- Guangzhou Higher Education Mega Center, State Key Laboratory of Biocontrol, School of Life Sciences, Sun Yat-sen University, Guangzhou, China
African swine fever (ASF) is a highly contagious disease and provokes severe economic losses and health threats. At present no effective vaccine or treatment is available to prevent or cure ASF. Consequently, there is an urgent need to develop effective drugs against ASF virus (ASFV). Chlorine dioxide (ClO2), an ideal biocide, has broad-spectrum antibacterial activity and no drug resistance. Here, we found that ClO2 strongly inhibited ASFV replication in porcine alveolar macrophages (PAMs). The inhibitory effect of ClO2 occurred during viral attachment rather than entry, indicating that ClO2 suppressed the early stage of virus life cycle. ClO2 showed a potent anti-ASFV effect when added either before, simultaneously with, or after virus infection. Furthermore, ClO2 could destroy viral nucleic acids and proteins, which may contribute to its capacity of inactivating ASFV virions. The minimum concentration of degradation of ASFV nucleic acids by ClO2 is 1.2 μg/mL, and the degradation is a temperature-dependent manner. These have guiding significance for ClO2 prevention and control of ASFV infection in pig farms. In addition, ClO2 decreased the expression of ASFV-induced inflammatory cytokines. Overall, our findings suggest that ClO2 may be an ideal candidate for the development of novel anti-ASFV prophylactic and therapeutic drugs in swine industry.
Introduction
African swine fever (ASF) can be considered as one of the most feared epidemic diseases of the pig industry worldwide. ASF is extremely dangerous due to its highly contagious characteristics, high morbidity and mortality rates, extreme resilience to endure high and low temperatures, and ability to be easily spread via a variety of vectors (1). The disease was first described in Kenya in the 1920s and then Outbreaks have been reported periodically outside Africa. In 2007, ASF was introduced into the Caucasus region of Eurasia via the Republic of Georgia. Subsequently, it spread throughout the Caucasus into the Russian Federation, Ukraine, and Belarus (2, 3). In 2018, an outbreak of ASF in pigs was reported in China and then occurred in other Asian countries and regions, causing huge economic losses to the global swine industry (4, 5).
African swine fever virus (ASFV), the etiological agent of ASF, is the sole member of its genus Asfivirus and family Asfarviridae (Asfar, African swine fever and related viruses) and the only known DNA arbovirus (6). The genome of the virus is a linear double-stranded DNA molecule containing around 200 open reading frames and the size of which is about 170–190 kilo base pair depending on the virus strain (7, 8). The virus has a highly genetic and antigenic diversity. To date, there are 24 genotypes based on the viral p72 protein (B646L gene), while at least 8 serotypes have been identified by hemadsorption inhibition assay (9). ASFV isolates vary in virulence thus the pathogenetic process of infected animals may be peracute, acute, subacute or chronic (10–12). ASFV is mainly thought to enter the animal body via the upper respiratory tract and can persist in swill and uncooked meat products for several months, thus might be transmitted and spread through the contaminated swine feed or meat products ingested (13). Due to the lack of detailed knowledge concerning the virulence of the virus, viral pathogenesis and immune response to virus infection, and the available cell lines supporting the ASFV replication, there is currently no effective vaccine available to prevent the transmission and spread of ASFV (14, 15). With that in mind, new virus control strategies such as antivirals are urgently needed.
Chlorine dioxide (ClO2), a strong oxidant, has a wide application prospect in different fields such as food and environment disinfection, medicine, as well as wastewater or water treatment (16). Most of the disinfectants such as peracetic acid and hydrogen peroxide used nowadays are toxic even at low concentrations (16). However, ClO2 has very low toxicity to the animal organism even at adequate antiviral and antibacterial activities (17). Therefore, it is widely used to sterilize the drinking water (18). Due to the property of its chlorination, ClO2 can inhibit or destroy microbes such as inactivating bacteria, virus, fungi, parasites and other cellular pathogens (19, 20). We previously demonstrated that ClO2 displays potent antiviral and virucidal activities to porcine reproductive and respiratory syndrome virus infection in both Marc-145 cells and porcine alveolar macrophages (PAMs) (21). Previous studies also showed that ClO2 is capable of killing feline calicivirus, human herpesvirus, human influenza virus, measles virus, canine parvovirus and human adenovirus (20, 22). However, whether ClO2 inhibits ASFV replication remains unknown.
Here, we investigate the anti-ASFV activity of ClO2 in vitro and address the molecular mechanism of its inhibitory effect on ASFV. We found that ClO2 has a great clinical application prospect in the prevention and treatment of ASFV infection in pig industry.
Materials and Methods
Cells, Viruses and Compounds
PAMs were isolated from the lungs of 3–8-week-old ASFV-negative piglets (Guangxi State Farms, China) by lung lavage. PAMs were cultured in RPMI-1640 supplemented with 100 U/ml penicillin, 100 μg/ml streptomycin sulfate, and 10% heat-inactivated fetal bovine serum (FBS; PAA, Pasching, Austria). Animals were euthanized and carcasses were treated innocuously. All animal experiments were approved by the Institutional Animal Care and Use Committee of Sun Yat-sen University. ASFV isolate GD19 was propagated and titrated in PAMs and was used throughout the study. ClO2 was provided by Guangzhou WellFar Co, Ltd., Guangzhou, China.
Cytotoxicity Assay
The cytotoxicity of ClO2 was tested using the alamarBlue® assay (Invitrogen, USA) according to the manufacturer's instructions. Briefly, PAMs at the density of 1 × 105 per well were seeded in 96-well plates respectively, ClO2 at different concentrations were added. Mock-treated cells were set up simultaneously. After incubation for 48 h, 10 μl of alamarBlue® was added to each well and the cultures were incubated for another 3 h. Fluorescence intensity was measured at 570 nm excitation and 590 nm emission wavelengths using a microplate reader (Synergy2, BioTek, USA) and normalized to the control for each sample.
Quantitative Reverse-Transcription Polymerase Chain Reaction (qRT-PCR)
Total RNA was extracted from PAMs using TRIzol reagent (Magen, China) according to the manufacturer's instructions. Briefly, Reverse Transcription System (A3500, Promega, USA) was used for reverse transcription in 20 μL reaction volume. The reverse-transcription primers were Oligo (dT) 15 primer (C110A, Promega, USA) and Random primer (C118A, Promega, USA). SYBR Green (TaKaRa, Osaka, Japan) real-time PCR was performed using a Light-Cycler 480 PCR system (Roche, Basel, Switzerland). Relative quantities of mRNA accumulation were evaluated using the 2−ΔCt method. The primers used for qRT-PCR are listed in Table 1.
Immunofluorescence Assay (IFA)
PAMs were fixed with 4% paraformaldehyde at room temperature (RT) for 15 min and then permeabilized with 0.3% Triton X-100 at RT. After washed three times with PBS, PAMs were blocked with 1% Bovine serum albumin (BSA) in PBS for 30 min at RT, then incubated overnight with an anti-ASFV p30 protein mAb (diluted 1: 200 in PBS; MEDIAN, Republic of Korea) at 4°C and followed by Alexa Fluor® 488-conjugated anti-mouse IgG secondary antibody for 2 h. The nuclei were stained with Hoechst dye 33342 (Sigma-Aldrich, St. Louis, MO, USA). Finally PAMs were examined using fluorescence microscopy (Carl Zeiss, Jena, Germany).
