- College of Coastal Agricultural Sciences, Guangdong Ocean University, Zhanjiang, China
An infinite cell line is one of the most favored experimental tools and plays an irreplaceable role in cell-based biological research. Primary cells from normal animal tissues undergo a limited number of divisions and subcultures in vitro before they enter senescence and die. On the contrary, an infinite cell line is a population of non-senescent cells that could proliferate indefinitely in vitro under the stimulation of external factors such as physicochemical stimulation, virus infection, or transfer of immortality genes. Cell immortalization is the basis for establishing an infinite cell line, and previous studies have found that methods to obtain immortalized cells mainly included physical and chemical stimulations, heterologous expression of viral oncogenes, increased telomerase activity, and spontaneous formation. However, some immortalized cells do not necessarily proliferate permanently even though they can extend their lifespan compared with primary cells. An infinite cell line not only avoids the complicated process of collecting primary cell, it also provides a convenient and reliable tool for studying scientific problems in biology. At present, how to establish a stable infinite cell line to maximize the proliferation of cells while maintaining the normal function of cells is a hot issue in the biological community. This review briefly introduces the methods of cell immortalization, discusses the related progress of establishing immortalized cell lines in livestock and poultry, and compares the characteristics of several methods, hoping to provide some ideas for generating new immortalized cell lines.
Introduction
As the basic structural and functional unit of life activities, cells are widely used as experimental tools in various studies, especially in the fields of molecular biology and biomedical research. Currently, there are two types of animal cells commonly used in laboratories: primary cells and infinite cell lines (1). Primary cells refer to cells that are directly collected from organism tissues and cultured in a simulated in vivo environment (2). Most of them are collected from tissues of experimental animals such as mice and rabbits, and chicken embryos (3, 4). Take myoblast cells as an example to briefly describe the general process for collecting adherent cells. First, collect fresh muscle tissue samples from a slaughterhouse and transport them to a cell culture laboratory under sterile conditions (5). Small-sized experimental animals such as chicken embryos, whose muscle tissue can also be separated directly on the laboratory sterile bench (6). Then, wash the muscle tissue with 70% ethanol or 1 × phosphate buffered saline (PBS) containing 1% penicillin-streptomycin to remove surface dirt, and cut it into small pieces. Obtain the suspension containing myoblasts after mechanical dispersion and enzymatic digestion (commonly used are 0.1% collagenase and 0.25% trypsin solutions) (7, 8). Finally, remove tissue and cell debris in the suspension using a 40-μM cell strainer and perform low-speed centrifugation to collect primary myoblast cells (9–12). It is worth mentioning that the collected primary cells are suspended in a complete medium supplemented with an appropriate amount of fetal bovine serum (FBS) and cultured in monolayers at 37°C in a humidified atmosphere containing 5% carbon dioxide (simulating the environment in which cells survive and replicate in vivo) (13, 14).
The collected primary cells are almost identical to their source cells in morphology and characteristics. However, their ability to rapidly proliferate and differentiate in vitro is limited (15, 16). Even primary tumor-derived cells cannot continue to proliferate after a certain number of passages in vitro (17). In contrast, an infinite cell line is a population of non-senescent cells that escape cell cycle restriction and can proliferate indefinitely in vitro (18). In other words, achieving cell immortality is the basis for establishing infinite cell lines. Cell immortalization is one of the hotspots in biological research. It refers to the process of making cells cultured in vitro escape the senescence period of cell proliferation under the influence of external factors to obtain the ability of infinite division (1). Previous research has revealed that telomeres and telomerase activity were closely related to cell immortalization (19). Telomeres, special DNA-protein complexes presenting at the ends of eukaryotic chromosomes, are comprised of simple repetitive and highly conserved DNA sequences with guanine (G) base-rich and related proteins. They are involved in DNA replication and play important roles in maintaining a stable and complete replication of chromosomes (20). Along with proliferation and division of cells from normal animal tissues (nerve tissue, muscle tissue, etc.), telomeres get shortened, and cell proliferation will be inhibited to enter the senescence period. At this time, if the activity of telomerase is extremely low, the cell will reach the crisis stage and finally enter apoptosis under gene regulation. On the contrary, immortalized cells or tumor cells can maintain constant telomere length because of the activation of telomerase (21). In addition, the expression of tumor suppressor gene p53 or Rb is also an important regulatory point in the process of cell immortalization (22, 23).
Cell lines bypass ethical issues associated with the use of animal and human tissues, providing an endless supply of a homogeneous cellular material that is cost-effective and very convenient to use. In addition, a cell line avoids collection of animal tissues from slaughterhouses, reducing the risk of endogenous contamination (24). Previous studies have suggested that many established immortalized cell lines could maintain the shape, characteristics, and functions of primary cells, and replace primary cells to provide convenient and reliable experimental materials for basic scientific research studies, clinical treatments, bioengineering pharmaceuticals, and vaccine research and development (25–27). However, some immortalized cells do not proliferate permanently despite their extended lifespan compared with primary cells (28). After multiple population doublings (PDs), cells will gradually senesce and loss important genetic characteristics (15, 18). Therefore, we summarized the established livestock and poultry cell lines and compared different methods to generate a stable infinite cell line hoping to find a better way to maximize the PDs of cells while maintaining their normal functions.
Methods for obtaining immortalized cells
Currently, the methods for obtaining the immortalization of human and animal cells are mainly divided into four categories (1, 29): (i) destroying the regulation of proto-oncogenes or tumor suppressor genes on the cell cycle through physical and chemical stimulation, which was a technique often utilized in early research (Figure 1), (ii) inducing the heterologous expression of viral oncogenes to help cells escape the cell cycle control (Figure 2), (iii) stimulating the activity of cellular telomerase to overcome the replicative senescence caused by telomere shortening and realize the infinite proliferation of cells in vitro (Figure 3), and (iv) spontaneous formation.
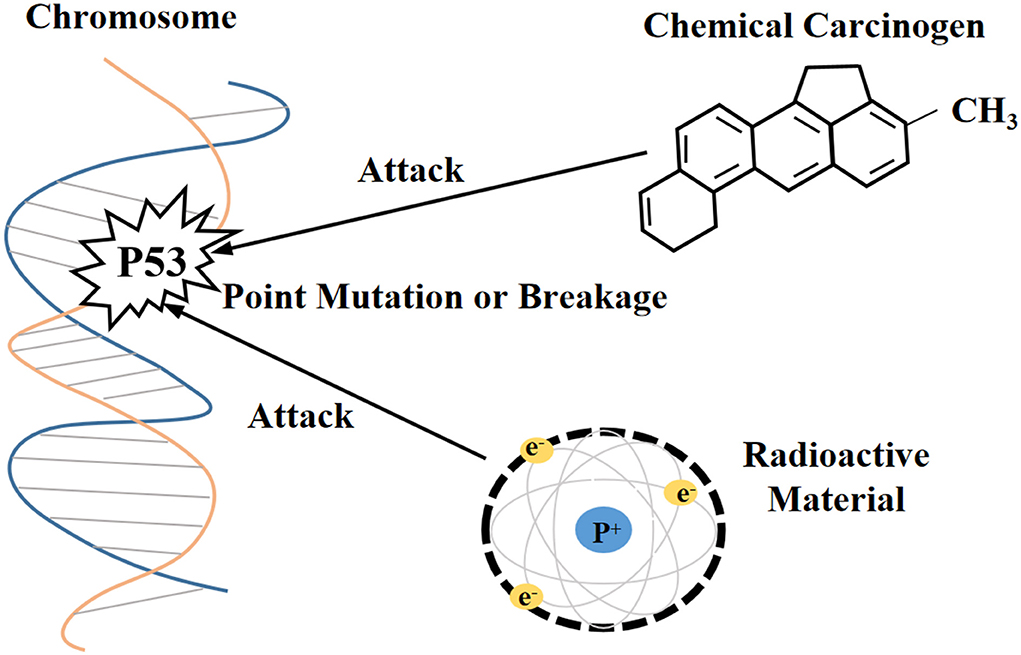
Figure 1. Physicochemical stimulation disrupts the molecular structure of proto-oncogenes and tumor suppressor genes.
