- 1Laboratory of Climate Change and Livestock Production, Department of Animal Nutrition, Faculty of Veterinary Medicine and Animal Science, University of Yucatan, Mérida, Yucatan, Mexico
- 2National Institute of Research in Forestry, Agriculture and Livestock-INIFAP, Experimental Field Valle del Guadiana, Durango, Mexico
- 3National Center for Disciplinary Research in Physiology and Animal Breeding, National Institute for Forestry, Agriculture and Livestock Research-INIFAP, Queretaro, Mexico
- 4Institute of Silviculture and Wood Industry, National Council of Science and Technology—Durango State Juarez University, Durango, Mexico
- 5National Technology of Mexico, Technological Institute of Valle del Guadiana, Durango, Mexico
- 6Tropical Forage Program—International Center for Tropical Agriculture (CIAT), Palmira, Colombia
- 7Food Technology Unit, Centro de Investigación y Asistencia en Tecnología y Diseño del Estado de Jalisco, Jalisco, Mexico
- 8Department of Animal Nutrition, National Council of Science and Technology—Durango State Juarez University, Durango, Mexico
- 9Department of Small Ruminant Nutrition, Faculty of Veterinary Medicine and Animal Science, Durango State Juarez University, Durango, Mexico
In this study, the effects of orange essential oil (OEO) on the rumen fermentation, nutrient utilization, and methane (CH4) emissions of beef heifers fed a diet of bermudagrass (Cynodon dactylon) were examined. In addition, in vitro and in situ experiments were conducted. The in vitro experiment consisted of three treatments: control (CTL, no OEO), OEO1 (0.25% OEO), and OEO2 (0.5% OEO). The forage to concentrate ratio was 70:30 (dry matter [DM] basis) in all treatments. No changes in pH, proportions of volatile fatty acids, and the acetate:propionate ratio were observed (P > 0.05). The addition of 0.25% OEO resulted in a reduction in CH4 production (mL/g) relative to the control (P < 0.05). In the in situ experiment, 5 g of total mixed ration (CTL, OEO1, and OEO2) were incubated for 6, 12, 24, 48, and 72 h. Potential and effective degradability were not affected by OEO supplementation (P > 0.05). In the in vivo study, six crossbred beef heifers (Bos indicus × Bos taurus), fitted with rumen cannulas, were assigned to three different treatments: no additive (CTL), 0.25% OEO (OEO1), and 0.5% OEO (OEO2) in a replicated 3 × 3 Latin square (21-day periods). Heifers were fed at 2.8% body weight. In vivo CH4 production was measured in open-circuit respiration chambers. Reductions in gross energy consumption, apparent total tract digestibility, and rumen valerate concentration were observed for OEO2 compared to the control (P < 0.05). Additionally, decreases in CH4 emissions (g/day; P < 0.05) and CH4 (MJ gross energy intake/day; P < 0.05) were observed in response to supplementation of 0.5% OEO as compared to the CTL treatment. Thus, supplementation of 0.5% OEO reduced CH4 emissions (g/day) by 12% without impacting the DM intake of heifers fed bermudagrass hay as a basal ration.
Introduction
Greenhouse gas (GHG) emissions from livestock production account for 10–12% of global GHG emissions and are expected to increase by 40% by 2050 (1). Methane (CH4) emitted by ruminants is a substantial GHG source. Given that CH4 is a short-lived GHG, reducing its emissions is important for mitigating the adverse effects of climate change (2, 3). The development of effective CH4 mitigation strategies that do not adversely affect animal performance is important, especially in tropical countries where livestock plays an important role in ensuring food security and contributes to poverty reduction. Ideally, GHG mitigation strategies should reduce the environmental impact of livestock farming, enhance the productivity and profitability of production systems, and meet the needs of consumers (4–6). Over 200,000 secondary plant metabolites have been identified as potential modulators of the rumen microbiome, and some of these metabolites can potentially enhance animal production efficiency and reduce energy losses in the form of enteric CH4 (7). Essential oils (EOs) are complex mixtures of volatile or aromatic chemicals, and their main constituents are terpenoids (6). Essential oils are present in various parts of plants—such as the roots, peels, seeds, buds, fruit, leaves, twigs, wood, and bark—and are frequently extracted through steam distillation (6–9). Essential oils are generally recognized as safe for mammals and exhibit antimicrobial, antiparasitic, and antifungal activity (5, 10, 11). Furthermore, some EOs possess antioxidant properties and can induce changes in the rumen microbiome, resulting in a reduction in CH4, and increases in propionate or rumen bypass protein (12). Although numerous EOs have been studied in vitro, only a few EOs have been studied in vivo (13). Citrus sinensis L. is one of the world's most important fruit crops. In addition to its use as a food source for humans, its by-products, such as peel, seeds, and pulp, account for 50% of the output of orange fruit production (14). Sweet orange essential oil (OEO) is also an important orange by-product and contains numerous different compounds (between 20 and 60) that are mainly present in the flavedo or exocarp.
A large number of compounds contained in OEO, such as monoterpenes (limonene as the main component), sesquiterpenes, and oxygenated derivatives are volatile but also a small portion of non-volatile compounds are contained in OEO (11, 15). Although OEO can reduce CH4 production in vitro (16), determining whether it has the same effect when used as a feed additive in vivo is critically important (16, 17). Wu (17) found that the use of citrus essential oil (D-limonene, 80.13%) in sheep has no adverse effects on body weight (BW), dry matter intake (DMI), average daily gain, and total-tract nutrient digestibility, and that it has intermittent anti-methanogenic effects. Kotsampasi (11) reported that milk yield, milk fat yield, and feed efficiency of ewes were all enhanced when 450 mg/kg OEO (d-limonene, 95.17 g/100 g) was added to a ration with a 57:43 concentrate-to-forage ratio. The replacement of 20% extruded corn with citrus pulp containing bioactive compounds, such as limonene, reduced CH4 production in dairy sheep (18). In tropical regions, forages are typically low in crude protein but high in neutral detergent fiber (NDF). Thus, the targeted supplementation of concentrates or the use of feed additives in roughage-based diets could enhance animal performance and promote rural development in the tropics (19). The aim of this study was to evaluate the effect of dietary OEO supplementation on rumen fermentation, nutrient utilization, and enteric CH4 production in beef heifers, fed tropical bermudagrass hay as basal ration. In addition, we examined the effect of OEO on total gas and CH4 production, and fermentation patterns in vitro, as well as potential and effective degradability in situ.
Materials and methods
The experimental procedures were approved by the Bioethics Committee at the University of Yucatan (approval no. CB-CCBA-D-2019-01). The research was carried out at the University of Yucatan's Faculty of Veterinary Medicine and Animal Science's Laboratory of Climate Change and Livestock Production, as well as at the Durango State Juarez University's Faculty of Veterinary Medicine and Animal Science. The experimental ration administered in the in vivo experiment was also used as a substrate in the in vitro and in situ experiments.
