- 1Department of Veterinary Clinical Sciences, College of Veterinary Medicine, Iowa State University, Ames, IA, United States
- 2Department for Small Animals, College of Veterinary Medicine, University of Leipzig, Leipzig, SN, Germany
Over the last decade, chronic inflammatory enteropathies (CIE) in dogs have received great attention in the basic and clinical research arena. The 2010 ACVIM Consensus Statement, including guidelines for the diagnostic criteria for canine and feline CIE, was an important milestone to a more standardized approach to patients suspected of a CIE diagnosis. Great strides have been made since understanding the pathogenesis and classification of CIE in dogs, and novel diagnostic and treatment options have evolved. New concepts in the microbiome-host-interaction, metabolic pathways, crosstalk within the mucosal immune system, and extension to the gut-brain axis have emerged. Novel diagnostics have been developed, the clinical utility of which remains to be critically evaluated in the next coming years. New directions are also expected to lead to a larger spectrum of treatment options tailored to the individual patient. This review offers insights into emerging concepts and future directions proposed for further CIE research in dogs for the next decade to come.
Update/perspective on disease pathogenesis
CIE characterizes an exaggerated immune response and has a multifactorial pathophysiology (Figure 1). The immunopathology of CIE results from a complex interplay between elements of innate and adaptive immunity (1). The innate immune system as the first line of host defense is characterized by a system of circulating cells and molecules, sentinel cells, and cellular molecules that orchestrate a complex immune reaction. These signaling pathways including the inflammasome modulate the adaptive immune response, which in turn is subject to adaptive control. Advances in the fields of genomics, microbiome, and metabolomics over the last decade have revealed further important aspects of CIE pathogenesis, but evaluation of the canine CIE exposome (i.e., the life-course of environmental effects or modulators of disease risk) is still in its infancy.
Disease prevalence and subclassification
Chronic inflammatory enteropathies are diagnosed based on the presence of chronic gastrointestinal signs (≥3 weeks), histopathologic evidence of intestinal mucosal inflammation, and the exclusion of other underlying causes (1–3). The true prevalence of CIE in dogs remains unknown, but it is estimated to present 1–2% of the cases in referral settings (3), and up to 20–30% of veterinary visits in companion animals are reported to be related to vomiting/diarrhea (4). Any dog, regardless of breed, can be affected by CIE (5).
Canine CIE is currently further subclassified (usually retrospectively) based on the response to treatment. Dogs are categorized as food-responsive enteropathy (FRE) if the clinical signs resolve or significantly improve within 2–4 weeks after starting an elimination diet trial with either a limited-ingredient novel protein and carbohydrate source (commercial or home-cooked diet) or a hydrolyzed protein diet. FRE is the most prevalent group (50–65%) of CIE in dogs (Figure 2), and affected dogs are typically younger and have less severe clinical signs than dogs with other CIE subclasses (2, 3, 6–8).
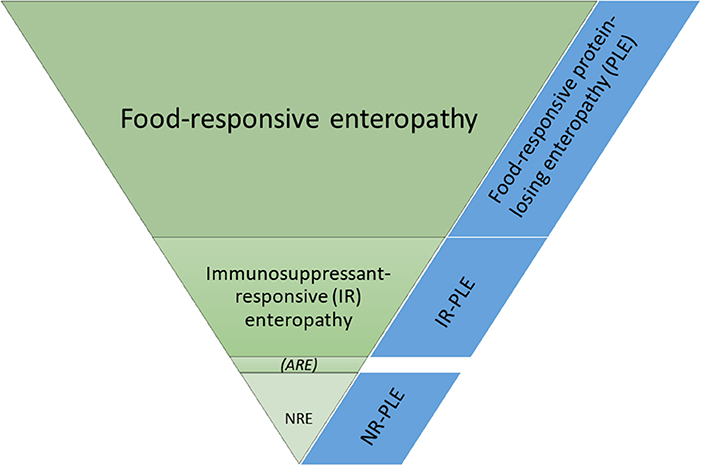
Figure 2. Frequencies of the different subgroups of chronic inflammatory enteropathies in dogs. ARE, antibiotic-responsive enteropathy (idiopathic intestinal dysbiosis); NR, non-responsive; NRE, NR enteropathy.
Excellent long-term responses in dogs with FRE can be achieved with commercial hydrolyzed protein or limited-ingredient novel protein diets (2, 9, 10). Use of a hydrolyzed protein diet is the preferred approach by the authors and some clinicians given the clinical impression of a superior response over novel protein/novel carbohydrate diets and the incomplete dietary histories (e.g., treats) in many affected dogs (11). Beneficial effects of feeding a limited ingredient elimination or hydrolyzed protein diet on the gastrointestinal microbiome and metabolome have been demonstrated (12–14). However, the response to different hydrolyzed protein diets varies, and an insufficient clinical response to one diet does not exclude the possibility of remission with another type of hydrolyzed protein diet (15), making a second elimination diet trial essential before either reaching for immunomodulatory drugs and/or more invasive diagnostics (Figure 3).
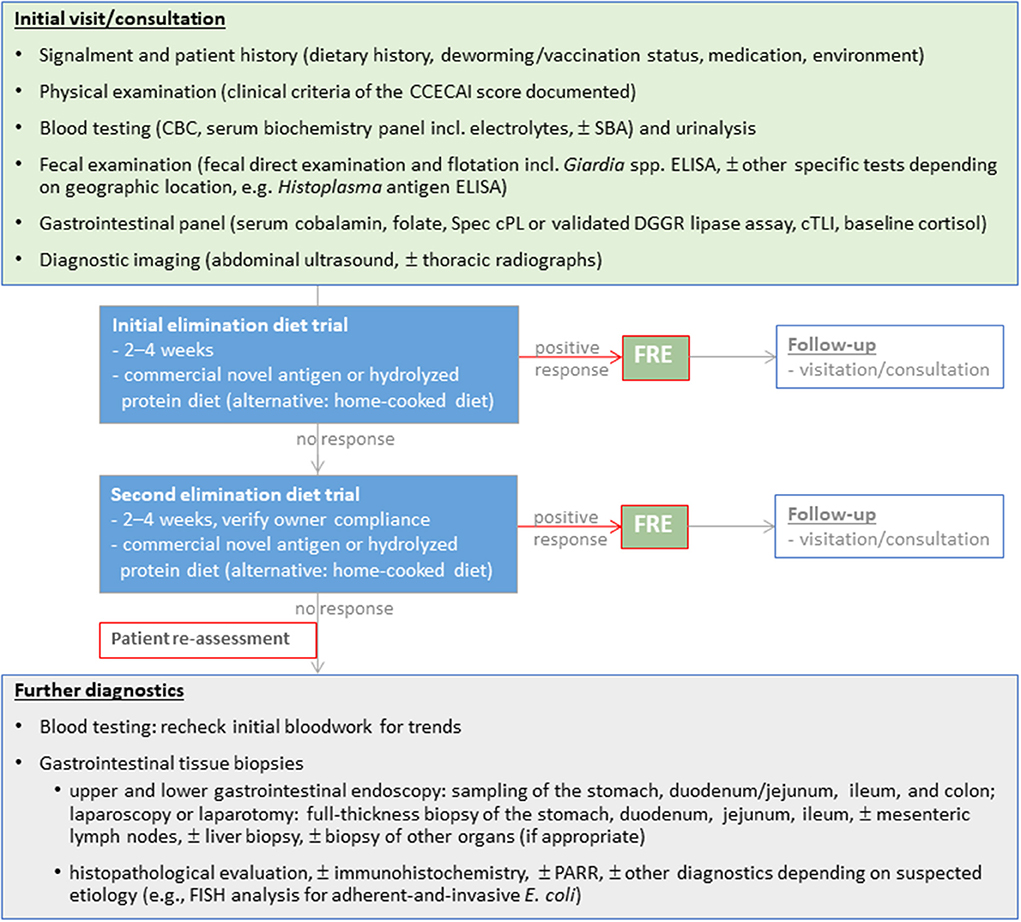
Figure 3. Diagnostic evaluation of dogs suspected with chronic inflammatory enteropathy (CIE). Selection and sequence of the individual diagnostic steps might vary and have to be tailored to the individual patient.
Dogs that show a marked and long-lasting improvement or resolution of their clinical signs after administering an antibiotic (metronidazole and/or tylosin) for 2 weeks were previously classified as ARE (3). However, antimicrobial treatment can have a long-lasting effect on the intestinal microbiome (16–20), increasing antimicrobial resistance is of major global concern, and most dogs that initially respond to an antibiotic trial will relapse after discontinuing treatment (8). Thus, the true existence of the ARE subgroup (proposed to be renamed “idiopathic intestinal dysbiosis”) of canine CIE and the appropriateness of empirical antimicrobial treatment trials in the diagnostic work-up of canine CIE is currently debated (8, 21, 22).
Dogs that require treatment with glucocorticoids or other immunosuppressive drugs are diagnosed as steroid-responsive (SRE) or immunosuppressant-responsive enteropathy (IRE) (2, 3, 21), which is a diagnosis of exclusion and often referred to as idiopathic inflammatory bowel disease (IBD). Thus, the diagnostic evaluation of dogs suspected to have SRE/IRE is complex and presents a challenge for many owners of affected dogs. Medical treatment of dogs with SRE/IRE usually involves the administration of glucocorticoids (predniso[lo]ne or budesonide) and/or other immunosuppressive drugs (cyclosporine, chlorambucil, or azathioprine) and follows a top-down approach (2, 3, 21, 23). Mycophenolate mofetil is typically not a good choice due to the high rate of gastrointestinal adverse effects (24). Targeted pathway-specific treatment strategies (e.g., therapeutic monoclonal antibodies targeting pro-inflammatory cytokines [TNF-α blockers] and integrins [integrin blockers]) would be desirable glucocorticoid-sparing treatment options for canine SRE/IRE but are currently not available in veterinary medicine and the pathways addressed using biologicals in human gastroenterology are those of Th1-driven intestinal inflammation that has not been shown to be present in dogs.
Approximately 15–43% of dogs classified as SRE/IRE have no adequate response to medical treatment and are categorized as non-responsive enteropathy (NRE), carrying a worse long-term prognosis and a high rate of euthanasia (25, 26). Further studies need to determine if these dogs will benefit from additional or alternative immunomodulatory treatments, including probiotics (e.g., Enterococcus faecium or the highly concentrated probiotic strains found in Visbiome), prebiotics (e.g., β-1,3/1,6-D-glucan), or synbiotics (27–34), fecal microbiota transplantation (FMT) (35–37), or stem cell therapy (38).
Protein-losing enteropathy (PLE), as a special form of chronic enteropathies in dogs, can be secondary to a severe diffuse infiltrative intestinal disease process causing lymphatic vascular obstruction (e.g., lymphoplasmacytic enteritis with SRE/IRE) or due to dysfunction of the intestinal lymphatics with primary intestinal lymphangiectasia (39, 40). Based on the response to treatment, PLE is subcategorized as either “food-responsive” PLE (FR-PLE) or non-FR-PLE requiring additional immunosuppressant treatment. Another subtype of PLE in dogs is focal lipogranulomatous lymphangitis (FLL) which is clinically indistinguishable from CIE and PLE, but abdominal ultrasonography typically reveals an increased wall thickness of the distal small intestine (jejunum, ileum, or both) with focal or solitary mass-like serosal lesions that can extend to the mesentery (41, 42).
Role of genetics—Breed-specific enteropathies
Canine CIE is a multifactorial disease likely caused by complex interactions between mucosal immunity and the environment (i.e., diet, gut microbiota) in genetically susceptible hosts, like human IBD (43, 44). Genetic susceptibility plays an important role in the development of ulcerative colitis and Crohn's disease in humans as genome-wide association studies (GWAS) have identified >100 risk alleles involving genes important in host-microbiome interactions (45, 46). Supporting the notion that host genetics play a similar role in CIE pathogenesis is the observation that certain dog breeds are predisposed to idiopathic IBD (5). However, few causal genetic defects have been identified at this time.
The association between specific breeds [e.g., Boxers and German shepherd dogs (GSD)] and their clinical response to antibiotics points to a potential interaction between host immunity and the microbiota (44). Previous studies have demonstrated upregulated expression of Toll-like receptors (TLR) 2, 4, and 9 in different breeds of dogs with CIE (47, 48), including GSD with IBD where TLR4 was upregulated and TLR5 downregulated at the mRNA level (49). Further studies have identified two non-synonymous single nucleotide polymorphisms (SNPs) in the TLR4 gene and three non-synonymous SNPs in the TLR5 gene that are significantly associated with IBD pathogenesis in GSD (50). Interestingly, all the SNPs associated with IBD involved a change in the class of amino acid coded which could change the structural properties of the TLR4 and TLR5 receptors, thereby altering innate immune responses. A preliminary GWAS suggests that five candidate genes associated with IBD in humans could potentially be associated with PLE in Gordon Setters (51). Boxer dogs and French Bulldogs with granulomatous colitis (GC) show sustained remission following eradication of mucosal invasive E. coli that are phylogenetically related to an adherent and invasive pathotype seen with Crohn's ileitis (52, 53). While preliminary GWAS in Boxers suggested neutrophil cytosolic factor 2 (NCF2) involved with killing intracellular bacteria as a candidate, subsequent studies now implicate various virulent genes in these bacteria as causing GC-associated mucosal inflammation (54).
Celiac disease in humans causes small intestinal inflammation with high heritability and a strong human leukocyte antigen (HLA) component (55). This strong genetic association reflects the central role of CD4+ T cells as the HLA molecules associated with celiac disease bind specific gluten peptides that activate proinflammatory T cells (56). Soft-coated Wheaton terriers (SCWT) have a well-recognized hypersensitivity reaction to different foods that may cause PLE and protein-losing nephropathy (57). Pedigree analysis from 188 SCWT demonstrated a common male ancestor, although the mode of inheritance remains unknown (58). Perinuclear cytoplasmic antibodies (pANCA) are observed with ulcerative colitis in humans (59) and in dogs with IBD, where their occurrence preceded the development of hypoalbuminemia by an average of 2.4 years (60). In a separate study, pANCA was investigated in 65 dogs with CIE at initial examination and in response to treatment. Elevated pANCA levels were observed in 62% and 23% of dogs with FRE and SRE/IRE, respectively; however, there was no difference in pANCA titers between disease groups after treatment (61). Expression of pANCA was detected in 38/104 (37%) dogs with IBD as compared to 4/23 (17%) of dogs with intestinal lymphoma in another investigation (62). These shared observations suggest that autoantibody formation indicative of immune dysgregulation occurs in some dogs with different forms of CIE but is most common in dogs with FRE. Other breed-associated GI diseases having a suspected genetic basis for their occurrence include Shar peis with cobalamin deficiency (63) and Irish setters with gluten-sensitive enteropathy (64).
While the role of genetics in CIE is currently being unraveled, there is evidence in humans and dogs that early life exposures can impact the intestinal microbiome and host response to influence the risk of disease later in life. After birth, the colonizing microbiota promotes development of mucosal immunity (a “weaning reaction”), which imprints the immune system to maintain its responsiveness after weaning and into adulthood. When host-microbe crosstalk is perturbed in early life, inhibition of the weaning reaction leads to pathological imprinting, which causes increased susceptibility to inflammatory pathologies in individuals that have not been exposed to a microbiota, or possibly a dysbiotic microbiota (65, 66). Both experimental (67) and epidemiological studies (68) support this concept of a period of vulnerability for IBD development. One recent study has investigated the long-term effects of parvovirus infection in dogs and its association with chronic gastroenteritis (69). Results of this study suggested that dogs that survived canine parvoviral infection were at increased risk (odds ratio = 5.33) for developing CIE compared to control dogs. Another study found that dogs having an episode of acute hemorrhagic diarrhea were at increased risk (odds ratio = 2.57) for developing chronic GI disease vs. controls later in life (70). These collective observations would support the two-hit model (i.e., the combination of genetic and environmental factors) proposed for IBD pathogenesis (71).
Immunopathogenesis of chronic intestinal inflammation
The pathogenesis of canine CIE is multifactorial and involves a loss of tolerance to diet and microbial components that cause an aberrant immune response in genetically susceptible hosts (Figure 1). As previously noted, several lines of evidence support innate immune dysregulation as contributing to the development of CIE, like IBD in humans (5, 72). Candidate gene analysis has shown that defects in pattern recognition receptors (TLR4 and TLR5) of the innate immune system are associated with a breed-specific form of CIE in GSDs (50). In a follow-up study, these same investigators showed, in both in vitro and ex vivo assays, that the canine risk-associated TLR5 haplotype results in hyper-responsiveness to flagellin vs. the response associated with the CIE risk-protective TLR5 haplotype (73). Candidate gene mutations and altered mRNA expression were observed for NOD2 with IBD in GSDs (74, 75).
Regarding adaptive immunity, there has been considerable work to define the role of immune cells and cytokines in CIE. Immunohistochemical investigation of intestinal biopsies has shown no overall difference in total lamina propria (LP) cells in dogs with IBD compared to controls. However, there are differences in the phenotype of LP immune cell subsets with increased numbers of T cells (especially those expressing the αβTCR and CD4+), IgG+ plasma cells, macrophages, and granulocytes, but fewer mast cells (76). Increased intraepithelial lymphocyte counts are also noted. In dogs with lymphoplasmacytic colitis, there are increased numbers of IgA+ and IgG+ plasma cells and T cells within the LP and epithelial compartments (77). Analysis of the clonality of B- and T-cell receptors showed reduced diversity in the intestines of dogs with IBD, suggesting expansion of lymphocyte clones with select antigenic specificities (78). Studies of cytokine gene expression in mucosal biopsies have yielded mixed results. One study examining mucosal cytokine expression in GSDs diagnosed with either ARE or IBD and healthy dogs using semi-quantitative reverse transcriptase-polymerase chain reaction (RT-PCR) showed significantly higher mucosal mRNA expression of IL-2, IL-5, IL-12p40, TNF-α, and TGF-β1 in dogs with CIE than in healthy controls (79). A similar study using quantitative RT-PCR (qPCR) showed no differences in IL-2, IL-4, IL-5, IL-6, IL-10, IL-12, IL-18, IFN-γ, TNF-α, TGF-β, and glyceraldehyde-3-phosphate dehydrogenase expression in dogs with and without CIE (80). Separate reviews on this topic have concluded that most dogs with CIE express a balanced Th1/Th2 cytokine profile (81, 82). There is also no evidence of a Th17 signature in dogs with CIE (83). This observation in canine CIE contrasts to human IBD, where distinct Th1 (i.e., cell-mediated) and Th2 (i.e., humoral) cytokine polarization occurs in Crohn's disease and ulcerative colitis patients, respectively (84).