Western Blot
ASFV-infected or mock-infected PAMs treated with or without ClO2 were lysed in RIPA lysis buffer (Beyotime, China), and then electrophoresed onto a 12% SDS-PAGE gel and transferred to a polyvinylidene-fluoride (PVDF) membranes (Roche, USA). The membranes were blocked and incubated with a monoclonal antibody against ASFV p72 protein or GAPDH (Cell Signaling Technology, USA). After washing, membranes were incubated with anti-mouse or anti-rabbit IgG, HRP-conjugated antibody (Cell Signaling Technology, USA). Signal detection was performed using a chemiluminescence reagent (Fdbio Science, China).
Antiviral Assay
The inhibitory effect of treatment with ClO2 on ASFV was analyzed with three different approaches. (I) Pre-treatment: PAMs were pre-treated with ClO2 at a concentration of 0.2 μg/mL for 4 h, ASFV at a multiplicity of infection (MOI) of 1 was then added and cells were cultured for another 36 h. (II) Co-treatment: Cells were inoculated with ASFV (MOI = 1) in the presence or absence of ClO2 (0.2 μg/mL) for 36 h. (III) Post-treatment: PAMs were infected with ASFV at an MOI of 1 for 4 h at 37°C, and then the viral inoculum was removed and fresh RPMI-1640 medium containing ClO2 (0.2 μg/mL) was added and cells were further incubated for 36 h. In all above antiviral assays, the cells were collected for the IFA and qRT-PCR analysis.
Virus Adsorption Assay
PAMs were cooled at 4°C for 30 min, and then infected with ASFV (MOI = 1) in the presence or absence of ClO2 for 3 h at 4°C. After washing three times with ice-cold PBS to remove unbound viral particles, cells were switched to 37°C for a further 4 h. Cells were harvested for IFA and qRT-PCR analysis.
Virus Entry Assay
Cells were initially infected with ASFV (MOI = 1) for 3 h at 4°C. After binding to cell surface, the viral inoculum was removed and then cells were washed with ice-cold PBS three times and incubated for 4 h at 37°C in the presence or absence of ClO2. After washed with PBS three times, cells were harvested to detect ASFV infection by IFA and qRT-PCR.
Virucidal Assay
ASFV was pre-incubated with various concentrations of ClO2 for 2 h at 37°C or pre-incubated with ClO2 at the indicated concentration for 1 or 4 h at 37°C. The mixtures were added to PAMs which were then incubated for 2 h at 37°C. The mixtures were finally removed and cells were cultured with RPMI-1640 medium containing 2% FBS for additional 24 h at 37°C, and harvested for the analysis of IFA and qRT-PCR.
Statistical Analysis
All experiments were performed with at least three independent replicates. Student's t-test and one-way ANOVA were used to analyze the data. All data were presented as means ± standard errors (SE). Statistical analysis was performed using SPSS 17.0 and GraphPad Prism 6.0. Differences with p-values < 0.05 were considered significant.
Results
ClO2 Shows a Potent Antiviral Effect on ASFV Infection
To examine the inhibitory effect of ClO2 on ASFV replication, the half maximal inhibitory concentration (IC50) and half maximal cytotoxic concentration (CC50) values of ClO2 were assessed using a non-linear regression model in GraphPad Prism 9.0. As shown in Figure 1A, ASFV infection was inhibited by ClO2 at an IC50 of 0.08 μg/ml, as determined by analyzing the cell infection rate from IFA images, and the CC50 value of ClO2 measured using the alamarBlue® assay was calculated to be 0.56 μg/ml in PAMs. We next performed IFA and qRT-PCR assays to determine the effect of ClO2 on ASFV, the concentration of ClO2 used in subsequent experiments was non-cytotoxic to PAMs. Upon ClO2 treatment, ASFV p30-specific staining was remarkably decreased in a dose-dependent manner in PAMs compared to that in mock-treated cells following ASFV infection (Figure 1B), suggesting that the infection and the spread of the virions to the neighboring cells are blocked by ClO2. The number of infected cells was notably diminished with ClO2 treatment (Figure 1C). Consistently, the mRNA and protein levels of the viral p72 were significantly reduced by ClO2 treatment compared with the mock-treated cells post ASFV infection, and the inhibition pattern is also in a dose-dependent manner (Figures 1D,E). To further test its antiviral activity on ASFV replication, PAMs were infected with ASFV at different MOIs (at MOIs of 1, 1.5 or 2) in the presence or absence of ClO2 (0.2 μg/mL). As shown in Figure 2, consistent with these findings above, ClO2 significantly suppressed the viral infection and replication in PAMs infected with ASFV at various MOIs.
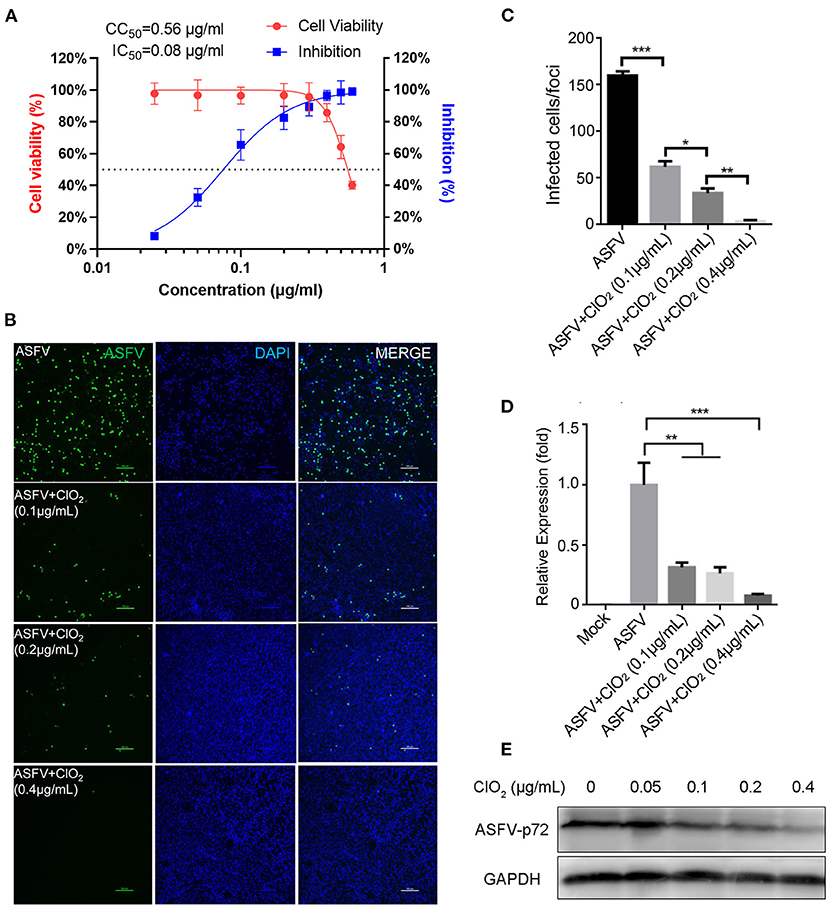
Figure 1. ClO2 restrains ASFV replication in a dose-dependent manner in PAMs. (A) Dose response curves showing the effect of ClO2 on inhibition of ASFV infection (blue) and cytotoxicity (red) in PAMs. The IC50 was determined by analyzing the cell infection rate from IFA images, and the CC50 was evaluated using the CCK-8 assay. The left and right Y-axis represent virus inhibition (%) and cell viability (%) of ClO2, respectively. (B) PAMs were infected with ASFV (MOI = 1) in the presence of various concentrations of ClO2. After incubation for 24 h, cells were harvested for fluorescence microscope examination. Bar, 100 μm. (C) Average number of infected cells per foci in cells treated as B. (D,E) PAMs were mock-infected or infected with ASFV at an MOI of 1, and simultaneously co-treated with different concentrations of ClO2 for 24 h. Cells were then collected for the detection of viral p72 mRNA (D) and protein (E) levels by qRT-PCR and western blot analysis, respectively. Data are representative of the results of three independent experiments (means ± SE). Significant differences compared with control group are denoted by *P < 0.05, **P < 0.01, and ***P < 0.001.
