- 1Hebei Key Laboratory of Preventive Veterinary Medicine, College of Animal Science and Technology, Hebei Normal University of Science and Technology, Qinhuangdao, China
- 2Institute of Microbiology, University of Agriculture, Faisalabad, Pakistan
- 3Livestock and Dairy Development Department, Lahore, Pakistan
- 4Key Laboratory of Agro-Products Quality and Safety Control in Storage and Transport Process, Ministry of Agriculture and Rural Affairs, Institute of Food Science and Technology, Chinese Academy of Agricultural Sciences, Beijing, China
- 5Department of Plant Pathology, University of Agriculture, Faisalabad, Pakistan
- 6State Key Laboratory of Veterinary Etiological Biology of Veterinary Parasitology of Gansu Province, Lanzhou Veterinary Research Institute, Chinese Academy of Agricultural Sciences, Lanzhou, China
- 7Jiangsu Co-Innovation Center for the Prevention and Control of Important Animal Infectious Disease and Zoonoses, Yangzhou University, Yangzhou, China
- 8Department of Clinical Medicine and Surgery, University of Agriculture, Faisalabad, Pakistan
- 9The Equine Clinic, Al-Hashar Stables, Muscat, Oman
- 10Department of Veterinary Medicine, University of Veterinary and Animal Sciences, Lahore, Pakistan
- 11Health Security Partners, Washington, DC, United States
Clostridium perfringens produces core virulence factors that are responsible for causing hemorrhagic abomasitis and enterotoxemia making food, animals, and humans susceptible to its infection. In this study, C. perfringens was isolated from necropsied intestinal content of buffalo and cattle belonging to four major bovine-producing regions in the Punjab Province of Pakistan for the purpose offind out the genetic variation. Out of total 160 bovine samples (n: 160), thirty-three (n: 33) isolates of C. perfringens were obtained from buffalo (Bubales bubalis) and cattle (Bos indicus) that were further subjected to biochemical tests; 16S rRNA based identification and toxinotyping was done using PCR (Polymerase Chain Reaction) and PFGE (Pulse Field Gel Electrophoresis) pulsotypesfor genetic diversity. Occurrence of C. perfringens was found to be maximum in zone-IV (Bhakkar and Dera Ghazi Khan) according to the heatmap. Correlation was found to be significant and positive among the toxinotypes (α-toxin, and ε-toxin). Response surface methodology (RSM) via central composite design (CCD) and Box-Behnken design (BBD) demonstrated substantial frequency of C. perfringens based toxinotypes in all sampling zones. PFGE distinguished all isolates into 26 different pulsotypes using SmaI subtyping. Co-clustering analysis based on PFGE further decoded a diversegenetic relationship among the collected isolates. This study could help us to advance toward disease array of C. perfringens and its probable transmission and control. This study demonstrates PFGE patterns from Pakistan, and typing of C. perfringens by PFGE helps illustrate and mitigate the incidence of running pulsotypes.
Introduction
Pakistan is an agriculture-based country and its livestock industry occupies a pivotal place in the economy, contributing 11.7% in the total gross domestic product (GDP) during the financial year 2019–2020 (1). In Pakistan, there are currently 90.8 million heads of buffalo and cattle, sharing 96.87 and 48.91% of the total milk and beef gross production, respectively (2). Due to the heavy potential of the livestock sector, a major proportion of the Pakistani population is directly or indirectly associated with the livestock sector. C. perfringens is an important etiological agent of causing different diseases in animals and humans (3). Diarrhea and pneumonia are considered to be a primary cause of mortality and morbidity in dairy calf worldwide (4). In domestic species, C. perfringens is the main cause of enteritis and enterotoxemia (5). In developed countries, food poisoning by C. perfringens type A is frequently reported foodborne illness; however, this type of food poisoning is not much reported in the developing countries (6, 7).
In recent times, the nomenclature of C. perfringens was augmented to seven types (A–G) by the addition of C. perfringens enterotoxin (CPE) and NetB hauling strains as peculiar toxin types (8). The pathogenic clostridial species can be classified into three groups, based on their toxin activity (enterotoxic, histotoxic, neurotoxic) on target tissues (9). C. perfringens type A has been and is still frequently blamed for enteritis, abomasitis, and/or enterotoxemia in cattle (10). The coding gene (CPA) is highly conserved and thus present in all strains of C. perfringens (11, 12). CPA plays a critical role in the pathogenesis of gas gangrene in humans and animals (13, 14). However, in recent years, multiple lines of evidence came from several well-designed studies mainly based on molecular Koch's postulates, which demonstrated the critical role of CPA in the pathogenesis of bovine necro-hemorrhagic enteritis (15–17). C. perfringens toxinotypes D isolates tend to produce alpha- and epsilon-toxin, which are encoded by plc and ε-toxin gene leading to the development of enterotoxemia (“pulpy kidney”) in sheep and goats (18–20).
Optimization is a method for response surface methodology (RSM) that focuses on the set of independent variables that serve as a mediator for discovering optimum conditions for the best possible response. This method is applied to gain maximum benefits from a particular process thus improving the performance of a system. This method could serve as a predictive study for determining the incidence of prevalent toxinotypes produced by C. perfringens (21). Many types of vaccines, medicines, and other biological agents have been tested for optimization to make the best combination that could be beneficial to every consumer (22). It consists of a polynomial model that is validated by variables for a particular response. It has a vast application in the field of microbiology and veterinary sciences. In this study, the toxinotypes of C. perfringens were subjected to an optimization process by Box-Behnken design (BBD) and central composite design (CCD) to determine the abundance of clostridial toxins (type A and type D) in B. bubalis and B. indicus.
C. perfringens type D (epsilon toxin) is responsible for enterotoxemia in sheep, goats, and cattle (19). ε-toxin is synthesized as a single-chain inactive prototoxin of 32–33 kDa in the gastrointestinal lumen, which is subsequently converted into an active toxin (29 kDa) by serine-type proteolytic enzymes (notably α-chymotrypsin, trypsin, and λ-protease). Once activated in the intestinal tract, ε-toxin increases intestinal vascular permeability, which in turn facilitates the entry of the toxin into the bloodstream. This event provides an opportunity for activated ε-toxin to rapidly damage different organs, including the brain, kidney, and lungs, through affecting vascular permeability and direct action on tissues (23). ε-toxin gene is encoded on the plasmids and is often regarded as the third-most potent toxin produced by Clostridial species after tetanus and botulinum toxins (24), with the epsilon toxin gene ε-toxin typically encoded on plasmids. Pathotyping is a traditional procedure of classification of C. perfringens (25). Over the past few years, advanced techniques like pulsed-field gel electrophoresis (PFGE) and multilocus sequence typing (MLST) have been introduced, with the capacity to ascertain the emergence and spread of infectious agents and explicate the immanent epidemiology of bacterial species (26, 27). Amid several accessible molecular typing tools, PFGE is still one of the most significant third-generation technique to decrypt the genetic diversity principally all of the bacterial species and also concede as gold standard due to brilliant discriminatory power and reliability of procedures upon developing macro-restriction pattern of the bacterial genome (28, 29).
The study was designed to assess the abundance of major toxin-producing genes related to C. perfringens using PFGE and check the interrelationship of pulsotypes to determine the genetic diversity to incide disease in buffalo and cattle of Pakistani origin.
Materials and Methods
Site Selection
The sites for sampling were divided into four sampling zones, namely, Zone-I (Lahore, Pattoki) Zone-II (Sargodha, Jhang), Zone-III (Sahiwal, Bahawalnagar), and Zone-IV (Bakkhar, Dera-Ghazi Khan). These zones are considered rich regarding buffalo and cattle population (Figure 1).
