- 1Department of Traditional Chinese Veterinary Medicine, College of Veterinary Medicine, Southwest University, Chongqing, China
- 2Immunology Research Center, Medical Research Institute, Southwest University, Chongqing, China
In broiler chicks, Escherichia coli lipopolysaccharide is a prominent cause for inflammatory damage and loss of immune homeostasis in broiler chicks. Ginsenosides have been shown to have anti-inflammatory and antioxidant effects. However, it has not been demonstrated that ginsenosides protect broiler chicks against stress induced by Escherichia coli lipopolysaccharide challenge. The aim of this is to investigate the protective effect of ginsenosides Rg1, Re, and Rg3 on Escherichia coli lipopolysaccharide-induced stress. Our results showed that Rg3 ameliorated growth inhibition and fever, as well as decreased the production of stress-related hormones in broilers with stress. The protective effect of Rg3 on the stressed chicks may be largely mediated by regulating inflammatory response and oxidative damage. Moreover, real-time quantitative-polymerase chain reaction (RT-qPCR) results demonstrated that Rg3 upregulated mRNA expression of mTOR, HO-1, and SOD-1. These results suggested that ginsenoside Rg3 and ginsenoside products contains Rg3 deserve further study for the control of immunological stress and inflammation in broiler chicks.
Introduction
Gram-negative environmental pathogens, such as Escherichia coli (E. coli), are common pathogens that attack dairy cows, pigs, and chickens (1–3). These pathogenic microorganisms not only cause morbidity and mortality in animals but also lead to the loss of immune homeostasis, and trigger stress responses (4). Under stress, the anabolism of proteins and fats is weakened, while catabolism is promoted to meet the need for nutrients to synthesize immune effector molecules (5). In addition, clinical signs of stress such as depression and diarrhea induced growth inhibition in broilers, resulting in significant economic losses (6, 7). Thus, controlling the stress induced by Escherichia coli and other pathogenic microorganisms has become an urgent issue.
Lipopolysaccharide (LPS) is a main constituent of gram-negative bacterial cell walls and stimulates production of several proinflammatory cytokines such as interleukin-1β (IL-1β), interleukin-6 (IL-6), interleukin-8 (IL-8), and tumor necrosis factor alpha (TNF-α) via the toll-like receptor 4 (TLR4) signaling pathway (8). IL-6 may accelerate glycogen hydrolysis and glucose production while TNF-α may promote adipolysis and proteolysis, leading to reduced synthesis of protein and lipid (9, 10). Moreover, inflammatory cytokines could promote glucocorticoid secretion and reduce the secretion of growth hormone through the neuroendocrine system, resulting in decreased appetite and feed intake (11). In addition, LPS promoted secretion of several proinflammatory cytokines by increasing the phosphorylation of Jun N-terminal kinase (JNK), p38 mitogen-activated protein kinase (MAPK), nuclear factors kappa B (NF-κB) while decreasing the phosphorylation level of mammalian target of rapamycin (mTOR) (12). Because the overproduction proinflammatory cytokines can generate reactive oxygen species (ROS), the oxidative damage of multiple tissues should also be considered in the pathogenesis of LPS-induced stress (13). Therefore, inhibition of inflammatory response, oxidative damage as well as related pathways may be considered as important drug targets for the prevention of infection and immunological stress (14).
Ginsenosides Rg1, Re, and Rg3 are extracts made from the root of Panax ginseng C.A. Meyer, which has been used for thousands of years in traditional Chinese medicine (15). Previous studies have found that these agents exert anti-inflammatory and antioxidant effects via multiple pathways including TLR4/NF-κB (16–18). However, it has not been reported whether ginsenosides can protect broiler chicks against LPS-induced stress. In this study, we investigated the effects of ginsenosides Rg1, Rg3, and Re on stressed broiler chicks induced by E. coli LPS. Our results may reveal the potential role of ginsenosides in the prevention of immunological stress and inflammation in broiler chicks, as well as the underlying mechanism.
Materials and Methods
Reagents
Ginsenoside Rg1 (98.92%), Ginsenoside Rg3 (98.18%), and Ginsenoside Re (98%) were purchased from Chengdu Purify Biotechnology Co., Ltd. (Chengdu, China). The chemical structure of ginsenosides was shown in Figures 1A–C. Ginsenosides Rg1 (C42H72O14; molecular weight, 801.024), Re (C48H82O18; molecular weight, 947.166), and Rg3 (C42H72O13; molecular weight, 785.025) are extracted from the root of Panax ginseng C.A. Meyer. The majority of ginsenosides are dammarane-type saponins. According to the saponins, dammarane-type ginsenosides can be divided into two types, protopanaxadiol (PPD) and protopanaxatriol (PPT). Rg3 are PPD-type ginsenosides with sugar moieties attached to the β-OH at C-3 and/or C-20 in the aglycon PPD. Ginsenosides Re and Rg1 are PPT-type ginsenosides with sugar moieties linked to the α-OH at C-6 and/or β-OH at C-20 in the aglycon PPT. Although Rg1 and Re share a similar chemical structure with a dammarane skeleton, Re has an additional rhamnose at C-6 position.