Evidence of immunological dysfunction in CIE also includes the expression of mucosal nitrite, a precursor of the inflammatory mediator of nitric oxide (NO) in dogs with idiopathic IBD (85) and increased numbers of inducible nitric oxide synthase (iNOS)-expressing cells associated with PAS+ macrophages in biopsies of dogs with GC (86). Other systemic and locally produced biomarkers of inflammation, including C-reactive protein (CRP), pANCA, and fecal calprotectin, are discussed in a comprehensive review (87). A study using gene expression microarray to investigate intestinal gene expression profiles in dogs with CIE compared to healthy controls showed >1,500 genes (85%) to be downregulated with CIE, including neurotensin, fatty acid-binding protein 6, fatty acid synthase, aldehyde dehydrogenase-1 family member B1, metallothionein, and claudin 8 (88). Few genes were upregulated in dogs with CIE, including those involved in extracellular matrix degradation (matrix metallopeptidases 1, 3, and 13), inflammation (TNF, IL-8, peroxisome proliferator-activated receptor-γ, and S100 calcium-binding protein G), iron transport (solute carrier family 40 member 1), and immunity (CD96 and carcinoembryonic antigen-related cell adhesion molecule [CEACAM]) (88). Dogs with severe CIE and PLE had the largest number of differentially expressed genes. Results of qPCR testing for select genes confirmed the microchip analysis.
MicroRNAs (miRNAs) are small, non-encoding RNAs that regulate gene and protein expression. As such, they play a crucial role in the development of cells involved in innate and adaptive immunity, and in regulating the immune response (89). Studies in human IBD indicate that tissue miRNA profiles are dysregulated in patients with active ulcerative colitis, Crohn's ileitis, and Crohn's colitis (90–92). Increased expression of miR-21 is the most consistently replicated finding in human IBD (93). Tissue and serum miRNA levels of dogs with CIE have been recently investigated. Compared to healthy controls, dogs with large intestinal IBD showed increased miR-16, miR-21, miR-122, and miR-147 expression in the colonic mucosa and serum, while the expression of miR-185, miR-192, and miR-223 was decreased (94). Furthermore, the expression of serum miR-192 and miR-223 correlated to both clinical disease and endoscopic score. These observations suggest that miRNAs are involved in the pathogenesis of canine idiopathic IBD, like human IBD.
A key element in the pathogenesis of CIE is an impaired intestinal epithelial barrier contributing to the loss of immunological tolerance. Different studies using selective sugar permeability testing to evaluate intestinal permeability in dogs with CIE have yielded conflicting results (95–97). More accurate estimates of intestinal permeability have been made using iohexol, a radiographic contrast medium, administered orally and measured in the serum of healthy dogs (98). Future studies investigating the value of iohexol clearance as an intestinal permeability test in dogs with CIE are warranted. Techniques to evaluate intestinal barrier function in vivo in humans include orally ingested probes (polyethylene glycols, 51Cr-EDTA, sugars) assessed in urine and measurement of fatty acid bindings proteins, glucagon-like peptide (GLP)-2, and LPS-binding protein (LBP) concentrations in serum (99). Apart from sugar permeability testing, these other function tests have not been critically assessed in dogs with CIE.
Hypovitaminosis B12
Assessment of the cobalamin (vitamin B12) status as a marker of intestinal absorptive capacity is of diagnostic and therapeutic value in small animal medicine (100). Cobalamin is a water-soluble B vitamin available through mostly dietary sources of animal origin and absorbed mostly via specific receptors that internalize the intrinsic factor (IF)-cobalamin complex in the distal small intestine (100). The intestinal microbiota can provide small amounts of cobalamin, but the body hardly benefits from this source because of the distal production site. Body stores, particularly in the liver, can ensure cobalamin availability for several months (100).
Cobalamin deficiency in dogs is currently determined by routine serum cobalamin measurement, often together with serum folate (vitamin B9), for which several commercial tests are available. An automated chemiluminescence test is routinely used in Europe and North America, and the reference intervals for serum cobalamin concentrations in dogs used by the various veterinary diagnostic laboratories are comparable (100).
Methylmalonic acid (MMA) is a metabolite that accumulates when the activity of cytosolic methylmalonyl-CoA mutase is decreased because of intracellular cobalamin deficiency (100). Thus, paired methylmalonic acidemia and/or aciduria due to an intracellular lack of cobalamin is a superior test for cobalamin deficiency at the cellular level (101, 102), and ideally, serum cobalamin and serum or urine MMA are used to properly assess the cobalamin status in dogs. However, measurement of MMA is not routinely performed in small animal medicine, but serum MMA tests have recently become available through some veterinary laboratories. Careful interpretation of serum MMA concentrations is warranted in dogs with renal insufficiency (103). Evaluation of serum holo-transcobalamin (cobalamin-saturated transcobalamin) in addition to or instead of serum cobalamin and MMA concentrations may more accurately reflect the cobalamin status (104). Suboptimal serum cobalamin levels were associated with higher serum transcobalamin concentrations than normocobalaminemia in dogs with CIE, but further evaluation of the clinical utility of this marker in dogs is needed.
Hypocobalaminemia or cobalamin deficiency can have several causes, the most common in dogs being chronic gastrointestinal diseases and exocrine pancreatic insufficiency [because in dogs, IF is primarily produced by the pancreas (105)]. Commercial dog foods are fortified with cobalamin; thus, dietary causes of cobalamin deficiency are unlikely unless fed a vegetarian or vegan diet without added cobalamin. Hypocobalaminemia frequently occurs in dogs with CIE (19–54%) and affects 22–75% of dogs with PLE (7, 25, 103, 106–108), which has been presumed to result from distal small intestinal cobalamin malabsorption due to ileal cobalamin receptor deficiency, secondary small intestinal dysbiosis (with increased utilization of cobalamin by the intestinal microbiota), or both. Contrary to the previously proposed pathogenetic mechanism, a recent study showed that cobalamin receptor downregulation is not the primary cause of hypocobalaminemia in canine CIE. Overexpression of the cobalamin receptor subunits amnionless (AMN) and cubilin (CUBN) in the ileum was detected and presumed to be a mechanism to compensate for CIE-associated hypocobalaminemia (109). Hypocobalaminemia was also shown to be a negative prognostic factor in dogs with CIE and to be associated with hypoalbuminemia (7, 25). However, documenting normoalbuminemia does not rule out a diagnosis of CIE. Concurrent hyperfolatemia and hypocobalaminemia suggest intestinal dysbiosis (110).
Subnormal or low normal serum cobalamin concentrations (i.e., < 350–400 ng/L) are often associated with increased serum MMA concentrations (101). These indicate a need for cobalamin replacement therapy to replete body cobalamin stores and aid with mucosal regeneration. Supplementation of cobalamin is recommended with cyanocobalamin. It was previously recommended to be given parenterally [50 μg/kg SQ once weekly for 6 weeks and another dose 1 month later, then continued every 2–4 weeks if needed (111)] while also addressing the underlying CIE. However, recent studies suggest that supplementation with oral cyanocobalamin (50 μg/kg PO q24 h for at least 12 weeks) can be as effective as parenteral administration and present a simple, non-invasive, and pain-free alternative to weekly SQ injections (112–114). Measurement of serum cobalamin concentration is recommended 1 month after the last dose to decide whether to continue cobalamin supplementation every 2–4 weeks (111). Successful treatment leads to a normalization of the biochemical findings and in most dogs, supranormal serum cobalamin concentrations. If supplementation with cyanocobalamin is not effective and serum cobalamin concentrations remain below the target range, hydroxycobalamin (presenting the natural form of cobalamin) given at a dose of 1,000 μg (for a medium-sized dog) IM q14d could be considered an alternative (115), followed by a recheck of serum cobalamin to screen for successful normalization. Oral cobalamin supplementation may particularly be effective in dogs with CIE and very high expression of the ileal cobalamin-IF-receptor, but passive diffusion across the intestinal mucosal epithelium could also explain why oral cobalamin supplementation might be effective.
Microbiome and metabolome
Intestinal dysbiosis is associated with mucosal inflammation and GI dysfunction in dogs with CIE. Microbial imbalances are characterized by broad shifts in the composition of microbes, reduced species diversity, and changes in the relative proportion of select microbial members compared to feces from healthy dogs (116–118). Most sequencing-based studies indicate reduced relative abundances of Bacteroidetes, Fusobacterium spp., and Firmicutes, and an increased abundance of Proteobacteria in fecal samples of dogs with CIE (118). A study using fluorescence in situ hybridization (FISH) to characterize the mucosal biogeography in colonic biopsies of dogs with CIE showed affected dogs to have decreased numbers of total bacteria within colonic crypts but no difference in total bacterial counts on the mucosal surface as compared to healthy control dogs (119). Numbers of mucosal E. coli were increased in dogs with CIE, like in a previous report (120). In contrast, the numbers of mucosal Helicobacter spp and Akkermansia spp were decreased in both colonic crypts and the mucosal surface, suggesting that those bacteria are beneficial resident species in the canine colon. GC in Boxers and French bulldogs is a unique form of CIE characterized by adherent and invasive E. coli (AIEC) residing within the colonic mucosa. A causal role for these bacteria in GC pathogenesis is supported by the association of remission with eradicating invasive E. coli when enrofloxacin was used (53, 121). Other studies in GSD with CIE have shown a unique dysbiosis characterized by increased Lactobacillus spp. relative to Greyhound controls (49) and a complex but variable dysbiosis in dogs with tylosin-responsive diarrhea (ARE) (122). Whether intestinal dysbiosis is a cause or consequence of mucosal inflammation in dogs with CIE remains unknown.
Assessment of the gut microbiome is best performed using molecular methods, including sequencing techniques detecting 16S ribosomal RNA genes and metagenomic shotgun sequencing (123). As both next-generation and metagenomic sequencing methods are expensive and time-consuming to perform, more rapid results with lower costs may be realized using a qPCR assay-based algorithm called the fecal dysbiosis index (DI) (124). The DI measures the abundance of 7 bacterial taxa (Fecalibacterium spp, Turicibacter spp, E. coli, Streptococcus spp, Blautia spp, Fusobacterium spp, and Clostridium hiranonis [now Peptacetobacter hominis]) and the total bacterial abundance in canine feces. The DI calculates a single number that expresses the extent of intestinal dysbiosis present: a DI < 0 indicates a normal microbiota while a DI >2 indicates dysbiosis (124). The DI can be used to assess normal vs. abnormal microbiota at a single time point or longitudinally in response to disease progression or therapeutic intervention (125–127).
Along with changes in microbial composition, functional changes in the microbiome have also been reported with canine CIE. In one study, dogs with idiopathic IBD exhibited differences in their fecal microbiome and serum metabolome compared to healthy controls (128). The serum metabolites 3-hydroxybutyrate, hexuronic acid, ribose, and gluconic acid lactone were significantly more abundant in dogs with IBD suggesting the presence of oxidative stress. Moreover, while the IBD dogs improved clinically with treatment, this was not accompanied by improvement in the microbiome or serum metabolite profiles. One group of metabolites of particular interest to host health is the short-chain fatty acids (SCFAs; e.g., butyrate, acetate, and propionate) derived from bacterial fermentation of complex carbohydrates that modulate mucosal immune responses. SCFAs are an essential energy source for colonocytes; they enhance epithelial barrier function by strengthening tight junctions and regulate the size and function of the regulatory T-lymphocyte pool to protect against mucosal inflammation (129–131). Relative abundances of SCFA-producing bacteria are decreased in dogs with both acute and chronic diarrhea (132). Examples of other beneficial microbe-derived metabolites include B vitamins and vitamin K.
Select amino acids, including tryptophan, are reduced in canine CIE (133). Tryptophan is an essential amino acid in dogs and serves as a precursor for kynurenine, serotonin, melatonin, and indole. Increased tryptophan catabolism limits the production and availability of serotonin which is an essential neurotransmitter mediating GI secretion, motility, and pain. Tryptophan also serves as a precursor to producing indole compounds by the gut microbiota that enhance mucosal homeostasis by improving gut permeability and increasing mucin production (134). Depletion of tryptophan occurs in dogs with PLE, whereas dogs with idiopathic IBD have decreased indole compounds present in their feces (133).
Intestinal dysbiosis can also trigger chronic mucosal inflammation and bile acid (BA) dysmetabolism (125). Luminal BAs are essential for lipid digestion and play major roles in maintaining mucosal homeostasis through their antimicrobial actions. In the healthy gut, select bacteria are required for deconjugation and dehydroxylation of primary BAs into secondary BAs. With dysbiosis, the decreased abundance of Peptacetobacter (Clostridium) hiranonis, a bacterium associated with normal BA metabolism, causes increased concentrations of primary BAs in the colon, which are pro-inflammatory and may cause secretory diarrhea (134). Bile acid diarrhea (BAD) occurs in a subset of humans with Crohn's disease and diarrhea-predominant irritable bowel syndrome (135). A recent report describes treatment of presumptive BAD in two dogs with CIE using the bile acid sequestrant cholestyramine (136). Taken together, these observations suggest that intestinal dysbiosis perturbs BA metabolism which in turn further promotes intestinal inflammation and GI signs. Dogs with CIE that are non-responsive to traditional therapies might respond to empirical treatment with bile acid sequestrants. Lastly, clinical remission accompanied by improved microbial composition, expansion of BA-producing bacteria, and increased levels of secondary BAs has been reported in dogs with FRE fed a hydrolyzed protein diet (14).
Update/perspective on diagnostic strategies
The diagnostic work-up of dogs with suspected CIE remains complex (Figure 3) and comprises a thorough patient history, physical examination, clinicopathologic evaluation and diagnostic imaging to rule out other gastrointestinal or extra-gastrointestinal etiologies that can mimic CIE (1, 3, 21). Histologic evidence of mucosal inflammation confirms the diagnosis, and appropriately designed treatment trials aid in classifying the disease to determine the optimal individual treatment plan (3, 21). Disease-independent clinical scoring systems can semi-objectively assess the clinical disease severity at the time of diagnosis and monitor patient improvement during treatment (25, 137). Several biomarkers can aid in the diagnostic evaluation and/or monitoring dogs with CIE (87).
Gastrointestinal endoscopy, mucosal assessment, and mucosal healing
The decision to perform GI endoscopy in dogs with CIE is best determined on a case-by-case basis. Treatment trials are the best tools to differentiate the varied forms of CIE as clinical history, laboratory findings, biomarkers of inflammation, and histologic abnormalities have not proven reliable to distinguish one form of CIE from another. While many dogs will quickly respond to elimination dietary trials, non-responders will require reassessment and further diagnostic testing including GI endoscopy to rule out other causes for chronic GI signs (Figure 3). Endoscopic biopsy of the GI tract provides rapid, relatively non-invasive, direct assessment of mucosal lesions and permits directed biopsy for histopathologic evaluation (138, 139). Other advantages of flexible endoscopy include the collection of multiple tissue samples from different alimentary (stomach, duodenum/proximal jejunum, ileum, and colon) sites and minimal risks (perforation) associated with the procedure. It is particularly useful in diagnosing infiltrative, erosive, or anatomic (lymphangiectasia) lesions seen with different forms of CIE, but especially SRE/IRE or IBD, as well as low-grade intestinal lymphoma (140). Severe weight loss, prolonged anorexia, poor body condition, hypoalbuminemia, ultrasonographic evidence of substantial infiltrative disease, or a combination of these findings indicates that GI endoscopy should be performed earlier during diagnostic testing rather than after (unsuccessful) therapeutic trials. Practical considerations for endoscopy include the role of ileal biopsy (141), acquisition of good quality tissue specimens (142), proper tissue processing that avoids artifacts and permits optimal tissue orientation in the laboratory (143), and the effect of endoscopic forceps on quality of mucosal biopsy (144).
Careful and thorough examination of mucosal surfaces should be performed and documented using standardized reporting. In this regard, the original World Small Animal Veterinary Association (WSAVA) guidelines recommend using a standardized endoscopic report form with check boxes to identify the presence/severity of mucosal lesions when endoscopy is performed (https://wsava.org/global-guidelines/gastrointestinal-guidelines/) (145). Determining the presence of mucosal inflammation at diagnosis or following treatment is an important consideration in humans with IBD. Measures of mucosal erythema, increased granularity, vascular pattern (colon), spontaneous bleeding, and/or mucosal damage (mucus, fibrin, exudates, erosions/ulceration) have been used in clinical trials (146, 147). Mucosal healing (MH) is an important treatment endpoint in human IBD and achieving MH has been demonstrated to improve patient-related outcomes (146, 148). Broadly, MH represents the absence of friability, erosions, and ulceration in patients with ulcerative colitis and Crohn's disease.
There are few studies evaluating the GI mucosal appearance of dogs with CIE following treatment. One prospective single-center study evaluated the sequential treatment response of 70 dogs with CIE placing them into FRE, ST (steroid-treated), and PLE cohorts (25). Gastroduodenoscopy and colonoscopy were performed in all dogs at diagnosis (except those with panhypoproteinemia), while repeat endoscopy was performed in ST dogs and a subset of ST dogs requiring treatment escalation. Endoscopic indices included the presence of mucosal erythema, friability, granularity, ulceration, white speckling, and degree of insufflation. While there was no difference in endoscopy scores among the FRE, ST, and treatment escalation groups, an increased endoscopy score indicating severe duodenal inflammation was associated with negative long-term outcome. A similar study evaluated the clinical, endoscopic, and histopathologic response to treatment in non-hypoproteinemic dogs with lymphoplasmacytic enteritis (149). Different macroscopic lesions to the gastric and duodenal mucosa were identified at diagnosis and following 120 days of diet and medical (prednisone and metronidazole) therapy. Seventy-five percent of dogs showed improvement in endoscopic gastric and duodenal lesions (defined as a reduction in the total macroscopic score post-treatment) indicative of MH. These two studies demonstrate the feasibility of using GI endoscopy to assess MH and outcome in dogs with CIE.
The WSAVA endoscopic examination reporting forms for upper and lower GI endoscopy include various descriptors (hyperemia, edema, discoloration) and subjective gradations (mild, moderate, severe) that may make it difficult to accurately quantitate mucosal inflammation. Furthermore, studies evaluating the interobserver agreement for grading mucosal lesions amongst endoscopists using the WSAVA endoscopic score are lacking. One recent study investigated the interobserver agreement in the endoscopic assessment of mucosal appearance of the GI tract to design and validate a simple and easy-to-use endoscopic scoring system for dogs with idiopathic IBD (150). Validation studies using endoscopic videoclips to assess the same endoscopic parameters defined by quantitative (lesion number and severity) vs. qualitative (present or absent) methods (Figures 4A–F) showed moderate-to-substantial agreement between experienced endoscopists. Since the quantitative and qualitative scores of mucosal appearances were very similar, an endoscopic activity score that incorporates only the presence/absence of four mucosal variables, and not their magnitude or the extent of their severity, is proposed for use in the endoscopic assessment of mucosal inflammation (Table 1). Future studies are required to confirm correlation of the simple endoscopic score to clinical activity and laboratory biomarkers of inflammation in dogs with CIE.