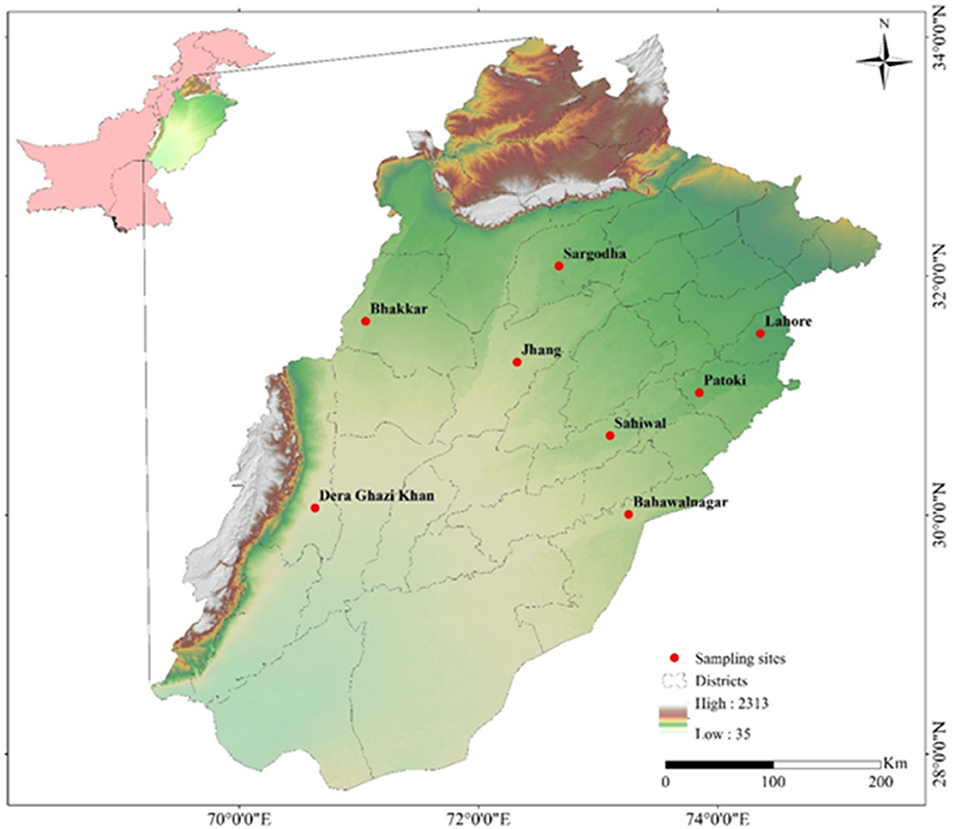
Figure 1. Map showing sampling sites (Buffalo, Cattle) of different cities of Punjab province, Pakistan generated by ArcGIS 10.2.2 software (ESRI, Redlands, CA, USA) by using Shuttle Radar Topography Mission (SRTM) Digital Elevation Model.
Sample Collection
A total of 160 animals comprising of 80 buffalo and 80 cattle were used in the study. Out of total 160 animals, 18 buffalo, and 15 cattle were found infected with C. perfringens. Intestinal contents (small intestine) of necropsied buffalo (n = 18) and cattle (n = 15) with a history of the intestinal problem were collected in sampling bags and transported in an ice box with a maintained temperature to the laboratory for further processing. Gross pathology is characterized by segmental to diffuse, hemorrhagic, or necrotizing inflammation of small intestinal mucosa.
Isolation of Bacterial Strains
Fecal swabs infused with intestinal contents were inoculated into 5 ml thioglycollate (FTA) broth and incubated at 37°C (Don Whitely DG-250 anaerobic workstation, UK) for 24 h. Subsequently, 100 μl of pre-enriched FTA broth was spread on tryptose sulphite cycloserine agar base enriched with 7% egg yolk and supplemented with D-cycloserine (Solarbio, Beijing, China). Petri plates containing multiple black colonies harboring lecithinase positive reaction were selected and cultured. For identification and purity of C. perfringens, streaking was done on Columbia blood agar [Huan Kai Microbial (HKM) Sci & Tech, Guangzhou, China] containing 5% defibrinated sheep blood and evaluated for typical double zone hemolysis associated with C. perfringens isolates. Moreover, Gram staining and biochemical tests, i.e., glucose, maltose, H2S reduction test, nitrate reduction test, gelatin liquefaction, and saccharose test (Hangwei, Microbiological Co. Ltd., Hangzhou, China) were performed. Isolates were preserved in 50% glycerol at −80°C for further use.
Extraction of Bacterial DNA
Genomic DNA of C. perfringens was extracted from overnight FTA broth culture inoculated with a single colony plate by Ultraclean Microbial DNA Isolation Kit (MoBio, Germantown, Maryland, USA) rendering to the manufacturer's directives with minor modification to attain high concentration. In brief, the bacterial suspension and lysis buffer mixed in addition of 20 μl of 20 mg/ml proteinase-K (Fisher Scientific, USA). Quality and quantity was determined by NanoDrop™ 2000 (Thermo Scientific Inc. Waltham, MA, USA). Protein contamination and the recommended purity level was estimated at the A260/A280 ratio of 1.8. The DNA was stored at −20°C for further genotyping analysis.
16S rRNA Gene Sequence Analysis
The 16S rRNA gene was amplified with primer from the genomic DNA of each strain using 16S rRNA gene species-specific primer (3). The sequences of the 16S rRNA gene region were determined by Sanger sequencing (Tsingke Biotechnology Company, Xian, China). The species were recognized by nucleotide alignments with the sequences of species banked in the GenBank NCBI, USA.The primers and conditions of amplification and sequencing of 16S rRNA genes references provided by Meer and Songer (30) and Kikuchi et al. (31), respectively. Primer sequence for genes regarding 16SrRNA (Table 1).
Assessment of Toxin Production by C. perfringens Isolates
Primers corresponding to the α toxin (CPA), β toxin (cpb), or ε toxin (ε-toxin) gene were used as described by Svensson et al. (4). Genes encoding toxin proteins, including cpb2, were detected by PCR (5–7). American Type Culture Collection ATCC-3624 (toxin type A, α-toxin positive) and China Institute of Veterinary Drug Control, Beijing, China, including CVCC-54 (toxin type B, α-, β-, and ε-toxin positive), CVCC-61 (toxin type C, α-, and β toxin positive), and CVCC-81 (toxin type D, α- and ε toxin positive) were used as reference strains for toxinotyping (A, B, C, and D, respectively), and as positive controls for cpb2 and cpe. Amplification was performed in a 25 μl reaction mixture containing 50 ng template DNA, PCR Premix Taq (Ex Taq V.2.0 plus Dye Takara, Japan), and 0.5 μM of each primer on a thermocycler (Takara, Japan); the total reaction volume was adjusted with the addition of RNase free water. The reaction conditions were as follows: initial denaturation at 96°C for 5 min; 35 cycles of denaturation at 96°C for 1 min., annealing at 56°C for 1 min and elongation at 72°C for 1 min; and a final extension at 72°C for 10 min.
For ε-toxin, the assay conditions were modified as follows: initial denaturation at 95°C for 3 min; 35 cycles of denaturation at 95°C for 40 s, annealing at 57°C for 30 s, and elongation at 72°C for 30 s; and a final extension at 72°C for 5 min. The amplified products were analyzed on a 1.2% agarose gel stained with ethidium bromide (10 mg/ml) by (GenStar, Beijing, China) PCR-amplified products on the gels were extracted and purified by E.Z.N.A® Gel Extraction Kit (Omega Bio-Tek, USA), and sequenced (Tsingke Biotechnology Company Xian, China) to ensure the identity with reference sequences.
Genotyping of C. perfringens by PFGE
PFGE was performed according to the method described by Gholamiandekhordi et al. (12) with slight modifications (8). Inserts of genomic DNA were digested with 37°C for 2.5 h with 20 U of SmaI enzyme. Electrophoresis was performed in a CHEF-DRIII apparatus (Bio-Rad, USA). DNA fingerprints were compared by the BioNumerics 7.6, Austin, TX, USA, using a cut-off value with 90% similarity based on Dice coefficient identify PFGE genotypes. Plug preparation, restriction digestion, and electrophoresis conditions were essentially performed for C. perfringens according to the previously described protocol by Lindström et al. (6). Cells were suspended in suspension buffer [75 mM NaCl and 25 mM EDTA, (pH 8.0)] to an estimated absorbance of 1.3 at 610 nm. Bacterial cells were embedded in 1.2% chromosomal-grade Pulse Field Certified Agarose (Bio-Rad, USA) by mixing equal volumes (0.5 ml) of the cell suspension and melted agarose equilibrated to 55–65°C. In some preparations, bacterial cells were pretreated with formalin to reduce the interference by endogenous DNase activity before they were mixed with agarose.