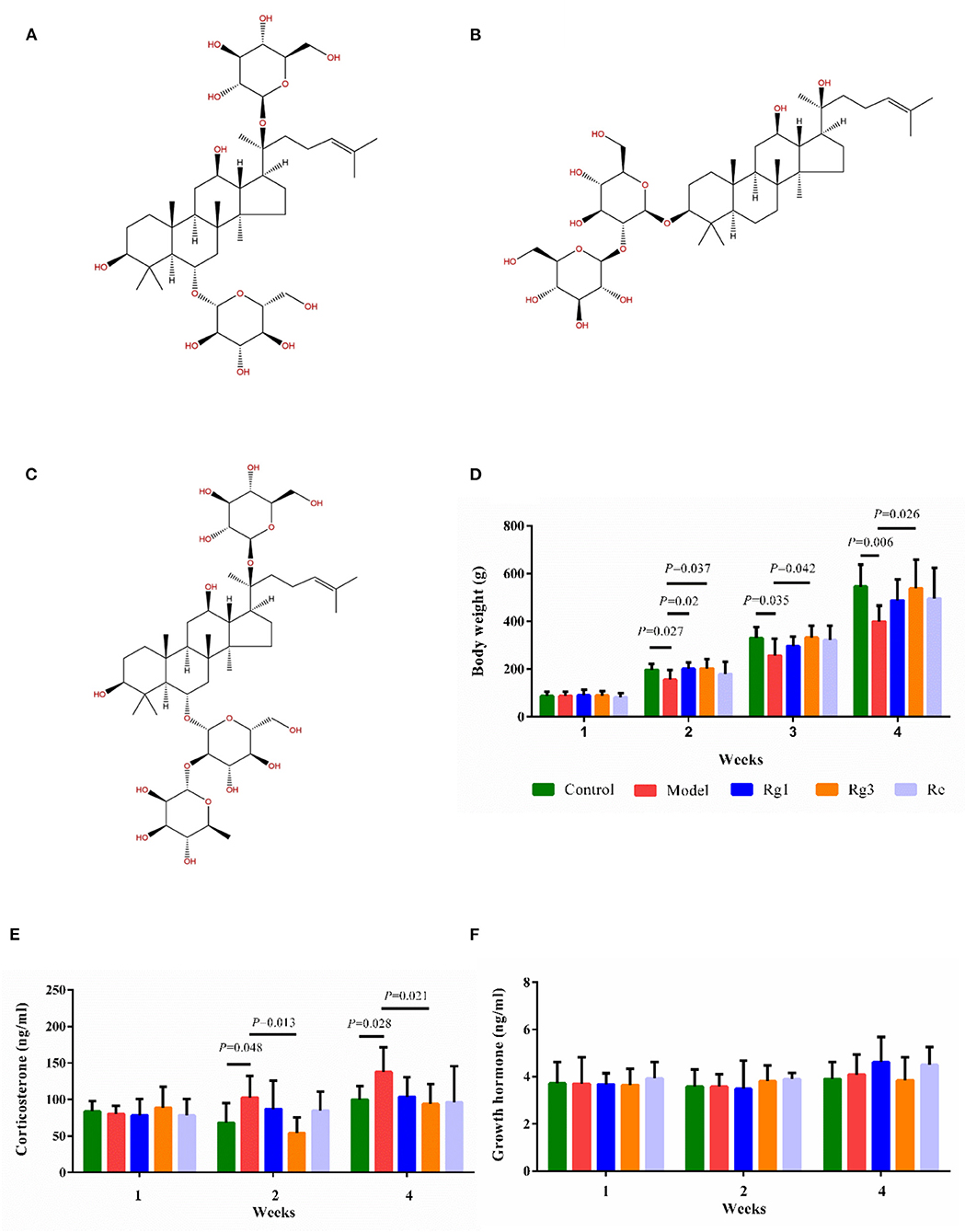
Figure 1. Effect of ginsenosides on growth and stress of broiler chicks. Chemical structure of ginsenoside Rg1 (C42H72O14; molecular weight, 801.024) (A); Rg3 (C42H72O13; molecular weight, 785.025) (B); Re (C48H82O18; molecular weight, 947.166) (C). Determination of body weight (D), serum corticosterone (E), and serum growth hormone (F) by ELISA. Values are means ± standard error (SE) (n = 8).
Lipopolysaccharide from E. coli serotype O55:B5 was purchased from Sigma-Aldrich Chemical Co. (St. Louis, MO, USA). Detection kits for corticosterone (CORT), growth hormone (GH), and adrenocorticotropic hormone (ACTH) were purchased from Nanjing Jiancheng Bioengineering Institute (Nanjing, China). IL-6, IL-1β, TNF-α, and inducible nitric oxide synthase (iNOS) enzyme-linked immunosorbent assay (ELISA) kits were from Shanghai Lengdon Bioscienci Co., Ltd. (Shanghai, China). Detection kits for nitric oxide (NO), total superoxide dismutase (T-SOD), catalase (CAT), total antioxidant capacity (T-AOC), the contents of malondialdehyde (MDA), glutathione (GSH), glutathione peroxidase (GSH-PX), xanthine oxidase (XOD), and carbonyl were products of Nanjing Jiancheng Bioengineering Institute. All other chemicals were analytic grades.
Experimental Design
Sichuan Lihua Poultry Co., Ltd. Provided one-day-old male Hongyu commecial broiler chickens, which were housed separately in three-layer cages. The indoor temperature was maintained at 32–34°C for a week, and then gradually decreased by 1°C every 2 day until a final temperature of 26°C was achieved. All broilers had free access to feed and water. The animal experiments were performed in accordance with the Animal Care and Use Committee of Southwest University (permit number IACUC-20200701-01). All experimental animals were euthanized at the end of the experiment.
In experiment 1, 40 broiler chicks were randomly divided into 5 groups, each consisting of 8 birds (Table 1). Chicks were received intraperitoneally administered saline, 1 mg Rg1/kg body weight (1 mg/kg Rg1), 1 mg/kg Re, or 1 mg/kg Rg3 2 h before LPS challenge. The chicks were then given intraperitoneal injection of 250 μg/kg LPS at the ages of 12, 14, 33, and 35 days to induce immunological stress (19). Control group was injected with an equivalent amount of sterile saline. Body weight was measured every week. Blood samples were collected from 8 chickens at the age of 1, 2, and 4 weeks for determination of CORT and GH.
In experiment 2, one hundred and sixty broiler chicks were randomly allocated to 4 treatments. Each treatment contained 4 replicates of 10 broilers per replicate (Table 2). Chickens in Rg3-treated groups were received intraperitoneally administered 1 mg/kg Rg3 2 h before or after LPS challenge. Control group was injected with an equivalent amount of sterile saline. Then the broilers were intraperitoneally injection of 250 μg/kg LPS at the age of 12, 14, 33, and 35 days to induce immunological stress. Birds were group weighed by cage at the age of 7, 14, 21, 28, and 35 days. Feed intake was monitored by cage at the age of 7, 14, 21, 28, and 35 days. Average daily weight gain (DWG), daily feed intake (DFI), and feed conversion ratio (FCR) were calculated for each period and for the overall experiment. At the age of 12 days, 16 chickens were randomly selected from each group. A digital thermometer for livestock (LY-302C, Lvyuan Hengtai, Co., Ltd., China) was used to measure body temperature by inserting it into the cloaca. The results were recorded every 4 h and monitored for 24 h continuously. Blood samples were collected using wing vein puncture at 1, 2, and 4 weeks and the serum was obtained for detection of hormone content, inflammatory mediators and antioxidant parameters. Total RNA was extracted from liver for real-time quantitative polymerase chain reaction (RT-qPCR analysis).
Determination of CORT, GH, and ACTH
About 4 mL of blood was centrifuged at 3,000 g for 10 min to get serum. The concentrations of serum CORT (H205), GH (H091-1-2), and ACTH (H097-1-2) were determined by using ELISA kits (Jiancheng Bioengineering Institute, Nanjing, China) according to the manufacturer's instructions.