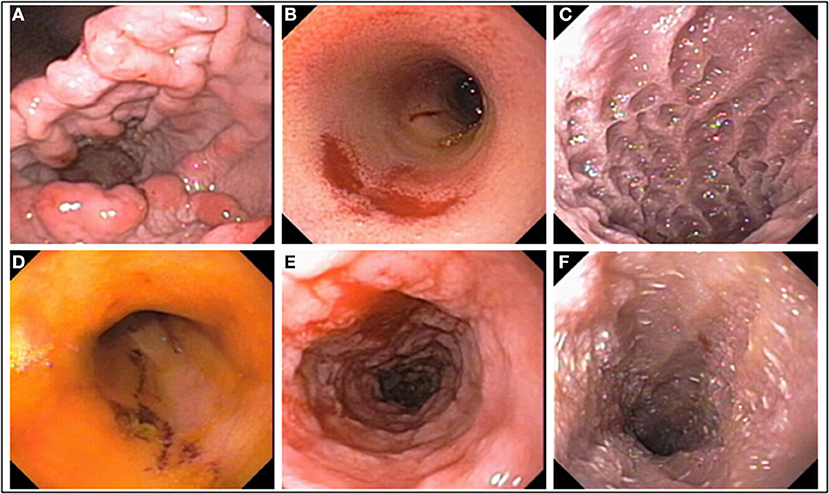
Figure 4. Mucosal abnormalities used in calculation of the simple endoscopic score. (A) Gastric granularity, (B) duodenal erosions, (C) duodenal granularity, (D) ileal erosions, (E) colonic granularity, and (F) lymphangiectasia, duodenum.
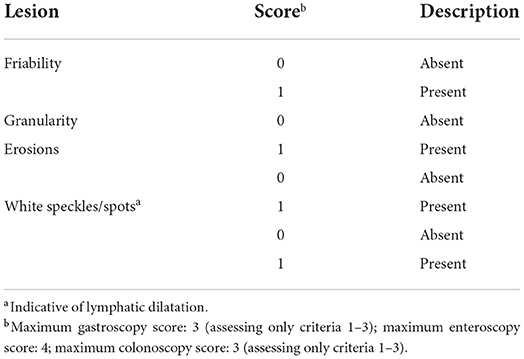
Table 1. Simple endoscopic score for canine chronic enteropathy [modified from (151)].
Histologic guidelines for gastrointestinal inflammation
Diagnosis of intestinal inflammation in dogs with CIE has historically posed great challenges for clinicians and veterinary pathologists. While intestinal biopsies can be acquired endoscopically or surgically, GI endoscopy is most often utilized. Several fundamental issues in the diagnostic process have included: (1) How can small and fragile endoscopic specimens of variable quantity from different regions of the GI tract be used for histologic assessment? (2) What constitutes an acceptable biopsy with regards to size and diagnostic quality? (3) Are standardized grading schemes of value in defining histologic inflammation? If so, what are the key criteria used for assessment (i.e., type/quantity of cellular infiltrate, mucosal architectural changes, other changes) and current recommendations for their use? (4) Is there a relationship between histologic changes and GI signs? and (5) Is there a relationship between histologic changes and response to treatment? To address these concerns, numerous histologic grading schemes characterizing the nature and severity of GI inflammation have been designed. Within most of these model systems, there is an emphasis on the type and severity of lamina propria cellularity that is variable and subjectively graded as normal, mild, moderate, or severe. It is now recognized that different types of cellular populations (e.g., lymphoplasmacytic, eosinophilic, and granulomatous) may overlap and occur in different types of GI diseases. For example, lymphoplasmacytic enteritis is a common feature in dogs with idiopathic IBD (25, 77, 151, 152) and is described in association with PLE (153). The reliance on mucosal cellularity as a key feature of GI inflammation has meant that abnormalities in mucosal architecture (i.e., epithelial lesions, villous morphology, lymphatic dilatation, fibrosis, and crypt changes) have been overlooked but may correlate best with inflammatory markers and clinical severity of GI disease (53, 154). The quality of endoscopic specimens (influenced by operator experience in detecting mucosal lesions) (143) and the effect of tissue processing (155) are additional limitations affecting histologic assessment. These findings and the observation that substantial variation occurs between pathologists when interpreting GI histologic findings (156) emphasized the need to develop uniform guidelines for evaluating GI histologic findings. Against this background, the WSAVA GI Standardization Group provided the first comprehensive guidelines for the standardized interpretation of inflammatory changes in the GI mucosa of dogs and cats (145). These guidelines included a visual and textual description of morphologic and inflammatory changes in the stomach, duodenum, and colon ranging from mild to severe as compared to normal GI histology.
A limitation of the WSAVA score is that it did not consider goblet cells as a morphologic parameter, where their numbers decrease with inflammation but increase in response to successful treatment (121, 154). Using the 253 animals from the original WSAVA study, a simplified histopathologic model for GI inflammation was designed to improve the consistency of interpreting GI inflammation between pathologists (157). This refined and reductionist model used statistical analysis to identify those WSAVA parameters that showed the least interpretive variation between pathologists of the original report (Table 2). The simplified model incorporates the number of goblet cells as a morphologic feature to grade when evaluating colonic endoscopic specimens. While histopathologic guidelines for interpretation of ileal mucosal specimens were not proposed in the WSAVA scheme, it is recommended to use the simplified model criteria of duodenal inflammation for microscopic evaluation of ileal biopsy specimens. Besides the original report, this model has been applied successfully to characterize the severity of histologic inflammation in biopsy-diagnosed CIE with and without hypoalbuminemia (158) and has shown to positively correlate with clinical disease activity in dogs with idiopathic IBD (159).
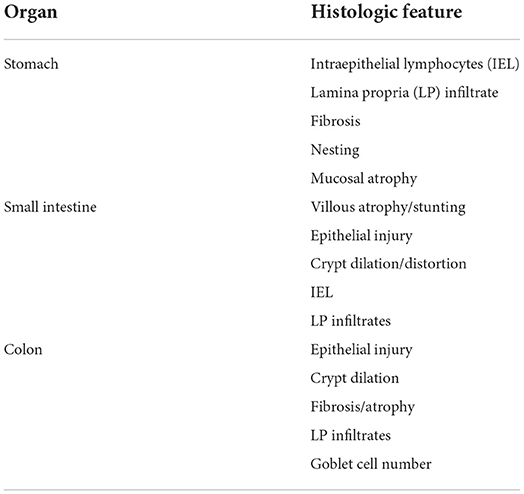
Table 2. Histopathologic features of the simplified histopathologic model for gastrointestinal inflammation [from (157)].
Endoscopic biopsy is often performed in dogs with chronic or intermittent GI signs that fail to respond to sequential treatment interventions. In these instances, it is expected that the histologic findings contained in tissue samples will confirm a diagnosis and guide treatment decisions in these patients. However, separate trials performed by different investigators have failed to show a convincing association of histopathologic findings in endoscopic specimens with clinical disease activity, biomarkers of inflammation, or response to therapy and outcome in dogs with idiopathic IBD (25, 26, 32, 150, 160, 161). The failure to demonstrate an association in these earlier studies might relate to the use of non-standardized histologic grading systems and/or differences in study design. In one recent study, the simplified GI scoring system demonstrated significant correlations with histologic scores and clinical indices (25). Compared to earlier grading schemes, the simplified score showed improved utility in correlating histologic features (total summative histologic scores in the duodenum and colon and select histologic scores) to IBD clinical activity.
Other factors contributing to potential discordance between histologic findings and clinical signs include the quality of endoscopic specimens and failure to biopsy the intestinal compartment most affected by a pathologic process. The effect of sample quality in detecting select histologic lesions in gastric and duodenal biopsies of dogs was investigated in one study showing that endoscopic biopsy quality (i.e., adequate vs. inadequate specimens) significantly affects the sensitivity to detect certain histologic lesions (142). As the quality of the endoscopic sample improved from inadequate to marginal to adequate, fewer endoscopic samples were required to diagnose duodenal lesions and most gastric lesions. Other investigations have focused on the value (extent of agreement in histologic changes) of simultaneous duodenal and ileal biopsies in dogs with CIE. In a separate study, there was poor agreement between pathologic lesions from duodenal vs. ileal biopsies, with a greater number of histologic abnormalities detected in the ileum of dogs with concurrent small and large intestinal diarrhea (162). Finally, in dogs with chronic small intestinal enteropathy, there was only slight agreement between duodenal and ileal histologic scores, except for the correlation of hypoalbuminemia with ileal lacteal dilatation and the number of ileal intraepithelial lymphocytes (141). These combined observations indicate that collection of concurrent duodenal and ileal biopsies is warranted in dogs with CIE requiring GI endoscopic biopsy.
Changes in the ultrastructural lesions of duodenal biopsies (i.e., enterocytes) have been reported in dogs with diet-responsive CIE before and after dietary treatment (163). Using both qualitative and quantitative measures of ultrastructural features, dietary therapy in dogs with FRE decreased the mean mitochondrial injury score and intermicrovillar space of intestinal epithelia and increased the mean microvillar height indicating recovery of enterocyte mitochondrial and brush border health.
Biomarkers of inflammation
Clinically useful biomarkers help detect disease, determine the severity of the disease process, and monitor the response to treatment. Several inflammatory biomarkers have been researched during the last decade and hold significant promise as diagnostic and/or monitoring tools in canine CIE (87). These include CRP, calprotectin, S100A12, N-methylhistamine (NMH), 3-bromotyrosine (3-BrY), and soluble receptor of advanced glycation end-products (sRAGE).
Serum C-reactive protein (CRP) concentration is a non-specific marker of inflammation (87). CRP is a positive type II acute-phase protein expressed in the liver in response to infection, inflammation, or cancer (164, 165). Several assay formats are available for the quantification of canine CRP in serum (166–172), which have reference intervals of approximately < 10 mg/L (173). A high-sensitivity CRP (hs-CRP) assay with improved sensitivity for lower serum CRP concentrations would be highly desirable but is currently not routinely available in small animal medicine (174).
Due to the high inter-individual biological variability of serum CRP concentrations in dogs (175), its utility as a diagnostic biomarker in canine CIE is limited. Still, a serum CRP concentration ≥9.1 mg/L was highly specific (100%) to detect dogs requiring anti-inflammatory or immunosuppressive treatment (SRE/IRE and NRE) among all CIE cases including those responding to an elimination diet (FRE) or antimicrobial treatment (ARE) (87, 108). Serum CRP is also useful as a surrogate biomarker to assess disease progression and response to treatment in canine CIE (108, 176, 177), but serum CRP concentration needs to increase or decrease at least 2.7-fold to be considered a clinically relevant change (175). Increased serum CRP levels are not specific for the gastrointestinal tract, but the marker can be used in corticosteroid-treated dogs.
Fecal calprotectin concentration appears to be a useful biomarker of intestinal inflammation in dogs (108, 177, 178). The calprotectin protein (S100A8/A9) complex belongs to the damage-associated molecular pattern (DAMP) molecules (alarmins) and accumulates at sites of inflammation (179). It is a ligand for Toll-like receptor (TLR)-4 (180), which plays a role in the pathogenesis of canine CIE (44, 46). The stability of the protein complex allows for its extraction and measurement in canine fecal samples (181, 182).
Using a cut-off concentration of ~50 μg/g, fecal calprotectin is a good surrogate marker of severe clinical disease in canine CIE (108, 177, 178). Fecal calprotectin may help predict the response to treatment in dogs with CIE, with levels ≥15.2 μg/g distinguishing partial responders or non-responders (NRE) from SRE/IRE dogs that achieve complete clinical remission with high sensitivity (80%) and moderate specificity (75%) (108). Careful selection of canine patients for fecal calprotectin testing is important to gain relevant information from this biomarker as increases also occur with acute gastrointestinal inflammatory conditions (183). Fecal calprotectin measurement in dogs is currently not routinely available, but a human immunoturbidimetric assay that can be run on standard laboratory chemistry analyzers has shown promise for fecal samples from dogs (182, 184).
Fecal S100A12 (calgranulin C) is another sensitive and specific marker of localized inflammatory processes in dogs (107, 185) and has a cellular distribution similar to calprotectin (179). The S100A12 protein is a DAMP molecule with several target proteins, including the receptor for advanced glycation end-products (RAGE) with a central role in innate and acquired immune responses (186). S100A12 is stable in fecal samples and can be measured after extraction (187).
The severity of clinical signs and endoscopic lesions, but not histopathologic changes, correlates with fecal S100A12 levels in dogs with CIE (107, 185), and the marker may also aid in predicting the response to treatment and disease outcome. Dogs with CIE and a fecal S100A12 concentration < 490 ng/g are very likely to respond to an elimination diet (and/or antibiotic) trial (i.e., diagnosis of FRE/ARE) and not require anti-inflammatory or immunosuppressive treatment (negative predictive value >80%) (87, 107). On the other hand, dogs with SRE/IRE and a fecal S100A12 concentration < 2,700 ng/g are very likely to at least partially respond (i.e., show clinical improvement or remission) to treatment (negative predictive value ~100%) (87, 107). Increased fecal S100A12 levels were also linked to individual patient outcomes in canine SRE/IRE (87, 188). Careful patient selection for using this marker is also important, given that fecal S100A12 levels can increase with acute gastrointestinal inflammation (183). Fecal S100A12 levels may be more specific for inflammation than fecal calprotectin concentrations, but measurement of fecal S100A12 in dogs is currently not routinely available.
N-methylhistamine (NMH) can be measured in serum, urine, and fecal samples (189, 190) and, as a stable product of histamine metabolism, reflects mast cell activation and degranulation in canine CIE (190–192). Increased urine NMH concentrations are detected in ~40% of dogs with CIE and correlate with the severity of histologic changes, but not the numbers of mast cells in the duodenum or the clinical disease severity (189, 190). Further studies are needed to determine the clinical utility of measuring NMH levels in dogs with CIE.
Serum 3-bromotyrosine (3-BrY), a stable metabolite of eosinophil peroxidase, is a biomarker of eosinophilic inflammation (193). Dogs with CIE have increased serum 3-BrY concentrations, likely reflecting an eosinophilic component or mixed inflammatory infiltrate (194). Requiring anti-inflammatory or immunosuppressive treatment (i.e., SRE/IRE diagnosis) was associated with higher serum 3-BrY concentrations than responding to an elimination diet (i.e., FRE) (195), but the diagnostic accuracy of serum 3-BrY and corresponding cut-off levels to differentiate CIE subgroups remain to be determined.
Serum soluble RAGE (sRAGE) as an anti-inflammatory decoy receptor can sequester ligands such as DAMP molecules (e.g., S100A12) and abrogate cellular proinflammatory RAGE responses (196, 197). Dogs with CIE have an upregulated intestinal transmembrane RAGE expression (198) and decreased serum sRAGE levels that correlate weakly with histologic lesions in the duodenum but not with the severity of clinical signs or patient outcomes (188, 197). With serum sRAGE levels increasing (i.e., normalizing) only in CIE dogs that achieve complete clinical remission, serum sRAGE may be a useful surrogate marker to determine the response to treatment (188). However, further research is needed to confirm the clinical utility of serum sRAGE as a biomarker in canine CIE and determine if sRAGE/RAGE pathways present a potential novel therapeutic target in dogs with chronic gastrointestinal inflammation (198, 199).
Update/perspective on therapeutic strategies
For some CIE subgroups, research over the last decade led to a change in the role of diet and recommended dietary strategies. Demonstrating the existence of food-responsive PLE (FR-PLE) and several effects of diet on the intestinal microbiome and metabolome (e.g., influence on fecal concentrations of secondary bile acids and abundances of Peptaceobacter [Clostridium] hiranonis) emphasizes the importance of selecting an optimal dietary strategy for dogs with CIE.
Strategies for PLE
Disease severity and chronicity dictate the diagnostic and therapeutic approach to dogs suspected of having protein-losing enteropathy (PLE). It requires sequential laboratory tests, diagnostic imaging, and often more invasive diagnostics (Figure 5). Increased fecal α1PI concentrations can detect PLE even before affected dogs develop clinical or clinicopathologic signs (106). Hypoalbuminemia is of prognostic relevance in dogs with PLE (25, 158), and hypovitaminosis D is common (200).
The cornerstone of PLE treatment is lifelong dietary management with a high-protein, low-fat diet ( ≤ 20% fat on a caloric basis or ≤ 20 g fat per 1,000 kcal) (201–203), which can break the cycle of intestinal protein leakage and catabolism. No clinical, clinicopathologic, histologic, or other markers can currently predict food-responsiveness in dogs with PLE (FR-PLE) (40). Serum CRP and fecal calprotectin levels often increase in dogs with PLE as with CIE, particularly those requiring immunomodulatory treatment (108), but both markers add little value to current diagnostic algorithms for PLE.
Response rates with the dietary management of non-infectious, non-neoplastic PLE are high (73–85%) (201–203), and the success of this treatment can be even further improved if multiple small meals are given throughout the day (40). Restoration of a positive protein balance is often achieved or supported with an easily digestible ultra-low fat ( ≤ 10 g fat per 1,000 kcal) commercial diet or a home-made ration (e.g., tilapia and rice or low-fat chicken breast, rice, cottage cheese, and white or sweet potato) (40, 202). Patient preferences and appetite will dictate dietary choices. A board-certified nutritionist should be consulted to verify or design a balanced recipe if the owners elect long-term feeding of a home-made diet. Critically ill dogs may require supplemental or exclusive feeding of either a full elemental diet (e.g., Vivonex1), semi-elemental diet, or polymeric formula (e.g., Ensure2) and the enteral route of feeding can be ensured by a feeding tube (204). Elemental diets can also be given intermittently or, if needed, continuously to dogs with PLE, but this choice can be expensive.
