- 1Guangxi Key Laboratory of Veterinary Biotechnology, Key Laboratory of China (Guangxi)-ASEAN Cross-Border Animal Disease Prevention and Control, Ministry of Agriculture and Rural Affairs of China, Guangxi Veterinary Research Institute, Nanning, China
- 2Brain Function and Disease Laboratory, Shantou University Medical College, Shantou, Guangdong, China
- 3Guangxi Mountain Comprehensive Technology Development Center, Nanning, China
Wumei San (WMS) is a traditional Chinese medicine that has been widely applied in the treatment of piglet diarrhea (PD). However, the mechanism of WMS in PD has not been investigated. In this study, the main active compounds of WMS and the target proteins were obtained from the Traditional Chinese Medicine Systematic Pharmacology, PubChem, and SwissTargetPrediction databases. The molecular targets of PD were identified using GeneCards, OMIM, and NCBI databases. The common targets of WMS and PD were screened out and converted into UniProt gene symbols. PD-related target genes were constructed into a protein-protein interaction network, which was further analyzed by the STRING online database. Gene Ontology and the Kyoto Encyclopedia of Genes and Genomes enrichment analyses were performed to construct the component-target gene-disease network. Molecular docking was then used to examine the relationship between the core compounds and proteins. As a result, a total of 32 active compounds and 638 target genes of WMS were identified, and a WMS-compound-target network was successfully constructed. Through network pharmacology analysis, 14 core compounds in WMS that showed an effect on PD were identified. The targets revealed by GO and KEGG enrichment analysis were associated with the AGE-RAGE signaling pathway, PI3K-Akt signaling pathway, TNF signaling pathway, NOD-like receptor signaling pathway, IL-17 signaling pathway, and other pathways and physiological processes. Molecular docking analysis revealed that the active compounds in WMS spontaneously bind to their targets. The results indicated that WMS may regulate the local immune response and inflammatory factors mainly through the TNF signaling pathway, IL-17 signaling pathway, and other pathways. WMS is a promising treatment strategy for PD. This study provides new insights into the potential mechanism of WMS in PD.
Introduction
Piglet diarrhea (PD) is a common disease, especially in 1- to 3-month-old piglets, with a high incidence in farming production (1). The incidence of diarrhea in weaned piglets is more than 30% and the mortality is 15%. PD may lead a decrease in the growth of piglets and a decrease in the feed reward. PD is also accompanied by physical decline, depressed immunity, weakened resistance to disease, and susceptibility to secondary and mixed infection of other infectious diseases. Without treatment, PD leads to a high death rate of piglets and severe economic injury to pig production (2, 3). PD is caused by general internal medicine diseases, sewage consumption, improper feed change, sudden weather changes, and cold stimulation. Other cases are caused by infectious diseases. Piglet yellow dysentery and piglet white dysentery are common bacterial diseases, and transmissible gastroenteritis, porcine epidemic diarrhea and rotavirus infection are common viral diseases in PD. The main strategies for treating PD in pig farms include antibiotics and medical levels of zinc oxide (4, 5). However, long-term medication and high zinc diet causes adverse effects on human health and the environment by contributing to the development of antimicrobial resistance among bacteria and to high soil concentrations of zinc, a heavy metal.
Because of their natural, eco-friendly, and safe nature, Chinese herbal feed additives and formulations have been used in increasing numbers in recent years for the prevention and treatment of PD (6, 7). According to the theory of traditional Chinese medicine, spleen and stomach weakness, cold dampness, dampness heat, and food injury are the important pathological factors of PD. The principle of treating PD is clearing heat and removing toxin, and astringing intestines and checking diarrhea. Wumei San (WMS) is a classic prescription for treating diarrhea in young animals. Its application history can be traced back to Hezi San from the earliest existing veterinary pharmacy Anji Prescription in Tang-Song period. WMS including Mume Fructus (MF), Coptis chinensis Franch. (CC), Curcuma longa L. (CL), Terminalia chebula Retz. (TC) and Diospyros kaki L.f. (DK) is now the latest recorded formula in Chinese Veterinary Pharmacopeia (2020 edition) II. Over thousands of years of evolution, only one or two herbs have changed in the prescription. MF, composed of organic acids, flavonoids and fatty acids, used to treat chronic cough, prolonged diarrhea, and other inflammation-related diseases (8). Previous studies have shown that weaned piglets fed with antibiotic-free diets supplemented with MF gained more weight and were healthier by modifying the gut microbial composition (9). CC is a Chinese herbal medicine with strong anti-inflammatory activity, and has obvious clinical medicinal value. Berberine, an isoquinoline alkaloid, mainly found in CC, with antibacterial effects on Shigella and Escherichia coli, is believed to exert gut health-promoting effects through modulation of the gut microbiota (10). CL has been used as a traditional Chinese medicinal material to treat gastrointestinal diseases for many years (11). TC is a widely used herbal drug in traditional medicine prescriptions. Chebulinic acid, a phenolic compound found in TC, is reported to exhibit both anti-inflammatory, anti-oxidant activity and anti-tumor property (12). DK is a popular cultivated and consumed fruits in China. Clinical studies showed that DK can help the gastrointestinal tract to digest and promote the recovery of appetite after diarrhea in sick pigs (13). The prescription of the compound WMS includes 15 g of MF, 24 g of DK, 6 g of CC, 6 g of CL, and 9 g of TC. The above five herbs are evenly combined, crushed, sieved, and combined. In this prescription, MF plays the role of promoting fluid production to quench thirst and astringing intestines to treat diarrhea as the main drug. TC and DK, as the auxiliary drugs, work through astringing intestines and consolidating. CL and CC are the assistant medicinal. CC takes effect of clearing heat and removing the toxin, and drying dampness to treat diarrhea. CL moves qi and activate blood to relieve pain. However, the mechanism of WMS is still unclear.
The multi-component, multi-target, and multi-channel characteristics of Chinese traditional veterinary medicine have made it difficult to elucidate the complex mechanisms, leading to a lack of data in pharmacological research. Network pharmacology has created a new framework for investigating how medications and disorders interact (14, 15).
In this study, network pharmacology and molecular docking were applied to examine the potential mechanism of WMS in the treatment of PD. The study overview is shown in Figure 1.
Methods
Active components and target gene analysis of WMS
The formula of WMS containing MF, CC, CL, TC, and DK was obtained from Chinese Veterinary Pharmacopeia (2020 edition) II. The Traditional Chinese Medicine Systematic Pharmacology (TCMSP) (https://www.tcmspw.com/tcmsp.php) database, which is a systems pharmacology resource for traditional Chinese medicines or related compounds and offers details on the absorption, distribution, metabolism, and excretion properties of a drug with potential biological effects at a systematic level, was used to identify the compounds in the herbs of MF, CC, CL, TC, and DK. Oral bioavailability (OB) and drug likeness (DL) are two essential characteristics of medications taken orally. OB and DL can assess the effectiveness of a drug's systemic circulation, and the resemblance between a molecule and a known medication, respectively. The putative active components were screened using OB and DL in this research. Active substances were identified from prior investigations and data from key databases of Chinese herbal medicine. Active compounds were then screened and considered as putative main constituents and retained using OB ≥30 % and DL ≥0.18 based on data from prior studies and pertinent Chinese herbal medicine databases (16, 17). For example, an herb name was entered into the search box, and the compounds in the herb were examined. The potential active compounds were obtained then by setting OB ≥30 % and DL ≥0.18. The primary functional components for the treatment of PD that are not found in TCMSP were sorted out by examining previous studies (18, 19). Active compounds were input into the TCMSP database to obtain known targets. The structures of the active compounds were obtained from PubChem (https://pubchem.ncbi.nlm.nih.gov/) and imported into the SwissTargetPrediction database with relevant parameters for prediction of target genes (probability > 0). The potential targets were then collected. The target genes corresponding to the compounds were uniformly standardized in UniProt (http://www.uniprot.org/).
