- 1State Key Laboratory of Animal Nutrition, College of Animal Science and Technology, China Agricultural University, Beijing, China
- 2Department of Animal Sciences, Faculty of Veterinary and Animal Sciences, University of Agriculture, Dera Ismail Khan, Pakistan
Aims: This study aims to evaluate the effect of lactic acid bacteria (LAB) and LAB-molasses (LAB + M) combination on the fermentation quality, chemical composition, physicochemical properties, in vitro degradability of rice straw and the characteristics of rumen microbial colonization on rice straw surface.
Methods and Results: There were three pretreatments, including control (not treated, Con), treated with LAB, or LAB + M. The results showed that both LAB and LAB + M treatments altered the physical and chemical structures of rice straw and were revealed by scanning electron microscopy (SEM) and X-ray diffraction analysis (XRD) spectroscopy, respectively. Moreover, both LAB and LAB + M pretreated rice straw increased the crude protein (CP) content, dry matter (DM) recovery, and in vitro digestibility and decreased the pH value, neutral detergent fiber (NDF), and acid detergent fiber (ADF) contents. The LAB + M pretreated rice straw increased the gas production (GP72) and rumen microbial colonization on the rice straw surface.
Conclusions: It is observed that LAB + M treatment could increase digestibility and the rumen microbial colonization on the rice straw surface. Therefore, LAB + M treatment can provide an alternative strategy to improve the quality of rice straw. Significance and impact of the study: This study provides an optimal pretreatment to improve the rice straw digestibility and rumen microbial colonization.
Introduction
Rice straw is one of the most abundant crop residues in the world, especially in China. It is commonly used as the source of roughage in ruminants' nutrition due to its richness and low cost. However, the recalcitrant cellulose—hemicellulose—lignin structure protects the rice straw biomass from the access of microorganisms (1), results in low degradation during ruminal fermentation, and subsequently limits intake in animals (2). Increasing the degradation of rice straw would help to increase the utilization of this abundant agricultural by-product in ruminants and hence reduce the status of shortage of high-quality forage.
The lactic acid bacteria (LAB) plays a role in the preservation and fermentation of forage crops within inoculated silages (3). LAB help to suppress the growth of spoilage microorganisms by reducing pH levels in fermented forages (4). Previous studies have shown that exogenous LAB can improve the quality of rice straw (5) and grass (6) silages. However, the insufficient contents of water-soluble carbohydrates (WSCs) in raw rice straw may lead to the accumulation of low lactic acid and increased pH value, allowing undesirable microorganisms such as clostridia to grow in the silage (7). Therefore, it might be helpful to add cheap sources of exogenous WSC, such as molasses (8). Sufficient WSC not only provides sufficient substrates for LAB fermentation but also increases the nutritional quality of rice straw silage.
Ruminal microorganisms are the most dominant domain contributing to the digestion and conversion of feedstuff to volatile fatty acids and microbial proteins (9). The attachment of microorganisms to the consumed forage is a key step in the forage degradation process and affects the degradation of the dietary (10). However, many studies have mainly focused on the changes in the colonization of microorganisms in the rumen degrading process of roughage that has not been pretreated. For example, Huws et al. reported that colonization of bacteria in the freshly ingested perennial ryegrass (11). However, not a single study has reported the rumen microbial colonization after pretreatment of roughage raw materials. Moreover, the physical structure and chemical characteristics of the feed particles are the key factors that affect microbial colonization (12). Therefore, further research on the changes in microbial colonization and the digestion of roughage after pretreatment is very important for the improvement in the efficiency of feed nutrient utilization by ruminants.
In this study, LAB or LAB + M pretreated rice straw with respect to its fermentation quality, chemical composition, physicochemical properties, in vitro degradability of rice straw, and the characteristics of rumen microbial colonization on rice straw surface.
Materials and Methods
All animal procedures used in this study were reviewed and approved by the Animal Care Committee of the College of Animal Science and Technology of China Agriculture University (protocol number: 2013-5-LZ).
Substrate and Treatment
Rice straw was collected from suburban farmland in Henan province, China. Mature whole rice plant was thrashed to remove the grain and the rice straw was sun-cured. The rice straw was cut into 3–5 cm using a guillotine cutter. The LAB and LAB + M were evenly sprayed on the rice straw, as per their respective treatments. For each treatment, the amounts of molasses and water were calculated in advance and weighed, whereas materials were separately added into the distilled water to adjust the moisture content to 70%.
The LAB were (1) a mixture of both homofermentative and heterofermentative LAB inoculants that were consisted of three strains of Lactobacillus (L. plantarum PS-8, L. plantarum PS-F, and L. buchneri HM-01), whose details have been described previously (2, 13) molasses (purchased from the Hebei Shuntong Encyclopedia Trading Co., Ltd., China). The three treatments were as follows: rice straw treated without (control, Con; supplemented with distilled water to adjust the moisture content to 70%) or with LAB (added at 1 × 1011 CFU/g of fresh material) or LAB + M group (mixture of molasses and LAB, molasses was added to 3% of the fresh material). The rice straw was ensiled in triplicate for each treatment in laboratory polyethylene bags (25 cm by 35 cm; Beijing Shengya Yuda Biological Technology Co., Ltd, Beijing, China) and sealed by a food vacuum sealing machine (Konka KZ-ZK007; Dongguan Yijian Packaging Machinery Co. Ltd, Dongguan, China). All forages were ensiled for 7, 15, 30, and 45 days before opening.