Because there is no clear evidence for an immune-mediated etiology of PLE (40), immunosuppressive treatment should be very carefully considered. Dogs with PLE that show a marked inflammatory infiltrate on intestinal tissue biopsies and lack an adequate clinical response to dietary intervention within 2–4 weeks should be started on anti-inflammatory or immunosuppressive treatment (201, 202). Glucocorticoids (prediso[lo]ne at an initial dose of 1–2 mg/kg PO q12–24h, then attempt to taper by 25% q2–4 weeks) have catabolic, hyperlipidemic, and prothrombotic effects. Thus, other immunosuppressive drugs (e.g., cyclosporine at 5 mg/kg PO q12–24h or chlorambucil at 4–6 mg/m2 PO q24h for 1–3 weeks, then taper) might be better first-line steroid-sparing drug choices. Mycophenolate carries the risk of significant GI adverse effects (24) and is generally not a good choice to treat inflammatory PLE (and CIE in general). Combination immunomodulatory treatment is reserved for dogs with inflammatory PLE that are refractory to dietary and mono-drug therapy (23, 205, 206). Supplemental fiber (e.g., psyllium) or pro-/prebiotics may benefit dogs with PLE, but further research needs to evaluate these treatment options. Absent an infectious etiology, antimicrobial treatment plays no role in canine PLE (21, 22). The somatostatin analog octreotide can be a beneficial rescue treatment (2–4 μg/kg SQ q8–12h) in dogs with inflammatory PLE that are refractory to immunomodulatory treatment, and a study further evaluating its utility in canine PLE is currently underway.
Supportive care should be offered as clinically indicated. Thromboelastography can identify dogs with PLE and a hypercoagulable state (207). Given the increased thrombotic risk, thromboprophylaxis (clopidogrel, low-dose acetylsalicylic acid, or enoxaparin) might be considered as part of the treatment plan in dogs with PLE (208) though evidence for a clear benefit is currently lacking. Dogs with a suboptimal serum vitamin B12 status (< 400 ng/L) should receive supplemental cobalamin (50 μg/kg SC weekly for ≥6 weeks or 50 μg/kg PO q24h for ≥12 weeks) (100). Moderate to marked hypofolatemia might require folic acid supplementation (200–400 μg PO q24h for 7–28 days). Vitamin D supplementation may be considered in patients with marked hypovitaminosis D given its association with negative outcomes (200) but further evidence is needed to determine the benefit (and potential risk) of this strategy. Hypocalcemia and/or hypomagnesemia that cause clinical signs should be corrected (calcium gluconate, magnesium sulfate or magnesium chloride). The benefits and risks of supporting plasma oncotic pressure (i.e., synthetic colloids, canine plasma, human or canine albumin) should be carefully weighed in dogs with severe hypoalbuminemia and marked cavitary effusion.
Probiotics and synbiotics
As microbial imbalances are associated with intestinal inflammation in dogs with CIE, treatments attempting to reduce mucosal inflammation by normalizing dysbiotic states are a rapidly growing research area (Table 3). Probiotics and synbiotics (combinations of a prebiotic and probiotic) are therapies that modify the intestinal microbiota and exert effects on the host immune response to enhance mucosal health. Probiotics are defined as live microorganisms that, when consumed in adequate quantities, confer health benefits on the host (209). Their proposed mechanisms of action include enhancement of mucosal innate and adaptive immune responses (210, 211), production of antimicrobial substances including defensins (210, 212) and organic acids (213), displacement of intestinal pathogens (214), inactivation of bacterial toxins (215), and/or up-regulation of non-specific cellular defenses including heat shock proteins or the inflammasome (216). Very few of these probiotic effects have been demonstrated in companion animals.
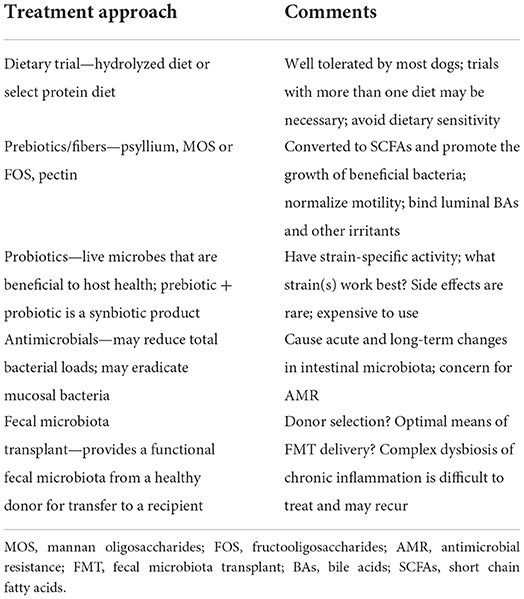
Table 3. General treatment approaches to intestinal dysbiosis in dogs with CIE [data from (14)].
Peer-reviewed publications investigating the use of probiotics or synbiotics in dogs with CIE are sparse (Table 4). The potential benefits of these supplements have been investigated using both clinical trials and ex vivo experiments. Clinical studies have included three randomized controlled trials for dogs with FRE (29, 33, 218), two trials for dogs with idiopathic IBD (28, 220), and one trial for dogs with tylosin-responsive diarrhea (ARE) (122). Additionally, two trials have involved ex vivo stimulation of endoscopic duodenal biopsies from dogs with FRE (219) or CIE (217). Interpretation of the results and comparison between studies is difficult for varied reasons: (1) probiotic effects are strain-specific, and the documented effects will depend on the specific strain used, which was not always specified, (2) different probiotics were used in different studies to treat different forms of CIE, (3) composition of the vehicle used for a specific probiotic could influence probiotic effects, and (4) most studies were substantially underpowered (221, 222).
In separate clinical trials involving dogs with FRE, use of a synbiotic (E. faecium, FOS, and gum Arabic) (29) or a lactic acid bacteria (LAB) mixture of three strains of Lactobacillus spp. (218) failed to improve clinical outcome or proinflammatory intestinal cytokine patterns compared to placebo (elimination diet). This same synbiotic did not significantly alter fecal microbiota richness or diversity in dogs with FRE when administered over 6 weeks (33). In dogs with tylosin-responsive diarrhea, the administration of L. rhamnosus GG did not extend an absence of GI signs once clinical remission was achieved and the antibiotic was discontinued (122).
In canine idiopathic IBD, probiotic therapy has resulted in modulation of host responses and enhancement of the intestinal epithelial barrier integrity. In one trial, probiotic therapy with VSL#3 (Visbiome, an eight-strain LAB mixture) was investigated in comparison to combination drug therapy (28). Dogs treated with the probiotic showed remission accompanied by changes in tolerogenic mucosal responses (increased numbers of FoxP3+ and TGF-β+ regulatory T cells) and increased mucosal expression of the tight junction protein occludin. In a separate trial, probiotic mixture VSL#3 and hydrolyzed diet was administered to dogs with idiopathic IBD and compared to a treatment regimen using hydrolyzed diet and prednisone. While both treatment groups saw remission, probiotic therapy was associated with increased beneficial mucosal bacteria and up-regulated expression of several tight junction proteins suggesting improved intestinal barrier function (32). Finally, the yeast probiotic Saccharomyces boulardii (as part of a synbiotic preparation) improved clinical severity and stool frequency at days 45 and 60 when administered to IBD dogs with and without PLE (220). In the PLE subgroup, all 3 dogs treated with S. boulardii showed improvement in serum albumin vs. 2/3 dogs in the placebo group.
Evidence-based data regarding probiotic use for canine CIE is limited and larger clinical trials are needed. The significant decrease in clinical scores of most dogs with CIE treated with probiotics is best explained by a positive response to dietary intervention. It remains possible that additional health benefits beyond the clinical effects of probiotics might be realized, including systemic immune modulation (223) and potential inhibition of parasite shedding (224).
Fecal microbiota transplantation
The efforts to promote recovery of dysbiosis and remission in dogs with chronic GI disease by administration of FMT have been investigated only to a limited extent. Guidelines regarding optimal donor screening and clinical indications, as well as the method of delivery (oral vs. rectal catheter vs. endoscopy) and frequency of administration of FMT, are currently lacking (22). Studies defining the potential role for FMT in dogs with CIE, including SRE/IRE or idiopathic IBD, involve two reports (including a total of 17 dogs) where the severity of clinical disease improved following FMT in most dogs (225, 226). The limitations in these studies were significant and include small sample size, lack of a standardized method of fecal delivery, failure to include a control group, and the lack of rigorous molecular testing to assess fecal microbial populations.
Emerging concepts and future directions
New concepts that have emerged from recent studies on the microbiome-host-interaction, metabolic pathways, and crosstalk within the mucosal immune system are expected to pave the way to a large spectrum of novel treatment strategies that are tailored to the individual patient. Digital health options and artificial intelligence algorithms will likely also play a role in the management of dogs with CIE. Novel diagnostics have been developed, but the clinical utility of these tests and their utility, when included in a panel of tests, remains to be critically evaluated in the future. A new direction is also the further evaluation of gastrointestinal endocrine pathways and the gut-brain axis in canine CIE.
2D and 3D organoids for regenerative medicine and drug discovery
Accumulating evidence in humans suggests that intestinal epithelial cell (IEC) dysfunction plays a crucial role in the pathogenesis and progression of IBD (227, 228). Different studies have emphasized the role of MH with the regeneration of IECs in IBD remission (228) and that alterations in gene expression and DNA methylation might persist in IECs despite endoscopic and histologic remission, which might influence the course of disease (229, 230). The absence of drugs that directly influence IEC function and the use of immortalized cell lines or animal models that have limited translatability to human IBD have fostered the development of primary IEC cultures called intestinal organoids: enteroids (small intestine) and colonoids (colon). These long-term ex vivo human 3D enteroid/colonoid structures, derived from human adult intestinal stem cells (ISCs), have tremendous potential to recapitulate in vivo physiologic conditions used for fundamental research, precision medicine, regenerative medicine, and drug discovery. Under appropriate culture conditions, enteroids/colonoids produce all cell types normally found within the intestinal epithelium. Dogs represent a unique large animal model that naturally develops CIE, sharing remarkable similarities in etiology, clinical course, histologic lesions, and interventional strategies to human IBD (231). The advantages of the dog in relation to other common animal models of intestinal inflammation include their large body size, longer life span, spontaneous (natural) development of CIE, and they possess a GI tract of comparable size, structure, and function to that of humans.
Canine intestinal organoids derived from healthy dogs and dogs with chronic GI diseases have been recently investigated (232–236). In a seminal study, derivation of canine intestinal organoids was described using tissues obtained endoscopically from the duodenum, ileum, and colon of healthy adult dogs and dogs with idiopathic IBD or intestinal mast cell tumor (232). Using an optimized culture protocol, organoids were extensively investigated for their phenotypic characteristics using H&E histology, immunohistochemistry (IHC), RNA in situ hybridization (RNA-ISH), and transmission electron microscopy (TEM) for comparison to whole jejunal tissue. Moreover, functional assays evaluating cellular metabolic changes (i.e., optical metabolic imaging [OMI]), transmembrane chloride ion conductance via activation of chloride channels in intestinal epithelial cells (cystic fibrosis transmembrane conductance [CFTR] assay), and uptake and transport of parasitic exosomes in response to exposure to the swine nematode, Ascaris suum, were performed. There were no differences in morphology of organoids between healthy dogs and dogs with idiopathic IBD visualized by bright-field microscopy. Ultrastructurally, TEM identified features of differentiated cell types, microvilli, and intercellular structures (i.e., adherens junctions, tight junctions, and desmosomes) between epithelial cells in both enteroids and native jejunum. Characterization of molecular markers and IEC subtypes in enteroids and tissues using RNA-ISH/IHC showed that canine enteroids and intestinal tissue share similar phenotypic expression and cell types (Table 5). Lastly, OMI distinguished and quantitated the cellular metabolism between young, rapidly growing enteroids and aged enteroids. Forskolin-induced swelling of enteroids was observed, indicating functional CFTR chloride channels, like in human colonoids (233). Finally, parasitic exosomes were readily absorbed by enteroids demonstrating their successful passage across the epithelium into the organoid lumen as a measure of absorptive capacity and barrier integrity. The same authors also reported development of a polarized 2D epithelial interface (monolayer) of canine biopsy-derived intestinal organoids to study epithelial-luminal interactions (234, 235). This model system confirmed the polarized expression of the P-glycoprotein efflux pump, offers a standardized system for measuring epithelial barrier function, and allows access to the apical side of the epithelium for investigating drug absorption and metabolism, toxicity testing, and host-microbe crosstalk. Another study has investigated the growth and differentiation of intestinal organoids derived from the canine duodenum, jejunum, and colon (236). Different culture conditions optimized organoid growth in a refined (vs. differentiation) medium whereby long-term organoid expansion and differentiation could be maintained. Future organoid technologies (organoid 2D monolayers in transwell) are currently being modeled and will allow for development of new therapeutic options (drug discovery) and improved understanding of gastrointestinal diseases in dogs and other companion animal species.
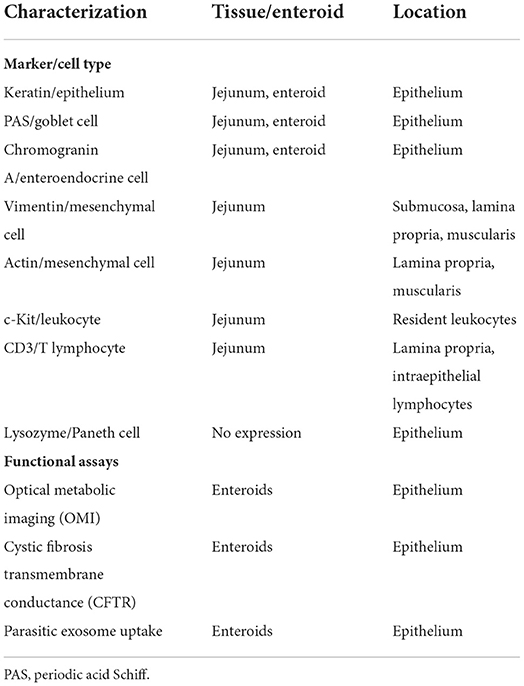
Table 5. Phenotypic/functional characterization of canine organoids and whole jejunum [modified from (237)].
Digital health
Raising the bar on the standard of care in canine CIE will involve introducing novel digital technologies. Artificial intelligence (AI) describes a variety of digital applications where human tasks (e.g., decision trees) are performed computer-based (238). As a particular AI application, machine learning (ML) algorithms can be developed to predict data (e.g., disease, disease subclassification) or outcomes based on previously generated patient data (239). The availability of novel biomarkers in addition to established clinical, endoscopic, and histologic scoring systems lends itself to integrating all these variables (potentially together with the results of other diagnostics such as abdominal ultrasonography) into meaningful ML algorithms (240) for the diagnosis and subclassification of canine CIE or the prediction of individual outcomes. It also offers the potential to establish an ML algorithm for determining the optimal treatment plan and predicting the individual response to treatment and patient outcome (241). Tailoring the diagnostic work-up, accurate ML algorithms may also reduce the overall veterinary healthcare costs for the diagnostic evaluation of the patient and promote an evidence-based medicine approach.
Digital health refers to a conceptional infrastructure that combines different digital technologies (242). The use of digital health structures in human medicine has been shown to promote positive health outcomes via accessible and efficient delivery of healthcare services and remote patient monitoring (242). Because digital health is particularly useful for monitoring and supporting chronically diseased patients (e.g., through mobile-based remote monitoring applications via smartphone), dogs with CIE and their pet owners are expected to benefit tremendously from such services. The enormous potential of these tools (like AI) is likely to unfold in supporting pet owners throughout disease management for improved patient monitoring, owner compliance, and patient outcomes (242). Patient-side biomarker assays (e.g., at-home fecal inflammatory biomarker monitoring), telemedicine and telemanagement (e.g., mentoring) services, and monitoring options (e.g., via a web-based diary function) would be very valuable additional tools in canine CIE management. An online survey at the University of Leipzig, Germany that included a cross-sectional questionnaire Disease tracker app for the management of chronic gastrointestinal disease in dogs and cats—a pet owner's perspective and yielded 2,416 completed votes (85% of respondents were GenZ/GenY < 35 years old, 78% females) revealed those aims to be aligned with most pet owners' expectations. Top-ranking net promoter scores (NPS) in this questionnaire were obtained for the possibility to be reminded of recurring treatments at home (e.g., weekly cobalamin injection), exchange of medical data with the veterinarian, and receiving individualized treatment recommendations and monitoring instructions. The motivation for most pet owners to use this digital health app was a simple and intuitive design, effective integration of this tool into the daily routine, and improved health management of their pet.
Individualized medicine
Diagnostic panels
Several biomarkers evaluated over the last two decades appear clinically useful surrogate tools for guiding the management of dogs with CIE. Some functional biomarkers (e.g., serum cobalamin or fecal α1PI concentrations) are clinically routinely used in dogs with suspected CIE. Genomic biomarkers are currently not readily available but hold great promise for an individualized approach to risk assessment, treatment, and monitoring in canine CIE. Of the inflammatory biomarkers, only serum CRP is currently widely offered, but a human fecal calprotectin assay holds promise for use with specimens from dogs (182, 184), increasing the diagnostic armamentarium in canine gastroenterology.
For the next decade, clinical biomarker research in canine CIE should focus on prospective longitudinal studies to critically evaluate the utility of currently established biomarker assays in dogs with CIE in the routine clinical setting. Incorporating the information gained from several markers into algorithms of “individualized biomarker panels” [as reported for the combination of serum CRP and fecal calprotectin concentration (108)] is expected to further increase the diagnostic accuracy of each individual biomarker and eventually culminate in a holistic individual approach to affected dogs via ‘liquid biopsy' (243).
Therapeutic strategies
Presenting a multifactorial disease with complex pathogenesis renders canine CIE inherently difficult for determining the individual risk of disease development, optimal treatment strategy, and predicted course of the disease. Beyond extending the diagnostic armamentarium, novel pathway-specific treatment options would provide an improved strategy to manage affected dogs. Three-dimensional (3D) models of canine CIE with complete cytodifferentiation from intestinal stem cells have been developed and are currently being refined allowing to reduce the complexity of the intestinal mucosa to modular functioning microphysiological systems (234, 236). These systems allow for individual or cross-pathway analyses (e.g., to further study the immune components in the pathogenesis of CIE), analysis of the interaction with luminal components (particularly with an “inside-out” enteroid model), exploration and validation of novel therapeutic targets (e.g., alternative anti-inflammatory drugs, certain probiotic strains, and even combinations of different treatment options) (237). Beyond implementing the 3R principle in research, biomimetic gut-on-a-chip technology (e.g., OrganoPlate platform3) allows to specifically model aspects of canine CIE that cannot be evaluated in vivo, in existing animal models, or in 2D-cell culture systems (e.g., barrier function, complex imbalance in the mucosal immune response) (244) and to apply gene-editing tools (e.g., CRISPR-Cas) (245). These innovative approaches to a “canine-ized” model are not without challenges and limitations, but a window of opportunity for a future approach to treatment that is fine-tuned to the individual patient (“Dog X's gut-on-a-chip”).
