- 1Department of Biotechnology, College of Science, Taif University, Taif, Saudi Arabia
- 2Department of Science and Technology, University College-Ranyah, Taif University, Taif, Saudi Arabia
- 3Department of Biology, College of Science, Taif University, Taif, Saudi Arabia
- 4Department of Genetics, Faculty of Agriculture, University of Alexandria, Alexandria, Egypt
- 5Clinical Laboratory Sciences Department, Turabah University College, Taif University, Taif, Saudi Arabia
Acrylamide (ACR) has various effects on biological systems, including oxidative stress and its associated metabolic disorders. Previous research reports that plants growing at high altitude have a different profile of antioxidants. In the current report, the Taify pomegranate juice (TPJ) of the Taify pomegranate growing at the Taif region (high altitude), Saudi Arabia, was investigated for its protective activity from ACR-induced oxidative stress. Rats were treated with ACR, TPJ, or TPJ+ACR, and various assays, including blood chemistry, liver function biomarkers, gene expression of endogenous antioxidant enzymes, oxidative stress regulatory genes, inflammation biomarkers, and apoptosis, were estimated using biochemical, real-time PCR, histopathological, and immunohistochemical analysis. TPJ showed a protective function of ACR-induced alteration of AST, ALT, GGT, urea, total proteins, albumin, MDA, and NO. It also increased the level of the endogenous antioxidative enzymes, including SOD, catalase, and GSH. It showed anti-inflammatory activity by reduction the TNF-α, IL-6 secretion and the enhancing of IL-10 levels. At the gene expression level, TPJ upregulated the expression of endogenous antioxidant genes (SOD and catalase) and of antioxidant-regulating genes Nrf2 and HO-1; downregulated the expression of inflammatory genes TGF-β1, COX2, and the apoptotic gene caspase-3; and upregulated the expression of antiapoptotic gene Bcl2. At the histological level, TPJ showed a protective effect from the ACR-induced hepatic histological damage. Results of this study conclude that TPJ has a protective effect from ACR-induced oxidative stress and its associated metabolic alterations through its antioxidant and anti-inflammatory activities.
Introduction
Acrylamide (ACR) represents a major threat for human health. It is considered to be one of the most important chemical materials that is widely used in industry. It is also one of the inevitable contaminants in the occupational environment (1). Besides this, it is detected in the cooked starchy foods (1, 2). It was declared a carcinogenic agent in 1994 by The International Agency for Research on Cancer (3). Various studies report the wide range of ACR's negative impact on health, including oxidative stress and cancer (1, 4–8), neurotoxicity (1, 3, 4, 9, 10), reproductive toxicity (1, 4, 11), and developmental toxicity (1, 12). Acrylamide exerts its harmful effects by increasing the level of reactive oxygen species (ROS) and decreasing the antioxidant capacity via its deleterious effects to the endogenous antioxidant enzymes, including superoxide dismutase (SOD) and glutathione peroxidase (GSH-Px) (13, 14). Also, it provokes the production of inflammatory cytokines, including TNF-α and IL-1β (12, 14), and induces mitochondrial and caspase-dependent apoptosis (15, 16).
During the last two decades, the use of herbal therapy in counteracting the negative impact of oxidative stress, pollution, obesity, aging, and tissue deterioration and synthetic drugs as well as their associated metabolic disorders has gained a lot of research interest worldwide (17–21). Various terms were coined for natural products that are used in herbal therapy, including functional foods, food supplements, or nutraceuticals (17, 21). They are used for treatment of metabolic disorders associated with oxidative stress without having the side effects of chemically synthesized drugs (20). Their antioxidant activity is due to the presence of various phytochemicals (22), mainly including flavonoids and phenolic compounds (23–25). They are detected in a wide range of plant species, including cereals, fruits, vegetables, and oil seeds (19, 21, 26–29).
A plethora of investigations has been published reporting different strategies to combat ACR toxicity and its associated deleterious effects, especially the use of plant-driven phytochemicals (15). Among these natural compounds are thermoquinone and capsaicin (30), N-acetylcysteine (31–34), quercetin (35–37), punicalagin (38), vitamin E (39), carboxyflurene (40), cyaniding-3-O-glucoside (41), and thymoquinone (8, 42). Similarly, plant extracts are used to counteract the impact of ACR, including Trigonella foenum-graecum seed oil (43); Spirulina platensis (44); Tetrastigma hemsleyanum leaf extract (45); Portulaca oleracea seed extract (46); white tea and raspberry ketone (47); hesperidin and tiger nut (48); olive oil hydroxytyrosol (49); tea polyphenols and resveratrol (50); and strawberry, grape, and blueberry powder (51).
Chemical constituents of plant extracts and essential oil differ based on geographical locations, altitude, and genotypes. Samples collected from 20 different geographical locations of Ducrosia anethifolia showed variations in essential oil (52). Chemical constituents of pomegranate fruits vary with climate conditions (53). Phytochemical variations among genotypes are also reported in tomato (54). Metabolite analysis of Curcuma longa shows differences up to 20% due to different agroclimatic regions (55).
Plants respond to high altitude by producing higher levels of a wide array of antioxidants compared with plants growing at lower altitude (56, 57) or producing de novo compounds not detected at normal elevations (58, 59). For example, a significant increase in total phenolics and antioxidant activity was reported in the methanolic extract of Scrophularia striata growing at an elevation of 600 m above sea level (60). Similarly, high altitude is negatively correlated with the content of tannin and positively correlated with flavonoids, rutin, total phenolic content, and antioxidant capacity of Potentilla fruticosa L. extract (61). In addition, phytochemical activity of Thalictrum foliolosum root extract collected from different altitudes shows that berberine content was negatively correlated with altitude while total phenolics and flavonoid as well as antioxidant capacity were positively correlated with altitude (62). The phenolic content and antioxidant capacity of two major Rhodiola species differed in samples collected from different elevations based on the differential abundance of 178 flavonoids, which positively correlated with elevation (63). The phenol and flavonoid contents Thalictrum foliolosum as well as antioxidant capacity increased at higher altitudes (62). Therefore, phytochemical content of plants depends on various factors, especially their elevation above sea level.
Pomegranate juice (PJ) shows a wide spectrum of effects, including antioxidant activity (64–75), antimutagenic activity (65), antiviral activity (76), antiobesity (19, 77), anticryptosporidial (78), and amelioration of neurodegenerative diseases (79). Also, pomegranate peel shows antioxidant (64, 68, 80, 81) and antimicrobial activity (82). In addition, several reports document that pomegranate seed extract presents antioxidant activity (64, 68, 83, 84).
Taif is a high-altitude region elevated about 1,200–2,300 m above sea level, which makes it suitable for a wide variety of indigenous plant species (85). This makes it unique in weather and plant flora. Among the most important Taif indigenous fruit plants are grape and pomegranate, Punica granatum L. (Lythraceae). The most cultivated variety of pomegranate is known as Taify, which has special cultural, nutritional, and commercial value in the Kingdom of Saudi Arabia (19). The elevated location of Taif as an indigenous habitat for Taify pomegranate makes this variety a potential special antioxidant source.
The previous theoretical framework detailed that few studies have been conducted to investigate the different aspects of Taify pomegranate (19, 86), which does not correlate with its economic, nutritional, and cultural value. Therefore, the focus of the current study was to investigate the antioxidant activity of Taify pomegranate juice (TPJ) against ACR-induced oxidative stress on the liver of rats. Various assays including biochemical, molecular, inflammatory, apoptotic, and histopathological were employed to achieve this goal.
Materials and Methods
Plant Samples and Extraction
Taify pomegranate fruits were collected from their natural habitat in the Taif region, Taif Governorate, Saudi Arabia. Intact seeds were collected from healthy ripened fruits, and TPJ was extracted by hand squeezing in cheesecloth without disrupting seed structure to get only the juice components. TPJ was filtered using a 0.22-μm filter and administered fresh to rats.
Animals and Treatments
Forty male rats (Ratus norvegicus) 10 weeks of age were used in this study. Animals were cared for at room temperature with free access to food and water for 7 days for acclimation at Turabah University labs. Animals were randomly and equally distributed among four groups. Group 1 served as the negative control and was given an equal volume of water. Group 2 orally received 20 mg/kg bw of acrylamide and served as the positive control (ACR group) (87, 88). This was reported to induce total liver toxicity (89). Group 3 (TPJ) orally received 2 ml/Kg bw of TPJ for 3 weeks (90). Group 4 (TPJ+ACR), the protective group, received 20 mg/kg bw of ACR and 2 ml/kg bw of pomegranate juice. TPJ administration started 1 week earlier than ACR administration for the protective effect. Treatments continued for 3 weeks. After the experimental period, animals were anesthetized using isoflurane, decapitated, and dissected. Blood samples were collected, and serum was separated and stored at −20°C for blood chemistry assays. Liver tissue samples were collected in QIAZOL reagent for RNA isolation and quantitative real-time PCR (qRT-PCR) analysis of gene expression. Liver samples were kept in Bowan's solution for histological and immunohistochemical analysis.
Blood Biochemical Analysis
The serum levels of ALT, AST, GGT, and urea were measured using a colorimetric spectrophotometer as described in the instruction manual. Malondialdehyde (MDA) was measured according to a previously described method (91). Catalase, superoxide dismutase (SOD), and nitric oxide (NO) were estimated according to previously reported methods (92, 93). Well-established methods were employed to measure albumin (94) and total proteins (95). GSH was estimated according to the Tietze method (96) using spectrophotometric absorbance at 412 nm.