Volatile characterization of OEO
The same batch of OEO was used throughout the study. The OEO was provided by CITROFRUT, S.A. de C.V., Monterrey, NL, Mexico. The composition of the OEO was analyzed using a GC/MSD system (GC 7890B and MSD 5977A, Agilent Technologies, Santa Clara, CA, USA). Separation was achieved using an HP-INNOWAX capillary column (60 m × 0.25 mm ID × 0.25-μm film thickness). The temperatures of the injector and detector were 230 and 260°C, respectively. The initial oven temperature was 60°C. The temperature was increased to 250°C at a rate of 4°C/min and held for 25 min. The carrier gas was helium at a flow rate of 0.8 mL/min. The injection volume was 0.3 μL using a split ratio of 1:200, with a 70 eV ionization potential. The mass spectrometer was operated in SCAN mode, with a scanning range of m/z 30–480. Volatile components of the OEO were preliminarily identified by matching their mass spectra with those recorded in the NIST14L MS Data Library, as well as by elution order based on published retention index datasets. Identities were also confirmed by the injection of pure compounds obtained from Sigma-Aldrich, USA (95% minimum purity) when possible under the same analytical conditions. Volatile compounds were quantified using the same chromatographic system with flame ionization detection. Chromatographic conditions were the same as those in the GC–MS analysis, with air and hydrogen flows of 400 and 40 mL/min, respectively. Substances were quantified by averaging the area percentage of each component after a duplicate analysis of OEO.
In vitro experiment
In vitro fermentation patterns
Six heifers (Bos indicus × Bos taurus) fitted with a permanent rumen cannula (10 cm i.d., Bar Diamond Inc., Parma, ID, USA) were adapted to the three different diets (Table 1) for 15 days, before rumen liquor was collected. The experiment was arranged as a completely randomized design with three treatments: (1) Control (CTL, no additive); (2) OEO1 at 0.25% incubated DM (IDM); and (3) OEO2 at 0.50% IDM. The substrate of each experimental treatment (1 g DM) was incubated at 39°C in ANKOM glass incubators (ANKOM Technology, USA) with 120 mL of ruminal liquor pooled from each pair of heifers and buffer solution in a 2:1 ratio. The modules were opened after 24 h of incubation, and the pH of the incubation fluid was measured immediately and evaluated using a portable pH meter (Hannah® Instruments, Woonsocket, RI, USA). Aliquots of the incubation fluid (10 mL) were collected and mixed with 2.5 mL of metaphosphoric acid [25% weight per volume (w/v)] following a previously described method (20). The volatile fatty acid (VFA) content of the samples was quantified using a gas chromatography system (7820A GC system Agilent Technologies Inc, Santa Clara, CA, USA) equipped with a flame ionization detector. The samples were analyzed in triplicates.
In vitro gas and CH4 production
The in vitro incubations were carried out using the ANKOM RF Gas Production System (ANKOM Technology, Macedon, NY, USA). Samples (ca. 1 g DM) from each treatment (CTL, OEO1, and OEO2) were incubated in triplicates in ANKOM glass modules equipped with a pressure transducer. This batch culture procedure was repeated in two consecutive runs on two different days (n = 6 per treatment). Fermentations were carried out according to the manufacturer's instructions by incubating the sample with a 2:1 mixture of buffer and ruminal inoculum solution. Four cows (Bos indicus × Bos taurus) fitted with a permanent rumen cannula (10 cm i.d., Bar Diamond Inc., Parma, ID, USA) were adapted to the diet for 15 days (70:30 forage:concentrate ratio), and rumen liquor was withdrawn from these cows in the morning before they were fed (between 0800 and 0830 h). The ruminal inoculum was collected as a pooled sample and immediately transported in thermos bottles to the laboratory, where it was mixed, flushed with CO2, and filtered with four layers of cheesecloth (21). Buffer solutions were prepared following the instructions provided by Ankom (ANKOM Technology, Macedon, NY, USA). The pressure was measured every hour during the 96 h incubation period. The kinetics of in vitro gas production were determined by fitting data to the Gompertz function (22) using the following equation:
where GP = gas production at time t (mL); Gmax= maximum gas production (mL); k = constant gas production rate (h−1); and A = latency time before gas production begins (h). To measure the proportions of CH4 and CO2, the pressure relief valve of the modules was opened for 2 s as per the manufacturer's instructions. The expelled gas was sent through a tube to a portable gas analyzer (GEMTM5000, LANDTEC, USA) as per a previously described method (23).
In situ experiment
Six rumen-cannulated heifers were used to estimate in situ rumen DM disappearance. Five grams DM of TMR with or without OEO (Table 1) were ground and screened using a 1-mm sieve before being placed in nylon bags (10 × 20 cm, 50 × 10-micron average pore size; R1020 Ankom Technology, Macedon, NY, USA) at each time point. Duplicate samples were incubated in the ventral sac of the rumen. Each heifer (n = 6) was considered a replicate. The bags were incubated in the rumen for 0, 12, 24, 48, 72, and 96 h in reverse order to ensure that all bags were removed at the same time. After incubation, the nylon bags were manually cleaned in tap water, dried at 60°C for 48 h, and then weighed for DM determination. The exponential model proposed by Ørskov and McDonald (24) was used to estimate in situ degradation curves (DM) as follows:
where a is the soluble and rapidly degradable fraction; b is an insoluble but potentially degradable fraction; c is the fractional disappearance rate constant at which b is degraded, and P is the proportion (%) of dry matter degraded at time t (incubation h). The sum of a + b was used to obtain the potential degradability (PD, %). The following equation was used to estimate effective degradability (ED):
where a, b, and c are the same values indicated in the aforementioned equation, and k is the predicted passage rate for ruminants fed at low output levels, which was 0.05 h−1 (25).
In vivo experiment
Animals, experimental design, and treatments
Six crossbred heifers (Bos taurus × Bos indicus) with average body weight (BW) of 383 ± 21.6 kg (mean ± SD) were used in this experiment. The heifers were randomly assigned to three treatments (no additive [CTL], 0.25% OEO [OEO1], and 0.5% OEO [OEO2]) in a replicated 3 × 3 (n = 6) Latin square design. Each period lasted for 21 days. The acclimation period was between 1 and 14 days. Measurements were taken between day 15 and day 21. The heifers were acclimated to indirect open-circuit respiration chambers for CH4 measurements before the beginning of the experiment.
Feed intake and apparent total tract digestibility
Heifers were provided unlimited access to water and fed the TMR (Table 1) at 2.8% of their body weight. Heifers were fed once daily at 0800 h. The TMR was formulated to satisfy the energy and protein requirements for maintenance and growth (25). The difference between the amount of feed supplied and that rejected the next day was used to compute daily feed intake. Between day 17 and day 21 of each period, the total amount of feces was collected in metabolic crates to determine the apparent total tract digestibility of nutrients. A sub-sample of 10% of the total feces was taken after homogenization. The fecal samples were dried in a forced-air oven (55°C for 72 h) and ground through a 1-mm screen (Willey mill, Arthur H. Thomas Co., Philadelphia, PA, USA) before chemical analysis. To avoid contamination of the feces with urine, each of the metabolic cages had a metal grid that allows urine and feces to pass through, but the heifers stayed dry and comfortable. Below the first grid, two steel mesh grills with 11.5-mm diameter holes collected feces and permitted the passage of urine into a container.
Rumen fermentation parameters
Five hours after feeding on day 18 of each period, 8 mL of ruminal liquor was collected using a syringe connected to a stainless-steel tube through the rumen cannula (20-mm internal diameter), to which 2 mL of 25% of metaphosphoric acid was added. The samples were then stored at −20°C before the determination of VFA content by gas chromatography (7820A GC system Agilent Technologies Inc, Santa Clara, CA, USA). An additional sample (50 mL) of rumen liquor was taken to measure the pH using a portable pH meter (Hannah® Instruments, Woonsocket, RI, USA).