Systemic complications of CIE
Extraintestinal manifestations of IBD in humans include the joints (polyarthritis), skin (erythema nodosum), or eyes (uveitis, episcleritis), but can also involve other organs, such as the liver (cholangitis, cholangiohepatitis), pancreas (pancreatitis), and lung (246–248). In 1 of every 4 patients (25%) the clinical signs associated with the extraintestinal manifestation of the disease can even occur before any gastrointestinal signs develop (248). The occurrence and prevalence of extraintestinal manifestations in canine CIE, as well as their effect on patient management and prognosis, have not been reported and warrant further study.
Discussion and conclusions
Tremendous research efforts over the last decade resulted in a better understanding of the multifactorial disease complex of canine CIE and the differentiation of breed-specific enteropathies. Milestones reached in researching aspects of the immunopathogenesis, microbiome, metabolome, hypovitaminoses B12 and D, and several biomarkers of inflammation are an essential basis for future routes aiming for an individualized medical approach to canine CIE. Refinement of previously established endoscopic and histologic evaluation and scoring criteria has further advanced the standardized assessment and improved the diagnostic algorithms in dogs suspected of CIE. Future investigations in this area need to validate these scoring systems and develop a consensus definition for the assessment of disease remission. More work is also needed to determine the potential benefits of using probiotics, synbiotics, and FMT in dogs with CIE. Subcategorization of food-responsiveness in PLE has changed the approach to affected dogs, and novel dietary strategies and treatment options for canine PLE are to be explored. Future avenues to further evaluate pathogenetic aspects with a focus on drug discovery will benefit from established canine organoid models. AI algorithms are an avenue to improved patient diagnosis and individualized treatment approaches, and digital health tools will likely play a key role in the future of canine CIE management. Important milestones are thus expected in the next decade of canine CIE research.
Author contributions
AEJ and RMH contributed equally to the manuscript design, writing, generation of figures/tables, editing, and proofing. Both authors contributed to the article and approved the submitted version.
Conflict of interest
The authors declare that the research was conducted in the absence of any commercial or financial relationships that could be construed as a potential conflict of interest.
Publisher's note
All claims expressed in this article are solely those of the authors and do not necessarily represent those of their affiliated organizations, or those of the publisher, the editors and the reviewers. Any product that may be evaluated in this article, or claim that may be made by its manufacturer, is not guaranteed or endorsed by the publisher.
Footnotes
1. ^Vivonex® T.E.N, Nestlé Health Science.
2. ^Ensure®, Abbott Laboratories.
3. ^OrganoPlate® platform designed in a 384-well plate format, Mimetas, Leiden, The Netherlands.
References
1. Washabau RJ, Day MJ, Willard MD, Hall EJ, Jergens AE, Mansell J, et al. Endoscopic, biop-sy, and histopathologic guidelines for the evaluation of gastrointestinal inflammation in companion animals. J Vet Intern Med. (2010) 24:10–26. doi: 10.1111/j.1939-1676.2009.0443.x
2. Allenspach K, Culverwell C, Chan D. Long-term outcome in dogs with chronic enteropathies: 203 cases. Vet Rec. (2016) 178:368. doi: 10.1136/vr.103557
3. Dandrieux JR. Inflammatory bowel disease versus chronic enteropathy in dogs: are they one and the same? J Small Anim Pract. (2016) 57:589–99. doi: 10.1111/jsap.12588
4. O'Neill DG, Church DB, McGreevy PD, Thomson PC, Brodbelt DC. Prevalence of disorders recorded in dogs attending primary-care veterinary practices in England. PLoS ONE. (2014) 9:e90501. doi: 10.1371/journal.pone.0090501
5. Kathrani A, Werling D, Allenspach K. Canine breeds at high risk of developing inflammatory bowel disease in the south-eastern UK. Vet Rec. (2011) 169:635. doi: 10.1136/vr.d5380
6. Kawano K, Shimakura H, Nagata N, Masashi Y, Suto A, Suto Y, et al. Prevalence of food-responsive enteropathy among dogs with chronic enteropathy in Japan. J Vet Med Sci. (2016) 78:1377–80. doi: 10.1292/jvms.15-0457
7. Volkmann M, Steiner JM, Fosgate GT, Zentek J, Hartmann S, Kohn B. Chronic diarrhea in dogs - retrospective study in 136 cases. J Vet Intern Med. (2017) 31:1043–55. doi: 10.1111/jvim.14739
8. Dandrieux JRS, Mansfield CS. Chronic enteropathy in canines: prevalence, impact and management strategies. Vet Med (Auckl). (2019) 10:203–14. doi: 10.2147/VMRR.S162774
9. Marks SL, Laflamme DP, McAloose D. Dietary trial using a commercial hypoallergenic diet containing hydrolyzed protein for dogs with inflammatory bowel disease. Vet Ther. (2002) 3:109–18.
10. Mandigers PJJ, Biourge V, van den Ingh TSGAM, Ankringa N, German AJ A. randomized, open-label, positively-controlled field trial of a hydrolyzed protein diet in dogs with chronic small bowel enteropathy. J Vet Intern Med. (2010) 24:1350–7. doi: 10.1111/j.1939-1676.2010.0632.x
11. Marchesi MC, Timpano CC, Busechian S, Pieramati C, Rueca F. The role of diet in managing inflammatory bowel disease affected dogs: a retrospective cohort study on 76 cases. Vet Ital. (2017) 53:297–302. doi: 10.12834/VetIt.566.2700.1
12. Bresciani F, Minamoto Y, Suchodolski JS, Galiazzo G, Vecchiato CG, Pinna C, et al. Effect of an extruded animal protein-free diet on fecal microbiota of dogs with food-responsive enteropathy. J Vet Intern Med. (2018) 32:1903–10. doi: 10.1111/jvim.15227
13. Kalenyak K, Isaiah A, Heilmann RM, Suchodolski JS, Burgener IA. Comparison of the intestinal mucosal microbiota in dogs diagnosed with idiopathic inflammatory bowel disease and dogs with food-responsive diarrhea before and after treatment. FEMS Microbiol Ecol. (2018) 94:173. doi: 10.1093/femsec/fix173
14. Wang S, Martins R, Sullivan MC, Friedman ES, Misic AM, El-Fahmawi A, et al. Diet-induced remission in chronic enteropathy is associated with altered microbial community structure and synthesis of secondary bile acids. Microbiome. (2019) 7:126. doi: 10.1186/s40168-019-0740-4
15. Kathrani A, Hall E A. preliminary study assessing cytokine production following ex vivo stimulation of whole blood with diet in dogs with chronic enteropathy. BMC Vet Res. (2019) 15:185. doi: 10.1186/s12917-019-1940-7
16. Suchodolski JS, Dowd SE, Westermarck E, Steiner JM, Wolcott RD, Spillmann T, et al. The effect of the macrolide antibiotic tylosin on microbial diversity in the canine small intestine as demonstrated by massive parallel 16S rRNA gene sequencing. BMC Microbiol. (2009) 9:210. doi: 10.1186/1471-2180-9-210
17. Manchester AC, Webb CB, Blake AB, Sarwar F, Lidbury JA, Steiner JM, et al. Long-term impact of tylosin on fecal microbiota and fecal bile acids of healthy dogs. J Vet Intern Med. (2019) 33:2605–17. doi: 10.1111/jvim.15635
18. Werner M, Suchodolski JS, Straubinger RK, Wolf G, Steiner JM, Lidbury JA, et al. Effect of amoxicillin-clavulanic acid on clinical scores, intestinal microbiome, and amoxicillin-resistant Esche-richia coli in dogs with uncomplicated acute diarrhea. J Vet Intern Med. (2020) 34:1166–76. doi: 10.1111/jvim.15775
19. Pilla R, Guard BC, Blake AB, Ackermann MA, Webb C, Hill S, et al. Long-term recovery of the fecal microbiome and metabolome of dogs with steroid-responsive enteropathy. Animals. (2021) 11:2498. doi: 10.3390/ani11092498
20. Ziese AL, Suchodolski JS. Impact of changes in gastrointestinal microbiota in canine and feline digestive diseases. Vet Clin North Am Small Anim Pract. (2021) 51:155–69. doi: 10.1016/j.cvsm.2020.09.004
21. Erdmann C, Heilmann RM. Diagnostic and therapeutic approach to chronic inflammatory enteropathies in dogs. Tierarztl Prax Ausg K Kleintiere Heimtiere. (2017) 45:317–27. doi: 10.15654/TPK-170366
22. Cerquetella M, Rossi G, Suchodolski JS, Schmitz SS, Allenspach K, Rodríguez-Franco F, et al. Proposal for rational antibacterial use in the diagnosis and treatment of dogs with chronic diar-rhoea. J Small Anim Pract. (2020) 61:211–5. doi: 10.1111/jsap.13122
23. Dandrieux JR, Noble PJ, Scase TJ, Cripps PJ, German AJ. Comparison of a chlorambucil-prednisolone combi-nation with an azathioprine-prednisolone combination for treatment of chronic enteropathy with con-current protein-losing enteropathy in dogs: 27 cases (2007–2010). J Am Vet Med Assoc. (2013) 242:1705–14. doi: 10.2460/javma.242.12.1705
24. Swann JW, Garden OA, Fellman CL, Glanemann B, Goggs R, LeVine DN, et al. ACVIM consensus statement on the treatment of immune-mediated hemolytic anemia in dogs. J Vet Intern Med. (2019) 33:1141–72. doi: 10.1111/jvim.15463
25. Allenspach K, Wieland B, Gröne A, Gaschen F. Chronic enteropathies in dogs: evaluation of risk factors for negative outcome. J Vet Intern Med. (2007) 21:700–8. doi: 10.1111/j.1939-1676.2007.tb03011.x
26. Craven M, Simpson JW, Ridyard AE, Chandler ML. Canine inflammatory bowel disease: retrospective analysis of diagnosis and outcome in 80 cases (1995-2002). J Small Anim Pract. (2004) 45:336–42. doi: 10.1111/j.1748-5827.2004.tb00245.x
27. Rychlik A, Nieradka R, Kander M, Nowicki M, Wdowiak M, Kołodziejska-Sawerska A. The effectiveness of natural and synthetic immunomodulators in the treatment of inflammatory bowel disease in dogs. Acta Vet Hung. (2013) 61:297–308. doi: 10.1556/avet.2013.015
28. Rossi G, Pengo G, Caldin M, Palumbo Piccionello A, Steiner JM, Cohen ND, et al. Compari-son of microbiological, histological, and immunomodulatory parameters in response to treatment with either combination therapy with prednisone and metronidazole or probiotic VSL#3 strains in dogs with idiopathic inflammatory bowel disease. PLoS ONE. (2014) 9:e94699. doi: 10.1371/journal.pone.0094699
29. Schmitz S, Glanemann B, Garden OA, Brooks H, Chang YM, Werling D, et al. A prospective, randomized, blinded, placebo-controlled pilot study on the effect of Enterococcus faecium on clinical activity and intestinal gene expression in canine food-responsive chronic enteropathy. J Vet Intern Med. (2015) 29:533–43. doi: 10.1111/jvim.12563
30. Schmitz S, Werling D, Allenspach K. Effects of ex-vivo and in-vivo treatment with probiotics on the inflammasome in dogs with chronic enteropathy. PLoS ONE. (2015) 10:e0120779. doi: 10.1371/journal.pone.0120779
31. Segarra S, Martínez-Subiela S, Cerdá-Cuéllar M, Martínez-Puig D, Muñoz-Prieto A, Rodríguez-Franco F, et al. Oral chondroitin sulfate and prebiotics for the treatment of canine inflammatory bowel disease: a randomized, controlled clinical trial. BMC Vet Res. (2016) 12:49. doi: 10.1186/s12917-016-0676-x
32. White R, Atherly T, Guard T, Rossi G, Wang C, Mosher C, et al. Randomized, controlled trial evaluating the effect of multi-strain probiotic on the mucosal microbiota in canine idiopathic inflammatory bowel disease. Gut Microbes. (2017) 8:451–66. doi: 10.1080/19490976.2017.1334754
33. Pilla R, Guard BC, Steiner JM, Gaschen F, Olson E, Werling D, et al. Administration of a synbiotic containing Enterococcus faecium does not significantly alter fecal microbiota richness or diversity in dogs with and without food-responsive chronic enteropathy. Front Vet Sci. (2019) 6:277. doi: 10.3389/fvets.2019.00277
34. Glanemann B, Seo YJ, Priestnall SL, Garden OA, Kilburn L, Rossoni-Serao M, et al. Clinical efficacy of prebiotics and glycosaminoglycans versus placebo in dogs with food responsive enteropathy receiving a hydrolyzed diet: a pilot study. PLoS ONE. (2021) 16:e0250681. doi: 10.1371/journal.pone.0250681
35. Pereira GQ, Gomes LA, Santos IS, Alfieri AF, Weese JS, Costa MC. Fecal microbiota trans-plantation in puppies with canine parvovirus infection. J Vet Intern Med. (2018) 32:707–11. doi: 10.1111/jvim.15072
36. Berlanda M, Innocente G, Simionati B, Di Camillo B, Facchin S, Giron MC, et al. Faecal microbiome transplantation as a solution to chronic enteropathies in dogs: a case study of beneficial microbial evolution. Animals. (2021) 11:1433. doi: 10.3390/ani11051433
37. Diniz AN, da Costa Ferreira de Souza A, Neopomuceno AC, Marcelino SAC, Pierezan F, Lobato FCF, et al. Fecal microbiota transplantation via colonoscopy in a dog with Clostridioides (Clostridium) difficile infection. Microbiology Cienc Rural. (2021) 51:e20200783. doi: 10.1590/0103-8478cr20200783
38. Pérez-Merino EM, Usón-Casaús JM, Zaragoza-Bayle C, Duque-Carrasco J, Mariñas-Pardo L, Hermida-Prieto M, et al. Safety and efficacy of allogeneic adipose tissue-derived mesenchymal stem cells for treatment of dogs with inflammatory bowel disease: clinical and laboratory outcomes. Vet J. (2015) 206:385–90. doi: 10.1016/j.tvjl.2015.08.003
39. Dossin O, Lavoué R. Protein-losing enteropathies in dogs. Vet Clin North Am Small Anim Pract. (2011) 41:399–418. doi: 10.1016/j.cvsm.2011.02.002
40. Craven MD, Washabau RJ. Comparative pathophysiology and management of protein-losing enteropathy. J Vet Intern Med. (2019) 33:383–402. doi: 10.1111/jvim.15406
41. Watson VE, Hobday MM, Durham AC. Focal intestinal lipogranulomatous lymphangitis in 6 dogs (2008-2011). J Vet Intern Med. (2014) 28:48–51. doi: 10.1111/jvim.12248
42. Lecoindre A, Lecoindre P, Cadoré JL, Chevallier M, Guerret S, Derré G, et al. Focal intestinal lipogranulomatous lymphangitis in 10 dogs. J Small Anim Pract. (2016) 57:465–71. doi: 10.1111/jsap.12522
43. Jergens AE. Inflammatory bowel disease—current perspectives. Vet Clin North Am Small Anim Pract. (1999) 29:501–21, vii. doi: 10.1016/S0195-5616(99)50032-6
44. Simpson KW, Jergens AE. Pitfalls and progress in the diagnosis and management of canine inflammatory bowel disease. Vet Clin North Am Small Anim Pract. (2011) 41:381–98. doi: 10.1016/j.cvsm.2011.02.003
45. McGovern DPB, Kugathasan S, Cho JH. Genetics of inflammatory bowel diseases. Gastroenterology. (2015) 149:1163–76. doi: 10.1053/j.gastro.2015.08.001
46. Xavier RJ, Podolsky DK. Unravelling the pathogenesis of inflammatory bowel disease. Nature. (2007) 448:427–34. doi: 10.1038/nature06005
47. McMahon LA, House AK, Catchpole B, Elson-Riggins J, Riddle A, Smith K, et al. Expres-sion of Toll-like receptor 2 in duodenal biopsies from dogs with inflammatory bowel disease is associated with severity of disease. Vet Immunol Immunopathol. (2010) 135:158–63. doi: 10.1016/j.vetimm.2009.11.012
48. Burgener IA, König A, Allenspach K, Sauter SN, Boisclair J, Doherr MG, et al. Upregulation of toll-like receptors in chronic enteropathies in dogs. J Vet Intern Med. (2008) 22:553–60. doi: 10.1111/j.1939-1676.2008.0093.x
49. Allenspach K, House A, Smith K, McNeill FM, Hendricks A, Elson-Riggins J, et al. Evalua-tion of mucosal bacteria and histopathology, clinical disease activity and expression of Toll-like re-ceptors in German shepherd dogs with chronic enteropathies. Vet Microbiol. (2010) 146:326–35. doi: 10.1016/j.vetmic.2010.05.025
50. Kathrani A, House A, Catchpole B, Murphy A, German A, Werling D, et al. Polymorphisms in the TLR4 and TLR5 gene are significantly associated with inflammatory bowel disease in German shepherd dogs. PLoS ONE. (2010) 5:e15740. doi: 10.1371/journal.pone.0015740
51. Donnini EK, Walugembe M, Rothschild MF, Jergens AE, Allenspach K. An initial genome-wide investigation of protein-losing enteropathy in Gordon setters: exploratory observations. Can J Vet Res. (2021) 85:51–60.