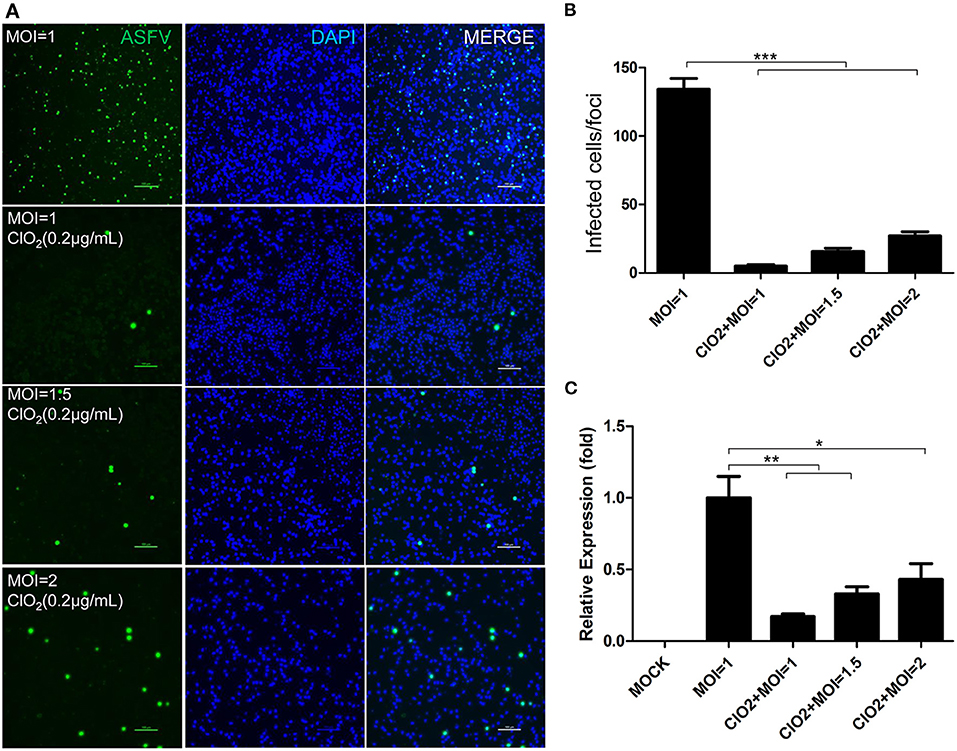
Figure 2. ASFV at different MOIs is inhibited by ClO2 in PAMs. (A) Cells were inoculated with ASFV at different MOIs in the presence or absence of ClO2. Cells were then cultured for 24 h and IFA was performed to evaluate the viral p30 expression. Bar, 100 μm. (B) Average number of infected cells per foci. (C) PAMs were mock-infected or infected with ASFV at various MOIs, and simultaneously co-treated with ClO2 at the indicated concentration. At 24 hpi, cells were measured by qRT-PCR for the determination of the transcription level of viral p72 gene. Data are representative of the results of three independent experiments (means ± SE). Significant differences compared with control group are denoted by *P < 0.05, **P < 0.01, and ***P < 0.001.
Since the order in which the virus and the compound are added to the cells may affect the antiviral effect of the compound, we therefore assessed the effects of ClO2 treatment on ASFV replication using three different approaches as described in the Materials and Methods. As shown in Figure 3, ClO2 displayed a strong antiviral activity both at the protein level of viral p30 and at the transcription and protein levels of viral p72 when administered with either the pre-, co-, or post-treatment approach. Taken together, these data demonstrate that ClO2 shows a powerful antiviral effect on ASFV infection in PAMs in vitro.
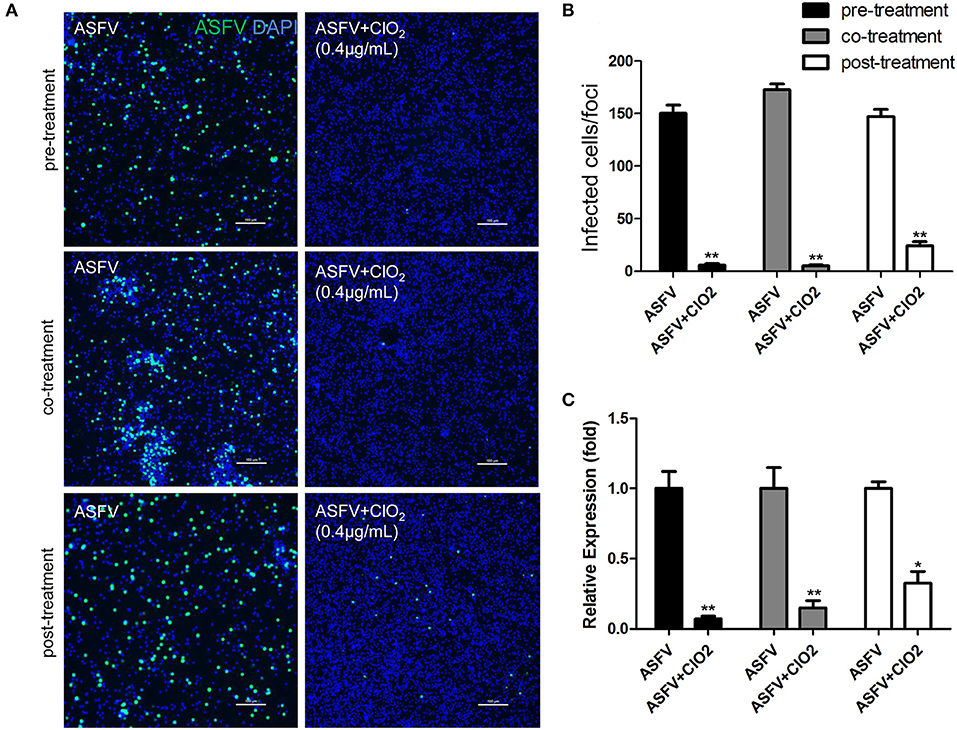
Figure 3. ClO2 shows effective antiviral activity against ASFV regardless of pre-treatment, co-treatment or post-treatment. (A,C) ClO2 treatment was performed either before (pre-treatment), simultaneously with (co-treatment), or after ASFV infection (post-treatment) in PAMs. After cultured for 24 h, cells were fixed for the determination of the expression of virus p30 protein using fluorescence microscope (A) or harvested for the evaluation of the mRNA expression of the viral p72 by qRT-PCR (C). Bar, 100 μm. (B) Average number of infected cells per foci. Data are representative of the results of three independent experiments (means ± SE). Significant differences compared with control group are denoted by *P < 0.05, **P < 0.01, and ***P < 0.001.
