- 1China Animal Health and Epidemiology Center, Qingdao, China
- 2College of Veterinary Medicine, Yangzhou University, Yangzhou, Jiangsu, China
Introduction: Three members of Capripoxvirus (CaPV) genus, including lumpy skin disease virus (LSDV), goatpox virus (GTPV), and sheeppox virus (SPPV), are mentioned as notifiable forms by World Organization for Animal Health. These viruses have negatively impacted ruminant farming industry worldwide, causing great economic losses. Although SPPV and GTPV cause more severe clinical disease in only one animal species, they can transfer between sheep and goats. Both homologous and heterologous immunization strategies are used to protect animals against CaPVs. However, development of accurate and rapid methods to distinguish these three viruses is helpful for the early detection, disease surveillance, and control of CaPV infection. Therefore, we developed a novel triplex real-time PCR (qPCR) for the differentiation of LSDV, GTPV, and SPPV.
Methods: Universal primers were designed to detect pan-CaPV sequences. Species-specific minor groove binder (MGB)-based probes were designed, which were labeled with FAM for LSDV, HEX for GTPV, and ROX for SPPV. The sensitivity, specificity, reproducibility, and ability of detecting mixed infections were evaluated for the triplex qPCR. Further, 226 clinical samples of the infection and negative controls were subjected to the triplex qPCR, and the results were verified using PCR-restriction fragment length polymorphism (PCR-RFLP) and sequencing methods for PRO30 gene.
Results: The triplex qPCR could successfully distinguish LSDV, GTPV, and SPPV in one reaction, and the assay sensitivity was 5.41, 27.70, and 17.28 copies/μL, respectively. No cross-reactivity was observed with other viruses causing common ruminant diseases, including des petits ruminants virus, foot-and-mouth disease virus, bluetongue virus, ovine contagious pustular dermatitis virus, infectious bovine rhinotracheitis virus, and bovine viral diarrhea-mucosal disease virus. Inter-and intra-assay variabilities were < 2.5%. The results indicated that the triplex qPCR was highly specific, sensitive, and reproducible. Simulation experiments revealed that this assay could successfully distinguish two or three viruses in case of mixed infections without any cross-reaction. For clinical samples, the results were completely consistent with the results of PCR-RFLP and sequencing. This demonstrated that the assay was reliable for clinical application.
Discussion: The triplex qPCR is a robust, rapid, and simple tool for identifying various types of CaPV as it can successfully distinguish LSDV, GTPV, and SPPV in one reaction. Furthermore, the assay can facilitate more accurate disease diagnosis and surveillance for better control of CaPV infection.
1. Introduction
Lumpy skin disease virus (LSDV), goatpox virus (GTPV), and sheeppox virus (SPPV) are members of the genus Capripoxvirus (CaPV). Symptoms of CaPV infection mainly include fever, enlarged lymph nodes, and nodules (or papules) on the skin and mucous membrane (1, 2). Sometimes, CaPV infection can lead to death; particularly GTPV and SPPV infections may cause 100% mortality in fully susceptible breeds of sheep and goats (2). Moreover, it can lead to economic losses due to the performance degradation in production, such as decreased milk yield, weight loss, skin damage, abortion in female animals, and temporary or permanent infertility in male animals. Due to the economic importance of cattle, sheep, and goat farming industries and potential of rapid transboundary spread of CaPVs, all CaPVs are in notifiable form as per World Organization for Animal Health (WOAH).
Previously, lumpy skin disease (LSD) mainly prevailed in Africa, Middle East, and south-eastern Europe (3, 4). From 2019 to 2021, LSD has spread rapidly in continental Asia, invading China, India, Vietnam, Thailand, Malaysia, and Mongolia. Prevention and control of LSD have been under focus in China and other Asian countries. Goatpox (GTP) and sheeppox (SPP) are widespread throughout Northern and Central Africa, Middle East, Central Asia, East Asia, and Southeast Asia (5). In China, GTP and SPP have been persistent epidemics in some provinces for years (6, 7).
Outbreaks of LSDV, GTPV, and SPPV have been occurring worldwide and have caused significant economic losses. LSDV mainly infects cattle and do not infect and transmit between sheep and goats (1). Strains of SPPV and GTPV can pass between sheep and goats, although most strains cause more severe clinical disease in only one animal species (2, 4). Control and eradication of CaPVs depend on vector control, early detection of outbreaks, restrictions on animal movement, and vaccination (8). Live attenuated vaccines are commercially available and mostly used to control CaPV infections. As the genomes of these three viruses are highly homologous, both homologous and heterologous immunization strategies are used in various countries (3). However, the protection conferred by heterologous vaccines for LSDV is not as effective as that conferred by homologous vaccines in animals (9–12). Thus, early detection and clear differentiation of these three viruses is important for selecting and implementing targeted prevention and control strategies. In the current study, we developed a novel triplex real-time quantitative PCR (qPCR) assay for sensitive, specific, robust, and fast differentiation of LSDV, GTPV, and SPPV in one reaction.