Measurement of Inflammatory Responses
The contents of IL-6, IL-1β, TNF-α, NO, and iNOS in the serum samples were measured by ELISA using chicken-specific quantification kits (Lengdon Bioscienci Co., Ltd., Shanghai, China).
Assay of Antioxidant Enzymes
The enzyme activities of T-SOD (A001-1-2), CAT (A007-1-1), T-AOC (A015-2-1), GSH-Px (A005-1-2), XOD (A002-1-1), and the contents of MDA (A003-1-2), GSH (A006-2-1), carbonyl (A087-1-2) in the serum were determined spectrophotometrically with the commercial kits (Jiancheng Bioengineering Institute, Nanjing, China) according to the instructions of the manufacturer.
RNA Extraction and RT-qPCR
Total RNA was extracted from the liver using TRIzol reagent (Takara, Shiga, Japan) following the manufacturer's guidelines. PrimeScript™RT Master Mix (Takara, Dalian, China) was utilized to convert RNA into cDNA on a T100™ thermal cycler (Bio Rad, Hercules, CA). The Chicken β-actin was served as the internal control gene. RT-qPCR with SYBR®Premix Ex Taq™ II (Tli RNaseH Plus) (Takara, Dalian, China) on selected genes was performed on a Multiple Real-Time PCR System (Applied Biosystems, Carlsbad, CA). A relative quantitative method (2−ΔΔCT) was employed to evaluate the quantitative variation (20). The primer sequences were list in Table 3. The final primer concentration was 10 pmol/μl of each primer.
Statistics Analysis
The one-way analysis of variance with Duncan's multiple range test was employed using SPSS software (version 20.0, SPSS Inc., Chicago, IL). Data was expressed as mean ± standard error (SE). Differences between means at p < 0.05 or p < 0.01 were statistically significant.
Results
Body Weight and Growth Performance
In experiment 1, the effect of each treatment on body weight was shown in Figure 1D. The LPS challenge significantly decreased body weight of broilers at the age of 2 (p < 0.05), 3 (p < 0.05), and 4 (p < 0.01) weeks. However, administration of Rg1 (2 weeks of age, p < 0.05) or Rg3 (2, 3, and 4 weeks of age, p < 0.05) attenuated LPS-induced growth inhibition.
In experiment 2, the effect of Rg3 on growth performance of broiler chicks was depicted in Table 4. The chicks with stress (Model) had lower DWG (3 weeks, p < 0.05; 4 weeks, p > 0.05; 5 weeks, p > 0.05) and DFI (3 weeks, p < 0.05; 4 weeks, p > 0.05; 5 weeks, p > 0.05) than the Controls. However, chicks pre-treated or post-treated with Rg3 had higher DFI at 3 weeks (p < 0.05), 4 weeks (p > 0.05), and 5 weeks (p > 0.05) than the Models. Furthermore, pre-treatment with Rg3 numerically increased the DWG of stressed chicks during any periods. There were no significant differences in FCR of broilers between treatments (p> 0.05).
Serum CORT and GH
In experiment 1, LPS challenge distinctly increased serum CORT (2 weeks, p < 0.05; 4 weeks, p < 0.05) content in broilers compared to the Control (Figure 1E). However, pre-treatment with Rg3 significantly decreased serum CORT (2 weeks, p < 0.05; 4 weeks, p < 0.05) content of broilers after LPS challenge. In addition, there was no significant difference in serum GH of broilers among the different groups (Figure 1F).
Body Temperature
The body temperature of broilers at 2 weeks was recorded. As depicted in Figure 2, there was no significant difference (p> 0.05) in body temperature among the four groups of broilers before LPS challenge. After the LPS injection, the body temperature increased rapidly, peaked at 4 h (p < 0.01), and returned to baseline levels at 16 h. However, the body temperature of broilers pre-treated with Rg3 decreased at 12 h, 4 h earlier than broilers injected with LPS only (p < 0.05). In addition, the body temperature of stressed broilers post-treated with Rg3 was lower than broilers that only injected with LPS at 4 h (p < 0.01), 8 h (p > 0.05), 12 h (p > 0.05), 16 h (p> 0.05), and 24 h (p > 0.05). The body temperature of stressed broilers pre-treated with Rg3 was numerically lower than broilers that only injected with LPS at different timepoint (p > 0.05).
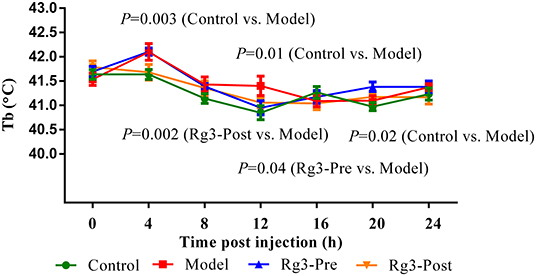
Figure 2. Continuous body temperature (Tb) record in broilers. Continuous monitoring of body temperature post-lipopolysaccharide injection. Values are represented as means ± SE (n = 16).
ACTH and Inflammatory Responses in Serum of Broilers
In experiment 2, LPS challenge increased serum ACTH (2 weeks, p > 0.05; 4 weeks, p < 0.05) content, when compared with the Control. However, pre-treatment with Rg3 decreased serum ACTH (2 weeks, p > 0.05; 4 weeks, p < 0.05) content of broilers after LPS challenge. Post-treated with Rg3 also decreased serum ACTH (2 weeks, p > 0.05; 4 weeks, p < 0.01) content after LPS challenge (Figure 3A).