Chemical Composition and in vitro Digestibility Analyses
Dry matter and crude protein contents were determined according to AOAC methods (14). The weight and DM content before and after ensiling were weighed to calculate the rate of DM loss, whereas NDF and ADF were determined using the procedures adopted by Van Soest et al. (15).
Rumen fluids from three healthy Holstein cows were collected 2 h after the morning feeding, mixed, and placed in a 39°C pretemperature vacuum flask and then immediately transported to the laboratory. Cows were fed a TMR containing 5.6% oat hay, 11.5% alfalfa hay, 8.3% alfalfa silage, 24.5% corn silage, and 50.1% concentrate (13.7% of stem-flaked corn, 5.0% of corn, 8.4% of soybean meal, 5.2% of soybean hull, 4.4% of corn DDGS, 3.3% of sprayed corn skin, 3.3% of cottonseed meal, 2.9% of molasses, 0.5% of Berg + Schmidt, 0.3% of XP XPC, 2.4% of premix, 0.4% of NaHPO3, and 0.3% OPTIGEN of DM) which offered three times a day (07:00, 14:00, and 18.30). Cows had free access to water throughout the day.
The in vitro gas production was determined using an automated trace gas recording system (AGRS) for microbial fermentation, as described previously (16). Briefly, 500 mg (DM basis) of representative samples (rice straw samples had been fermented for 45 days) (6 replicates) of each treatment group was weighed and placed in 120-ml glass bottles. About 50-ml of a freshly prepared buffer solution was added to each bottle (17). All bottles were purged with anaerobic N2 for 5 s, sealed with rubber plug and Hungate screw caps, and individually connected with medical plastic infusion pipes to the AGRS, according to the procedure introduced by Zhang and Yang (18). All the bottles were incubated at 39°C for 72 h, and each batch culture system run had 6 bottles of samples for blank correction. The bottles were removed from the AGRS system after 48 h of incubation. The pH value of the culture medium was immediately determined. The in vitro digestibility of DM (IVDMD), NDF (NDFD), and ADF (ADFD) was calculated using differential subtraction according to the DM content of substrates before and after in vitro incubation.
Structural Analyses
The morphological and structural images of the rice straw were obtained by emission scanning electron microscopy (SEM, ASU 3500, Japan) according to the manufacturer's instructions. Briefly, morphological changes in biomass before and after pretreatment with additives were observed at a magnification of 1,500. Prior to imaging, the samples (rice straw samples had been fermented for 45 days) were sputter-coated with platinum to make them electrically conductive.
The X-ray diffraction (XRD) method was applied for the detection of cellulose crystallinity index (CrI) of samples (rice straw samples had been fermented for 45 days) as described by Zhang et al. (19). The XRD was conducted with a Siemens D-5000 diffractometer (Bruker, Ettlingen, Germany), and Cu-K radiation was generated at 40 kV and 20 mA. Samples were scanned from 3° to 40° with a step size of 0.02 and 3 s per step. The cellulose crystallinity index (CrI) was calculated using the following formula (20):
where I002 is the scattered intensity at the main peak for cellulose type I and Iam is the scattered intensity due to the amorphous portion evaluated as the minimum intensity between the main and secondary peaks.
In situ Rumen Incubation
The experimental procedures of in situ rumen incubation were done according to method exercised by Gharechahi et al. (21). In brief, rice straw air-dried samples that had been fermented for 45 days were ground into a 2-mm sieve using a Wiley mill (KRT-34; KunJie, Beijing, China). About 5 g of the milled samples was weighed and placed in nylon bags (8 × 16 cm; pore size = 45 mm) in six replications. These bags were incubated for 0.5, 4, 12, and 24 h in the rumen of three fistulas cows (two replications per cow). After removing from the rumen, the bags were washed with sterile saline to remove loosely attached microbiota and then immediately frozen on dry ice and transported to the laboratory for storage at −80°C until DNA extraction.
DNA Extraction and Quantitative Real-Time PCR Analysis
Total microbial genomic DNAs were extracted from 200 mg of the rumen-incubated rice straw sample. The DNA was extracted according to the protocol for pathogen detection in stool using an EZNA Stool DNA kit (Omega Biotek, Norcross, GA, US). For bacteria, the V3-V4 variable region of the 16S rDNA was targeted using primers Eub338F (ACTCCTACGGGAGGCAGCAG) and Eub806R (GGACTACHVGGGTWTCTAAT). Quantitative real-time PCR (q-PCR) was performed according to the procedures described by Jiao et al. (22). A standard curve was generated using plasmid DNA containing the exact 16S/18S rRNA gene inserts, and the standard curve met the following requirements: R2 > 0.99. The qPCR assay was performed to generate fragments of 460 base pairs (bp) suitable for paired-end sequencing on the Illumina Miseq system (Shanghai Majorbio Bio-pharm Technology Co., Ltd). The reactions were performed in a 20 μl mixture containing 10 μl of 2X Taq Plus Master Mix, 0.8 μl of each primer (5 μM), 7.4% of ddH2O, and 1 μl of each reaction which was used as a template of PCR. Each sample was performed in triplicate for PCRs.
Data Analysis
The cumulative gas production (GP72) (mL/g) data were recorded using the AGRS system and fitted to the Groot model as per Equation (1) (23).