2. Materials and methods
2.1. Viruses and clinical samples
Lumpy skin disease virus/China/XJ/2019-1 and peste des petits ruminants virus (PPRV/China/2013) were isolated and identified at China Animal Health and Epidemiology Center (CAHEC). GTPV vaccine (AV41 strain), foot-and-mouth disease virus bivalent vaccine (FMDV Re-O/MYA98/JSCZ 2013 and Re-A/WH/09 strains), infectious bovine rhinotracheitis virus and bovine viral diarrhea-mucosal virus mixed vaccine (BVDV/NMG and IBRV/LY strains), and ovine contagious pustular dermatitis virus vaccine (ORFV/GO-BT15-30 strain) were purchased from the market. Nucleic acid (NA) of SPPV (GL strain) was provided by Lanzhou Veterinary Research Institute. NA of bluetongue virus (BTV) was provided by Institute of Military Veterinary Medicine. In total, 184 clinical samples from cattle and 42 clinical samples from goats or sheep suspected to be infected with CaPVs were provided by CAHEC and Animal Disease Prevention and Control Center of Inner Mongolia. The types of samples included whole blood, serum, saliva, and skin tissue. All the samples were stored at −70°C until further analysis.
2.2. Primer and probe designing
To design the candidate primers and probes, the complete genomic sequences of LSDV, GTPV, and SPPV obtained from GenBank were aligned using MegAlign software of Lasergene. A conserved region of the CaPV ORF091 gene (accession number: AF369026.1) was obtained via the designed similarity comparative algorithm approach. The primers and probes were designed using Primer Premier 5 and Beacon Designer 7.7. Universal primers were designed for all CaPV strains, and three minor groove binder (MGB)-based probes were designed to individually detect LSDV, GTPV, and SPPV. The probes were labeled with fluorescent dyes [6-carboxy fluorescein (FAM), hexachloro fluorescein (HEX), and carboxy-x-rhodamine (ROX) for detecting LSDV, GTPV and SPPV, respectively] on the 5′ end and with a compatible MGB quencher at the 3′ end. The sequences of primers and probes are given in Table 1. The primers and probes were synthesized by Ruibiotech (Qingdao, China).
2.3. NA extraction
The NA from whole blood, serum, saliva, virus, and other liquid samples was directly extracted with commercialized magnetic-bead-based viral DNA/RNA Extraction Kit (Tianlong, Xian, China) using automatic NA extractor (Tianlong, Xian, China). The skin tissue samples were initially homogenized and centrifuged, and the NA was extracted from the supernatant as per the method described above. The NA samples were stored at −70°C until further analysis.
2.4. Construction of positive controls
Positive control plasmids containing the target fragment of the ORF091 gene were constructed as follows. Overall, 2 μL NA of LSDV, GTPV, and SPPV was added to 25 μL reaction mixture containing 2× Platinum Super Green PCR MIX (Thermo Fisher Scientific, United States) and 500 nM of each 091F/091R primer. ddH2O was added to make up the volume to 50 μL. The conventional PCR conditions were 95°C for 5 min; 35 cycles of denaturation at 95°C for 30 s, annealing at 55°C for 30 s, extension at 72°C for 30 s; and final extension at 72°C for 5 min. The PCR products were recovered and ligated to pMD19-T vector. The concentration of the positive plasmids was estimated using NanoDrop one (Thermo Fisher Scientific, United States), and the copy number was determined as per the equation given below.
2.5. Triplex qPCR
In total, 2 μL of the sample NA was added to 10 μL reaction mixture containing 2× AceQ® Universal U+ Probe Master Mix V2 (Vazyme, Nanjing, China) and 250 nM of each primer and each probe. ddH2O was added to make up the volume to 20 μL. The triplex qPCR was performed on the CFX 96™ real-time PCR machine (Bio-Rad, Hercules, CA, United States) with the following conditions: 37°C for 2 min, 95°C for 5 min, and 40 cycles of denaturation at 95°C for 10 s and annealing at 58°C for 30 s. The data acquisition was performed during the annealing step on three different channels including red for FAM, green for HEX, and orange for ROX.
2.6. Assay sensitivity
To determine the assay sensitivity of the triplex qPCR, 10-fold serially diluted positive control plasmids containing the ORF091 gene of LSDV, GTPV, and SPPV were tested to determine the lowest detection limit (LOD). The standard curves were generated. The efficiency of the assay was determined using the following calculation:
2.7. Assay reproducibility
Assay reproducibility was determined by calculating the intra-and inter-assay coefficients of variation (CVs), using at least three replicates of each of the 10-fold serial dilutions of positive controls containing the ORF091 gene of LSDV, GTPV, and SPPV.
2.8. Assay specificity
To determine the specificity of the triplex qPCR, the assay was initially performed using virus-derived NA templates of LSDV, GTPV, and SPPV. Further, the assay was performed using NA templates of non-CaPV pathogens, including FMDV, PPRV, IBRV, BTV, BVDV, and ORFV. To evaluate the assay specificity in case of clinically mixed infections, the assay was performed using virus-derived NA templates of various viruses mixed in 1:1 ratio.
2.9. Evaluation of simulated mixed viral samples
Three positive control plasmids (of concentration 105 copies/μL) were mixed in various proportions and were used to simulate clinical samples of double and triple infection. The ability of the triplex qPCR to differentiate mixed infection samples was assessed. Three replicates of each proportion were used, and the CVs were calculated.