Candidate targets of PD
“Piglet diarrhea” was used as the keyword to explore disease-related genes at GeneCards (https://www.genecards.org/), OMIM (https://www.omim.org/), and NCBI (https://www.ncbi.nlm.nih.gov/gene/). All potentially relevant genes were obtained. All the disease gene targets were then normalized and converted into gene symbols through UniProt database after removing the redundancy.
Venn Diagram analysis
The predicted target genes of WMS and the projected target genes of PD were analyzed by Venn Diagram analysis (https://www.bioinformatics.com.cn/?keywords=%E6%96%87%E6%81%A9%E5%9B%BE).
Construction of a network of herbs, natural compounds, and targets
From the above data, a network of herbs, active compounds, and common targets was constructed using Cytoscape (v 3.9.1), and the relationships among them were analyzed. Herbs, compounds, and targets were represented by nodes in the network, and their interactions were represented by edges connecting nodes.
Construction of the protein—Protein interaction network
The potential target genes of WMS in PD were uploaded to STRING database (https://string-db.org/) (v 11.5) to draw a protein—Protein interaction (PPI) network. The organism was limited to “Sus scrofa.” The results showed the confidence of the interaction between the proteins by scores. The minimum required interaction score was selected at medium confidence data >0.4 to ensure the reliability of the analysis. The data from STRING database were then analyzed by the “Analysis Network” tool in Cytoscape 3.9.1 software to obtain a PPI network. The relevant parameters of degree (DC), betweenness centrality (BC), closeness centrality (CC), and stress were calculated for topology analysis on the PPI network to obtain the key targets.
GO and KEGG pathway enrichment analyses
The potential targets from the intersection were imported into DAVID database (2021) (https://david.ncifcrf.gov/tools.jsp) for Gene Ontology (GO) and the Kyoto Encyclopedia of Genes and Genomes (KEGG) enrichment analysis with “Sus scrofa” as the species. P ≤ 0.01 was considered to be significantly enriched. The top 10 most significantly enriched GO biological processes (BP), cell component (CC), and molecular function (MF) and top 20 items of KEGG pathway results were then mapped (https://www.bioinformatics.com.cn/?keywords=pathway) to draw the enrichment bubble diagram.
Construction of a network of herbs, compounds, pathways, and targets
The interaction information between intersection targets and the top 20 most significantly enriched KEGG pathways were combined with the screened drug components and intersection targets. Data were then uploaded into Cytoscape 3.9.1 to construct a network of herbs, active compounds, target, and pathway.
Molecular docking
The 10 important compounds in accordance with the degree values in WMS were selected to dock to the top 10 core targets from the PPI analysis. The three-dimensional crystal structure of the target protein was downloaded from the RCSB database (https://www.rcsb.org/) and saved in pdb format after removing solvent and organic through the PyMol software. The 2D chemical structure of the compounds was obtained from PubChem and converted to mol2 format after minimizing energy through Chem3D 18.0. The ligand and receptor were then converted to pdbqt file format through AutoDockTools-1.5.7. Molecular docking was performed by AutoDock Vina 1.1.2. A binding energy of <-4.25 kcal/mol indicates that the ligand and receptor molecules bind spontaneously. The binding energy of <-5.0 kcal/mol indicates that ligand and receptor molecules are stably bound. The binding energy of <-7.0 kcal/mol indicates that the two have strong binding activity. Results with strong binding force were selected and visualized by Pymol software.
Results
The natural active ingredients in WMS
Using the databases, previous reports, and the criteria (OB ≥30%; DL ≥0.18) described above, the compounds of WMS were screened out. After the removal of non-target compounds, 8, 14, 3, 8, and 4 natural compounds of TC, CC, CL, MF, and DK were obtained, respectively. After removing any duplicate compounds, a total of 34 active compounds in the WMS formula were obtained (Table 1).
Targets of the effective compounds of WMS
TCMSP, PubChem, and SwissTargetPrediction databases were used to predict the target genes of compounds in WMS. The results identified 638 targets, including 325, 447, 58, 75 and 271 targets for TC, CC, CL, DK and MF, respectively.
From the GeneCards website and OMIM, 352 genes were determined as highly likely to be associated with PD. The 352 candidate PD-associated genes were compared with the 638 target genes from WMS using Venn diagram analysis (Figure 2A). A total of 94 (11%) overlapping genes were extracted. The intersection between PD, CC, TC, CL, DK and MF which contains 3 common genes (Figure 2B).
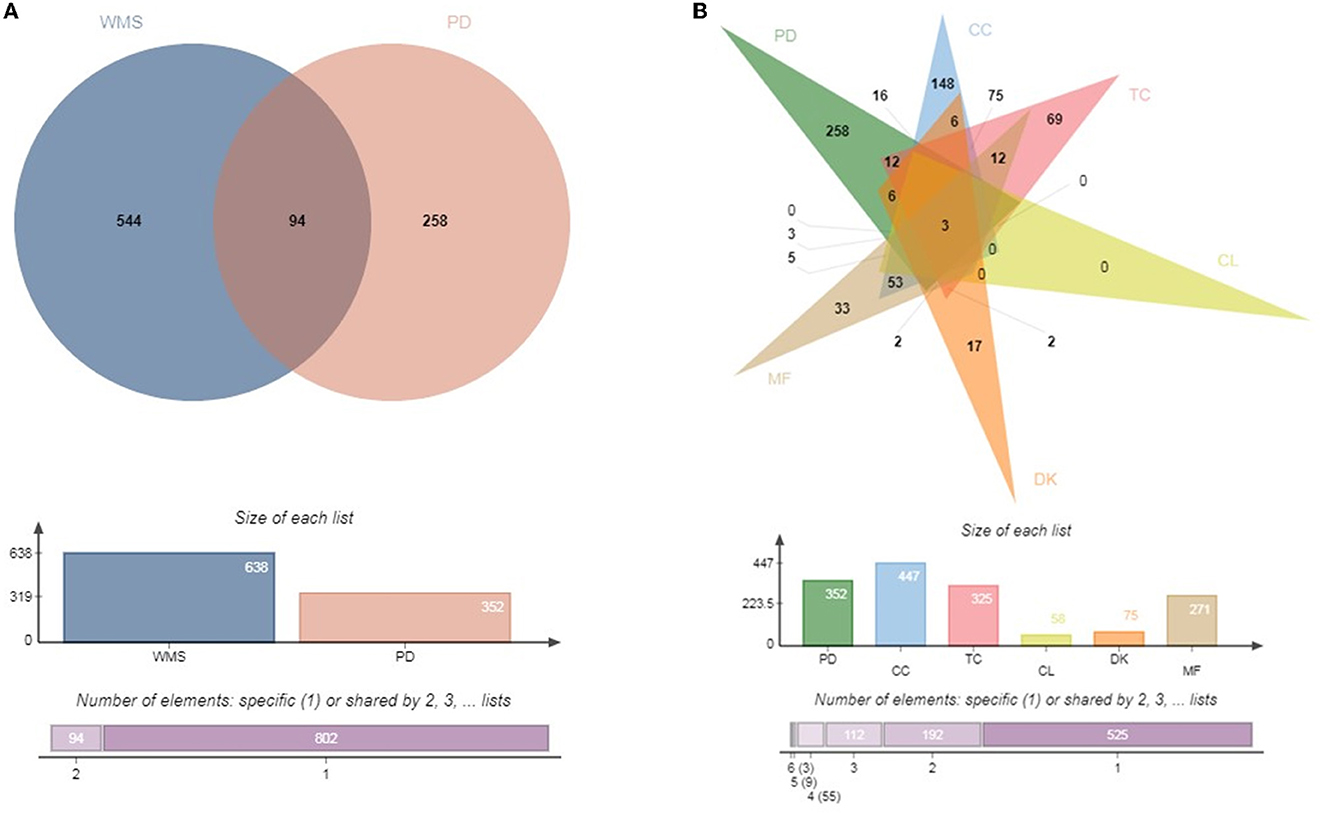
Figure 2. Venn diagram. (A) Common target genes of WMS and PD. (B) Common target genes of CC, TC, CL, MF, DK, and PD.