Analysis of Inflammation and Anti-inflammation Cytokines
IL-6 and TNF-α were estimated using specific ELISA (ab100768 and ab46070, respectively) kits and spectrophotometric analysis according to the kits' instructions. IL-10 was measured using a commercial kit obtained from Abcam, USA (Rat IL-10 ELISA Kit, ab100765). Data obtained from the ELISA reader were calculated as described in the kit instructions.
Gene Expression Quantitation by Real-Time PCR
Total RNA was isolated from liver samples using QIAZOL according to the manufacturer's instructions, 50 μg per 1 ml of QIAZOL. Concentration of the isolated RNA was estimated spectrophotometrically (BIORAD, USA) at 260 nm. Total RNA, 2 μg, was used as a template for reverse transcriptase for cDNA synthesis (MyTaq Red Mix, Bioline). cDNA was used as a template for qPCR amplification of various liver using SYBR Green master mix (Thermo scientific, USA). Primers were synthesized at Macrogen Company (Seoul, Korea). Information of primers used in qPCR amplification are summarized in Table 1. The obtained qPCR data was analyzed using the 2−ΔΔCt method of the CFX96 Touch Real-Time PCR (Bio-Rad, USA). Beta-actin gene expression was used as a reference for estimation of gene expression.
Histopathology and Immunohistochemistry
Liver tissue samples were fixed in neutral buffered formalin (10%). Fixed samples were processed and stained with hematoxylin and eosin as described by Bancroft et al. (97). The immunohistochemical protocol was conducted following the method of Saber et al. (98). Wax was removed from tissue sections, and they were rinsed in 0.05 M citrate buffer, pH 6.8. Non-specific binding was blocked by treating the sections with 0.3% H2O2 and a protein block. Sections were subjected to rabbit monoclonal (anti-bcl2, Abcam, Cat# ab182858, dilution 1:500) primary antibody. Sections were washed in phosphate-buffered saline and subjected to goat antirabbit secondary antibody (EnVision System Horseradish Peroxidase Labeled Polymer; Dako) for 30 min at room temperature. Slides were visualized with DAB kit and counterstained using Mayer's hematoxylin. The immunolabeling indices of Bcl2 are presented as a percentage of positive expression in a total of 1,000 cells per eight high power fields (HPF).
Statistical Analysis
The SPSS program (IBM, Chicago, IL, USA) was used to perform one way ANOVA of the obtained data. Means were separated using Duncan multiple range test (DMRT) at P ≤ 0.05. Values were presented as means ± SE.
Results
Blood Chemistry
The capability of TPJ to rectify the impact of ACR on the level of liver function enzymes as well as some essential blood parameters was tested. TPJ alone did not induce significant changes in all estimated parameters compared with the control group. ACR increased AST (4.7-fold) and ALT (4.2-fold) compared with controls. Co-treatment of TPJ+ACR reduced the high scores of AST and ALT compared with the ACR group (Table 2). Also, ACR reduced the GGT level to almost 0.5-fold compared with the control and TPJ groups while co-treatment of TPJ+ACR highly increased the GGT level to an insignificant level compared with the control and TPJ groups (Table 2). Urea level was increased by ACR to about 3.45-fold compared with the control. The TPJ had a protective effect against ACR by which it reduced the urea level to 1.3-fold of the control group. Albumin and total proteins were reduced by ACR to 0.39-fold and 0.45-fold of the control, consecutively, while the TPJ had a protective effect against the ACR effect by increasing albumin and total proteins to an insignificant level compared with the control and TPJ (Table 2).
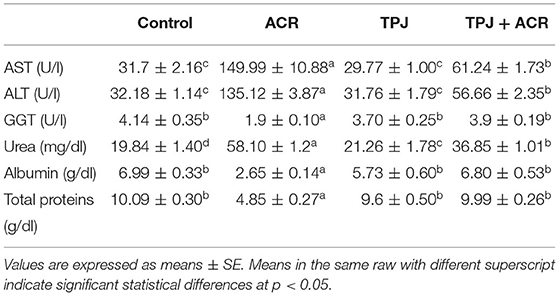
Table 2. Protective effect of Taify Pomegranate juice (TPJ) on ACR-induced liver dysfunction in rats.
In vivo Antioxidant Capacity of TPJ
ACR induced a significant increase in MDA (3-fold) and NO (3-fold) levels. It also decreased the level of antioxidant enzymes, including catalase (0.4-fold), SOD (0.56-fold), and GSH (0.5-fold) compared with the control (Table 3). TPJ alone reduced the MDA level below its level in the control and increased the SOD and GSH levels compared with the control, whereas it did not have significant changes on the catalase and the NO levels (Table 3). Co-treatment of TPJ+ACR showed a protective effect of the ACR oxidative stress. This was indicated by significant reduction of MDA, increase of catalase, SOD, and GSH, and decrease of NO compared with the ACR group (Table 3).

Table 3. Protective effects of Taify pomegranate juice (TPJ) against ACR-induced alterations on serum MDA, catalase, SOD, GSH, and NO levels.
Anti-inflammatory Effect of TPJ
TPJ alone enhanced the level of the anti-inflammatory cytokine IL-10 while ACR reduced its level compared with the control. Co-administration of TPJ and ACR abrogated the ACR effect on the IL-10 (Table 4). TPJ reduced the inflammatory cytokine TNF-α compared with the control, whereas ACR significantly induced higher levels of TNF-α compared with the control. Co-administration of TPJ and ACR significantly reduced TNF-α levels compared with the control group (Table 4). Similarly, ACR induced high levels of the inflammatory cytokine IL-6, and PJ alone reduced the IL-6 levels to insignificant state compared with the control. ACR administration with TPJ highly and significantly reduced IL-6 production, indicating that TPJ has an anti-inflammatory effect that was able to rectify the ACR increase of IL-6, TNF-α, and the decrease of the anti-inflammatory IL-10.
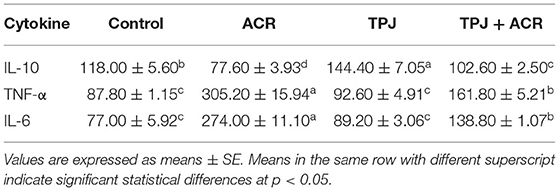
Table 4. Protective effects of Taify Pomegranate juice (TPJ) against ACR-induced alterations in serum cytokine levels.
TPJ Abrogated the ACR-Induced Suppression of Endogenous Antioxidant Genes SOD and Catalase
To investigate the capability of TPJ to rectify the ACR-induced oxidative stress, its effect on the expression of the endogenous antioxidative enzymes was investigated. Treatment with ACR reduced catalase expression, whereas treatment with TPJ alone induced its expression compared with the control. On the other hand, co-treatment of TPJ and ACR rectified the catalase expression to a level close to the control and significantly higher than its level in the ACR group (Figure 1A). Also, the expression of SOD was downregulated by ACR while TPJ alone upregulated its expression compared with the control group. Co-treatment of TPJ and ACR had a protective effect of ACR-induced downregulation of SOD compared with the control, where the TPJ was able to rectify the ACR-induced reduction in SOD level (Figure 1B).
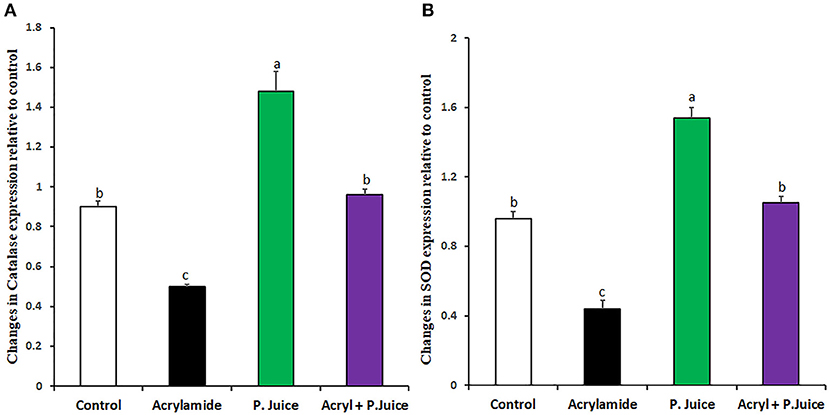
Figure 1. Antioxidant enzyme gene expression in response to ACR and TPJ of Catalase (A) and SOD (B). Values are expressed as means ± SE. Means with different letters are significant (p < 0.05).
TPJ Rectified the ACR-Downregulation of Nrf2/HO-1 Axis
ACR and TPJ had diverse effects on the cytoprotective antioxidant marker genes nuclear factor-erythroid 2-related factor 2 (Nrf2) and Heme Oxygenase-1 (HO-1). While ACR greatly suppressed the Nrf2 to 0.31-fold of the control, TPJ enhanced its expression to 1.2-fold compared with the control group. Co-administration of TPJ and ACR significantly normalized the expression of Nrf2 compared with the control or the TPJ groups, yet it was still significantly higher compared with the ACR group (Figure 2A) giving an indication that TPJ had a protective effect from ACR-induced suppression of cytoprotective antioxidant Nrf2 gene. Regarding HO-1 gene, ACR and TPJ had similar expression profile to that of Nrf2. ACR significantly suppressed HO-1 to 0.44-fold of the control; meanwhile, TPJ enhanced its expression level to 1.2-fold of the control. Co-administration of PJ and ACR enhanced its expression to 0.84-fold of the control (Figure 2B), providing evidence that TPJ had a protective effect against ACR-induced oxidative stress.