“A” is the asymptotic gas production (ml/g); “B” is a sharpness parameter determining the curve's shape; “C” is the time (h) at which half of A is reached; and “t” is in vitro incubation time (h).
The results of the 6 bottles per treatment within each run were averaged and then analyzed using a mixed model with SPSS 24.0 (SPSS Inc., Chicago, IL). The two-way ANOVA analysis was performed to examine the effect of treatment with treated groups and the ensiling period on the chemical composition of rice straw. Duncan's multiple comparison method was carried out to compare the differences between the means; p <0.05 was used to show the significance levels. The DNA sequencing data were analyzed on a free online platform of Majorbio tools https://cloud.majorbio.com/page/project/p.html.
Results
Physical Structure and Physicochemical Properties
The scanning electron microscopy images showed that LAB and LAB + M treatments disrupted the physical structure of rice straw in this study (Figure 1), whereas the morphology of untreated rice straw exhibited a compact, rigid, and angular fibril structure with pilling. In addition, the rice straw treated with both LAB and LAB + M reflected somewhat melted and patchy surfaces.
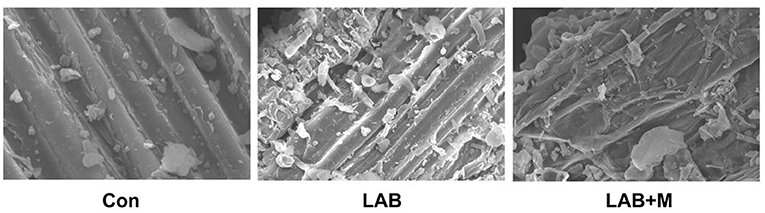
Figure 1. SEM imagines of rice straw residues obtained from treatment with LAB or LAB + M. Con: no additive, control; LAB: added LAB, LAB + M: a combination of LAB and molasses.
To reveal the changes in cellulose structure, XRD diffraction data were acquired (Figure 2). The CrI of LAB + M was decreased (p <0.05) compared to Con.
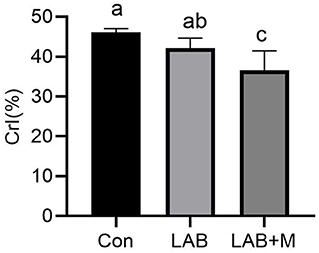
Figure 2. Effect of LAB or LAB + M treatments on the Cellulose crystalline index (CrI, %) of rice straw. Con: no additive, control; LAB: added LAB, LAB + M: a combination of LAB and molasses.
The NDF (p <0.001) and ADF (p <0.001) contents (Table 1) were decreased in LAB and LAB + M treatments compared to Con. While the content of DM was lowest in LAB + M (p <0.05), no difference was found between LAB and Con (p > 0.05). While the content of CP was increased in LAB and LAB + M treatments compared to Con (p <0.001), no difference was found between LAB and LAB + M (p > 0.05). Meanwhile, after 30 days of ensiling, the NDF (p <0.001) and ADF (p <0.001) contents of rice straw were lowest in LAB and LAB + M treatments. Additionally, the content of CP was stable at 15 days.
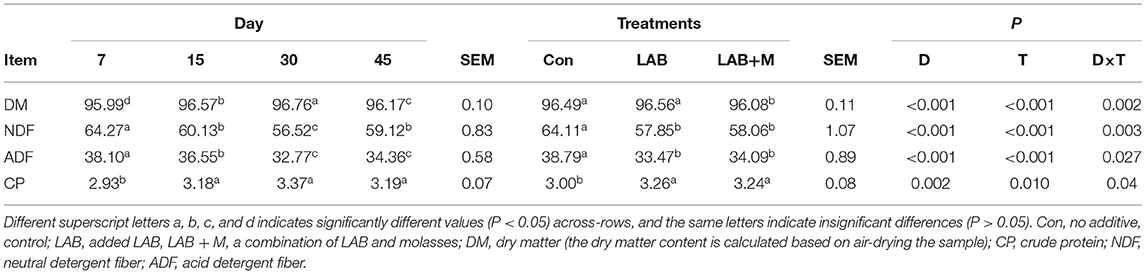
Table 1. Effect of LAB or LAB + M treatments and anaerobic storage days on the chemical composition of rice straw.
In general, with the extension of the fermentation days, the pH value of each group showed a downward trend (Figure 3). The LAB (3.77) (p <0.001) and LAB + M (3.41) (p <0.001) treatments were decreased pH value in rice straw after ensiling for 30 days. The LAB and LAB + M treatments were shown lower pH values compared to Con (p <0.05) after ensiling for 45 days. Specifically, the pH values were lower 4.0 in LAB + M group.
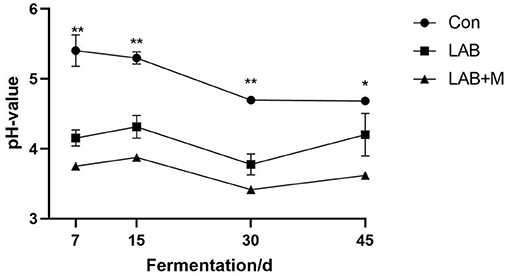
Figure 3. Effect of LAB or LAB + M treatments on the pH value of rice straw. Con: no additive, control; LAB: added LAB, LAB + M: a combination of LAB and molasses. While * and ** indicated the significant correlations at p < 0.05 and p < 0.01, respectively.