Construction of a network of herbs, natural compounds, and targets
From the above data, a network of the complex interactions among components of WMS, effective compounds, and target genes was visualized using Cytoscape; the network comprised 133 nodes and 393 edges (Figure 3). The top 10 compounds by degree value are (2R)-5,7-dihydroxy-2-(4-hydroxyphenyl) chroman-4-one, moupinamide, epiberberine, sennoside E_qt, cheilanthifoline, quercetin, berlambine, obacunone, kaempferol, and 7-dehydrosigmasterol (Table 2). These compounds may be the core compounds of WMS responsible for anti-PD effects. This network allowed for easy observation of relationships among herbs, ingredients, and targets. These findings suggested that the pharmacological effects of WMS in the treatment of PD are the result of multi-component and multi-target effects.
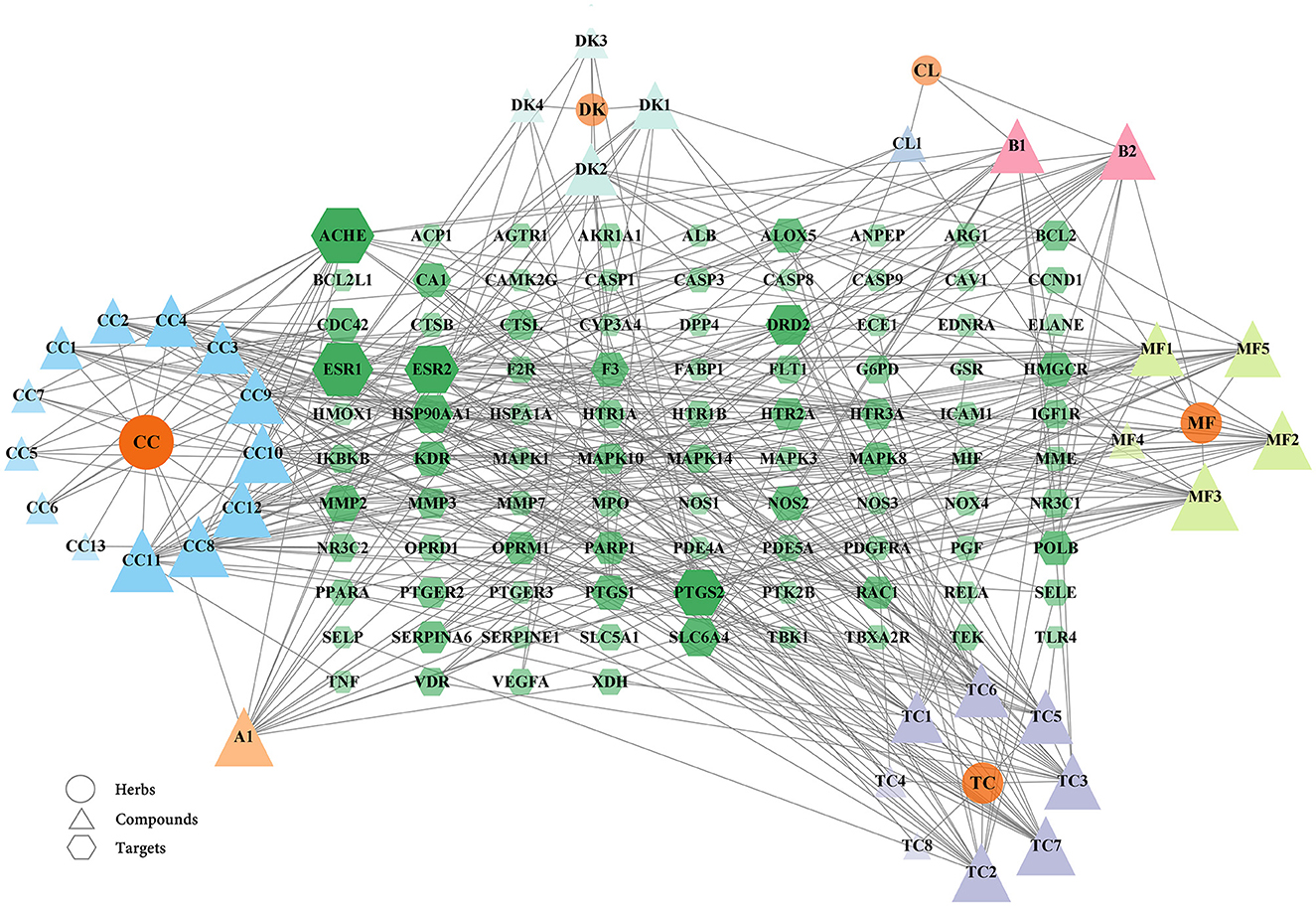
Figure 3. The herb-compound-disease target network. The ellipse nodes, the triangle nodes, and the hexagon nodes represent the herbs, compounds, and targets, respectively. The connections represent the interactions among the three. The node size and color are related to the degree value. The larger the node area and the darker the color in the figure, the more important it is in the network.
Construction and analysis of the target PPI network
The 94 intersection genes were uploaded to the STRING database, and a PPI network was obtained depicting the BP of WMS treatment of PD in vivo. The network comprised 90 nodes and 607 edges. The results were then imported into Cytoscape to construct a network diagram.
The PPI network and relevant parameters were obtained with the Analysis Network tool in Cytoscape software. Using the four parameters DC, BC, CC and Stress, the indicators above the median value were selected as the key indicators, and two screenings were performed. The critical values of the first screening were DC >11, CC >0.4623, BC >0.0032, and Stress >255. Through topological analysis, 30 key targets were obtained and screened again. The screening criteria were DC >15, CC >0.6744, BC >0.0093, and Stress >60. Finally, a total of 14 key targets of WMS acting on PD were obtained. The specific screening strategy is shown in Figure 4.
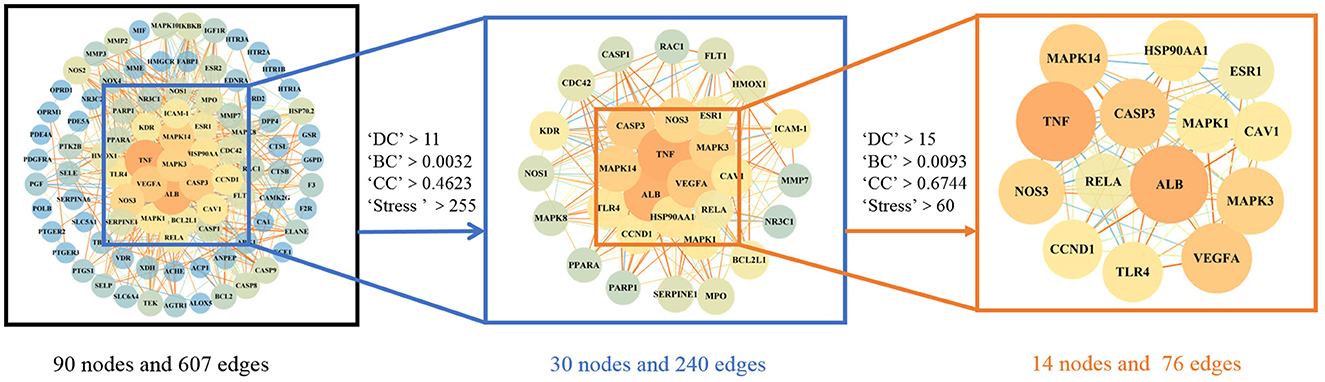
Figure 4. The topology screening process of the protein interaction network. The node size and color were related to the degree value. The color and size of the node are adjusted in accordance with the degree value. The darker colors and larger nodes indicate a larger degree value.
GO and KEGG pathway enrichment analyses
After inputting common targets into the DAVID 2021 database, 145 KEGG pathways, 184 GO BP, 31 GO cell components and 57 GO molecular functions that met the enrichment criteria of P < 0.01 were identified.
The top 10 most significantly enriched GO BP were selected for analysis (Figure 5A). The major biological processes enriched were response to hypoxia, positive regulation of interleukin-8 production, inflammatory response, positive regulation of angiogenesis, and vascular endothelial growth factor receptor signaling pathway. The major molecular functions were MAP kinase activity, steroid binding, protein homodimerization activity, protein kinase binding, and protein homodimerization activity. The cellular components were mainly enriched in the perinuclear region of cytoplasm, caveola, extracellular space, receptor complex, and membrane.