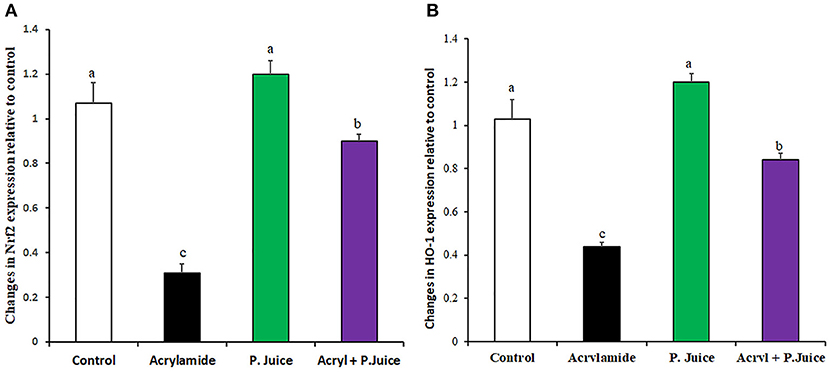
Figure 2. Gene expression of oxidative stress marker genes Nrf2 (A) and HO-1 (B) in response to ACR and the TPJ in rat liver. Values are expressed as means ± SE. Means with different letters are significant (p < 0.05).
Anti-inflammatory Activity of TPJ
The protective effect of TPJ from inflammation was estimated by modulating gene expression of the inflammatory transforming growth factor β1 (TGF-β1) and cyclooxygenase2 (COX2). ACR upregulated the TGF-β1 about 2.3-fold while TPJ downregulated its expression to 0.88-fold of the control. Co-treatment of ACR and TPJ indicated that TPJ abrogated the ACR effect and downregulated the TGF-β1 expression to 1.35-fold of the control, concluding its protective effect (Figure 3A). Treatment with ACR highly induced the expression of the inflammatory COX2 compared with the control with about 1.9-fold compared to the control. TPJ reduced the expression of COX2 to a level close to the control level (1.06-fold). Co-administration of ACR and TPJ reduced the COX2 expression to 1.32-fold of the control, indicating the protective effect of the TPJ (Figure 3B).
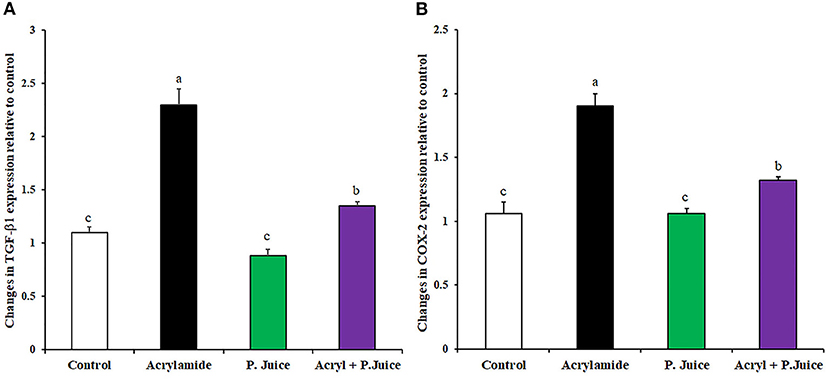
Figure 3. Gene expression of fibrosis-associated genes TGF-B1 (A) and COX2 (B) in response to ACR and the TPJ in rat liver. Values are expressed as means ± SE. Means with different letters are significant (p < 0.05).
Anti-apoptotic Activity of TPJ
The apoptotic caspase-3 gene expression was induced by ACR about 1.85-fold, whereas the TPJ significantly reduced its expression to 0.54-fold compared with the control. When TPJ was co-administered with ACR, the TPJ rectified the caspase-3 expression to 1.09-fold of the control (Figure 4A). On the contrary, to the caspase-3 case, ACR was able to suppress the antiapoptotic gene Bcl2 to 0.47-fold while TPJ upregulated the Bcl2 gene 1.39-fold compared with the control. Co-treatment of ACR along with the TPJ showed a protective effect through the enhancement of the Bcl2 gene to a comparable level (1.05-fold) to the control group (Figure 4B).
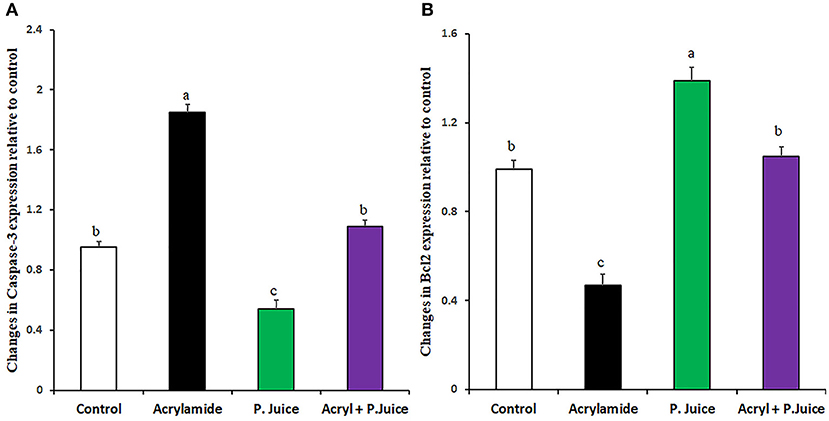
Figure 4. Gene expression of the apoptotic Caspase-3 (A) and the antiapoptotic Bcl2 (B) genes in response to ACR and the TPJ in rat liver. Values are expressed as means ± SE. Means with different letters are significant (p < 0.05).
Histopathological Protection of TPJ
Sections from normal and TPJ administered rats (Figures 5A,B) displayed normal features of hepatic parenchyma organized in hepatic cords radiating from the central veins (CV) at the center of the hepatic lobule toward the lobular periphery. Sections from acrylamide-treated rats (Figure 5C) showed degenerative changes as indicated from vacuolar hepatocytes (arrows). Sections from acrylamide-treated rats (Figure 5C) showed that vascular degeneration (v) and hydrobic degeneration (hy) of hepatocytes. The liver tissues showed proliferation of the vonkupher cells (k). The blood sinusoids showed cellular infiltrates. Some hepatocyte cells were characterized by necrosis (n). The co-treatment of rats with acrylamide and TPJ (Figure 5D) showed less vacuolar and hydrobic degeneration in some hepatic cells. The Kupffer cells still proliferated but were not numerous compared with the acrylamide group. The detected degenerative changes are illustrated in Table 5. Co-treatment of rats with acrylamide and TPJ (Figure 5D) reduced the degenerative changes and protected rats from the bad effects of ACR. Scale bar = 20 μm (original magnification = 200 ×).
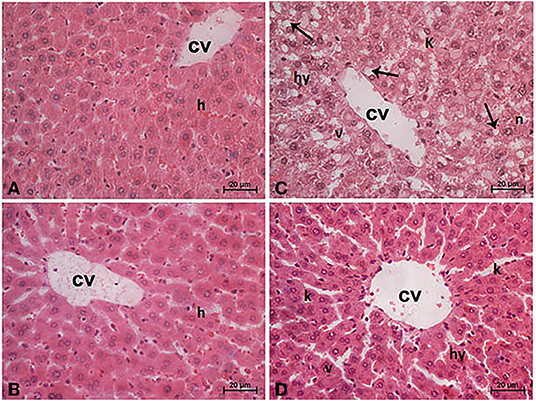
Figure 5. Photomicrographs of liver tissues stained with hematoxylin and eosin in the control and TPJ group (A,B), the acrylamide-treated group (C), and the group co-treated with acrylamide and TPJ (D). Sections from control and TPJ groups (A,C) show the liver consisted of CV surrounded by hepatic cords (h). The cords consisted of large hepatocytes with centrally located nuclei and acidophilic cytoplasm. Sections from the acrylamide-treated rats (B) the liver tissue showed vacular degeneration (v), hydrobic degeneration (hy). The liver tissues showed proliferation of the vonkupher cells (k). The blood sinusoids showed cellular infiltrates (Table 5). Co-treatment of rats with acrylamide and TPJ (D) showed less vacuolar and hydrobic degeneration in some hepatocytes. Some vonkupher cells still proliferated but were not numerous compared with the acrylamide group. Scale bar = 20 μm (original magnification = 200 ×). The morphometric and scoring of hepatic lesions are shown in Table 5.
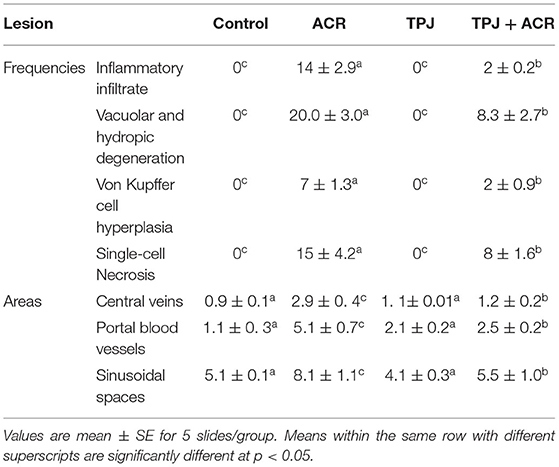
Table 5. Ameliorative impacts of TPJ against morphometric analysis and score lesions induced by acrylamide rat's liver.