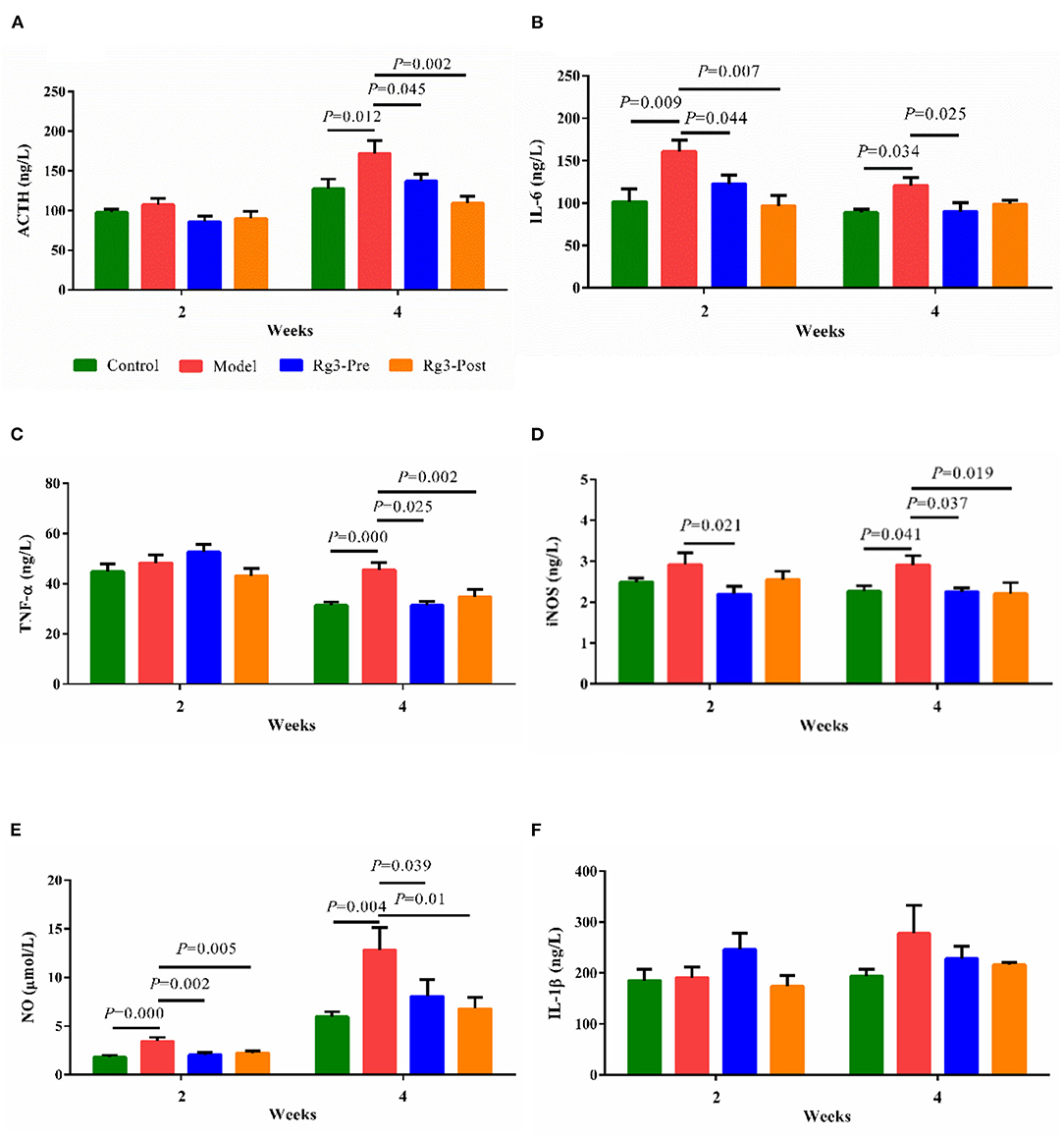
Figure 3. Effect of ginsenoside Rg3 on adrenocorticotropic hormone (ACTH) and inflammatory responses in serum of broilers with stress. Determination of serum ACTH (A), interleukin-6 (IL-6) (B), tumor necrosis factor α (TNF-α) (C), inducible nitric oxide synthase (iNOS) (D), nitric oxide (NO) (E), and interleukin-1β (IL-1β) (F) by ELISA. The values are represented as means ± SE (n = 10).
The effect of Rg3 on inflammatory responses of stressed broilers was shown in Figures 3B–F. Results showed that the LPS challenge promoted the production of serum IL-6 (2 wk, p < 0.01; 4 wk, p < 0.05), TNF-α (2 weeks, p > 0.05; 4 weeks, p < 0.01), iNOS (2 weeks, p > 0.05; 4 weeks, p < 0.05), and NO (2 week, p < 0.01; 4 weeks, p < 0.01). However, pre-treatment of Rg3 inhibited the increased IL-6 (2 weeks, p < 0.05; 4 weeks, p < 0.05), TNF-α (2 weeks, p > 0.05; 4 weeks, p < 0.05), iNOS (2 weeks, p < 0.05; 4 weeks, p < 0.05), and NO (2 weeks, p < 0.01; 4 weeks, p < 0.05). In addition, post-treatment of Rg3 also reversed the increase of IL-6 (2 weeks, p < 0.01; 4 weeks, p > 0.05), TNF-α (2 weeks, p > 0.05; 4 weeks, p < 0.01), iNOS (2 weeks, p > 0.05; 4 weeks, p < 0.05), and NO (2 weeks, p < 0.01; 4 weeks, p < 0.05). There was no significant difference in serum IL-1β of broilers among the different groups.
Effect of Rg3 on Serum Oxidation and Antioxidant Indices
As shown in Figures 4A–E, injection of LPS decreased the activities of T-AOC (2 weeks, p < 0.01; 4 weeks, p < 0.01), T-SOD (2 weeks, p < 0.01; 4 weeks, p < 0.01), CAT (2 weeks, p < 0.05; 4 weeks, p < 0.05), GSH-PX (2 weeks, p > 0.05; 4 weeks, p < 0.01), and increased the activity of XOD (2 weeks, p < 0.05; 4 weeks, p < 0.05) compared to the Control. However, pre-treatment of Rg3 inhibited decreases in activities of T-AOC (2 weeks, p < 0.01; 4 weeks, p > 0.05), T-SOD (2 weeks, p < 0.01; 4 weeks, p > 0.05), CAT (2 weeks, p > 0.05; 4 weeks, p < 0.05), and GSH-PX (2 weeks, p > 0.05; 4 weeks, p < 0.05) in broilers challenged with LPS. Meanwhile, post-treated with Rg3 inhibited decreases in activities of T-SOD (2 weeks, p < 0.05; 4 weeks, p < 0.05), CAT (2 weeks, p < 0.05; 4 weeks, p > 0.05), GSH-PX (2 weeks, p > 0.05; 4 weeks, p < 0.05), and decreased the activities of XOD (2 weeks, p < 0.05; 4 week, p > 0.05) in chicks challenged with LPS.