The top 20 most significantly enriched KEGG pathways were selected for analysis (Figure 5B). The potential target genes of WMS in PD were involved in the AGE-RAGE signaling pathway, PI3K-Akt signaling pathway, TNF signaling pathway, NOD-like receptor signaling pathway, and IL-17 signaling pathway.
Using the herb, compound, target, and pathway analyses, an entire herb, chemical, target and pathway network was then constructed by Cytoscape using the top 20 signaling pathways. As shown in Figure 6, the interaction network has 114 nodes and 593 edges. The results showed that 55 of the 94 potential targets were involved in the top 20 pathways. These findings indicate that WMS has multi-component, multi-target, and multi-channel effects in the treatment of PD.
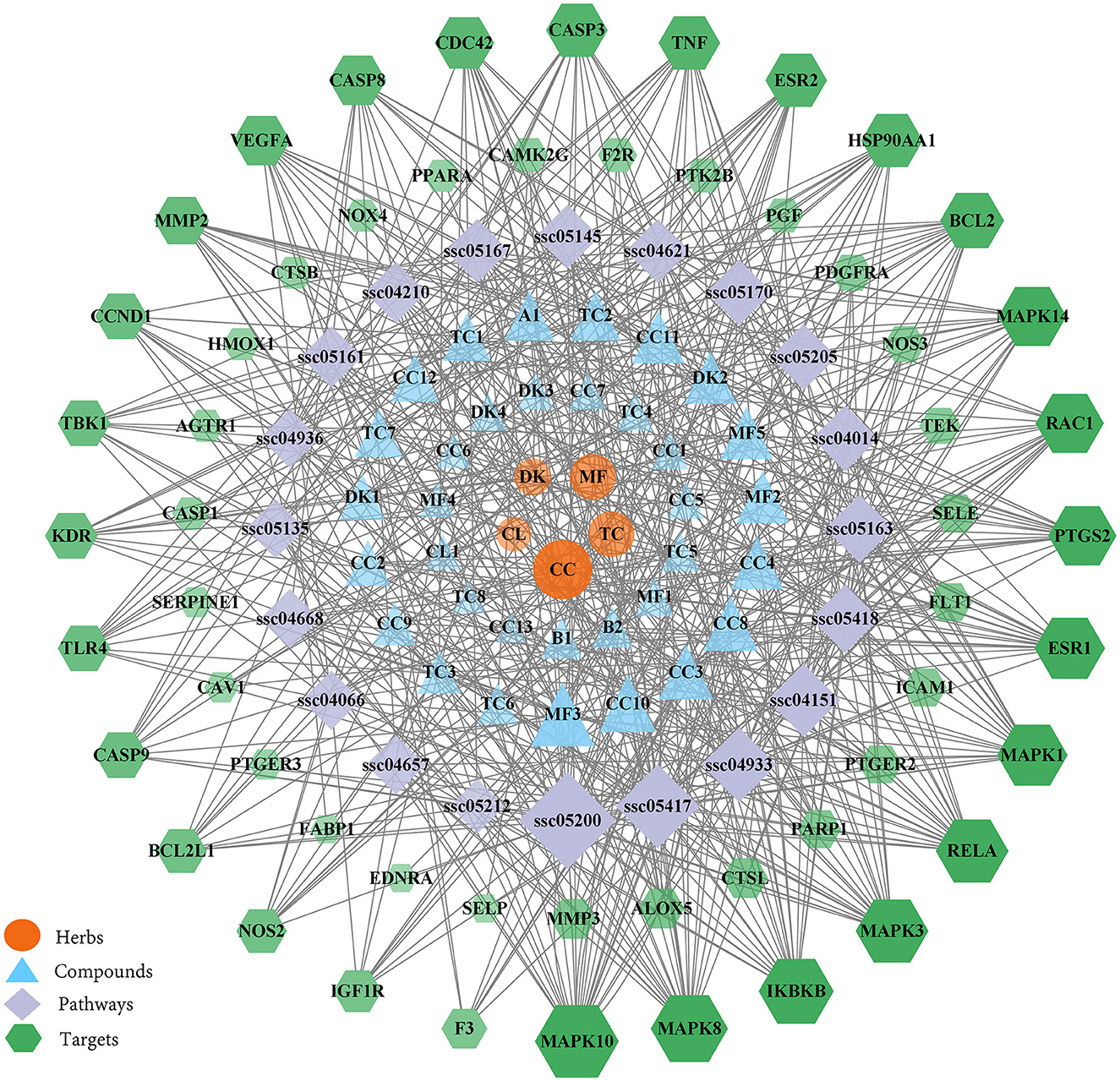
Figure 6. The herb-compound-disease target-pathway network. The orange ellipse, blue triangle, purple diamond, and green hexagon correspond to herbs, compounds, pathways, and target genes, respectively.
Molecular docking
From the degree analysis in the network, the 10 main active compounds (2R)-5,7-dihydroxy-2-(4-hydroxyphenyl)chroman-4-one (MF3), epiberberine (CC8), moupinamide (CC11), berlambine (CC10), sennoside E_qt (TC2), quercetin (A1), obacunone (CC12), kaempferol (MF2), cheilanthifoline (TC7), and 7-dehydrosigmasterol (TC3) were chosen for molecular docking verification with the 10 core targets MAPK14, CASP3, ESR1, MAPK3, VEGFA, TNF, CCND1, HSP90AA, CAV1, and NOS3. The statistical results of binding energy are shown in Figure 7.
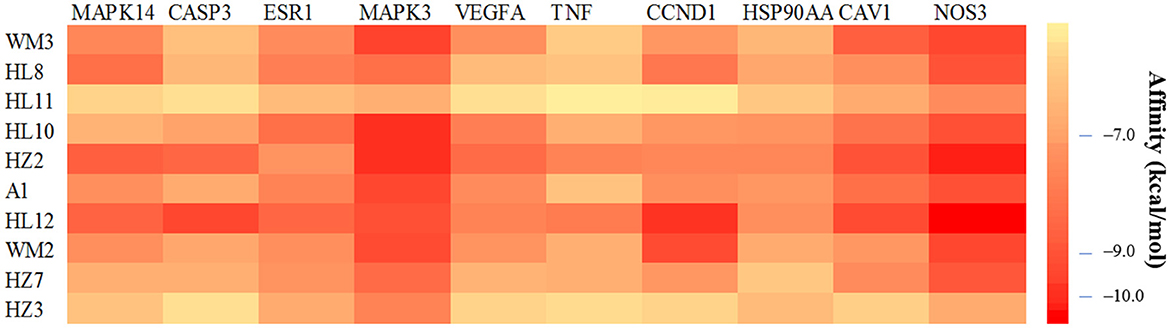
Figure 7. Molecular docking heat map. A darker color indicates a stronger binding force between the ligand molecule and the receptor protein.
Among the 100 receptor-ligand docking combinations, the binding energy of all molecules to proteins was <−5.0 kcal/mol, and 62 groups (62% of all combinations) had binding energy <−7.0 kcal/mol, which indicated that the main active ingredients in WMS have a strong binding activity with the core targets. The affinity of the compound obacunone to target NOS3 had the lowest binding energy of −11.1 kcal/mol. The binding modes of some key targets to the core active compounds are shown in Figure 8.