2.10. Evaluation of clinical samples using triplex qPCR
The detection power and usability of the assay was evaluated using NA extracted from blood, serum, saliva, and skin tissue collected from cattle, goats, and sheep with suspected CaPVs infection. All samples were validated using PCR-restriction fragment length polymorphism (PCR-RFLP) for PRO30 gene as described previously (13). Furthermore, all positive PCR products were sequenced and aligned using NCBI server.
3. Results
3.1. Primer and probe designing
The whole genomic sequences of various strains of GTPV, SPPV, and LSDV were downloaded from GenBank and compared using the MegAlign software. The primers designed in this study were conserved and suitable for the amplification of various strains of CaPV based on BLAST hits, whereas the probes were unique for LSDV, GTPV, and SPPV (Figure 1).
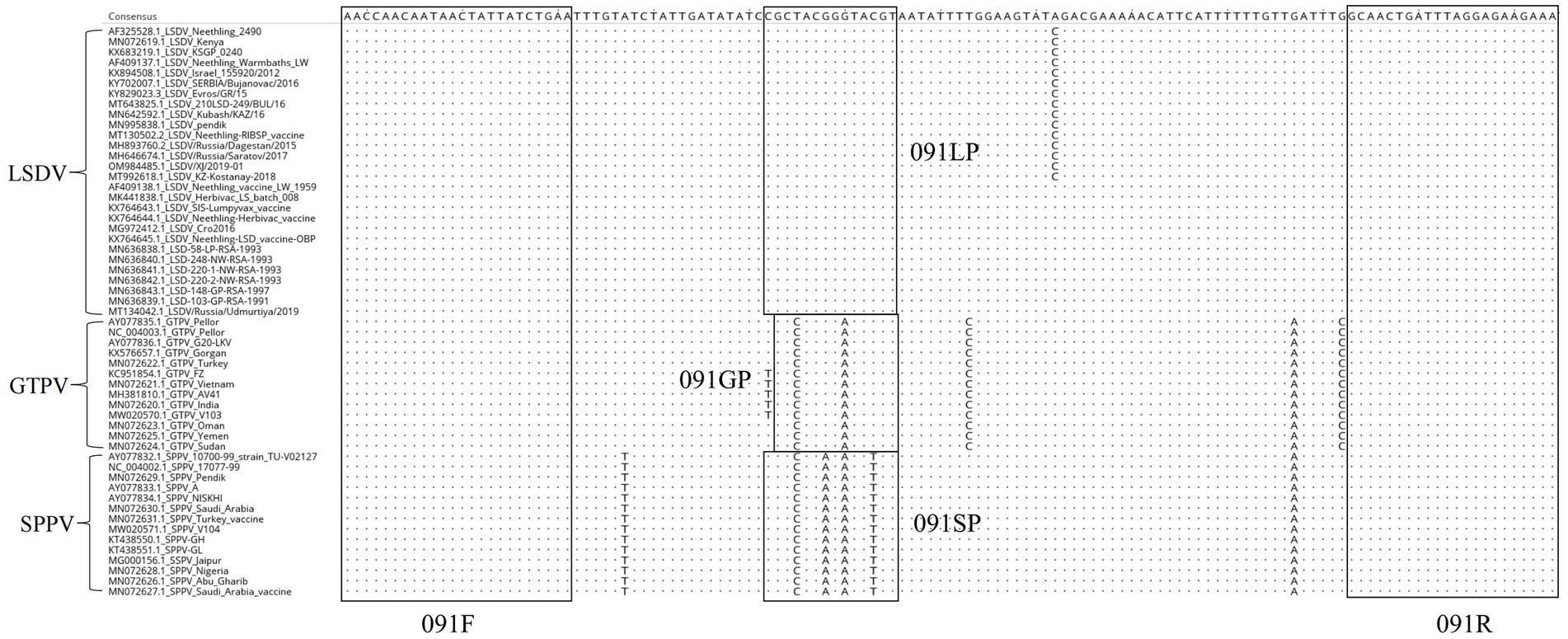
Figure 1. Alignment of ORF091 sequences of lumpy skin disease virus (LSDV), goatpox virus (GTPV), and sheeppox virus (SPPV).
3.2. Construction of positive controls
Three plasmids containing related ORF091 gene fragments were successfully constructed and used as the positive controls for LSDV, GTPV, and SPPV, respectively. The concentration of LSDV, GTPV, and SPPV positive controls was 11.82 (5.41 × 109 copies/μL), 60.48 (2.77 × 1010 copies/μL), and 37.52 (1.73 × 1010 copies/μL) ng/μL, respectively. These positive controls were further used for evaluation of the assay.
3.3. Linearity and LOD of the assay
The linearity of the assay was established by amplifying 10-fold serial dilutions of positive controls. The resulting Cq value was plotted against the log input copy number. Using 102 to 109 dilutions, the efficiencies were determined as 97.9, 83.3, and 83.8% for LSDV, GTPV, and SPPV, respectively (Figure 2). The correlation coefficient (R2) values were > 0.998 (Figure 2). The LOD was 5.41, 27.70, and 17.28 copies/μL for LSDV, GTPV, and SPPV, respectively (Figure 3). The results revealed that the assay was sensitive and could accurately estimate sample concentration in a large-scale linear range.