Plugs were solidified at 4°C in 1.5 mm thick molds (Bio-Rad Laboratories, Hercules, CA, USA). The agarose-embedded cells were lysed by incubation of the plugs overnight at 55°C with gentle shaking in lysis buffer comprising 50 mM Tris-HCl [pH 8.0], 50 mM EDTA [pH 8.0], 1% N-lauryl sarcosine, and proteinase K (1 mg/ml Merck, Germany). The plugs were washed three times at 50°C with vigorous shaking for 15 min each in sterile reagent-grade water followed by three times of washing with TE buffer comprising of 10 mM Tris, 1 mM EDTA (pH 8.0) Tris EDTA for 15 min each time. Plugs were stored in TE buffer at 4°C in refrigerator for further experimental usage. These plugs were cut into 3–4 mm slices and equilibrated with appropriate restriction buffer containing (20 mg/ml) bovine serum albumin (BSA, Amresco, Solon, Ohio, USA). The SmaI (New Bio, England) restriction enzyme was used at appropriate conditions recommended by the manufacturer. Restriction fragments were separated by electrophoresis through a 1% Pulse Field Certified Agarose (Bio-Rad, USA) solution of Tris-borate and EDTA (TBE buffer, Solarbio, Beijing, China). TBE buffer containing 200 μM thiourea (Sigma-Aldrich, St. Louis, MO, United States) at 14°C in a contour-clamped homogeneous electric field MAPPER XA PFGE (Bio-Rad, USA) apparatus was used to increase the band quality and resolution. The run time was 18 h with a voltage of 6 V/cm and a linearly ramped pulse time of 0.5–38 s. The restricted fragments were separated in a 1% agarose gel in 0.5 × TBE buffer by using a CHEF-DR III system (Bio-Rad, USA). Following the electrophoresis, the gel was stained in aqueous ethidium bromide (10 mg/ml) by (GenStar, Beijing, China) followed by a destained step in water for 20 min, and the image was captured by a ChemiDoc CRX+ Image analyzer (Bio-Rad USA) as TIFF files.
PFGE Agarose and DNA Migration
Based on equations and reprise DNA model migration in traditional electrophoresis, the linear DNA molecular i (Di) migration in a counter-clamed homogeneous electric field (CHEF) dynamic regulation (DR-III) gels are described by Lindström et al. (6), Roeder et al. (10), Canard et al. (11), Lindström et al. (6), Roeder et al. (10), and Canard and Cole (11).
Computational Analysis of PFGE Patterns
An analysis obtained from the restrictive SmaI endonuclease was carried out with PFGE standards and analyzed using BioNumerics version 7.6 (Applied Math's, Inc., Austin, TX, USA). The assessment was based on a band for each type A and the similarity analysis was performed using the Dice coefficients (SD) with a custom tolerance of 1.5%. The type of dendrogram was made by unweighted pair group impressions formed by the unweighted pair group method with arithmetic mean (UPGMA). The discriminatory power was calculated by the Simpson diversity index (SD) (32).
Statistical Analysis
Analysis of data was performed by one-way analysis of variance (ANOVA) along with the means of treatments were compared using Tukey's Honestly Significant Difference (HSD) test using SPSS [Statistical Package for Social Sciences (SPSS) version 26.0 Armonk, NY, USA]. Correlation, regression, and cluster analysis using heatmap were performed using R Studio suite 1.3.1093. Optimization of the parameters was done using RSM via CCD and BBD using the Design-Expert software (Design Expert® version 12.0, Stat-Ease Inc. Suite 6400, Minneapolis, MN55413, USA).
Results
Distribution of C. perfringens From Four Sampling Zones of Punjab Province in Pakistan
A total of 160 animals (buffalo = 80 and cattle = 80) were examined for C. perfringens infection. Of the total animals, only positive tested samples were isolated from both buffalo and cattle. The zonal distribution of collected samples is stated in Table 2. On processing the collected samples, after careful isolation and identification (classical method, i.e., culturing, biochemical tests), we performed PCR of toxin-encoded genes to confirm their serotypes (8). Furthermore, PFGE performed with SmaI that gives different fingerprint patterns leads to the development of 26 pulsotypes (PFGE patterns) which indicates genetic diversity among study samples.
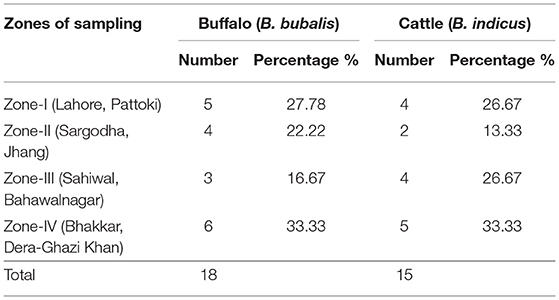
Table 2. Distribution of C. perfringens in buffalo and cattle from four sampling zones of Punjab province in Pakistan.
Isolate Isolation and Identification
Culturing, biochemical testing, i.e., glucose (+), maltose (+), H2S reduction test (–), nitrate reduction test (+), gelatin liquefaction (+), and saccharose test (+), amplification (PCR), and subsequent sequencing of the 16S rRNA gene confirmed that all 33 isolates were of C. perfringens. The representative sequences can be accessed at NCBI under accession number MT158886-MT158897.
Cluster Analysis of C. perfringens Based Toxinotypes in Buffalo and Cattle From Four Zones in Punjab Province
For cluster analysis of the toxinotypes in C. perfringens, a heatmap was used. In the case of α-toxin and ε-toxin of C. perfringens, the heatmap depicts that zone-IV is abundant in both toxinotypes, while Zone-II is less abundant. α-toxin was mostly observed in cattle and buffalo belonging to zone-IV, while ε-toxin was also found maximum in cattle and buffalo belonging to zone-IV. This illustration depicts that buffalo and cattle of Zone-IV are more vulnerable to C. perfringens (Figure 2).
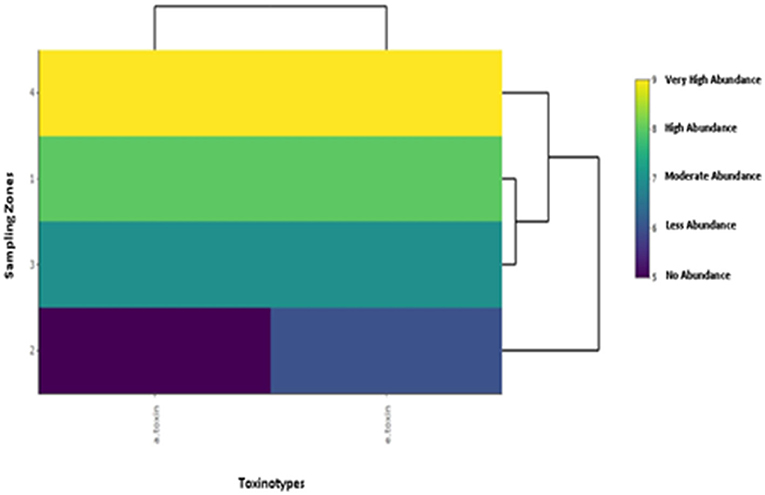
Figure 2. Illustration of cluster analysis by heatmap of toxinotypes in C. perfringens isolated from buffalo and cattle in four different zones of Punjab province in Pakistan. The color scheme represents the abundance of toxinotypes in buffalo and cattle belonging to four sampling zones.
Optimization of C. perfringens Toxinotypes (α-Toxin and ε-Toxin) in Sampled Zones of Punjab Province by Response Surface Methodology
For the toxinotypes, the abundance in buffalo and cattle was determined based on RSM via CCD. The coding of variables is shown in Table 3. The data were applied to the following equation:
where “Y” is the abundance of C. perfringens toxinotypes., “β0” is the intercept constant, “βi,” “βii,” “βij” are the regression coefficients of “F1,” “F2,” “Fi,” “Fj” are coded values of independent variables, which are α-toxin and ε-toxin (Table 3), respectively, while “ε” is the error term.