The intensity of Bcl2 immunostaining was remarkable in control (Figure 6A) and TPJ (Figure 6B) as indicated from the strongly positive hepatocytes (arrows), reduced in the acrylamide group (Figure 6C) and partial restored to fair levels in pretreated rats (Figure 6D). The reactive liver cells (arrows) are usually next to CV. Reactive hepatocytes are marked with arrows. Scale bar = 20 μm (original magnification = 400 ×). This immunostaining of Bcl2 is shown in the form of densitometric score and is correlated with the estimated immunoreactivity (Figure 6E) of immunostained liver sections (Figures 6A–D), confirming the protective effect of TPJ.
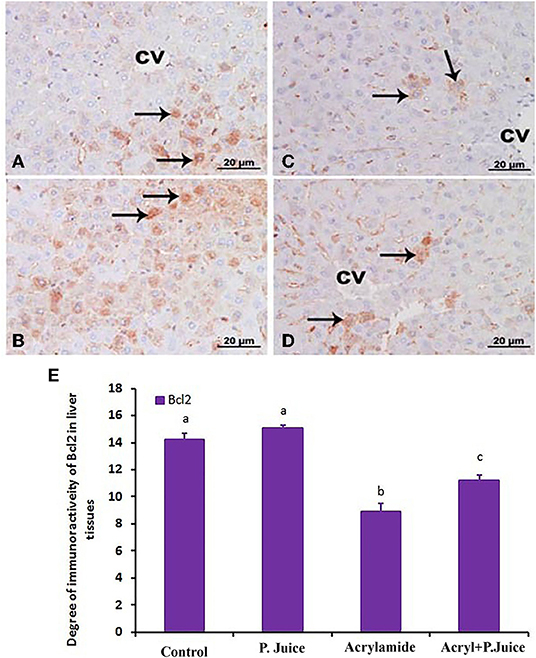
Figure 6. Photomicrographs of hepatic sections immunostained with Bcl2 antibody in the control group (A), the TPJ group (B), the acrylamide-treated group (C), and the group co-treated with TPJ and acrylamide (D). The intensity of immunostaining was remarkable in the control and TPJ-treated groups (A,B), less in the acrylamide-treated group (C), and ameliorated and restored in the co-treated rats (D). Reactive liver cells (arrows) are frequently seen next to CVs. Scale bar = 20 μm (original magnification = 200 ×). The degree of positive immunoreactivity for Bcl2 is graphed in (E). Densitometric values are statistically significant at *p < 0.05 vs. the control and TPJ-treated groups; #p < 0.05 vs. the acrylamide-administered group. In panel E, means with different letters indicate significance (p < 0.05).
Discussion
Plant-derived phytochemicals collectively known as nutraceuticals have biological functions for protection against chronic disease, improving health, delaying aging, or supporting various functions of the body (99). They have gained increasing interest for their nutritional, pharmaceutical, safety, and protective roles against oxidative stress-related metabolic disorders, including allergy, cardiovascular diseases, cancer, diabetes, inflammation, and obesity (99). It has been established that nutraceuticals exert their biological functions through their content of bioactive compounds, mainly polyphenols and flavonoids (100). Besides their direct scavenging activity of ROS, they also have the capability to inhibit ROS production by neutrophils and the inhibition inducible-nitric oxide synthases (iNOS) (100). Pomegranate is consumed fresh or used in cosmetic and nutraceutical products because of its antioxidant, anti-inflammatory, anti-microbial, and antiproliferative attributes, which is based on its content of functional phytochemicals (101).
Our results show that TPJ was able to decrease the ACR-induced increase of AST, ALT, GGT, urea, and total protein when co-administered with ACR. Our results agree with the results obtained from other several studies. PJ showed a protective effect from non-alcoholic fatty liver disease (NAFLD) induced by a high fat diet via lowering the level of AST, ALT, insulin, triglycerides, and glucose compared with the control (102). Also, in NAFLD, PJ decreased the liver enzymes AST and ALT while it increased the antioxidant capacity (103). In addition, similar results were observed using plant-derived products. Trigonella foenum-graecum seed oil showed a protective effect against ACR toxicity. While ACR increased serum levels AST, ALT, GGT, and urea, Tigonella seed oil supplementation rectified the changed serum parameters and enhanced the antioxidant capacity in the hepatic cells, indicating its protective effects against ACR-induced oxidative stress (43). Furthermore, previous reports show that some PJ active constituents had consistent activity against ACR-induced oxidative stress and its associated metabolic alterations. For example, administration of 50 mg/kg bw quercetin had a protective effect against ACR-induced toxicity through the reduction of oxidative stress and protection from mitochondrial dysfunction (35, 37). Ellagic acid showed protection from ACR-induced neurotoxicity (13). Myricetin had a counteracting activity of ACR-induced oxidative stress through the inhibition of the MEK/ERK signaling pathway (104).
In the current study, ACR increased urea and decreased albumin and total proteins, and TPJ normalized these serum parameters. This aligned with other reported biological activities of PJ. It presented protective effects against sodium fluoride oxidative damages in liver tissue and erythrocytes of rats. It showed an ameliorative effect on hematological parameters, rectified the total protein, albumin, bilirubin levels, and the activities of hepatic marker enzymes (105).
The increase of MDA and NO as well as the decrease in catalase, SOD, and GSH in the current study is in line with the results obtained from previous studies. PJ had protective effect against ACR-induced oxidative stress through the reduction of MDA with increased antioxidant capacity in NAFLD patients (103). Also, PJ compromised the higher levels of lead acetate–induced MDA and GSH, indicating a protective role against lead acetate–induced oxidative stress (106). Similarly, PJ presented a protective effect against the anxiety and depression induced by aluminum trichloride exposure through the enhancement of catalase, SOD, GST, and GSH (107). In addition, PJ showed antifibrotic activity against NDEA-induced liver fibrosis via increasing SOD, GST, and catalase levels (108) and enhanced the antioxidant enzymes, including SOD, catalase, and the GSH levels in liver, kidney, and testis tissues against lead-induced oxidative stress (109). PJ had a protective effect of diethylnitrosamine and phenobarbital-induced hepatic damage by reducing the level of MDA, glutathione reductase (GSR), decreased SOD and GST that were altered by diethylnitrosamine and phenobarbital (110). Prolonged ingestion of PJ alleviated the impact of systemic oxidative stress in mice by lowering oxidative biomarkers including catalase, SOD, GSH, and GSSG (111). High altitude-induced hypoxia and its associated metabolic alterations were protected by PJ via lowering the level of lipid peroxidation in muscle, rectifying the GSH:GSSG ratio (112).
Endogenous antioxidant enzymes, including SOD, catalase, and glutathione peroxidase are induced by oxidative stress through the binding of nuclear factor-erythroid 2-related factor 2 (Nrf2) transcription factor to the antioxidant responsive elements (ARE) in their promoter regions (100, 113). Nrf2 regulates ARE-regulated genes (113, 114). Normally, Nrf2 is bound to kelch-like protein-1 (KEAP1) and localized in the cytoplasm and under oxidative stress, and Nrf2 is released and translocated to the nucleus. In the nucleus, it induces the transcription of antioxidant enzymes and provokes the transcription of HO-1 expression. Also, oxidative stress is the stimulant of nuclear factor-kappa B (NF-κB), inflammatory cytokines, such as interleukin (IL)-6, IL-17, and tumor necrosis factor-alpha (TNF-α) (115). TNF-α is an inflammatory cytokine that is responsible for a wide range of molecular and signaling pathways underlying necrosis and/or apoptosis. It also plays an indispensable role in cancer and infection resistance (116).
ACR downregulated the antioxidant activating genes Nrf2, HO-1; upregulated the inflammatory genes TGF-1β, and COX2; induced the inflammatory cytokines IL-6 and TNF-α, and reduced the anti-inflammatory cytokines IL-10. On the other hand, preadministration of TPJ rectified these inflammatory and anti-inflammatory parameters. This provides strong evidence that TPJ exerts an anti-inflammatory activity against ACR-induced oxidative and inflammatory stress. Several previous studies report results in line with ours. CuO-NPs-induced oxidative stress was compromised using PJ via the downregulation of the Nrf2/HO-1 axis (117). In addition, PJ downregulated the expression of COX2 and Nrf2 induced by NDEA in fibrotic rat liver (108). Trigonella foenum-graecum seed oil reduced the ACR-elevated levels of inflammatory cytokines, including IL-1β, IL-6, and TNF-α (43).
TPJ normalized the altered expression profile of caspase-3 and Bcl2 genes altered by ACR, which agrees with previous published studies. PJ downregulated the caspase-3 induced by diethylnitrosamine and phenobarbital (110) and downregulated the CuO-NPs-induced high expression of caspase-3 (117). Garlic and Spirulina maxima had a protective effect from Pb-induced neurotoxicity, including the proapoptotic associated increase of caspase-3 expression as well as the altered acetyl cholinesterase enzyme activity and oxidative stress parameters (118).
