Effects of Different Oligosaccharides on Growth Performance and Intestinal Function in Broilers
- 1College of Animal Science and Technology, Hunan Agricultural University, Changsha, China
- 2Provincial and Ministerial Co-construction of Collaborative Innovation Center for High-quality Animal Products Production, Changsha, China
- 3Hunan Engineering Research Center of Poultry Production Safety, Changsha, China
- 4Guangxi Fufeng Agriculture and Animal Husbandry Group Co., Ltd., Nanning, China
Objective: This study was conducted to investigate the effects of different oligosaccharides on the growth performance and intestinal function in broilers.
Methods: A total of 360 1-day-old yellow-feather chickens were randomly divided into 5 groups and fed with a basal diet supplemented with 50 mg/kg chlortetracycline (ANT), 3 g/kg isomalto-oligosaccharide (IMO), 3 g/kg raffinose oligosaccharide (RFO), and 30 mg/kg chitooligosaccharide (COS). The experiment lasted for 56 days, with 1–28 days as the starter phase and 29–56 days as the grower phase.
Results: The results showed that dietary supplementation with RFO and COS significantly improved average daily gain (ADG) and average daily feed intake (ADFI) (p < 0.05). Relative to the control group, diets supplemented with oligosaccharides dramatically increased the level of serum IgM (RFO, COS), T-SOD (COS), and GSH-Px (IMO and RFO) and the expression of ZO-1(IMO) and claudin-1 (RFO) (p < 0.05). Adding antibiotics or oligosaccharides to the diet could remarkedly increase the villus height and villus height (VH)/crypt depth (CD) ratio of each group (p < 0.05). Through the ileum α-diversity analysis and comparison of OTU number in each group showed that the microbial richness of the IMO group increased in the starter phase, and that of the RFO and CSO group increased in the grower phase. Additionally, compared with the control group, IMO supplementation increased the level of ileum sIgA (p < 0.05) and the content of valeric acid (p < 0.05) in the cecum.
Conclusions: In summary, the addition of oligosaccharides in diet can improve the immune function and antioxidant capacity and improve intestinal health of broilers.
Introduction
An intestinal tract is an important place for digestion and absorption of nutrients in poultry. The intestine plays a dual role that not only maintains the proper permeability to ensure that nutrients are fully digested, but also generates a certain type of cell junction, thus forming the intestinal defense barrier, which is consisted of a mechanical barrier, chemical barrier, immune barrier, and microbial barrier (1). Claudins and occludins are the transmembrane proteins that form the backbone of the intestinal epithelium with tight connections (2). Closed-loop proteins (ZO) are intracellular proteins that provide structural support for tight junctions (3). Mucin secreted by intestinal goblet cells, and mucopolysaccharide, lysozyme, bile salt, and gastric acid secreted by the digestive tract constitute the chemical barrier (4). The microbiota is a stable microecological barrier in the intestine. Meanwhile, gut-associated lymphoid tissue (GALT) and its secreted immune factors form the immune barrier of the intestine, such as cytokines, secretory immunoglobulin A (sIgA) (5). Various barriers are coordinated with each other through different signaling pathways, molecular regulatory mechanisms, and biological functions of organic combination to effectively prevent the invasion of harmful substances through the intestine to poultry.
As feed additives, antibiotics have been widely used in global animal husbandry, which can not only improve the growth performance of animals but also prevent and treat diseases, bringing huge economic benefits to producers (6). These effects are related to the antibiotic function in the gut. In addition, antibiotics serve as an inhibitor to suppress the growth of intestinal bacteria. Bacteria can affect the synthesis of bacterial peptidoglycan, which results in incomplete cell wall components and declined osmotic pressure, which leads to bacterial deformation, rupture, and death (7). However, the excessive use of antibiotics will lead to an imbalance of microecological balance, drug residues in animal products, and antibiotic resistance (8). Thus, it is critical to find alternative substituent products with similar antibiotic effects but without severe contaminations to the environment or public health. Functional oligosaccharides, which are natural, versatile, non-toxic, and non-resistant compounds, are considered as new feed additives that can replace antibiotics environmental-friendly under certain conditions (9). Studies have shown that oligosaccharides could improve animal performance, intestinal microflora, immune regulation, antioxidant, antimicrobial, anti-inflammatory, and cholesterol reduction (10, 11). At present, many studies about the effects of oligosaccharides on animal production are to investigate the consequences of dietary supplementation of a single oligosaccharide (12, 13). There are few studies on the comparison of the effects of various oligosaccharides. In our research, three different kinds of oligosaccharides, isomalto-oligosaccharide (IMO), raffinose oligosaccharide (RFO), and chitooligosaccharide (COS), were added to the basal diet to investigate their effects on growth performance, immune and antioxidant functions, intestinal morphology, and microbiota.
Materials and Methods
Animal, Diets, and Experimental Design
The IMO and COS were provided by the Baolingbao Biology Company (Shandong, China). The RFO was provided by Xinrui Biotech (Hunan, China). Based on a single-factor completely randomized design, a total of 360 1-day-old yellow-feather broilers were randomly assigned to five groups with six replicates per treatment and 12 birds per replicate. Five treatments included: (1) CON group: supplied corn-soybean meal basal diet; (2) ANT group: supplied with chlortetracycline (50 mg/kg) based on basal diet; (3) IMO group: supplied with IMO [3 g/kg, according to Thitaram et al. (14) and Zhang et al. (15)] based on basal diet; (4) RFO group: supplied with RFO [3 g/kg, according to Hua et al. (16)] based on basal diet; (5) COS group: supplied with COS [30 mg/kg, according to Khambualai et al. (17)] based on basal diet. The basal diet used in the current study was formulated to meet the recommended nutrient requirements for broilers according to the NRC guidelines [Table 1; National Research Council (18)]. Broilers were reared in a temperature-controlled house under a light schedule of 24 h of light and were provided with mash feed and water ad libitum. The whole test period is 56 days, which is divided into two phases: the starter phase (1–28 days) and the grower phase (29–56 days).
Growth Performance
The experiment lasted for 8 weeks. At the beginning of the experiment, the fourth week of the experiment, and the end of the experiment, broilers were weighed by pen (replication), and the feed consumption was recorded by replication, respectively. Average daily feed intake (ADFI), average daily gain (ADG), and feed to gain ratio (F/G) were calculated for periods of 1–28, 29–56, and 1–56 days. In addition, the average body weight on days 28 (D28) and 56 (D56) was calculated.
Sample Collection
At 28, 56 days of age, after 8 h of starvation, 6 birds (1 bird per replicate) were randomly selected from each treatment group and collected about 5 ml of blood at the venous sinus of the wing vein. The serum, intestinal samples, ileal chyme, and cecal chyme were collected and stored at −80°C for further analysis.
Determination of Antioxidants and Immunity in the Serum
The serum IgA and IgM were measured using the commercial assay kits purchased from Well Biotech (Jiangsu, China) (19). The levels of MDA, GSH-Px, T-AOC, and T-SOD were using the commercial assay kits purchased from Jiancheng Bioengineering Research Institute (Nanjing, China). The product serial numbers are A003-1-1, A005-1-2, A015-1-2, and A001-1-2, respectively.