Methane production
Methane measurements were carried out in two open-circuit respiration chambers with an internal volume of 9.97 m3. Each chamber was equipped with a steel feeder (90 cm length, 70 cm height, and 50 cm width), automatic bowl-type waterer (20 cm diameter), fan (30 cm diameter), air conditioner (12,000-BTU), and dehumidifier. The temperature and relative humidity were maintained at 23 ± 1°C and 55 ± 10%, respectively. A polyvinyl chloride tube and a half-turn valve (75-mm diameter) positioned in the upper-right corner of the front wall regulated the supply of ambient air and air pressure within the chamber (−275 Pa). Ambient air was drawn from the chambers by mass flowmeters (Flowkit; Sable Systems International; Las Vegas, NV, USA), adjusted to the animal's body weight (1 L min/kg BW), and sent to the sample device using a flexible industrial pipe (50-mm diameter). A CH4 analyzer that uses infrared light (MA-10 Sable Systems International®, Las Vegas, NV, USA) and respiration chambers were calibrated by infusing a known amount of pure CH4 (Praxair® Gases Industrial Inc., Monterrey, NL, Mexico; 99.997% purity), with recovery values ranging from 97 to 102%. Every morning before each run, the calibration was verified by zeroing the apparatus with pure N2 (Praxair®, State of Mexico, Mexico) and then releasing CH4 (1000 ppm, Praxair®, State of Mexico, Mexico) diluted in N2 until stable readings (plateau) were obtained after 5 min. The measurements were carried out for three consecutive days (day 19 to day 21 of each period), and chambers were cleaned in 1 h. Expe Data® software was used to extrapolate the data to a 24 h period (26). According to IPCC (27), GEI/day lost as CH4 was computed from its heat of combustion (CH4 = 55.65 MJ/kg).
Chemical analyses
The total feed provided, refused feed, and fecal samples were oven-dried for 72 h at 60°C, ground through a 1-mm screen (Wiley mill; A. H. Thomas, Philadelphia, PA, USA), and analyzed for DM (method 930.15), ash (method 923.03), and crude protein (CP) using a C/N-analyzer (CN-2000 series 3740, LECO® Corp., St. Joseph, MI, USA; AOAC, method number 992.15), as well as ether extract (EE, AOAC, method number 920.39). An adiabatic bomb calorimeter was used to determine gross energy (model 6400 Parr Instrument Company, Moline, IL, USA). The concentrations of NDF and acid detergent fiber (ADF) (AN 3805 ANKOM, ANKOM Technology, Wayne County, NY, USA) were determined following the method proposed by Van Soest et al. (28). Briefly, NDF and ADF were determined using ~0.5-g samples with fiber analysis bags (F57). Samples were treated with a neutral detergent solution and rinsed with a heat-stable amylase to remove the soluble non-structural carbohydrates from the matrix; the remaining compounds were cell wall compounds, such as cellulose, hemicellulose, and lignin. Thereafter, hemicellulose was removed from the matrix by making it soluble with an acid detergent solution. Cellulose and lignin remaining in the matrix were treated with sulfuric acid to remove cellulose using an ANKOM200 fiber analysis system and commercially available solutions (ANKOM Technology, Wayne County, NY, USA).
Statistical analyses
All data from the in vitro studies were analyzed using the GLM procedure in SAS (version 9.4; SAS Inst., Inc., Cary, NC, USA) for a completely randomized design. The model used was as follows:
where μ is the overall mean, Ti is the treatment effect, and e is the error term. Least-squares means tests were used to determine the standard error of the difference between means. Tukey tests were used to compare means. Polynomial contrasts were performed to evaluate the linear and quadratic effects of the treatments. Data from the in vivo experiment were subjected to analysis of variance for a replicated 3 × 3 Latin square design using the mixed procedure in the SAS® 9.4 Software (29). The statistical model was as follows:
where Y is the dependent variable, μ is the general mean, P is the effect of period, A is the random effect of animal, T is the effect of treatment, and E is the random residual error. Results were compared using least-squares means tests, and polynomial contrasts were used to assess the treatment effect.
Kinetics of degradation and potential rumen degradability in situ were obtained from the equation proposed by Ørskov and McDonald (24) for each treatment using the non-linear Marquardt procedure of SAS (version 9.4; SAS Inst., Inc., Cary, NC, USA), and the in situ degradation kinetic parameters were analyzed using the mixed procedure in SAS (version 9.4; SAS Inst., Inc.), in which treatment was a fixed effect and incubation replicate in the rumen was a random effect. The model used for the analysis was as follows:
where Y = the observation of the dependent variables ij; μ = the overall mean of Y; Fij= the effect of treatment (i = 3), R = the effect of incubation run as replicate (j =6 animals), and eij = the random error associated with the observation ij. Standard error of the difference among means was carried out using least-squares means tests.
Results
Volatile composition of OEO
The main volatile compounds identified in OEO are shown in Table 2. The compounds representing more than 99% of the total composition of OEO were D-limonene (78.84%), β-myrcene (6.55%), α-pinene (2.4%), linalool (1.61%), sabinene (1.17%), and β-phellandrene (1.08%).
In vitro experiment
In vitro fermentation patterns
The inclusion of OEO had no effect (P > 0.05) on in vitro pH, which ranged between 6.7 and 6.8. The proportions of acetate, propionate, isobutyrate, butyrate, isovalerate, and valerate were not altered by OEO supplementation (P > 0.05); the acetate:propionate ratio ranged from 1.9 to 2.0 (Table 3) and was also not affected by OEO supplementation.
Gas and methane production
No differences in Gmax (mL/g IDM) and GP24 (mL/g IDM) between the OEO treatments and the control were observed (P > 0.05; Table 4). However, A (h) and K (% h−1) were higher in the OEO1 and OEO2 treatments compared with the control (P < 0.05). The production of CH4 decreased by 41.7% (mL at 24 h), 41.6% (mL/g IDM), and 45.9% (mL/g degraded DM), respectively, when OEO was supplemented at 0.25% DM (P < 0.05). There was a quadratic relationship between the dose of OEO and CH4 production (ml/g IDM and ml/g of degraded DM; P < 0.05).
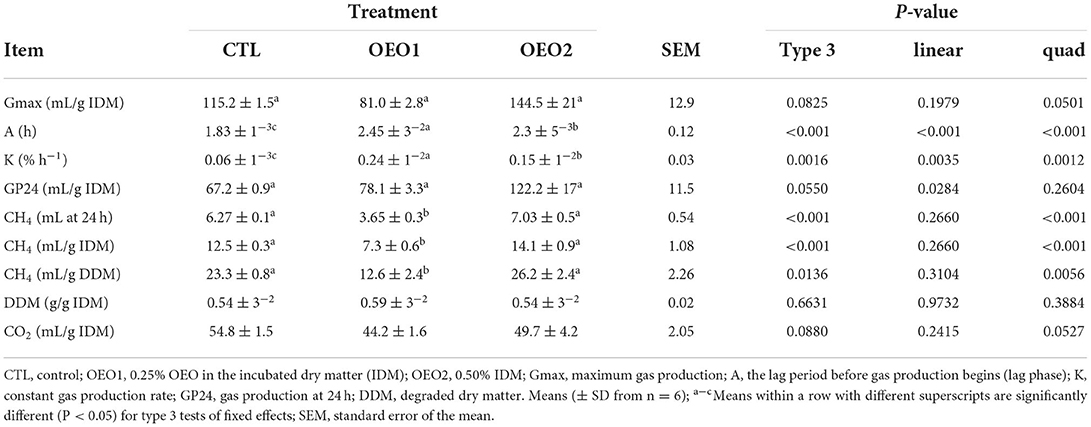
Table 4. Maximum in vitro gas production, lag phase, constant gas production rate, and in vitro CH4 production in response to supplementation with orange essential oil (OEO; n = 6).