ClO2 Shows a Potent Extracellular Virucidal Activity Against ASFV Infection
Previous studies have shown that ClO2 has great ability to inactivate bacteria, virus, fungi, parasites and other cellular pathogens (23–25). To demonstrate that whether ClO2 can kill the ASFV in vitro, a virucidal assay was performed on PAMs. As shown in Figure 4, a lot of fluorescence representing viral burden in visual field was observed following the treatment of ASFV only using a fluorescence microscope. However, the viral fluorescence was dramatically reduced in ASFV-infected cells upon ClO2 treatment. The decline was even more pronounced when the virus was incubated with ClO2 for 4 h at 37°C. Consistently, the mRNA expression of the viral p72 was notably repressed in the presence of ClO2 treatment for 1 or 4 h (Figure 4C). Similar phenomenon was also observed when the virus was incubated with different concentrations of ClO2 for 2 h (Figure 5). Overall, ClO2 can effectively inactivate the ASFV virions in vitro.
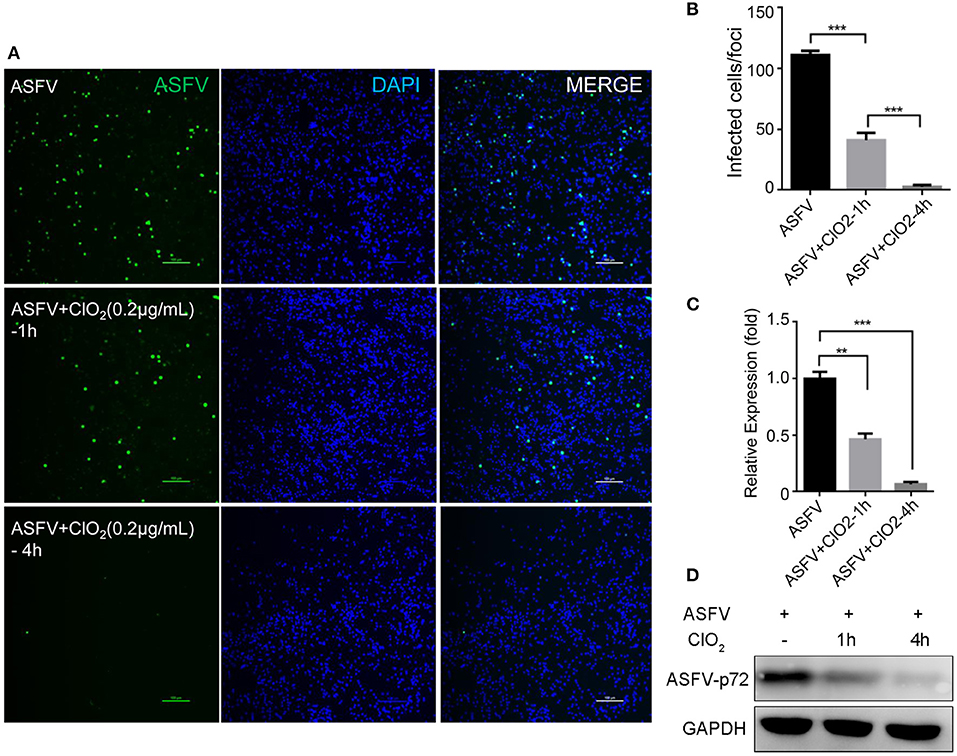
Figure 4. ClO2 displays a strong extracellular virucidal effect on ASFV. (A,C,D) ClO2 at the concentration of 0.2 μg/mL was pre-incubated with ASFV (MOI = 1) for 1 or 4 h at 37°C, and then the mixtures were diluted and added to PAMs for 2 h at 37°C. Finally the mixtures were removed and cells were replenished with fresh RPMI-1640 supplemented with 2% FBS and cultured for additional 24 h. IFA was performed to detect the expression of viral protein using an anti-ASFV p30 mAb (A). The mRNA and protein levels of virus p72 were examined by qRT-PCR (C) and western blot (D) analysis, respectively. Bar, 100 μm. (B) Average number of infected cells per foci. Data are representative of the results of three independent experiments (means ± SE). Significant differences compared with control group are denoted by **P < 0.01 and ***P < 0.001.
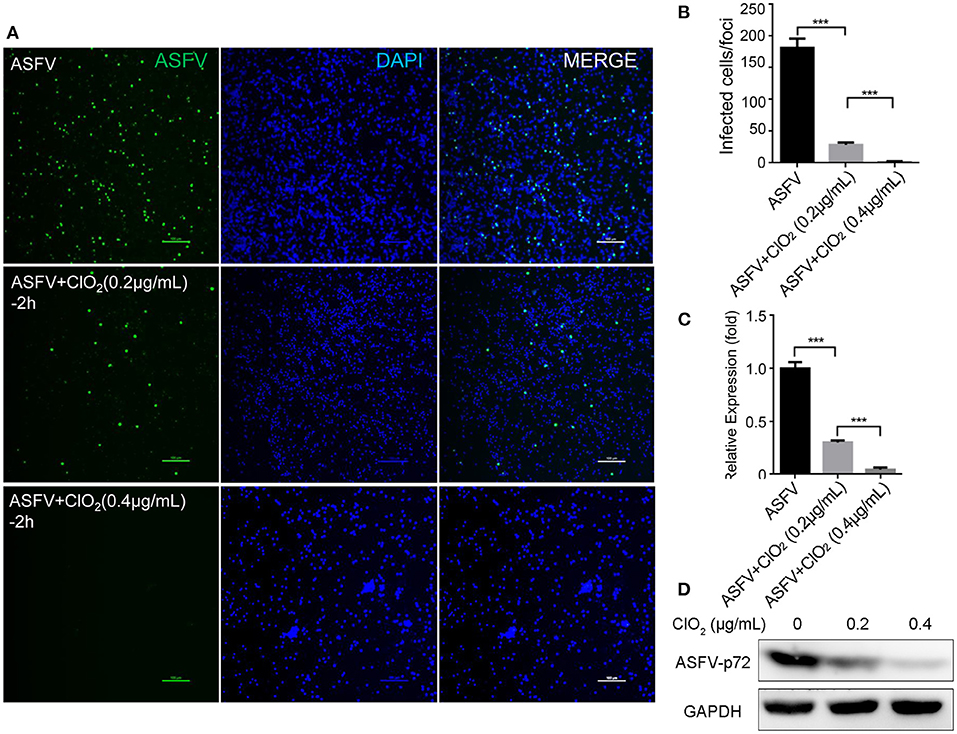
Figure 5. ClO2 inactivates ASFV virions in a dose-dependent manner. (A,C,D) ASFV was pre-incubated with various concentrations of ClO2 for 2 h at 37°C. The mixtures were then added to PAMs. After incubation for 2 h at 37°C, cells were supplied with fresh RPMI-1640 medium containing 2% FBS and cultured for another 24 h at 37°C. Cells were finally harvested for the analysis of IFA (A), qRT-PCR (C) and western blot (D). Bar, 100 μm. (B) Average number of infected cells per foci. Data are representative of the results of three independent experiments (means ± SE). Significant differences compared with control group are denoted by ***P < 0.001.