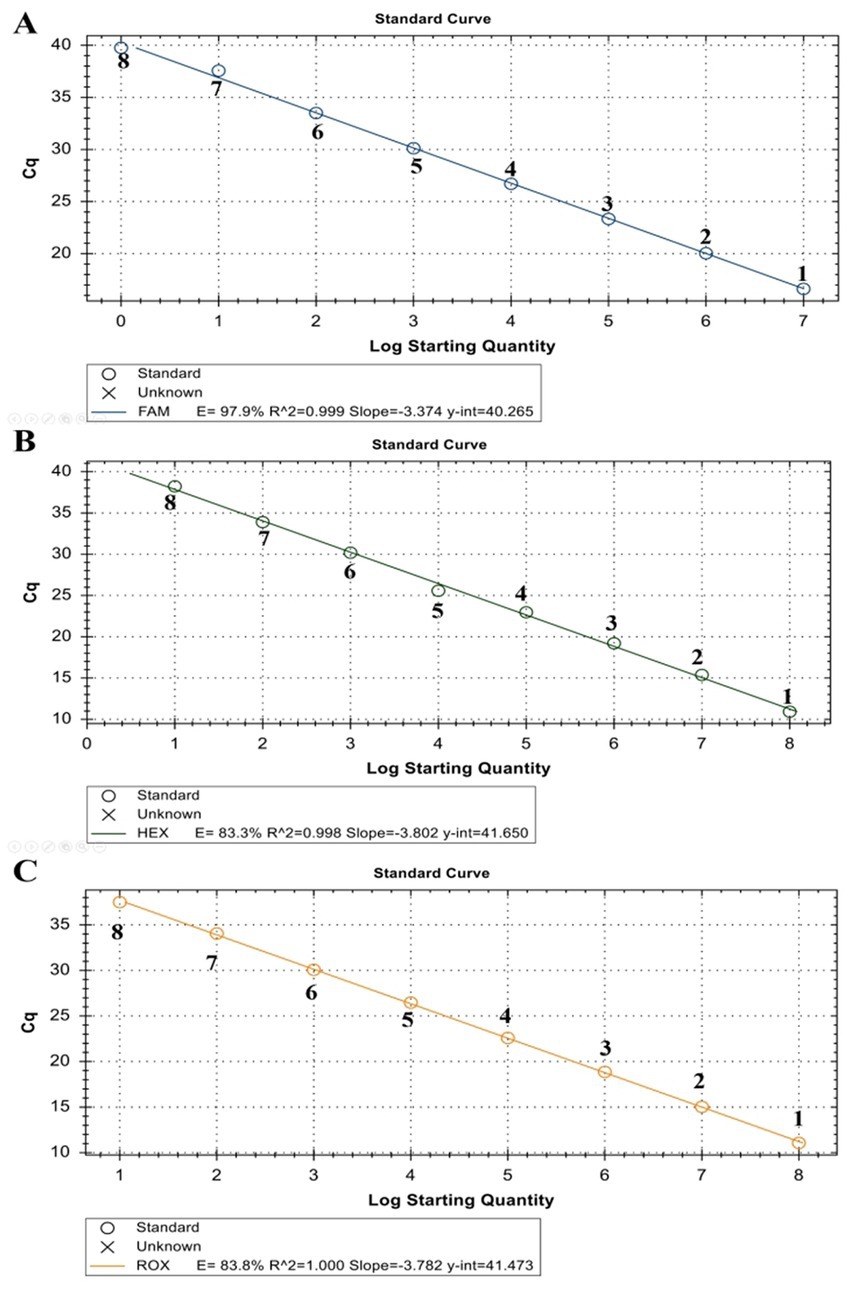
Figure 2. Standard curve of ORF091 positive controls in the triplex qPCR. (A) LSDV ORF091 positive control; (B) GTPV ORF091 positive control; (C) GTPV ORF091 positive control. Dilution factor 1: 102; 2: 103; 3: 104; 4: 105; 5: 106; 6: 107; 7: 108; and 8: 109.