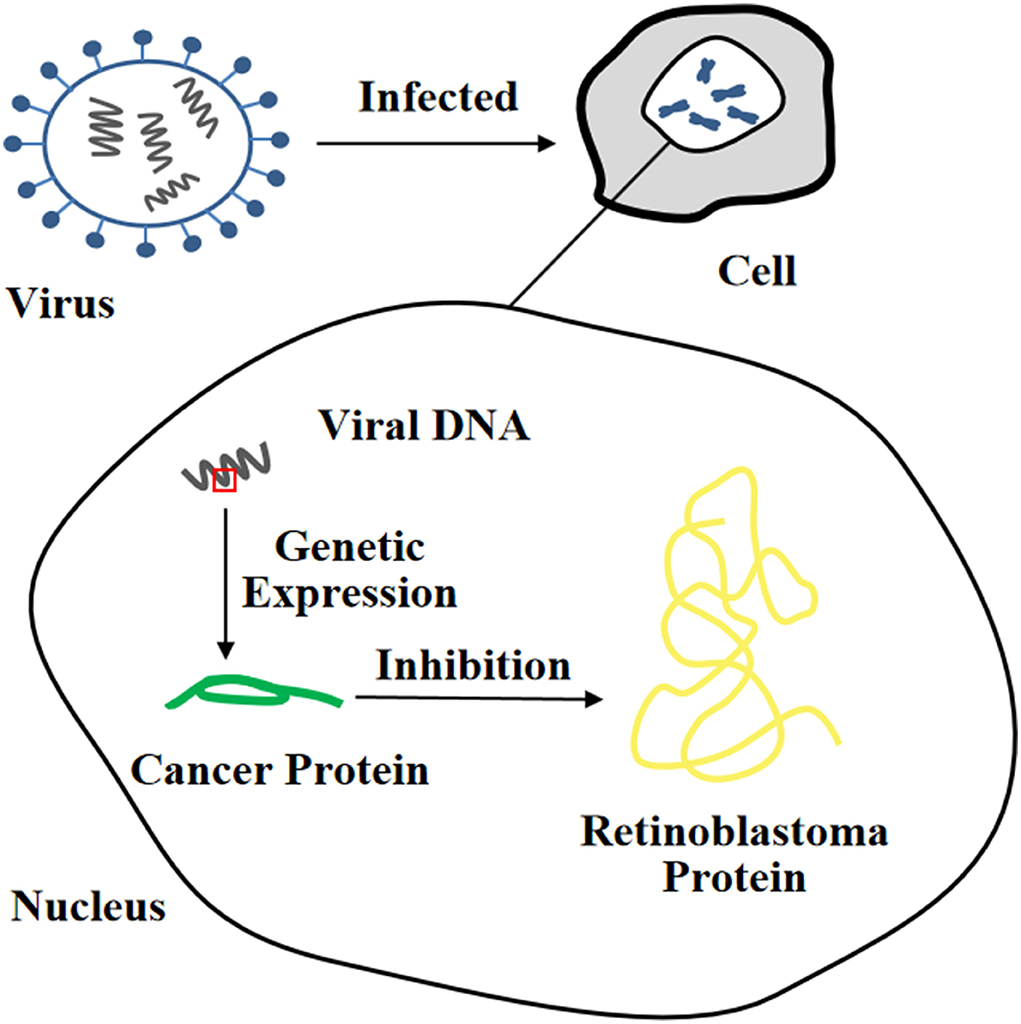
Figure 2. Heterologous expression of viral oncogene inhibits the function of tumor suppressor protein.
Physical and chemical stimulation
Immortalization of cells induced by radioactive factors
In previous studies, researchers have attempted to induce cells with unlimited proliferation using X-rays or gamma rays. For example, results from an experiment indicated that human skin fibroblasts with a mutant p53 allele could proliferate continually and exceeded 450 PDs in vitro after periodic X-ray irradiation, whereas the unirradiated control group cells could only be cultured to 37 PDs (30). Relevant phenotypes of immortalized cells obtained with such methods could be transferred by DNA transfection, which has been demonstrated in mouse cells (31). Previous study has shown that in place of it was suggested that treatment with Harvery murine sarcoma virus (Ha-MSV) alone did not promote the transformation of normal human fibroblasts into immortalized or tumorigenic cells, while immortalized fibroblasts KMST-6 formed by Co60γ-ray irradiation after treatment of Ha-MSV, and transplanted them into nude mice could acquire anchorage independent growth potential and eventually generated tumors (32). Therefore, radioactive factor-induced immortalized cells may increase the risk of tumorigenesis.
Immortalization of cells induced by chemical carcinogens
N-methyl-N-nitro-N-nitrosoguanidine (MNNG) (33) and 3-methylcholanthrene (34) are chemical carcinogens that induce cell immortalization. A previous study has observed that rabbit tracheal epithelial cells proliferated exponentially in the second week of culture and reached plateau in the third week. However, after experiencing the MNNG process, some rabbit tracheal epithelial cells showed a relative delay in the onset of proliferation and recovered clonal activity in a later stage of the plateau phase (35). Nevertheless, immortalized cells induced by chemical carcinogens do not necessarily retain normal morphology and are adhesion-dependent (33). Therefore, their carcinogenesis risk cannot be neglected.
Heterologous expression of viral oncogenes
It is well-known that the simian virus 40 large T antigen (SV40-LT), human papilloma virus E6 or E7 protein (HPV E6/E7), and Epstein-Barr Virus (EBV) are oncogenes. Among them, the SV40-LT gene fragment is one of the most commonly used target fragments for inducing cell immortalization. Integrating it into the target cell nucleus for expression can cause inactivation of the p53 and Rb proteins, thereby changing cell proliferation activity and prolonging cell lifespan (36). However, the length of telomeres will gradually shorten until cells stop growing, and only a few cells can completely leave the cell cycle and continue to proliferate, eventually forming immortalized cell lines (37). In recent years, SV40-LT has been successfully used in the establishment of immortalized cell lines of livestock and poultry such as pigs (38), cattle (25), sheep (24), and ducks (39).