ClO2 Inhibits Viral Attachment but Not Entry
Viral infection of target cells involves the following processes: adsorption, entry, and uncoating. To characterize the molecular mechanism of anti-ASFV activity of ClO2 and to identify which stage of the viral life cycle is suppressed by ClO2, a viral adsorption assay described in Materials and Methods was first performed to test whether ClO2 is able to inhibit ASFV attachment to PAMs. As shown in Figures 6A,B compared with the mock-treated cells inoculated with ASFV (MOI = 1), the translation level of viral p30 protein and the transcription level of viral p72 were sharply decreased in infected cells in the presence of ClO2 during the period of virus adsorption. The data indicate that ClO2 is capable of diminishing the quantity of infectious virions attached to cell surface. We next investigated whether ClO2 can restrain the entry phase of virus life cycle (the protocol was described in Materials and Methods). The ASFV p30-specific staining which represents the virus yield in virus-infected cells with the treatment of ClO2 was comparable to that of cells following the treatment of ASFV only, during the entry of the virus (Figure 6C). The level of mRNA expression of the viral p72 protein showed a similar phenomenon (Figure 6D). Taken together, these data indicate that ClO2 shows a potent inhibitory effect on the viral adsorption rather than the internalization process of ASFV life cycle in PAMs.
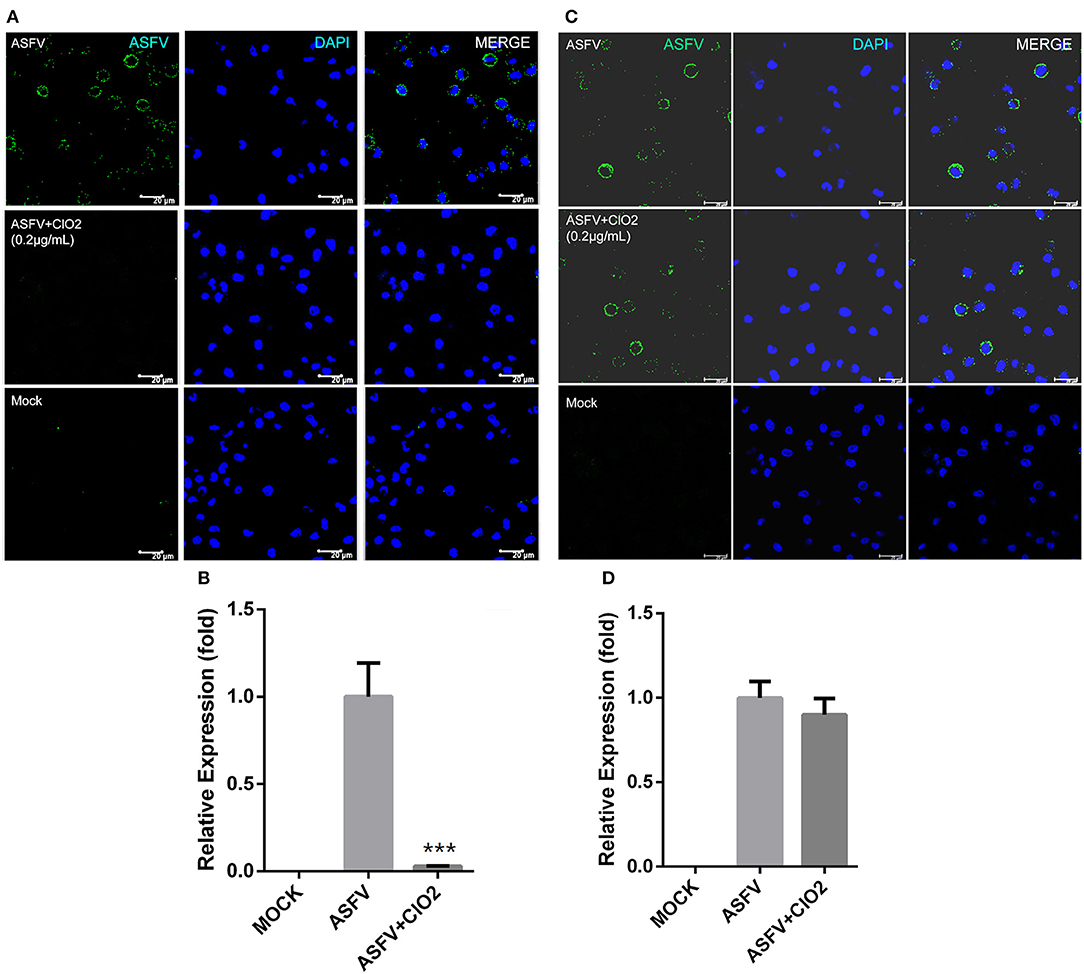
Figure 6. ClO2 inhibits virus adsorption rather than entry during ASFV life cycle. (A,B) Cells were pre-chilled for 30 min at 4°C and mock-infected or infected with ASFV (MOI = 1) in the presence or absence of ClO2. After cultured for 3 h at 4°C, cells were then washed and switched to 37°C for additional 4 h. The inhibitory effect was determined by IFA (A) and qRT-PCR (B) assays. Bar, 20 μm. (C,D) For the viral entry assay, cells were cooled for 30 min at 4°C and mock-infected or infected with ASFV at an MOI of 1 for 3 h at 4°C. Cells were then washed three times to remove unbound virions and incubated at 37°C in the presence or absence of ClO2 for 4 h. Cells were fixed and stained for ASFV p30 protein using fluorescence microscope (C) or collected to determine the mRNA level of viral p72 protein (D). Bar, 20 μm. Data are representative of the results of three independent experiments (means ± SE). Significant differences compared with control group are denoted by ***P < 0.001.