At the histopathology level, TPJ protected hepatic tissue from the ACR-induced histopathological effects. Similar activities of PJ are reported by other authors. PJ reduced the histological changes associated with non-alcoholic fatty liver disease, including disturbed hepatic architecture, dilatation and congestion of central veins, blood sinusoids and portal veins, cytoplasmic vacuolation, mitochondrial structural changes, dilatation of endoplasmic reticulum in addition to nuclear structural changes like condensed chromatin, irregular shrunken nuclei and vacuolated nuclei, inflammatory cellular infiltrations, deposition of collagen fibers around the central vein, blood sinusoids, portal areas and in between the hepatocytes in addition to significant increase in number of hepatic stellate cells that was proved by electron microscope and confirmed by immunohistochemical study (119). Also, PJ was able to protect tissue damage induced by lead acetate in kidney, liver, and heart as well as reduced the accumulation of lead in kidney and testis (109). In addition, PJ had a significant ameliorative effect against cisplatin-induced renal damage at the histopathological level, giving evidence that PJ could be used as dietary supplement for individuals during chemotherapy treatments (120). Moreover, PJ reduce the NAFLD-associated hepatic steatosis, ballooning, lobular inflammation, and portal inflammation (102).
The obtained results indicate that TPJ had an integrated protection effect against ACR at various levels, including serum parameters, gene expression in tissues, and histological parameters. This integrated protection of PJ was observed in other previous studies (90, 106, 109).
The represented biological activities of TPJ, including antioxidative, anti-inflammatory, and antiapoptotic activities could be attributed to its components of various compounds with previously shown beneficial biological activities. Also, prepared from plants growing at high altitude, the TPJ has some unique components, such as syringic acid, quercetin, naringenin, and myricetin, that might have contributed to its biological activities reported in the current study. In conclusion, TPJ has the potential to ameliorate the toxicity of acrylamide through the regulation of anti-inflammatory, anti-apoptotic, and antioxidant molecular pathways.
Data Availability Statement
The raw data supporting the conclusions of this article will be made available by the authors, without undue reservation.
Ethics Statement
The animal study was reviewed and approved by Ethical committee, Taif University.
Author Contributions
All authors listed have made a substantial, direct, and intellectual contribution to the work and approved it for publication.
Funding
This study was supported by the Deputyship for Research and Innovation, Ministry of Education, in Saudi Arabia under project number 1-441-128.
Conflict of Interest
The authors declare that the research was conducted in the absence of any commercial or financial relationships that could be construed as a potential conflict of interest.
Publisher's Note
All claims expressed in this article are solely those of the authors and do not necessarily represent those of their affiliated organizations, or those of the publisher, the editors and the reviewers. Any product that may be evaluated in this article, or claim that may be made by its manufacturer, is not guaranteed or endorsed by the publisher.
Acknowledgments
The authors extend their appreciation to the Deputyship for Research and Innovation, Ministry of Education, in Saudi Arabia for funding this research work through project number 1-441-128.
References
2. Kopanska M, Muchacka R, Czech J, Batoryna M, Formicki G. Acrylamide toxicity and cholinergic nervous system. J Physiol Pharmacol. (2018) 69. doi: 10.26402/jpp.2018.6.03
3. Koszucka A, Nowak A, Nowak I, Motyl I. Acrylamide in human diet, its metabolism, toxicity, inactivation and the associated European Union legal regulations in food industry. Crit Rev Food Sci Nutr. (2020) 60:1677–92. doi: 10.1080/10408398.2019.1588222
4. Imai T, Kitahashi T. A 13-week toxicity study of acrylamide administered in drinking water to hamsters. J Appl Toxicol. (2014) 34:57–65. doi: 10.1002/jat.2831
5. Huang M, Jiao J, Wang J, Xia Z, Zhang Y. Characterization of acrylamide-induced oxidative stress and cardiovascular toxicity in zebrafish embryos. J Hazard Mater. (2018) 347:451–60. doi: 10.1016/j.jhazmat.2018.01.016
6. Komoike Y, Matsuoka M. In vitro and in vivo studies of oxidative stress responses against acrylamide toxicity in zebrafish. J Hazard Mater. (2019) 365:430–9. doi: 10.1016/j.jhazmat.2018.11.023
7. Rifai L, Saleh FA. A review on acrylamide in food: occurrence, toxicity, and mitigation strategies. Int J Toxicol. (2020) 39:93–102. doi: 10.1177/1091581820902405
8. Tabeshpour J, Mehri S, Abnous K, Hosseinzadeh H. Role of oxidative stress, mapkinase and apoptosis pathways in the protective effects of thymoquinone against acrylamide-induced central nervous system toxicity in rat. Neurochem Res. (2020) 45:254–67. doi: 10.1007/s11064-019-02908-z
9. Murray SM, Waddell BM, Wu CW. Neuron-specific toxicity of chronic acrylamide exposure in C. elegans. Neurotoxicol Teratol. (2020) 77:106848. doi: 10.1016/j.ntt.2019.106848
10. Lin Z, Luo P, Huang D, Wu Y, Li F, Liu H. Multi-omics based strategy for toxicity analysis of acrylamide in Saccharomyces cerevisiae model. Chem Biol Interact. (2021) 349:109682. doi: 10.1016/j.cbi.2021.109682
11. Song HX, Wang R, Cao SX, Liu TZ. [Toxicity of acrylamide on male reproduction]. Zhonghua Nan Ke Xue. (2008) 14:159–62.
12. Zhu F, Wang J, Jiao J, Zhang Y. Exposure to acrylamide induces skeletal developmental toxicity in zebrafish and rat embryos. Environ Pollut. (2021) 271:116395. doi: 10.1016/j.envpol.2020.116395
13. Goudarzi M, Mombeini MA, Fatemi I, Aminzadeh A, Kalantari H, Nesari A, et al. Neuroprotective effects of Ellagic acid against acrylamide-induced neurotoxicity in rats. Neurol Res. (2019) 41:419–28. doi: 10.1080/01616412.2019.1576319
14. Guo J, Cao X, Hu X, Li S, Wang J. The anti-apoptotic, antioxidant and anti-inflammatory effects of curcumin on acrylamide-induced neurotoxicity in rats. BMC Pharmacol Toxicol. (2020) 21:62. doi: 10.1186/s40360-020-00440-3
15. Kunnel SG, Subramanya S, Satapathy P, Sahoo I, Zameer F. Acrylamide induced toxicity and the propensity of phytochemicals in amelioration: a review. Cent Nerv Syst Agents Med Chem. (2019) 19:100–13. doi: 10.2174/1871524919666190207160236
16. Deng L, Zhao M, Cui Y, Xia Q, Jiang L, Yin H, et al. Acrylamide induces intrinsic apoptosis and inhibits protective autophagy via the ROS mediated mitochondrial dysfunction pathway in U87-MG cells. Drug Chem Toxicol. (2021) 1–12. doi: 10.1080/01480545.2021.1979030
17. Hardy G. Nutraceuticals and functional foods: introduction and meaning. Nutrition. (2000) 16:688–9. doi: 10.1016/S0899-9007(00)00332-4
18. Bukhari SB, Iqbal S, Bhanger MI. Antioxidant potential of commercially available cumin (Cuminum cyminuml inn) in Pakistan. Int J Food Sci Nutr. (2009) 60:240–7. doi: 10.1080/09637480701695583
19. Ahmed MM, Samir el SA, El-Shehawi AM, Alkafafy ME. Anti-obesity effects of Taif and Egyptian pomegranates: molecular study. Biosci Biotechnol Biochem. (2015) 79:598–609. doi: 10.1080/09168451.2014.982505
20. Santini A, Cammarata SM, Capone G, Ianaro A, Tenore GC, Pani L, et al. Nutraceuticals: opening the debate for a regulatory framework. Br J Clin Pharmacol. (2018) 84:659–72. doi: 10.1111/bcp.13496
21. Sayed S, Ahmed M, El-Shehawi A, Alkafafy M, Al-Otaibi S, El-Sawy H, et al. Ginger water reduces body weight gain and improves energy expenditure in rats. Foods. (2020) 9:38. doi: 10.3390/foods9010038
22. Zheng W, Wang SY. Antioxidant activity and phenolic compounds in selected herbs. J Agric Food Chem. (2001) 49:5165–70. doi: 10.1021/jf010697n
23. Ramesh B, Satakopan VN. Antioxidant activities of hydroalcoholic extract of Ocimum sanctum against cadmium induced toxicity in rats. Indian J Clin Biochem. (2010) 25:307–10. doi: 10.1007/s12291-010-0039-5
24. Cohen MM. Tulsi - Ocimum sanctum: a herb for all reasons. J Ayurveda Integr Med. (2014) 5:251–9. doi: 10.4103/0975-9476.146554
25. Ge G, Zhang Q, Ma J, Qiao Z, Huang J, Cheng W, et al. Protective effect of Salvia miltiorrhiza aqueous extract on myocardium oxidative injury in ischemic-reperfusion rats. Gene. (2014) 546:97–103. doi: 10.1016/j.gene.2014.05.021
26. Naczk M, Shahidi F. Phenolics in cereals, fruits and vegetables: occurrence, extraction and analysis. J Pharm Biomed Anal. (2006) 41:1523–42. doi: 10.1016/j.jpba.2006.04.002
27. Borneo R, Aguirre A, León AE. Chia (Salvia hispanica L.) gel can be used as egg or oil replacer in cake formulations. J Am Diet Assoc. (2010) 110:946–9. doi: 10.1016/j.jada.2010.03.011
28. Kratchanova M, Denev P, Ciz M, Lojek A, Mihailov A. Evaluation of antioxidant activity of medicinal plants containing polyphenol compounds. Comparison of two extraction systems. Acta Biochim Pol. (2010) 57:229–34. doi: 10.18388/abp.2010_2399
29. Khalaf AA, Moselhy WA, Abdel-Hamed MI. The protective effect of green tea extract on lead induced oxidative and DNA damage on rat brain. Neurotoxicology. (2012) 33:280–9. doi: 10.1016/j.neuro.2012.02.003
30. Abd Al Haleem EN, Hasan WYS, Arafa HMM. Therapeutic effects of thymoquinone or capsaicin on acrylamide-induced reproductive toxicity in rats mediated by their effect on oxidative stress, inflammation, and tight junction integrity. Drug Chem Toxicol. (2021) 1–13. doi: 10.1080/01480545.2021.1942485
31. Lee KY, Shibutani M, Kuroiwa K, Takagi H, Inoue K, Nishikawa H, et al. Chemoprevention of acrylamide toxicity by antioxidative agents in rats–effective suppression of testicular toxicity by phenylethyl isothiocyanate. Arch Toxicol. (2005) 79:531–41. doi: 10.1007/s00204-005-0656-6
32. Williams JR, Rayburn JR, Cline GR, Sauterer R, Friedman M. Potential protective effect of L-cysteine against the toxicity of acrylamide and furan in exposed Xenopus laevis embryos: an interaction study. J Agric Food Chem. (2014) 62:7927–38. doi: 10.1021/jf5013743
33. Altinoz E, Turkoz Y, Vardi N. The protective effect of N-acetylcysteine against acrylamide toxicity in liver and small and large intestine tissues. Bratisl Lek Listy. (2015) 116:252–8. doi: 10.4149/BLL_2015_049
34. Wang D, Qi J, Pan X, Yan D, Yan H. [The antagonistic effect and mechanism of N-acetylcysteine on acrylamide-induced hepatic and renal toxicity]. Zhonghua Lao Dong Wei Sheng Zhi Ye Bing Za Zhi. (2016) 34:13–7.