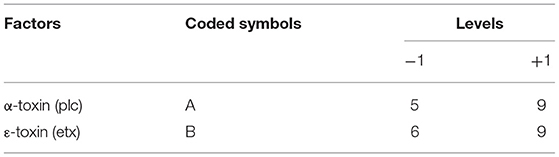
Table 3. The design approach for determining the optimization of C. perfringens toxinotypes in central–composite design (CCD).
By applying ANOVA (Table 4) for the model, it was found to be significant (P < 0.05). It indicated that this model was adequate and reproducible. The abundance of C. perfringens predicted by the regression equation was close to the observed ones (R2 = 0.98). Based on the ANOVA, two independent variables, namely, α-toxin and ε-toxin had a significant linear effect and quadratic effect on abundance. The above parameters for abundance of C. perfringens were evaluated concluding an optimized value could be achieved at α-toxin level of 7 and ε-toxin level of 5. In Figure 3, the zones of optimization are shown in the contour and surface plots to illustrate the effects of independent variables (factors), i.e., α-toxin and ε-toxin on the dependent variable (response), i.e., abundance in cow and buffalo. The interactive effect of these toxinotypes was found to be nonsignificant at P < 0.05. Both α-toxin and ε-toxin have their own impact. Runs 1, 5, and 8 of table display low abundance, while other runs illustrate high abundance of α-toxin and ε-toxin, respectively (Table 5).
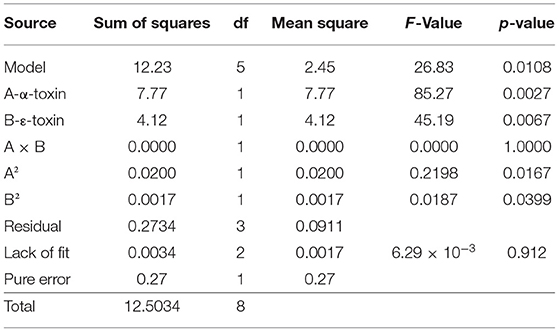
Table 4. Analysis of variance for prevalence of C. perfringens toxinotypes in four different zones of Punjab province in Pakistan.
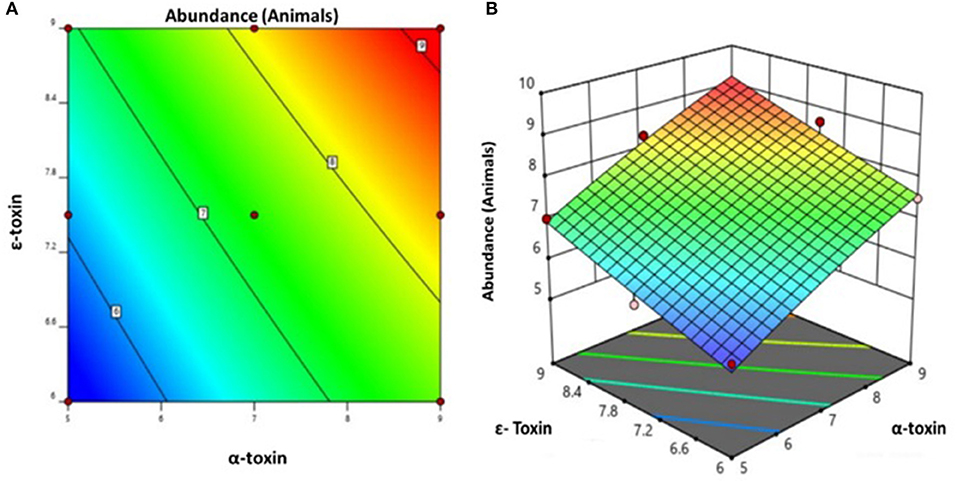
Figure 3. Prevalence of C. perfringens toxinotypes in four different zones of Punjab province in Pakistan (A) contour plot (B) surface plot.
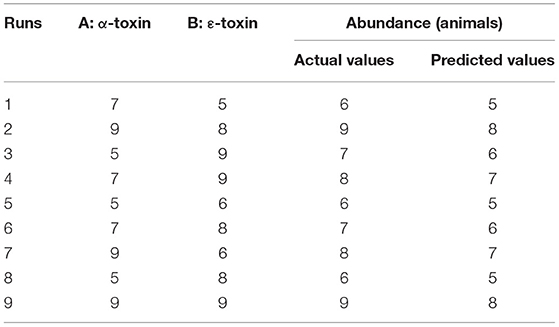
Table 5. Actual and predicted values for the abundance of C. perfringens toxinotypes in four different zones of Punjab province in Pakistan.
Based on the ANOVA, the following regression equation was obtained for the optimization of C. perfringens toxinotypes:
Optimization of Sampling Zones Against the C. perfringens Toxins by Response Surface Methodology
For all the zones of sampling, the abundance was assessed based on RSM via Box Behnken design (BBD). The coding of variables is shown in Table 6. The data were applied to the following equation:
where “Y” is the abundance in buffalo and cattle, “β0” is the intercept constant, “βi,” “βii,” “βij” are the regression coefficients of “F1,” “F2,” “F3,” “Fi,” “Fj” are coded values of independent variables which are four sampling zones in Punjab Province, respectively, while “ε” is the error term.
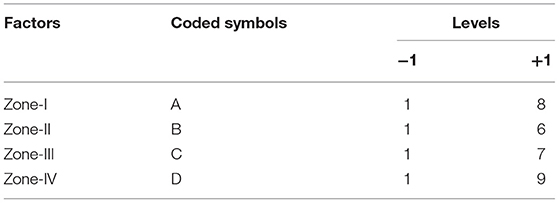
Table 6. The design approach for determining the optimization of four sampling zones in the abundance of C. perfringens in Box–Behnken Design (BBD).
By applying ANOVA (Table 7) for the model, it was found to be significant (P < 0.05). It indicated that this model was adequate and reproducible. The abundance of C. perfringens toxins predicted by the regression equation was close to the observed ones (R2 = 0.99). Based on the ANOVA, four independent variables, namely, zone-I, zone-II, zone-III, and zone-IV had a significant linear effect and quadratic effect on the abundance of toxins in animals. The above parameters for abundance were evaluated concluding an optimized value could be achieved at 1 unit of zone-I, 6 units of zone-II, 1 unit of zone-III, and 5 units of zone-IV. In Figure 4, the zones of optimization are shown in the surface plots to illustrate the effects of independent variables (factors), i.e., sampling zones on the dependent variable (response), i.e., abundance in animals. The interactive effect of all zones was found to be positive and significant regarding the abundance of toxins in cows and buffalo and the significance value was found to be <5%. All these factors show relatively significant abundance in both buffalo and cattle. In runs 14, 18, and 25, the animals showed less abundance of toxinotypes based on four zones of sampling (Table 8).
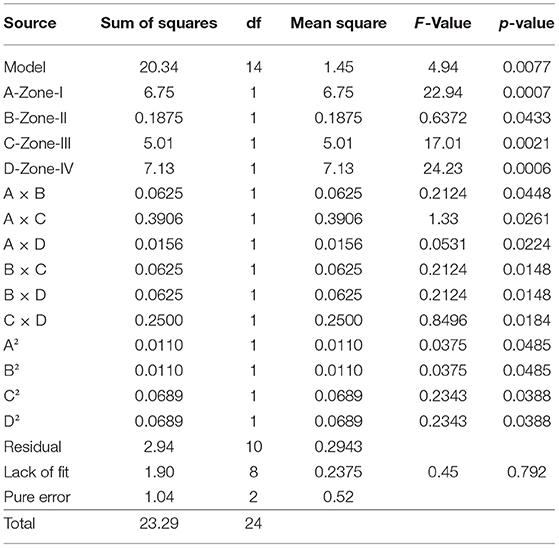
Table 7. Analysis of variance for the prevalence of C. perfringens toxinotypes in four different zones of Punjab province in Pakistan.
