- 1West Central Research and Outreach Center, University of Minnesota, Morris, MN, United States
- 2Department of Biology, Kutztown University of Pennsylvania, Kutztown, PA, United States
- 3Rodale Institute, Kutztown, PA, United States
Parasite infection is a common problem in organic pig production, which can compromise health and growth of pigs, threaten food safety of pork products, and cause economic losses to organic farmers. To develop management strategies for controlling parasites, we evaluated intestinal parasite infection in pigs at different ages and of different sexes, and investigated whether parasite infection influences growth performance and carcass traits in a cross-sectional study. Fecal samples were collected from pigs (n = 298) raised under near-organic standards during nursery, growing, finishing, and gestating phases for analysis of fecal egg counts (FEC) of Ascaris suum, Trichuris suis, and Oesophagostomum spp. Ascaris suum eggs were not detected in the feces of nursery pigs. Eggs of Ascaris suum were found in 45%, 74%, and 0% of fecal samples of growing pigs, finishing pigs, and gestating sows, respectively, after false-positive adjustment (P < 0.001). Mean FEC of Ascaris suum was higher in infected finishing pigs than in infected growing pigs [2,502 vs. 724 eggs per gram (epg), P < 0.001]. No differences in percent of Ascaris suum positive samples or FEC of Ascaris suum were detected between sexes. Growth performance and carcass traits were not different between non-infected pigs and those infected with Ascaris suum. All pigs (n = 32) examined at slaughter had white spots on the liver, and 78% harbored Ascaris suum worms. Trichuris suis eggs were not detected in any fecal samples. Eggs of Oesophagostomum spp. were found in 7%, 0%, 1%, and 9% of fecal samples of nursery pigs, growing pigs, finishing pigs, and gestating sows, respectively, with a maximum FEC of 40 epg in all age groups. These results indicate Ascaris suum was the predominant parasite infecting growing and finishing pigs in the herds studied. To control A. suum infection, future research should investigate the efficacy of treating pigs with organically approved anthelmintics during the growing phase of production.
Introduction
Parasite infection is a persistent problem in organic swine production (1–3), mainly due to requirement for housing pigs in bedded barns or outdoors while restricting anthelmintic use. In the United States, the National Organic Standards (4) require housing organic pigs in bedded barns with access to outdoors throughout all production stages and do not allow application of synthetic anthelmintics to pigs from 38 days prenatal (the third trimester of gestation) to slaughter. The most common parasites in organic pig farms are gastrointestinal (helminth) parasites with life stages outside of the host, such as embryonated eggs or free-living larvae in fecal material, that are directly responsible for new infections (5). Thus, pigs on bedded floors with exposure to feces face a greater risk of infection with intestinal parasites (6, 7).
Three species of intestinal parasites are commonly observed in pigs: Ascaris suum (A. suum), Oesophagostomum spp. (O. spp.), and Trichuris suis (T. suis), with A. suum being dominant in growing-finishing pigs, O. spp. in sows, and T. suis in pigs and sows with access to outdoors (5). Adult worms of A. suum live in the small intestine and O. spp. and T. suis live in different sections of the large intestine of pigs; and infections with a single species or co-infections with multiple species can occur (7–9). Pigs infected with A. suum show pathological lesions, commonly known as white spots, on the liver, which are caused by A. suum larvae migrating to the lungs via the liver, before settling in the small intestine (10–12). Livers with white spots caused by A. suum infection can be rejected for human food processing and lose commercial value (13). Additionally, infection can compromise the health of pigs because parasites can damage intestinal membranes and consequently compromise the immune system of the hosts (14–16). Pigs with damaged intestinal membranes may have diarrhea and reduce digestibility of dietary nutrients, which could ultimately reduce growth performance (17–19). A meta-analysis (20) indicated that intestinal parasite infection can reduce average daily feed intake (ADFI) by 5%, average daily gain (ADG) by 31%, and feed efficiency (gain:feed) by 6% in pigs.
A recent survey in the United States across fives states indicates that parasite infection is so common that all organic pig farms surveyed were infected with at least one species of intestinal parasites (2). However, research on parasite infection in organic pigs in the United States barely exists. To develop efficient management strategies for controlling parasite infection in organic pigs, we need to know occurrence and intensity of each parasite species infection, which age group of pigs should be targeted for treatment, and how parasite infection may affect growth performance, carcass traits, and liver condemnations at slaughter. The objectives of this study were to measure parasite infection in pigs at different production stages and of different sexes, and assess whether infection influences growth performance and carcass traits in an enclosed swine herd.
Materials and Methods
This study was conducted between 2019 and 2020 at the University of Minnesota's West Central Research and Outreach Center located in the Midwestern region (Morris, Minnesota) of the United States. The experimental protocol used in the study was reviewed and approved by the University of Minnesota Institutional Animal Care and Use Committee (IACUC#: 2106-39200A).
Animals, Housing, and Management
Pigs housed in the swine facilities with bedded floors were used for this study. The swine facilities included a hoop barn for gestating sows, an indoor barn for lactating sows and nursery pigs, and two hoop barns for growing-finishing pigs. All barns were bedded with wheat straw and had no confinement structures, except stalls in the gestation barn that were used during feeding to control individual feed intake of gestating sows. Within each barn except the gestation barn, pigs were managed all-in/all-out (AI/AO). All pigs were managed according to the National Organic Program (4), except conventional straw for bedding, piglets being weaned one week earlier than the National Organic Program requires, and pigs and sows being denied for access to outdoors due to the facility structures.
Gestating sows (Yorkshire × Landrace) were housed in 4 pens on bedded concrete floors, and each pen accommodated 15 sows. Fifteen feeding stalls and a water fountain with two drinking spaces were present in each pen. Floor space allowance in each pen was 3.7 m2 per sow, excluding the area occupied by feeding stalls and the water fountain. Sows entered the gestation barn after weaning and remained until 3 to 7 days before the expected farrowing dates when the sows were moved to the farrowing barn. Sows farrowed in two batches, each consisting of 30 sows from 2 gestation pens. The second batch of sows farrowed 10 weeks after the first batch. The gestation barn was managed on a continuous basis (not AI/AO). Bedding in the gestation barn was cleaned out every 1 to 3 months according to the season, less frequently in winter to preserve the heat and more frequently in summer to reduce moisture in the barn.