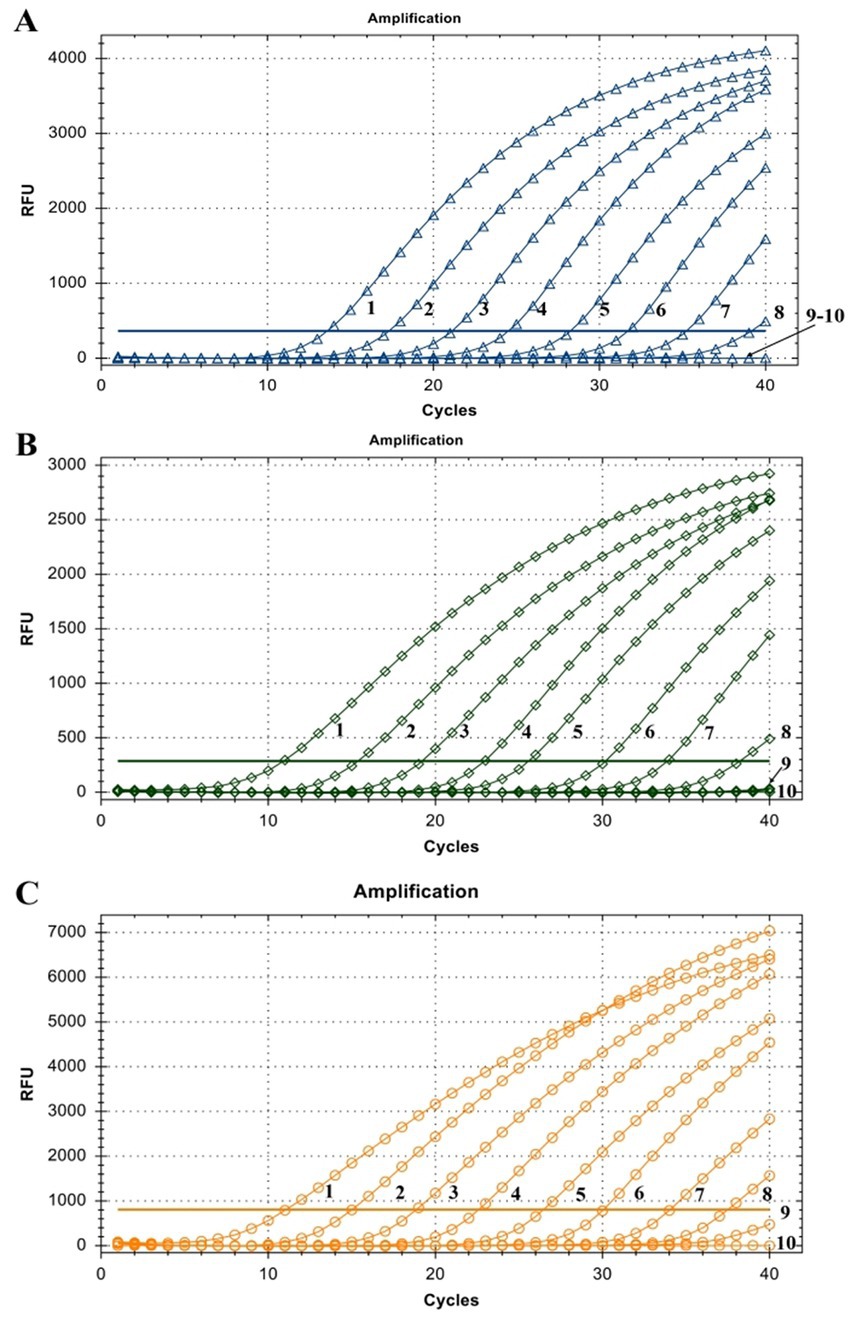
Figure 3. Assay sensitivity of the triplex qPCR. (A) LSDV ORF091 positive control; (B) GTPV ORF091 positive control; (C) GTPV ORF091 positive control. Dilution factor 1: 102; 2: 103; 3: 104; 4: 105; 5: 106; 6: 107; 7: 108; 8: 109; 9: 1010; 10: ddH2O. FAM, HEX, and ROX fluorescence signals were represented by △, ◇, and ○, respectively.
3.4. Cross-reactivity between homologous viruses or viruses causing other common diseases
To demonstrate the assay specificity in homologous viruses, NAs extracted from LSDV, GTPV, and SPPV were evaluated, and the concentrations were 24.35, 11.82, and 20.32 ng/μL, respectively. The LSDV sample produced a strong FAM fluorescence with no HEX or ROX fluorescence. The GTPV sample produced a strong HEX fluorescence with no FAM or ROX fluorescence, and the SPPV sample produced a strong ROX fluorescence with no FAM or HEX fluorescence (Figure 4A). To distinguish LSDV, GTPV, and SPPV from other viruses causing common ruminant diseases, the assay was tested using FMDV, PPRV, IBRV, BTV, BVDV, and ORFV. The samples of these other viruses exhibited negative FAM, HEX, or ROX fluorescence (Figure 4A). In case of mixed-virus-derived NA, the LSDV+GTPV sample produced strong FAM and HEX fluorescence with no ROX fluorescence (Figure 4B); the LSDV+SPPV sample produced strong FAM and ROX fluorescence with no HEX fluorescence (Figure 4C), and the GTPV+SPPV sample produced strong HEX and ROX fluorescence with no FAM fluorescence (Figure 4D). Neither false positivity nor interference was observed among the three fluorophore signals, indicating high specificity.