As illustrated in Figure 4, dry matter recovery showed a dynamic change during the whole silage period that first decreased and then keep stable. After 45 days, the dry matter recovery was higher in LAB and LAB + M groups (p <0.001) in comparison with Con.
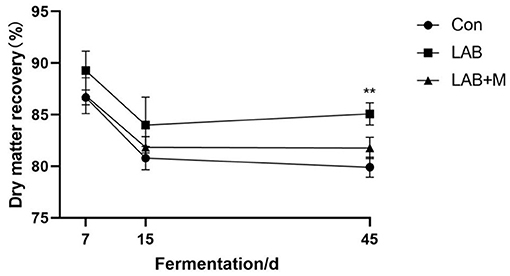
Figure 4. Effect of LAB or LAB + M treatments on the Dry matter recovery (%) of rice straw after anaerobic fermentation 7, 15 and 45 days. Con: no additive, control; LAB: added LAB, LAB + M: a combination of LAB and molasses, while * and ** indicated the significant correlations at p < 0.05 and p < 0.01, respectively.
In vitro Ruminal Degradation and Total gas Production
The LAB + M and LAB treatments were increased DM (p <0.001), NDF (p <0.001), and ADF (p <0.001) degradation (Table 2) of rice straw compared to Con. The LAB + M was increased gas production (p = 0.001) over the 72-h incubation period and asymptotic gas production (p = 0.003) compared to LAB and Con, whereas no difference was found between LAB and Con treatments (p > 0.05) for gas production and asymptotic gas production.
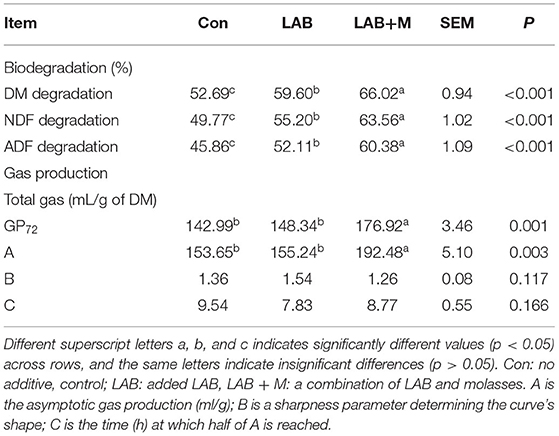
Table 2. Effects of LAB or LAB + M treatments on the biodegradation and gas production after 72 h of in vitro ruminal incubation of rice straw.
Particle-Attached Bacterial Density
Total bacterial population in the rice straw samples was estimated by real-time PCR by measuring the total copy number of bacterial 16S rRNA genes (Figure 5). The highest microbial colonization in the samples of rumen-incubated rice straw was observed at 24 h of incubation for LAB +M than LAB and Con treatments. Higher numbers of colonization bacteria (p <0.05) were also observed in the LAB + M treatment than Con and LAB groups at 4 to 12 h of incubation. Interestingly, in the Con, the microbial colonization of rice straw surface was the highest at 0.5 h (p <0.05) and decreased (p <0.05) after 4 h in the rumen.
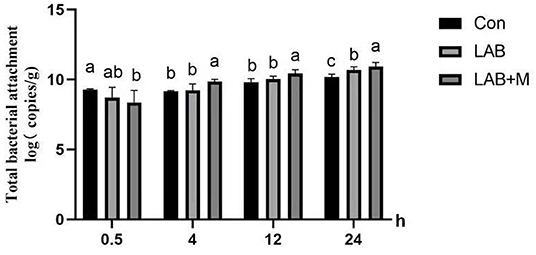
Figure 5. Effect of LAB or LAB + M treatments on rice straw's microbial colonization (MC). Different lowercase letters a, b, and c above the columns indicate significant differences (p < 0.05), and the same letters indicate no significant differences (p > 0.05). Con: no additive, control; LAB: added LAB, LAB + M: a combination of LAB and molasses.
Discussion
Physical Structure and Physicochemical Properties
The degradation difficult of lignocellulose is complex cell wall structure of cellulose–hemicellulose–lignin (24). In this study, the morphology of untreated rice straw exhibited a compact, rigid, and angular fibril structure with pilling, whereas rice straw treated with LAB and LAB + M reflected somewhat melted and patchy surfaces. It indicates that treated rice straw could provide more rumen microbial colonization sites. Notably, the increase in microbial colonization on the surface of rice straw had a positive effect on degradation (25). Furthermore, the relative lower acidic environment may cause the damage to the cell wall structure of rice straw (26). Indeed, the LAB and LAB + M treatments have shown lower pH values in this study (Figure 3); especially, the pH values was lower 4.0 in LAB + M group, whereas the straw surface damage in LAB + M group was the most obvious compared to Con and LAB groups. Also, a study reported that acid pretreatment resulted into solubility of 70–80% of xylan in barley straw (27), which imply that a lower acidic environment could soluble the hemicellulose in forage.
To reveal the changes in cellulose structure, XRD diffraction data were acquired. Unlike starch and hemicellulose, cellulose has a crystalline structure. Its crystallinity is believed to play a major role in its biological conversion. Cellulose CrI is the important parameter on cellulose structural features (28). In this study, the CrI of LAB + M was significantly decreased as expected compared to Con. Notably, the lower CrI could be attributed to the partial hydrolysis of cellulose, reduction in the crystalline material, and increase in the amorphous substances, and suggestion has a positive effect on fiber degradation (29).