Intestinal Morphology
Briefly, the intestinal samples were dehydrated with increasing concentrations of ethanol, cleared with xylene (Surgipath Medical Industries, Richmond, IL, USA), embedded with paraffin wax (Thermo Fisher Scientific, Kalamazoo, MC, USA), and cut into 4-μm-thick histological sections for hematoxylin and eosin staining. Three straight and well-formed villi were observed for calculating the villous height (VH) and corresponding crypt depth (CD) under the microscope.
Determination of SIgA Content
Under cold conditions in an ice water bath, the tissue homogenate was prepared using 0.9% NaCl at a weight (g)-to-volume (ml) ratio of 1:9. The homogenate supernatant was obtained by centrifugation (3,500 rpm) for 10 min. The level of sIgA was assayed in the homogenate supernatant of the ileum using the commercial assay kits purchased from Well Biotech (Jiangsu, China).
Gene Expression
Total RNA of the ileum was isolated using the SteadyPure Universal RNA Extraction Kit (Accurate Bioengineering Co., Ltd., Hunan, China). The mRNA expression levels of mucin-2, claudin-1, occludin, ZO-1, and β-actin were measured by quantitative real-time PCR (RT-qPCR) technique with the primers shown in Table 2. The RT-qPCR was performed using the TB Green Premix Ex Taq (TaKaRa, Biotechnology, Dalian, China). The mRNA levels were calculated using the 2−ΔΔCt method.
Determination of Volatile Fatty Acids (VFAs) in the Cecum
The cecum from the frozen tube, thaw, and centrifuge was removed, then, 1 ml of the supernatant was filtered, and the gas chromatography method was used as mentioned in the previous study (20). The content of acetic acid, propionic acid, n-butyric acid, and isobutyric acid was measured by law.
16S rRNA Sequencing Analysis
Five samples of cecal contents obtained separately from six random replicates were used to extract DNA for subsequent 16S rRNA sequencing analysis. DNA was extracted from samples of broilers using a Stool DNA Isolation Kit (Tiangen Biotech Co., Ltd., Beijing, China). Amplicons of the V4 hypervariable region of 16S rRNA were amplified using the sample-specific sequence barcoded fusion primers (forward 5'-GTGCCAGCMGCCGCGGTAA-3' and reverse 5'-GGACTACHVGGGTWTCTAAT-3', provided by Allwegene Company, Beijing, China) (21). The volume of PCR reaction was 25 μl, which contains 12.5 μl of Phusion® High-Fidelity PCR Master Mix (New England Biolabs Inc., Beverly, MA,USA), 2 μl of forward and reverse primers, 30 ng of template DNA, and 7.5 μl double distilled H2O (ddH2O). Cycling parameters were 98°C for 1 min, followed by 30 cycles at 98°C for 10 s, 57°C for 30 s, and 72°C for 30 s, and a final extension at 72°C for 10 min. PCR products were mixed in equidensity ratios and purified with the GeneJET Gel Extraction Kit (Thermo Fisher Scientific Inc., Schwerte, Germany), quantified using real-time PCR, and sequenced at Allwegene Company, Beijing. The sequences were clustered into operational taxonomic units (OTUs) at a similarity level of 97% to generate rarefaction curves (22) and to calculate the richness and diversity indices (23). OTUs representing <0.005% of the population were removed, and taxonomy was assigned by the Ribosomal Database Project (RDP) classifier.
Statistical Analysis
All statistical analyses were performed using SPSS 20.0 software (SPSS Inc., Chicago, IL, United States). Alpha and beta diversities were analyzed with QIIME (v1.7.0) and displayed with R software (v3.5.1). The differences among groups were compared using one-way ANOVA and Duncan's multiple range test. p-values <0.05 were used to indicate statistical significance. β-diversity was assessed by MANOVA and principal coordinate analysis. Significant differences in α-diversity and OTU counts between the different groups were determined by one-way analysis followed by Duncan's multiple comparison test using the SPSS.
Results
Growth Performance
As shown in Table 3, in the starter phase (1–28 days), compared with the CON group, the ADG of the RFO group increased by 17.25% (p < 0.05), and the ADFI of the RFO group increased by 14.39% (p < 0.01). There was no significant difference in growth performance between the experimental groups in the grower phase (29–56 days) (p > 0.05). From the whole phase, compared with the CON group, the ADG of the RFO group and ANT group showed an increasing trend (p > 0.05). Compared with the IMO group, the ADG of the RFO group increased by 19.44% (p < 0.05).
Immunoglobulin Concentration
As shown in Table 4, at 28 days, compared to the control group, diets supplemented with oligosaccharides could improve the concentration of IgM, and the effect of COS was the most significant (p < 0.05). Additionally, compared to the control group, the concentration of sIgA increased in broiler diets supplemented with oligosaccharides (p < 0.05) except RFO. At 56 days, IgM levels in the ANT group and RFO group were significantly higher than those in the CON group (p < 0.05). There was no significant difference in IgA between the starter and grower phases (p > 0.05).
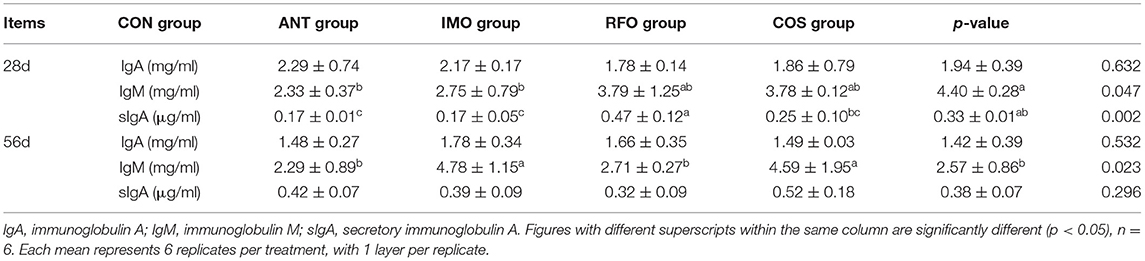
Table 4. Effects of different oligosaccharides on serum IgA, serum IgM, and secretory IgA levels in broilers.
Antioxidant Capacity
The antioxidant indices that include the MDA, T-AOC, T-SOD, and GSH-Px content in the serum of broilers are presented in Figure 1. Compared to the control group, dietary supplementation with oligosaccharides, which include IMO, RFO, and COS, remarkably improved the content of T-SOD in the starter and grower phase (p < 0.05). Additionally, the RFO group markedly increased the content of GSH-Px in the starter and grower phase, and the IMO group sharply increased the content of GSH-Px in the starter phase compared to the control group. There was no significant difference in MDA levels among groups (p > 0.05).