52. Baumgart M, Dogan B, Rishniw M, Weitzman G, Bosworth B, Yantiss R, et al. Culture independent analysis of ileal mucosa reveals a selective increase in invasive Escherichia coli of novel phylogeny relative to depletion of Clostridiales in Crohn's disease involving the ileum. ISME J. (2007) 1:403–18. doi: 10.1038/ismej.2007.52
53. Mansfield CS, James FW, Craven M, Davies DR, O'Hara AJ, Nicholls PK, et al. Remission of histiocytic ulcerative colitis in Boxer dogs correlates with eradication of invasive intramucosal Escherichia coli. J Vet Intern Med. (2009) 23:964–9. doi: 10.1111/j.1939-1676.2009.0363.x
54. Dogan B, Zhang S, Kalla SE, Dogan EI, Guo C, Ang CR, et al. Molecular and phenotypic characterization of Escherichia coli associated with granulomatous colitis of Boxer dogs. Antibiotics. (2020) 9:540. doi: 10.3390/antibiotics9090540
55. Kuja-Halkola R, Lebwohl B, Halfvarson J, Wijmenga C, Magnusson PK, Ludvigsson JF. Heritability of non-HLA genetics in coeliac disease: a population-based study in 107 000 twins. Gut. (2016) 65:1793–8. doi: 10.1136/gutjnl-2016-311713
56. Sollid LM, Markussen G, Ek J, Gjerde H, Vartdal F, Thorsby E. Evidence for a primary asso-ciation of celiac disease to a particular HLA-DQ alpha/beta heterodimer. J Exp Med. (1989) 169:345–50. doi: 10.1084/jem.169.1.345
57. Vaden SL, Hammerberg B, Davenport DJ, Orton SM, Trogdon MM, Melgarejo LT, et al. Food hypersensitivity reactions in Soft Coated Wheaten Terriers with protein-losing enteropathy or protein-losing nephropathy or both: gastroscopic food sensitivity testing, dietary provocation, and fecal immunoglobulin E. J Vet Intern Med. (2000) 14:60–7. doi: 10.1111/j.1939-1676.2000.tb01501.x
58. Littman MP, Dambach DM, Vaden SL, Giger U. Familial protein-losing enteropathy and protein-losing nephropathy in Soft Coated Wheaten Terriers: 222 cases (1983-1997). J Vet Intern Med. (2000) 14:68–80. doi: 10.1111/j.1939-1676.2000.tb01502.x
59. Anand A, Russell AS, Tsuyuki R, Fedorak R. Perinuclear antineutrophil cytoplasmic autoantibodies and anti-Saccharomyces cerevisiae antibodies as serological markers are not specific in the identification of Crohn's disease and ulcerative colitis. Can J Gastroenterol. (2008) 22:33–6. doi: 10.1155/2008/974540
60. Allenspach K, Lomas B, Wieland B, Harris T, Pressler B, Mancho C, et al. Evaluation of perinuclear anti-neutrophilic cytoplasmic autoantibodies as an early marker of protein-losing enteropathy and protein-losing nephropathy in Soft Coated Wheaten Terriers. Am J Vet Res. (2008) 69:1301–4. doi: 10.2460/ajvr.69.10.1301
61. Luckschander N, Allenspach K, Hall JA, Seibold F, Gröne A, Doherr MG, et al. Perinuclear antineutrophilic cytoplasmic antibody and response to treatment in diarrheic dogs with food responsive disease or inflammatory bowel disease. J Vet Intern Med. (2006) 20:221–7. doi: 10.1111/j.1939-1676.2006.tb02849.x
62. Mancho C, Sainz Á, García-Sancho M, Villaescusa A, Rodríguez-Franco F. Evaluation of perinuclear antineutrophilic cytoplasmic antibodies in sera from dogs with inflammatory bowel disease or intestinal lymphoma. Am J Vet Res. (2011) 72:1333–7. doi: 10.2460/ajvr.72.10.1333
63. Grützner N, Bishop MA, Suchodolski JS, Steiner JM. Association study of cobalamin deficiency in the Chinese Shar Pei. J Hered. (2009) 101:211–7. doi: 10.1093/jhered/esp100
64. Garden OA, Pidduck H, Lakhani KH, Walker D, Wood JL, Batt RM. Inheritance of gluten-sensitive enteropathy in Irish Setters. Am J Vet Res. (2000) 61:462–8. doi: 10.2460/ajvr.2000.61.462
65. Gensollen T, Iyer SS, Kasper DL, Blumberg RS. How colonization by microbiota in early life shapes the immune system. Science. (2016) 352:539–44. doi: 10.1126/science.aad9378
66. Nabhani ZA, Dulauroy S, Marques R, Cousu C, Bounny SA, Déjardin F, et al. A weaning reaction to microbiota is required for resistance to immunopathologies in the adult. Immunity. (2019) 50:1276–88. doi: 10.1016/j.immuni.2019.02.014
67. Scheer S, Medina TS, Murison A, Taves MD, Antignano F, Chenery A, et al. Early-life anti-biotic treatment enhances the pathogenicity of CD4(+) T cells during intestinal inflammation. J Leu-koc Biol. (2017) 101:893–900. doi: 10.1189/jlb.3MA0716-334RR
68. Li X, Sundquist J, Hemminki K, Sundquist K. Risk of inflammatory bowel disease in first- and second-generation immigrants in Sweden: a nationwide follow-up study. Inflamm Bowel Dis. (2011) 17:1784–91. doi: 10.1002/ibd.21535
69. Kilian E, Suchodolski JS, Hartmann K, Mueller RS, Wess G, Unterer S. Long-term effects of canine parvovirus infection in dogs. PLoS ONE. (2018) 13:e0192198. doi: 10.1371/journal.pone.0192198
70. Skotnitzki E, Suchodolski JS, Busch K, Werner M, Zablotski Y, Ballhausen BD, et al. Frequency of signs of chronic gastrointestinal disease in dogs after an acute episode acute hemorrhagic diarrhea. J Vet Intern Med. (2022) 36:59–65. doi: 10.1111/jvim.16312
71. Kaser A, Zeissig S, Blumberg RS. Genes and environment: how will our concepts on the pathophysiology of IBD develop in the future? Dig Dis. (2010) 28:395–405. doi: 10.1159/000320393
72. Cader MZ, Kaser A. Recent advances in inflammatory bowel disease: mucosal immune cells in intestinal inflammation. Gut. (2013) 62:1653–64. doi: 10.1136/gutjnl-2012-303955
73. Kathrani A, Holder A, Catchpole B, Alvarez L, Simpson K, Werling D, et al. TLR5 risk-associated haplotype for canine inflammatory bowel disease confers hyper-responsiveness to flagellin. PLoS ONE. (2012) 7:e30117. doi: 10.1371/journal.pone.0030117
74. Okanishi H, Hayashi K, Sakamoto Y, Sano T, Maruyama H, Kagawa Y, et al. NOD2 mRNA expression and NFkappaB activation in dogs with lymphocytic plasmacytic colitis. J Vet Intern Med. (2013) 27:439–44. doi: 10.1111/jvim.12082
75. Kathrani A, Lee H, White C, Catchpole B, Murphy A, German A, et al. Association between nucleotide oligomerisation domain two (Nod2) gene polymorphisms and canine inflammatory bowel disease. Vet Immunol Immunopathol. (2014) 161:32–41. doi: 10.1016/j.vetimm.2014.06.003
76. German AJ, Hall EJ, Day MJ. Immune cell populations within the duodenal mucosa of dogs with enteropathies. J Vet Intern Med. (2001) 15:14–25. doi: 10.1111/j.1939-1676.2001.tb02292.x
77. Jergens AE, Gamet Y, Moore FM, Niyo Y, Tsao C, Smith B. Colonic lymphocyte and plasma cell populations in dogs with lymphocytic-plasmacytic colitis. Am J Vet Res. (1999) 60:515–20.
78. Olivero D, Turba M, Gentilini F. Reduced diversity of immunoglobulin and T-cell receptor gene rearrangements in chronic inflammatory gastrointestinal diseases in dogs. Vet Immunol Immunopathol. (2011) 144:337–45. doi: 10.1016/j.vetimm.2011.08.011
79. German AJ, Helps CR, Hall EJ, Day MJ. Cytokine mRNA expression in mucosal biopsies from German shepherd dogs with small intestinal enteropathies. Dig Dis Sci. (2000) 45:7–17. doi: 10.1023/A:1005436721798
80. Peters IR, Helps CR, Calvert EL, Hall WJ, Day MJ. Cytokine mRNA quantification in duo-denal mucosa from dogs with chronic enteropathies by real-time reverse transcriptase polymerase chain reaction. J Vet Intern Med. (2005) 19:644–53. doi: 10.1111/j.1939-1676.2005.tb02742.x
81. Jergens AE, Sonea IM, O'Connor AM, Kauffman LK, Grozdanic SD, Ackermann MR, et al. Intestinal cytokine mRNA expression in canine inflammatory bowel disease: a meta-analysis with critical appraisal. Comp Med. (2009) 59:153–62.
82. Heilmann RM, Suchodolski JS. Is inflammatory bowel disease in dogs and cats associated with a Th1 or Th2 polarization? Vet Immunol Immunopathol. (2015) 168:131–4. doi: 10.1016/j.vetimm.2015.10.008
83. Schmitz S, Garden OA, Werling D, Allenspach K. Gene expression of selected signature cytokines of T cell subsets in duodenal tissues of dogs with and without inflammatory bowel disease. Vet Immunol Immunopathol. (2012) 146:87–91. doi: 10.1016/j.vetimm.2012.01.013
84. Neurath MF, Finotto S, Glimcher LH. The role of Th1/Th2 polarization in mucosal immunity. Nat Med. (2002) 8:567–73. doi: 10.1038/nm0602-567
85. Gunawardana SC, Jergens AE, Ahrens FA, Niyo Y. Colonic nitrite and immunoglobulin G concentrations in dogs with inflammatory bowel disease. J Am Vet Med Assoc. (1997) 211:318–21.
86. Nolte A, Junginger J, Baum B, Hewicker-Trautwein M. Heterogeneity of macrophages in canine histiocytic ulcerative colitis. Innate Immun. (2017) 23:228–39. doi: 10.1177/1753425916686170
87. Heilmann RM, Steiner JS. Clinical utility of currently available biomarkers in inflammatory enteropathies of dogs. J Vet Intern Med. (2018) 32:1495–508. doi: 10.1111/jvim.15247
88. Wilke VL, Nettleton D, Wymore MJ, Gallup JM, Demirkale CY, Ackermann MR, et al. Gene expression in intestinal mucosal biopsy specimens obtained from dogs with chronic enteropathy. Am J Vet Res. (2012) 73:1219–29. doi: 10.2460/ajvr.73.8.1219
89. Lu LF, Liston A. MicroRNA in the immune system, microRNA as an immune system. Immu-nology. (2009) 127:291–8. doi: 10.1111/j.1365-2567.2009.03092.x
90. Takagi T, Naito Y, Mizushima K, Hirata I, Yagi N, Tomatsuri N, et al. Increased expression of microRNA in the inflamed colonic mucosa of patients with active ulcerative colitis. J Gastroen-terol Hepatol. (2010) 25 Suppl 1:S129–133. doi: 10.1111/j.1440-1746.2009.06216.x
91. Fasseu M, Tréton X, Guichard C, Pedruzzi E, Cazals-Hatem D, Richard C, et al. Identifica-tion of restricted subsets of mature microRNA abnormally expressed in inactive colonic mucosa of patients with inflammatory bowel disease. PLoS ONE. (2010) 5:e13160. doi: 10.1371/journal.pone.0013160
92. Wu F, Zikusoka M, Trindade A, Dassopoulos T, Harris ML, Bayless TM, et al. MicroRNAs are differentially expressed in ulcerative colitis and alter expression of macrophage inflammatory peptide-2 alpha. Gastroenterology. (2008) 135:1624–35. doi: 10.1053/j.gastro.2008.07.068
93. Kalla R, Ventham NT, Kennedy NA, Quintana JF, Nimmo ER, Buck AH, et al. MicroRNAs: new players in IBD. Gut. (2015) 64:504–13. doi: 10.1136/gutjnl-2014-307891
94. Konstantinidis AO, Pardali D, Adamama-Moraitou KK, Gazouli M, Dovas CJ, Legaki E, et al. Colonic mucosal and serum expression of microRNAs in canine large intestinal inflammatory bowel disease. BMC Vet Res. (2020) 16:69. doi: 10.1186/s12917-020-02287-6
95. Sørensen SH, Proud FJ, Rutgers HC, Markwell P, Adam A, Batt RM, et al. blood test for intesti-nal permeability and function: a new tool for the diagnosis of chronic intestinal disease in dogs. Clin Chim Acta. (1997) 264:103–15. doi: 10.1016/S0009-8981(97)00077-6
96. Kobayashi S, Ohno K, Uetsuka K, Nakashima K, Setoguchi A, Fujino Y, et al. Measurement of intestinal mucosal permeability in dogs with lymphocytic-plasmacytic enteritis. J Vet Med Sci. (2007) 69:745–9. doi: 10.1292/jvms.69.745
97. Allenspach K, Steiner JM, Shah BN, Berghoff N, Ruaux CG, Williams DA, et al. Evaluation of gastrointestinal permeability and mucosal absorptive capacity in dogs with chronic enteropathy. Am J Vet Res. (2006) 67:479–83. doi: 10.2460/ajvr.67.3.479
98. Klenner S, Frias R, Coenen M, Failing K, Hewicker-Trautwein M, Ternes W, et al. Estimation of intestinal permeability in healthy dogs using the contrast medium iohexol. Vet Clin Pathol. (2009) 38:353–60. doi: 10.1111/j.1939-165X.2009.00136.x
99. Schoultz I, Keita ÅV. The intestinal barrier and current techniques for the assessment of gut permeability. Cells. (2020) 9:1909. doi: 10.3390/cells9081909
100. Kather S, Grützner N, Kook PH, Dengler F, Heilmann RM. Review of cobalamin status and disorders of cobalamin metabolism in dogs. J Vet Intern Med. (2020) 34:13–28. doi: 10.1111/jvim.15638
101. Berghoff N, Suchodolski JS, Steiner JM. Association between serum cobalamin and methylmalonic acid concentrations in dogs. Vet J. (2012) 191:306–11. doi: 10.1016/j.tvjl.2011.03.005
102. Grützner N, Heilmann RM, Stupka KC, Rangachari VR, Weber K, Holzenburg A, et al. Serum homocysteine and methylmalonic acid concentrations in Chinese Shar-Pei dogs with cobalamin deficiency. Vet J. (2013) 197:420–6. doi: 10.1016/j.tvjl.2013.02.002
103. Berghoff N, Parnell NK, Hill SL, Suchodolski JS, Steiner JM. Serum cobalamin and methylmalonic acid concentrations in dogs with chronic gastrointestinal disease. Am J Vet Res. (2013) 74:84–9. doi: 10.2460/ajvr.74.1.84
104. Fedosov SN, Brito A, Miller JW, Green R, Allen LH. Combined indicator of vitamin B12 status: modification for missing biomarkers and folate status and recommendations for revised cut-points. Clin Chem Lab Med. (2015) 53:1215–25. doi: 10.1515/cclm-2014-0818
105. Batt RM, Horadagoda NU. Gastric and pancreatic intrinsic factor-mediated absorption of cobalamin in the dog. Am J Physiol. (1989) 257:344–9. doi: 10.1152/ajpgi.1989.257.3.G344
106. Heilmann RM, Parnell NK, Grützner N, Mansell J, Berghoff N, Schellenberg S, et al. Serum and fecal canine alpha1-proteinase inhibitor concentrations reflect the severity of intestinal crypt ab-scesses and/or lacteal dilation in dogs. Vet J. (2016) 207:131–9. doi: 10.1016/j.tvjl.2015.10.042
107. Heilmann RM, Volkmann M, Otoni CC, Grützner N, Kohn B, Jergens AE, et al. Fecal S100A12 concentration predicts a lack of response to treatment in dogs affected with chronic enteropathy. Vet J. (2016) 215:96–100. doi: 10.1016/j.tvjl.2016.03.001
108. Heilmann RM, Berghoff N, Mansell J, Grützner N, Parnell NK, Gurtner C, et al. Association of fecal calprotectin concentrations with disease severity, response to treatment, and other biomarkers in dogs with chronic inflammatory enteropathies. J Vet Intern Med. (2018) 32:679–92. doi: 10.1111/jvim.15065
109. Kather S, Kacza J, Pfannkuche H, Böttcher D, Steiner JM, Gäbel G, et al. Upregulation of intestinal cobalamin receptor expression in hypocobalaminemic dogs with idiopathic inflammatory bowel disease. Gastroenterology. (2022) 162:S459 (abstract). doi: 10.1016/S0016-5085(22)61091-6
110. Suchodolski JS. Diagnosis and interpretation of intestinal dysbiosis in dogs and cats. Vet J. (2016) 215:30–7. doi: 10.1016/j.tvjl.2016.04.011
111. Toresson L, Steiner JM, Spodsberg E, Olmedal G, Suchodolski JS, Lidbury JA, et al. Effects of oral versus parenteral cobalamin supplementation on methylmalonic acid and homocysteine concentrations in dogs with chronic enteropathies and low cobalamin concentrations. Vet J. (2019) 243:8–14. doi: 10.1016/j.tvjl.2018.11.004