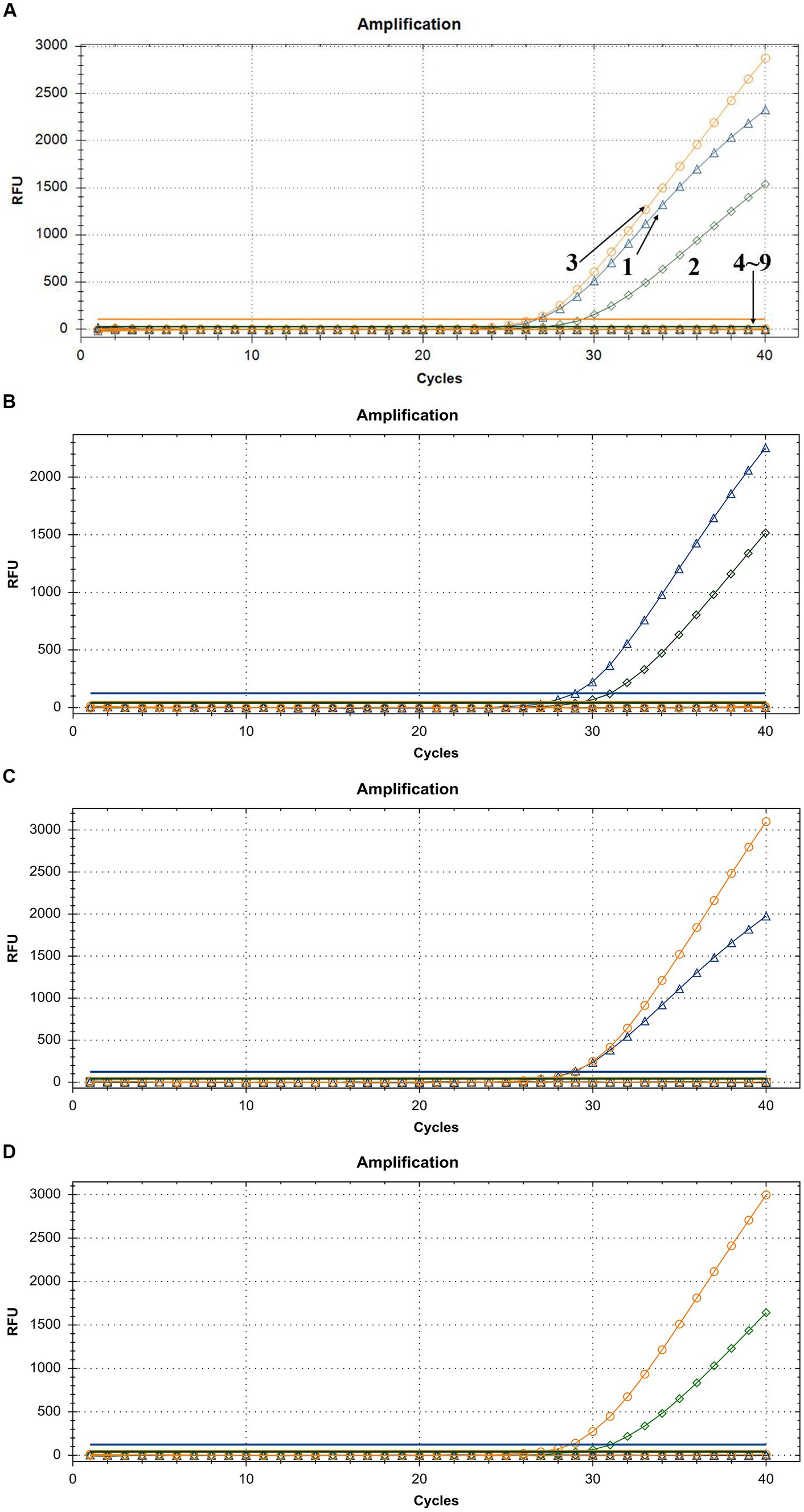
Figure 4. Assay specificity of the triplex qPCR. (A) 1: LSDV; 2: GTPV; 3: SPPV; 4: BTV; 5: FMDV; 6: BVDV and IBRV; 7: ORFV; 8: PPRV; and 9: ddH2O. (B) LSDV + GTPV. (C) LSDV + SPPV. (D) GTPV+SPPV. FAM, HEX, and ROX fluorescence signals were represented by △, ◇, and ○, respectively.
3.5. Inter-and intra-assay variability
The assay reproducibility was evaluated using 10-fold serial dilutions of positive controls. The CVs are summarized in Table 2. The CVs between runs were mostly in the range of 0.18–2.31% for LSDV, 0.16–1.78% for GTPV, and 0.37–2.29% for SPPV (Table 2). The assay exhibited a high reproducibility in inter-and intra-assay testing.
3.6. Simulation of mixed infections
The artificially mixed plasmids that simulated clinical double and triple mixed infections were analyzed using the triplex qPCR. The assay successfully distinguished two or three types of plasmids in various mixed schemes without any cross-reaction (Table 3). The CVs of the assay for detecting simulated double and triple mixed infection were < 2.68 and < 3.92%, respectively (Table 3).