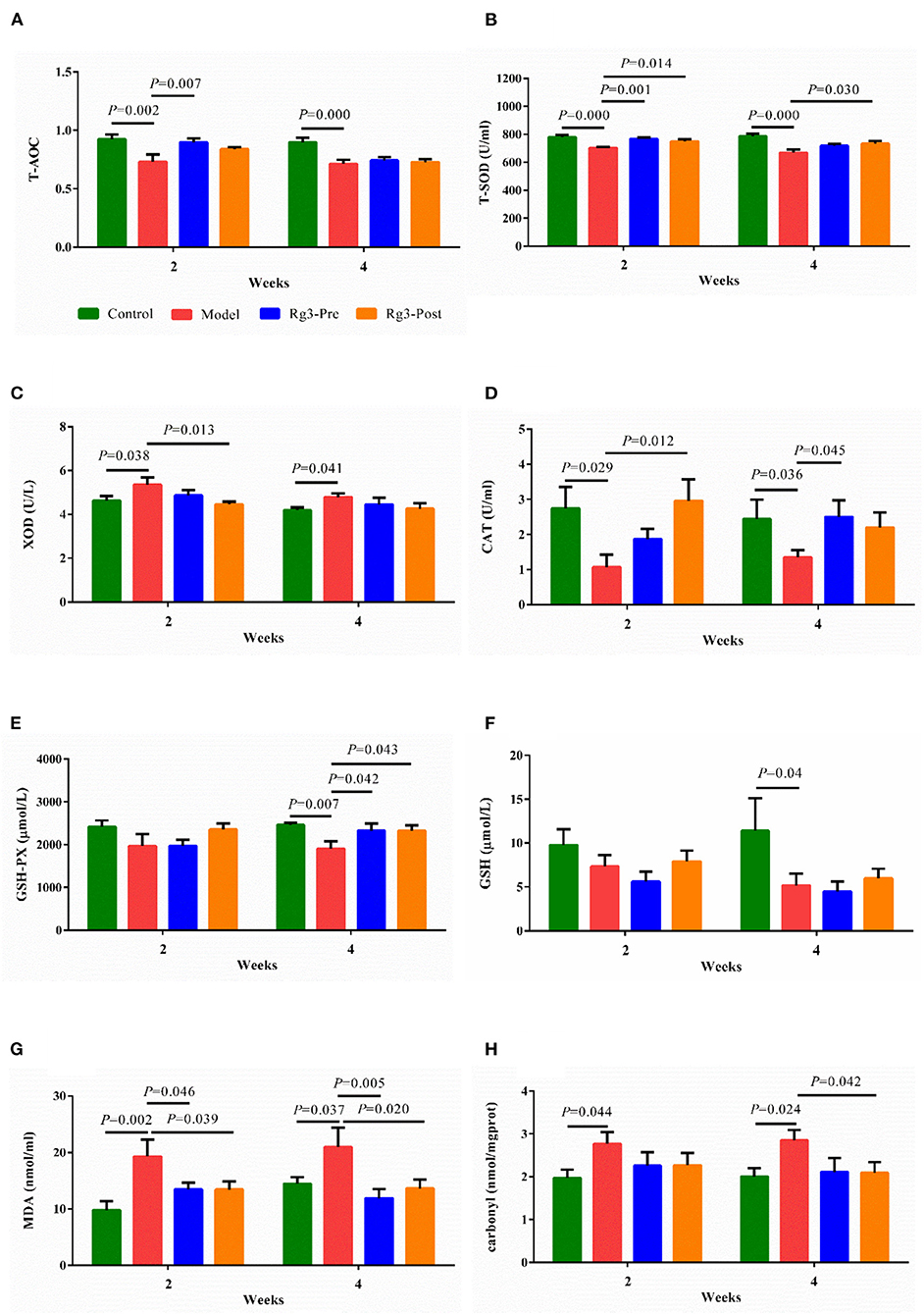
Figure 4. Effect of ginsenoside Rg3 on enzymatic antioxidants and non-enzymatic antioxidants in broilers with stress. Detection for serum total antioxidant capacity (T-AOC) (A), total superoxide dismutase (T-SOD) (B), xanthine oxidase (XOD) (C), catalase (CAT) (D), glutathione peroxidase (GSH-PX) (E), glutathione (GSH) (F), malondialdehyde (MDA), (G) and carbonyl (H). Values are means ± SE (n = 10).
As illustrated in Figures 4F–H, LPS challenge reduced the content of serum GSH (2 weeks, p > 0.05; 4 weeks, p < 0.05), and increased the levels of MDA (2 weeks, p < 0.01; 4 weeks, p < 0.05), and Carbonyl (2 weeks, p < 0.05; 4 weeks, p < 0.05), when compared with the Control. However, pre-treatment of Rg3 inhibited elevation of MDA (2 weeks, p < 0.05; 4 weeks, p < 0.01) in serum of chickens challenged with LPS. In addition, post-treated with Rg3 reversed increase of MDA (2 weeks, p < 0.05; 4 weeks, p < 0.05), and carbonyl (2 weeks, p> 0.05; 4 weeks, p < 0.05) induced by LPS challenge.
Relative Expression of mRNA
As shown in Figure 5, significantly elevated mRNA expression of NF-κB p65 (p < 0.01), MyD88 (p < 0.01), and significantly decreased mRNA expression of mTOR (p < 0.01), Akt (p < 0.01), PI3K (p < 0.01), SOD-1 (p < 0.05), and HO-1 (p < 0.01) were detected in the liver of broilers with stress (Model), when compared with the Control. However, pretreatment of Rg3 significantly increased mRNA expression of mTOR (p < 0.05), SOD-1 (p < 0.01), and HO-1 (p < 0.05). There was no significant difference in mRNA expression of NF-κB p65, MyD88, Akt, and PI3K between the Model and Rg3-Pre-group.
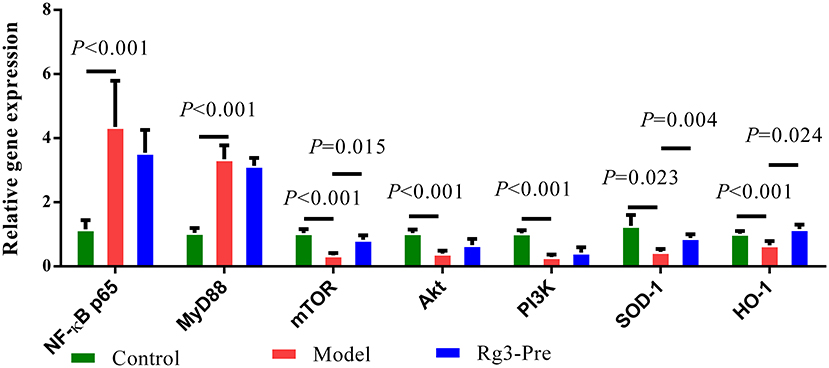
Figure 5. Relative mRNA expression in the liver. Total RNA was extracted from the liver. The Chicken β-actin was served as the internal control gene. Expression of nuclear factors kappa B (NF-κB) p65, myeloid differential protein-88 (MyD88), mammalian target of rapamycin (mTOR), AKT serine-threonine kinase 1 (Akt), phosphoinositol 3 kinase (PI3K), and SOD-1 mRNA were assessed by real-time quantitative PCR. A relative quantitative method (2−ΔΔCT) was employed to evaluate the quantitative variation. Data are represented as means ± SE (n = 6).