The ASFV Inactivation by ClO2 Treatment May Be Attributed to Its Effects on Viral Nucleic Acids and Proteins
Previous studies have shown that ClO2 produces its antiviral activity against hepatitis A virus by acting on viral nucleic acids and proteins (19, 26). Since ClO2 has the ability to kill ASFV, we next investigated that whether ClO2 can damage the nucleic acids of the virus. ClO2 at the concentration of 0.1 μg/mL was incubated with ASFV for 30 or 60 min at 37°C, and then the mixtures were harvested for viral nucleic acids analysis by PCR. The starting amount of ASFV added to each reaction is 5.8 × 106 copies. As shown in Figure 7A, there were no detectable fragments of viral p32 gene and partial p72 gene upon ClO2 treatment, indicating that the ASFV nucleic acids can be destroyed by ClO2 treatment. To determine the minimum concentration at which ClO2 destroys the viral nucleic acids, quantitative PCR was performed (27). As shown in Figure 7B, ClO2 at the concentration of 1.2 μg/mL completely destroyed the viral genome (no Ct values were detectable). The viral nucleic acids were almost degraded by ClO2 treatment at the concentration of 0.2 μg/mL for 60 min at 37°C (Figure 7C). Since the effect of ClO2 in killing ASFV virions is also affected by temperature, we next investigate the ability of viral nucleic acids damage by ClO2 in different temperatures. As expected, the Ct values decreased with the drop of temperature post ClO2 treatment (Figure 7D), indicating that ClO2 degrades ASFV nucleic acids in a temperature-dependent manner. The effect of ClO2 on the viral proteins was also tested. ClO2 at different concentrations was incubated with ASFV for 30 min at 37°C. As expected, ASFV was degraded with ClO2 treatment, and the minimum concentration of degradation is 0.2 μg/mL (Figure 7E). Taken together, ClO2 is capable of damaging the ASFV nucleic acids and degrading the viral proteins, which may contribute to its inactivation of ASFV.
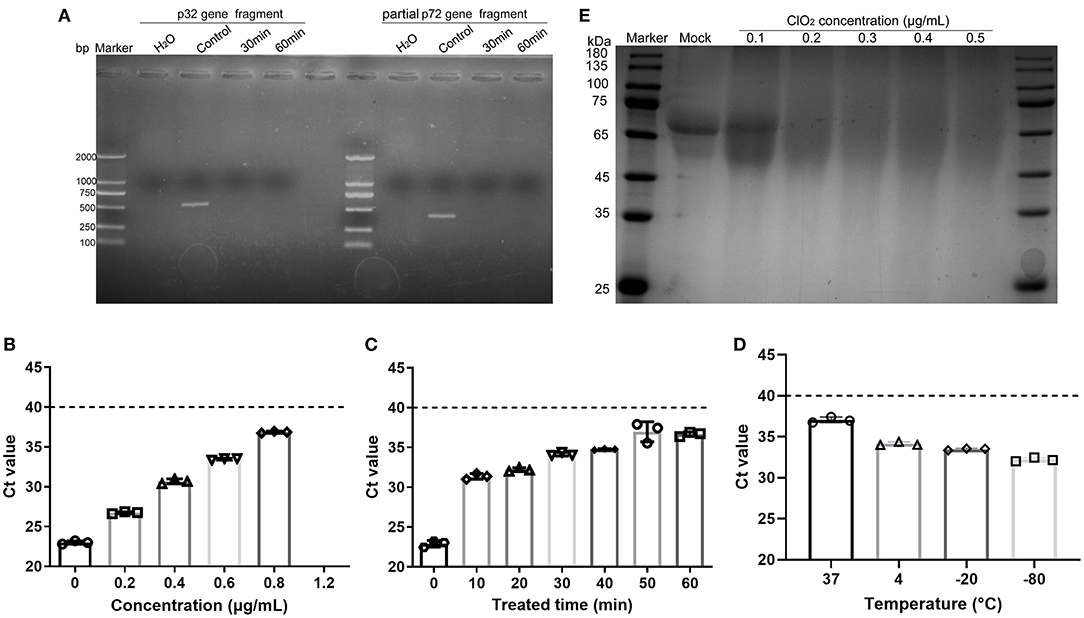
Figure 7. ClO2 inactivation of ASFV virions may due to its effects on viral nucleic acids and proteins. (A) ASFV was incubated with ClO2 at the indicated concentration for 30 or 60 min at 37°C, the fragments of virus p32 gene (558 bp) and partial p72 gene (478 bp) were amplified by PCR to test the viral nucleic acids damage by ClO2. The starting amount of ASFV added to each reaction is 5.8 × 106 copies. (B–D) To determine the minimum concentration at which ClO2 destroys the viral nucleic acids and the effect of ClO2 in killing ASFV in various temperature, different concentrations of ClO2 were incubated with ASFV for 30 min at 37°C (B), or ClO2 at the indicated concentration was incubated with the virus at 37°C for various times (C) or at different temperature for 60 min (D), the mixtures were then collected and quantitative PCR was performed to measure the level of viral p72 gene. The threshold cycle (Ct) values which are below the cut-off (dashed line) mean ASFV-positive. (E) The effects of ClO2 on the viral proteins were also tested. ClO2 at different concentrations was incubated with ASFV for 30 min at 37°C, the mixtures were subjected to SDS-PAGE analysis. Data are representative of the results of three independent experiments (means ± SE).
ClO2 Decreases the Level of Inflammatory Cytokines Induced by ASFV
Since PAMs are immune cells and play an important role in the innate immune response, we next investigated the effects of ClO2 on the expression of cytokines such as interleukin-6 (IL-6) and interferon-β (IFN-β), which are known to be related to the host antiviral and inflammatory reactions. As shown in Figure 8, the upregulation of IL-6 and IFN-β induced by ASFV infection was significantly diminished in the presence of ClO2 in PAMs at 6 hpi. The expression level of IL-6 and IFN-β in cells treated with ClO2 alone was comparable to that of mock-treated cells. These data suggest that ClO2 inhibition of cytokines expression caused by ASFV may contribute to its capacity of suppressing virus replication.
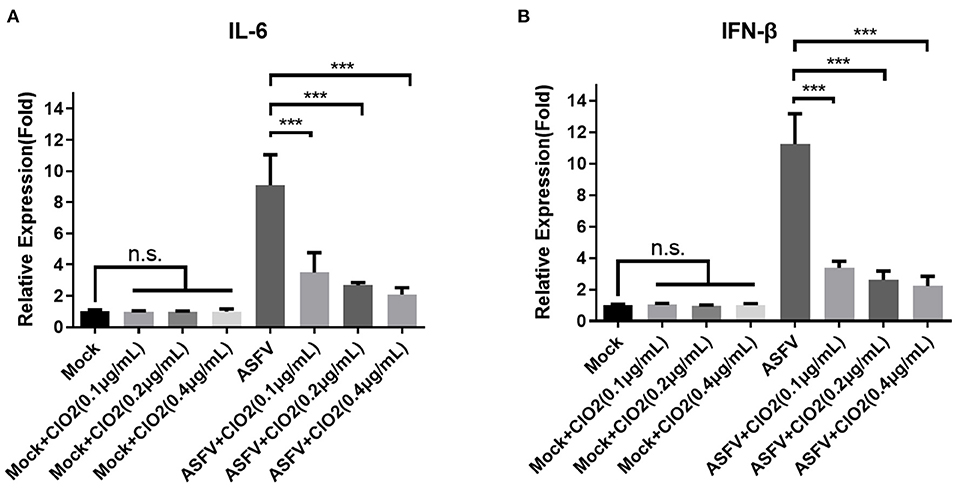
Figure 8. Cytokines expression in PAMs treated with ClO2. (A,B) PAMs were mock infected or infected with ASFV at an MOI of 1 in the presence of various concentrations of ClO2. After cultured for 6 h, cells were lysed for the determination of the expression of IL-6 (A) and IFN-β (B) using qRT-PCR. Data are representative of the results of three independent experiments (means ± SE). Significant differences compared with control group are denoted by ***P < 0.001.