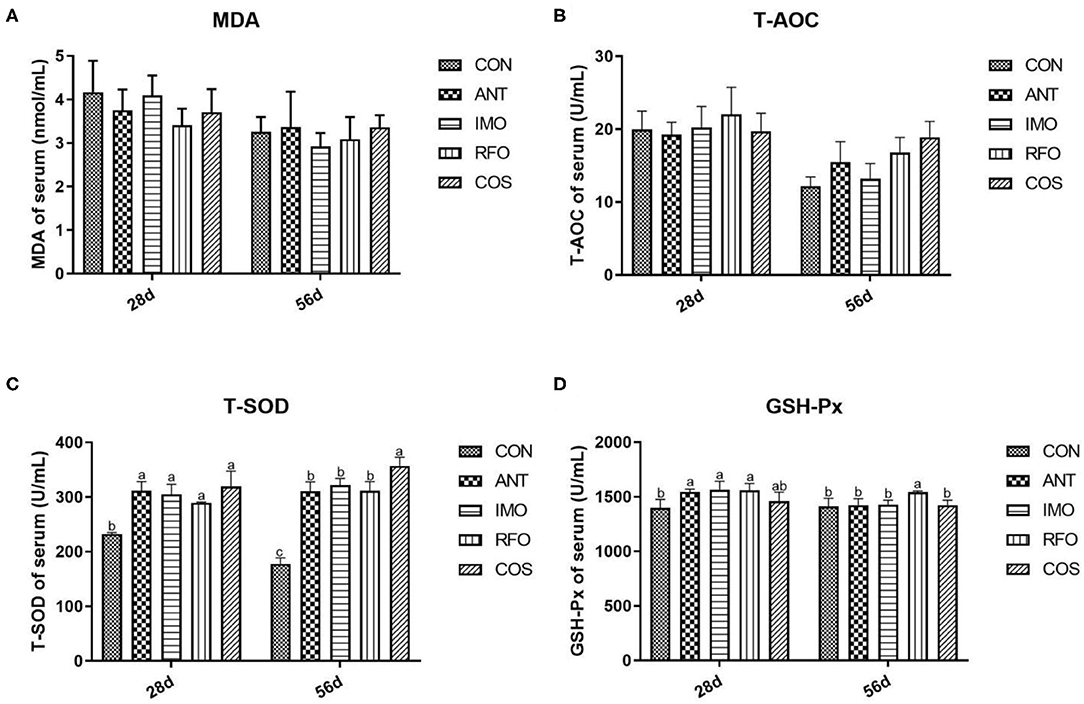
Figure 1. Effects of different treatments on antioxidant indices in serum (n = 6). (A) MDA, (B) T-AOC, (C) T-SOD, and (D) GSH-Px. Graph bars marked with different letters on top represent statistically significant results (p < 0.05) based on ANOVA with Duncan's range tests. CON, control group; ANT, ANT group; IMO, IMO group; RFO, RFO group; COS, COS group.
Intestinal Histomorphology
The normal function and structure of the intestinal tract were indicated by the villus height, CD, and villus length/CD (V/C), as shown in Table 5. In the starter phase, compared with the CON group, the dietary supplementation of oligosaccharides significantly increased the villus height and V/C (p < 0.01), and the dietary supplementation of oligosaccharides significantly decreased the CD (p < 0.01).In the grower phase, compared with the CON group, the villus height was significantly increased in the RFO group (p < 0.01), the CD was significantly decreased in the ANT, IMO, RFO, and COS groups (p < 0.01), and the V/C was significantly increased in the ANT, IMO, RFO, and COS groups (p < 0.01).
Intestinal Mucosal Barrier Function
The effects of dietary supplementation of oligosaccharides on intestinal mucosal barrier function are presented in Figure 2. Relative to the CON group, the IMO group markedly increased the mRNA expression of ZO-1 and claudin-1 (p < 0.05) in the grower phase, but there was no significant difference in the starter phase (p > 0.05). RFO group improved the mRNA expression of claudin-1 (p < 0.05) in the grower phase compared to the control group.
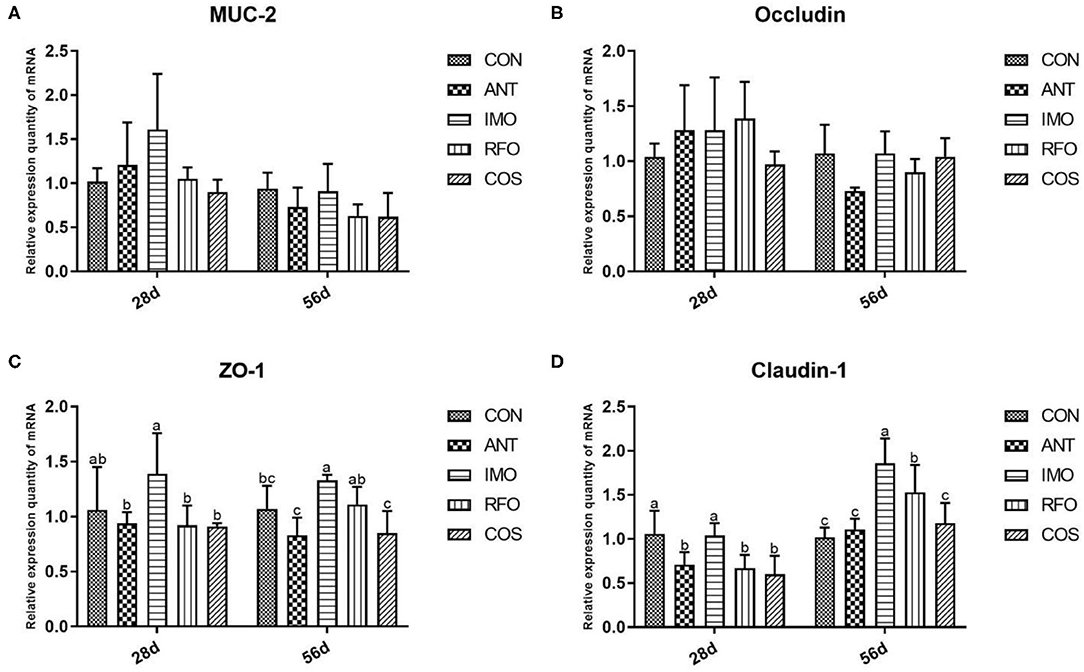
Figure 2. Effects of different treatments on the expression of intestinal related genes and the immune function of ileum in broilers (n = 6). (A) MUC-2, (B) occludin, (C) ZO-1, and (D) claudin-1. Graph bars marked with different letters on top represent statistically significant results (p < 0.05) based on ANOVA with Duncan's range tests. CON, control group; ANT, ANT group; IMO, IMO group; RFO, RFO group; COS, COS group.
Ileum Gut Microbiota
Increasing evidence shows that the addition of functional oligosaccharides to diet can affect the structure and composition of gut microbiota (24). Therefore, we sequenced ileum content samples to elucidate the effects of IMO, RFO, and COS on the gut microbiota structure. At the starter phase, 225 OTUs were detected in all groups (Figure 3A). There were 76, 20, 20, 75, and 3 unique OTUs in the CON, ANT, IMO, RFO, and COS groups, respectively. There was no significant difference in the value of Chao1, Goods_coverage, and Shannon between the CON, ANT, and IMO groups. However, significant differences in Chao1, Goods_coverage, and Observed_species were found between broiler in the ANT and COS groups (Supplementary Table 1). At the grower phase, 232 OTUs were detected in all groups (Figure 3B). There were 6, 95, 11, 134, and 22 unique OTUs in the CON, ANT, IMO, RFO, and COS groups, respectively. Significant differences were also found in the Chao1, Goods_coverage measures of the CON and RFO groups (Supplementary Table 2).