The chemical composition in forage is related to forage quality and degradation (30). In this study, the removal of hemicellulose from the cellulose–hemicellulose–lignin structure led to a reduction in NDF and ADF contents in rice straw treated with LAB and LAB + M. Notably, the low NDF and ADF were associated with higher feed quality and degradation, as well as higher dry matter intake (31). Similar results were obtained in rice straw silage, in which LAB + M reduced the NDF and ADF content of rice straw silage (32). This may be due to the enzymes secreted by microbes degrading the crude fiber during fermentation process (33). Meanwhile, after 30 days of ensiling, the NDF and ADF contents were lowest in LAB and LAB + M treatments. In a previous study, ensiling of grass for 7 to 28 days resulted in the degradation of structural carbohydrates by acid hydrolysis at low pH (34). On the other hand, the CP content was significantly increased in the LAB and LAB +M treatments. This might be a suggestion that rice straw treated with LAB and LAB +M rice straw has a positive effect on degradation.
In this study, LAB (3.77) and LAB + M (3.41) treatments decreased the pH value in rice straw after ensiling for 30 days. Notably, the pH values might be used as an indicator to roughly monitor the auto-hydrolysis following pretreatment with feed additives, contributing to the screening of optimum conditions for different kinds of feedstock (35). Moreover, a pH of 4.0 may inhibit microbial activity as well as provide long-term preservation or inhibit downstream biochemical processes (36). Pretreatment biomass losses should be taken into account, which would affect the soluble sugars yield (37). In this study, dry matter recovery showed a dynamic change during the whole silage period that first decreased and then keep stable. This might be attributed to more lignocellulose undergoing hydrolysis, and more volatile products such as organic acids and compounds such as furfural are generated (38). The dry matter recovery is higher in LAB and LAB + M groups than Con after 45 days, and it implies that more nutrition was retained in rice straw after pretreated by LAB or LAB + M.
In vitro Ruminal Degradation and Total gas Production
The release of hemicellulose from the cell wall matrix promotes enzymatic hydrolysis and fermentation of the feed substrate. Neutral detergent soluble, including water-soluble carbohydrates (WSC), proteins, and other extracts, can be degraded easily by microorganisms (39). In this study, LAB and LAB + M treatments increased DM, NDF, and ADF degradation of rice straw, and LAB + M increased the gas production over the 72-h incubation period, asymptotic gas production. Notably, these changes proved that LAB or LAB + M treatments could improve the nutritional value of rice straw.
In vitro gas production has been used to predict the rumen degradability and metabolizable energy of different animal feeds (40). A strong correlation between nutritional content and in vitro cumulative gas production exists, and these parameters have gained wide acceptance in the nutritional evaluation of animal feeds (41–43). In vitro gas production is highly dependent on the availability of soluble fractions, which favor ruminal fermentation at the early fermentation stages (44). In this study, the LAB + M group significantly increased the GP72 of rice straw, which suggests that LAB + M treatment could improve the nutritional value of rice straw.
Pretreatment prepares the carbohydrates, particularly cellulose, for an enzymatic or microbial attack. It is well established that CrI is among the parameters that are widely measured and related to the bioconversion of the lignocelluloses (45). Many studies have shown that CrI has a negative effect on digestibility (46, 47). In this study, the LAB + M group significantly reduced the CrI of rice straw, which partially explained the improved digestibility in rice straw.
Particle-Attached Bacterial Density
Normally, bacteria attached to the feed particles account for about 75% of the total microorganisms in the rumen (48). This indicates that the bacteria attached to the feed particles play a vital role in the digestion and utilization of feed. The highest microbial colonization on the samples of rumen-incubated rice straw was observed at 24 h of incubation, and the LAB + M was highest. Higher microbial colonization was also observed in the LAB + M group than other groups from 4 to 12 h of rumen incubation. The results imply that the degradation of rice straw in the rumen mainly occurs within 24 h. Indeed, the degradation of feed incubated in rumen for 24 h was related to the nutritional value of the feed (49). Interestingly, in control group, the microbial colonization on the rice straw was the highest at 0.5 h. This suggested that the turning point of microbial colonization of low quality pasture was 0.5 h (50).
The rice straw incubated with LAB + M showed the most significant changes in its structure and hence had a larger surface area that allowed for more microbial attachment sites. Unfortunately, we incubated the samples in the rumen for only 24 h. Hence, we cannot independently state whether degradation of rice straw in the rumen could have increased or decreased thereafter. Thus, the LAB or LAB + M pretreatments of rice straw were significantly increased the degradation by ruminal microbiome in this study. The LAB or LAB + M not only destructs the structure of rice straw and provides more rumen microbial colonization sites but also changes the chemical composition, such as decreased NDF, ADF and CrI contents, and increased CP content of rice straw. Importantly, these changes could improve the degradation of rice straw. Indeed, the results of in vitro digestion and gas production of rice straw confirmed it. Lactic acid bacteria and molasses in this study may be used to develop large-scale processes to improve the nutritional value of rice straw as forage for ruminants.
Data Availability Statement
The raw data supporting the conclusions of this article will be made available by the authors, without undue reservation.