In situ experiment
There were no changes in the parameters assessed in situ (Table 5), including potential and effective degradability in response to OEO supplementation (P > 0.05).
In vivo experiment
Feed intake and apparent total tract digestibility
Supplementation of OEO had no effect on DM, OM, CP, NDF, and ADF intake (P > 0.05; Table 6); however, there was a negative quadratic relationship between GE intake and the level of OEO supplementation (P < 0.05; Table 6). The apparent total tract digestibility of DM decreased quadratically (P < 0.05; Table 7) when 0.5% OEO was added. The apparent total tract digestibility of OM, CP, NDF, and ADF and digestible energy were not affected (P > 0.05) by OEO supplementation.
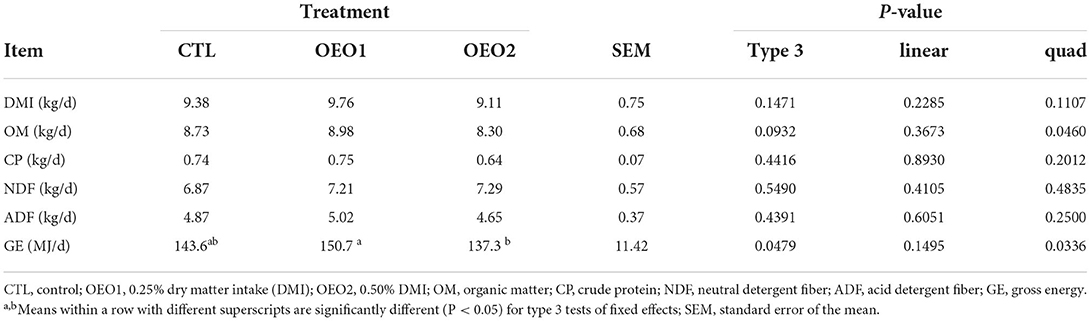
Table 6. Effect of orange essential oil (OEO) supplementation on the feed intake of heifers (n = 6).
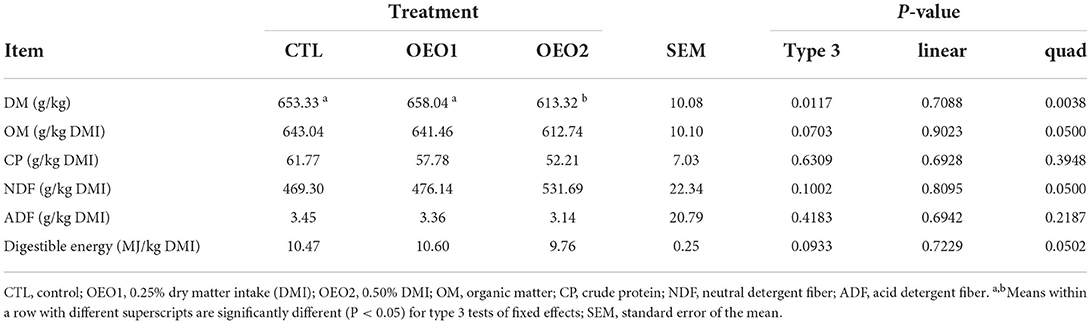
Table 7. Effect of orange essential oil (OEO) on the apparent total tract digestibility of heifers (n = 6).
Rumen fermentation parameters
Rumen pH, VFA, and the acetate:propionate ratio are shown in Table 8. Rumen pH was similar across treatments (P > 0.05) and ranged from 6.57 to 6.68. There was a quadratic response in acetate proportion when OEO was added (P < 0.05). The proportion of valerate decreased quadratically in response to OEO addition (P < 0.05). Feeding OEO1 resulted in an increase of valerate by 21% relative to the control, whereas valerate decreased by 14% in response to OEO2 compared to the control (P < 0.05). Supplementation of OEO had no effect on the C3:C2 ratio (P > 0.05).
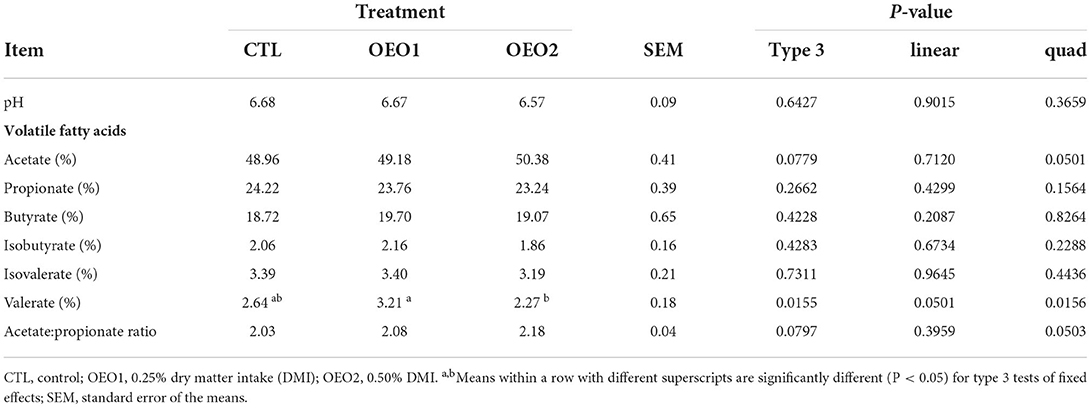
Table 8. Effect of orange essential oil (OEO) on rumen pH and volatile fatty acid (VFA) production in heifers (n = 6).
Methane production
When 0.5% OEO was fed, CH4 (g/day) was reduced by 12% as compared to control (P < 0.05; Table 9). The CH4 yield (g/kg DMI) decreased linearly with the level of OEO supplementation. No changes were observed in emissions per kilogram of fermented OM in the rumen (P > 0.05). There was a linear decrease in CH4 yield (% GE/day) in response to OEO addition. There was a quadratic decrease in energy loss in form of CH4 (MJ of GEI/day) in response to OEO2 supplementation (P < 0.05).
Discussion
Volatile composition of OEO
The composition of volatile compounds in OEO was consistent with that reported in previous studies (30–33); D-limonene, β-myrcene, and α-pinene were the most abundant volatile compounds. Variation in the concentrations of volatile compounds is associated with variation in species, geographical origin, climatic conditions, extraction technique, and fruit maturity (34).