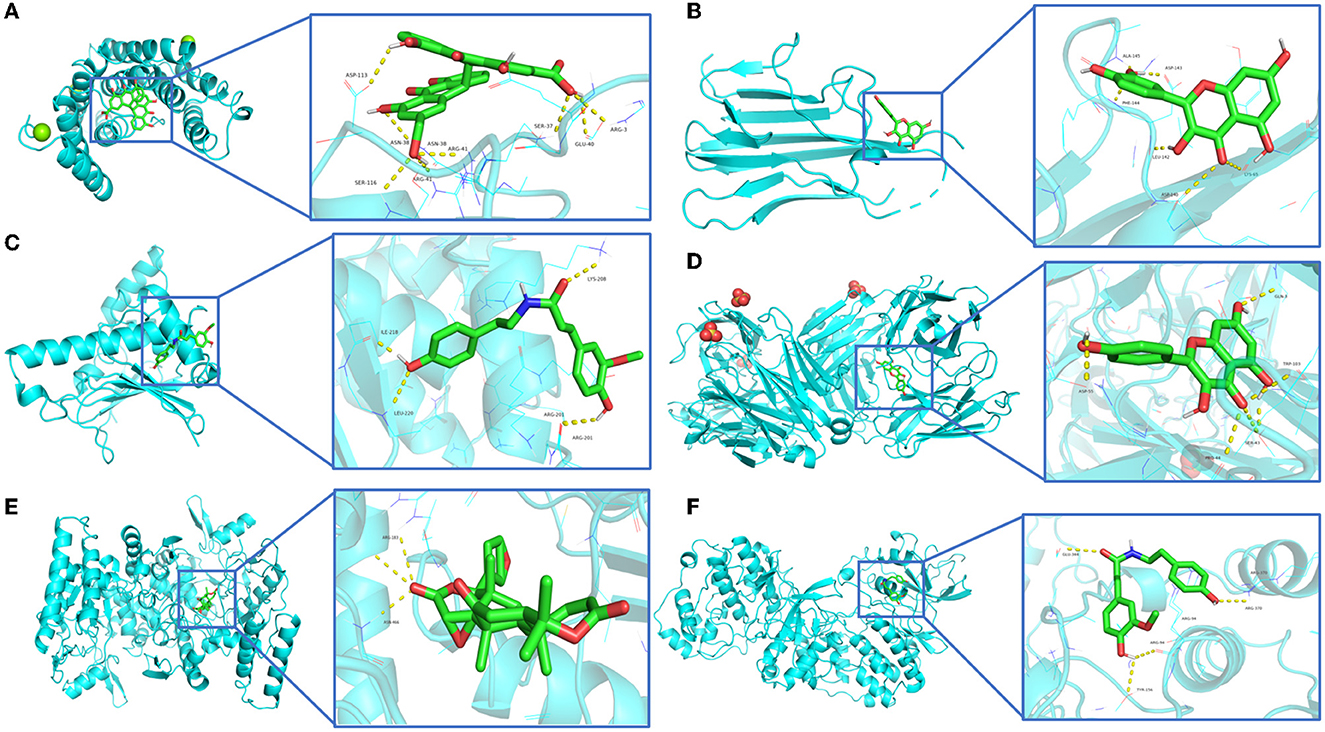
Figure 8. Molecular and key target docking verifications. (A) Sennoside E_q-ESR1, (B) Quercetin-TNF, (C) Moupinamide-HSP90AA1, (D) Kaempferol-CAV1, (E) Obacunone-NOS3, (F) Moupinamide-MAPK3.
Discussions
PD is a common disease in pig farms with a high morbidity and mortality that leads to productivity loss. Antibiotics and medical zinc oxide are the main treatment strategies for PD in pig production (20). However, long-term medication and a high zinc diet leads to adverse effects on human health and the environment by contributing to the development of antimicrobial resistance among bacteria and high zinc levels in soil. Therefore, the identification of a natural drug for PD prevention and treatment is critical. Some traditional Chinese medicines and formulations have been found to be effective in the treatment of PD. The WMS formula, which is composed of MF, CC, CL, DK, and TC, has the functions of regulating metabolism and enhancing immune effects. WMS has been clinically used in the treatment of PD in China and has a significant effect. However, the mechanism has been unclear.
In this study, network pharmacology was applied to analyze the effective components and mechanisms of WMS in the treatment of PD. A total of 32 active compounds in the WMS formula were obtained and 10 core compounds were identified. The compounds included terpenoids, flavonoids, alkaloids, and tannins. Flavonoids have the effects of reducing ulcerative colitis and anti-gastric ulcers, improving functional dyspepsia, inhibiting ileal motility, protecting gastric mucosa, and exerting antibacterial and antiviral effects (21). Quercetin is a flavonoid polyphenol molecule commonly found in vegetables, fruits, and Chinese herbal medicines; it promotes the reconstruction of epithelial tight junctions, enhances barrier integrity, and inhibits the production of proinflammatory cytokines such as IL17, TNF-α, and IL6 (22). Some of its metabolites reduce inflammation and prevent colitis (23). Previous studies showed that adding quercetin to the diet of weaned piglets increases the antioxidant capacity of piglets, regulates the structure and metabolism of intestinal microorganisms, and alleviates diarrhea and intestinal injury (24). Kaempferol is a polyphenol that is widely distributed in many vegetables, fruits, and beans. It has various pharmacological activities such as antiviral, apoptosis, anti-inflammation, and anti-oxidation effects (25). These effects may be related to kaempferol's inhibition of oxidative stress and attenuation of inflammatory factors, such as tumor necrosis factor-alpha (TNF-α), interleukin-6 (IL-6), cyclooxygenase-2 (COX-2), and nuclear factor κB (NF-κB) and the modulation of apoptosis and mitogen-activated protein kinase (MAPK) signaling pathways (26). Berberine is an alkaloid that reduces the expression of TNF-α, IL1β, and IL8 genes and the occurrence of intestinal inflammation through inhibiting the expression of TLR4 and NOD1 genes in intestinal mucosa (27, 28). Epiberberine is an isomer of berlambine; it exhibits anti-adipogenesis effects by modulating the Akt and ERK pathways, anti-dyslipidemia effects by inhibition on cholesterol synthesis, anti-cancer effects by impacting the p53/Bax apoptosis pathway, and antibacterial activities (29). 7-dehydrosigmasterol is a sterol compound. Phytosterols exhibit anti-inflammatory activities, improve the immunity of weaned piglets, and reduce the rate of PD (30). Epicatechin is a natural plant tannin compound that exerts anti-inflammatory effects by reducing the secretion of inflammatory cytokines and inhibiting the phosphorylation of P38 MAPK, extracellular signal-regulated kinase (ERK), and c-jun N-terminal kinase protein (JNK) in the MAPK signaling pathway (31). Supplementation of some tannins may help prevent PD in 21-day-old weaned piglets (32). These compounds form the material basis of the mechanism of action of WMS on PD, and further research is necessary to elucidate the detailed mechanisms.