Ethics Statement
The animal study was reviewed and approved by all animal procedures used in the current study were reviewed and approved by the Animal Care Committee of the College of Animal Science and Technology of China Agriculture University (Protocol number: 2013-5-LZ).
Author Contributions
ZC, XC, and YM mainly designed this experiment. The animal experiment was conducted by XC and YM. Data were collected and analyzed by XC and YM. The manuscript was mainly written by YM and edited by JX, GA, MK, YW, ZC, and SL. All authors contributed to the article and approved the submitted version.
Funding
This work was funded by the 2115 Talent Development Program of China Agricultural University.
Conflict of Interest
The authors declare that the research was conducted in the absence of any commercial or financial relationships that could be construed as a potential conflict of interest.
Publisher's Note
All claims expressed in this article are solely those of the authors and do not necessarily represent those of their affiliated organizations, or those of the publisher, the editors and the reviewers. Any product that may be evaluated in this article, or claim that may be made by its manufacturer, is not guaranteed or endorsed by the publisher.
Acknowledgments
We thank the students from China Agricultural University (Beijing, P. R. China) and the rice straw from Zhulin Agriculture Co., Ltd (Gushi, Henan, P. R. China).
References
1. Yu Q, Zhuang X, Wang W, Qi W, Wang Q, Tan X, et al. Hemicellulose and lignin removal to improve the enzymatic digestibility and ethanol production. Biomass Bioenergy. (2016) 94:105–9. doi: 10.1016/j.biombioe.2016.08.005
2. Chen Y, Gong X, Huang Y, Jiang M, Zhan K, Lin M, et al. Growth performance, rumen fermentation and inflammatory response on holstein growing cattle treated with low and high non-fibrous carbohydrate to neutral detergent fiber ratio pelleted total mixed ration. Animals. (2022) 12:12081036. doi: 10.3390/ani12081036
3. Guo L, Yao D, Li D, Lin Y, Yang F. Effects of lactic acid bacteria isolated from rumen fluid and feces of dairy cows on fermentation quality, microbial community, and in vitro digestibility of alfalfa silage. Front Microbiol. (2020) 10:2998–3312. doi: 10.3389/fmicb.2019.02998
4. Blajman JE, Páez R, Vinderola CG, Lingua MS, Signorini ML. A meta-analysis on the effectiveness of homofermentative and heterofermentative lactic acid bacteria for corn silage. J Appl Microbiol. (2018) 125:1655–69. doi: 10.1111/jam.14084
5. Kholif AE, Gouda GA, Patra AK. The sustainable mitigation of in vitro ruminal biogas emissions by ensiling date palm leaves and rice straw with lactic acid bacteria and Pleurotus ostreatus for cleaner livestock production. J Appl Microbiol. (2021) 30:2925–39. doi: 10.1111/jam.15432
6. Liu B, Huan H, Gu H, Xu N, Shen Q, Ding C. Dynamics of a microbial community during ensiling and upon aerobic exposure in lactic acid bacteria inoculation-treated and untreated barley silages. Bioresour Technol. (2019) 273:212–9. doi: 10.1016/j.biortech.2018.10.041
7. Yan Y, Li X, Guan H, Huang L, Ma X, Peng Y, et al. Microbial community and fermentation characteristic of Italian ryegrass silage prepared with corn stover and lactic acid bacteria. Bioresour Technol. (2019) 279:166–73. doi: 10.1016/j.biortech.2019.01.107
8. Yuan XJ, Dong ZH, Tajebe DS, Wen AY, Zhu X, Rong T, et al. Adding distiller's grains and molasses on fermentation quality of rice straw silages. Cincia Rural. (2016) 46:2235–40. doi: 10.1590/0103-8478cr20150851
9. Kim H, Lee I, Kwon Y, Kim BC, Su H, Lee JH, et al. Immobilization of glucose oxidase into polyaniline nanofiber matrix for biofuel cell applications. Biosens Bioelectron. (2011) 26:3908–13. doi: 10.1016/j.bios.2011.03.008
10. Mcallister TA, Bae HD, Jones GA, Cheng KJ. Microbial attachment and feed digestion in the rumen. J Anim Sci. (1994) 11:343–72. doi: 10.2527/1994.72113004x
11. Huws SA, Theodorou LA. Successional colonization of perennial ryegrass by rumen bacteria. Lett Appl Microbiol. (2013) 56:343–54. doi: 10.1111/lam.12033
12. Yang HE, Son YS, Beauchemin KA. Effects of exogenous enzymes on ruminal fermentation and degradability of alfalfa hay and rice straw. Asian Australas J Anim Sci. (2011) 24:56–64. doi: 10.5713/ajas.2011.90369
13. Bao W, Mi Z, Xu H, Zheng Y, Kwok LY, Zhang H, et al. Assessing quality of Medicago sativa silage by monitoring bacterial composition with single molecule, real-time sequencing technology and various physiological parameters. Sci Rep. (2016) 6:28358. doi: 10.1038/srep28358
15. Van Soest PJ, Robertson JB, Lewis BA. Methods for dietary fiber, neutral detergent fiber, and nonstarch polysaccharides in relation to animal nutrition. J Dairy Sci. (1991) 74:3583–97. doi: 10.3168/jds.S0022-0302(91)78551-2
16. Bai S, Cao ZJ, Cao BB, Yang HJ, Li SL, Liu JX. Effects of different forage combinations in total mixed rations on in vitro gas production kinetics, ruminal and milk fatty acid profiles of lactating cows. Anim Sci J. (2018) 89:1261–70. doi: 10.1111/asj.13036
17. Menke KH, Steingass H. Estimation of the energetic feed value obtained from chemical analysis and in vitro gas production using rumen fluid. Anim Res Dev. (1988) 13:34–7.