35. Yang S, Cao C, Chen S, Hu L, Bao W, Shi H, et al. Serum metabolomics analysis of quercetin against acrylamide-induced toxicity in rats. J Agric Food Chem. (2016) 64:9237–45. doi: 10.1021/acs.jafc.6b04149
36. Bao W, Cao C, Li S, Bo L, Zhang M, Zhao X, et al. Metabonomic analysis of quercetin against the toxicity of acrylamide in rat urine. Food Funct. (2017) 8:1204–14. doi: 10.1039/C6FO01553K
37. Uthra C, Shrivastava S, Jaswal A, Sinha N, Reshi MS, Shukla S. Therapeutic potential of quercetin against acrylamide induced toxicity in rats. Biomed Pharmacother. (2017) 86:705–14. doi: 10.1016/j.biopha.2016.12.065
38. Foroutanfar A, Mehri S, Kamyar M, Tandisehpanah Z, Hosseinzadeh H. Protective effect of punicalagin, the main polyphenol compound of pomegranate, against acrylamide-induced neurotoxicity and hepatotoxicity in rats. Phytother Res. (2020) 34:3262–72. doi: 10.1002/ptr.6774
39. Rahangadale S, Jangir BL, Patil M, Verma T, Bhandarkar A, Sonkusale P, et al. Evaluation of protective effect of vitamin e on acrylamide induced testicular toxicity in wister rats. Toxicol Int. (2012) 19:158–61. doi: 10.4103/0971-6580.97216
40. Sumizawa T, Igisu H. Suppression of acrylamide toxicity by carboxyfullerene in human neuroblastoma cells in vitro. Arch Toxicol. (2009) 83:817–24. doi: 10.1007/s00204-009-0438-7
41. Sun J, Li M, Zou F, Bai S, Jiang X, Tian L, et al. Protection of cyanidin-3-O-glucoside against acrylamide- and glycidamide-induced reproductive toxicity in leydig cells. Food Chem Toxicol. (2018) 119:268–74. doi: 10.1016/j.fct.2018.03.027
42. Tabeshpour J, Mehri S, Abnous K, Hosseinzadeh H. Neuroprotective effects of thymoquinone in acrylamide-induced peripheral nervous system toxicity through MAPKinase and apoptosis pathways in rat. Neurochem Res. (2019) 44:1101–12. doi: 10.1007/s11064-019-02741-4
43. Abdel-Daim MM, Abd Eldaim MA, Hassan AG. Trigonella foenum-graecum ameliorates acrylamide-induced toxicity in rats: roles of oxidative stress, proinflammatory cytokines, and DNA damage. Biochem Cell Biol. (2015) 93:192–8. doi: 10.1139/bcb-2014-0122
44. Bin-Jumah MN, Al-Huqail AA, Abdelnaeim N, Kamel M, Fouda MMA, Abulmeaty MMA, et al. Potential protective effects of Spirulina platensis on liver, kidney, and brain acrylamide toxicity in rats. Environ Sci Pollut Res Int. (2021) 28:26653–63. doi: 10.1007/s11356-021-12422-x
45. Chu Q, Chen W, Jia R, Ye X, Li Y, Liu Y, et al. Tetrastigma hemsleyanum leaves extract against acrylamide-induced toxicity in HepG2 cells and Caenorhabditis elegans. J Hazard Mater. (2020) 393:122364. doi: 10.1016/j.jhazmat.2020.122364
46. Farag OM, Abd-Elsalam RM, Ogaly HA, Ali SE, El Badawy SA, Alsherbiny MA, et al. Metabolomic profiling and neuroprotective effects of purslane seeds extract against acrylamide toxicity in rat's brain. Neurochem Res. (2021) 46:819–42. doi: 10.1007/s11064-020-03209-6
47. Hamdy SM, El-Khayat Z, Farrag AR, Sayed ON, El-Sayed MM, Massoud D. Hepatoprotective effect of Raspberry ketone and white tea against acrylamide-induced toxicity in rats. Drug Chem Toxicol. (2020) 1–9. doi: 10.1080/01480545.2020.1772279
48. Hamdy SM, Shabaan AM, Abdel Latif AKM, Abdel-Aziz AM, Amin AM. Protective effect of Hesperidin and Tiger nut against Acrylamide toxicity in female rats. Exp Toxicol Pathol. (2017) 69:580–8. doi: 10.1016/j.etp.2017.05.004
49. Rodriguez-Ramiro I, Martin MA, Ramos S, Bravo L, Goya L. Olive oil hydroxytyrosol reduces toxicity evoked by acrylamide in human Caco-2 cells by preventing oxidative stress. Toxicology. (2011) 288:43–8. doi: 10.1016/j.tox.2011.07.002
50. Xie Q, Liu Y, Sun H, Liu Y, Ding X, Fu D, et al. Inhibition of acrylamide toxicity in mice by three dietary constituents. J Agric Food Chem. (2008) 56:6054–60. doi: 10.1021/jf0730542
51. Zhao M, Liu X, Luo Y, Guo H, Hu X, Chen F. Evaluation of protective effect of freeze-dried strawberry, grape, and blueberry powder on acrylamide toxicity in mice. J Food Sci. (2015) 80:H869–874. doi: 10.1111/1750-3841.12815
52. Arbabi M, Badi HN, Labbafi M, Mehrafarin A, Saboki E. Morphophysiological and phytochemical variability in some wild populations of Ducrosia anethifolia from Iran. Chem Biodivers. (2018) 15:e1800301. doi: 10.1002/cbdv.201800301
53. Joshi M, Schmilovitch Z, Ginzberg I. Pomegranate fruit growth and skin characteristics in hot and dry climate. Front Plant Sci. (2021) 12:725479. doi: 10.3389/fpls.2021.725479
54. Chandra HM, Shanmugaraj BM, Srinivasan B, Ramalingam S. Influence of genotypic variations on antioxidant properties in different fractions of tomato. J Food Sci. (2012) 77:C1174–8. doi: 10.1111/j.1750-3841.2012.02962.x
55. Sandeep IS, Sanghamitra N, Sujata M. Differential effect of soil and environment on metabolic expression of turmeric (Curcuma longa cv. Roma). Indian J Exp Biol. (2015) 53:406–11.