The mapped PPI network was analyzed and 14 core targets were identified: MAPK14, HSP90AA1, TNF, NOS3, RELA, CCND1, TLR4, VEGFA, MAPK3, CAV1, MAPK1, ESR1, CASP3, and ALB. Intestinal inflammation is one of the main internal causes of piglet diarrhea, and the expression of the intestinal cytokines is one of the main characteristics of intestinal inflammatory response. Previous studies showed that, the gene expression of IL-6, TNF-α and IL-β in jejunum of piglets were increased (33, 34). The weaning stress may activate MAPK signaling pathways, NF-κB pathway and other pathways in the intestine (35, 36). Bacteria and lipopolysaccharide invade the intestinal mucosa of weaned piglets, activate the intestinal inflammatory signaling pathway, promote the transcription of downstream inflammatory factors, and cause intestinal inflammation in piglets. As can be seen from the Figure 3, the 14 core targets are the targets of the compounds in WMS that can directly act on PD. TLR4 corresponds to peraksine; MAPK14 corresponds to magnograndiolide, berberine, obacunone, and methyl arachidonate; VEGFA corresponds to (2R)-5,7-dihydroxy-2-(4-hydroxyphenyl)chroman-4-one, procyanidin B1 and β-carotene; TNF corresponds to obacunone; RELA corresponds to palmidin A; CAV1 corresponds to β-carotene. The results showed the characteristics of multi-component and multi-target effects of WMS in the treatment of PD. TNF is a critical cytokine with a wide range of sources. TNF-α is an indispensable immunomodulatory factor that maintains internal stability and functions in the resistance against various pathogenic factors. It has dual biological activities. At low concentrations, TNF-α is involved in resisting pathogenic microbial infection, promoting tissue repair, and regulating the inflammatory response (37). The initial line of defense against diarrhea is comprised of intestinal epithelial cells. TNF-α can induce epithelial cell apoptosis and destroys intestinal barrier function by rearranging the adhesion proteins in intestinal epithelial cells (38). The expression of NHE3 and DRA, the main transporter proteins regulating colonic Na+ inward transport and the main transporter protein regulating Cl−/ exchange in the apical membrane of mammalian intestinal epithelial cells, respectively, can be inhibited by the rising TNF-α expression in ulcerative colitis (39, 40). RELA is a member of the NF-κB family. Abnormal inflammation associated with inflammatory bowel disease is caused by excessive activation of RELA/NF-κB (41). MAPK14, also named P38α, plays an important role in the normal immune and inflammatory responses. The MAPK14 pathway plays a role in inflammatory bowel disease (42). TLR4 maintains immune tolerance and intestinal homeostasis, and inflammation is alleviated by TLR4 regulating the TLR4/NF-κB pathway (43, 44). CAV1 is a multifunctional protein, with roles in cellular defense by its inhibition of nitrosative stress and mucosal barrier injury (45). VEGFR is a receptor that binds to VEGF and initiates a signaling cascade that stimulates angiogenesis. A lack of VEGFR in newborn animals leads to intestinal microvascular dysplasia and colitis (46). Epidermal growth factor can promote the development of intestinal mucosal morphology in weaned piglets, activate gastric and intestinal digestive enzymes and disaccharidase, and improve weaning stress (47). We speculate that WMS exerts its effects on PD by acting on MAPK14, TNF, RELA, TLR4, VEGFA, CAV1, and other factors to regulate the inflammatory response, maintain the intestinal mucosal barrier, and improve stress.
GO enrichment analysis showed that WMS may play a therapeutic role by regulating the immune response of piglets, modulating cell growth, and regulating apoptosis. PD is caused by impaired intestinal barrier function, disruption of intestinal flora homeostasis, and disturbances in intestinal chemical, mechanical, and immune barriers (48, 49). The impaired intestinal barriers may promote bacterial translocation and the entry of allergic compounds from the intestine into the body, leading to increased immune response and susceptibility. GO enrichment analysis showed that the active ingredients of WMS exert activities on cell components and structures such as the cytoplasm, caveola, cell surface, extracellular space, receptor complex, and membrane, which are related to the maintenance of intestinal epithelial tissue integrity. Thus, WMS may prevent intestinal barrier damage and the entry of sensitive substances such as bacteria from the intestine into the body.
PD progresses through a variety of pathophysiological processes, including stimulation of intestinal structural damage, digestive dysfunction, oxidative stress, and inflammation response. KEGG pathway enrichment analysis showed that the pharmacological effects of WMS in treating PD included effects on the AGE-RAGE signaling pathway, PI3K-Akt signaling pathway, TNF signaling pathway, NOD-like receptor signaling pathway, and IL-17 signaling pathway. As can be seen from the Figure 6, the compounds berberine, moupinamide, palmidin A, obacunone, β-carotene, catechin, and methyl arachidonate in WMS modulate the AGE-RAGE signaling pathway by regulating MAPK14, TNF, VEGFA, RELA and other targets to treat piglets. Peraksine, β-carotene, catechin, and (2R)-5,7-dihydroxy-2-(4-hydroxyphenyl)chroman-4-one modulate the PI3K-Akt signaling pathway by regulating the TLR4, VEGFA, and other targets. Berberine, ellagic acid, sennoside E_qt, quercetin, chebulic acid, β-carotene, catechin, and epicatechin modulate the TNF signaling pathway, IL-17 signaling pathway and NOD-like receptor signaling pathway by regulating TNF, MAPK14, RELA, MMP3, CASP8, PTGS2, and other targets to treat PD. The AGE-RAGE signaling pathway elicits activation of multiple intracellular signal pathways involving NADPH oxidase, protein kinase C, and MAPKs, then resulting in NF-κB activity. NF-κB promotes the expression of pro-inflammatory cytokines such as IL-1, IL-6, and TNF-α (50). TNF induces a wide range of intracellular signal pathways including apoptosis, cell survival, inflammation, and immunity pathways. TNF-α is an indispensable immunomodulatory factor and an important downstream factor of the NF-κB pathway. When the intestinal epithelial cells was attacked by transmissible gastroenteritis virus, adding leucine can inhibit the expression of the pro-inflammatory factor TNF-α and anti-inflammatory factor IL10 by inhibiting the phosphorylation level of NF-κB, thus reducing the inflammatory response in the small intestine (51). The PI3K-Akt signaling pathway is activated by many types of cellular stimuli or toxic insults and regulates fundamental cellular functions such as transcription, translation, proliferation, growth, and survival. Previous studies showed that flavonoids ameliorate dysregulated inflammatory responses, the intestinal barrier, and gut microbiome in ulcerative colitis via the PI3K-AKT pathway (52). IL17 is associated with bacterial infection, and γδT cells secreting IL17 are the key component of the mucosal defense against infection (53). NOD-like receptors are representatives of mediating inflammatory responses. After activation, they can induce the release of inflammatory factors by mediating the NF-κB pathway and MAPK pathway (54). Together these results indicate that the AGE-RAGE signaling pathway, PI3K-Akt signaling pathway, TNF signaling pathway, IL-17 signaling pathway, and other pathways may play key roles in the effects of WMS on PD.
Conclusion
WMS is a traditional Chinese medicine formula that is effective in treating PD. In this study, network pharmacology combined with molecular docking was applied to explore the mechanism underlying the effect of WMS on PD. The results indicated that WMS may exert its anti-PD activities through the TNF signaling pathway and IL-17 signaling pathway. The AGE-RAGE signaling pathway, PI3K-Akt signaling pathway, and NOD-like receptor signaling pathway may also be involved. Further experiments are required to elucidate the precise mechanism by which WMS functions in PD.
Data availability statement
The original contributions presented in the study are included in the article/supplementary material, further inquiries can be directed to the corresponding author.
Author contributions
HHY wrote the manuscript. HHY, WL, XYJ, and GQY designed the figures and edited the manuscript. HHY supervised data analysis and manuscript editing, in cooperation with XYZ, WZ, and YHW. All authors contributed to the article and approved the submitted version.
Funding
This work was supported by the Guangxi Science and Technology Bureau Program (No. AA17204057) and the Guangxi Fundamental Research Funds (No. 20-5).
Acknowledgments
We thank Gabrielle White Wolf, from Liwen Bianji (Edanz) (www.liwenbianji.cn) for editing the English text of a draft of this manuscript.
Conflict of interest
The authors declare that the research was conducted in the absence of any commercial or financial relationships that could be construed as a potential conflict of interest.
Publisher's note
All claims expressed in this article are solely those of the authors and do not necessarily represent those of their affiliated organizations, or those of the publisher, the editors and the reviewers. Any product that may be evaluated in this article, or claim that may be made by its manufacturer, is not guaranteed or endorsed by the publisher.