The farrowing barn served as both a farrowing and a nursery barn. Eight sows were housed in each of three identical rooms (9.8 m × 11.0 m) on bedded concrete floors. Sows in each farrowing room shared two feeders with 4 feeding spaces each, and one water fountain with two drinking spaces in the communal area. Sows farrowed and nursed their piglets in individual pens during the first 10 days after farrowing. Then, farrowing pens were removed so that sows and their piglets within each room mingled in a large group until weaning. Piglets were ear-tagged for individual identification, supplemented with iron via intramuscular injection, and male piglets were castrated within 24 h after birth. No piglets were tail docked or ear-notched according to the National Organic Program (4). Piglets were weaned at 5 weeks of age by removing the sows from the farrowing rooms. After weaning, piglets remained in each farrowing room for another 3 weeks. The farrowing barn was power-washed and dried after bedding being cleaned out between groups.
At 8 weeks of age, healthy pigs (Yorkshire × Landrace × Duroc) with no visual signs of illness, lameness, or any other physical injuries were transferred to growing-finishing hoop barns. Each growing-finishing barn had two pens, and each pen (6 m × 24 m) was equipped with a round bulk feeder with 12 feeding spaces and a water fountain with 4 drinking spaces on a raised concrete platform (6 m × 4 m). The rest area of the pen was bedded with straw on pressed clay floors. Fifty pigs balanced for sex and body weight were assigned to each pen within a hoop barn. Once assigned, pigs remained in their pens until they reach slaughter weight (average 125 kg). After bedding being cleaned out between groups, the growing-finishing hoop barns remained empty for 2 to 4 weeks to dry the floors.
Room temperature was controlled by a central heating system and exhaust fans to achieve a room temperature of 20°C in the farrowing and nursery barn. Unlike the farrowing and nursery barn, the hoop barns for gestation and growing-finishing pigs were ventilated naturally through openings on the sides and ends of the barns with no mechanical ventilation, heating, or cooling systems. Thermal environment in all hoop barns was maintained by adjusting the openings of the barn and the amount of bedding provided. The depth of straw bedding in the hoop barns was maintained at 40 to 60 cm during winter months, 10 to 30 cm during summer months, and 20 to 40 cm during other months. Fresh bedding was added when a new group of sows or pigs entered the barns. Throughout the rearing period, fresh bedding was added as needed to maintain clean and dry lying areas in each room and pen, and to achieve the desired microthermal environment for sows and pigs. Diets were formulated to meet or exceed nutrient requirement of pigs at each production stage recommended by the National Research Council (21). Except sows that were limited fed to achieve desired body condition during gestation, pigs and sows were provided feed ad libitum and water all the time. Pigs from birth to slaughter and sows from the 3rd trimester of gestation (38 days prior to the expected farrowing date) to weaning were fed organically approved feed and were not treated with any antibiotics or anthelminthics. Prior to the 3rd trimester of gestation, sows were treated with anthelmintics (Safe-Guard 0.9% Swine Dewormer, Zoetis Inc, with Fenbendazole as the active ingredient) by top-dressing 100 mg/kg body weight for 3 consecutive days.
Experimental Design and Data Collection
A cross-sectional study was conducted involving pigs at four production stages: gestating sows (n = 45) prior to deworming, nursery pigs (n = 29) at 6 weeks of age, growing pigs (n = 91) at 15 weeks of age, and finishing pigs (n = 133) at 23 weeks of age. All fecal samples (n = 298) were collected over 8 days between 2019 and 2020. In 2019, 104 fecal samples were collected from gestating sows (n = 45) in 4 pens, nursery pigs (n = 29) in 2 rooms, and growing pigs (n = 30) in 2 pens over 3 days in June, September, and November, respectively. In 2020, 194 fecal samples from growing pigs (n = 61) in 4 pens and finishing pigs (n = 133) in 6 pens were collected over 5 days in January, June, August, September, and October, respectively. Among the finishing pigs that were fecal sampled, 103 pigs (25 to 27 pigs from each pen) in 4 pens were identified individually and recorded for growth performance and carcass traits (see Section Growth performance, carcass traits, liver white spots, and A. suum worm presence).
Fecal Sampling
Fecal samples were collected either during or immediately after pigs and sows defecated using the method described by Katakam et al. (1). Pigs were selected for fecal sampling based on their defecation during the time of sampling. For all pigs and most dry sows, samples were collected either before the feces was dropped on the floor or from the top of the feces that have just dropped on the floor. For 6 sows that did not defecate during the sampling periods, fecal samples were collected from the rectum. To avoid fecal samples being collected repeatedly from the same pig at a sampling time point, pigs were marked with a crayon on their back after their fecal samples were collected. Each sample (10 to 30 g/sample) was placed in a plastic zip-lock bag and stored on ice in a cooler immediately after sampling. Then the samples were stored in a refrigerator at 4°C for 6 to 72 h (median = 24 h) before being shipped to Kutztown, Pennsylvania. All fecal samples were shipped overnight on cool pads to Kutztown University for analysis of fecal egg counts (FEC) of each parasite species.
Lab Analysis for Parasite Fecal Egg Counts
Fecal samples were processed and analyzed within a few days (2 to 10 days, median = 4 days) of sampling. Fecal egg counts of A. suum, O. spp., and T. suis were determined according to the concentration McMaster technique described by Roepstorff and Nansen (22). Saturated NaCl-glucose solution (50 g NaCl, 75 g glucose monohydrate, and 131 g water) was used as a flotation fluid and the detection limit was 20 egg per gram (epg) of fecal sample for all three species of parasites. Fecal samples were analyzed to determine positive samples, egg abundance, and intensity for each parasite species. Positive samples were the number of fecal samples detected with parasite eggs as percent of total fecal samples analyzed. Egg abundance was the average FEC of all fecal samples collected. Egg intensity was the average FEC of fecal samples that were detected with parasite eggs (22). False-positive adjustment was performed for evaluation of A. suum infection, using FEC of 200 epg as the criteria according to Boes et al. (23) and Katakam et al. (1). Fecal samples with FEC of A. suum < 200 epg were considered false-positive of A. suum infection.
Growth Performance, Carcass Traits, Liver White Spots, and A. suum Worm Presence
For finishing pigs (n = 103) that were individually identified for fecal sampling, sex, growth performance and carcass traits were recorded for each pig. Pigs were weighed individually at 8 weeks of age when entering the hoop barn, every 4 weeks thereafter, and at 23 weeks of age prior to slaughter. Average daily gain was calculated for each pig from weight changes and days between each weighing. At 23 weeks of age, all pigs, except two pigs that did not meet requirements for slaughter and 32 pigs that were used for evaluation of liver white spots and A. suum worm presence, were slaughtered at a commercial meat processing plant where hot carcass weight and backfat thickness at the last rib were recorded for each pig. Dressing percentage was calculated for each pig based on carcass weight and live weight before slaughter of each pig using the equation: dressing (%) = (hot carcass weight/live weight) × 100%.