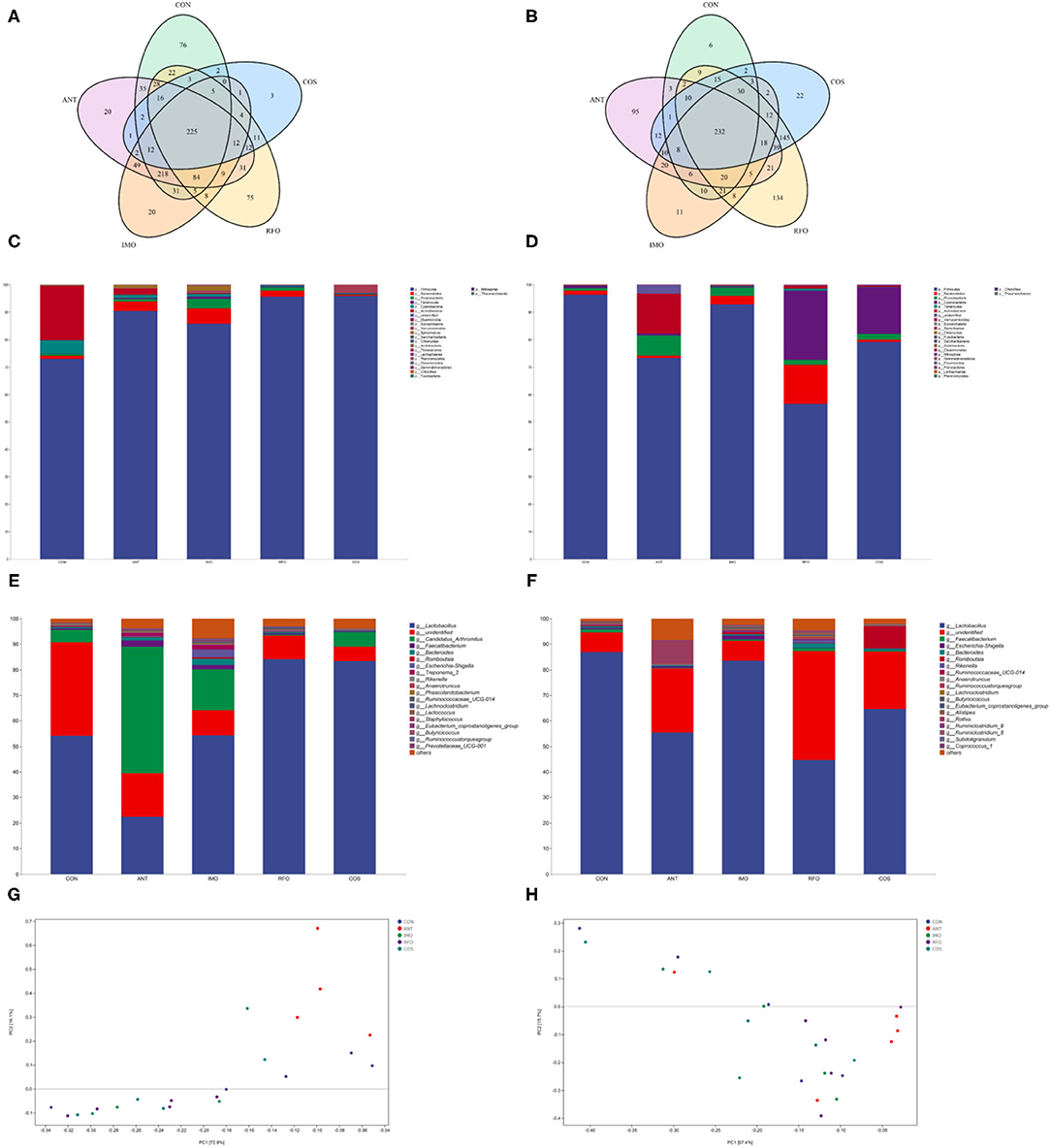
Figure 3. Oligosaccharides alter the composition of the gut microbiota in the broiler (n = 5). Venn diagrams showing the unique and shared OTUs in the gut microbiota of the groups (A,B). Community taxonomic composition and abundance distribution map at the phylum level (C,D). Community taxonomic composition and abundance distribution map at the genus level (E,F). PCA clustering analysis (G,H). ANT, IMO, RFO, and COS correspond to supplemented with chlortetracycline, isomalto-oligosaccharide, raffinose oligosaccharide, and chitooligosaccharide in diet, respectively.
The addition of oligosaccharides in diet could reduce the level of Proteobacteria in the starter phase and increase the level of Firmicutes in broilers. Compared with the CON group, the abundance of Bacteroidetes, Tenericutes, Euryarchaeota, and Spirochaetae in the IMO group was significantly increased (Figure 3C, Supplementary Table 3). There was no significant difference in gut microbial community abundance among broilers in the grower phase (Figure 3D, Supplementary Table 4). Moreover, the ANT group had significantly reduced Lactobacillus and significantly increased Candidatus_Arthromitus, Faecalibacterium, Bacteroides, Ruminococcaceae_UCG_014, and Treponema_2 compared to the CON group in the starter phase (Figure 3E, Supplementary Table 5). Compared with the CON group, supplemented oligosaccharides in the diet could significantly increase the abundance of Lactobacillus, except the IMO group. However, IMO and RFO supplementation significantly increased the abundance of Ruminocaceae_UCG_014 and Lachnoclostridium (Figure 3E, Supplementary Table 5). There was no significant difference in species level of gut microbial community abundance among broilers in the grower phase (Figure 3F, Supplementary Table 6). PCA revealed a separation in the microbiota of the groups (Figures 3G,H). Collectively, these results indicated that oligosaccharides could modulate the gut microbiota of the broiler, especially during the starter phase.
Volatile Fatty Acids
Figure 4 shows that compared with CON and ANT groups, adding IMO in broiler diet can significantly increase the content of valeric acid (p < 0.05).
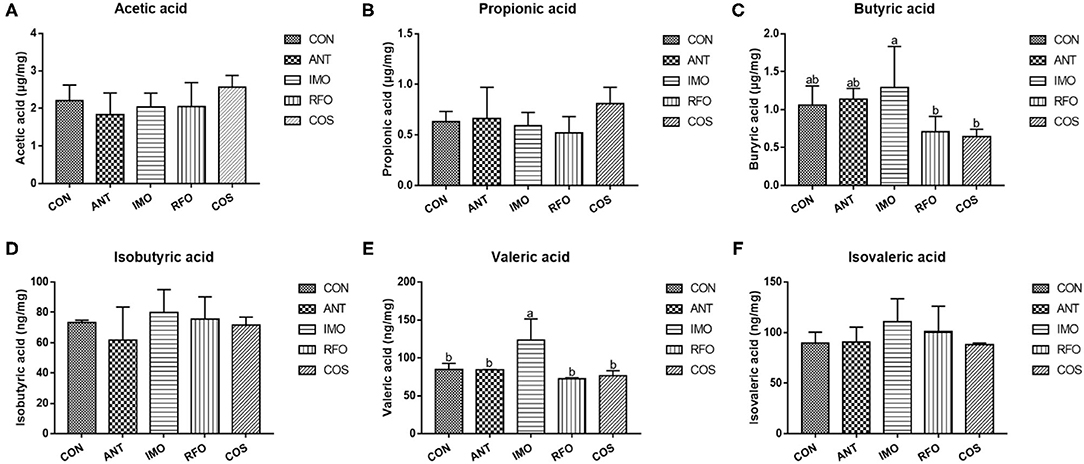
Figure 4. Effects of different oligosaccharides on cecal VFA in yellow-feather broilers (n = 6). (A) Acetic acid, (B) propionic acid, (C) butyric acid, (D) isobutyric acid, (E) valeric acid, and (F) isovaleric acid. Graph bars marked with different letters on top represent statistically significant results (p < 0.05) based on ANOVA with Duncan's range tests. CON, control group; ANT, ANT group; IMO, IMO group; RFO, RFO group; COS, COS group.