112. Toresson L, Steiner JM, Suchodolski JS, Spillmann T. Oral cobalamin supplementation in dogs with chronic enteropathies and hypocobalaminemia. J Vet Intern Med. (2016) 30:101–7. doi: 10.1111/jvim.13797
113. Toresson L, Steiner JM, Razdan P, Spodsberg E, Olmedal G, Suchodolski JS, et al. Comparison of efficacy of oral and parenteral cobalamin supplementation in normalising low cobalamin concentrations in dogs: a randomised controlled study. Vet J. (2018) 232:27–32. doi: 10.1016/j.tvjl.2017.12.010
114. Kook PH, Hersberger M. Daily oral cyanocobalamin supplementation in Beagles with heredi-tary cobalamin malabsorption (Imerslund-Gräsbeck syndrome) maintains normal clinical and cellular cobalamin status. J Vet Intern Med. (2019) 33:751–7. doi: 10.1111/jvim.15380
115. Kook PH, Reusch CE, Hersberger M. Prospective long-term evaluation of parenteral hydrox-ocobalamin supplementation in juvenile beagles with selective intestinal cobalamin malabsorption (Imerslund-Gräsbeck syndrome). J Vet Intern Med. (2018) 32:1033–40. doi: 10.1111/jvim.15090
116. Xenoulis PG, Palculict B, Allenspach K, Steiner JM, van House AM, Suchodolski JS. Molec-ular-phylogenetic characterization of microbial communities imbalances in the small intestine of dogs with inflammatory bowel disease. FEMS Microbiol Ecol. (2008) 66:579–89. doi: 10.1111/j.1574-6941.2008.00556.x
117. Suchodolski JS, Dowd SE, Wilke V, Steiner JM, Jergens AE. 16S rRNA gene pyrosequenc-ing reveals bacterial dysbiosis in the duodenum of dogs with idiopathic inflammatory bowel disease. PLoS One. (2012) 7:e39333. doi: 10.1371/journal.pone.0039333
118. Honneffer JB, Minamoto Y, Suchodolski JS. Microbiota alterations in acute and chronic gastrointestinal inflammation of cats and dogs. World J Gastroenterol. (2014) 20:16489–97. doi: 10.3748/wjg.v20.i44.16489
119. Giaretta PR, Suchodolski JS, Jergens AE, Steiner JM, Lidbury JA, Cook AK, et al. Bacterial biogeography of the colon in dogs with chronic inflammatory enteropathy. Vet Pathol. (2020) 57:258–65. doi: 10.1177/0300985819891259
120. Cassmann E, White R, Atherly T, Wang C, Sun Y, Khoda S, et al. Alterations of the ileal and colonic mucosal microbiota in canine chronic enteropathies. PLoS ONE. (2016) 11:e0147321. doi: 10.1371/journal.pone.0147321
121. Simpson KW, Dogan B, Rishniw M, Goldstein RE, Klaessig S, McDonough PL, et al. Adherent and invasive Escherichia coli is associated with granulomatous colitis in boxer dogs. Infect Immun. (2006) 74:4778–92. doi: 10.1128/IAI.00067-06
122. Westermarck E, Skrzypczak T, Harmoinen J, Steiner JM, Ruaux CG, Williams DA, et al. Tylosin-responsive chronic diarrhea in dogs. J Vet Intern Med. (2005) 19:177–86. doi: 10.1111/j.1939-1676.2005.tb02679.x
123. Costa M, Weese JS. Methods and basic concepts for microbiota assessment. Vet J. (2019) 249:10–5. doi: 10.1016/j.tvjl.2019.05.005
124. AlShawaqfeh MK, Wajid B, Minamoto Y, Markel M, Lidbury JA, Steiner JM, et al. A dysbiosis index to assess microbial changes in fecal samples of dogs with chronic inflammatory en-teropathy. FEMS Microbiol Ecol. (2017) 93:fix136. doi: 10.1093/femsec/fix136
125. Guard BC, Honneffer JB, Jergens AE, Jonika MM, Toresson L, Lawrence YA, et al. Longi-tudinal assessment of microbial dysbiosis, fecal unconjugated bile acid concentrations, and disease activity in dogs with steroid-responsive chronic inflammatory enteropathy. J Vet Intern Med. (2019) 33:1295–305. doi: 10.1111/jvim.15493
126. Giaretta PR, Rech RR, Guard BC, Blake AB, Blick AK, Steiner JM, et al. Comparison of intestinal expression of the apical sodium-dependent bile acid transporter between dogs with and without chronic inflammatory enteropathy. J Vet Intern Med. (2018) 32:1918–26. doi: 10.1111/jvim.15332
127. Minamoto Y, Minamoto T, Isaiah A, Sattasathuchana P, Buono A, Rangachari VR, et al. Fecal short-chain fatty acid concentrations and dysbiosis in dogs with chronic enteropathy. J Vet Intern Med. (2019) 33:1608–18. doi: 10.1111/jvim.15520
128. Minamoto Y, Otoni CC, Steelman SM, Buyukleblebici O, Steiner JM, Jergens AE, et al. Alteration of the fecal microbiota and serum metabolite profiles in dogs with idiopathic inflammatory bowel disease. Gut Microbes. (2015) 6:33–47. doi: 10.1080/19490976.2014.997612
129. Rivière A, Selak M, Lantin D, Leroy F, de Vuyst F. Bifidobacteria and butyrate-producing colon bacteria: importance and strategies for their stimulation in the human gut. Front Microbiol. (2016) 7:979. doi: 10.3389/fmicb.2016.00979
130. Peng L, Li ZR, Green RS, Holzman IR, Lin J. Butyrate enhances the intestinal barrier by facilitating tight junction assembly via activation of AMP-activated protein kinase in Caco-2 cell monolayers. J Nutr. (2009) 139:1619–25. doi: 10.3945/jn.109.104638
131. Arpaia N, Campbell C, Fan X, Dikiy S, van der Veeken J. deRoos P, et al. Metabolites produced by commensal bacteria promote peripheral regulatory T-cell generation. Nature. (2013) 504:451–5. doi: 10.1038/nature12726
132. Suchodolski JS, Markel ME, Garcia-Mazcorro JF, Unterer S, Heilmann RM, Dowd SE, et al. The fecal microbiome in dogs with acute diarrhea and idiopathic inflammatory bowel disease. PLoS ONE. (2012) 7:e51907. doi: 10.1371/journal.pone.0051907
133. Kathrani A, Allenspach K, Fascetti AJ, Larsen JA, Hall EJ. Alterations in serum amino acid concentrations in dogs with protein-losing enteropathy. J Vet Intern Med. (2018) 32:1026–32. doi: 10.1111/jvim.15116
134. Whitfield-Cargile CM, Cohen ND, Chapkin RS, Weeks BR, Davidson LA, Goldsby JS, et al. The microbiota-derived metabolite indole decreases mucosal inflammation and injury in a murine model of NSAID enteropathy. Gut Microbes. (2016) 7:246–61. doi: 10.1080/19490976.2016.1156827
135. Walters RJ, Pattni SS. Managing bile acid diarrhoea. Therap Adv Gastroenterol. (2010) 3:349–57. doi: 10.1177/1756283X10377126
136. Toresson L, Steiner JM, Suchodolski JS. Cholestyramine treatment in two dogs with presumptive bile acid diarrhoea: a case report. Canine Med Genet. (2021) 8:1. doi: 10.1186/s40575-021-00099-x
137. Jergens AE, Schreiner CA, Frank DE, Niyo Y, Ahrens FE, Eckersall PD, et al. A scoring index for disease activity in canine inflammatory bowel disease. J Vet Intern Med. (2003) 17:291–7. doi: 10.1111/j.1939-1676.2003.tb02450.x
139. Jergens AE, Willard MD, Allenspach K. Maximizing the diagnostic utility of endoscopic biopsy in dogs and cats with gastrointestinal disease. Vet J. (2016) 214:50–60. doi: 10.1016/j.tvjl.2016.04.008
140. Lane J, Price J, Moore A, Dandrieux JRS, Clifford C, Curran K, et al. Low-grade gastrointestinal lymphoma in dogs: 20 cases (2010 to 2016). J Small Anim Pract. (2018) 59:147–53. doi: 10.1111/jsap.12769
141. Procoli F, Motskula PF, Keyte SV, Priestnall S, Allenspach K. Comparison of histopathologic findings in duodenal and ileal endoscopic biopsies in dogs with chronic small intestinal enteropathies. J Vet Intern Med. (2013) 27:268–74. doi: 10.1111/jvim.12041
142. Willard MD, Mansell J, Fosgate GT, Gualtieri M, Olivero D, Lecoindre P, et al. Effect of sample quality on the sensitivity of endoscopic biopsy for detecting gastric and duodenal lesions in dogs and cats. J Vet Intern Med. (2008) 22:1084–9. doi: 10.1111/j.1939-1676.2008.0149.x
143. Willard MD, Lovering SL, Cohen ND, Weeks BR. Quality of tissue specimens obtained endoscopically from the duodenum of dogs and cats. J Am Vet Med Assoc. (2001) 219:474–9. doi: 10.2460/javma.2001.219.474
144. Goutal-Landry CM, Mansell J, Ryan KA, Gaschen FP. Effect of endoscopic forceps on quality of duodenal mucosal biopsy in healthy dogs. J Vet Intern Med. (2013) 27:456–61. doi: 10.1111/jvim.12085
145. Day MJ, Bilzer T, Mansell J, Wilcock B, Hall EJ, Jergens A, et al. Histopathological standards for the diagnosis of gastrointestinal inflammation in endoscopic biopsy samples from the dog and cat: a report from the World Small Animal Veterinary Association Gastrointestinal Standardization Group. J Comp Pathol. (2008) 138 Suppl 1:S1–43. doi: 10.1016/j.jcpa.2008.01.001
146. Walsh A, Palmer R, Travis S. Mucosal healing as a target of therapy for colonic inflammatory bowel disease and methods to score disease activity. Gastrointest Endosc Clin N Am. (2014) 24:367–78. doi: 10.1016/j.giec.2014.03.005
147. Rutgeerts R, Vermeire S, Van Assche G. Mucosal healing in inflammatory bowel disease: impossible ideal or therapeutic target? Gut. (2007) 56:453–5. doi: 10.1136/gut.2005.088732
148. Neurath MF, Travis SP. Mucosal healing in inflammatory bowel diseases: a systematic review. Gut. (2012) 61:1619–35. doi: 10.1136/gutjnl-2012-302830
149. Garcia-Sancho M, Rodriguez-Franco F, Sainz A, Mancho C, Rodriguez A. Evaluation of clinical, macroscopic, and histopathologic response to treatment in nonhypoproteinemic dogs with lymphocytic-plasmacytic enteritis. J Vet Intern Med. (2007) 21:11–7. doi: 10.1111/j.1939-1676.2007.tb02922.x
150. Slovak JE, Wang C, Sun Y, Otoni C, Morrison J, Deitz K, et al. Development and validation of an endoscopic activity score for canine inflammatory bowel disease. Vet J. (2015) 203:290–5. doi: 10.1016/j.tvjl.2014.12.030
151. Jergens AE, Moore FM, Haynes JS, Miles KG. Idiopathic inflammatory bowel disease in dogs and cats: 84 cases (1987-1990). J Am Vet Med Assoc. (1992) 201:1603–8.
152. Jergens AE, Moore FM, Kaiser MS, Haynes JS, Kinyon JM. Morphometric evaluation of immunoglobulin A-containing and immunoglobulin G-containing cells and T cells in duodenal mucosa from healthy dogs and from dogs with inflammatory bowel disease or non-specific gastroenteri-tis. Am J Vet Res. (1996) 57:697–704.
153. Peterson PB, Willard MD. Protein-losing enteropathies. Vet Clin North Am Small Anim Pract. (2003) 33:1061–82. doi: 10.1016/S0195-5616(03)00055-X
154. Roth L, Walton AM, Leib MS, Burrows CF A. grading system for lymphocytic plasmacytic colitis in dogs. J Vet Diagn Invest. (1990) 2:257–62. doi: 10.1177/104063879000200401
155. Willard MD, Moore GD, Denton BD, Day MJ, Mansell J, Bilzer T, et al. Effect of tissue pro-cessing on assessment of endoscopic intestinal biopsies in dogs and cats. J Vet Intern Med. (2010) 24:84–9. doi: 10.1111/j.1939-1676.2009.0432.x
156. Willard MD, Jergens AE, Duncan RB, Leib MS, McCracken MD, DeNovo RC, et al. Interobserver variation among histopathologic evaluations of intestinal tissues from dogs and cats. J Am Vet Med Assoc. (2002) 220:1177–82. doi: 10.2460/javma.2002.220.1177
157. Jergens AE, Evans RB, Ackermann M, Hostetter J, Willard M, Mansell J, et al. Design of a simplified histopathologic model for gastrointestinal inflammation in dogs. Vet Pathol. (2014) 51:946–50. doi: 10.1177/0300985813511123
158. Wennogle SA, Priestnall SL, Webb CB. Histopathologic characteristics of intestinal biopsy samples from dogs with chronic inflammatory enteropathy with and without hypoalbuminemia. J Vet Intern Med. (2017) 31:371–6. doi: 10.1111/jvim.14669
159. Allenspach K, Mochel JP, Du Y, Priestnall SL, Moore F, Slayter M, et al. Correlating gastro-intestinal histopathologic changes to clinical disease activity in dogs with idiopathic inflammatory bowel disease. Vet Pathol. (2019) 56:435–43. doi: 10.1177/0300985818813090
160. McCann TM, Ridyard AE, Else RW, Simpson JW. Evaluation of disease activity markers in dogs with idiopathic inflammatory bowel disease. J Small Anim Pract. (2007) 48:620–5. doi: 10.1111/j.1748-5827.2007.00335.x
161. Schreiner NM, Gaschen F, Gröne A, Sauter SN, Allenspach K. Clinical signs, histology, and CD3-positive cells before and after treatment of dogs with chronic enteropathies. J Vet Intern Med. (2008) 22:1079–83. doi: 10.1111/j.1939-1676.2008.0153.x
162. Casamian-Sorrosal D, Willard MD, Murray JK, Hall EJ, Taylor SS, Day MJ. Comparison of histopathologic findings in biopsies from the duodenum and ileum of dogs with enteropathy. J Vet Intern Med. (2010) 24:80–3. doi: 10.1111/j.1939-1676.2009.0427.x
163. Walker D, Knuchel-Takano A, McCutchan A, Chang YM, Downes C, Miller S, et al. A com-prehensive pathological survey of duodenal biopsies from dogs with diet-responsive chronic enterop-athy. J Vet Intern Med. (2013) 27:862–74. doi: 10.1111/jvim.12093
164. Rhodes B, Fürnrohr BG, Vyse TJ. C-reactive protein in rheumatology: biology and genetics. Nat Rev Rheumatol. (2011) 7:282–9. doi: 10.1038/nrrheum.2011.37
165. Lewis DH, Chan DL, Pinheiro D, Armitage-Chan E, Garden OA. The immunopathology of sepsis: pathogen recognition, systemic inflammation, the compensatory anti-inflammatory response, and regulatory T cells. J Vet Intern Med. (2012) 26:457–82. doi: 10.1111/j.1939-1676.2012.00905.x
166. Berghoff N, Suchodolski JS, Steiner JM. Assessment of stability and determination of a reference range for canine C-reactive protein in serum. J Vet Intern Med. (2006) 20:791 (abstract). doi: 10.1111/j.1939-1676.2006.tb02919.x
167. Parra MD, Tuomola M, Cabezas-Herrera J, Cerón JJ. Analytical and clinical validation of a time-resolved immunofluorometric assay (TR-IFMA) for canine C-reactive protein in serum. Vet Res Commun. (2006) 30:113–26. doi: 10.1007/s11259-006-3201-z
168. Plickert HD, Einspanier R, Arndt G, Brunnberg L, Kohn B. Evaluation of a point-of-care test for canine C-reactive protein. Vet Clin Pathol. (2011) 40:384–8. doi: 10.1111/j.1939-165X.2011.00339.x
169. Klenner S, Bauer N, Moritz A. Evaluation of three automated human immunoturbidimetric assays for the detection of C-reactive protein in dogs. J Vet Diagn Invest. (2010) 22:544–52. doi: 10.1177/104063871002200408
170. Hindenberg S, Klenner-Gastreich S, Kneier N, Zielinsky S, Gommeren K, Bauer N, et al. Evaluation of a species-specific C-reactive protein assay for the dog on the ABX Pentra 400 clinical chemistry analyzer. BMC Vet Res. (2017) 13:146. doi: 10.1186/s12917-017-1065-9
171. Pineiro M, Pato R, Soler L, Pena R, García N, et al. A new automated turbidimetric immunoassay for the measurement of canine C-reactive protein. Vet Clin Pathol. (2018) 47:130–7. doi: 10.1111/vcp.12576
172. Covin MA, Gomez RR, Suchodolski JS, Steiner JM, Lidbury JA. Analytical validation of a point-of-care test and an automated immunoturbidimetric assay for the measurement of canine C-reactive protein in serum. Can J Vet Res. (2021) 85:285–92.