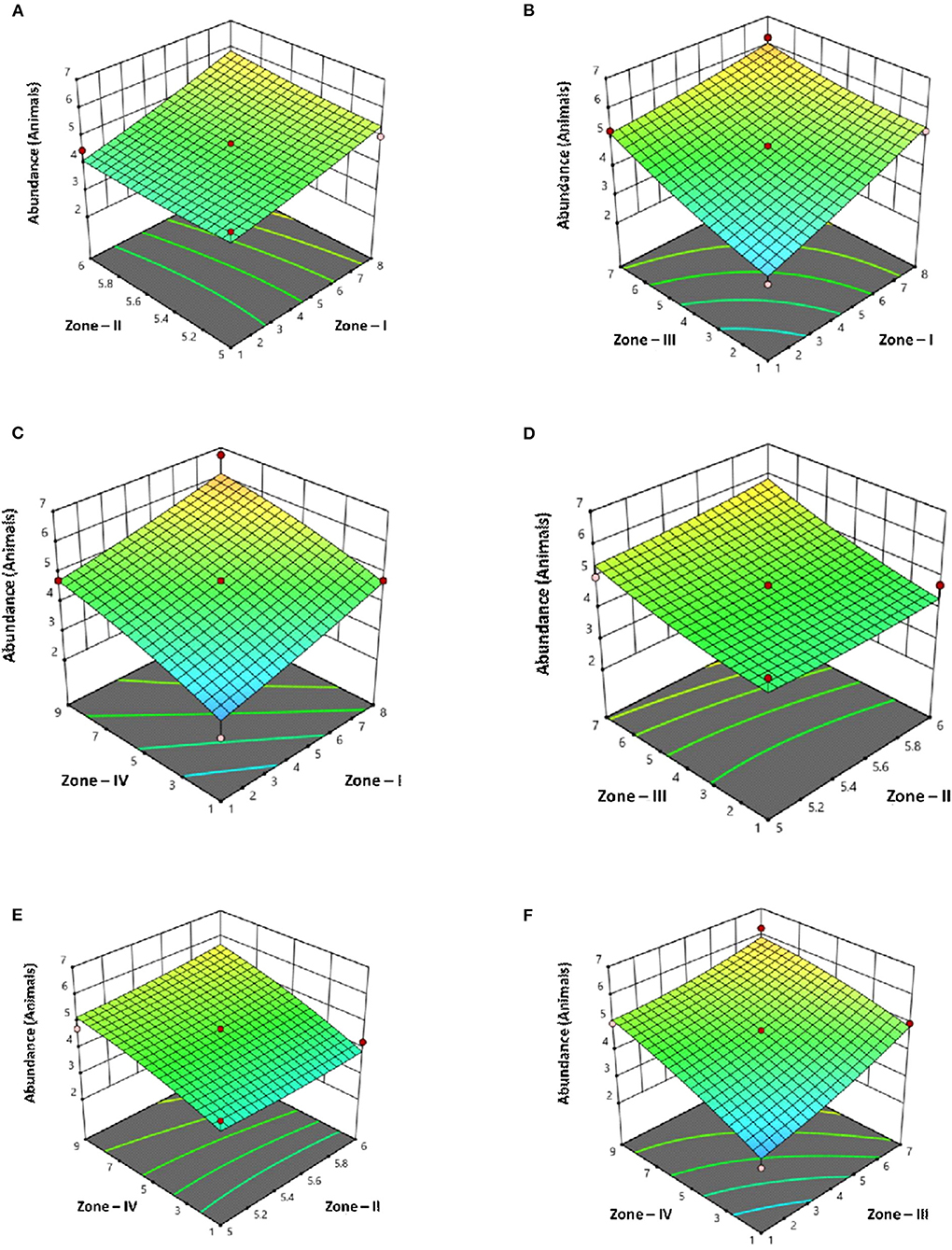
Figure 4. Surface plots of C. perfringens toxins in four different zones with Punjab province in Pakistan with their interaction (A) Zone–I × Zone–II (B) Zone–I × Zone–III (C) Zone–I × Zone–IV (D) Zone–II × Zone–III (E) Zone–II × Zone–IV (F) Zone–III × Zone–IV.
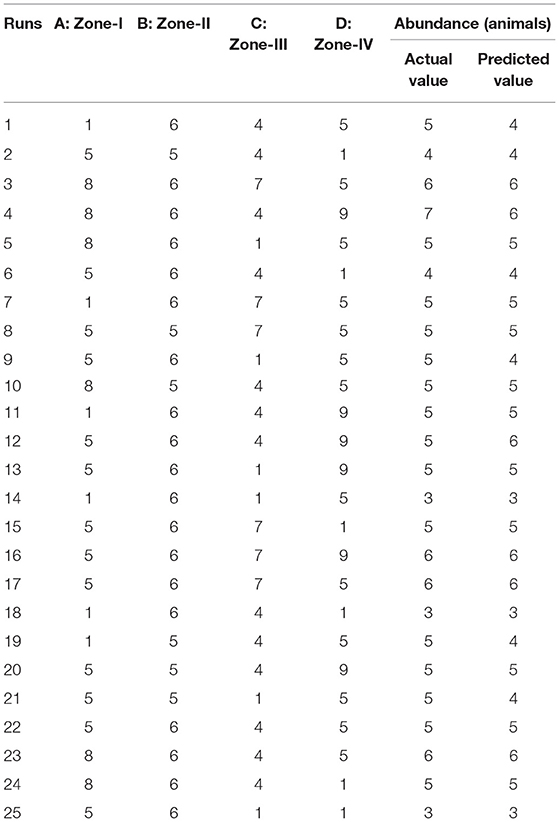
Table 8. Actual and predicted values for C. perfringens toxins in four different zones of Punjab province in Pakistan.
Figure 5 and Table 9 illustrate the summary statistics of the model applied to the variables, which clarifies the significance of both RSM-based designs.
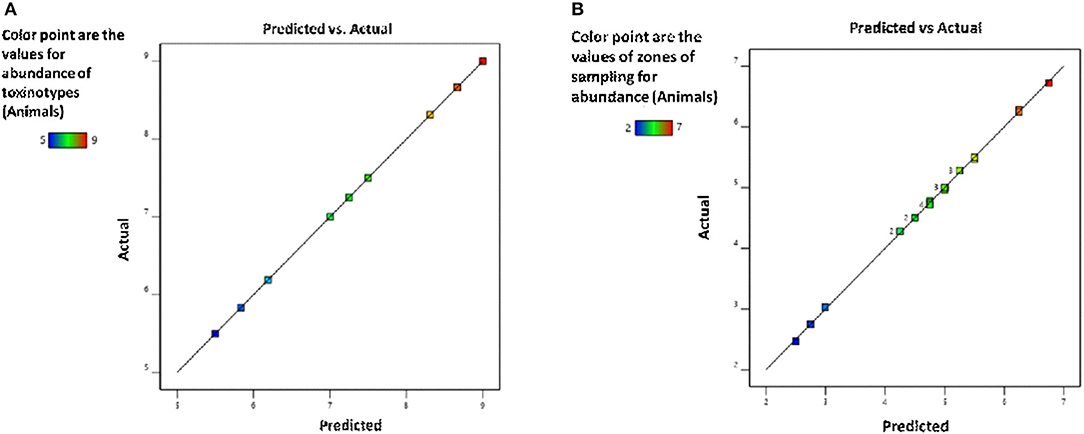
Figure 5. Validation of the model for (A) toxinotypes and (B) zones of sampling for the assessment of C. perfringens abundance in the buffalo and cattle population of Punjab province, Pakistan.
Based on the ANOVA, following regression equation was obtained for the optimization of sampling zones:
Toxinotyping and Confirmation of Genes Encoding Toxin Proteins by PCR
In the current study, isolates were divided into four sampling zones, namely, Zone-I, Zone-II, Zone-III, and Zone-IV. These zones are considered rich regarding buffalo and cattle population. The toxinotypes were confirmed by toxin-specific PCR and sequencing. The results revealed that the 33 isolated C. perfringens strains were either type A or type D. Major proportion (55%, 28/33) belonged to type D (plc+, ε-toxin+), while only 15% (5/33) of samples were identified as type A (plc+).
Pulsed Field Gel Electrophoresis
Analysis of 33 C. perfringens strains provided reasonable pattern that led to formation of 26 pulsotypes showed higher discriminatory power of SmaI digestion activity and proved to be capable to C. perfringens type A strains to achieve the satisfactory type ability. Restriction enzyme profiles of the isolates' genomic DNA obtained by using SmaI showed 9–13 fragments ranging in size from ~48 to 485 kb (Figure 6).