Discussion
In the post-antibiotics era, prebiotics are proposed as an alternative to antibiotic growth promoters in poultry production. Oligosaccharides can regulate the balance of intestinal flora and build a healthy and stable ecological environment for animals (25–27). Supplemented COS in the diet could improve the production performance, breast meat quality (28), and regulation of intestinal microflora in broilers (29). Small dose IMO (0.1 or 0.2%) could improve the performance of laying hens (30). In this study, the results show that RFO supplementation significantly increased ADG, but decreased F/G in yellow-feather broilers. It is worth mentioning that during the whole rearing period, the RFO group showed improved ADG by 8%, and F/G was significantly decreased by 2.6%, as compared with the control group, even slightly better than the ANT group. Similar to our findings, the previous studies showed that 1.9 mg/embryo RFO significantly increased the bodyweight of broilers (31). These data suggested that RFO could be used as a potential alternative to antibiotics.
The animal immune response is closely associated with immunoglobulins. IgA is associated with mucosal immunity and IgM is correlated with acute infection (32, 33). sIgA plays an important role in intestinal mucosal defense and is the first line of defense on the intestinal surface. sIgA deficiency resulted in bacterial overgrowth, adherence, and translocation (34). IMO was found to play an active role in humoral and cell-mediated immunity for host animals (35). It was reported that the level of IgA in feces was increased when mice were fed a diet supplemented with 20% IMO (36). Meanwhile, 100 mg/kg COS could also promote the development of immune organs in broilers (37, 38). RFO could improve the immunity of the small intestine (39). Similarly, in the current study, 50 mg/kg COS showed improved IgM by 88.8%, and sIgA was significantly increased by 94.1%, as compared with the control group in the starter phase. Simultaneously, IMO and RFO supplementation also had a positive effect on IgM levels. Wu et al. (40) suggested that dietary supplementation of IMO improved the immune function in weaned pigs. Teng and Kim (41) also indicated that oligosaccharides could improve gut development and the immunity of broilers. Our study agreed with the above reports.
GSH-Px and SOD can reduce toxic peroxide and decompose hydrogen peroxide, respectively, eliminate hydroxyl and oxygen free radicals, and protect the cell membrane structure from damage (42). T-AOC is a comprehensive index to reflect the antioxidant activity in vivo (43). MDA is the product of membrane peroxidation, and the higher content of MDA would indicate the higher the degree of lipid attacked by reactive oxygen species (44). In our study, the addition of these three oligosaccharides was found to improve the antioxidant capacity of broilers. The previous studies have shown that RFO and IMO supplementation in the diet could significantly improve the antioxidant capacity of yellow-feathered broilers (45, 46). Consistent with the previous studies, supplementation with all three oligosaccharides was found to increased serum T-AOC, T-SOD, and GSH-Px levels in yellow-feather broilers. Noteworthy, chitooligosaccharide was a potential antioxidant and its antioxidant activity was linked to the average molecular weight, the degree of acetylation, and the degree of polymerization (47). In this study, COS with a low degree of polymerization was selected, but the effect was also the most remarkable. It supported the hypothesis that oligosaccharides had effects to improve the antioxidant ability of broilers.
The increase in villus height means the increase in the contact area of nutrients to the intestinal epithelium. The differentiation ability of intestinal epithelial cells depends on the depth of the crypt, which determines the numbers of the intestinal stem cells. When the depth of the crypt is over than the normal CD, it may indicate intestinal mucosal lesions (48, 49). It has been found that the villus height and VH/CD value of broilers increased in a dose-dependent manner with RFO after treatment of fertilized eggs with different doses of RFO, but did not affect the depth of the crypt after 21 days (39). In this research, supplementation with IMO, RFO, and COS significantly increased villus height and VH/CD ratio and decreased CD in the ileum. These data might be owned to the change of intestinal microbiota, which contribute greatly to changes in intestinal morphology when the dietary was supplemented with oligosaccharides.
Volatile fatty acids were one of the most important end products of indigestible foods such as carbohydrates fermented by intestinal microorganisms (50). The fermented short-chain fatty acids can provide energy for intestinal epithelial cells (51). Moreover, intestinal microorganisms can influence the barrier function of the host intestine and regulate intestinal immune response, even systematic immune response (52). Therefore, the logistic characteristics of microbiota can affect the content and composition of volatile fatty acids and the expression patterns of intestine-related genes (53). In our study, PCA of the five treatments showed significant differences in principle components, and three oligosaccharides improved the microbial abundance in the ileum. The previous studies had confirmed that COS had obvious antimicrobial activity, while the intestinal microbiota of broiler chickens was relatively simple structure and low abundance (54). The antimicrobial activity of COS was reflected in its ability to inhibit the growth of pathogens but also slightly inhibit the development of beneficial bacteria (55, 56). However, there is an absence of research on the antimicrobial activity of RFO. According to the differences in performance during the starter and grower phase, RFO was similar to the COS group. The content of butyric acid in the IMO group was significantly greater than that in the RFO and COS groups, and the content of valeric acid was the highest.
Intestinal microbiota, which is the key factor to regulate the host intestinal health, can affect the barrier function of the intestinal tract and regulate intestinal immune response, and even systematic immune response (57). From our study, Spearman's correlation thermogram analysis showed that Lactococcus and Leuconostoc were a significant negative correlation with F/G (Figure 5). Lactobacillus were found to be still the dominant genus in poultry ileum and showed a significant positive correlation with poultry growth performance, in agreement with the previous results (58, 59). In the starter phase, the effects of three different oligosaccharide supplements on the intestinal microbiota of broilers were quite different. The proportion of Lactobacillus in the RFO group was dominant. Although the proportion of Lactobacillus in the COS group was almost the same as that in the RFO group, the ratio of Candidatus_Arthromitus was much higher than that of the RFO group. Both segmented filamentous bacteria SFB and Arthromitus filaments from arthropod guts were grouped under the provisional name “Candidatus_Arthromitus” as they share a striking similarity in morphology and close contact with the host gut wall (60). It is suggested that Candidatus_Arthromitus might be a cause of intestinal inflammation (61). These bacteria could adhere to the intestinal epithelium, especially the ileum. SFB played a key role in the maturation of gut innate and adaptive immune function in the postnatal gut. Notably, SFB can induce strong IgA responses (62, 63), and it may be one of the reasons for the difference in growth performance of the RFO group.