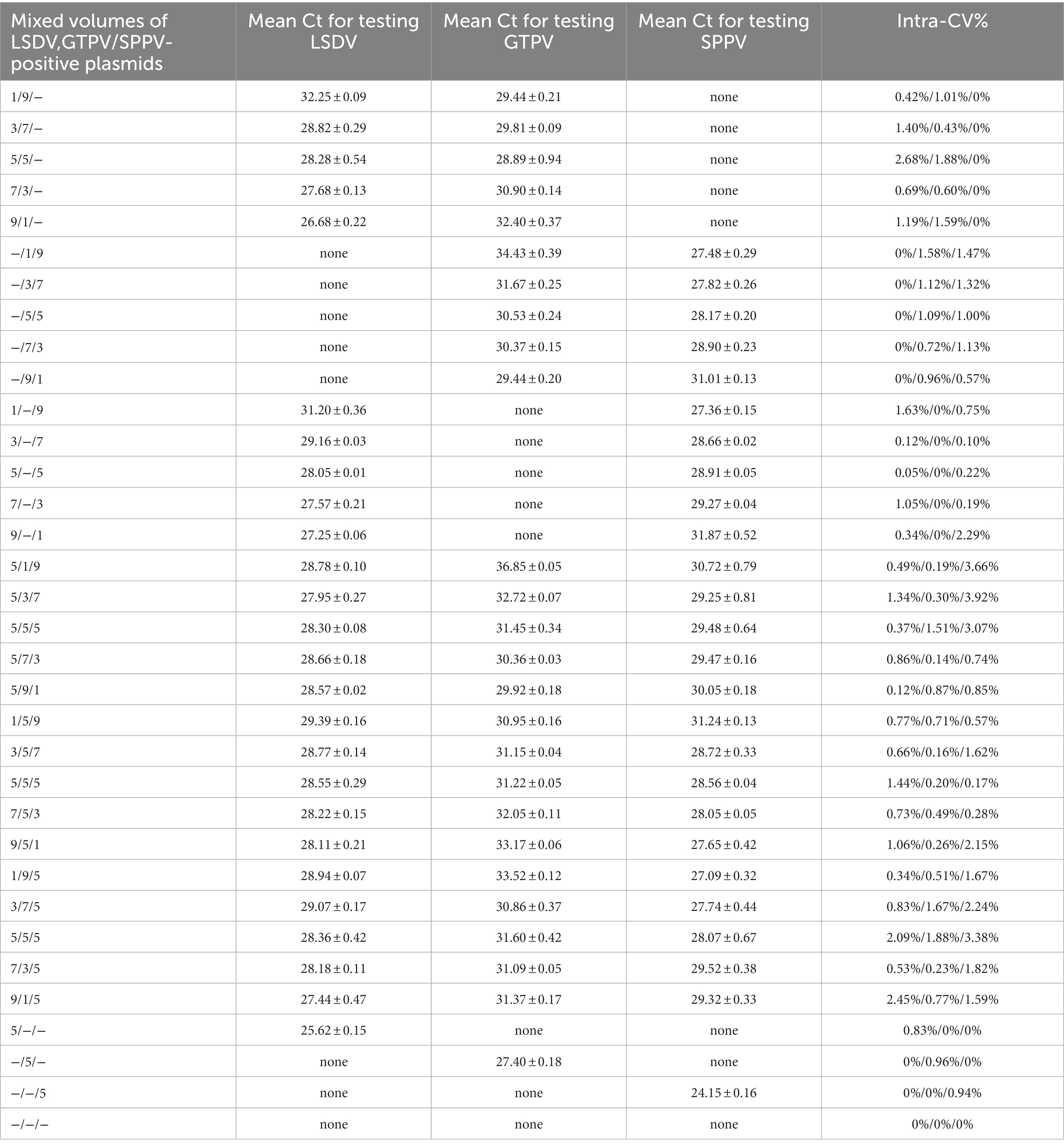
Table 3. Mean Ct values and CV% of the triple qPCR for testing artificial mixtures of control plasmids.
3.7. Virus detection in clinical samples
The detection of viruses in 226 clinical samples (including 95 samples infected with LSDV, six samples infected with GTPV, seven samples infected with SPPV, and 118 negative samples) exhibited a detection rate of 100%. This was consistent with the results obtained by PCR-PFLP and sequencing methods.
4. Discussion
Capripoxvirus infection in ruminants causes significant morbidity and mortality in Africa, Middle East, and Asia; therefore, it has a great economic importance (14). Currently, all CaPVs are ranked as class II animal diseases in China. Attenuated GTPV strain AV41 is widely used to immunize cattle, goats, and sheep to prevent CaPV. Combined with movement control, quarantine supervision, sterilization, insect control, and other measures, the number of CaPV outbreaks has greatly reduced, particularly LSDV outbreaks in China in recent years.
Lumpy skin disease virus infection seems to be restricted to cattle and large wild ruminants. SPPV and GTPV infect mainly small domestic and wild ruminants, which may share similar geographical locations and same animal species. Both lead to the formation of generalized or localized cutaneous lesions (14). For controlling CaPV infection, immunization is a critical measure and live attenuated vaccines are being used for years. Globally, sheep, goats, and cattle are mostly vaccinated with Romania, Bakirkoy, Yugoslavian RM65, KSGP O-240, and KSGP O-180 strains; Gorgan, Mysore, Uttarkashi, and KSGP O-240 strains; and Neethling, KSGP O-240 and KSGP O-180, Romania, Bakirkoy, and Gorgan strains (3), respectively, which are homologous and heterologous immunization strategies. Thus, development of accurate and rapid methods to distinguish these three viruses is helpful for the early detection, surveillance, and control of CaPV infection, as well as for the differentiation of wild-type strains and vaccine strains when heterologous immunization strategies are used.
Capripoxviruses are closely related, with genomic sequence identities ranging from 96% (between species) to 99% (between the strains of same species) (15). It is difficult to serologically distinguish these three viruses. For the viral detection, conventional PCR, qPCR, and HRM methods have been developed and validated (13, 14, 16–19). Conventional PCR involves gel electrophoresis. HRM assay strongly depends on PCR conditions, instruments, and dyes, and the accuracy is critically dependent on the resolution of the instrument (20, 21). In recent years, because of the outbreaks of African swine fever and Coronavirus disease 2019, qPCR application is greatly promoted and widely accepted, particularly in China (22–25). qPCR is considered one of the most useful tools for clinical molecular detection, which is reliable, sensitive, and cost-effective.