In vitro experiment
In vitro fermentation patterns
Changes in rumen pH in our study were consistent with those observed by Castillejos et al. (35), who used the most abundant monocyclic monoterpene in OEO (limonene) in doses of 5–500 mg/L rumen liquor in a basal diet with 60:40 forage:concentrate ratio. However, our findings differ from those of Kamalak et al. (16), who found that increases in OEO supplementation (200, 400, 800, and 1,200 mg/L) increase rumen pH. In our experiment, VFA (% of total) was not significantly affected by OEO supplementation, suggesting that the doses evaluated did not affect rumen bacteria (OEO1: 2,500 mg/L and OEO2: 5,000 mg/L). Kamalak et al. (16), who used rumen fluid from sheep, reported that high doses of OEO (800 and 1,200 mg/L) reduce the production of VFA (mmol/L) and alter the molar proportion of VFA; specifically, high OEO doses increase the proportion of acetate and the acetate:propionate ratio. Castillejos et al. (36) found that EO supplementation of a diet containing 10% barley straw and 90% concentrate with thyme oil (Thymus vulgaris), savory oil (Satureja montana), and Lavandin oil (Lavandula hybrida) lowered the pH. Furthermore, they found that clover leaf oil (Eugenia caryophyllus) and oregano oil (Origanum vulgare) increased pH when supplied at 500 mg.
In vitro gas and methane production
Essential oils, which are typically obtained by steam distillation, are known to have antibacterial properties due to their ability to modify the permeability of cells (37, 38). A previous study (16) showed that OEO supplementation at doses ranging from 100 to 1,200 mg/L reduces CH4 production (mmol/L), which is consistent with the results of our study. Methane production was reduced by 41.7% (mL), 41.6% (mL/g incubated DM), and 45.9% (mL/g degraded DM) in response to OEO1. García-Rodríguez et al. (18) evaluated the effect of substituting extruded corn for dry citrus pulp (20%) and found that reductions in CH4 were associated with the antimicrobial effects of bioactive compounds such as terpenoids, limonene, and citral. However, this was not observed for the OEO2 treatment, as there were no differences in CH4 between OEO2 and the control. The quadratic response in CH4 production might stem from the fact that a higher concentration of OEO might have induced toxicity to the microbiota. This finding confirms that the effects of EO on ruminal microbiota are dose-dependent. An alternative explanation for the differences between the results of our study and the results of previous studies might be associated with differences in the concentrations of bioactive compounds, the substrates used (i.e., soybean meal vs. forage: concentrate:forage ratio 70:30), and the techniques used for quantification.
In situ experiment
Few studies have examined the effects of OEO on the in situ digestibility of DM. Previous studies of other EOs such as lemongrass oil and a mixture of garlic and ginger oil have shown that supplementation of these EOs (200 mg/kg) enhances the in situ DM digestibility of grass hay a TMR (39). Studies using different EOs reported inconsistent findings regarding the degradation of protein-rich substrates using dacron bags in the rumen. This might be due to differences in substrate composition (40). However, in this study, the level of OEO supplementation did not appear to affect in situ rumen degradation kinetics.
In vivo experiment
Feed intake and apparent digestibility
Increases in the level of OEO supplementation did not affect DMI as has been shown in a previous study that applied 200–1,200 ppm of OEO in a swamp grass:concentrate ratio 60:40 (41). A different study found that supplementation of 4.5 g of citrus extract per day did not improve the DMI of the dairy cows (42). Other EOs have been reported to increase feed intake (carvacrol, eugenol, thymol, coriander seed oil, geranyl acetate, and geraniol), but some studies have demonstrated that blends of thymol, eugenol, carvacrol, garlic, citral, and cinnamaldehyde can induce reductions in feed intake and digestibility (43, 44). This suggests that the effects of different EOs or their constituents on feed intake are variable and will depend on the type and dosage of EO (37). Other studies have suggested that the palatability of feed can be impacted by the addition of EO; however, other factors that also affect DMI—such as animal growth stage, body weight, or the specific properties of diets such as the fiber content and particle size—also require consideration (41, 45, 46). One possible explanation for the reduced rumen degradability associated with the OEO2 treatment might be the broad and non-specific antimicrobial capacity of OEO on rumen microorganisms. The use of 0.5% OEO reduced rumen microbial activity, which affected the fermentability, degradability, and CH4 emissions (47). This is consistent with findings of in vitro studies showing that 200 and 300 ppm of orange peel oil inhibits CH4 generation by more than 50% due to the low digestibility of DM and NDF, which was possibly associated with a microbial imbalance in the rumen (48). The discrepancies among studies might be caused by different types of EOs that were used in the respective studies, dosages tested, and differences in diet and host interactions (49).
Rumen fermentation parameters
In ruminants fed low-quality tropical grasses as a basal ration, the rate and extent of fermentation of OM in the rumen are usually reduced, which helps maintain pH within the physiological range. Some have suggested that EOs have stronger effects in animals fed high concentrations of grains as in intensive systems; given that bacteria that grow under low pH are favored under such conditions, limonene has been reported to reduce Fusobacterium necrophorum populations as well as the prevalence of liver abscesses (50, 51). The rumen pH was not affected by OEO supplementation (41), and it remained within the optimal range for fermentation (6.6 ± 0.5) (52). The above findings are also consistent with the results of other studies that have used a mixture (750 mg/day) of EOs (thymol, eugenol, vanillin, guaiacol, and limonene) (53). Afzalani et al. (41) found that the use of 200 and 400 ppm OEO in cattle increased the concentration of propionic acid in the rumen and affected the C3:C2 ratio. No changes in the rumen microbial catabolism of branched-chain amino acids were observed in response to OEO supplementation; the concentration of valerate increased with the level of OEO1 supplementation, which indicates that valerate acted as a sink for H2 and contributed to reductions in CH4 (54). Wu et al. (2018) suggested that EO can affect rumen fermentation by inhibiting microbial growth.
Methane production
Previous authors who supplemented EO and detected reductions in enteric CH4 suggested the following reasons for the observed reduction: (1) a reduction in hydrogen production (alternative sinks), (2) the direct inhibition of archaea, and (3) a disruption of the symbiosis between protozoa and archaea (55). Few studies of the effects of EO and OEO from Citrus sinensis have been conducted (2). Wu et al. (17) reported that citrus EO (limonene) had anti-methanogenic effects in Hu sheep, but this effect was not consistent among periods, suggesting that microbial adaptation can occur following short-term exposure to EO. Phytogenic feed additives, such as for example Agolin Ruminant (Agolin, Bière, Switzerland) or Mootral (Mootral SA, Rolle Switzerland), are often mixtures of different plant extracts containing EOs. The fact that commercial phytogenic additives are typically complex mixtures of different compounds, makes it challenging to identify the bioactive compounds responsible for beneficial effects like CH4 reduction. A large amount of bioactive compounds in those additives makes it challenging to identify their mode of action and predict the effectiveness of those mixtures when added to different basal diets. In this study, a trend for a quadratic response in CH4 (g/day) for an increase in OEO supplementation was detected. The use of high doses of OEO with antimicrobial activity likely decreased the microbial activity and fermentability of the diet. It has been described that the interactions among different components in EOs may affect their antimicrobial activity (56). The availability of raw materials is also important, although this is not a problem for OEO given that it can be obtained from one of the most common subtropical crops in the world (13, 57). The quality of meat is not affected by EO (58, 59). The inclusion of OEO in the diet of sheep has been shown to improve milk yield, fat milk yield, and feed efficiency (11). In our study, discrepancies between in vitro and in vivo results might be caused by the absence of VFA absorption and passage in in vitro batch systems compared to in vivo, as well as differences in the composition of the microbial population (60, 61). Our findings demonstrate that OEO decreased CH4 emissions, which also might lead to improved feed efficiency (2).