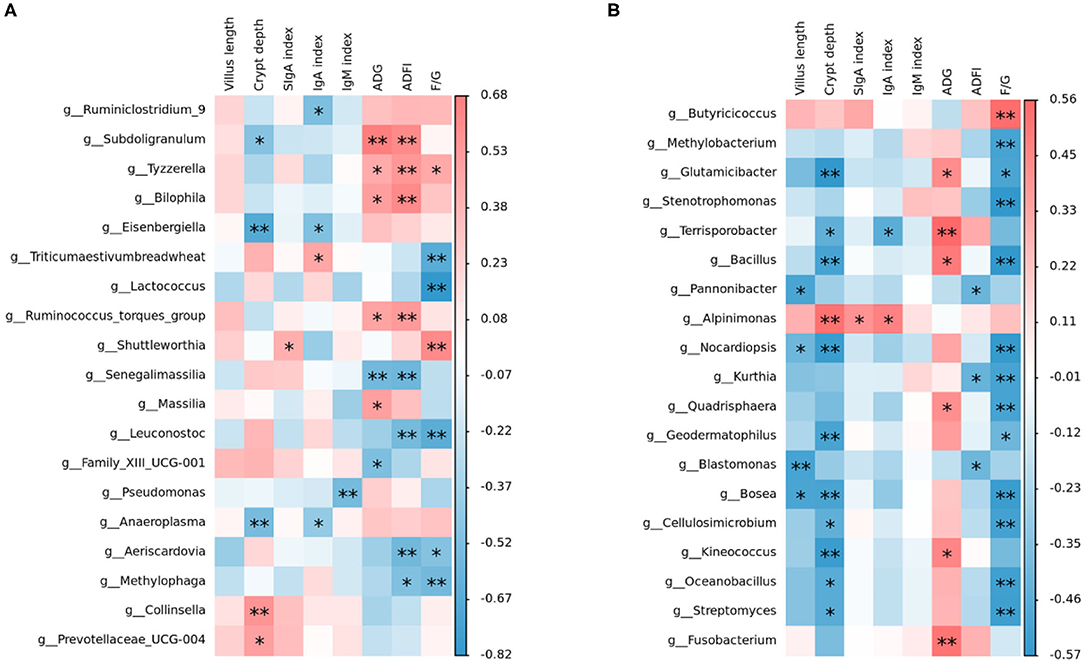
Figure 5. Relationship between intestinal microbiota and indices, including growth performance, serum immunity, sIgA in the ileum, and ileum morphological structure analyzed with a Spearman's correlation heatmap. (A) Correlation of microbiota with indices on starter phase (1–28 days). (B) Correlation of microbiota with indices on grower phase (29–56 days). The colors range from blue (negative correlation) to red (positive correlation). *represents p < 0.05 and ** represents p < 0.01.
Conclusions
In summary, both oligosaccharides and antibiotics can alter the intestinal microbiota, improve the antioxidant capacity, and then improve the growth performance of yellow-feathered broilers in our study. However, in terms of improved growth performance, RFO and COS showed superior effects over IMO. Additionally, the effect of RFO on growth performance was comparable to the use of antibiotics. IMO was greater than RFO and COS in improved intestinal mucosal barrier function. It is very potential to use oligosaccharides as an alternative to antibiotics to maintain growth performance and health in broilers.
Data Availability Statement
The datasets generated for this study can be found in the NCBI- SAMN2589823.
Ethics Statement
The animal study was reviewed and approved by Ethics of Animal Experiments of Hunan Agriculture University.
Author Contributions
LC, YD, ZS, HZ, and XH designed the experiment and revised the manuscript. LC and YW carried out the animal trials, sample analysis, did some data analysis work, and edited the manuscript. LC, YW, ZS, and XH are responsible for the integrity of the work as a whole. All authors reviewed and approved the final manuscript.
Funding
This study was supported by the Guangxi for Research Bases and Talents (Guike AD20238092), the China Agriculture Research System of MOF and MARA (CARS-41), and the Scientific and Technical Talents in Hunan Province (2020TJ-Q02).
Conflict of Interest
FL was employed by Guangxi Fufeng Agriculture and Animal Husbandry Group Co., Ltd.
The remaining authors declare that the research was conducted in the absence of any commercial or financial relationships that could be construed as a potential conflict of interest.
Publisher's Note
All claims expressed in this article are solely those of the authors and do not necessarily represent those of their affiliated organizations, or those of the publisher, the editors and the reviewers. Any product that may be evaluated in this article, or claim that may be made by its manufacturer, is not guaranteed or endorsed by the publisher.
Supplementary Material
The Supplementary Material for this article can be found online at: https://www.frontiersin.org/articles/10.3389/fvets.2022.852545/full#supplementary-material
References
1. Ren Z, Guo C, Yu S, Zhu L, Wang Y, Hu H, et al. Progress in mycotoxins affecting intestinal mucosal barrier function. Int J Mol Sci. (2019) 20:2777–91. doi: 10.3390/ijms20112777
2. Piontek J, Krug SM, Protze J, Krause G, Fromm M. Molecular architecture and assembly of the tight junction backbone. Biochimica et Biophysica Acta (BBA) Biomembranes. (2020) 1862:4–18. doi: 10.1016/j.bbamem.2020.183279
3. Suzuki T. Regulation of the intestinal barrier by nutrients: the role of tight junctions. Anim Sci J. (2020) 91:1–12. doi: 10.1111/asj.13357
4. Paone P, Cani PD. Mucus barrier, mucins and gut microbiota: the expected slimy partners? Gut. (2020) 69:2232–43. doi: 10.1136/gutjnl-2020-322260
5. Javanshir N, Hosseini G, Sadeghi M, Esmaeili R, Satarikia F, Ahmadian G, et al. Evaluation of the function of probiotics, emphasizing the role of their binding to the intestinal epithelium in the stability and their effects on the immune system. Biol Proced Online. (2021) 23:1–17. doi: 10.1186/s12575-021-00160-w
6. Low CX, Tan LT, Ab MN, Pusparajah P, Goh BH, Chan KG, et al. Unveiling the impact of antibiotics and alternative methods for animal husbandry: a review. Antibiotics. (2021) 10:578–95. doi: 10.3390/antibiotics10050578
7. Linklater DP, Baulin VA, Juodkazis S, Crawford RJ, Stoodley P, Ivanova EP. Mechano-bactericidal actions of nanostructured surfaces. Nat Rev Microbiol. (2021) 19:8–22. doi: 10.1038/s41579-020-0414-z
8. Lima T, Domingues S, Da SG. Manure as a potential hotspot for antibiotic resistance dissemination by horizontal gene transfer events. Vet Sci. (2020) 7:110–31. doi: 10.3390/vetsci7030110
9. Stokniene J, Powell LC, Aarstad OA, Aachmann FL, Rye PD, Hill KE, et al. Bi-Functional alginate oligosaccharide-polymyxin conjugates for improved treatment of multidrug-resistant gram-negative bacterial infections. Pharmaceutics. (2020) 12:1080-101. doi: 10.3390/pharmaceutics12111080
10. Camacho F, Macedo A, Malcata F. Potential industrial applications and commercialization of microalgae in the functional food and feed industries: a short review. Mar Drugs. (2019) 17:312–37. doi: 10.3390/md17060312
11. Maochen X, Qi C, Yu W, Han X, Jiarui Z, Qing Z, et al. Advances in research on the bioactivity of alginate oligosaccharides. Mar Drugs. (2020) 18:144–70. doi: 10.3390/md18030144
12. Liu JB, Cao SC, Liu J, Xie YN, Zhang HF. Effect of probiotics and xylo-oligosaccharide supplementation on nutrient digestibility, intestinal health and noxious gas emission in weanling pigs. Asian-Australas J Anim Sci. (2018) 31:1660–9. doi: 10.5713/ajas.17.0908
13. Al-Khalaifa H, Al-Nasser A, Al-Surayee T, Al-Kandari S, Al-Enzi N, Al-Sharrah T Ragheb G, et al. Effect of dietary probiotics and prebiotics on the performance of broiler chickens. Poultry Sci. (2020) 100:1329–44. doi: 10.3382/ps/pez282
14. Thitaram SN, Chung CH, Day DF, Hinton A, Bailey JS, Siragusa GR. Isomaltooligosaccharide increases cecal bifidobacterium population in young broiler chickens. Poult Sci. (2005) 84:998–1003. doi: 10.1093/ps/84.7.998
15. Zhang WF, Li DF, Lu WQ, Yi GF. Effects of isomalto-oligosaccharides on broiler performance and intestinal microflora. Poult Sci. (2003) 82:657–63. doi: 10.1093/ps/82.4.657
16. Hua J, He X, Yang ZJ, Zhang SP, Yang WY, Liu L. The effect of raffinose on growth performance and ability of antioxidation in broilers. J Beijing Univ Agric. (2010) 25:15–17. doi: 10.13473/j.cnki.issn.1002-3186.2010.04.016
17. Khambualai O, Yamauchi K-e, Tangtaweewipat S, Cheva-Isarakul B. Effects of dietary chitosan diets on growth performance in broiler chickens. J poult sci. (2008) 45:206–9. doi: 10.2141/jpsa.45.206