In addition, infection with HPV E6/E7 can also immortalize a large number of different types of cells (40, 41). The HPV E6 protein, as one of the most common transforming proteins, can cause degradation of the p53 protein and upregulate the expression level of cellular-myelocytomatosis viral oncogene (c-myc) (42). Furthermore, it can also induce the expression of human telomerase reverse transcriptase (hTERT) and enable cells to acquire the ability of indefinite proliferation (43). There are many binding sites for c-myctranscription factor on the promoter of hTERT, so c-myc can mediate hTERT transcriptional activation and rapidly induce hTERT mRNA to express (44). The HPV E7 protein can lead to degradation of the Rb protein (45). It was reported that retroviruses containing the HPV E6/E7 gene was used to infect human pancreatic duct epithelial cells to establish the corresponding immortalized cell line, which could be passaged more than 20 times, retaining the anchorage dependence of mammalian cells with non-carcinogenic effects (40). Currently, the EBV is mostly used to immortalize B lymphocytes. The EBV genome contains more than 100 genes, and only a few genes (so-called latent genes) can be expressed in EBV-infected B lymphocytes. For instance, it is capable of infecting B lymphoblastoid cells in vitro, activating the interaction of cytokines with their receptors by expressing latent proteins, and forming immortalized lymphoblastoid cell lines. It is worth noting that the most notable feature of immortalized B cells induced by EBV is increased telomerase activity (46).
Telomerase causing cell immortalization
Telomerase
Telomerase is a kind of a specific reverse transcriptase and includes three components: telomerase RNA (TR), telomerase-associated protein, and telomerase reverse transcriptase (TERT) or telomerase catalytic subunit. Using its own RNA as a template to extend telomeres from the 3′-OH end of telomeric DNA or synthesize new telomeric DNA, it can compensate for the shortening of chromosome ends during cell division, so as to maintain the length of telomeres and prevent cells from the apoptosis caused by telomere depletion (47). Telomerase almost has no activity in normal cells but with expression in stem cells and germ cells. The activity of telomerase is elevated in most immortalized cell lines and various human tumor tissues, suggesting that telomerase activity is closely related to occurrence and development of tumors (48).
Rebuild telomerase activity to immortalize cells
In 1998, it was first reported that after the exogenous hTERT gene was introduced into telomerase-negative normal human retinal pigment epithelial cells, the intracellular telomerase was activated and the endogenous β-galactosidase (senescent marker) was significantly reduced (49). Besides, a previous study has claimed that after transfection with retrovirus-mediated exogenous hTERT gene, normal human breast epithelial cells gained stable telomere length, longer lifespan (40 PDs more than primary cells), less obvious β-galactosidase staining, and unchanged plasminogen activator inhibitor expression (PAI, another senescent marker) (50).
Furthermore, it has been determined that hTERT could improve telomerase activity, stabilize telomere length in cells, increase the number of cellular PDs, slow down cell senescence, and prolong the lifespan of culture in vitro (51–56). Certain cells can maintain their original morphology and function while obtaining the ability to proliferate indefinitely (57, 58). For example, immortalized human bone marrow mesenchymal stem cell line carrying hTERT has been subjected to 290 PDs without losing cell contact inhibitory function. By observing cell morphology at 95 and 275 PDs, it was found that transfected cells had the ability to transform into adipocytes, chondrocytes, and osteoblasts (59). Currently, hTERT transfection alone can immortalize many livestock and poultry cells (Table 1), or it can be combined with viral oncogenes to improve the success rate of obtaining immortalized cells (69).
Spontaneously generated immortalized cells
During cell culture in vitro, some spontaneously immortalized cells are occasionally generated and show high proliferative potential without gene transfer (70–73). These cells achieve serum-independent growth and have higher saturation densities (74).
Rodent cells have a higher incidence of spontaneous immortalization, up to 10−5 or 10−6 (44). Previous research has discussed that human cells could escape aging only if both the p53 and Rb genes were inactivated simultaneously, and that dysregulation of the ARF-p53 pathway alone in rodent cells was sufficient for eternal proliferation (75). By comparing the expression of multiple genes in early passage bovine mammary epithelial cells (bMECs), senescent bMECs, spontaneously immortalized bMECs (BME65Cs), and human breast cancer MCF-7 cell line (76), it was found that BME65Cs had the general features of normal BMECs in terms of morphology and karyotype etc., accompanied by endogenous TERT activity and telomeres stability. Compared with MCF-7 cells, the oncogene c-myc was only slightly upregulated in BME65Cs, and the breast tumor-related genes Bcl-2-associated athanogene 1 (Bag-1) and transcriptional repressor 1 (TRPS-1) were not detected. Likewise, the expression of tumor suppressor gene p53 and cycle-dependent kinase inhibitory factor p16INK4a (also known as cyclin-dependent kinase inhibitor 2A, CDKN2A) in BME65Cs was decreased but not completely inactivated compared to earlier passages, indicating that spontaneous immortalized cell lines were not caused by mutations in the p53 or p16INK4a gene. In addition, the expression level of DNA methyltransferase was upregulated, suggesting that the co-suppression of cell aging and mitochondrial apoptosis pathways orchestrated the immortalization process of BME65Cs (76). That means the mechanism by which spontaneously immortalized cells escape replicative senescence is poorly understood.
Establishment and current status of livestock and poultry immortalized cell lines
The common methods for establishing non-carcinogenic immortalized cell lines
It is well known that cancer cells also have the ability to proliferate indefinitely, and that cells may become cancerous during the process of establishing cell lines. Soft agar assay and nude mouse tumorigenesis assay are widely recognized methods for testing whether immortalized cell lines are tumorigenic (77, 78). Studies have found that immortalized cell lines induced by radioactive substances and chemical carcinogens may increase the formation of cancer cells, which are rarely used today (32, 33). Immortalized cell lines established by inducing the combined expression of immortality genes, proto-oncogenes, and cell cycle regulators are also tumorigenic, such as porcine pancreatic ductal epithelial cells, which are often used to generate tumor models (79, 80).
However, some immortalized cell lines can still avoid the generation of cancer cells while maintaining the morphological and physiological characteristics of primary cells (63, 81). The current common immortalization methods that do not cause any cancer growth are mainly by hTERT or SV40-LT expression induction, such as porcine oral mucosal epithelial cell line (hTERT-POMEC) (61), canine bronchiolar epithelial cell line (hTERT-CBECs) (77), and pig liver cell line (GalT-KO-hep) (82). So far, anchorage-independent growth, chromosomal abnormalities, and tumorigenic transformation have not been observed during the culture of these cell lines.
Small mammalian and livestock cell lines
By comparing the establishment status of common small mammal (rats, mice, and rabbits) and livestock (such as pigs, cattle, and sheep) immortalized cell lines (Table 2), it is not difficult to find that most expression vectors carrying the SV40-LT or hTERT gene are transfected into cells to prolong their lifespan. Notably, the cell immortalization induced by the tetracycline Tet-on 3G system is reversible, and cell proliferation can be controlled with doxycycline (Dox), which is more flexible (93).
As an example, the characteristics of immortalized pig cell lines separately obtained by transfecting SV40-LT and hTERT are compared (Table 3). It is observed that immortalization effects can be evaluated from the aspects of cell lifespan, telomerase activity, passage times, PDs, cell morphology, and tumorigenicity.