Conclusion
Orange essential oil, containing 78.84% D-limonene, supplemented at 0.25% did not have adverse effects on DMI, apparent total tract digestibility, rumen fermentation parameters, or enteric CH4 production. Supplementation of 0.5% OEO reduced CH4 emissions by 12%; however, 0.5% OEO had a negative effect on the apparent total tract digestibility of DM.
Data availability statement
The raw data supporting the conclusions of this article will be made available by the authors, without undue reservation.
Ethics statement
The animal study was reviewed and approved by Bioethics Committee at the University of Yucatan (approval no. CB-CCBA-D-2019-01).
Author contributions
RJ-O, MM-F, EA-R, and ME-E: investigation, writing-original draft, review, editing, conceptualization, and data elaboration. JK-V and JA: supervision, funding acquisition, and review and editing. EH-T, MG-C, EA-R, and GP-C: in vitro experiments, writing-review and editing, and data elaboration. CA-P: review, editing, validation, and data elaboration. All authors contributed to the editing of the paper and the intellectual content, and have approved it for publication.
Funding
This research was funded by the one CGIAR initiative on Livestock, Climate, and System Resilience (LCSR). We thank all donors that globally Support our work through their contributions to the CGIAR system.
Acknowledgments
We thank CONACYT for the RJ-O. Fellowship and the CLIFF-GRADS Program. We thank CCAFS and the Global Research Alliance on Agricultural Greenhouse Gases for Enhancing the Research Abilities and Competencies of RJ-O (www.Globalresearchalliance.org). We thank the Firms CITROFRUT S.A. of C.V. and ARPEN S.P.R. of R.L. of C.V. for Assistance with EO.
Conflict of interest
The authors declare that the research was conducted in the absence of any commercial or financial relationships that could be construed as a potential conflict of interest.
Publisher's note
All claims expressed in this article are solely those of the authors and do not necessarily represent those of their affiliated organizations, or those of the publisher, the editors and the reviewers. Any product that may be evaluated in this article, or claim that may be made by its manufacturer, is not guaranteed or endorsed by the publisher.
References
1. IPCC. Climate Change and Land. Chapter 5: Food Security. (2019). Available online at: https://www.ipcc.ch/srccl/chapter/chapter-5/ (accessed April 26, 2022).
2. Honan M, Feng X, Tricarico JM, Kebreab E. Feed additives as a strategic approach to reduce enteric methane production in cattle: modes of action, effectiveness and safety. Anim Prod Sci. (2021) doi: 10.1071/AN20295
3. Min B-R, Lee S, Jung H, Miller DN, Chen R. Enteric methane emissions and animal performance in dairy and beef cattle production: strategies, opportunities, and impact of reducing emissions. Animals. (2022) 12:948. doi: 10.3390/ani12080948
4. Carrazco AV, Peterson CB, Zhao Y, Pan Y, McGlone JJ, DePeters EJ, et al. The impact of essential oil feed supplementation on enteric gas emissions and production parameters from dairy cattle. Sustainability. (2020) 12:10347. doi: 10.3390/su122410347
5. McGrath J, Duval SM, Tamassia LFM, Kindermann M, Stemmler RT, de Gouvea VN, et al. Nutritional strategies in ruminants: a lifetime approach. Res Vet Sci. (2018) 116:28–39. doi: 10.1016/j.rvsc.2017.09.011
6. Burt S. Essential oils: their antibacterial properties and potential applications in foods—a review. Int J Food Microbiol. (2004) 94:223–53. doi: 10.1016/j.ijfoodmicro.2004.03.022
7. Ku-Vera JC, Castelán-Ortega OA, Galindo-Maldonado FA, Arango J, Chirinda N, Jiménez-Ocampo R, et al. Review: strategies for enteric methane mitigation in cattle fed tropical forages. Animal. (2020) 14:s453–63. doi: 10.1017/S1751731120001780
8. Ku-Vera JC, Jiménez-Ocampo R, Valencia-Salazar SS, Montoya-Flores MD, Molina-Botero IC, Arango J, et al. Role of secondary plant metabolites on enteric methane mitigation in ruminants. Front Vet Sci. (2020) 7:584. doi: 10.3389/fvets.2020.00584
9. Souza KA de, Monteschio J de O, Mottin C, Ramos TR, Pinto LA de M, Eiras CE, et al. Effects of diet supplementation with clove and rosemary essential oils and protected oils (eugenol, thymol and vanillin) on animal performance, carcass characteristics, digestibility, and ingestive behavior activities for Nellore heifers finished in feedlot. Livest Sci. (2019) 220:190–5. doi: 10.1016/j.livsci.2018.12.026
10. Horky P, Skalickova S, Smerkova K, Skladanka J. Essential oils as a feed additives: pharmacokinetics and potential toxicity in monogastric animals. Animals. (2019) 9:352. doi: 10.3390/ani9060352
11. Kotsampasi B, Tsiplakou E, Christodoulou C, Mavrommatis A, Mitsiopoulou C, Karaiskou C, et al. Effects of dietary orange peel essential oil supplementation on milk yield and composition, and blood and milk antioxidant status of dairy ewes. Anim Feed Sci Technol. (2018) 245:20–31. doi: 10.1016/j.anifeedsci.2018.08.007
12. Lin B, Lu Y, Salem AZM, Wang JH, Liang Q, Liu JX. Effects of essential oil combinations on sheep ruminal fermentation and digestibility of a diet with fumarate included. Anim Feed Sci Technol. (2013) 184:24–32. doi: 10.1016/j.anifeedsci.2013.05.011
13. Hassan F, Arshad MA, Ebeid HM, Rehman MS, Khan MS, Shahid S, et al. Phytogenic additives can modulate rumen microbiome to mediate fermentation kinetics and methanogenesis through exploiting diet–microbe interaction. Front Vet Sci. (2020) 7:575801. doi: 10.3389/fvets.2020.575801
14. Hosni K, Zahed N, Chrif R, Abid I, Medfei W, Kallel M, et al. Composition of peel essential oils from four selected tunisian citrus species: evidence for the genotypic influence. Food Chem. (2010) 123:1098–104. doi: 10.1016/j.foodchem.2010.05.068
15. de Araújo JSF, de Souza EL, Oliveira JR, Gomes ACA, Kotzebue LRV, da Silva Agostini DL, et al. Microencapsulation of sweet orange essential oil (Citrus aurantium var. dulcis) by liophylization using maltodextrin and maltodextrin/gelatin mixtures: Preparation, characterization, antimicrobial and antioxidant activities. Int J Biol Macromol. (2020) 143:991–9. doi: 10.1016/j.ijbiomac.2019.09.160
16. Kamalak A, Atalay AI, Ozkan CO, Tatliyer A. Kaya E. Effect of essential orange (Citrus sinensis L) oil on rumen microbial fermentation using in vitro gas production technique. J Anim Plant Sci. (2011) 21:764–9.