A subset of individually identified pigs (n = 32, all gilts) was examined for liver white spots caused by migration of A. suum larvae and for presence of A. suum worms in the small intestine when pigs were slaughtered at the Meat Lab of the University of Minnesota at 24 weeks of age [average weight = 128 kg ± 8.7 (SD)]. Liver white spots were classified arbitrarily into two categories based on the number: many (more than 10 white spots on a liver) and few (10 or fewer than 10 white spots on a liver). Ascaris suum worms in the small intestines were recorded as presence or absence. Meanwhile, hot carcass weight and backfat thickness at the last rib were recorded for each pig as in the commercial processing plant.
Data Analysis
All data were analyzed using SAS software (Version 9.4; SAS Institute Inc., Cary, NC) and tested for normal distribution using the Univariate Procedure. Data for percent of positive samples were analyzed using the Frequency Procedure with Chi-square (χ2) test. Because data for FEC (both raw and logarithm transformed) were not normally distributed, non-parametric Friedman rank test with Cochran-Mantel-Haenszel (CMH) test based on rank scores was used to detect differences among production stages and between sexes with pen serving as a stratifying variable. Due to sparse data (majority of the data with 0 epg of FEC), sampling date was not considered in any statistical model. For finishing pigs that were individually identified, A. suum was the only parasite species detected in fecal samples. As a result, A. suum infection on growth performance (initial and final weight, ADG) and carcass traits (hot carcass weight, backfat thickness, and dressing percentage) was analyzed. Pigs were categorized as infected or non-infected based on FEC of A. suum. Pigs with A. suum FEC equal to or > 200 epg were considered infected, and pigs with A. suum FEC < 200 epg were defined as non-infected (23). Data for growth performance and carcass were normally distributed and analyzed using the Mixed procedure. In the statistical model, the effect of A. suum infection on growth performance, except for ADG, and carcass traits was analyzed with infection as the fixed effect and pen as a random effect. Data for ADG were analyzed using the Mixed procedure with repeated measures in time, and the statistical model included infection, week, and their interaction as fixed effects and pen as a random effect. In both models, infection nested within pen was used as the experimental unit and differences between least square means were tested using Tukey's test. Initial weight was used as a covariate for data analysis of final weight, hot carcass weight and ADG, final weight as a covariate for dressing percentage, and hot carcass as a covariate for backfat thickness. To analyze correlations between A. suum infection and pig performance, correlations of FEC with initial body weight, final body weight, ADG, carcass weight, dressing percentage, and backfat thickness at the last rib were estimated using the Spearman rank correlation procedure. Furthermore, data for the number of liver white spots and presence of A. suum worm in the small intestine were analyzed using the Frequency and Logistic procedures. The likelihood of A. suum presence associated with liver white spots was tested using the Wald Chi-square (χ2) for odds ratios with 95% confidence intervals. All tests were two-tailed tests. Differences were considered significant when P < 0.05.
Results
Parasite Infection and Fecal Egg Counts
Percent of positive samples and FEC (abundance and intensity) of three species of parasites in four production stages are listed in Table 1. Ascaris suum eggs were not detected in the feces of nursery pigs. Percent of A. suum positive samples was the highest in finishing pigs (91.7%), lowest in gestating sows (6.7%), and intermediate in growing pigs (75.8%; P < 0.001) without false-positive adjustment. After false-positive adjustment, percent of A. suum positive samples remains higher in finishing pigs than in growing pigs (74.4% vs. 45.1%; P < 0.001), and dropped to 0% in gestating sows. Mean FEC (both abundance and intensity) of A. suum were higher (both P < 0.001) in finishing pigs than in growing pigs. Confidence limits for mean FEC and range for the inner quartile (between 25th and 75th percentile) were wider for finishing pigs than for growing pigs, suggesting large variation in A. suum FEC among individual finishing pigs than among growing pigs.
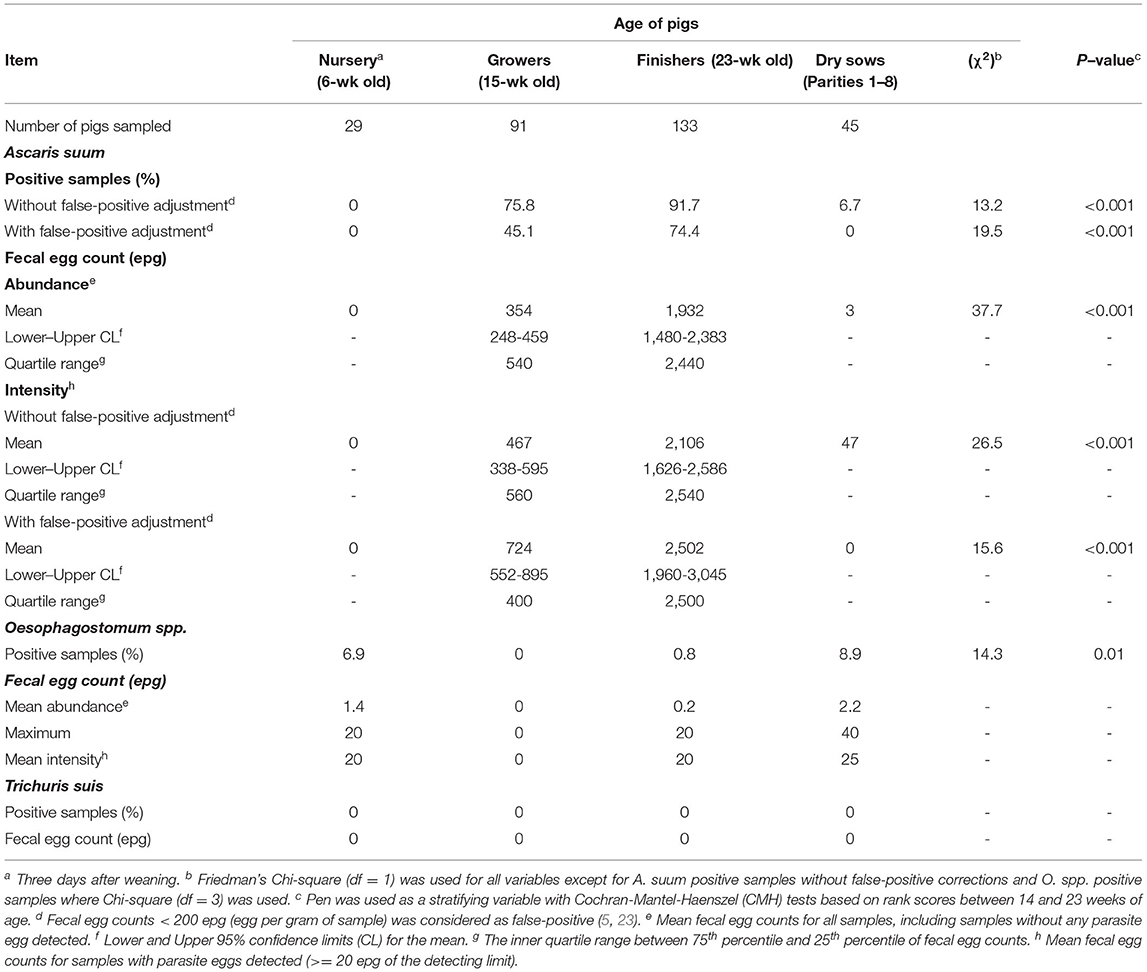
Table 1. Positive samples and fecal egg counts, measured in eggs per gram (epg), of intestinal parasites in pigs at different ages.