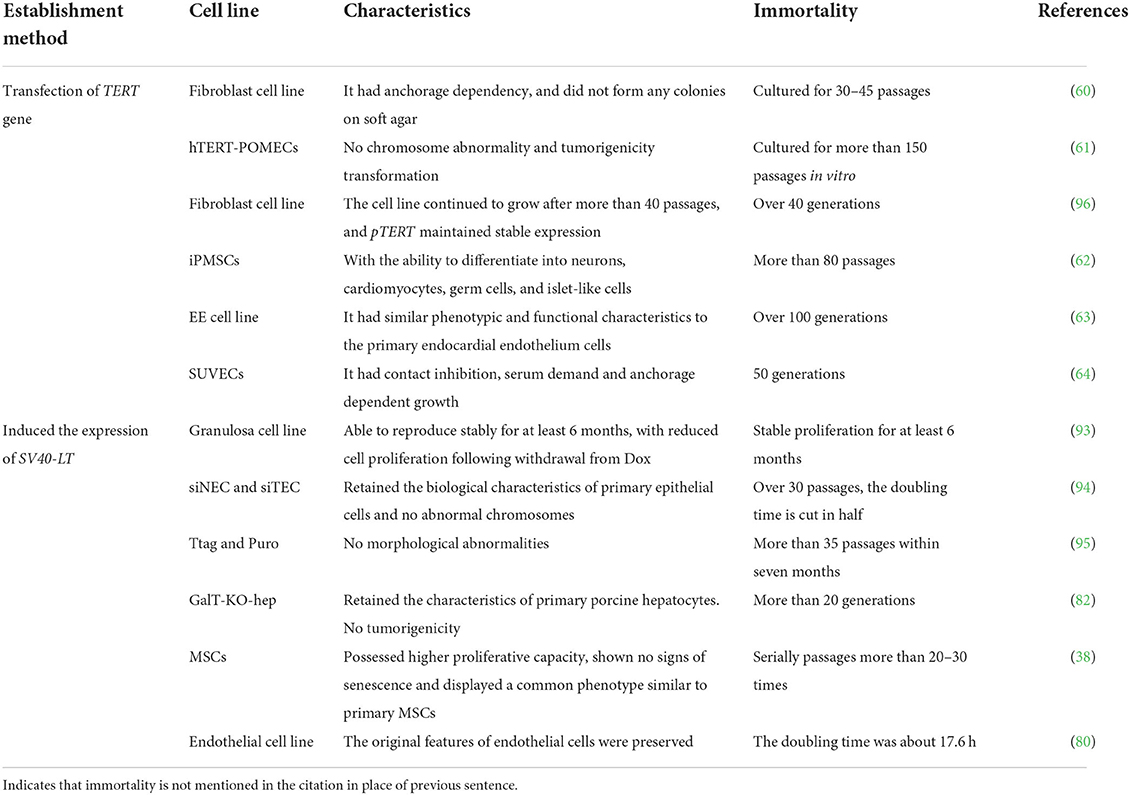
Table 3. Characteristics of immortalized pig cell lines established by transfecting SV40-LT and hTERT.
Establishment of poultry cell lines
We summarized poultry cell lines and their characteristics, including chickens, ducks, geese, and quails (Table 4). It was found that few immortalized cell lines were successfully established in poultry compared with mammals, and that the existing poultry cell lines were mainly obtained from tumor tissues; some chemical carcinogens or oncogenic viruses were used to immortalize specific types of bird cells, and some continuous cell lines were spontaneously generated. There are two points worth noting: (i) the preadipocyte lines “ICP1” and “ICP2” successfully established by transfection with the chicken telomerase reverse transcriptase (chTERT) have a high proliferation potential without malignant transformation after long-term culture, which provides a new idea and theoretical reference for the acquisition of other immortalized poultry cell lines (7), and (ii) during the whole process of establishing immortalized cell lines, specific antibiotics can be used to screen out positive cells expressing the SV40-LT gene or other target genes and then select a single transforming focus for subculture, which is not only simple but also safer (27).
Conclusions
Establishing an ideal immortalized cell line with infinite proliferation ability and maintaining the characteristics of its source tissue cells cannot only avoid the complicated process of primary cell separation and purification, reduce the time and energy consumption of researchers, and save the cost of experiments, it is also conducive to the research on scientific issues such as gene function of livestock and poultry, and rapidly promotes the development of science. Since immortalized cells can be passaged multiple times in vitro, researchers can immortalize cells that are difficult to passage, slow to proliferate, and prone to senescence, and provide more cell resources for related experiments. Nevertheless, whether the functional cells from different species adopt the same immortalization method, and how to quickly and efficiently prepare immortalized cells and to ensure the immortalized cells maintaining the original characteristics have not yet been solved and require more in-depth research. In summary, the application of immortalized cells has broad prospects. The continuous improvement of immortalized cell line establishment technology is conducive to further research in molecular biology and other scientific fields.
Author contributions
DG participated in literature collection, drafted the manuscript, and revised it. LZ participated in the design of this review and revised it. XW participated in literature collection. JZ helped draft the manuscript. SL conceived the review, participated in literature collection, revised the manuscript, and finally agreed to publish it. All authors read and approved the final version of the manuscript.
Funding
This research was funded by the National Natural Science Foundation of China (Grant No. 31972550), Guangdong Province (Grant Nos. 2020A1515011576 and 2020B1515420008), and College of Coastal Agricultural Sciences—Ph.D. Start-up Fee and Postgraduate Training Fund (Grant No. 060302052104).
Acknowledgments
Thanks to SL for the English revision.
Conflict of interest
The authors declare that the research was conducted in the absence of any commercial or financial relationships that could be construed as a potential conflict of interest.
Publisher's note
All claims expressed in this article are solely those of the authors and do not necessarily represent those of their affiliated organizations, or those of the publisher, the editors and the reviewers. Any product that may be evaluated in this article, or claim that may be made by its manufacturer, is not guaranteed or endorsed by the publisher.
Abbreviations
PBS, Phosphate buffered solution; FBS, fetal bovine serum; PDs, population doublings; MNNG, N-methyl-N-nitro-N-nitrosoguanidine; SV40-LT, Simian virus 40 large T antigen; HPV E6/E7, human papilloma virus E6 or E7 protein; EBV, Epstein-Barr virus; c-myc, cellular-myelocytomatosis viral oncogene; hTERT, human telomerase reverse transcriptase; TR, telomerase RNA; PAI, plasminogen activator inhibitor; iPMSCs, immortalized porcine mesenchymal stem cells; EE, endocardial endothelium; SUVECs, swine umbilical vein endothelial cells; AEC, alveolar epithelial cell; BME, bovine microvascular endothelium; STCs, sheep trophoblast cells; bMECs, bovine mammary epithelial cells; Bag-1, Bcl-2-associated athanogene 1; TRPS-1, transcriptional repressor 1; CDKN2A, cyclin-dependent kinase inhibitor 2A; Dox, doxycyline; RKCs, rat Kupffer cells; EOE, enamel organ epithelial; MSCs, mesenchymal stem cells; CDK4, cyclin-dependent kinase 4; chTERT, chicken telomerase reverse transcriptase; ASV, avian sarcoma virus; ALSV, avian leukosis sarcoma virus; DEFs, duck embryo fibroblasts.
References
1. Stacey G, MacDonald C. Immortalization of primary cells. Cell Biol Toxicol. (2001) 17:231–46. doi: 10.1023/A:1012525014791
2. Matsuda A, Shimizu Y, Kanda T, Ohnishi A, Maeta N, Miyabe M, et al. Establishment of a canine lens epithelial cell line. Can J Vet Res. (2021) 85:236–40.