Discussion
Young broilers are more susceptible to pathogens than adults because of their immature immune system and high demand for nutrients (25–27). Thus, nutritional interventions were commonly used to maintain growth performance and alleviate the harmful consequences of bacterial LPS challenge (13, 28, 29). Nevertheless, the relief of fever, depression, and diarrhea plays a critical role for animals to recover from immunological stress (19, 30, 31). Plant extracts such as ginseng stem and leaf saponin (GSLS) and astragalus polysaccharide are found effective to regulate inflammation and oxidative stress (19, 32). In 2017, the Agricultural Ministry of China issued a certificate approving the product made from GSLS to be used in poultry (2017–20). It is interesting whether ginsenosides Rg1, Rg3, and Re in GSLS could protect broilers chicks from stress induced by E. coli LPS. The results showed that Rg3 could improve the growth inhibition, shorten the duration of fever and the maximum body temperature, and decrease the serum CORT and ACTH in stressed broilers. Ultimately, Rg3 reversed the growth inhibition and attenuated the stress. In the future, detection of Rg3 content in ginsenosides can be used as one of approaches to control quantity of ginsenosides products.
Although remarkedly decreased body weight gain was observed in broilers challenged with LPS, there was no significant difference in serum growth hormone between Model and Control groups. Generally, the growth inhibition of broilers with immunological stress might be attributed to the activation of inflammatory responses (13, 31). Increased inflammatory cytokines alter metabolic process in cells, depleting nutrition of the immune system, and inhibiting the growth and development of animals (9, 10). Therefore, regulation of pro-inflammatory cytokines and inflammatory mediators is wanted to prevent LPS-induced stress (27, 33). In this study, Rg3 inhibited the production of IL-6, TNF-α, NO, and iNOS in a bird model, which gives us an enlightenment to use Rg3 and other ginsenosides to control inflammation-related diseases in the poultry industry. Our data was consistent with previous results. Yoon et al. (34) found that two optical isomers of Rg3 could suppress NO generation and iNOS expression in LPS-stimulated peritoneal macrophages and inhibit IL-1β production induced by inflammasome activation. Shi et al. (35) reported the inhibiting effect of Rg3 on inflammation evidenced by decreased IL-1 secretion and blocked activation of the caspase-1 and the NLRP3 inflammasome in human and mouse macrophages.
The production of proinflammatory cytokines (TNF-α, IL-1β, and IL-6) and inducible proinflammatory enzymes such as iNOS-released free radicals and induced oxidative stress, causing cell apoptosis and tissue damage (36). Interestingly, we observed the reduced content of MDA and Carbonyl, and the increased activity of T-AOC, T-SOD, GSH-Px, and CAT in the serum of LPS-challenged broilers pre-treated or treated with Rg3. Therefore, we inferred that Rg3 protected stressed broilers also by attenuating the oxidative damage. Numerous studies have confirmed the ability of Rg3 to protect against oxidative stress in vitro and in vivo (18, 37).
Cytokines such as IL-1β, IL-6, and TNF-α have been implicated as mediators to induce fever, and subsequently increase basal metabolite rate and decrease growth performance. Thus, inhibition of cytokines was generally thought as a strategy to attenuate fever and stress (27, 33). In the current study, reduction of maximum body temperature and the duration of fever were associated with decreased production of cytokines in Rg3-treated groups, which is consistent with previous reports. Thus, we presumed Rg3 might regulate the body temperature by suppression of neuroinflammation (38).
The mTOR is a conserved Ser/Thr kinase and is most well-known for mediating the signaling of nutrition, especially branched chain amino acids and lipid metabolism (39, 40). In addition, plant extracts have been reported to regulate inflammation through multiple signal pathways including mTOR. Resveratrol protected the myocardium in sepsis by activating the PI3K/AKT/mTOR pathway and inhibiting the NF-κB signaling pathway (41). Scoparone ameliorated hepatic inflammation of mice with nonalcoholic steatohepatitis by regulating the ROS/P38/Nrf2 axis and PI3K/AKT/mTOR pathway in macrophages (42). In the current study, RT-qPCR assays showed that mRNA expression of mTOR, SOD-1, and HO-1 was increased in the stress of broilers with the administration of ginsenoside Rg3. These results suggested that the protective effect of Rg3 on stress might be related to upregulation of mTOR and antioxidant enzymes. Activation of mTOR or HO-1 has been shown to suppress NF-κB, thus eventually inhibit the inflammation (41, 43). We observed inhibited inflammatory responses in chickens treated with Rg3, however, the motion that whether Rg3 inhibited inflammatory responses by suppressing activation of NF-κB needs to further demonstrate.
In conclusion, ginsenoside Rg3 could reduced the stress of broiler chicks induced by E. coli LPS. The effect of Rg3 on stress may be related to the inhibition of inflammatory response, protection of oxidative damage, and upregulation of mTOR, HO-1, SOD-1. In addition, plant extracts contain Rg3 deserve further study for the control of immunological stress and inflammation in broiler chicks.
Data Availability Statement
The original contributions presented in the study are included in the article/supplementary material, further inquiries can be directed to the corresponding author/s.
Ethics Statement
The animal study was reviewed and approved by Southwest University Committee on Animal Care and Use.
Author Contributions
SB and LC conceived and designed the experiments. SB, YQ, JS, JZ, and WL performed the experiments. YQ, JS, LZ, and JN analyzed the data. SB wrote the paper. All authors read and approved the final manuscript.
Funding
This work was supported by the National Natural Science Foundation of China (32002325), Chongqing Research Program of Basic and Frontier Technology (cstc2020jcy-jmsxmX0418), and Fundamental Research Funds for the Central Universities (SWU119054).
Conflict of Interest
The authors declare that the research was conducted in the absence of any commercial or financial relationships that could be construed as a potential conflict of interest.
Publisher's Note
All claims expressed in this article are solely those of the authors and do not necessarily represent those of their affiliated organizations, or those of the publisher, the editors and the reviewers. Any product that may be evaluated in this article, or claim that may be made by its manufacturer, is not guaranteed or endorsed by the publisher.