Oesophagostomum spp. eggs were not detected in the feces of growing pigs (Table 1). Percent of O. spp. positive samples was higher in nursery pigs (7%) and gestating sows (9%) than in finishing pigs (1%; P < 0.01), with the maximum FEC of 40 epg across all age groups. Cautions should be taken when interpreting the differences in O. spp. infection among production stages because most fecal samples had 0 epg of O. spp. Trichuris suis eggs were not detected in any fecal samples in this study.
Among the finishing pigs that were individually identified, 58 were barrows and 45 were gilts (Table 2). There were no differences in percentage of A. suum positive samples between sexes, regardless of false-positive adjustment (both P > 0.37). Likewise, no difference in A. suum FEC (abundance or intensity) was detected between sexes (all P > 0.41).
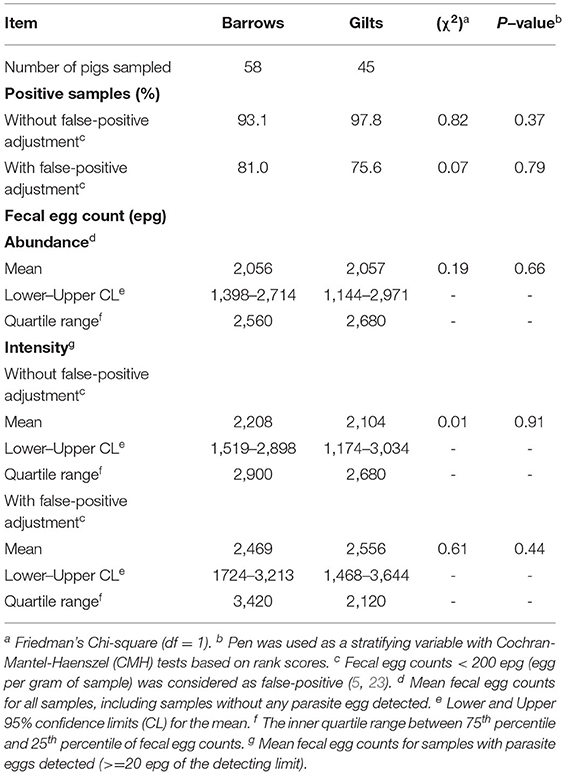
Table 2. Positive samples and fecal egg counts, measured in eggs per gram (epg), of Ascaris suum in barrows vs gilts at 23 weeks of age.
Growth Performance and Carcass Traits
Twenty-two pigs (21%) were categorized as non-infected and 81 pigs (79%) were categorized as infected with A. suum (Table 3). No difference in initial or final body weight, ADG, hot carcass weight, dressing percentage, or backfat thickness at the last rib was detected between pigs that were infected and not infected with A. suum. Likewise, Spearman rank correlation analysis did not detect correlations of A. suum FEC with growth performance or carcass traits (Table 4). Two pigs were not sold for slaughter, due to mobility problems or lighter (91 kg) than the minimum slaughter weight (100 kg) required by the processing plant. Both pigs were infected with A. suum, with FEC of 1,060 and 2,360 epg for the pigs with mobility problems and lightweight, respectively.
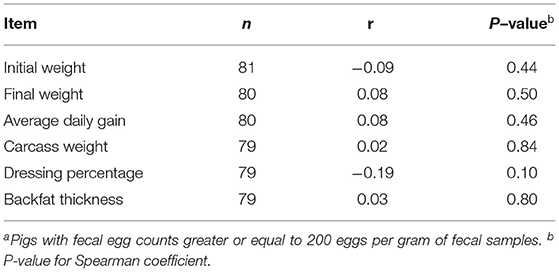
Table 4. Coefficient (r) of Spearman rank correlation of Ascaris suum fecal egg count with growth performance and carcass traits of infected finishing pigsa at 23 weeks of age.
For pigs (n = 32) that were examined for liver white spots and A. suum worm presence, 100% of the pigs had white spots on the liver, with 78% (25 out of 32 pigs) having many (more than 10) white spots on each liver. Likewise, 78% of these pigs hosted A. suum worms. Pigs with many white spots on the liver were more likely to harbor A. suum worms in the small intestine (odds ratio = 144, lower 95% confidence limit = 7.8; Wald χ2= 11.2, df=1; P < 0.001) compared to pigs with fewer white spots on the liver.
Discussion
Parasite Infection at Different Production Stages
Parasite infection in pigs at different production stages is influenced by both the immunogenic property of the parasite and management practices to control parasites. Generally, in organic swine production systems where pigs are raised on bedded floors and anthelmintic drugs are prohibited, A. suum infection is expected to be higher in pigs (nursery, growing and finishing pigs) than in breeding sows due to the high immunogenicity of A. suum (1, 3, 5). On the other hand, O. spp. infection is expected to be higher in sows than in pigs due to the low immunogenicity of the parasite (5, 7). In conventional production systems where pigs are raised in confinement barns on slatted floors to separate pigs from feces and can be treated with anthelmintic drugs when needed, A. suum infection does not occur until pigs reach the growing or finishing phase, and O. spp. remains at a very low level until pigs reach the breeding phase (5, 24). In the current study, we observed distinct patterns of intestinal parasite infections in pigs at different production stages. For instance, A suum infection was detected in growing and finishing pigs, but not detected in newly weaned nursery pigs or gestating sows after false-positive adjustment. On the contrary, O. spp. infection was detected in nursery pigs and gestating sows, but not detected in growing pigs. Trichuris suis was not detected in pigs of any production stages in this study, possibly because all pigs were housed in barns without access to outdoor environments (25, 26). These patterns of intestinal parasite infection in pigs at different production stages in the current study are more similar to the patterns observed in conventional production systems than the patterns in organic production systems, possibly due to housing, management, and the parasite control protocol applied to the swine herds studied.