3. Wang JM, Chen AF, Zhang K. Isolation and primary culture of mouse aortic endothelial cells. J Vis Exp. (2016) 19:e52965. doi: 10.3791/52965
4. Su F, Liu X, Liu G, Yu Y, Wang Y, Jin Y, et al. Establishment and evaluation of a stable cattle type II alveolar epithelial cell line. PLoS ONE. (2013) 8:e76036. doi: 10.1371/journal.pone.0076036
5. Sadkowski T, Ciecierska A, Oprzadek J, Balcerek E. Breed–dependent microRNA expression in the primary culture of skeletal muscle cells subjected to myogenic differentiation. BMC Genomics. (2018) 19:109. doi: 10.1186/s12864-018-4492-5
6. Souza YRM, Manso PPA, Oliveira BCD, Terra MABL, Paschoal T, Caminha G, et al. Generation of yellow fever virus vaccine in skeletal muscle cells of chicken embryos. Mem Inst Oswaldo Cruz. (2019) 114:e190187. doi: 10.1590/0074-02760190187
7. Zhang Y, Shi J, Liu S. Establishment and characterization of a telomerase–immortalized sheep trophoblast cell line. Biomed Res Int. (2016) 2016:5808575. doi: 10.1155/2016/5808575
8. Luo W, Lin Z, Chen J, Chen G, Zhang S, Liu M, et al. TMEM182 interacts with integrin beta 1 and regulates myoblast differentiation and muscle regeneration. J Cachexia Sarcopenia Muscle. (2021) 12:1704–23. doi: 10.1002/jcsm.12767
9. Choi KH, Yoon JW, Kim M, Lee HJ, Jeong J, Ryu M, et al. Muscle stem cell isolation and in vitro culture for meat production: a methodological review. Compr Rev Food Sci Food Saf. (2021) 20:429–57. doi: 10.1111/1541-4337.12661
10. Liu P, Bian Y, Zhong J, Yang Y, Mu X, Liu Z. Establishment and characterization of a rat intestinal microvascular endothelial cell line. Tissue Cell. (2021) 72:101573. doi: 10.1016/j.tice.2021.101573
11. Lojk J, Mis K, Pirkmajer S, Pavlin M. siRNA delivery into cultured primary human myoblasts—-optimization of electroporation parameters and theoretical analysis. Bioelectromagnetics. (2015) 36:551–63. doi: 10.1002/bem.21936
12. Bautista–Amorocho H, Silva–Sayago JA, Goyeneche–Patino DA, Pérez–Cala TL, Macías–Gómez F, Arango–Viana JC, et al. A novel method for isolation and culture of primary swine gastric epithelial cells. BMC Mol Cell Biol. (2021) 2021:1. doi: 10.1186/s12860-020-00341-7
13. Wang W, Zhang T, Wu C, Wang S, Wang Y, Li H, et al. Immortalization of chicken preadipocytes by retroviral transduction of chicken TERT and TR. PLoS ONE. (2017) 12:e0177348. doi: 10.1371/journal.pone.0177348
14. Chenari N, Khademi B, Razmkhah M. EZB–ICR cell line: a new established and characterized oral squamous cell carcinoma cell line from tongue. Asian Pac J Cancer Prev. (2021) 22:99–103. doi: 10.31557/APJCP.2021.22.1.99
15. Bukowy–Bieryłło Z. Long–term differentiating primary human airway epithelial cell cultures: how far are we? Cell Commun Signal. (2021) 19:63. doi: 10.1186/s12964-021-00740-z
16. Martinovich KM, Iosifidis T, Buckley AG, Looi K, Ling KM, Sutanto EN, et al. Conditionally reprogrammed primary airway epithelial cells maintain morphology, lineage and disease specific functional characteristics. Sci Rep. (2017) 7:17971. doi: 10.1038/s41598-017-17952-4
17. Liu Y, Wang CH, Fan J, Peng JX, Pan J, Zhang X, et al. Establishing a papillary craniopharyngioma cell line by SV40LT–mediated immortalization. Pituitary. (2021) 24:159–69. doi: 10.1007/s11102-020-01093-5
18. Hu H, Zheng N, Gao H, Dai W, Zhang Y, Li S, et al. Immortalized bovine mammary epithelial cells express stem cell markers and differentiate in vitro. Cell Biol Int. (2016) 40:861–72. doi: 10.1002/cbin.10624
19. de Lange T. Protection of mammalian telomeres. Oncogene. (2002) 21:532–40. doi: 10.1038/sj.onc.1205080
20. Blackburn EH. Structure and function of telomeres. Nature. (1991) 350:569–73. doi: 10.1038/350569a0
21. Cerni C. Telomeres, telomerase, and myc. An update. Mutat Res. (2000) 462:31–47. doi: 10.1016/S1383-5742(99)00091-5
22. Sharpless N.E, Ramsey MR, Balasubramanian P, Castrillon DH, DePinho RA. The differential impact of p16 (INK4a) or p19 (ARF) deficiency on cell growth and tumorigenesis. Oncogene. (2004) 23:379–85. doi: 10.1038/sj.onc.1207074
23. D'Amico M, Wu K, Fu M, Rao M, Albanese C, Russell RG, et al. The inhibitor of cyclin–dependent kinase 4a/alternative reading frame (INK4a/ARF) locus encoded proteins p16INK4a and p19ARF repress cyclin D1 transcription through distinct cis elements. Cancer Res. (2004) 64:4122–30. doi: 10.1158/0008-5472.CAN-03-2519
24. Seridi N, Hamidouche M, Belmessabih N, El Kennani S, Gagnon J, Martinez G, et al. Immortalization of primary sheep embryo kidney cells. In Vitro Cell Dev Biol Anim. (2021) 57:76–85. doi: 10.1007/s11626-020-00520-y
25. Takenouchi T, Iwamaru Y, Sato M, Yokoyama T, Shinagawa M, Kitani H. Establishment and characterization of SV40 large T antigen–immortalized cell lines derived from fetal bovine brain tissues after prolonged cryopreservation. Cell Biol Int. (2007) 31:57–64. doi: 10.1016/j.cellbi.2006.09.006
26. Muñoz–Gutiérrez JF, Schneider DA, Baszler TV, Greenlee JJ, Nicholson EM, Stanton JB. hTERT–immortalized ovine microglia propagate natural scrapie isolates. Virus Res. (2015) 198:35–43. doi: 10.1016/j.virusres.2014.10.028
27. Wu LA, Yuan G, Yang G, Ortiz–Gonzalez I, Yang W, Cui Y, et al. Immortalization and characterization of mouse floxed Bmp2/4 osteoblasts. Biochem Biophys Res Commun. (2009) 386:89–95. doi: 10.1016/j.bbrc.2009.05.144
28. Dahodwala H, Lee KH. The fickle CHO: a review of the causes, implications, and potential alleviation of the CHO cell line instability problem. Curr Opin Biotechnol. (2019) 60:128–37. doi: 10.1016/j.copbio.2019.01.011
29. Maqsood MI, Matin MM, Bahrami AR, Ghasroldasht MM. Immortality of cell lines: challenges and advantages of establishment. Cell Biol Int. (2013) 37:1038–45. doi: 10.1002/cbin.10137
30. Tsutsui T, Tanaka Y, Matsudo Y, Hasegawa K, Fujino T, Kodama S, et al. Extended lifespan and immortalization of human fibroblasts induced by X–ray irradiation. Mol Carcinog. (1997) 18:7–18. doi: 10.1002/(SICI)1098-2744(199701)18:1<7::AID-MC2>3.0.CO;2-F
31. Too C.K, Sierra–Rivera E, Oberley LW, Guernsey DL. Passage of X–ray–induced immortal, non–transformed phenotype by DNA–mediated transfection. Cancer Lett. (1995) 97:39–47. doi: 10.1016/0304-3835(95)03949-W
32. Namba M, Nishitani K, Fukushima F, Kimoto T. Multistep carcinogenesis of normal human fibroblasts. Human fibroblasts immortalized by repeated treatment with Co−60 gamma rays were transformed into tumorigenic cells with Ha–ras oncogenes. Anticancer Res. (1988) 8:947–58.