173. Covin MA, Steiner JM. Measurement and clinical applications of C-reactive protein in gastro-intestinal diseases of dogs. Vet Clin Pathol. (2022) 50 Suppl 1:29–36. doi: 10.1111/vcp.13100
174. Hillström A, Hagman R, Söder J, Häggström J, Ljungvall I, Kjelgaard-Hansen M. Validation and application of a canine-specific automated high-sensitivity C-reactive protein assay. J Vet Diagn Invest. (2015) 27:182–90. doi: 10.1177/1040638715575751
175. Carney PC, Ruaux CG, Suchodolski JS, Steiner JM. Biological variability of C-reactive protein and specific pancreatic lipase immunoreactivity (Spec cPL) in apparently healthy dogs. J Vet Intern Med. (2011) 25:825–30. doi: 10.1111/j.1939-1676.2011.0729.x
176. Jergens AE, Crandell J, Morrison JA, Deitz K, Pressel M, Ackermann M, et al. Comparison of oral prednisone and prednisone combined with metronidazole for induction therapy of canine inflammatory bowel disease: a randomized-controlled trial. J Vet Intern Med. (2010) 24:269–77. doi: 10.1111/j.1939-1676.2009.0447.x
177. Otoni CC, Heilmann RM, García-Sancho M, Sainz A, Ackermann MR, Suchodolski JS, et al. Serologic and fecal markers to predict response to induction therapy in dogs with idiopathic inflammatory bowel disease. J Vet Intern Med. (2018) 32:999–1008. doi: 10.1111/jvim.15123
178. Grellet A, Heilmann RM, Lecoindre P, Feugier A, Day MJ, Peeters D, et al. Fecal calprotectin concentrations in adult dogs with chronic diarrhea. Am J Vet Res. (2013) 74:706–11. doi: 10.2460/ajvr.74.5.706
179. Heilmann RM, Nestler J, Schwarz J, Grützner N, Ambrus A, Seeger J, et al. Mucosal expres-sion of S100A12 (calgranulin C) and S100A8/A9 (calprotectin) and correlation with serum and fecal concentrations in dogs with chronic inflammatory enteropathy. Vet Immunol Immunopathol. (2019) 211:64–74. doi: 10.1016/j.vetimm.2019.04.003
180. Vogl T, Tenbrock K, Ludwig S, Leukert N, Ehrhardt C, van Zoelen MAD, et al. Mrp8 and Mrp14 are endogenous activators of Toll-like receptor 4, promoting lethal, endotoxin-induced shock. Nat Med. (2007) 13:1042–9. doi: 10.1038/nm1638
181. Heilmann RM, Suchodolski JS, Steiner JM. Development and analytic validation of a radio-immunoassay for the quantification of canine calprotectin in serum and feces from dogs. Am J Vet Res. (2008) 69:845–53. doi: 10.2460/ajvr.69.7.845
182. Truar K, Nestler J, Schwarz J, Grützner N, Gabris C, Kock K, et al. Feasibility of measuring fecal calprotectin concentrations in dogs and cats by the fCAL® turbo immunoassay. J Vet Intern Med. (2018) 32:580 (abstract). doi: 10.1111/jvim.14858
183. Heilmann RM, Guard MM, Steiner JM, Suchodolski JS, Unterer S. Fecal inflammatory biomarkers and microbial changes in dogs with acute hemorrhagic diarrhea syndrome (AHDS). J Vet Emerg Crit Care. (2017) 27:586–9. doi: 10.1111/vec.12636
184. Enderle LL, Köller G, Heilmann RM. Verification of the fCAL turbo immunoturbidimetric assay for measurement of the fecal calprotectin concentration in dogs and cats. J Vet Diagn Invest. (2022) 34:813–24. doi: 10.1177/10406387221114031
185. Heilmann RM, Grellet A, Allenspach K, Lecoindre P, Day MJ, Priestnall SL, et al. Association between fecal S100A12 concentration and histologic, endoscopic, and clinical disease severity in dogs with idiopathic inflammatory bowel disease. Vet Immunol Immunopathol. (2014) 158:156–66. doi: 10.1016/j.vetimm.2014.01.006
186. Gebhardt C, Riehl A, Durchdewald M, Németh J, Fürstenberger G, Müller-Decker K, et al. RAGE signaling sustains inflammation and promotes tumor development. J Exp Med. (2008) 205:275–85. doi: 10.1084/jem.20070679
187. Heilmann RM, Cranford SM, Ambrus A, Grützner N, Schellenberg S, Ruaux CG, et al. Methodological and preanalytical validation of an enzyme-linked immunosorbent assay for the measurement of canine S100A12. Vet Clin Pathol. (2016) 45:135–47. doi: 10.1111/vcp.12320
188. Heilmann RM, Otoni CC, Jergens AE, Grützner N, Suchodolski JS, Steiner JM. Systemic levels of the anti-inflammatory decoy receptor sRAGE (soluble receptor for advanced glycation end products) are decreased in dogs with inflammatory bowel disease. Vet Immunol Immunopathol. (2014) 161:184–92. doi: 10.1016/j.vetimm.2014.08.003
189. Anfinsen KP, Berghoff N, Priestnall SL, Suchodolski JS, Steiner JM, Allenspach K. Urinary and faecal N-methylhistamine concentrations do not serve as markers for mast cell activation or clinical disease activity in dogs with chronic enteropathies. Acta Vet Scand. (2014) 56:90. doi: 10.1186/s13028-014-0090-y
190. Berghoff N, Hill S, Parnell NK, Mansell J, Suchodolski JS, Steiner JM. Fecal and urinary N-methylhistamine concentrations in dogs with chronic gastrointestinal disease. Vet J. (2014) 201:289–94. doi: 10.1016/j.tvjl.2014.05.016
191. Vaden SL, Hammerberg B, Orton SM, Stone EA. Mast cell degranulation responses in Soft-Coated Wheaten Terriers with protein-losing enteropathy and/or nephropathy. J Vet Intern Med. (2000) 14:348 (abstract). doi: 10.1111/j.1939-1676.2000.tb01174.x
192. Berghoff N, Suchodolski JS, Steiner JM. Fecal N-methylhistamine concentrations in Norwegian Lundehunds with gastrointestinal disease. J Vet Intern Med. (2008) 22:748 (abstract). doi: 10.1111/j.1939-1676.2008.0103.x
193. Bastan I, Robinson NA, Ge XN, Rendahl AK, Rao SP, Washabau RJ, et al. Assessment of eosinophil peroxidase as a potential diagnostic and prognostic marker in dogs with inflammatory bowel disease. Am J Vet Res. (2017) 78:36–41. doi: 10.2460/ajvr.78.1.36
194. Sattasathuchana P, Grützner N, Lopes R, Guard BC, Suchodolski JS, Steiner JM. Stability of 3-bromotyrosine in serum and serum 3-bromotyrosine concentrations in dogs with gastrointestinal diseases. BMC Vet Res. (2015) 11:1–7. doi: 10.1186/s12917-015-0321-0
195. Sattasathuchana P, Allenspach K, Lopes R, Suchodolski JS, Steiner JM. Evaluation of serum 3-bromotyrosine concentrations in dogs with steroid-responsive diarrhea and food-responsive diarrhea. J Vet Intern Med. (2017) 31:1056–61. doi: 10.1111/jvim.14742
196. Heilmann RM, Allenspach K. Pattern recognition receptors: signaling pathways and dysregu-lation in canine chronic enteropathies - brief review. J Vet Diagn Invest. (2017) 29:781–7. doi: 10.1177/1040638717728545
197. Cabrera-García AI, Protschka M, Alber G, Kather S, Dengler F, Müller U, et al. Dysregulation of gastrointestinal RAGE (receptor for advanced glycation end products) expression in dogs with chronic inflammatory enteropathy. Vet Immunol Immunopathol. (2021) 234:110216. doi: 10.1016/j.vetimm.2021.110216
198. Cabrera-García AI, Suchodolski JS, Steiner JM, Heilmann RM. Association between serum soluble receptor for advanced glycation end-products (RAGE) deficiency and severity of clinicopathologic evidence of canine chronic inflammatory enteropathy. J Vet Diagn Invest. (2020) 32:664–74. doi: 10.1177/1040638720943584
199. Body-Malapel M, Djouina M, Waxin C, Langlois A, Gower-Rousseau C, Zerbib P, et al. The RAGE signaling pathway is involved in intestinal inflammation and represents a promising therapeutic target for inflammatory bowel diseases. Mucosal Immunol. (2019) 12:468–78. doi: 10.1038/s41385-018-0119-z
200. Allenspach K, Rizzo J, Jergens AE, Chang YM. Hypovitaminosis D is associated with negative outcome in dogs with protein losing enteropathy: a retrospective study of 43 cases. BMC Vet Res. (2017) 13:96. doi: 10.1186/s12917-017-1022-7
201. Okanishi H, Yoshioka R, Kagawa Y, Watari T. The clinical efficacy of dietary fat restriction in treatment of dogs with intestinal lymphangiectasia. J Vet Intern Med. (2014) 28:809–17. doi: 10.1111/jvim.12327
202. Rudinsky AJ, Howard JP, Bishop MA, Sherding RG, Parker VJ, Gilor C. Dietary management of presumptive protein-losing enteropathy in Yorkshire terriers. J Small Anim Pract. (2017) 58:103–8. doi: 10.1111/jsap.12625
203. Nagata N, Ohta H, Yokoyama N, Teoh YB, Nisa K, Sasaki N, et al. Clinical characteristics of dogs with food-responsive protein-losing enteropathy. J Vet Intern Med. (2020) 34:659–68. doi: 10.1111/jvim.15720
204. Economu L, Chang YM, Priestnall SL, Kathrani A. The effect of assisted enteral feeding on treatment outcome in dogs with inflammatory protein-losing enteropathy. J Vet Intern Med. (2021) 35:1297–305. doi: 10.1111/jvim.16125
205. Salavati Schmitz S, Gow A, Bommer N, Morrison L, Mellanby R. Diagnostic features, treatment, and outcome of dogs with inflammatory protein-losing enteropathy. J Vet Intern Med. (2019) 33:2005–13. doi: 10.1111/jvim.15571
206. Kathrani A. Dietary and nutritional approaches to the management of chronic enteropathy in dogs and cats. Vet Clin North Am Small Anim Pract. (2021) 51:123–36. doi: 10.1016/j.cvsm.2020.09.005
207. Goodwin LV, Goggs R, Chan DL, Allenspach K. Hypercoagulability in dogs with protein-losing enteropathy. J Vet Intern Med. (2011) 25:273–7. doi: 10.1111/j.1939-1676.2011.0683.x
208. Jacinto AML, Ridyard AE, Aroch I, Watson PJ, Morrison LR, Chandler ML, et al. Thromboembolism in dogs with protein-losing enteropathy with non-neoplastic chronic small intestinal disease. J Am Anim Hosp Assoc. (2017) 53:185–92. doi: 10.5326/JAAHA-MS-6328
209. FAO/WHO. Evaluation of Health and Nutritional Properties of Powder Milk and Live Lactic Acid Bacteria. Geneva: WHO (2001). p. 1–4.
210. Oelschlaeger TA. Mechanisms of probiotic actions—a review. Int J Med Microbiol. (2010) 300:57–62. doi: 10.1016/j.ijmm.2009.08.005
211. Pagnini C, Saeed R, Bamias G, Arseneau KO, Pizarro TT, Cominelli F. Probiotics promote gut health through stimulation of epithelial innate immunity. Proc Natl Acad Sci USA. (2010) 107:454–9. doi: 10.1073/pnas.0910307107
212. Thomas CM, Versalovic J. Probiotics-host communication: modulation of signaling pathways in the intestine. Gut Microbes. (2010) 1:148–1463. doi: 10.4161/gmic.1.3.11712
213. Saarela M, Mogensen G, Fondén R, Mättö J, Mattila-Sandholm T. Probiotic bacteria: safety, functional and technological properties. J Biotechnol. (2000) 84:197–215. doi: 10.1016/S0168-1656(00)00375-8
214. Lee YK, Puong KY, Ouwehand AC, Salminen S. Displacement of bacterial pathogens from mucus and Caco-2 cell surface by lactobacilli. J Med Microbiol. (2003) 52:925–30. doi: 10.1099/jmm.0.05009-0
215. Jones SE, Versalovic J. Probiotic Lactobacillus reuteri biofilms produce antimicrobial and anti-inflammatory factors. BMC Microbiol. (2009) 9:35. doi: 10.1186/1471-2180-9-35
216. Soo I, Madsen KL, Tejpar Q, Sydora BC, Sherbaniuk R, Cinque B, et al. VSL#3 probiotic upregulates intestinal mucosal alkaline sphingomyelinase and reduces inflammation. Can J Gastroenterol. (2008) 22:237–42. doi: 10.1155/2008/520383
217. Sauter SN, Allenspach K, Gaschen F, Gröne A, Ontsouka EC, Blum JW. Cytokine expression in an ex vivo culture system of duodenal samples from dogs with chronic enteropathies: modulation by probiotic bacteria. Domest Anim Endocrinol. (2005) 29:605–22. doi: 10.1016/j.domaniend.2005.04.006
218. Sauter SN, Benyacoub J, Allenspach K, Gaschen F, Ontsouka E, Reuteler G, et al. Effects of probiotic bacteria in dogs with food responsive diarrhoea treated with an elimination diet. J Anim Physiol Anim Nutr. (2006) 90:269–77. doi: 10.1111/j.1439-0396.2005.00595.x
219. Schmitz S, Henrich M, Neiger R, Werling D, Allenspach K. Stimulation of duodenal biopsies and whole blood from dogs with food-responsive chronic enteropathy and healthy dogs with Toll-like receptor ligands and probiotic Enterococcus faecium. Scand J Immunol. (2014) 80:85–94. doi: 10.1111/sji.12186
220. D'Angelo S, Fracassi F, Bresciani F, Galuppi R, Diana A, Linta N, et al. Effect of Saccharomyces boulardii in dog with chronic enteropathies: double-blinded, placebo-controlled study. Vet Rec. (2018) 182:258. doi: 10.1136/vr.104241
221. Schmitz SS. Value of probiotics in canine and feline gastroenterology. Vet Clin North Am Small Anim Pract. (2021) 51:171–217. doi: 10.1016/j.cvsm.2020.09.011
222. Jensen AP, Bjørnvad CR. Clinical effect of probiotics in prevention or treatment of gastrointestinal disease in dogs: a systematic review. J Vet Intern Med. (2019) 33:1849–64. doi: 10.1111/jvim.15554
223. Benyacoub J, Czarnecki-Maulden GL, Cavadini C, Sauthier T, Anderson RE, Schiffrin EJ, et al. Supplementation of food with Enterococcus faecium (SF68) stimulates immune functions in young dogs. J Nutr. (2003) 133:1158–62. doi: 10.1093/jn/133.4.1158
224. Simpson KW, Rishniw M, Bellosa M, Liotta J, Lucio A, Baumgart M, et al. Influence of Enterococcus faecium SF68 probiotic on giardiasis in dogs. J Vet Intern Med. (2009) 23:476–81. doi: 10.1111/j.1939-1676.2009.0283.x
225. Bottero E, Benvenuti E, Ruggiero P. Fecal microbiota transplantation (FMT) in 16 dogs with idiopatic IBD. Veterinaria. (2017) 31:31–45.
226. Niina A, Kibe R, Suzuki R, Yuchi Y, Teshima T, et al. Improvement in clinical symptoms and fecal microbiome after fecal microbiota transplantation in a dog with inflammatory bowel disease. Vet Med (Auckl). (2019) 10:197–201. doi: 10.2147/VMRR.S230862
227. Okamoto R, Watanabe M. Role of epithelial cells in the pathogenesis and treatment of inflammatory bowel disease. J Gastroenterol. (2016) 51:11–21. doi: 10.1007/s00535-015-1098-4
228. Dotti I, Salas A. Potential use of human stem cell-derived intestinal organoids to study inflammatory bowel diseases. Inflamm Bowel Dis. (2018) 24:2501–9. doi: 10.1093/ibd/izy275
229. Planell N, Lozano JJ, Mora-Buch R, Masamunt MC, Jimeno M, Ordás I, et al. Transcriptional analysis of the intestinal mucosa of patients with ulcerative colitis in remission reveals lasting epithelial cell alterations. Gut. (2013) 62:967–76. doi: 10.1136/gutjnl-2012-303333
230. Cooke J, Zhang H, Greger L, Silva AL, Massey D, Dawson C, et al. Mucosal genome-wide methylation changes in inflammatory bowel disease. Inflamm Bowel Dis. (2012) 18:2128–37. doi: 10.1002/ibd.22942
231. Jergens AE, Parvinroo S, Kopper J, Wannemuehler MJ. Rules of engagement: epithelial-microbe interactions and inflammatory bowel disease. Front Med. (2021) 8:669913. doi: 10.3389/fmed.2021.669913
232. Sahoo DK, Borcherding DC, Chandra L, Jergens AE, Atherly T, Bourgois-Mochel A, et al. Differential transcriptomic profiles following stimulation with lipopolysaccharide in intestinal organoids from dogs with inflammatory bowel disease and intestinal mast cell tumor. Cancers. (2022) 14:3525. doi: 10.3390/cancers14143525
233. Dekkers JF, Wiegerinck CL, de Jonge HR, Bronsveld I, Janssens HM, de Winter-de Groot KM, et al. A functional CFTR assay using primary cystic fibrosis intestinal organoids. Nat Med. (2013) 19:939–45. doi: 10.1038/nm.3201
234. Chandra L, Borcherding DC, Kingsbury D, Atherly T, Ambrosini YM, Bourgois-Mochel A, et al. Derivation of adult canine intestinal organoids for translational research in gastroenterology. BMC Biol. (2019) 17:33. doi: 10.1186/s12915-019-0652-6
235. Ambrosini YM, Park Y, Jergens AE, Shin W, Min S, Atherly T, et al. Recapitulation of the accessible interface of biopsy-derived canine intestinal organoids to study epithelial-luminal interactions. PLoS ONE. (2020) 15:e0231423. doi: 10.1371/journal.pone.0231423
236. Kramer N, Pratscher B, Meneses AMC, Tschulenk W, Walter I, Swoboda A, et al. Generation of differentiating and long-living intestinal organoids reflecting the cellular diversity of canine intestine. Cells. (2020) 9:822. doi: 10.3390/cells9040822
237. Kopper JJ, Iennarella-Servantez C, Jergens AE, Sahoo DK, Guillot E, Bourgois-Mochel A, et al. Harnessing the biology of canine intestinal organoids to heighten understanding of inflammatory bowel disease pathogenesis and accelerate drug discovery: a one health approach. Front Toxicol. (2021) 3:773953. doi: 10.3389/ftox.2021.773953
238. Christou CD, Tsoulfas G. Challenges and opportunities in the application or artificial intelligence in gastroenterology and hepatology. World J Gastroenterol. (2021) 27:6191–223. doi: 10.3748/wjg.v27.i37.6191
239. Kraszewski S, Szczurek W, Szymczak J, Reguła M, Neubauer K. Machine learning prediction model for inflammatory bowel disease based on laboratory markers. Working model in a discovery cohort study. J Clin Med. (2021) 10:4745. doi: 10.3390/jcm10204745
240. Gubatan J, Levitte S, Patel A, Balabanis T, Wei MT, Sinha SR. Artificial intelligence applications in inflammatory bowel disease: emerging technologies and future directions. World J Gastroenterol. (2021) 27:1920–35. doi: 10.3748/wjg.v27.i17.1920
241. Con D, van Langenberg DR, Vasudevan A. Deep learning vs. conventional learning algorithms for clinical prediction in Crohn's disease: a proof-of-concept study. World J Gastroenterol. (2021) 27:6476–88. doi: 10.3748/wjg.v27.i38.6476
242. Majidova K, Handfield J, Kafi K, Martin RD, Kubinski R. Role of digital health and artificial intelligence in inflammatory bowel disease: a scoping review. Genes. (2021) 12:1465. doi: 10.3390/genes12101465
243. Kinugasa H, Hiraoka S, Nouso K, Yamamoto S, Hirai M, Terasawa H, et al. Liquid biopsy for patients with IBD-associated neoplasia. BMC Cancer. (2020) 20:1188. doi: 10.1186/s12885-020-07699-z
244. Beaurivage C, Naumovska E, Chang YX, Elstak ED, Nicolas A, Wouters H, et al. Development of a gut-on-a-chip model for high throughput disease modeling and drug discovery. Int J Mol Sci. (2019) 20:5661. doi: 10.3390/ijms20225661
245. van Dongen JE, Berendsen JTW, Steenbergen RDM, Wolthuis RMF, Eijkel JCT, Segerink LI. Point-of-care CRISPR/Cas nucleic acid detection: recent advances, challenges and opportunities. Biosens Bioelectron. (2020) 166:112445. doi: 10.1016/j.bios.2020.112445
246. Calvo P, Pablo L. Managing IBD outside the gut: ocular manifestations. Dig Dis. (2013) 31:229–32. doi: 10.1159/000353375
247. Sharma SM, Jackson D. Uveitis and spondyloarthropathies. Best Pract Res Clin Rheumatol. (2017) 31:846–62. doi: 10.1016/j.berh.2018.08.002
Keywords: biomarker, disease modeling, drug discovery, individualized approach, innovation
Citation: Jergens AE and Heilmann RM (2022) Canine chronic enteropathy—Current state-of-the-art and emerging concepts. Front. Vet. Sci. 9:923013. doi: 10.3389/fvets.2022.923013
Received: 18 April 2022; Accepted: 22 August 2022;
Published: 21 September 2022.
Edited by:
Matthew Irick Jackson, Hill's Pet Nutrition, Inc., United StatesReviewed by:
Frederic Philippe Gaschen, Louisiana State University, United StatesSilke Salavati, University of Edinburgh, United Kingdom
Copyright © 2022 Jergens and Heilmann. This is an open-access article distributed under the terms of the Creative Commons Attribution License (CC BY). The use, distribution or reproduction in other forums is permitted, provided the original author(s) and the copyright owner(s) are credited and that the original publication in this journal is cited, in accordance with accepted academic practice. No use, distribution or reproduction is permitted which does not comply with these terms.
*Correspondence: Albert E. Jergens, YWplcmdlbnNAaWFzdGF0ZS5lZHU=
†These authors have contributed equally to this work