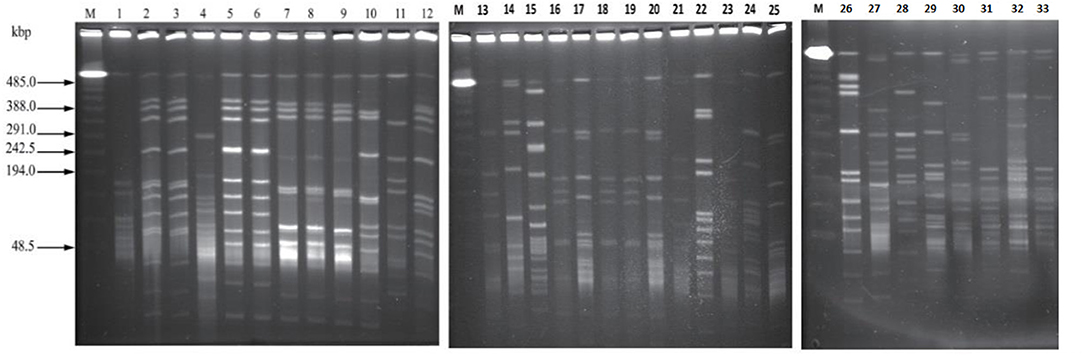
Figure 6. The pattern obtained by PFGE SmaI, Marker = (PFGE Lambda Marker New England Bio, UK) lanes: 1–33 strains of C. perfringens of bovine origin showing different DNA macrorestriction pattern.
According to the criteria of Layana et al. (18) and Tenover et al. (33), none of the isolates can be considered genetically indistinguishable. Two isolates were closely related, with 2- to 3-band differences. Dendrogram constructed, considering the adopted UPGMA parameters, grouped the isolates into seven different clusters (A to G) with the main clusters having similarities ranging from 80 to 95% (BioNumerics 7.6, Austin, TX, USA) and generated 26 pulsotypes based on the band (line) pattern (Figure 7). We collected the field samples and performed careful isolation and characterization via PCR and found type A (plc) and type D (plc + etx) on the basis of toxin-encoded gene amplification. On processing of collected samples, after careful isolation and identification (classical method, i.e., culturing, biochemical tests), we performed PCR of toxin-encoded genes to confirm their serotypes (8). Furthermore, PFGE performed with SmaI that gives different fingerprint patterns leads to development of 26 pulsotypes (PFGE patterns) that indicates genetic diversity among study samples.
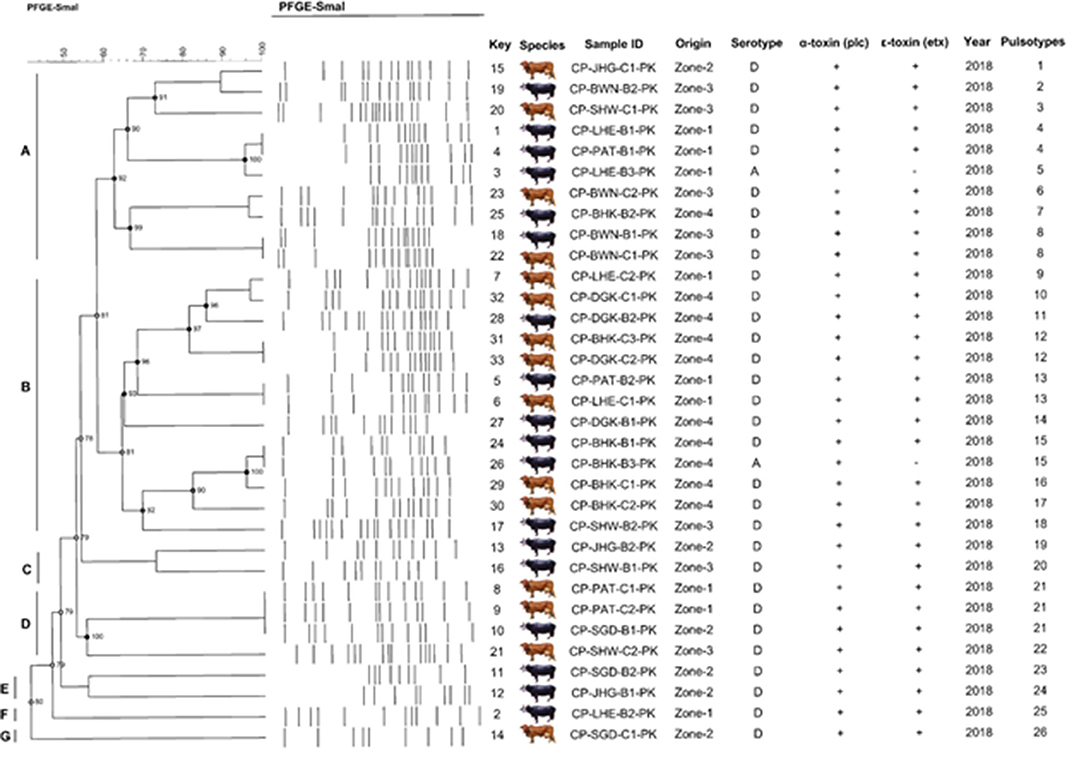
Figure 7. Schematic representation (genetic profile, key species, isolate, serotype, toxinotypes, and pulsotypes) of 33 C. perfringens isolates obtained from buffalo and cattle in Pakistan. The macro-restriction pattern was conducted with SmaI. Dendogram obtained using BioNumerics 7.6 software and unweighted pair group method with Arithmetic mean. Letters (A–G) represents the C. perfringens toxinotypes prevalent in bovines.
Cluster A was found to be highly diverse among the others, as it consisted of the samples from all the sampling zones included in the study. Cluster D and F consisted of only buffalo isolates, while the rest of the clusters included isolates from both buffalo and cattle. All isolates were found to be α-toxin and ε-toxin positive, while only 5 were ε-toxin negative. Out of 5 ε-toxin negative samples, 4 were of buffalo origin, while only one was of cattle origin. The animals from Zone-I fashioned 4 similar genomic patterns of DNA macrorestriction patterns on parallel Zone-IV also produced 2 similar PFGE profiles. Our data provide information about running PFGE profile (pulsotypes) in dairy animals of Punjab Province, Pakistan.
Simpson Index (SD) and Cophenetic Correlation (ρ)
A high SD value was achieved with SmaI (SD = 0.98) yielding 26 pulsotypes. The excellence of the cluster analysis was validated by the cophenetic correlation value (ρ) for the dendrogram. Each strain of DNA underwent duplication to verify the repeatability of the macrorestriction pattern of running DNA samples. PFGE profiles (pulsotypes) are given in the dendrogram provided by a cophenetic correlation coefficient (ρ) equal to 0.90. This explained the fitness and reliability of the clustering of original data presented by Muth and Morrill (20) and generally falls between 0.60 and 0.95 and values higher than 0.80 are regarded as satisfactory as reported by Hornitzky and Glastonbury (34) and Knapp et al. (35).
Discussion
C. perfringens is an important part of intestinal microflora and certain strains are well-documented for triggering diseases in animals and humans varying from myonecrosis and food poisoning to enterotoxemia and enteritis (36, 37). The association of disease is augmented by ε-toxin in numerous animal species advocated with the clinical and pathological investigation (19, 38). Investigational trials related to enterotoxemia in cattle indicate that ε-toxin was the crucial factor in the pathogenesis of this disease in (bovine) dairy farm animals (19). Although C. perfringens type D secretes ε-toxin similarly, the synergistic role of additional C. perfringens toxins as α-toxin and other twenty minor toxins cannot be precluded (19, 36). ε-toxin is provocative among researchers, but all acquiesce that “pulpy kidney” is materialized in the kidney of ruminants with type D enterotoxemia (37, 39).
The toxin profiles and PFGE were used to characterize isolates obtained from buffalo and cattle feces. The PFGE profiles of all isolates differed by no more than two bands and the three patterns observed shared a 90% similarity. This is in contrast to the study by Akiba et al. (37). Statistics on enterotoxemia by C. perfringens type D in farm animals are limited, but a study conducted by Filho et al. (19) supports our result about a disease model in buffalo and cattle, which confirmed that the lethal ε-toxin is an elite cause of enterotoxemia in dairy farm animals. Our results impart support to that conjecture and anticipate sagacity related to genetic diversity.