18. Zhang DF, Yang HJ. In vitro ruminal methanogenesis of a hay-rich substrate in response to different combination supplements of nitrocompounds; pyromellitic diimide and 2-bromoethanesulphonate. Anim Feed Sci Technol. (2011) 163:20–32. doi: 10.1016/j.anifeedsci.2010.09.019
19. Zhang W, Yi Z, Huang J, Li F, Hao B, Li M, et al. Three lignocellulose features that distinctively affect biomass enzymatic digestibility under NaOH and H2SO4 pretreatments in Miscanthus. Bioresour Technol. (2013) 130:30–7. doi: 10.1016/j.biortech.2012.12.029
20. Segal L, Creely JJ, Martin AE, Conrad CM. An empirical method for estimating the degree of crystallinity of native cellulose using the x-ray diffractometer. Text Res J. (1959) 29:786–94. doi: 10.1177/004051755902901003
21. Gharechahi J, Vahidi MF, Ding XZ, Han JL, Salekdeh GH. Temporal changes in microbial communities attached to forages with different lignocellulosic compositions in cattle rumen. FEMS Microbiol Ecol. (2020) 96:117–234. doi: 10.1093/femsec/fiaa069
22. Jiao J, Wang P, He Z, Tang S, Zhou C, Han X, et al. In vitro evaluation on neutral detergent fiber and cellulose digestion by post-ruminal microorganisms in goats. J Sci Food Agric. (2014) 94:1745–52. doi: 10.1002/jsfa.6485
23. Zheng Y, Xue S, Zhao Y, Li S. Effect of cassava residue substituting for crushed maize on in vitro ruminal fermentation characteristics of dairy cows at mid-lactation. Animals. (2020) 10:893. doi: 10.3390/ani10050893
24. Jie X, Xu X, Yue L, Li H, Liu H. Effect of microbiological inoculants DN-1 on lignocellulose degradation during co-composting of cattle manure with rice straw monitored by FTIR and SEM. Environ Prog Sustain. (2016) 35:112–34. doi: 10.1002/ep.12222
25. Bleuze L, Chabbert B, Lashermes G, Recous S. Hemp harvest time impacts on the dynamics of microbial colonization and hemp stems degradation during dew retting. Ind Crops Prod. (2020) 145:112122. doi: 10.1016/j.indcrop.2020.112122
26. Lavarack BP, Griffin GJ, Rodman D. The acid hydrolysis of sugarcane bagasse hemicellulose to produce xylose, arabinose, glucose and other products. Biomass Bioenergy. (2002) 23:367–80. doi: 10.1016/S0961-9534(02)00066-1
27. Martínez PM, Bakker R, Harmsen P, Gruppen H, Kabel M. Importance of acid or alkali concentration on the removal of xylan and lignin for enzymatic cellulose hydrolysis. Ind Crops Prod. (2015) 64:88–96. doi: 10.1016/j.indcrop.2014.10.031
28. Li Z, O'Dwyer J, Chang VS, Granda CB, Holtzapple MT. Structural features affecting biomass enzymatic digestibility. Bioresour Technol. (2008) 99:3817–28. doi: 10.1016/j.biortech.2007.07.033
29. Karimi K, Taherzadeh MJ. A critical review of analytical methods in pretreatment of lignocelluloses: composition, imaging, and crystallinity. Bioresour Technol. (2016) 200:1008–18. doi: 10.1016/j.biortech.2015.11.022
30. Amorim DS, Edvan RL, Nascimento R, Bezerra LR, Oliveira R. Sesame production and composition compared with conventional forages. Chil J Agr Res. (2019) 79:586–95. doi: 10.4067/S0718-58392019000400586
31. Ni K, Wang Y, Pang H, Cai Y. Effect of cellulase and lactic acid bacteria on fermentation quality and chemical composition of wheat straw silage. Am J Plant Sci. (2014) 5:1877–84. doi: 10.4236/ajps.2014.513201
32. Tian J, Xu N, Liu B, Huan H, Ding C. Interaction effect of silo density and additives on the fermentation quality, microbial counts, chemical composition and in vitro degradability of rice straw silage. Bioresour Technol. (2019) 297:122412. doi: 10.1016/j.biortech.2019.122412
33. Ashbell G, Weinberg ZG. The effect of microbial inoculants applied at ensiling on sorghum silage characteristics and aerobic stability. Agric Sci China. (2002) 47:1174–9.