17. Wu P, Liu ZB, He WF Yu SB, Gao G, Wang JK. Intermittent feeding of citrus essential oils as a potential strategy to decrease methane production by reducing microbial adaptation. J Clean Prod. (2018) 194:704–13. doi: 10.1016/j.jclepro.2018.05.167
18. García-Rodríguez J, Saro C, Mateos I, González JS, Carro MD, Ranilla MJ. Effects of replacing extruded maize by dried citrus pulp in a mixed diet on ruminal fermentation, methane production, and microbial populations in rusitec fermenters. Animals. (2020) 10:1316. doi: 10.3390/ani10081316
19. Ali W. Korir, Merbold, Goopy, Butterbach-Bahl, Dickhoefer, Schlecht. Supplementing tropical cattle for improved nutrient utilization and reduced enteric methane emissions. Animals. (2019) 9:210. doi: 10.3390/ani9050210
20. Araiza-Ponce K, Murillo-Ortiz M, Herrera-Torres E, Valencia-Vázquez R, Carrete-Carreón F, Pamanes-Carrasco G. Leucaena leucocephala y Opuntia ficus-indica reducen la producción de metano in vitro. Abanico Vet. (2020) 10:1–13. doi: 10.21929/abavet2020.18
21. Musco N, Koura IB, Tudisco R, Awadjihè G, Adjolohoun S, Cutrignelli MI, et al. Nutritional characteristics of Forage Grown in South of Benin. Asian-Australas J Anim Sci. (2015) 29:51–61. doi: 10.5713/ajas.15.0200
22. Schofield P, Pitt RE, Pell AN. Kinetics of fiber digestion from in vitro gas production. J Anim Sci. (1994) 72:2980–91. doi: 10.2527/1994.72112980x
23. González-Arreola A, Murillo-Ortíz M, Pámanes-Carrasco G, Reveles-Saucedo FO, Herrera-Torres E. Nutritive quality and gas production of corn silage with the addition of fresh and fermented prickly pear cladodes. J Anim Plant Sci. (2019) 40:6544–53.
24. Ørskov ER, McDonald I. The estimation of protein degradability in the rumen from incubation measurements weighted according to rate of passage. J Agric Sci. (1979) 92:499–503. doi: 10.1017/S0021859600063048
25. National Academies of Sciences E and Medicine. Nutrient Requirements of Beef Cattle: Eighth Revised Edition. Washington, DC: The National Academies Press (2016)
26. Arceo-Castillo JI, Montoya-Flores MD, Molina-Botero IC, Piñeiro-Vázquez AT, Aguilar-Pérez CF, Ayala-Burgos AJ, et al. Effect of the volume of methane released into respiration chambers on full system methane recovery. Anim Feed Sci Technol. (2019) 249:54–61. doi: 10.1016/j.anifeedsci.2019.02.001
27. IPCC. IPCC Guidelines for National Greenhouse Gas Inventories. (2006). Available online at: https://www.ipcc-nggip.iges.or.jp/public/2006gl/vol1.html (accessed April 15, 2019).
28. Van Soest PJ, Robertson JB, Lewis BA. Methods for dietary fiber, neutral detergent fiber, and nonstarch polysaccharides in relation to animal nutrition. J Dairy Sci. (1991) 74:3583–97. doi: 10.3168/jds.S0022-0302(91)78551-2
30. Droby S, Eick A, Macarisin D, Cohen L, Rafael G, Stange R, et al. Role of citrus volatiles in host recognition, germination and growth of Penicillium digitatum and Penicillium italicum. Postharvest Biol Technol. (2008) 49:386–96. doi: 10.1016/j.postharvbio.2008.01.016
31. Moufida S, Marzouk B. Biochemical characterization of blood orange, sweet orange, lemon, bergamot and bitter orange. Phytochemistry. (2003) 62:1283–9. doi: 10.1016/S0031-9422(02)00631-3
32. Pino J, Sánchez M, Sánchez R, Roncal E. Chemical composition of orange oil concentrates. Nahrung. (1992) 36:539–42. doi: 10.1002/food.19920360604
33. González-Mas MC, Rambla JL, López-Gresa MP, Blázquez MA, Granell A. Volatile compounds in citrus essential oils: a comprehensive review. Front Plant Sci. (2019) 10:12. doi: 10.3389/fpls.2019.00012
34. Ruiz B, Flotats X. Citrus essential oils and their influence on the anaerobic digestion process: an overview. Waste Manag. (2014) 34:2063–79. doi: 10.1016/j.wasman.2014.06.026
35. Castillejos L, Calsamiglia S, Ferret A. Effect of essential oil active compounds on rumen microbial fermentation and nutrient flow in in vitro systems. J Dairy Sci. (2006) 89:2649–58. doi: 10.3168/jds.S0022-0302(06)72341-4
36. Castillejos L, Calsamiglia S, Martín-Tereso J, Ter Wijlen H. In vitro evaluation of effects of ten essential oils at three doses on ruminal fermentation of high concentrate feedlot-type diets. Anim Feed Sci Technol. (2008) 145:259–70. doi: 10.1016/j.anifeedsci.2007.05.037
37. Thao NT, Wanapat M, Kang S, Cherdthong A. Effects of Supplementation of Eucalyptus E. Camaldulensis leaf meal on feed intake and rumen fermentation efficiency in Swamp Buffaloes Asian-Australas. J Anim Sci. (2015) 28:951–7. doi: 10.5713/ajas.14.0878
38. Thao NT, Wanapat M, Cherdthong A, Kang S. Effects of eucalyptus crude oils supplementation on rumen fermentation, microorganism and nutrient digestibility in Swamp Buffaloes. Asian-Australas J Anim Sci. (2014) 27:46–54. doi: 10.5713/ajas.2013.13301
39. Nanon A, Suksombat W, Yang WZ. Effects of essential oils supplementation on in vitro and in situ feed digestion in beef cattle. Anim Feed Sci Technol. (2014) 196:50–9. doi: 10.1016/j.anifeedsci.2014.07.006
40. Cobellis G, Acuti G, Forte C, Menghini L, De Vincenzi S, Orrù M, et al. Use of Rosmarinus officinalis in sheep diet formulations: Effects on ruminal fermentation, microbial numbers and in situ degradability. Small Rumin Res. (2015) 126:10–8. doi: 10.1016/j.smallrumres.2015.01.018
41. Afzalani, Zein M, Jamarun N, Musnandar E. Effect of increasing doses of essential oil extracted from berastagi orange (Citrus sinensis L.) peels on performance, rumen fermentation and blood metabolites in fattening Bali Cattle. Pak J Nutr. (2015) 14:480–86. doi: 10.3923/pjn.2015.480.486
42. Ying Y, Niu M, Clarke AR, Harvatine KJ. Short communication: effect of a citrus extract in lactating dairy cows. J Dairy Sci. (2017) 100:5468–71. doi: 10.3168/jds.2016-12233
43. Belanche A, Newbold CJ, Morgavi DP, Bach A, Zweifel B, Yáñez-Ruiz DR, et al. Meta-analysis describing the effects of the essential oils blend agolin ruminant on performance, rumen fermentation and methane emissions in dairy cows. Animals. (2020) 10:620. doi: 10.3390/ani10040620
44. Oh J, Harper M, Hristov AN. Effects of lowering crude protein supply alone or in a combination with essential oils on productivity, rumen function and nutrient utilization in dairy cows. Animal. (2019) 13:2510–8. doi: 10.1017/S1751731119001083