18. NRC. Nutrient Requirements of Poultry. 9th Ed. Washington, DC: National Academy of Sciences Press (1994).
19. Xie Q, Xie K, Yi J, Song Z, Zhang H, He X. The effects of magnolol supplementation on growth performance, meat quality, oxidative capacity, and intestinal microbiota in broilers. Poult Sci. (2022) 101:101722–37. doi: 10.1016/j.psj.2022.101722
20. Gallina A, Fiorese E, Pastore P, Magno F. Identification and semi-quantitative determination of gum Arabic in wines by GC-MS and size exclusion chromatography. Ann Chim. (2004) 94:177–84. doi: 10.1002/adic.200490021
21. Zhang K, Delgado-Baquerizo M, Zhu Y-G, Chu H. Space is more important than season when shaping soil microbial communities at a large spatial scale. Msystems. (2020) 5:e00783–19. doi: 10.1128/mSystems.00783-19
22. Colwell RK, Coddington JA. Estimating Terrestrial Biodiversity through Extrapolation. Philos Trans R Soc Lond A. (1994) 345:101–18. doi: 10.1098/rstb.1994.0091
23. Cole JR, Wang Q, Cardenas E, Fish J, Chai B, Farris JR, et al. The Ribosomal Database Project: improved alignments and new tools for rRNA analysis. Nucleic Acids Res. (2009) 37:141–5. doi: 10.1093/nar/gkn879
24. Rastall RA. Functional oligosaccharides: application and manufacture. Annu Rev Food Sci Technol. (2010) 1:305–39. doi: 10.1146/annurev.food.080708.100746
25. Cao BH, Karasawa Y, Guo YM. Effects of green tea polyphenols and fructo-oligosaccharides in semi-purified diets on broilers' performance and caecal microflora and their metabolites. Asian Austral J Anim. (2005) 18:85–89. doi: 10.5713/ajas.2005.85
26. Sharma AN, Kumar S, Tyagi AK. Effects of mannan-oligosaccharides and Lactobacillus acidophilus supplementation on growth performance, nutrient utilization and faecal characteristics in Murrah buffalo calves. J Anim Physiol Anim Nutr (Berl). (2018) 102:679–89. doi: 10.1111/jpn.12878
27. Rezaei S, Faseleh JM, Liang JB, Zulkifli I, Farjam AS, Laudadio V, et al. Effect of oligosaccharides extract from palm kernel expeller on growth performance, gut microbiota and immune response in broiler chickens. Poult Sci. (2015) 94:2414–20. doi: 10.3382/ps/pev216
28. Zhou TX, Chen YJ, Yoo JS, Huang Y, Lee JH, Jang HD, et al. Effects of chitooligosaccharide supplementation on performance, blood characteristics, relative organ weight, and meat quality in broiler chickens. Poultry Sci. (2009) 88:593–600. doi: 10.3382/ps.2008-00285
29. Liu W, Li X, Zhao Z, Pi X, Meng Y, Fei D, et al. Effect of chitooligosaccharides on human gut microbiota and antiglycation. Carbohydr Polym. (2020) 242:116413–422. doi: 10.1016/j.carbpol.2020.116413
30. Wu SG, Gao CQ, Zhang HJ, et al. Effect of dietary isomalto-oligosaccharide on performance, caecal microflora and immune function of laying hens. Chin J Anim Nutr. (2011) 23:1560–8. doi: 10.3969/j.issn.1006-267x.2011.09.015
31. Bednarczyk M, Stadnicka K, Kozlowska I, Abiuso C, Tavaniello S, Dankowiakowska A, et al. Influence of different prebiotics and mode of their administration on broiler chicken performance. Animal. (2016) 10:1271–9. doi: 10.1017/S1751731116000173
32. Zhang B, Chen G, Zhang H, Lan J, Yang C. Effects of rhamnolipids on growth performance and intestinal health parameters in Linnan yellow broilers. Poult Sci. (2021) 100:810–9. doi: 10.1016/j.psj.2020.10.041
33. Bian X, Wallstrom G, Davis A, Wang J, Park J, Throop A, et al. Immunoproteomic profiling of antiviral antibodies in new-onset type 1 diabetes using protein arrays. Diabetes. (2016) 65:285–96. doi: 10.2337/db15-0179
34. Min YN, Yang HL, Xu YX, Gao YP. Effects of dietary supplementation of synbiotics on growth performance, intestinal morphology, sIgA content and antioxidant capacities of broilers. J Anim Physiol an N. (2016) 100:1073–80. doi: 10.1111/jpn.12479
35. Wang S, Zhu H, Lu C, Kang Z, Luo Y, Feng L, et al. Fermented milk supplemented with probiotics and prebiotics can effectively alter the intestinal microbiota and immunity of host animals. J Dairy Sci. (2012) 95:4813–22. doi: 10.3168/jds.2012-5426
36. Mizubuchi H, Yajima T, Aoi N, Tomita T, Yoshikai Y. Isomalto-oligosaccharides polarize Th1-like responses in intestinal and systemic immunity in mice. J Nutr. (2005) 135:2857–61. doi: 10.1093/jn/135.12.2857
37. Deng X, Li X, Liu P, Yuan S, Zang J, Li S, et al. Effect of Chito-oligosaccharide Supplementation on Immunity in Broiler Chickens. Asian Austral J Anim. (2008) 21:1651–8. doi: 10.5713/ajas.2008.80056
38. Shenghe L, Erhui J, Enmei Q, Guozhong W, Kui L. Chitooligosaccharide promotes immune organ development in broiler chickens and reduces serum lipid levels. Histol Histopathol. (2017) 32:951–61. doi: 10.14670/HH-11-860
39. Berrocoso JD, Kida R, Singh AK, Kim YS, Jha R. Effect of in ovo injection of raffinose on growth performance and gut health parameters of broiler chicken. Poult Sci. (2017) 96:1573–80. doi: 10.3382/ps/pew430
40. Wu Y, Pan L, Shang QH, Ma XK, Long SF, Xu YT, et al. Effects of isomalto-oligosaccharides as potential prebiotics on performance, immune function and gut microbiota in weaned pigs. Anim Feed Sci Tech. (2017) 230:126–35. doi: 10.1016/j.anifeedsci.2017.05.013
41. Teng P, Kim WK. Review: roles of prebiotics in intestinal ecosystem of broilers. Front Vet Sci. (2018) 245:1–18. doi: 10.3389/fvets.2018.00245
42. Chi X, Ma X, Li Z, Zhang Y, Wang Y, Yuan L, et al. Protective effect of Epigallocatechin-3-Gallate in hydrogen Peroxide-Induced oxidative damage in chicken lymphocytes. Oxid Med Cell Longev. (2020) 2020:1–15. doi: 10.1155/2020/7386239
43. Li W, Zhang X, He Z, Chen Y, Li Z, Meng T, et al. In vitro and in vivo antioxidant activity of eucalyptus leaf polyphenols extract and its effect on chicken meat quality and cecum microbiota. Food Res Int. (2020) 136:109302–344. doi: 10.1016/j.foodres.2020.109302