Discussion
Chinese pig farming accounts for 60% of the global pig production industry. The first ASF case in China was reported in August 2018 and the virus has rapidly spread throughout the whole country, leading to serious economic losses to the pig industry in China (4). At present no effective vaccine or treatment is available to prevent or cure ASF, the only possible way to control this disease is the implementation of strict biosecurity measures, and the continuing threat of the disease is overwhelming (28, 29). Therefore, there is an urgent need to develop effective drugs against the virus.
Previous researches refer to ClO2 as an ideal biocide (16). ClO2 has a broad spectrum antimicrobial activity, and it needs only a few milliseconds to kill bacteria. Although the disinfectants such as sodium hypochlorite and hydrogen peroxide currently in use show an anti-microorganism effect, they are toxic even at low concentrations. However, ClO2 has little toxicity to humans and animals even at adequate pathogens inactivating activity. In addition, the main current health challenges are the rise of drug-resistant bacteria and the decline of effective new antibiotics. Since ClO2 damages pathogens via destroying nucleic acids and key proteins, the pathogens cannot resist ClO2 through any of their resistance mechanisms (16). Therefore, due to its broad-spectrum antimicrobial activity, and non-drug resistance side effects, ClO2 is widely used in many fields such as drinking water, fruits in food industry, and environment disinfection. In this study, we demonstrated that ClO2 could effectively inhibit ASFV infection and replication in PAMs (Figures 1–3) and inactivate ASFV by eliminating viral nucleic acids and proteins (Figure 7). To demonstrate that ClO2 is not a PCR inhibitor, we amplified the housekeeping gene GAPDH in Marc-145 cells in the presence or absence of ClO2. No changes were observed in the amplification efficiency of GAPDH upon ClO2 treatment (data not shown), suggesting that ClO2 indeed directly destroys ASFV genomic DNA.
If the purity of ClO2 is low and contains impurities, its use is harmful to the human body and food (18). In this study, to maximize the safety of ClO2 and to eliminate or reduce the impurities, we used ClO2 with a purity of 99%, and ClO2 is dissolved in water. Due to the volatilization of the gas, the residence time of ClO2 in aqueous solution is very short.
Inactivation of infectivity is the most important and direct index to evaluate the effects of virus disinfection (19). Here, we showed that ClO2 could inactivate the ASFV virions which may be due to its destructive effects on viral nucleic acids and proteins (Figures 4, 5, 7). Furthermore, we found that the minimum concentration of degradation of ASFV nucleic acids by ClO2 was 1.2 μg/mL (Figure 7B), which has guiding significance for ClO2 prevention and control of ASFV infection in pig farms. In addition, we showed that ClO2 treatment caused a reduction of cytokines expression induced by ASFV (Figure 8), which might contribute to its suppression of the virus. However, whether in vivo tests in pigs show consistent results needs further study.
In conclusion, our study proves for the first time that ClO2, the purity of which is 99%, has very little cytotoxicity and exhibits a very strong anti-ASFV activity via targeting attachment process of viral life cycle. ClO2 has the ability to damage viral nucleic acids and proteins to inactivate ASFV virions. Overall, ClO2 has the potential to develop into a novel drug against ASFV infection in swine industry in the future. The in vivo anti-ASFV effect of ClO2 on pigs requires further testing.
Data Availability Statement
The original contributions presented in the study are included in the article, further inquiries can be directed to the corresponding authors.
Ethics Statement
The animal study was reviewed and approved by the Institutional Animal Care and Use Committee of Sun Yat-sen University.
Author Contributions
CG: conceptualization, formal analysis, resources, writing—review and editing, visualization, and project administration. RW and XW: methodology and software. RW, XW, and CG: validation. XL and CG: investigation, data curation, supervision, and funding acquisition. RW: writing—original draft preparation. All authors have read and agreed to the published version of the manuscript.
Funding
This work was jointly supported by National Natural Science Foundation of China (32072695 and 31872329), Natural Science Foundation of Guangdong province (2019B1515210024), and a special grant (Grant No. 2019B020211003) for detection of ASFV from Guangdong Provincial Department of Science and Technology.
Conflict of Interest
The authors declare that the research was conducted in the absence of any commercial or financial relationships that could be construed as a potential conflict of interest.
Publisher's Note
All claims expressed in this article are solely those of the authors and do not necessarily represent those of their affiliated organizations, or those of the publisher, the editors and the reviewers. Any product that may be evaluated in this article, or claim that may be made by its manufacturer, is not guaranteed or endorsed by the publisher.