References
1. Kumar D, Shepherd FK, Springer NL, Mwangi W, Marthaler DG. Rotavirus infection in swine: genotypic diversity, immune responses, and role of gut microbiome in rotavirus immunity. Pathogens. (2022) 11:1078. doi: 10.3390/pathogens11101078
2. Jacobson M. On the infectious causes of neonatal piglet diarrhoea-a review. Vet Sci. (2022) 9:422. doi: 10.3390/vetsci9080422
3. Kong QH, Zhang WQ, An M, Fakhar-e-Alam Kulyar M, Shang ZD, Tan ZK, et al. Characterization of bacterial microbiota composition in healthy and diarrheal early-weaned Tibetan piglets. Front Vet Sci. (2022) 9:799862. doi: 10.3389/fvets.2022.799862
4. Zhang G, Zhao JB, Lin G, Guo YH Li DF, Wu Y. Effects of protein-chelated zinc combined with Mannan-rich fraction to replace high-dose zinc oxide on growth performance, nutrient digestibility, and intestinal health in weaned piglet. Animals-Basel. (2022) 12:3407. doi: 10.3390/ani12233407
5. Jakobsen N, Goecke NB, Pedersen KS. Evaluation of the diagnostic performance of a commercially available point-of-care test for post weaning diarrhoea in pigs-a pilot study. Porc Health Manag. (2022) 8:49. doi: 10.1186/s40813-022-00292-9
6. Xu QL, Cheng M, Jiang R, Zhao XL, Zhu JJ, Liu MZ, et al. Effects of dietary supplement with a Chinese herbal mixture on growth performance, antioxidant capacity, and gut microbiota in weaned pigs. Front Vet Sci. (2022) 9:971647. doi: 10.3389/fvets.2022.971647
7. Li Y, Sun TH, Hong YX, Qiao T, Wang YS Li W, et al. Mixture of five fermented herbs (Zhihuasi Tk) alters the intestinal microbiota and promotes the growth performance in piglets. Front Microbiol. (2021) 12:725196. doi: 10.3389/fmicb.2021.725196
8. Kim MS, Bang JH, Lee J, Han JS, Kang HW, Jeon WK. Fructus mume ethanol extract prevents inflammation and normalizes the septohippocampal cholinergic system in a rat model of chronic cerebral hypoperfusion. J Med Food. (2016) 19:196–204. doi: 10.1089/jmf.2015.3512
9. Zhang F, Jin E, Liu XD Ji X, Hu H. Effect of dietary Fructus mume and Scutellaria baicalensis Georgi on the fecal microbiota and its correlation with apparent nutrient digestibility in weaned piglets. Animals. (2022) 12:2418. doi: 10.3390/ani12182418
10. Dehau T, Cherlet M, Croubels S, van Immerseel F, Goossens E. A high dose of dietary berberine improves gut wall morphology, despite an expansion of and a reduction in beneficial microbiota in broiler chickens. mSystems. (2023) 3:e0123922. doi: 10.1128/msystems.01239-22
11. Jafarzadeh E, Shoeibi S, Bahramvand Y, Nasrollahi E, Maghsoudi AS, Yazdi F, et al. Turmeric for treatment of irritable bowel syndrome: a systematic review of population-based evidence. Iran J Public Health. (2022) 51:1223–31. doi: 10.18502/ijph.v51i6.9656
12. Feng XH, Xu HY, Wang JY, Duan S, Wang YC, Ma CM. In vivo hepatoprotective activity and the underlying mechanism of chebulinic acid from Terminalia chebula fruit. Phytomedicine. (2021) 83:153479. doi: 10.1016/j.phymed.2021.153479
13. Ao WP, Liu QJ. Experiment of compound traditional Chinese medicine to prevent piglet diarrhea. Feed Research. (2010) (12):41–3. doi: 10.13557/j.cnki.issn1002-2813.2010.12.014
14. Andrew L, Hopkins. Network pharmacology. Nat Biotechnol. (2007) 25:1110–1. doi: 10.1038/nbt1007-1110
15. Qi SM, Zhang JT, Zhu HY, Wang Z, Li W. Review on potential effects of traditional Chinese medicine on glaucoma. J Ethnopharmacol. (2022) 304:116063. doi: 10.1016/j.jep.2022.116063
16. Shi HS, Dong CD, Wang M, Liu RX, Wang Y, Kan ZQ, et al. Exploring the mechanism of Yizhi Tongmai decoction in the treatment of vascular dementia through network pharmacology and molecular docking. Ann Transl Med. (2021) 9:164. doi: 10.21037/atm-20-8165
17. Ru JL Li P, Wang JN, Zhou W, Li BH, Huang C, et al. TCMSP: a database of systems pharmacology for drug discovery from herbal medicines. J Cheminform. (2014) 6:13. doi: 10.1186/1758-2946-6-13
18. Fukai S, Tanimoto S, Maeda A, Fukuda H, Okada Y, Nomura M. Pharmacological activity of compounds extracted from persimmon peel. J Oleo Sci. (2009) 58:213–9. doi: 10.5650/jos.58.213
19. Smrke T, Persic M, Veberic R, Sircelj H, Jakopic J. Influence of reflective foil on persimmon (Diospyros kaki Thunb) fruit peel colour and selected bioactive compounds. Sci Rep. (2019) 9:19069. doi: 10.1038/s41598-019-55735-1
20. Canibe N, Hojberg O, Kongsted H, Vodolazska D, Lauridsen C, Nielsen TS, et al. Review on preventive measures to reduce post-weaning diarrhoea in piglets. Animals-Basel. (2022) 12:2585. doi: 10.3390/ani12192585
21. Shao J, Li Z, Gao Y, Zhao KR, Lin ML Li YD, et al. Construction of a “bacteria-metabolites” co-expression network to clarify the anti–ulcerative colitis effect of flavonoids of Sophora flavescens Aiton by regulating the “host–microbe” interaction. Front Pharmacol. (2021) 12:710052. doi: 10.3389/fphar.2021.710052
22. Feng W, Zhong XQ, Zheng XX, Liu QP, Liu MY, Liu XB, et al. Study on the effect and mechanism of quercetin in treating gout arthritis. Int Immunopharmacol. (2022) 111:109112. doi: 10.1016/j.intimp.2022.109112
23. Uyanga VA, Amevor FK, Liu M, Cui ZF, Zhao XL, Lin H. Potential implications of citrulline and quercetin on gut functioning of monogastric animals and humans: a comprehensive review. Nutrients. (2021) 13:3782. doi: 10.3390/nu13113782
24. Xu BY, Qin WX, Xu YZ, Yang WB, Chen YW, Huang JC, et al. Dietary quercetin supplementation attenuates diarrhea and intestinal damage by regulating gut microbiota in weanling piglets. Oxid Med Cell Longev. (2021) 2021:6221012. doi: 10.1155/2021/6221012
25. Wu CC, Lee TY, Cheng YJ, Cho DY. The dietary flavonol kaempferol inhibits epstein–barr virus reactivation in nasopharyngeal carcinoma cells. Molecules. (2022) 27:8158. doi: 10.3390/molecules27238158
26. Hosseini A, Alipour A, Baradaran Rahimi V, Askari VR. A comprehensive and mechanistic review on protective effects of kaempferol against natural and chemical toxins: Role of NF-κB inhibition and Nrf2 activation. Biofactors. (2022) 3:1923 doi: 10.1002/biof.1923
27. Tong YL, Liu LP, Wang RL, Yang T, Wen JX, Wei SZ, et al. Berberine attenuates chronic atrophic gastritis induced by MNNG and its potential mechanism. Front Pharmacol. (2021) 12:644638. doi: 10.3389/fphar.2021.644638
28. Chen L, Fan XD, Qu H, Bai RN, Shi DZ. Berberine protects against TNF-α-induced injury of human umbilical vein endothelial cells via the AMPK/NF-κB/YY1 signaling pathway. Evid Based Complement Alternat Med. (2021) 2021:6518355. doi: 10.1155/2021/6518355
29. Liu LQ Li JJ, He Y. Multifunctional epiberberine mediates multi-therapeutic effects. Fitoterapia. (2020) 147:104771. doi: 10.1016/j.fitote.2020.104771
30. Hu QL Li SS, Zhang YW, Zhou Z, Feng J. Phytosterols on growth performance, antioxidant enzymes and intestinal morphology in weaned piglets. J Sci Food Agric. (2017) 97:4629–34. doi: 10.1002/jsfa.8333
31. Mei SH, Chen XM. Investigation into the anti-inflammatory mechanism of coffee leaf extract in LPS-induced Caco-2/U937 co-culture model through cytokines and NMR-based untargeted metabolomics analyses. Food Chem. (2022) 404(Pt A):134592. doi: 10.1016/j.foodchem.2022.134592