34. Dewar WA, Mcdonald P, Whittenbury R. The hydrolysis of grass hemicelluloses during ensilage. J Sci Food Agric. (2010) 14:411–7. doi: 10.1002/jsfa.2740140610
35. Junior M, Pas J, Erickson J, Pas PM, Silveira M, Aguiar AD, et al. Nutritive value and fermentation characteristics of silages produced from different sweet sorghum plant components with or without microbial inoculation - ScienceDirect. Appl Anim Sci. (2020) 36:777–83. doi: 10.15232/aas.2020-02027
36. Kaewpila C, Gunun P, Kesorn P, Subepang S, Sumadong P. Improving ensiling characteristics by adding lactic acid bacteria modifies in vitro digestibility and methane production of foragesorghum mixture silage. Sci Rep. (2021) 11:34–45. doi: 10.1038/s41598-021-81505-z
37. Garlock RJ, Balan V, Dale BE, Pallapolu VR, Lee YY, Kim Y, et al. Comparative material balances around pretreatment technologies for the conversion of switchgrass to soluble sugars. Bioresour Technol. (2011) 102:11063–71. doi: 10.1016/j.biortech.2011.04.002
38. Oliveira A. Meta-analysis of the effect of homolactic and facultative heterolactic bacteria inoculation on silage quality: III Dry matter recovery, chemical composition and in-vitro digestibility. J Anim Sci. (2016) 94:310–1. doi: 10.2527/jam2016-0650
39. Leiva E, Hall MB, Horn HV. Performance of dairy cattle fed citrus pulp or corn products as sources of neutral detergent-soluble carbohydrates. J Dairy Sci. (2000) 83:2866–75. doi: 10.3168/jds.S0022-0302(00)75187-3
40. Contreras-Govea FE, Muck RE, Mertens DR, Weimer PJ. Microbial inoculant effects on silage and in vitro ruminal fermentation, and microbial biomass estimation for alfalfa, bmr corn, corn silages. Anim Feed Sci Technol. (2011) 163:2–10. doi: 10.1016/j.anifeedsci.2010.09.015
41. Vastolo A, Calabrò S, Pacifico S, Koura BI, Cutrignelli MI. Chemical and nutritional characteristics of Cannabis sativa L. co-products. J Anim Physiol Anim Nutr. (2021) 1:1–9. doi: 10.1111/jpn.13557
42. Ikanya LW, Maina JG, Gachuiri CK. Chemical composition and digestibility of preferred forage species by lactating somali camels in Kenya. Rangeland Ecol Manag. (2022) 80:61–7. doi: 10.1016/j.rama.2021.10.003
43. Moharrery A, Toghyani E. Evaluation of nutritional properties of alfalfa and sainfoin forages by gas production techniques. J Livest Sci Technol. (2013) 1:1–9.
44. Chen YY, Wang YL, Wang WK, Zhang ZW, Si XM, Cao ZJ, et al. Beneficial effect of Rhodopseudomonas palustris on in vitro rumen digestion and fermentation. Benef Microbes. (2020) 11:91–9. doi: 10.3920/BM2019.0044
45. An YX, Zong MH, Wu H, Li N. Pretreatment of lignocellulosic biomass with renewable cholinium ionic liquids: biomass fractionation, enzymatic digestion and ionic liquid reuse. Bioresour Technol. (2015) 192:165–71. doi: 10.1016/j.biortech.2015.05.064
46. Mihranyan A. Engineering of native cellulose structure for pharmaceutical applications: influence of cellulose crystallinity index, surface area and pore volume on sorption phenomena. Acta Univ Ups. (2005) 3:17–23.
47. Ma XJ, Cao SL, Lin L, Luo XL, Hu HC, Chen LH, et al. Hydrothermal pretreatment of bamboo and cellulose degradation. Bioresour Technol. (2013) 148:408–13. doi: 10.1016/j.biortech.2013.09.021
48. Forsberg CW, Lam K. Use of Adenosine 5′-Triphosphate as an indicator of the microbiota biomass in rumen contents. Appl Environ Microbiol. (1977) 33:528–37. doi: 10.1128/aem.33.3.528-537.1977
49. Cheng Y, Ying W, Li Y, Zhang Y, Liu T, Wang Y, et al. Progressive colonization of bacteria and degradation of rice straw in the rumen by illumina sequencing. Front Microbiol. (2017) 8:2165. doi: 10.3389/fmicb.2017.02165
Keywords: lactic acid bacteria, molasses, rumen microbial colonization, rice straw, in vitro digestibility
Citation: Chen X, Ma Y, Khan MZ, Xiao J, Alugongo GM, Li S, Wang Y and Cao Z (2022) A Combination of Lactic Acid Bacteria and Molasses Improves Fermentation Quality, Chemical Composition, Physicochemical Structure, in vitro Degradability and Rumen Microbiota Colonization of Rice Straw. Front. Vet. Sci. 9:900764. doi: 10.3389/fvets.2022.900764
Received: 21 March 2022; Accepted: 06 May 2022;
Published: 08 June 2022.
Edited by:
Bruno Solis-Cruz, Universidad Nacional Autonóma de México, MexicoReviewed by:
Suban Foiklang, Maejo University, ThailandVictor Manuel Petrone-García, National Autonomous University of Mexico, Mexico
Copyright © 2022 Chen, Ma, Khan, Xiao, Alugongo, Li, Wang and Cao. This is an open-access article distributed under the terms of the Creative Commons Attribution License (CC BY). The use, distribution or reproduction in other forums is permitted, provided the original author(s) and the copyright owner(s) are credited and that the original publication in this journal is cited, in accordance with accepted academic practice. No use, distribution or reproduction is permitted which does not comply with these terms.
*Correspondence: Zhijun Cao, Y2FvemhpanVuQGNhdS5lZHUuY24=
†These authors have contributed equally to this work