44. Pengxia L, Xuan Z, Huali H, Ya S, Yuning W, Yancun Z. High carbon dioxide and low oxygen storage effects on reactive oxygen species metabolism in Pleurotus eryngii. Postharvest Biol Tec. (2013) 85:141–146. doi: 10.1016/j.postharvbio.2013.05.006
45. Liu WC, Guo Y, Zhao ZH, Jha R, Balasubramanian B. Algae-derived polysaccharides promote growth performance by improving antioxidant capacity and intestinal barrier function in broiler chickens. Front Vet Sci. (2020) 7:601336–346. doi: 10.3389/fvets.2020.601336
46. Gu XL, Li H, Song ZH, Ding YN, He X, Fan ZY. Effects of isomaltooligosaccharide and Bacillus supplementation on sow performance, serum metabolites, and serum and placental oxidative status. Anim Reprod Sci. (2019) 207:52–60. doi: 10.1016/j.anireprosci.2019.05.015
47. Hao W, Li K, Ma Y, Li R, Xing R, Yu H, et al. Preparation and antioxidant activity of chitosan dimers with different sequences. Mar Drugs. (2021) 19:366–81. doi: 10.3390/md19070366
48. Riasi A, Mahdavi AH, Bayat E. Effect of different levels of raw and heated grass pea seed (Lathyrus sativus) on nutrient digestibility, intestinal villus morphology and growth performance of broiler chicks. J Anim Physiol Anim Nutr (Berl). (2015) 99:924–31. doi: 10.1111/jpn.12319
49. Walton KD, Whidden M, Kolterud A, Shoffner SK, Czerwinski MJ, Kushwaha J, et al. Villification in the mouse: Bmp signals control intestinal villus patterning. Development. (2016) 143:427–36. doi: 10.1242/dev.130112
50. Macfarlane GT, Macfarlane S. Bacteria, colonic fermentation, and gastrointestinal health. J Aoac Int. (2012) 95:50–60. doi: 10.5740/jaoacint.SGE_Macfarlane
51. Gill PA, van Zelm MC, Muir JG, Gibson PR. Review article: short chain fatty acids as potential therapeutic agents in human gastrointestinal and inflammatory disorders. Aliment Pharmacol Ther. (2018) 48:15–34. doi: 10.1111/apt.14689
52. Yang W, Yu T, Huang X, Bilotta AJ, Xu L, Lu Y, et al. Intestinal microbiota-derived short-chain fatty acids regulation of immune cell IL-22 production and gut immunity. Nat Commun. (2020) 11:1–18. doi: 10.1038/s41467-020-18262-6
53. Lindsay EC, Metcalfe NB, Llewellyn MS. The potential role of the gut microbiota in shaping host energetics and metabolic rate. J Anim Ecol. (2020) 89:2415–26. doi: 10.1111/1365-2656.13327
54. Yue L, Sun D, Mahmood KI, Liu X, Jiang Q, Xia W. Cinnamyl alcohol modified chitosan oligosaccharide for enhancing antimicrobial activity. Food Chem. (2020) 309:125513–37. doi: 10.1016/j.foodchem.2019.125513
55. Muanprasat C, Wongkrasant P, Satitsri S, Moonwiriyakit A, Pongkorpsakol P, Mattaveewong T, et al. Activation of AMPK by chitosan oligosaccharide in intestinal epithelial cells: mechanism of action and potential applications in intestinal disorders. Biochem Pharmacol. (2015) 96:225–36. doi: 10.1016/j.bcp.2015.05.016
56. Simunek J, Brandysová V, Koppová I, Simunek J. The antimicrobial action of chitosan, low molar mass chitosan, and chitooligosaccharides on human colonic bacteria. Folia Microbiol. (2012) 57:341–5. doi: 10.1007/s12223-012-0138-1
57. Wang J, Ji H, Wang S, Liu H, Zhang W, Zhang D, et al. Probiotic lactobacillus plantarum promotes intestinal barrier function by strengthening the epithelium and modulating gut microbiota. Front Microbiol. (2018) 9:1953–87. doi: 10.3389/fmicb.2018.01953
58. Johnson TJ, Youmans BP, Noll S, Cardona C, Evans NP, Karnezos TP, et al. A consistent and predictable commercial broiler chicken bacterial microbiota in Antibiotic-Free production displays strong correlations with performance. Appl Environ Microbiol. (2018) 84:e00362–18. doi: 10.1128/AEM.00362-18
59. Kalavathy R, Abdullah N, Jalaludin S, Ho YW. Effects of lactobacillus cultures on growth performance, abdominal fat deposition, serum lipids and weight of organs of broiler chickens. Br Poult Sci. (2003) 44:139–44. doi: 10.1080/0007166031000085445
60. Thompson CL, Vier R, Mikaelyan A, Wienemann T, Brune A. 'Candidatus Arthromitus' revised:Segmented filamentous bacteria in arthropod guts are members of Lachnospiraceae. Environ Microbiol. (2012) 14:1454–65. doi: 10.1111/j.1462-2920.2012.02731.x
61. Del-Pozo J, Crumlish M, Ferguson HW, Green DM, Turnbull JF. A prospective longitudinal study of “Candidatus arthromitus”-associated rainbow trout gastroenteritis in the UK. Prev Vet Med. (2010) 94:289–300. doi: 10.1016/j.prevetmed.2010.02.001
62. Lecuyer E, Rakotobe S, Lengline-Garnier H, Lebreton C, Picard M, Juste C, et al. Segmented filamentous bacterium uses secondary and tertiary lymphoid tissues to induce gut IgA and specific T helper 17 cell responses. Immunity. (2014) 40:608–20. doi: 10.1016/j.immuni.2014.03.009
Keywords: isomalto-oligosaccharide, raffinose oligosaccharide, chitooligosaccharide, gut microbiota, broiler
Citation: Chang L, Ding Y, Wang Y, Song Z, Li F, He X and Zhang H (2022) Effects of Different Oligosaccharides on Growth Performance and Intestinal Function in Broilers. Front. Vet. Sci. 9:852545. doi: 10.3389/fvets.2022.852545
Received: 11 January 2022; Accepted: 01 March 2022;
Published: 01 April 2022.
Edited by:
Monika Pogány Simonová, Slovak Academy of Sciences, SlovakiaReviewed by:
Birendra Mishra, University of Hawaii at Manoa, United StatesBaikui Wang, Zhejiang University, China
Copyright © 2022 Chang, Ding, Wang, Song, Li, He and Zhang. This is an open-access article distributed under the terms of the Creative Commons Attribution License (CC BY). The use, distribution or reproduction in other forums is permitted, provided the original author(s) and the copyright owner(s) are credited and that the original publication in this journal is cited, in accordance with accepted academic practice. No use, distribution or reproduction is permitted which does not comply with these terms.
*Correspondence: Xi He, hexi111@126.com; Haihan Zhang, zhhous@163.com
†These authors have contributed equally to this work