Application of the anthelmintic drug (fenbendazole) by the 3rd trimester of gestation could be a main reason for the absence of A. suum and T. suis infection in gestating sows in the current study. Fenbendazole can effectively protect sows from infections of most swine parasite species, with 100% protection against A. suum, and 94% to 100% protection against T. suis (27, 28). Additionally, washing and drying the farrowing barn could have removed and reduced the survival of parasite eggs, prevented parasite transmission from previous farrowing groups (24), and ultimately reduced parasite pressure in the sow herds in this study. Furthermore, sows housed in the bedded barns possibly could have developed immunity against intestinal parasites, especially A. suum and T. suis. which can stimulate strong immune reactions in pigs (5). Compared to A. suum and T. suis, O. spp. does not stimulate strong immune responses in pigs, which could contribute infection of O. spp. observed in gestating sows in the current study. However, percent of O. spp. positive samples (9%) in sows in the current study was much lower than reported for organic sows (e.g., 50% reported by 5; 20% reported by 6) and sows across different housing systems (e.g., 52% reported by 7). Additionally, O. spp. egg intensity in sows in the current study was barely detectable. The low percent of positive samples combined with low egg intensity suggests that O. spp. infection is not a concern for the sow herds in the current study.
Percent of positive samples and egg intensity of A. suum and O. spp. in nursery pigs followed similar patterns as in gestating sows in the current study. While A. suum was not detected in any fecal samples of nursery pigs, we could not confirm that nursery pigs were not infected with A. suum in the current study. This is because the prepatent period of A. suum is 6 to 8 weeks (5, 12), which is close to the age of nursery pigs in this study. Using experimental inoculation, Mejer and Roepstorff (29) and Nejsum et al. (12) observed that most pigs started to secrete A. suum eggs between 7 and 11 weeks of age post inoculation. Piglets born to contaminated pastures did not shed A. suum eggs in their feces until 9 weeks of age (29). Likewise, Lindgren et al. (3) reported that 12-week old pigs on organic farms shed significant amount (138 to 7,612 epg) of A. suum eggs in feces. If the nursery pigs in the current study had been infected with A. suum, we expect both percent of positive samples and egg intensity would be low due to the minimal shedding of A. suum eggs by the sows that farrowed and nursed the pigs. On the other hand, percent of O. spp. positive samples and egg intensity of O. spp. in nursery pigs in this study were much lower than in organic nursery pigs reported previously (6, 24). The low percent of positive samples (7%) combined with barely detected FEC (20 epg) renders O. spp. not being a concern for nursery pigs studied. Interestingly, percent of O. spp. positive samples in growing (0% prevalence) and finishing (<1% prevalence) pigs was even lower than in nursery pigs in the current study. These data suggest that growing and finishing pigs in the current study barely harbored O. spp. worms.
Adjustment for false-positive infection of A. suum was used in this study to exclude pigs that were not infected but only passed A. suum eggs through their digestive tracts. Boes et al. (23) and Katakam et al. (1) reported that pigs with FEC < 200 epg were not likely to harbor A. suum worms, instead they only passed parasite eggs through the digestive tracts due to coprophagy. Coprophagy is more frequently observed in pigs that are housed indoors with high stocking density compared to pigs on pastures or housed indoors with low stocking density (23). False-positive A. suum samples can be as high as 30% in growing-finishing pigs, and 15% in gestating sows (1). So, adjustment for false-positive infection of A. suum is recommended, especially for pigs housed indoors without outdoor access, as in the current study (1, 23). After adjustment for false-positive infection, percent of A. suum positive samples was reduced by 30% in growing pigs and 18% in finishing pigs in the current study, which were consistent with results reported by Katakam et al. (1).
Results of the current study suggest that A. suum infection was a major concern for growing and finishing pigs in the swine herds studied. We observed the same trend on commercial organic pig farms where A. suum infection was most common in growing-finishing pigs compared to nursery pigs and gestating sows (2). Infection of A. suum has also been confirmed in organic fattening pigs in European countries (30). In fact, A. suum is the toughest parasite to control in swine due to the large amount of eggs that each female A. suum worm sheds daily, and because the thick shell of A. suum eggs that can allow the eggs to remain viable for four to ten years (5). We suspect that most of the pigs in the current study had been infected with A. suum in the growing-finishing hoop barns because the barns with clay floors could not be washed or disinfected between groups. Thoroughly cleaning, washing, and drying floors between groups of pigs could have reduced A. suum infection in growing-finishing pigs in the current study. Pettersson et al. (24) attributed the reduction in parasite prevalence in Swedish pigs over the last 30 years to improved hygiene practices. To control A. suum in conventional swine production systems where there is no restriction on anthelmintic treatments, it is recommended to treat sows before moving into the farrowing barn, and to treat pigs at weaning and once or twice during the growing-finishing phase (5). In organic swine production systems where synthetic anthelmintics are prohibited for market pigs, A. suum could be managed by limited application of anthelmintics that are approved for organic production. Currently, some organically approved anthelmintics are commercially available and recommended to be used for treating pigs at weaning in the Midwestern region of the United States. However, results of the current study and previous work (28) suggest that treating pigs during the growing phase may be more effective to minimize transmission of A. suum infection than treating pigs at weaning. One reason for doing this is that pigs do not shed A. suum eggs at weaning as demonstrated in the current study and previous work (28). Instead, deworming pigs with organically approved anthelmintics during the growing phase can reduce the risk of A. suum transmission because about 30% to 50% of growing pigs shed A. suum eggs as observed in the current study and previously (6). Additionally, deworming during the growing period might prevent pigs from shedding A. suum eggs after anthelminthic treatment until slaughter due to the prepatent period length (5, 12). The efficacy of treating pigs during the growing phase of production with organically approved anthelmintics needs to be investigated in future research.