References
1. Blum SE, Heller ED, Leitner G. Long term effects of Escherichia coli mastitis. Vet J. (2014) 201:72–7. doi: 10.1016/j.tvjl.2014.04.008
2. Laird TJ, Abraham S, Jordan D, Pluske JR, Hampson DJ, Trott DJ, et al. Porcine enterotoxigenic Escherichia coli: antimicrobial resistance and development of microbial-based alternative control strategies. Vet Microbiol. (2021) 258:109117. doi: 10.1016/j.vetmic.2021.109117
3. Chen X, Liu W, Li H, Yan S, Jiang F, Cai W, et al. Whole genome sequencing analysis of avian pathogenic Escherichia coli from China. Vet Microbiol. (2021) 259:109158. doi: 10.1016/j.vetmic.2021.109158
4. Shini S, Kaiser P, Shini A, Bryden WL. Biological response of chickens (Gallus gallus domesticus) induced by corticosterone and a bacterial endotoxin. Comp Biochem Phys B. (2008) 149:324–33. doi: 10.1016/j.cbpb.2007.10.003
5. Yu G, Wang Y, Wang S, Duan C, Wei L, Gao J, et al. Effects of microbial aerosol in poultry house on meat ducks' immune function. Front Microbiol. (2016) 7:1245. doi: 10.3389/fmicb.2016.01245
6. Munyaka PM, Tactacan G, Jing M. OK, House JD, St Paul M, et al. (2013). Response of older laying hens to an Escherichia coli lipopolysaccharide challenge when fed diets with or without supplemental folic acid. Poult. Sci. 92, 105–113. doi: 10.3382/ps.2012-02579
7. Li R, Song Z, Zhao J, Huo D, Fan Z, Hou DX, et al. Dietary L-theanine alleviated lipopolysaccharide-induced immunological stress in yellow-feathered broilers. Anim Nutr. (2018) 4:265–72. doi: 10.1016/j.aninu.2018.05.002
8. Bryant CE, Spring DR, Gangloff M, Gay NJ. The molecular basis of the host response to lipopolysaccharide. Nat Rev Microbiol. (2010) 8:8–14. doi: 10.1038/nrmicro2266
9. Hennigar SR, McClung JP, Pasiakos SM. Nutritional interventions and the IL-6 response to exercise. FASEB 31 J. (2017) 3719–28. doi: 10.1096/fj.201700080R
10. Patel HJ, Patel BM. TNF-α and cancer cachexia: molecular insights and clinical implications. Life Sci. (2017) 170:56–63. doi: 10.1016/j.lfs.2016.11.033
11. Lu N, Li X, Yu J, Li Y, Wang C, Zhang L, et al. Curcumin attenuates lipopolysaccharide-induced hepatic lipid metabolism disorder by modification of m(6) A RNA methylation in piglets. Lipids. (2018) 53:53–63. doi: 10.1002/lipd.12023
12. Liu SQ, Wang LY, Liu GH, Tang DZ, Fan XX, Zhao JP, et al. Leucine alters immunoglobulin a secretion and inflammatory cytokine expression induced by lipopolysaccharide via the nuclear factor-κB pathway in intestine of chicken embryos. Animal. (2018) 12:1903–11. doi: 10.1017/S1751731117003342
13. Han H, Zhang J, Chen Y, Shen M, Yan E, Wei C, et al. Dietary taurine supplementation attenuates lipopolysaccharide-induced inflammatory responses and oxidative stress of broiler chickens at an early age. J Anim Sci. (2020) 98:skaa311. doi: 10.1093/jas/skaa311
14. Liu T, Zhang L, Joo D, Sun S. NF-κB signaling in inflammation. Signal Transduct Target Ther. (2017) 2:17023. doi: 10.1038/sigtrans.2017.23
15. Yuan L, Wang Y, Ma X, Cui X, Lu M, Guan R, et al. Sunflower seed oil combined with ginseng stem-leaf saponins as an adjuvant to enhance the immune response elicited by Newcastle disease vaccine in chickens. Vaccine. (2020) 38:5343–54. doi: 10.1016/j.vaccine.2020.05.063
16. Wei X, Su F, Su X, Hu T, Hu S. Stereospecific antioxidant effects of ginsenoside Rg3 on oxidative stress induced by cyclophosphamide in mice. Fitoterapia. (2012) 83:636–42. doi: 10.1016/j.fitote.2012.01.006
17. Su F, Xue Y, Wang Y, Zhang L, Chen W, Hu S. Protective effect of ginsenosides Rg1 and Re on lipopolysaccharide-induced sepsis by competitive binding to Toll-like receptor 4. Antimicrob Agents Ch. (2015) 59:5654–63. doi: 10.1128/AAC.01381-15
18. Kim JH, Yi Y, Kim M, Cho JY. Role of ginsenosides, the main active components of Panax ginseng, in inflammatory responses and diseases. J Ginseng Res. (2017) 41:435–43. doi: 10.1016/j.jgr.2016.08.004
19. Liu L, Shen J, Zhao C, Wang X, Yao J, Gong Y, et al. Dietary Astragalus polysaccharide alleviated immunological stress in broilers exposed to lipopolysaccharide. Int J Biol Macromol. (2015) 72:624–32. doi: 10.1016/j.ijbiomac.2014.08.057
20. Bi S, Ma X, Wang Y, Chi X, Hu S. Protective effect of ginsenoside Rg1 on oxidative damage induced by hydrogen peroxide in chicken splenic lymphocytes. Oxid Med Cell Longev. (2019) 2019:1–13. doi: 10.1155/2019/8465030
21. Yang S, Zhang J, Jiang Y, Xu YQ, Jin X, Yan SM, et al. Effects of Artemisia argyi flavonoids on growth performance and immune function in broilers challenged with lipopolysaccharide. Anim Biosci. (2021) 34:1169–80. doi: 10.5713/ab.20.0656
22. Wang, H.ongjing, Zhao, Y.izhi, Shao, J.uanjuan, and Liu, J.inglun, Liyang, and Luo. Copper or/and arsenic induces autophagy by oxidative stress-related PI3K/AKT/mTOR pathways and cascaded mitochondrial fission in chicken skeletal muscle. J Inorg Biochem. (2018) 188:1–8. doi: 10.1016/j.jinorgbio.2018.08.001
23. Li Z, Miao Z, Ding L, Teng X, Bao J. Energy metabolism disorder mediated ammonia gas-induced autophagy via AMPK/mTOR/ULK1-Beclin1 pathway in chicken livers. Ecotox Environ Safe. (2021) 217:112219. doi: 10.1016/j.ecoenv.2021.112219
24. Wan X, Zhang J, He J, Bai K, Zhang L, Wang T. Dietary enzymatically treated Artemisia annua L. supplementation alleviates liver oxidative injury of broilers reared under high ambient temperature. Int J Biometeorol. (2017) 61:1629–36. doi: 10.1007/s00484-017-1341-1