The isolates that had PFGE pattern type A and type D were indisputably associated with the bovine origin and an identical pattern was obtained with a few isolates (40). In this study, the variation between the band patterns of PFGE types was reflected as adequate to discriminate between two different PFGE types (pulsotypes). The results gained by PFGE divulge a huge genetic diversity. No relationship was found between the isolated source and the positions in the dendrogram.
At present, the main advantage of PFGE application is to perform bacterial DNA fingerprinting that is preceding to differentiating bacterial subtypes. The bacterial genome analysis by PFGE provides remarkable information that helps to distinguish the clonality of the isolates in an outbreak to identify the origin, cause, and route along with broadcasting of the disease (12, 29, 41). Currently, PFGE is not only the point of focus for public health surveillance, disease cluster identification, and outbreak investigations but also considered the gold standard for bacterial subtyping. Moreover, PFGE gel upon completion of electrophoresis provides precise results in real-time. We assume that PFGE remains the key method for bacterial subtyping and performs molecular epidemiological studies in developed countries, although the switching to WGS will be a key obstacle due to the shortage of funds and the allocation of multidisciplinary qualified teams in several countries.
In this study, huge genetic diversity was possible because of the wide diversity of the isolates analyzed. PFGE clearly distinguished among disparate isolates of C. perfringens and established a clonal relationship between related strains. Although unrelated isolates impart 60 to 70% similarities, numerous fragments comigrated between isolates, similar to those reported by Canard and Cole (11), signifying that several sequences in the genome are conserved conversely; according to prior studies by Canard et al. (11) and Maslanka et al. (42), it was not astounding to observe different patterns between unrelated isolates. Computer-aided programs are used to obtain a more objective estimation of clonality between the strains. Visual assessment of subtype patterns leads to deduce the relatedness (band difference) when in fact the isolates were obtained in different cities of Pakistan. Khan et al. (43) and Nasir et al. (44) also confirmed the incidence of epsilon toxin causing eneterotoxemia in bovine species of Pakistan origin, which could be a major threat in the near future.
Conclusion
The novelty of this study lies in the genomic diversity of C. perfringens by PFGE that would help us for better understanding related to epidemiology and disease control strategy. The establishment of deep knowledge at the genome level is required to figure out the host-pathogen interaction.
Data Availability Statement
The datasets presented in this study can be found in online repositories. The names of the repository/repositories and accession number(s) can be found at: The representative sequences can be accessed at NCBI under accession number MT158886-MT158897.
Ethics Statement
No animal studies are presented in this manuscript. However, the collection of intestinal contents from the necropsied cattle and buffaloes was done keeping the standards of animal welfare intact and strictly adhering to the international guidelines.
Author Contributions
MZ, SK, and MH: conceptualization. MZ and MH: methodology, software, and writing—original draft. YL and JZ: validation, investigation, resources, supervision, project administration, and funding acquisition. SY: formal analysis. MZ, SK, MH, WA, MA, TM, MI, and MT: data curation. SY, YL, JZ, SK, WA, MA, TM, MI, and MT: writing—review and editing. SY, YL, and JZ: visualization. All authors contributed to the article and approved the submitted version.
Funding
This study was supported by the Hebei Province Key R&D Program (Grant No. 21322913D), Gansu Province R&D Program (20YF3NA005), and the Project Funds (Nos. 2022YB009 and 2022YB010) supported by Hebei Normal University of Science and Technology.
Conflict of Interest
The authors declare that the research was conducted in the absence of any commercial or financial relationships that could be construed as a potential conflict of interest.
Publisher's Note
All claims expressed in this article are solely those of the authors and do not necessarily represent those of their affiliated organizations, or those of the publisher, the editors and the reviewers. Any product that may be evaluated in this article, or claim that may be made by its manufacturer, is not guaranteed or endorsed by the publisher.
Acknowledgments
The authors are grateful to Dr. Peter C. Thomson from School of Life and Environmental Sciences (SOLES), University of Sydney, Camden, Australia, for editorial assistance and valuable comments to the manuscript.
References
3. Petit L, Gibert M, Popoff MR. Clostridium perfringens: toxinotype and genotype. Trends Microbiol. (1999) 7:104–10. doi: 10.1016/S0966-842X(98)01430-9
4. Svensson C, Linder A, Olsson SO. Mortality in Swedish dairy calves and replacement heifers. J Dairy Sci. (2006) 89:4769–77. doi: 10.3168/jds.S0022-0302(06)72526-7
5. Fohler S, Klein G, Hoedemaker M, Scheu T, Seyboldt C, Campe A, et al. Diversity of Clostridium perfringens toxin-genotypes from dairy farms. BMC Microbiol. (2016) 16:199. doi: 10.1186/s12866-016-0812-6
6. Lindström M, Heikinheimo A, Lahti P, Korkeala H. Novel insights into the epidemiology of Clostridium perfringens type A food poisoning. Food Microbiol. (2011) 28:192–8. doi: 10.1016/j.fm.2010.03.020
7. Uzal FA, Freedman JC, Shrestha A, Theoret JR, Garcia J, Awad MM, et al. Towards an understanding of the role of Clostridium perfringens toxins in human and animal disease. Fut Microbiol. (2014) 9:361–77. doi: 10.2217/fmb.13.168
8. Rood JI, Adams V, Lacey J, Lyras D, McClane BA, Melville SB, et al. Expansion of the Clostridium perfringens toxin-based typing scheme. Anaerobe. (2018) 53:5–10. doi: 10.1016/j.anaerobe.2018.04.011
9. Rood JI, McClane BA, Songer JG, Titball RW. The Clostridia: Molecular Biology and Pathogenesis. San Diego, CA: Academic Press (1997).
10. Roeder BL, Chengappa MM, Nagaraja TG, Avery TB, Kennedy GA. Experimental induction of abdominal tympany, abomasitis, and abomasal ulceration by intraruminal inoculation of Clostridium perfringens type A in neonatal calves. Am J Vet Res. (1988) 49:201–7.
11. Canard B, Cole ST. Genome organization of the anaerobic pathogen Clostridium perfringens. Proc Natl Acad Sci USA. (1989) 86:6676–80. doi: 10.1073/pnas.86.17.6676
12. Gholamiandekhordi AR, Ducatelle R, Heyndrickx M, Haesebrouck F, Van Immerseel F. Molecular and phenotypical characterization of Clostridium perfringens isolates from poultry flocks with different disease status. Vet Microbiol. (2006) 113:143–52. doi: 10.1016/j.vetmic.2005.10.023
13. Awad MM, Ellemor DM, Boyd RL, Emmins JJ, Rood JI. Synergistic effects of alpha-toxin and perfringolysin O in Clostridium perfringens-mediated gas gangrene. Infect Immun. (2001) 69:7904–10. doi: 10.1128/IAI.69.12.7904-7910.2001
14. Cavalcanti MTH, Porto T, Porto ALF, Brandi IV, Lima Filho JL, Pessoa Junior A. Large scale purification of Clostridium perfringens toxins: a review. Rev Bras Ciências Farm. (2004) 40:151–64. doi: 10.1590/S1516-93322004000200004
15. Valgaeren B, Pardon B, Goossens E, Verherstraeten S, Schauvliege S, Timbermont L, et al. Lesion development in a new intestinal loop model indicates the involvement of a shared Clostridium perfringens virulence factor in haemorrhagic enteritis in calves. J Comp Pathol. (2013) 149:103–12. doi: 10.1016/j.jcpa.2012.11.237
16. Goossens E, Verherstraeten S, Valgaeren BR, Pardon B, Timbermont L, Schauvliege S, et al. The C-terminal domain of Clostridium perfringens alpha toxin as a vaccine candidate against bovine necrohemorrhagic enteritis. Vet Res. (2016) 47:52. doi: 10.1186/s13567-016-0336-y
17. Verherstraeten S, Goossens E, Valgaeren B, Pardon B, Timbermont L, Vermeulen K, et al. The synergistic necrohemorrhagic action of Clostridium perfringens perfringolysin and alpha toxin in the bovine intestine and against bovine endothelial cells. Vet Res. (2013) 44:45. doi: 10.1186/1297-9716-44-45
18. Layana JE, Miyakawa MEF, Uzal FA. Evaluation of different fluids for detection of Clostridium perfringens type D epsilon toxin in sheep with experimental enterotoxemia. Anaerobe. (2006) 12:204–6. doi: 10.1016/j.anaerobe.2006.05.001
19. Filho EJF, Carvalho AU, Assis RA, Lobato FF, Rachid MA, Carvalho AA, et al. Clinicopathologic features of experimental Clostridium perfringens type D enterotoxemia in cattle. Vet Pathol. (2009) 46:1213–20. doi: 10.1354/vp.08-VP-0304-U-FL
20. Muth OH, Morrill DR. Control of enterotoxemia (pulpy kidney disease) in lambs by the use of alum precipitated toxoid. Am J Vet Res. (1946) 7:355–7.