References
1. Gavier-Widen D, Stahl K, Dixon L. No hasty solutions for African swine fever. Science. (2020) 367:622–4. doi: 10.1126/science.aaz8590
2. Liu RL, Sun YP, Chai Y, Li S, Li SH, Wang L, et al. The structural basis of African swine fever virus pA104R binding to DNA and its inhibition by stilbene derivatives. P Natl Acad Sci USA. (2020) 117:11000–9. doi: 10.1073/pnas.1922523117
3. Frouco G, Freitas FB, Coelho J, Leitao A, Martins C, Ferreira F. DNA-Binding properties of African swine fever virus pA104R, a Histone-like protein involved in viral replication and transcription. J Virol. (2017) 91: e02498-16. doi: 10.1128/JVI.02498-16
4. Zhao DM, Liu RQ, Zhang XF, Li F, Wang JF, Zhang JW, et al. Replication and virulence in pigs of the first African swine fever virus isolated in China. Emerg Microbes Infect. (2019) 8:438–47. doi: 10.1080/22221751.2019.1590128
5. Wen XX, He XJ, Zhang X, Zhang XF, Liu LL, Guan YT, et al. Genome sequences derived from pig and dried blood pig feed samples provide important insights into the transmission of African swine fever virus in China in 2018. Emerg Microbes Infect. (2019) 8:303–6. doi: 10.1080/22221751.2019.1565915
6. Sanna G, Dei Giudici S, Bacciu D, Angioi PP, Giammarioli M, De Mia GM, et al. Improved strategy for molecular characterization of African swine fever viruses from Sardinia, based on analysis of p30, CD2V and I73R/I329L variable regions. Transbound Emerg Dis. (2017) 64:1280–6. doi: 10.1111/tbed.12504
7. Bao JY, Wang QH, Lin P, Liu CJ, Li L, Wu XD, et al. Genome comparison of African swine fever virus China/2018/AnhuiXCGQ strain and related European p72 Genotype II strains. Transbound Emerg Dis. (2019) 66:1167–76. doi: 10.1111/tbed.13124
8. Olasz F, Meszaros I, Marton S, Kajan GL, Tamas V, Locsmandi G, et al. A simple method for sample preparation to facilitate efficient whole-genome sequencing of African swine fever virus. Viruses. (2019) 11:1129. doi: 10.3390/v11121129
9. Karger A, Perez-Nunez D, Urquiza J, Hinojar P, Alonso C, Freitas FB, et al. An update on African swine fever virology. Viruses. (2019) 11:864. doi: 10.3390/v11090864
10. Netherton CL, Connell S, Benfield CTO, Dixon LK. The genetics of life and death: virus-host interactions underpinning resistance to African swine fever, a viral hemorrhagic disease. Front Genet. (2019) 10:402. doi: 10.3389/fgene.2019.00402
11. Franzoni G, Graham SP, Sanna G, Angioi P, Fiori MS, Anfossi A, et al. Interaction of porcine monocyte-derived dendritic cells with African swine fever viruses of diverse virulence. Vet Microbiol. (2018) 216:190–7. doi: 10.1016/j.vetmic.2018.02.021
12. Zhu ZZ, Xiao CT, Fan YS, Cai ZN, Lu CY, Zhang GH, et al. Homologous recombination shapes the genetic diversity of African swine fever viruses. Vet Microbiol. (2019) 236:108380. doi: 10.1016/j.vetmic.2019.08.003
13. Sanchez EG, Quintas A, Perez-Nunez D, Nogal M, Barroso S, Carrascosa AL, et al. African swine fever virus uses macropinocytosis to enter host cells. Plos Pathog. (2012) 8: e1002754. doi: 10.1371/journal.ppat.1002754
14. Wang N, Zhao DM, Wang JL, Zhang YL, Wang M, Gao Y, et al. Architecture of African swine fever virus and implications for viral assembly. Science. (2019) 366:640–44. doi: 10.1126/science.aaz1439
15. Rock DL. Challenges for African swine fever vaccine development-"…perhaps the end of the beginning”. Vet Microbiol. (2017) 206:52–8. doi: 10.1016/j.vetmic.2016.10.003
16. Palcso B, Moldovan Z, Suvegh K, Herczegh A, Zelko R. Chlorine dioxide-loaded poly (acrylic acid) gels for prolonged antimicrobial effect. Mat Sci Eng C-Mater. (2019) 98:782–8. doi: 10.1016/j.msec.2019.01.043
17. Dodd MC. Potential impacts of disinfection processes on elimination and deactivation of antibiotic resistance genes during water and wastewater treatment. J Environ Monitor. (2012) 14:1754–71. doi: 10.1039/c2em00006g
18. Ma JW, Huang BS, Hsu CW, Peng CW, Cheng ML, Kao JY, et al. Efficacy and safety evaluation of a chlorine dioxide solution. Int J Env Res Pub He. (2017) 14:329. doi: 10.3390/ijerph14030329
19. Li JW, Xin ZT, Wang XW, Zheng JL, Chao FH. Mechanisms of inactivation of hepatitis A virus by chlorine. Appl Environ Microb. (2002) 68:4951–5. doi: 10.1128/AEM.68.10.4951-4955.2002
20. Sanekata T, Fukuda T, Miura T, Morino H, Lee C, Maeda K, et al. Evaluation of the antiviral activity of chlorine dioxide and sodium hypochlorite against feline calicivirus, human influenza virus, measles virus, canine distemper virus, human herpesvirus, human adenovirus, canine adenovirus and canine parvovirus. Biocontrol Sci. (2010) 15:45–9. doi: 10.4265/bio.15.45
21. Zhu Z, Guo Y, Yu P, Wang X, Zhang X, Dong W, et al. Chlorine dioxide inhibits the replication of porcine reproductive and respiratory syndrome virus by blocking viral attachment. Infect Genet Evol. (2019) 67:78–87. doi: 10.1016/j.meegid.2018.11.002
22. Lim MY, Kim JM, Ko G. Disinfection kinetics of murine norovirus using chlorine and chlorine dioxide. Water Res. (2010) 44:3243–51. doi: 10.1016/j.watres.2010.03.003
23. Sigstam T, Rohatschek A, Zhong QX, Brennecke M, Kohn T. On the cause of the tailing phenomenon during virus disinfection by chlorine dioxide. Water Res. (2014) 48:82–9. doi: 10.1016/j.watres.2013.09.023
24. Montazeri N, Manuel C, Moorman E, Khatiwada JR, Williams LL, Jaykus LA. Virucidal activity of fogged chlorine dioxide-and hydrogen peroxide-based disinfectants against human norovirus and its surrogate, feline calicivirus, on hard-to-reach surfaces. Front Microbiol. (2017) 8:1031. doi: 10.3389/fmicb.2017.01031
25. Zhong QX, Carratala A, Shim H, Bachmann V, Jensen JD, Kohn T. Resistance of echovirus 11 to ClO2 Is associated with enhanced host receptor use, altered entry routes, and high fitness. Environ Sci Technol. (2017) 51:10746–55. doi: 10.1021/acs.est.7b03288
26. Li JW, Xin ZT, Wang XW, Zheng JL, Chao FH. Mechanisms of inactivation of hepatitis A virus in water by chlorine dioxide. Water Res. (2004) 38:1514–9. doi: 10.1016/j.watres.2003.12.021
27. Fernandez-Pinero J, Gallardo C, Elizalde M, Robles A, Gomez C, Bishop R, et al. Molecular diagnosis of African swine fever by a new real-time PCR using universal probe library. Transbound Emerg Dis. (2013) 60:48–58. doi: 10.1111/j.1865-1682.2012.01317.x
28. Galindo I, Alonso C. African swine fever virus: a review. Viruses. (2017) 9:109. doi: 10.3390/v9050103
Keywords: African swine fever (ASF), antiviral, chlorine dioxide, viral attachment, cytokine
Citation: Wei R, Wang X, Liu X and Guo C (2022) Chlorine Dioxide Inhibits African Swine Fever Virus by Blocking Viral Attachment and Destroying Viral Nucleic Acids and Proteins. Front. Vet. Sci. 9:844058. doi: 10.3389/fvets.2022.844058
Received: 27 December 2021; Accepted: 21 February 2022;
Published: 17 March 2022.
Edited by:
Shao-Lun Zhai, Guangdong Academy of Agricultural Sciences, ChinaReviewed by:
Christopher Lewis Netherton, The Pirbright Institute, United KingdomTongling Shan, Chinese Academy of Agricultural Sciences (CAAS), China
Copyright © 2022 Wei, Wang, Liu and Guo. This is an open-access article distributed under the terms of the Creative Commons Attribution License (CC BY). The use, distribution or reproduction in other forums is permitted, provided the original author(s) and the copyright owner(s) are credited and that the original publication in this journal is cited, in accordance with accepted academic practice. No use, distribution or reproduction is permitted which does not comply with these terms.
*Correspondence: Chunhe Guo, Z3VvY2h1bmhAbWFpbC5zeXN1LmVkdS5jbg==
†These authors have contributed equally to this work and share first authorship