56. Rebeiro KL. Occupational therapy and the multidisciplinary team. Psychiatr Serv. (2002) 53:767. doi: 10.1176/appi.ps.53.6.767
57. Kirsch A. Successful treatment of metastatic malignant melanoma with Viscum album extract (Iscador M). J Altern Complement Med. (2007) 13:443–5. doi: 10.1089/acm.2007.6175
58. Fischer UA, Carle R, Kammerer DR. Identification and quantification of phenolic compounds from pomegranate (Punica granatum L.) peel. mesocarp, aril and differently produced juices by HPLC-DAD-ESI/MS(n). Food Chem. (2011) 127:807–21. doi: 10.1016/j.foodchem.2010.12.156
59. Galani JHY, Patel JS, Patel NJ, Talati JG. Storage of fruits and vegetables in refrigerator increases their phenolic acids but decreases the total phenolics, anthocyanins and vitamin C with subsequent loss of their antioxidant capacity. Antioxidants. (2017) 6:59. doi: 10.3390/antiox6030059
60. Zargoosh Z, Ghavam M, Bacchetta G, Tavili A. Effects of ecological factors on the antioxidant potential and total phenol content of Scrophularia striata Boiss. Sci Rep. (2019) 9:16021. doi: 10.1038/s41598-019-52605-8
61. Liu W, Yin D, Li N, Hou X, Wang D, Li D, et al. Influence of environmental factors on the active substance production and antioxidant activity in Potentilla fruticosa L. and its quality assessment. Sci Rep. (2016) 6:28591. doi: 10.1038/srep28591
62. Pandey G, Khatoon S, Pandey MM, Rawat AKS. Altitudinal variation of berberine, total phenolics and flavonoid content in Thalictrum foliolosum and their correlation with antimicrobial and antioxidant activities. J Ayurveda Integr Med. (2018) 9:169–76. doi: 10.1016/j.jaim.2017.02.010
63. Dong X, Guo Y, Xiong C, Sun L. Evaluation of two major rhodiola species and the systemic changing characteristics of metabolites of Rhodiola crenulata in different altitudes by chemical methods combined with UPLC-QqQ-MS-based metabolomics. Molecules. (2020) 25:4062. doi: 10.3390/molecules25184062
64. Fernandes L, Pereira JA, Lopez-Cortes I, Salazar DM, Ramalhosa EC. physicochemical changes and antioxidant activity of juice, skin, pellicle and seed of pomegranate (cv. Mollar de Elche) at different stages of ripening. Food Technol Biotechnol. (2015) 53:397–406. doi: 10.17113/ftb.53.04.15.3884
65. Cano-Lamadrid M, Marhuenda-Egea FC, Hernandez F, Rosas-Burgos EC, Burgos-Hernandez A, Carbonell-Barrachina AA. biological activity of conventional and organic pomegranate juices: antioxidant and antimutagenic potential. Plant Foods Hum Nutr. (2016) 71:375–80. doi: 10.1007/s11130-016-0569-y
66. Alkathiri B, El-Khadragy MF, Metwally DM, Al-Olayan EM, Bakhrebah MA, Abdel Moneim AE. Pomegranate (Punica granatum) juice shows antioxidant activity against cutaneous leishmaniasis-induced oxidative stress in female BALB/c mice. Int J Environ Res Public Health. (2017) 14:1592. doi: 10.3390/ijerph14121592
67. Amri Z, Ghorbel A, Turki M, Akrout FM, Ayadi F, Elfeki A, et al. Effect of pomegranate extracts on brain antioxidant markers and cholinesterase activity in high fat-high fructose diet induced obesity in rat model. BMC Complement Altern Med. (2017) 17:339. doi: 10.1186/s12906-017-1842-9
68. Derakhshan Z, Ferrante M, Tadi M, Ansari F, Heydari A, Hosseini MS, et al. Antioxidant activity and total phenolic content of ethanolic extract of pomegranate peels, juice and seeds. Food Chem Toxicol. (2018) 114:108–11. doi: 10.1016/j.fct.2018.02.023
69. Dzugan M, Wesolowska M, Zagula G, Puchalski C. The comparison of the physicochemical parameters and antioxidant activity of homemade and commercial pomegranate juices. Acta Sci Pol Technol Aliment. (2018) 17:59–68. doi: 10.17306/J.A.F.S.2018.0529
70. Russo M, Fanali C, Tripodo G, Dugo P, Muleo R, Dugo L, et al. Analysis of phenolic compounds in different parts of pomegranate (Punica granatum) fruit by HPLC-PDA-ESI/MS and evaluation of their antioxidant activity: application to different Italian varieties. Anal Bioanal Chem. (2018) 410:3507–20. doi: 10.1007/s00216-018-0854-8
71. Di Stefano V, Pitonzo R, Novara ME, Bongiorno D, Indelicato S, Gentile C, et al. Antioxidant activity and phenolic composition in pomegranate (Punica granatum L.) genotypes from south Italy by UHPLC-Orbitrap-MS approach. J Sci Food Agric. (2019) 99:1038–45. doi: 10.1002/jsfa.9270
72. Pontonio E, Montemurro M, Pinto D, Marzani B, Trani A, Ferrara G, et al. Lactic acid fermentation of pomegranate juice as a tool to improve antioxidant activity. Front Microbiol. (2019) 10:1550. doi: 10.3389/fmicb.2019.01550
73. Benchagra L, Berrougui H, Islam MO, Ramchoun M, Boulbaroud S, Hajjaji A, et al. Antioxidant effect of moroccan pomegranate (Punica granatum L. sefri variety) extracts rich in punicalagin against the oxidative stress process. Foods. (2021) 10:2219. doi: 10.3390/foods10092219
74. Esposto S, Veneziani G, Taticchi A, Urbani S, Selvaggini R, Sordini B, et al. Chemical composition, antioxidant activity, and sensory characterization of commercial pomegranate juices. Antioxidant. (2021) 10:1381. doi: 10.3390/antiox10091381
75. Tarantino A, Difonzo G, Disciglio G, Frabboni L, Paradiso VM, Gambacorta G, et al. Fresh pomegranate juices from cultivars and local ecotypes grown in southeastern Italy: comparison of physicochemical properties, antioxidant activity and bioactive compounds. J Sci Food Agric. (2021) 102:1185–92. doi: 10.1002/jsfa.11456
76. Salles TS, Meneses MDF, Caldas LA, Sa-Guimaraes TE, de Oliveira DM, Ventura JA, et al. Virucidal and antiviral activities of pomegranate (Punica granatum) extract against the mosquito-borne Mayaro virus. Parasit Vectors. (2021) 14:443. doi: 10.1186/s13071-021-04955-4
77. Song H, Shen X, Chu Q, Zheng X. Pomegranate fruit pulp polyphenols reduce diet-induced obesity with modulation of gut microbiota in mice. J Sci Food Agric. (2021). doi: 10.1002/jsfa.11535
78. Aboelsoued D, Abo-Aziza FAM, Mahmoud MH, Abdel Megeed KN, Abu El Ezz NMT, Abu-Salem FM. Anticryptosporidial effect of pomegranate peels water extract in experimentally infected mice with special reference to some biochemical parameters and antioxidant activity. J Parasit Dis. (2019) 43:215–28. doi: 10.1007/s12639-018-01078-z
79. Kujawska M, Jourdes M, Witucki L, Karazniewicz-Lada M, Szulc M, Gorska A, et al. Pomegranate juice ameliorates dopamine release and behavioral deficits in a rat model of Parkinson's disease. Brain Sci. (2021) 11:1127. doi: 10.3390/brainsci11091127
80. Turgut SS, Isikci F, Soyer A. Antioxidant activity of pomegranate peel extract on lipid and protein oxidation in beef meatballs during frozen storage. Meat Sci. (2017) 129:111–9. doi: 10.1016/j.meatsci.2017.02.019
81. Zhai X, Zhu C, Li Y, Zhang Y, Duan Z, Yang X. Optimization for pectinase-assisted extraction of polysaccharides from pomegranate peel with chemical composition and antioxidant activity. Int J Biol Macromol. (2018) 109:244–53. doi: 10.1016/j.ijbiomac.2017.12.064
82. Jacob B, Malli Sureshbabu N, Ranjan M, Ranganath A, Siddique R. The antimicrobial effect of pomegranate peel extract versus chlorhexidine in high caries risk individuals using quantitative real-time polymerase chain reaction: a randomized triple-blind controlled clinical trial. Int J Dent. (2021) 2021:5563945. doi: 10.1155/2021/5563945
83. Kaseke T, Opara UL, Fawole OA. Effect of blanching pomegranate seeds on physicochemical attributes, bioactive compounds and antioxidant activity of extracted oil. Molecules. (2020) 25:2554. doi: 10.3390/molecules25112554
84. Kaseke T, Opara UL, Fawole OA. Effects of enzymatic pretreatment of seeds on the physicochemical properties, bioactive compounds, and antioxidant activity of pomegranate seed oil. Molecules. (2021) 26:46575. doi: 10.3390/molecules26154575
85. Al-Sodany YM, Bazaid SA, Mosallam HA. Medicinal plants in Saudi Arabia: I. Sarrwat mountains at Taif, KSA academic. J Plant Sci. (2013) 6:134–45. doi: 10.5829/idosi.ajps.2013.6.4.1115
86. Dessoky ES, Alqurashi M, Alotaibi SS, Sadik AS. DNA fingerprinting of in vitro micropropagated pomegranate genotypes. Pak J Biol Sci. (2020) 23:619–27. doi: 10.3923/pjbs.2020.619.627
87. Elhelaly AE, AlBasher G, Alfarraj S, Almeer R, Bahbah EI, Fouda MMA, et al. Protective effects of hesperidin and diosmin against acrylamide-induced liver, kidney, and brain oxidative damage in rats. Environ Sci Pollut Res Int. (2019) 26:35151–62. doi: 10.1007/s11356-019-06660-3
88. Abdel-Daim MM, Abo El-Ela FI, Alshahrani FK, Bin-Jumah M, Al-Zharani M, Almutairi B, et al. Protective effects of thymoquinone against acrylamide-induced liver, kidney and brain oxidative damage in rats. Environ Sci Pollut Res Int. (2020) 27:37709–17. doi: 10.1007/s11356-020-09516-3
89. Jiang G, Lei A, Chen Y, Yu Q, Xie J, Yang Y, et al. The protective effects of the Ganoderma atrum polysaccharide against acrylamide-induced inflammation and oxidative damage in rats. Food Funct. (2021) 12:397–407. doi: 10.1039/D0FO01873B
90. Pirinççioglu M, Kizil G, Kizil M, Kanay Z, Ketani A. The protective role of pomegranate juice against carbon tetrachloride-induced oxidative stress in rats. Toxicol Ind Health. (2014) 30:910–8. doi: 10.1177/0748233712464809
91. Ohkawa H, Ohishi N, Yagi K. Assay for lipid peroxides in animal tissues by thiobarbituric acid reaction. Anal Biochem. (1979) 95:351–8. doi: 10.1016/0003-2697(79)90738-3
92. Beutler E, Duron O, Kelly BM. Improved method for the determination of blood glutathione. J Lab Clin Med. (1963) 61:882–8.