21. Araujo PW, Brereton RG. Experimental design III. Quantification. Trends Anal Chem. (1996) 15:156–63. doi: 10.1016/0165-9936(95)00086-0
22. Almeida DG, da Silva RCFS, Luna JM, Rufino RD, Santos VA, Sarubbo LA. Response surface methodology for optimizing the production of biosurfactant by Candida tropicalis on industrial waste substrates. Front Microbiol. (2017) 8:157. doi: 10.3389/fmicb.2017.00157
23. Stiles BG, Barth G, Barth H, Popoff MR. Clostridium perfringens epsilon toxin: a malevolent molecule for animals and man? Toxins. (2013) 5:2138–60. doi: 10.3390/toxins5112138
24. Smedley JG, Fisher DJ, Sayeed S, Chakrabarti G, McClane BA. The enteric toxins of Clostridium perfringens. In: Reviews of Physiology, Biochemistry and Pharmacology. Springer. (2004) p. 183–204. doi: 10.1007/s10254-004-0036-2
25. Yamagishi T, Sugitani K, Tanishima K, Nakamura S. Polymerase chain reaction test for differentiation of five toxin types of Clostridium perfringens. Microbiol Immunol. (1997) 41:295–9. doi: 10.1111/j.1348-0421.1997.tb01204.x
26. Jost BH, Trinh HT, Songer JG. Clonal relationships among Clostridium perfringens of porcine origin as determined by multilocus sequence typing. Vet Microbiol. (2006) 116:158–65. doi: 10.1016/j.vetmic.2006.03.025
27. Maiden MCJ, Bygraves JA, Feil E, Morelli G, Russell JE, Urwin R, et al. Multilocus sequence typing: a portable approach to the identification of clones within populations of pathogenic microorganisms. Proc Natl Acad Sci USA. (1998) 95:3140–5. doi: 10.1073/pnas.95.6.3140
28. Nassonova ES. Pulsed field gel electrophoresis: theory, instruments and application. Cell Tissue biol. (2008) 2:557. doi: 10.1134/S1990519X08060011
29. Goering RV. Pulsed field gel electrophoresis: a review of application and interpretation in the molecular epidemiology of infectious disease. Infect Genet Evol. (2010) 10:866–75. doi: 10.1016/j.meegid.2010.07.023
30. Meer RR, Songer JG. Multiplex polymerase chain reaction assay for genotyping Clostridium perfringens. Am J Vet Res. (1997) 58:702–5.
31. Kikuchi E, Miyamoto Y, Narushima S, Itoh K. Design of species-specific primers to identify 13 species of Clostridium harbored in human intestinal tracts. Microbiol Immunol. (2002) 46:353–8. doi: 10.1111/j.1348-0421.2002.tb02706.x
32. Hunter PR, Gaston MA. Numerical index of the discriminatory ability of typing systems: an application of Simpson's index of diversity. J Clin Microbiol. (1988) 2611:2465–6.
33. Tenover FC, Arbeit RD, Goering RV, Mickelsen PA, Murray BE, Persing DH, et al. Interpreting chromosomal DNA restriction patterns produced by pulsed-field gel electrophoresis: criteria for bacterial strain typing. J Clin Microbiol. (1995) 33:2233. doi: 10.1128/jcm.33.9.2233-2239.1995
34. Hornitzky MA, Glastonbury J. Enterotoxaemia in sheep, goats and cattle. In: Australian Standard Diagnostic Techniques for Animal Diseases. Corner LA, Bagust TJ, editors. Melbourne, VIC: Standing Committee on Agricultural and Resource Management, CSIRO (1993).
35. Knapp O, Stiles B, Popoff M. The aerolysin-like toxin family of cytolytic, pore-forming toxins. Open Toxinol J. (2010) 3, 53–68. doi: 10.2174/1875414701003010053
36. Uzal FA, Kelly WR. Enterotoxaemia in goats. Vet Res Commun. (1996) 20:481–92. doi: 10.1007/BF00396291
37. Akiba M, Sameshima T, Nakazawa M. Clonal turnover of enterohemorrhagic Escherichia coli O157: H7 in experimentally infected cattle. FEMS Microbiol Lett. (2000) 184:79–83. doi: 10.1111/j.1574-6968.2000.tb08994.x
38. Hassan KA, Elbourne LDH, Tetu SG, Melville SB, Rood JI, Paulsen IT. Genomic analyses of Clostridium perfringens isolates from five toxinotypes. Res Microbiol. (2015) 166:255–63. doi: 10.1016/j.resmic.2014.10.003
39. Johansson A, Aspan A, Bagge E, Båverud V, Engström BE, Johansson. -E. Genetic diversity of Clostridium perfringens type A isolates from animals, food poisoning outbreaks and sludge. BMC Microbiol. (2006) 6:47. doi: 10.1186/1471-2180-6-47
40. Paine CM, Cherniak R. Composition of the capsular polysaccharides of Clostridium perfringens as a basis for their classification by chemotypes. Can J Microbiol. (1975) 21:181–5. doi: 10.1139/m75-026
41. Tang YW, Stratton CW, Tang YW. Advanced Techniques in Diagnostic Microbiology. New York, NY: Springer (2013). doi: 10.1007/978-1-4614-3970-7
42. Maslanka SE, Kerr JG, Williams G, Barbaree JM, Carson LA, Miller JM, et al. Molecular subtyping of Clostridium perfringens by pulsed-field gel electrophoresis to facilitate food-borne-disease outbreak investigations. J Clin Microbiol. (1999) 37:2209–14. doi: 10.1128/JCM.37.7.2209-2214.1999
43. Khan MUZ, Humza M, Yang S, Alvi MA, Iqbal MZ, Zain-Ul-Fatima H, et al. Occurrence and toxicogenetic profiling of Clostridium perfringens in buffalo and cattle: an update from Pakistan. Toxins. (2021) 13:212. doi: 10.3390/toxins13030212
Keywords: Clostridium perfringens, sampling zones, intestinal contents, toxinotyping, PFGE
Citation: Zafar Khan MU, Khalid S, Humza M, Yang S, Alvi MA, Munir T, Ahmad W, Iqbal MZ, Tahir MF, Liu Y and Zhang J (2022) Infection Dynamics of Clostridium perfringens Fingerprinting in Buffalo and Cattle of Punjab Province, Pakistan. Front. Vet. Sci. 9:762449. doi: 10.3389/fvets.2022.762449
Received: 07 October 2021; Accepted: 12 May 2022;
Published: 22 July 2022.
Edited by:
Ramon A. Juste, NEIKER Basque Institute for Agricultural Research and Development, SpainReviewed by:
Bharat Bhanderi, Kamdhenu University, IndiaKumaragurubaran Karthik, Tamil Nadu Veterinary and Animal Sciences University, India
Copyright © 2022 Zafar Khan, Khalid, Humza, Yang, Alvi, Munir, Ahmad, Iqbal, Tahir, Liu and Zhang. This is an open-access article distributed under the terms of the Creative Commons Attribution License (CC BY). The use, distribution or reproduction in other forums is permitted, provided the original author(s) and the copyright owner(s) are credited and that the original publication in this journal is cited, in accordance with accepted academic practice. No use, distribution or reproduction is permitted which does not comply with these terms.
*Correspondence: Yongsheng Liu, TGl1eXM0MDMxQGhldnR0Yy5lZHUuY24=; Jie Zhang, emhhbmdqaWUwM0BjYWFzLmNu
†These authors have contributed equally to this work