33. Marczynska B, Khoobyarian N, Chao TS, Tao M. Phorbol ester promotes growth and transformation of carcinogen–exposed nonhuman primate cells in vitro. Anticancer Res. (1991) 11:1711–7.
34. Kawaguchi T, Nomura K, Hirayama Y, Kitagawa T. Establishment and characterization of a chicken hepatocellular carcinoma cell line, LMH. Cancer Res. (1987) 47:4460–4.
35. Kitamura H, Gray TE, Jetten AM, Inayama Y, Nettesheim P. Enhanced growth potential of cultured rabbit tracheal epithelial cells following exposure to N–methyl–N'–nitro–N–nitrosoguanidine. Jpn J Cancer Res. (1993) 84:1113–9. doi: 10.1111/j.1349-7006.1993.tb02810.x
36. Manfredi JJ, Prives C. The transforming activity of simian virus 40 large tumor antigen. Biochim Biophys Acta. (1994) 1198:65–83. doi: 10.1016/0304-419X(94)90006-X
37. Bryan TM, Reddel RR. SV40–induced immortalization of human cells. Crit Rev Oncog. (1994) 5:331–57. doi: 10.1615/CritRevOncog.v5.i4.10
38. Moscoso I, Rodriguez–Barbosa JI, Barallobre–Barreiro J, Anon P, Domenech N. Immortalization of bone marrow–derived porcine mesenchymal stem cells and their differentiation into cells expressing cardiac phenotypic markers. J Tissue Eng Regen Med. (2012) 6:655–65. doi: 10.1002/term.469
39. Fu Y, Chen Z, Li C, Liu G. Establishment of a duck cell line susceptible to duck hepatitis virus type 1. J Virol Methods. (2012) 184:41–5. doi: 10.1016/j.jviromet.2012.05.004
40. Furukawa T, Duguid WP, Rosenberg L, Viallet J, Galloway DA, Tsao MS. Long–term culture and immortalization of epithelial cells from normal adult human pancreatic ducts transfected by the E6E7 gene of human papilloma virus 16. Am J Pathol. (1996) 148:1763–70.
41. Reznikoff CA, Belair C, Savelieva E, Zhai Y, Pfeifer K, Yeager T, et al. Long–term genome stability and minimal genotypic and phenotypic alterations in HPV16 E7–, but not E6–, immortalized human uroepithelial cells. Genes Dev. (1994) 8:2227–40. doi: 10.1101/gad.8.18.2227
42. Klingelhutz AJ, Foster SA, McDougall JK. Telomerase activation by the E6 gene product of human papillomavirus type 16. Nature. (1996) 380:79–82. doi: 10.1038/380079a0
43. Veldman T, Horikawa I, Barrett JC, Schlegel R. Transcriptional activation of the telomerase hTERT gene by human papillomavirus type 16 E6 oncoprotein. J Virol. (2001) 75:4467–72. doi: 10.1128/JVI.75.9.4467-4472.2001
44. Katakura Y, Alam S, Shirahata S. Immortalization by gene transfection. Methods Cell Biol. (1998) 57:69–91. doi: 10.1016/S0091-679X(08)61573-3
45. Boyer SN, Wazer DE, Band V. E7 protein of human papilloma virus−16 induces degradation of retinoblastoma protein through the ubiquitin–proteasome pathway. Cancer Res. (1996) 56:4620–4.
46. Sugimoto M, Ide T, Goto M, Furuichi Y. Reconsideration of senescence, immortalization and telomeres maintenance of Epstein–Barr virus–transformed human B–lymphoblastoid cell lines. Mech Ageing Dev. (1999) 107:51–60. doi: 10.1016/S0047-6374(98)00131-6
47. Greider CW, Blackburn EH. The telomeres terminal transferase of tetrahymena is a ribonucleoprotein enzyme with two kinds of primer specificity. Cell. (1987) 51:887–98. doi: 10.1016/0092-8674(87)90576-9
48. Kim NW, Piatyszek MA, Prowse KR, Harley CB, West MD, Ho PL, et al. Specific association of human telomerase activity with immortal cells and cancer. Science. (1994) 266:2011–5. doi: 10.1126/science.7605428
49. Bodnar AG, Ouellette M, Frolkis M, Holt SE, Chiu CP, Morin GB, et al. Extension of life–span by introduction of telomerase into normal human cells. Science. (1998) 279:349–52. doi: 10.1126/science.279.5349.349
50. Wang J, Xie LY, Allan S, Beach D, Hannon GJ. Myc activates telomerase. Genes Dev. (1998) 12:1769–74. doi: 10.1101/gad.12.12.1769
51. Vaziri H, Benchimol S. Reconstitution of telomerase activity in normal human cells leads to elongation of telomeres and extended replicative life span. Curr Biol. (1998) 8:279–82. doi: 10.1016/S0960-9822(98)70109-5
52. Halvorsen TL, Leibowitz G, Levine F. Telomerase activity is sufficient to allow transformed cells to escape from crisis. Mol Cell Biol. (1999) 19:1864–70. doi: 10.1128/MCB.19.3.1864
53. Ouellette MM, Aisner DL, Savre–Train I, Wright WE, Shay JW. Telomerase activity does not always imply telomeres maintenance. Biochem Biophys Res Commun. (1999) 254:795–803. doi: 10.1006/bbrc.1998.0114
54. Ouellette MM, McDaniel LD, Wright WE, Shay JW, Schultz RA. The establishment of telomerase–immortalized cell lines representing human chromosome instability syndromes. Hum Mol Genet. (2000) 9:403–11. doi: 10.1093/hmg/9.3.403
55. Simonsen JL, Rosada C, Serakinci N, Justesen J, Stenderup K, Rattan SI, et al. Telomerase expression extends the proliferative life–span and maintains the osteogenic potential of human bone marrow stromal cells. Nat Biotechnol. (2002) 20:592–6. doi: 10.1038/nbt0602-592
56. Mihara K, Imai C, Coustan–Smith E, Dome JS, Dominici M, Vanin E, et al. Development and functional characterization of human bone marrow mesenchymal cells immortalized by enforced expression of telomerase. Br J Haematol. (2003) 120:846–9. doi: 10.1046/j.1365-2141.2003.04217.x
57. Kawano Y, Kobune M, Yamaguchi M, Nakamura K, Ito Y, Sasaki K, et al. Ex vivo expansion of human umbilical cord hematopoietic progenitor cells using a coculture system with human telomerase catalytic subunit (hTERT)–transfected human stromal cells. Blood. (2003) 101:532–40. doi: 10.1182/blood-2002-04-1268
58. Wege H, Le HT, Chui MS, Liu L, Wu J, Giri R, et al. Telomerase reconstitution immortalizes human fetal hepatocytes without disrupting their differentiation potential. Gastroenterology. (2003) 124:432–44. doi: 10.1053/gast.2003.50064
59. Huang G, Zheng Q, Sun J, Guo C, Yang J, Chen R, et al. Stabilization of cellular properties and differentiation mutilpotential of human mesenchymal stem cells transduced with hTERT gene in a long–term culture. J Cell Biochem. (2008) 103:1256–69. doi: 10.1002/jcb.21502
60. Le QVC, Youk S, Choi M, Jeon H, Kim WI, Ho CS, et al. Development of an immortalized porcine fibroblast cell panel with different swine leukocyte antigen genotypes. Front Genet. (2022) 13:815328. doi: 10.3389/fgene.2022.815328
61. Cui H, Liang W, Wang D, Guo K, Zhang Y. Establishment and characterization of an immortalized porcine oral mucosal epithelial cell line as a cytopathogenic model for porcine circovirus 2 infection. Front Cell Infect Microbiol. (2019) 9:171. doi: 10.3389/fcimb.2019.00171
62. Cao H, Chu Y, Zhu H, Sun J, Pu Y, Gao Z, et al. Characterization of immortalized mesenchymal stem cells derived from foetal porcine pancreas. Cell Prolif. (2011) 44:19–32. doi: 10.1111/j.1365-2184.2010.00714.x
63. Kuruvilla L, Santhoshkumar TS, Kartha CC. Immortalization and characterization of porcine ventricular endocardial endothelial cells. Endothelium. (2007) 14:35–43. doi: 10.1080/10623320601177353
64. Hong HX, Zhang YM, Xu H, Su ZY, Sun P. Immortalization of swine umbilical vein endothelial cells with human telomerase reverse transcriptase. Mol Cells. (2007) 24:358–63.