Parasite Infection Between Sexes
We did not detect a difference in A. suum infection between barrows and gilts in the current study. This is consistent with results reported by Amadi et al. (31) and Aiyedun and Oludairo (32) who did not detect any difference in A. suum infection between sexes of pigs. Sex differences in parasite infection have been observed in vertebrates, including lab animals, field animals (field mice and voles) and pastured cattle, with males having higher infection prevalence and egg intensity of parasites than females due to the negative effect of testosterone and positive effect of progesterone against parasite infections (33). However, such difference in parasite infection between sexes has not been observed in pigs. On the contrary, Tamboura et al. (34) and Sah (35) reported that infection prevalence of A. suum was higher in gilts than in barrows, probably due to difference in coprophagy between sexes. Likewise, Baskota and Shrestha (36) noted that gilts showed more clinical symptoms of A. suum infection than barrows. Male pigs in the current study, as in commercial swine production in the United States, were castrated at birth, and castrated males (barrows) produce limited testosterone. Therefore, we do not expect any difference in testosterone concentrations between barrows and gilts. Additionally, finishing pigs in the current study did not reach their reproductive age, so progesterone effects of gilts on parasite infection are not expected.
Effects of Parasite Infection on Growth Performance and Carcass Traits
Parasite infection can potentially result in economic losses as indicated by reduced ADFI, ADG, and feed efficiency in previous work (18, 20, 37). Yang et al. (38, 39) reported that parasite infection can cause anorexia, resulting in reduced feed intake in pigs. Reduced feed intake is one of the major reasons for reduced growth rate. Additionally, parasites can negatively affect host's nutrient absorption by causing lesions on the intestinal membranes or microvillus atrophy (14–16). As a result, the negative effect of parasite infection on ADG can be escalated when pigs are fed diets that do not meet their nutrient requirements (40, 41). Furthermore, parasite infection evokes immune response of the host and activates macrophages to produce pro-inflammatory cytokines. The cytokines can reduce growth hormone secretion in the central nervous system (42), resulting in reduced growth in parasite infected pigs. However, in the current study, we did not detect differences in growth performance or carcass traits between pigs infected and non-infected with A. suum. We could not evaluate the difference in feed intake between infected and non-infected pigs in the current study because both infected and non-infected pigs were housed in the same pen (50 pigs/pen) and fed as a group. However, we speculate that both infected and non-infected pigs consumed enough feed to meet their nutrient requirements because pigs in the current study were fed ad libitum and all diets were formulated to meet or exceed nutrient requirements of pigs according to the NRC (21) recommendations. Consequently, there was no difference in growth performance or carcass traits between infected and non-infected pigs in the current study. Our results are consistent with results of Martinez-Perez et al. (43) who reported that A. suum infection was not correlated with ADG in pigs. On the other hand, Bernardo et al. (44) reported that the number of A. suum worms in the small intestine did not affect ADG, but the life-time burden of A. suum, measured by FEC and the duration of infection, was negatively associated with ADG. Additionally, Knecht et al. (17), Jankowska-Makosa and Knecht (18) reported that pigs infected with both A. suum and O. spp. reduced ADG, dressing and carcass lean in pigs. Because we did not observe O. spp. infection in growing pigs in the current study, our results are not comparable to results of Knecht et al. (17).
Another potential economic loss caused by A. suum infection is liver condemnation (43). In the current study, we observed white spots on all livers examined, suggesting that all pigs may have infected with A. suum during the rearing phase (10, 11, 45). However, not all these pigs harbored A. suum worms at slaughter. We observed pigs that had more than 10 white spots on the liver were likely to harbor A. suum worms at slaughter. In other words, livers from pigs hosting A. suum worms are more likely to be excluded from the food processing chain, resulting in economic losses to organic pork farmers. Several researchers reported evidence that pigs may develop a “pre-hepatic barrier” after continuous exposure to A. suum and presence of A. suum worms in the small intestine (45–47). The pre-hepatic barrier is considered to regulate A. suum worm population in the small intestine of the host by preventing ingested larvae migrating to the liver (45–47). However, some later studies (12, 29) demonstrated that the pre-hepatic barrier did not completely prevent ingested larvae from migrating to the liver. In fact, Nejsum et al. (12) reported that the presence of A. suum worms in the intestine does not affect larvae migrating to the lungs, the liver, or the intestine, but affects whether larvae could survive in the small intestine and grow to adult worms. This may explain results from the current study that pigs that hosted A. suum worms had more white spots on the liver, possibly because these pigs could have been continuously infected with A. suum, resulting in larvae continuously migrated to the liver. In the current study, 78% of focal pigs examined for A suum worm presence in the small intestine hosted A. suum worms, which was consistent with percent of A. suum positive samples (78.6% after false-positive adjustment) in the 103 pigs from which the focal pigs were derived. This further suggests that false-positive adjustment was essential for evaluation of A. suum infection using FEC in enclosed herds as in the current study.
Conclusion
In the enclosed swine herds studied, intestinal parasite infection was not a concern for gestating sows and nursery pigs because sows were treated with anthelmintic drugs by the 3rd trimester of gestation and farrowed in a clean farrowing barn. Growing and finishing pigs were heavily infected with A. suum in hoop barns on bedded clay floors which may have been contaminated with A. suum over the years of operation. However, A. suum infection did not affect growth performance or carcass traits, and there was no difference in A. suum infection between sexes in the current study. Pigs that harbored A. suum worms bore many (more than 10) white spots on the liver, which can result in rejection of the liver for use as human food. Management strategies for controlling A. suum infection in organic pigs, including application of organically approved anthelmintics during the growing phase of production, need to be investigated in the future.
Data Availability Statement
The raw data supporting the conclusions of this article will be made available by the authors, without undue reservation.
Ethics Statement
The animal study was reviewed and approved by University of Minnesota Institutional Animal Care and Use Committee.
Author Contributions
YL designed and conducted the animal trial, analyzed the data, and prepared the manuscript. AH and SM conducted lab analysis and corrected the manuscript. RC reviewed and corrected the manuscript. All authors contributed to and approved the submitted version.
Funding
This work was supported by the Organic Transition Program (Award#: 2018-51106-28772) from the USDA National Institute of Food and Agriculture.
Conflict of Interest
The authors declare that the research was conducted in the absence of any commercial or financial relationships that could be construed as a potential conflict of interest.
Publisher's Note
All claims expressed in this article are solely those of the authors and do not necessarily represent those of their affiliated organizations, or those of the publisher, the editors and the reviewers. Any product that may be evaluated in this article, or claim that may be made by its manufacturer, is not guaranteed or endorsed by the publisher.