32. Ma M, Chambers JK, Uchida K, Ikeda M, Watanabe M, Goda Y, et al. Effects of supplementation with a quebracho tannin product as an alternative to antibiotics on growth performance, diarrhea, and overall health in early-weaned piglets. Animals-Basel. (2021) 11:3316. doi: 10.3390/ani11113316
33. Pié S, Lallès JP, Blazy F, Laffitte J, Sève B, Oswald IP. Weaning is associated with an upregulation of expression of inflammatory cytokines in the intestine of Piglets. J Nutr. (2004) 134:641–7. doi: 10.1093/jn/134.3.641
34. Mei J, Xu RJ. Transient changes of transforming growth factor-beta expression in the small intestine of the pig in association with weaning. Br J Nutr. (2005) 93:37–45. doi: 10.1079/bjn20041302
35. Hu CH, Xiao K, Luan ZS, Song J. Early weaning increases intestinal permeability, alters expression of cytokine and tight junction proteins, and activates mitogen-activated protein kinases in pigs. J Anim Sci. (2013) 91:1094–101. doi: 10.2527/jas.2012-5796
36. Shi HR, Huang XY, Yan ZQ, Yang QL, Wang PF Li SG, et al. Effect of Clostridium perfringens type C on TLR4/MyD88/NF-κB signaling pathway in piglet small intestines. Microb Pathog. (2019) 135:103567. doi: 10.1016/j.micpath.2019.103567
37. Semita IN, Utomo DN, Suroto H, Sudiana IK, Gandi P. The mechanism of human neural stem cell secretomes improves neuropathic pain and locomotor function in spinal cord injury rat models: through antioxidant, anti-inflammatory, anti-matrix degradation, and neurotrophic activities. Korean J Pain. (2023) 36:72–83. doi: 10.3344/kjp.22279
38. Lu W, Wang Y, Zhang Q, Owen S, Green M, Ni T, et al. TNF-derived peptides inhibit tumour growth and metastasis through cytolytic effects on tumour lymphatics. Clin Exp Immunol. (2019) 198:198–211. doi: 10.1111/cei.13340
39. Amin MR, Dudeja PK, Ramaswamy K, Malakooti J. Involvement of Sp1 and Sp3 in differential regulation of human NHE3 promoter activity by sodium butyrate and IFN-γ/TNF-α. Am J Physiol Gastrointest Liver Physiol. (2007) 293:374–82. doi: 10.1152/ajpgi.00128.2007
40. Kumar A, Chatterjee I, Gujral T, Alakkam A, Coffing H, Anbazhagan AN, et al. Activation of nuclear factor-κB by tumor necrosis factor in intestinal epithelial cells and mouse intestinal epithelia reduces expression of the chloride transporter SLC26A3. Gastroenterology. (2017) 153:1338–50. doi: 10.1053/j.gastro.2017.08.024
41. Chawla M, Mukherjee T, Deka A, Chatterjee B, Sarkar UA, Singh AK, et al. An epithelial Nfkb2 pathway exacerbates intestinal inflammation by supplementing latent RelA dimers to the canonical NF-κB module. Proc Natl Acad Sci U S A. (2021) 118:e2024828118. doi: 10.1073/pnas.2024828118
42. Madkour MM, Anbar HS, El-Gamal MI. Current status and future prospects of p38a/MAPK14 kinase and its inhibitors. Eur J Med Chem. (2021) 213:113216. doi: 10.1016/j.ejmech.2021.113216
43. Zhou P, Lai J, Li YY, Deng JZ, Zhao CL, Huang QQ, et al. Methyl gallate alleviates acute ulcerative colitis by modulating gut microbiota and inhibiting TLR4/NF-κB pathway. Int J Mol Sci. (2022) 23:14024. doi: 10.3390/ijms232214024
44. Liu YJ, Yang M, Tang L, Wang FC, Huang SJ, Liu S, et al. TLR4 regulates RORγt+ regulatory T-cell responses and susceptibility to colon inflammation through interaction with Akkermansia muciniphila. Microbiome. (2022) 10:98. doi: 10.1186/s40168-022-01296-x
45. Song YH, Xie FR, Ma SY, Deng GH Li YJ, Nie YQ, et al. Caveolin-1 protects against DSS-induced colitis through inhibiting intestinal nitrosative stress and mucosal barrier damage in mice. Biochem Pharmacol. (2020) 180:114153. doi: 10.1016/j.bcp.2020.114153
46. Yan XC, Managlia E, Liu Shirley XL, Tan XD, Wang X, Marek C, et al. Lack of VEGFR2 signaling causes maldevelopment of the intestinal microvasculature and facilitates necrotizing enterocolitis in neonatal mice. Am J Physiol Gastrointest Liver Physiol. (2016) 310:716–25. doi: 10.1152/ajpgi.00273.2015
47. He LQ, Zhou XH, Wu ZP, Feng YZ, Liu D, Li TJ, et al. Glutamine in suppression of lipopolysaccharide-induced piglet intestinal inflammation: The crosstalk between AMPK activation and mitochondrial function. Anim Nutr. (2022) 10:137–47. doi: 10.1016/j.aninu.2022.03.001
48. Tang XP, Xiong KN, Fang RJ Li MJ. Weaning stress and intestinal health of piglets: a review. Front Immunol. (2022) 13:1042778. doi: 10.3389/fimmu.2022.1042778
49. Su WF, Gong T, Jiang ZP, Lu ZQ, Wang YZ. The role of probiotics in alleviating post-weaning diarrhea in piglets from the perspective of intestinal barriers. Front Cell Infect Microbiol. (2022) 12:883107. doi: 10.3389/fcimb.2022.883107
50. Chen MY, Meng XF, Han YP, Yan JL, Xiao C, Qian LB. Profile of crosstalk between glucose and lipid metabolic disturbance and diabetic cardiomyopathy: Inflammation and oxidative stress. Front Endocrinol. (2022) 13:983713. doi: 10.3389/fendo.2022.983713
51. Adegoke EO, Xue W, Machebe NS, Adeniran SO, Hao W, Chen W, et al. Sodium Selenite inhibits mitophagy, downregulation and mislocalization of blood-testis barrier proteins of bovine Sertoli cell exposed to microcystin-leucine arginine (MC-LR) via TLR4/NF-kB and mitochondrial signaling pathways blockage. Ecotoxicol Environ Saf. (2018) 166:165–75. doi: 10.1016/j.ecoenv.2018.09.073
52. Li CC, Wang L, Zhao JB, Wei YC, Zhai S, Tan M, et al. Lonicera rupicola Hookfet Thoms flavonoids ameliorated dysregulated inflammatory responses, intestinal barrier, and gut microbiome in ulcerative colitis via PI3K/AKT pathway. Phytomedicine. (2022) 104:154284. doi: 10.1016/j.phymed.2022.154284
53. Blanco-Domínguez R. de la Fuente H, Rodríguez C, Martín-Aguado L, Sánchez-Díaz R, Jiménez-Alejandre R, et al. CD69 expression on regulatory T cells protects from immune damage after myocardial infarction. J Clin Invest. (2022) 132:e152418. doi: 10.1172/JCI152418
Keywords: Wumei San, piglet diarrhea, traditional Chinese medicine systematic pharmacology, signal pathway, molecular docking
Citation: Yin HH, Liu W, Ji XY, Yan GQ, Zeng XY, Zhao W and Wang YH (2023) Study on the mechanism of Wumei San in treating piglet diarrhea using network pharmacology and molecular docking. Front. Vet. Sci. 10:1138684. doi: 10.3389/fvets.2023.1138684
Received: 06 January 2023; Accepted: 13 February 2023;
Published: 28 February 2023.
Edited by:
Cristina Carresi, University Magna Graecia of Catanzaro, ItalyReviewed by:
George Grant, University of Aberdeen, United KingdomKeyu Zhang, Shanghai Veterinary Research Institute (CAAS), China
Copyright © 2023 Yin, Liu, Ji, Yan, Zeng, Zhao and Wang. This is an open-access article distributed under the terms of the Creative Commons Attribution License (CC BY). The use, distribution or reproduction in other forums is permitted, provided the original author(s) and the copyright owner(s) are credited and that the original publication in this journal is cited, in accordance with accepted academic practice. No use, distribution or reproduction is permitted which does not comply with these terms.
*Correspondence: Wei Liu, d2VpbGl1XzA3NzFAMTYzLmNvbQ==