65. Buser R, Montesano R, Garcia I, Dupraz P, Pepper MS. Bovine microvascular endothelial cells immortalized with human telomerase. J Cell Biochem. (2006) 98:267–86. doi: 10.1002/jcb.20715
66. Jin X, Lee JS, Kwak S, Lee SY, Jung JE, Kim TK, et al. Establishment and characterization of three immortal bovine muscular epithelial cell lines. Mol Cells. (2006) 21:29–33.
67. Zhang N, Li J, Zhong X, An X, Hou J. Reversible immortalization of sheep fetal fibroblast cells by tetracycline–inducible expression of human telomerase reverse transcriptase. Biotechnol Lett. (2016) 38:1261–8. doi: 10.1007/s10529-016-2103-6
68. Cui W, Wylie D, Aslam S, Dinnyes A, King T, Wilmut I, et al. Telomerase–immortalized sheep fibroblasts can be reprogrammed by nuclear transfer to undergo early development. Biol Reprod. (2003) 69:15–21. doi: 10.1095/biolreprod.102.013250
69. Bi CM, Zhang SQ, Zhang Y, Peng SY, Wang L, An ZX, et al. Immortalization of bovine germ line stem cells by c–myc and hTERT. Anim Reprod Sci. (2007) 100:371–8. doi: 10.1016/j.anireprosci.2006.10.017
70. Georgescu HI, Mendelow D, Evans CH. HIG−82: an established cell line from rabbit periarticular soft tissue, which retains the “activatable” phenotype. In Vitro Cell Dev Biol. (1988) 24:1015–22. doi: 10.1007/BF02620875
71. Takahashi K, Sawasaki Y, Hata J, Mukai K, Goto T. Spontaneous transformation and immortalization of human endothelial cells. In Vitro Cell Dev Biol. (1990) 26:265–74. doi: 10.1007/BF02624456
72. Castro–Muñozledo F. Development of a spontaneous permanent cell line of rabbit corneal epithelial cells that undergoes sequential stages of differentiation in cell culture. J Cell Sci. (1994) 107:2343–51. doi: 10.1242/jcs.107.8.2343
73. Kageyama T, Hayashi R, Hara S, Yoshikawa K, Ishikawa Y, Yamato M, et al. Spontaneous acquisition of infinite proliferative capacity by a rabbit corneal endothelial cell line with maintenance of phenotypic and physiological characteristics. J Tissue Eng Regen Med. (2017) 11:1057–64. doi: 10.1002/term.2005
74. Nachtigal M, Nagpal ML, Greenspan P, Nachtigal SA, Legrand A. Characterization of a continuous smooth muscle cell line derived from rabbit aorta. In Vitro Cell Dev Biol. (1989) 25:892–8. doi: 10.1007/BF02624001
75. Delia D, Fontanella E, Ferrario C, Chessa L, Mizutani S. DNA damage–induced cell–cycle phase regulation of p53 and p21waf1 in normal and ATM–defective cells. Oncogene. (2003) 22:7866–9. doi: 10.1038/sj.onc.1207086
76. Zhao C, Meng L, Hu H, Wang X, Shi F, Wang Y, et al. Spontaneously immortalised bovine mammary epithelial cells exhibit a distinct gene expression pattern from the breast cancer cells. BMC Cell Biol. (2010) 11:82. doi: 10.1186/1471-2121-11-82
77. Xie X, Pang M, Liang S, Yu L, Zhao Y, Ma K, et al. Establishment and characterization of a telomerase–immortalized canine bronchiolar epithelial cell line. Appl Microbiol Biotechnol. (2015) 99:9135–46. doi: 10.1007/s00253-015-6794-8
78. Ma GL, Qiao ZL, He D, Wang J, Kong YY, Xin XY, et al. Establishment of a low–tumorigenic MDCK cell line and study of differential molecular networks. Biologicals. (2020) 68:112–21. doi: 10.1016/j.biologicals.2020.07.003
79. Bailey KL, Cartwright SB, Patel NS, Remmers N, Lazenby AJ, Hollingsworth MA, et al. Porcine pancreatic ductal epithelial cells transformed with KRASG12D and SV40T are tumorigenic. Sci Rep. (2021) 11:13436. doi: 10.1038/s41598-021-92852-2
80. Kim D, Kim JY, Koh HS, Lee JP, Kim YT, Kang HJ, et al. Establishment and characterization of endothelial cell lines from the aorta of miniature pig for the study of xenotransplantation. Cell Biol Int. (2005) 29:638–46. doi: 10.1016/j.cellbi.2005.03.016
81. Callesen MM, Árnadóttir SS, Lyskjaer I, Ørntoft MW, Høyer S, Dagnaes–Hansen F, et al. A genetically inducible porcine model of intestinal cancer. Mol Oncol. (2017) 11:1616–29. doi: 10.1002/1878-0261.12136
82. Wang Q, Zhang X, Wang B, Bai G, Pan D, Yang P, et al. Immortalization of porcine hepatocytes with a α-1,3–galactosyltransferase knockout background. Xenotransplantation. (2020) 27:e12550. doi: 10.1111/xen.12550
83. Peng Y, Murr MM. Establishment of immortalized rat Kupffer cell lines. Cytokine. (2007) 37:185–91. doi: 10.1016/j.cyto.2007.03.003
84. MacDougall M, Mamaeva O, Lu C, Chen S. Establishment and characterization of immortalized mouse ameloblast–like cell lines. Orthod Craniofac Res. (2019) 22:134–41. doi: 10.1111/ocr.12313
85. Sakai Y, Miyake R, Shimizu T, Nakajima T, Sakakura T, Tomooka Y. A clonal stem cell line established from a mouse mammary placode with ability to generate functional mammary glands. In Vitro Cell Dev Biol Anim. (2019) 55:861–71. doi: 10.1007/s11626-019-00406-8
86. Kawasaki H, Ohama T, Hori M, Sato K. Establishment of mouse intestinal myofibroblast cell lines. World J Gastroenterol. (2013) 9:2629–37. doi: 10.3748/wjg.v19.i17.2629
87. Komine A, Abe M, Saeki T, Terakawa T, Uchida C, Uchida T. Establishment of adipose–derived mesenchymal stem cell lines from a p53–knockout mouse. Biochem Biophys Res Commun. (2012) 426:468–74. doi: 10.1016/j.bbrc.2012.08.094
88. Bartlett PF, Reid HH, Bailey KA, Bernard O. Immortalization of mouse neural precursor cells by the c–myc oncogene. Proc Natl Acad Sci USA. (1988) 85:3255–9. doi: 10.1073/pnas.85.9.3255
89. Orimoto A, Katayama M, Tani T, Ito K, Eitsuka T, Nakagawa K, et al. Primary and immortalized cell lines derived from the amami rabbit (Pentalagus furnessi) and evolutionally conserved cell cycle control with CDK4 and cyclin D1. Biochem Biophys Res Commun. (2020) 525:1046–53. doi: 10.1016/j.bbrc.2020.03.036
90. Thenet S, Benya PD, Demignot S, Feunteun J, Adolphe M. SV40–immortalization of rabbit articular chondrocytes: alteration of differentiated functions. J Cell Physiol. (1992) 150:158–67. doi: 10.1002/jcp.1041500121
91. Chen Y, Hu S, Wang M, Zhao B, Yang N, Li J, et al. Characterization and establishment of an immortalized rabbit melanocyte cell line using the SV40 large T antigen. Int J Mol Sci. (2019) 20:4874. doi: 10.3390/ijms20194874
92. Araki K, Ohashi Y, Sasabe T, Kinoshita S, Hayashi K, Yang XZ, et al. Immortalization of rabbit corneal epithelial cells by a recombinant SV40–adenovirus vector. Invest Ophthalmol Vis Sci. (1993) 34:2665–71.