References
1. Katakam KK, Thamsborg SM, Dalsgaard A, Kyvsgaard NC, Majer H. Environmental contamination and transmission of Ascaris suum in Danish organic pig farms. Parasite Vectors. (2016) 9:1–12. doi: 10.1186/s13071-016-1349-0
2. Li YZ, Hernandez A, Carr R, Major S, DeWitte D. Parasite prevalence and fecal egg counts on organic pig farms. J Anim Sci. (2020) 98:463. doi: 10.1093/jas/skaa054.385
3. Lindgren K, Cunnarsson S, Hoglund J, Lindahl C, Roepstorff A. Nematode parasites eggs in pasture soils and pigs on organic farms in Sweden. Org Agr. (2020) 10:289–300. doi: 10.1007/s13165-019-00273-3
4. NOP (National Organic Program). Agricultural Marketing Service, USDA. (2020). Available online at: Section A. Standards | Agricultural Marketing Service (usda.gov). (accessed March 10, 2022).
5. Nansen P, Roepstorff A. Parasitic helminths of the pig: factors influencing transmission and infection levels. Intl J Parasitol. (1999) 29:877–91.
6. Carstensen L, Vaarst M, Roepstorff A. Helminth infections in danish organic swine herds. Vet Parasitol. (2002) 106:253–64. doi: 10.1016/S0304-4017(02)00080-8
7. Kochanowski M, Karamon J, Dabrowska J, Dors A, Czyzewska-Dors E, Cencek T. Occurrence of intestinal parasites in pigs in Poland – the influence of factors related to the production systems. J Vet Res. (2017) 61:459–66. doi. 10. 1515/jvetrs-2017-0053. doi: 10.1515/jvetres-2017-0053
8. Helwigh AB, Christensen CM, Roepstorff A, Nansen P. Concurrent Ascaris suum and Oesophagostomum dentatum infections in pigs. Vet Parasitol. (1999) 82:221–34. doi: 10.1016/S0304-4017(99)00007-2
9. Gowda CK, Pattalakshmamma GC, D'Souza PE, Mamatha GS, Chandranaik HM. Survey of gastrointestinal parasites of pigs slaughtered in Bengaluru. J Vet Parasitol. (2018) 32:23–37. doi: 10.5958/0974-0813.2018.00005.0
10. Mehl WM, Boch J, Heine J, Weiland G, Henkel G. Ascaris larvae as a cause of liver lesions (milk spots) in swine. Berl Munch Tierarztl Wochenschr. (1983) 96:405–9.
11. Roepstorff A, Eriksen L, Slotved HC, Nansen P. Experimental Ascaris suum infection in the pig: worm population kinetics following single inoculations with three doses of infective eggs. parasitol. (1997) 115:443–52. doi: 10.1017/S0031182097001480
12. Nejsum P, Thamsborg SM, Petersen HH, Kringel H, Fredholm M, Roepstorff A. Population dynamics of Ascaris suum in trickle-infected pigs. J Parasitol. (2009) 95:1048–53. doi. 10.1645/ge-1987.1 doi: 10.1645/GE-1987.1
13. Zumbado L, Oliveira JB, de Chacon F, Hermandez J, Quiros L, Murillo J. Identification of gastrointestinal parasites in pig farms and economic losses due to condemnation of livers parasitized by Ascaris suum in abottoirs in Cost Rica. Revista de Ciencias Veterinarias. (2009) 27:7–21.
14. Andreasen A, Petersen HH, Kringel H, Iburg TM, Skovgaard K, Dawson H, et al. Immune and inflammatory responses in pigs infected with Trichuris suis and Oesophagostomum dentatum. Vet Parasitol. (2015) 207:249–58. doi: 10.1016/j.vetpar.2014.12.005
15. William AR, Krych L, Ahmad HF, Nejsum P, Skovgaard K, Nielsen DS, et al. A polyphenol-enriched diet and Ascaris suum infection modulate mucosal immune response and gut microbiota composition in pigs. PLoS ONE. (2017) 12:e0186546. doi: 10.1371/journal.pone.0186546
16. Wang YY, Liu F, Urban JF, Paerewijck O, Geldhof P, Li RW. Ascaris suum infection was associated with a worm-independent reduction in microbial diversity and altered metabolic potential in the porcine gut microbiome. Int J Parasitol. (2019) 49:247–56. doi: 10.1016/j.ijpara.2018.10.007
17. Knecht D, Jankowska A, Zalesny G. The impact of gastrointestinal parasites infection on slaughter efficiency in pigs. Vet Parasitol. (2012) 184:291–7. doi: 10.1016/j.vetpar.2011.09.006
18. Jankowska-Makosa A, Knecht D. The influence of endoparasites on selected production parameters in pigs in various housing systems. Res Vet Sci. (2015) 100:153–60. doi: 10.1016/j.rvsc.2015.04.002
19. Ozvari L. Production losses of parasitisms in swine. Literat Rev Magyar Allatorvosok Lapja. (2017) 139:17–25.
20. Kipper M, Andretta I, Monteiro SG, Lovatto PA, Lehnen CR. Meta-analysis of the effects of endoparasites on pig performance. Vet Parasitol. (2011) 181:316–20. doi: 10.1016/j.vetpar.2011.04.029
21. NRC (National Research Council). Nutrient Requirements of Swine. 11th revised ed. Washington, DC: National Academies Press (2012).
22. Roepstorff A, Nansen P. The Epidemiology, Diagnosis and Control of Helminth Parqasites in Swine. Rome: An FAO handbook FAO (1998). p. 51–6.
23. Boes J, Nansen P, Stephenson LS. False-positive Ascaris sum egg counts in pigs. Intl J Parasitol. (1997) 27:833–8. doi: 10.1016/S0020-7519(97)00054-4
24. Pettersson E, Sjolund M, Dorea FC, Lind EO, Grandi G, Jacobson M, et al. Gastrointesinal parasites in Swedish pigs: prevalence and associated risk factors for infection in herds where animal welfare standards are improved. Vet Parasitol. (2021) 295:1–8. doi: 10.1016/j.vetpar.2021.109459
25. Roepstorff A, Murrell KD. Transmission dynamics of helminth parasites of pigs on continuous pasture: ascaris suum and trichuris suis. Intl J Parasitol. (1997) 27:563–72. doi: 10.1016/S0020-7519(97)00022-2
26. Kringel H, Roepstorff A. Trichuris suis population dynamics following a primary experimental infection. Vet Parasitol. (2006) 139:132–9. doi: 10.1016/j.vetpar.2006.03.002
27. Stewart TB, Marti OG, Hale OM. Efficacy of fenbendazole agaionst five genera of swine parasites. Am J Vet Res. (1981) 42:1160–2.