25. Leskovec J, Levart A, Nemec Svete A, Peric L, Ð*ukic Stojcic M, Žikic D, et al. Effects of supplementation with α-tocopherol, ascorbic acid, selenium, or their combination in linseed oil-enriched diets on the oxidative status in broilers. Poult Sci. (2018) 97:1641–50. doi: 10.3382/ps/pey004
26. Jazi V, Mohebodini H, Ashayerizadeh A, Shabani A, Barekatain R. Fermented soybean meal ameliorates Salmonella Typhimurium infection in young broiler chickens. Poult Sci. (2019) 98:5648–60. doi: 10.3382/ps/pez338
27. Da Rosa G, Alba DF, Silva AD, Gris A, Mendes RE, Mostardeiro VB, et al. Impact of Escherichia coli infection in broiler breeder chicks: the effect of oxidative stress on weight gain. Microb Pathogenesis. (2020) 139:103861. doi: 10.1016/j.micpath.2019.103861
28. Takahashi K, Aoki A, Takimoto T, Akiba Y. Dietary supplementation of glycine modulates inflammatory response indicators in broiler chickens. Brit J Nutr. (2008) 100:1019–28. doi: 10.1017/S0007114508966125
29. Chen Y, Zhang H, Cheng Y, Li Y, Wen C, Zhou Y. Dietary l-threonine supplementation attenuates lipopolysaccharide-induced inflammatory responses and intestinal barrier damage of broiler chickens at an early age. Brit J Nutr. (2018) 119:1254–62. doi: 10.1017/S0007114518000740
30. Groves PJ, Sharpe SM, Cox JM. Response of layer and broiler strain chickens to parenteral administration of a live Salmonella Typhimurium vaccine. Poult Sci. (2015) 94:1512–20. doi: 10.3382/ps/pev127
31. Mshelbwala FM, Ibrahim NDG, Saidu SN, Kadiri AKF, Kwanashie CN. Comparison of the clinical signs, pathological and immuohistochemical findings in visceral organs of chickens experimentally infected with Salmonella Zega through three routes. Acta Trop. (2019) 200:105123. doi: 10.1016/j.actatropica.2019.105123
32. Yu J, Chen Y, Zhai L, Zhang L, Xu Y, Wang S, et al. Antioxidative effect of ginseng stem-leaf saponins on oxidative stress induced by cyclophosphamide in chickens. Poult Sci. (2015) 94:927–33. doi: 10.3382/ps/pev055
33. Liu J, Wang S, Zhang Q, Li X, Xu S. Selenomethionine alleviates LPS-induced chicken myocardial inflammation by regulating the miR-128-3p-p38 MAPK axis and oxidative stress. Metallomics. (2020) 12:54–64. doi: 10.1039/c9mt00216b
34. Yoon S, Park J, Choi S, Lee J, Jung H, Kim T, et al. Ginsenoside Rg3 regulates s-nitrosylation of the NLRP3 inflammasome via suppression of iNOS. Biochem Bioph Res Co. (2015) 463:1184–9. doi: 10.1016/j.bbrc.2015.06.080
35. Shi Y, Wang H, Zheng M, Xu W, Yang Y, Shi F. Ginsenoside Rg3 suppresses the NLRP3 inflammasome activation through inhibition of its assembly. FASEB J. (2020) 34:208–21. doi: 10.1096/fj.201901537R
36. Brenner C, Galluzzi L, Kepp O, Kroemer G. Decoding cell death signals in liver inflammation. J Hepatol. (2013) 59:583–94. doi: 10.1016/j.jhep.2013.03.033
37. Li W, Wang J, Zhou Y, Hou J, Liu Y, Wang Y, et al. Rare ginsenoside 20(R)-Rg3 inhibits D-galactose-induced liver and kidney injury by regulating oxidative stress-induced apoptosis. Am J Chin Med. (2020) 48:1141–57. doi: 10.1142/S0192415X20500561
38. Kang A, Xie T, Zhu D, Shan J, Di L, Zheng X. Suppressive effect of ginsenoside Rg3 against lipopolysaccharide-induced depression-like behavior and neuroinflammation in mice. J Agric Food Chem. (2017) 65:6861–9. doi: 10.1021/acs.jafc.7b02386
39. Kim YM, Stone M, Hwang TH, Kim YG, Dunlevy JR, Griffin TJ, et al. SH3BP4 is a negative regulator of amino acid-Rag GTPase-mTORC1 signaling. Mol Cell. (2012) 46:833–46. doi: 10.1016/j.molcel.2012.04.007
40. Triki M, Rinaldi G, Planque M, Broekaert D, Winkelkotte AM, Maier CR, et al. mTOR signaling and SREBP activity increase FADS2 expression and can activate sapienate biosynthesis. Cell Rep. (2020) 31:107806. doi: 10.1016/j.celrep.2020.107806
41. Shang X, Lin K, Yu R, Zhu P, Zhang Y, Wang L, et al. Resveratrol protects the myocardium in sepsis by activating the phosphatidylinositol 3-kinases (PI3K)/AKT/Mammalian target of rapamycin (mTOR) pathway and inhibiting the nuclear factor-κB (NF-κB) signaling pathway. Med Sci Monit. (2019) 25:9290–8. doi: 10.12659/MSM.918369
42. Liu B, Deng X, Jiang Q, Li G, Zhang J, Zhang N, et al. Scoparone improves hepatic inflammation and autophagy in mice with nonalcoholic steatohepatitis by regulating the ROS/P38/Nrf2 axis and PI3K/AKT/mTOR pathway in macrophages. Biomed Pharmacother. (2020) 125:109895. doi: 10.1016/j.biopha.2020.109895
Keywords: ginsenoside, Escherichia coli lipopolysaccharide, broiler chicks, stress, inflammation
Citation: Bi S, Qu Y, Shao J, Zhang J, Li W, Zhang L, Ni J and Cao L (2022) Ginsenoside Rg3 Ameliorates Stress of Broiler Chicks Induced by Escherichia coli Lipopolysaccharide. Front. Vet. Sci. 9:878018. doi: 10.3389/fvets.2022.878018
Received: 17 February 2022; Accepted: 09 March 2022;
Published: 08 April 2022.
Edited by:
Ramesh Chandra Gupta, Murray State University, United StatesReviewed by:
Taniya Mitra, University of Veterinary Medicine Vienna, AustriaLv Zengpeng, China Agricultural University, China
Copyright © 2022 Bi, Qu, Shao, Zhang, Li, Zhang, Ni and Cao. This is an open-access article distributed under the terms of the Creative Commons Attribution License (CC BY). The use, distribution or reproduction in other forums is permitted, provided the original author(s) and the copyright owner(s) are credited and that the original publication in this journal is cited, in accordance with accepted academic practice. No use, distribution or reproduction is permitted which does not comply with these terms.
*Correspondence: Shicheng Bi, c2hpY2hlbmdiaUBzd3UuZWR1LmNu