93. Bai Y, Zhu C, Feng M, Pan B, Zhang S, Zhan X, et al. Establishment of A reversibly inducible porcine granulosa cell line. Cells. (2020) 9:156. doi: 10.3390/cells9010156
94. Zhang K, Li H, Dong S, Liu Y, Wang D, Liu H, et al. Establishment and evaluation of a PRRSV–sensitive porcine endometrial epithelial cell line by transfecting SV40 large T antigen. BMC Vet Res. (2019) 15:299. doi: 10.1186/s12917-019-2051-1
95. Zheng Y, Feng T, Zhang P, Lei P, Li F, Zeng W. Establishment of cell lines with porcine spermatogonial stem cell properties. J Anim Sci Biotechnol. (2020) 11:33. doi: 10.1186/s40104-020-00439-0
96. He S, Li Y, Chen Y, Zhu Y, Zhang X, Xia X, et al. Immortalization of pig fibroblast cells by transposon–mediated ectopic expression of porcine telomerase reverse transcriptase. Cytotechnology. (2016) 68:1435–45. doi: 10.1007/s10616-015-9903-8
97. Kuroda K, Kiyono T, Isogai E, Masuda M, Narita M, Okuno K, et al. Immortalization of fetal bovine colon epithelial cells by expression of human cyclin D1, mutant cyclin dependent kinase 4, and telomerase reverse transcriptase: an in vitro model for bacterial infection. PLoS ONE. (2015) 10:e0143473. doi: 10.1371/journal.pone.0143473
98. Pace MC, Chambliss KL, German Z, Yuhanna IS, Mendelsohn ME, Shaul PW. Establishment of an immortalized fetal intrapulmonary artery endothelial cell line. Am J Physiol. (1999) 277:L106–12. doi: 10.1152/ajplung.1999.277.1.L106
99. Da Silva Teixeira MF, Lambert V, Mselli–Lakahl L, Chettab A, Chebloune Y, Mornex JF. Immortalization of caprine fibroblasts permissive for replication of small ruminant lentiviruses. Am J Vet Res. (1997) 58:579–84.
100. Zhao R, Jin J, Sun X, Jin K, Wang M, Ahmed MF, et al. The establishment of clonally derived chicken embryonic fibroblast cell line (CSC) with high transfection efficiency and ability as a feeder cell. J Cell Biochem. (2018) 119:8841–50. doi: 10.1002/jcb.27137
101. Lee J, Foster DN, Bottje WG, Jang HM, Chandra YG, Gentles LE, et al. Establishment of an immortal chicken embryo liver–derived cell line. Poult Sci. (2013) 92:1604–12. doi: 10.3382/ps.2012-02582
102. Himly M, Foster DN, Bottoli I, Iacovoni JS, Vogt PK. The DF−1 chicken fibroblast cell line: transformation induced by diverse oncogenes and cell death resulting from infection by avian leukosis viruses. Virology. (1998) 248:295–304. doi: 10.1006/viro.1998.9290
103. Kawaguchi T, Nomura K, Hirayama Y, Kitagawa T. Establishment and characterization of a chicken hepatocellular carcinoma cell line, LMH. Cancer Res. (1987) 47:4460–4.
104. Wang W, Said A, Wang Y, Fu Q, Xiao Y, Lv S, et al. Establishment and characterization of duck embryo epithelial (DEE) cell line and its use as a new approach toward DHAV−1 propagation and vaccine development. Virus Res. (2016) 213:260–8. doi: 10.1016/j.virusres.2015.12.021
105. Wang W, Said A, Wang B, Qu G, Xu Q, Liu B, et al. Establishment and evaluation of the goose embryo epithelial (GEE) cell line as a new model for propagation of avian viruses. PLoS ONE. (2018) 13:e0193876. doi: 10.1371/journal.pone.0193876
106. Antin PB, Ordahl CP. Isolation and characterization of an avian myogenic cell line. Dev Biol. (1991) 143:111–21. doi: 10.1016/0012-1606(91)90058-B
107. Jaffredo T, Chestier A, Bachnou N, Dieterlen–Lièvre F. MC29–immortalized clonal avian heart cell lines can partially differentiate in vitro. Exp Cell Res. (1991) 192:481–91. doi: 10.1016/0014-4827(91)90067-5
108. Moscovici C, Moscovici MG, Jimenez H, Lai MM, Hayman MJ, Vogt PK. Continuous tissue culture cell lines derived from chemically induced tumors of Japanese quail. Cell. (1977) 11:95–103. doi: 10.1016/0092-8674(77)90320-8
Keywords: livestock and poultry, immortalization, cell line, methods, telomerase activity
Citation: Guo D, Zhang L, Wang X, Zheng J and Lin S (2022) Establishment methods and research progress of livestock and poultry immortalized cell lines: A review. Front. Vet. Sci. 9:956357. doi: 10.3389/fvets.2022.956357
Received: 30 May 2022; Accepted: 10 August 2022;
Published: 02 September 2022.
Edited by:
Abdul Rasheed Baloch, University of Karachi, PakistanReviewed by:
Shanti Choudhary, Guru Angad Dev Veterinary and Animal Sciences University, IndiaAnjali Somal, Chaudhary Sarwan Kumar Himachal Pradesh Krishi Vishvavidyalaya, India
Copyright © 2022 Guo, Zhang, Wang, Zheng and Lin. This is an open-access article distributed under the terms of the Creative Commons Attribution License (CC BY). The use, distribution or reproduction in other forums is permitted, provided the original author(s) and the copyright owner(s) are credited and that the original publication in this journal is cited, in accordance with accepted academic practice. No use, distribution or reproduction is permitted which does not comply with these terms.
*Correspondence: Shudai Lin, bGluc2Q4OXN5bHZpYUAxNjMuY29t
†These authors have contributed equally to this work