28. Vandekerckhove E, Vlaminck J, Sacristan R, del P, Geldhof P. Effect of stratigic deworming on Ascaris suum exposure and technical performance parameters in fattening pigs. Vet Parasitol. (2019) 268:67–72. doi: 10.1016/j.vetpar.2019.03.006
29. Mejer H, Roepstorff A. Ascaris suum infections in pigs born and raised on contaminated paddocks. Parasitol. (2006) 133:305–12. doi: 10.1017/S0031182006000394
30. Lindgren K, Bochicchio D, Hegelund L, Leeb C, Mejer H, Roepstorff A, et al. Animal health and welfare in production systems for organic fattening pigs. Org Ag. (2014) 4:135–47. doi: 10.1007/s13165-014-0069-z
31. Amadi AN, Ubiaru PC, Ugagu GM, Ibediugha BN, Obeagu IA, Njikeonye A. Preliminary study on the prevalence of gastrointestinal parasites of pigs managed and slaughtered in umuahia north local government area of abia state, Nigeria. Anim Res Intl. (2018) 15:3013–9.
32. Aiyedun JO, Oludairo OO. Prevalence of intestinal parasitism of swine in a north central state of Nigeria. J Adv Vet Anim Res. (2016) 3:278–81. doi: 10.5455/javar.2016.c163
33. Zuk M, McKean KA. Sex differenes in parasite infections: patterns and processes. Intl J Parasitol. (1996) 26:1009–24. doi: 10.1016/S0020-7519(96)80001-4
34. Tamboura HH, Banga-Mboko H, Maes D, Youssao I, Traore A, Bayala B, et al. Prevalence of common gastrointestinal namatode parasites in scavenging pigs of different ages and sexes in eastern centre province, Burkina Faso. Onderstepoort J Vet Res. (2006) 73:53–60. doi: 10.4102/ojvr.v73i1.169
35. Sah RP. Prevalence of common gasatrointestinal namatode parasitets in pigs based on different altitudes and seasons in Dhankuka and Sunsari districts of Nepal. Nepalese J Ag Sci. (2018) 16:77–84.
36. Baskota N, Shrestha SP. Helminth parasites of pigs and development of suitable strategies for its control. Nepalese Vet J. (2019) 36:163–9. doi: 10.3126/nvj.v36i0.27776
37. Hale OM, Stewart TB, Marti OG. Influence of an experimental infection of Ascaris suum on performance of pigs. J Anim Sci. (1985) 60:220–5. doi: 10.2527/jas1985.601220x
38. Yang S, Bottoms GD, Gaafar SM. Tumor necrosis factor-like cytotoxicity and anorexia in Ascaris suum infected pigs. Vet Parasitol. (1990) 36:37–44. doi: 10.1016/0304-4017(90)90092-P
39. Yang S, Gaafar SM, Bottoms GD. Serum levels of gastrin insulin and glucagons as possible factors of anorexia in pigs infected once with Ascaris suum. Vet Parasitol. (1990) 36:211–9. doi: 10.1016/0304-4017(90)90033-8
40. Nesheim MC. Some experimental approaches to the study of nutrition and parasitic infection. Fed Proc. (1984) 43:235–8.
41. Martin J, Crompton DWT, Carrera E, Mesheim MC. Mucosal surface lesions in young protein-deficient pigs infected with Ascaris suum (Nematoda). Parasitol. (1984) 88:333–40. doi: 10.1017/S0031182000054573
42. Johnson RW. Inhibition of growth by pro-inflammatory cytokines: an integrated view. J Anim Sci. (1997) 75:1244–55. doi: 10.2527/1997.7551244x
43. Martinez-Perez JM, Vandekerckhove E, Vlaminck J, Geldhof P. Serological detection of Ascaris suum at fattening pig farms is linked with performance and management indices. Vet Parasitol. (2017) 248:33–8. doi: 10.1016/j.vetpar.2017.10.009
44. Bernardo TM, Dohoo IR, Donald A. Effect of Ascaris and respiratory diseases on growth rates in swine. Can J Vet Res. (1990) 54:278–84.
45. Eriksen L, Nansen P, Roepstorff A, Lind P, Nilsson O. Response to repeated inoculations with Ascaris suum eggs in pigs during the fattening period. I studies on worm population kinetics. Parasitol Res. (1992) 78:241–6. doi: 10.1007/BF00931733
46. Urban JF, Alizadeh H, Romanowski RD. Ascaris suum: development of intestinal immunity to infectives second-stage larvae in swine. Experim Parasitol. (1988) 66:66–77. doi: 10.1016/0014-4894(88)90051-3
47. Jungersen G, Eriksen L, Roepstorff A, Lind P, Meeusen ENT, Rasmussen T, et al. Experimental Ascaris suum infection in pigs: Protective memory response after three immunizations and effect of intestinal adult worm populations. Parasit Immunol. (1999) 21:619–30. doi: 10.1046/j.1365-3024.1999.00261.x
Keywords: parasites, pigs, growth performance, carcass traits, production stage, sex
Citation: Li YZ, Hernandez AD, Major S and Carr R (2022) Occurrence of Intestinal Parasites and Its Impact on Growth Performance and Carcass Traits of Pigs Raised Under Near-Organic Conditions. Front. Vet. Sci. 9:911561. doi: 10.3389/fvets.2022.911561
Received: 02 April 2022; Accepted: 28 April 2022;
Published: 23 May 2022.
Edited by:
Vinícius Longo Ribeiro Vilela, Instituto Federal de Educação, Ciência e Tecnologia da Paraíba, BrazilReviewed by:
Hosaneide Gomes, Federal University of Campina Grande, BrazilRobert W. Li, United States Department of Agriculture, United States
Craig Robert Reinemeyer, East Tennessee Clinical Research, Inc., United States
Copyright © 2022 Li, Hernandez, Major and Carr. This is an open-access article distributed under the terms of the Creative Commons Attribution License (CC BY). The use, distribution or reproduction in other forums is permitted, provided the original author(s) and the copyright owner(s) are credited and that the original publication in this journal is cited, in accordance with accepted academic practice. No use, distribution or reproduction is permitted which does not comply with these terms.
*Correspondence: Yuzhi Z. Li, eXV6aGlsaUB1bW4uZWR1