93. Nishikimi M, Appaji N, Yagi K. The occurrence of superoxide anion in the reaction of reduced phenazine methosulfate and molecular oxygen. Biochem Biophys Res Commun. (1972) 46:849–54. doi: 10.1016/S0006-291X(72)80218-3
94. Young GA, Keogh JB, Parsons FM. Plasma amino acids and protein levels in chronic renal failure and changes caused by oral supplements of essential amino acids. Clin Chim Acta. (1975) 61:205–13. doi: 10.1016/0009-8981(75)90316-2
95. Lowry OH, Rosebrough NJ, Farr AL, Randall RJ. Protein measurement with the Folin phenol reagent. J Biol Chem. (1951) 193:265–75. doi: 10.1016/S0021-9258(19)52451-6
96. Tietze F. Enzymic method for quantitative determination of nanogram amounts of total and oxidized glutathione: applications to mammalian blood and other tissues. Anal Biochem. (1969) 27:502–22. doi: 10.1016/0003-2697(69)90064-5
97. Bancroft D, Stevens A, Turner R. Theory and Practice of Histological Techniques. Edinburgh; London; Melbourne: Churchill Livingstone (1996).
98. Saber S, Khalil R, Abdo W, Nassif D, El-Ahwany E. Olmesartan ameliorates chemically-induced ulcerative colitis in rats via modulating NFjB and Nrf-2/HO-1 signaling crosstalk. Toxicol Appl Pharmacol. (2019) 364:120–32.
99. Nasri H, Baradaran A, Shirzad H, Rafieian-Kopaei M. New concepts in nutraceuticals as alternative for pharmaceuticals. Int J Prev Med. (2014) 5:1487–99.
100. Serafini M, Peluso I. Functional foods for health: the interrelated antioxidant and anti-inflammatory role of fruits, vegetables, herbs, spices and cocoa in humans. Curr Pharm Des. (2016) 22:6701–15. doi: 10.2174/1381612823666161123094235
101. Les F, Prieto JM, Arbonés-Mainar JM, Valero MS, López V. Bioactive properties of commercialised pomegranate (Punica granatum) juice: antioxidant, antiproliferative and enzyme inhibiting activities. Food Funct. (2015) 6:2049–57. doi: 10.1039/C5FO00426H
102. Noori M, Jafari B, Hekmatdoost A. Pomegranate juice prevents development of non-alcoholic fatty liver disease in rats by attenuating oxidative stress and inflammation. J Sci Food Agric. (2017) 97:2327–32. doi: 10.1002/jsfa.8042
103. Ekhlasi G, Shidfar F, Agah S, Merat S, Hosseini AF. Effects of pomegranate and orange juice on antioxidant status in non-alcoholic fatty liver disease patients: a randomized clinical trial. Int J Vitam Nutr Res. (2015) 85:292–8. doi: 10.1024/0300-9831/a000292
104. Lim TG, Lee BK, Kwon JY, Jung SK, Lee KW. Acrylamide up-regulates cyclooxygenase-2 expression through the MEK/ERK signaling pathway in mouse epidermal cells. Food Chem Toxicol. (2011) 49:1249–54. doi: 10.1016/j.fct.2011.03.003
105. Bouasla A, Bouasla I, Boumendjel A, Abdennour C, El Feki A, Messarah M. Prophylactic effects of pomegranate (Punica granatum) juice on sodium fluoride induced oxidative damage in liver and erythrocytes of rats. Can J Physiol Pharmacol. (2016) 94:709–18. doi: 10.1139/cjpp-2015-0226
106. Annac E, Uckun M, Ozkaya A, Yologlu E, Pekmez H, Bulmus O, et al. The protective effects of pomegranate juice on lead acetate-induced neurotoxicity in the male rat: a histomorphometric and biochemical study. J Food Biochem. (2021) e13881. doi: 10.1111/jfbc.13881
107. Abu-Taweel GM, Al-Mutary MG. Pomegranate juice moderates anxiety- and depression-like behaviors in AlCl3-treated male mice. J Trace Elem Med Biol. (2021) 68:126842. doi: 10.1016/j.jtemb.2021.126842
108. Husain H, Latief U, Ahmad R. Pomegranate action in curbing the incidence of liver injury triggered by Diethylnitrosamine by declining oxidative stress via Nrf2 and NFkappaB regulation. Sci Rep. (2018) 8:8606. doi: 10.1038/s41598-018-26611-1
109. Aksu DS, Saglam YS, Yildirim S, Aksu T. Effect of pomegranate (Punica granatum L.) juice on kidney, liver, heart and testis histopathological changes, and the tissues lipid peroxidation and antioxidant status in lead acetate-treated rats. Cell Mol Biol. (2017) 63:33–42. doi: 10.14715/cmb/2017.63.10.5
110. Shaban NZ, El-Kersh MA, Bader-Eldin MM, Kato SA, Hamoda AF. Effect of Punica granatum (pomegranate) juice extract on healthy liver and hepatotoxicity induced by diethylnitrosamine and phenobarbital in male rats. J Med Food. (2014) 17:339–49. doi: 10.1089/jmf.2012.0306
111. Faria A, Monteiro R, Mateus N, Azevedo I, Calhau C. Effect of pomegranate (Punica granatum) juice intake on hepatic oxidative stress. Eur J Nutr. (2007) 46:271–8. doi: 10.1007/s00394-007-0661-z
112. Gaurava, Praveenvats, Shyam R, Suri S, Kumria M, Sridharan K, et al. Effect of pomegranate (Punica granatum L.) juice on changes in tissue glutathione levels of rats exposed to high altitude hypoxia. Anc Sci Life. (2001) 21:75–86.
113. Clementi ME, Sampaolese B, Sciandra F, Tringali G. Punicalagin protects human retinal pigment epithelium cells from ultraviolet radiation-induced oxidative damage by activating Nrf2/HO-1 signaling pathway and reducing apoptosis. Antioxidants. (2020) 9:473. doi: 10.3390/antiox9060473
114. Hu R, Saw CL, Yu R, Kong AN. Regulation of NF-E2-related factor 2 signaling for cancer chemoprevention: antioxidant coupled with antiinflammatory. Antioxid Redox Signal. (2010) 13:1679–98. doi: 10.1089/ars.2010.3276
115. Peluso I, Raguzzini A, Villano DV, Cesqui E, Toti E, Catasta G, et al. High fat meal increase of IL-17 is prevented by ingestion of fruit juice drink in healthy overweight subjects. Curr Pharm Des. (2012) 18:85–90. doi: 10.2174/138161212798919020
116. Idriss HT, Naismith JH. TNF alpha and the TNF receptor superfamily: structure-function relationship(s). Microsc Res Tech. (2000) 50:184–95. doi: 10.1002/1097-0029(20000801)50:3<184::AID-JEMT2>3.0.CO;2-H
117. Hassanen EI, Ibrahim MA, Hassan AM, Mehanna S, Aljuaydi SH, Issa MY. Neuropathological and cognitive effects induced by CuO-NPs in rats and trials for prevention using pomegranate juice. Neurochem Res. (2021) 46:1264–79. doi: 10.1007/s11064-021-03264-7
118. Galal MK, Elleithy EMM, Abdrabou MI, Yasin NAE, Shaheen YM. Modulation of caspase-3 gene expression and protective effects of garlic and spirulina against CNS neurotoxicity induced by lead exposure in male rats. Neurotoxicology. (2019) 72:15–28. doi: 10.1016/j.neuro.2019.01.006
119. Hassan NF, Soliman GM, Okasha EF, Shalaby AM. Histological, immunohistochemical, and biochemical study of experimentally induced fatty liver in adult male albino rat and the possible protective role of pomegranate. J Microsc Ultrastruct. (2018) 6:44–55. doi: 10.4103/JMAU.JMAU_5_18
Keywords: acrylamide (Acr), pomegranate juice, antioxidant, liver dysfunction, oxidative stress
Citation: El-Shehawi AM, Sayed S, Hassan MM, Al-Otaibi S, Althobaiti F, Elseehy MM and Soliman M (2022) Taify Pomegranate Juice (TPJ) Abrogates Acrylamide-Induced Oxidative Stress Through the Regulation of Antioxidant Activity, Inflammation, and Apoptosis-Associated Genes. Front. Vet. Sci. 9:833605. doi: 10.3389/fvets.2022.833605
Received: 11 December 2021; Accepted: 07 February 2022;
Published: 22 March 2022.
Edited by:
Mohamed Fathi Abdallah, Ghent University, BelgiumReviewed by:
Seema Rai, Guru Ghasidas Vishwavidyalaya, IndiaOla Habotta, Mansoura University, Egypt
Copyright © 2022 El-Shehawi, Sayed, Hassan, Al-Otaibi, Althobaiti, Elseehy and Soliman. This is an open-access article distributed under the terms of the Creative Commons Attribution License (CC BY). The use, distribution or reproduction in other forums is permitted, provided the original author(s) and the copyright owner(s) are credited and that the original publication in this journal is cited, in accordance with accepted academic practice. No use, distribution or reproduction is permitted which does not comply with these terms.
*Correspondence: Ahmed M. El-Shehawi, YS5lbHNoZWhhd2lAdHUuZWR1LnNh
†These authors have contributed equally to this work