45. Benchaar C, Calsamiglia S, Chaves AV, Fraser GR, Colombatto D, McAllister TA, et al. review of plant-derived essential oils in ruminant nutrition and production. Anim Feed Sci Technol. (2008) 145:209–28. doi: 10.1016/j.anifeedsci.2007.04.014
46. Cardozo PW, Calsamiglia S, Ferret A, Kamel C. Effects of alfalfa extract, anise, capsicum, and a mixture of cinnamaldehyde and eugenol on ruminal fermentation and protein degradation in beef heifers fed a high-concentrate diet1. J Anim Sci. (2006) 84:2801–8. doi: 10.2527/jas.2005-593
47. Hundal J, Wadhwa M, Bakshi M. Effect of supplementing essential oils on the in vitro methane production and digestibility of wheat straw. J Anim Res Nutr. (2016) 01:014. doi: 10.21767/2572-5459.100014
48. Rofiq MN, Negara W, Martono S, Gopar RA, Boga M. Potential effect of some essential oils on rumen methane reduction and digestibility by in vitro incubation technique. IOP Conf Ser: Earth Environ Sci. (2021) 905:012138. doi: 10.1088/1755-1315/905/1/012138
49. Hassan F, Arshad MA Li M, Rehman MS, Loor JJ, Huang J. Potential of mulberry leaf biomass and its flavonoids to improve production and health in ruminants: mechanistic insights and prospects. Animals. (2020) 10:2076. doi: 10.3390/ani10112076
50. Samii SS, Wallace N, Nagaraja TG, Engstrom MA, Miesner MD, Armendariz CK, et al. Effects of limonene on ruminal Fusobacterium necrophorum concentrations, fermentation, and lysine degradation in cattle. J Anim Sci. (2016) 94:3420–30. doi: 10.2527/jas.2016-0455
51. Spanghero M, Zanfi C, Fabbro E, Scicutella N, Camellini C. Effects of a blend of essential oils on some end products of in vitro rumen fermentation. Anim Feed Sci Technol. (2008) 145:364–74. doi: 10.1016/j.anifeedsci.2007.05.048
52. Van Soest PJ. Nutritional Ecology of the Ruminant. (2018). Available online at: https://doi.org/10.7591/9781501732355 (accessed February 20, 2020).
53. Benchaar C, Petit HV, Berthiaume R, Ouellet DR, Chiquette J, Chouinard PY. Effects of essential oils on digestion, ruminal fermentation, rumen microbial populations, milk production, and milk composition in dairy cows fed alfalfa silage or corn silage. J Dairy Sci. (2007) 90:886–97. doi: 10.3168/jds.S0022-0302(07)71572-2
54. Direkvandi E, Mohammadabadi T, Salem AZM. Influence of three microbial feed additives of Megasphaera elsdenii, Saccharomyces cerevisiae and Lactobacillus sp. on ruminal methane and carbon dioxide production, and biofermentation kinetics. J Appl Microbiol. (2021) 131:623–33. doi: 10.1111/jam.14990
55. Cobellis G, Trabalza-Marinucci M, Yu Z. Critical evaluation of essential oils as rumen modifiers in ruminant nutrition: a review. Sci Total Environ. (2016) 545:556–68. doi: 10.1016/j.scitotenv.2015.12.103
56. Cobellis G, Trabalza-Marinucci M, Marcotullio MC Yu Z. Evaluation of different essential oils in modulating methane and ammonia production, rumen fermentation, and rumen bacteria in vitro. Anim Feed Sci Technol. (2016) 215:25–36. doi: 10.1016/j.anifeedsci.2016.02.008
57. Rezzadori K, Benedetti S, Amante ER. Proposals for the residues recovery: orange waste as raw material for new products. Food Bioprod Process. (2012) 90:606–14. doi: 10.1016/j.fbp.2012.06.002
58. Rivaroli DC, del Mar Campo M, Sañudo C, Guerrero A, Jorge AM, Vital ACP, et al. Effect of an essential oils blend on meat characteristics of crossbred heifers finished on a high-grain diet in a feedlot. Anim Prod Sci. (2020) 60:595. doi: 10.1071/AN18620
59. Rivaroli DC, Guerrero A, Velandia Valero M, Zawadzki F, Eiras CE, Campo M., del M, et al. Effect of essential oils on meat and fat qualities of crossbred young bulls finished in feedlots. Meat Sci. (2016) 121:278–84. doi: 10.1016/j.meatsci.2016.06.017
60. Macome FM, Pellikaan WF., Schonewille JTh, Bannink A, van Laar H, Hendriks WH, et al. iIn vitro rumen gas and methane production of grass silages differing in plant maturity and nitrogen fertilisation, compared to in vivo enteric methane production. Anim Feed Sci Technol. (2017) 230:96–102. doi: 10.1016/j.anifeedsci.2017.04.005
61. Yáñez-Ruiz DR, Bannink A, Dijkstra J, Kebreab E, Morgavi DP, O'Kiely P, et al. Design, implementation and interpretation of in vitro batch culture experiments to assess enteric methane mitigation in ruminants—a review. Anim Feed Sci Technol. (2016) 216:1–18. doi: 10.1016/j.anifeedsci.2016.03.016
62. Babushok VI, Linstrom PJ, Zenkevich IG. Retention indices for frequently reported compounds of plant essential oils. J Phys Chem Ref Data. (2011) 40:043101. doi: 10.1063/1.3653552
Keywords: cattle, enteric methane, essential oil, feed additive, plant secondary metabolites
Citation: Jiménez-Ocampo R, Montoya-Flores MD, Pamanes-Carrasco G, Herrera-Torres E, Arango J, Estarrón-Espinosa M, Aguilar-Pérez CF, Araiza-Rosales EE, Guerrero-Cervantes M and Ku-Vera JC (2022) Impact of orange essential oil on enteric methane emissions of heifers fed bermudagrass hay. Front. Vet. Sci. 9:863910. doi: 10.3389/fvets.2022.863910
Received: 27 January 2022; Accepted: 26 July 2022;
Published: 16 August 2022.
Edited by:
Paula Macarena Toro-Mujica, Universidad de O'Higgins, ChileReviewed by:
Anusorn Cherdthong, Khon Kaen University, ThailandAvijit Dey, Central Institute for Research on Buffaloes (ICAR), India
Martin Hünerberg, University of Göttingen, Germany
Copyright © 2022 Jiménez-Ocampo, Montoya-Flores, Pamanes-Carrasco, Herrera-Torres, Arango, Estarrón-Espinosa, Aguilar-Pérez, Araiza-Rosales , Guerrero-Cervantes and Ku-Vera. This is an open-access article distributed under the terms of the Creative Commons Attribution License (CC BY). The use, distribution or reproduction in other forums is permitted, provided the original author(s) and the copyright owner(s) are credited and that the original publication in this journal is cited, in accordance with accepted academic practice. No use, distribution or reproduction is permitted which does not comply with these terms.
*Correspondence: Rafael Jiménez-Ocampo, cmFmYXg3N0Bob3RtYWlsLmNvbQ==; Juan C. Ku-Vera, a3ZlcmFAY29ycmVvLnVhZHkubXg=