In the present study, we developed a new MBG-based triplex qPCR that can successfully distinguish LSDV, GTPV, and SPPV in one reaction. The LOD was 5.41, 27.70, and 17.28 copies/μL for LSDV, GTPV, and SPPV, respectively. The probes were BLAST searched and found to be specific for the related species only. The assay exhibited no cross-reactivity with the viruses causing common ruminant-related diseases including PPRV, FMDV, ORFV, BTV, IBRV, and BVDV. Furthermore, the CVs of inter-and intra-assay were <2.5%. Through simulation experiments, the assay successfully distinguished CaPVs in case of mixed infections without any cross-reaction. The results indicated the triplex qPCR assay is highly specific, sensitive, and reproducible for distinguishing LSDV, GTPV, and SPPV. The assay was evaluated using clinical samples, and the results were completely consistent with the results of PCR-PFLP and sequencing methods. This indicated that the assay is reliable for clinical application.
Therefore, the assay provided a robust, rapid, and simple tool for the differentiation of LSDV, GTPV, and SPPV and facilitated more accurate disease diagnosis and surveillance for better control of CaPV.
Data availability statement
The original contributions presented in the study are included in the article/supplementary material, further inquiries can be directed to the corresponding authors.
Author contributions
YC, DP, and WN were responsible for the experimental design and drafting the manuscript. MG, YL, JL, LL, HQ, CL, YW, FW, XW, and ZW performed the experiments and analyzed data. All authors contributed to the article and approved the submitted version.
Funding
This work was supported by National Key Research and Development Program (No. 2022YFD1800500).
Conflict of interest
The authors declare that the research was conducted in the absence of any commercial or financial relationships that could be construed as a potential conflict of interest.
Publisher’s note
All claims expressed in this article are solely those of the authors and do not necessarily represent those of their affiliated organizations, or those of the publisher, the editors and the reviewers. Any product that may be evaluated in this article, or claim that may be made by its manufacturer, is not guaranteed or endorsed by the publisher.
References
1. World Organization of Animal Health (2019) Manual of diagnostic tests and vaccines, Ch. 3.04.12. Available at: https://www.oie.int/fileadmin/Home/eng/Health_standards/tahm/3.04.12_LSD.pdf
2. World Organization of Animal Health (2019) Manual of diagnostic tests and vaccines. Chapter 3.07.12 Available at: https://www.oie.int/fileadmin/Home/eng/Health_standards/tahm/3.07.12_S_POX_G_POX.pdf
3. Hamdi, J, Munyanduki, H, Omari Tadlaoui, K, El Harrak, M, and Fassi, FO. Capripoxvirus infections in ruminants: a review. Microorganisms. (2021) 9:902. doi: 10.3390/microorganisms9050902
4. Tuppurainen, E, Venter, EH, Shisler, JL, Gari, G, Mekonnen, GA, Juleff, N, et al. Review: Capripoxvirus diseases: current status and opportunities for control. Transbound Emerg Dis. (2017) 64:729–45. doi: 10.1111/tbed.12444
5. Hamdi, J, Bamouh, Z, Jazouli, M, Boumart, Z, Tadlaoui, KO, Fihri, OF, et al. Experimental evaluation of the cross-protection between Sheeppox and bovine lumpy skin vaccines. Sci Rep. (2020) 10:8888. doi: 10.1038/s41598-020-65856-7
6. Lu, G, Xie, J, Luo, J, Shao, R, Jia, K, and Li, S. Lumpy skin disease outbreaks in China, since 3 august 2019. Transbound Emerg Dis. (2021) 68:216–9. doi: 10.1111/tbed.13898
7. Li, L, Qi, C, Li, J, Nan, W, Wang, Y, Chang, X, et al. Quantitative real-time PCR detection and analysis of a lumpy skin disease outbreak in Inner Mongolia Autonomous Region. Front Vet Sci. (2022) 9:936581. doi: 10.3389/fvets.2022.936581
8. Tuppurainen, ES, and Galon, N. Lumpy skin disease: Current situation in Europe and neighbouring regions and necessary control measures to halt the spread in south-East Europe. Oie Reg. Comm. (2016).
9. Yeruham, I, Nir, O, Braverman, Y, Davidson, M, Grinstein, H, Haymovitch, M, et al. Spread of lumpy skin disease in Israeli dairy herds. Vet Rec. (1995) 137:91–3. doi: 10.1136/vr.137.4.91
10. Ayelet, G, Abate, Y, Sisay, T, Nigussie, H, Gelaye, E, Jemberie, S, et al. Lumpy skin disease: preliminary vaccine efficacy assessment and overview on outbreak impact in dairy cattle at Debre Zeit, Central Ethiopia. Antivir Res. (2013) 98:261–5. doi: 10.1016/j.antiviral.2013.02.008
11. Ben-Gera, J, Klement, E, Khinich, E, Stram, Y, and Shpigel, NY. Comparison of the efficacy of Neethling lumpy skin disease virus and x10RM65 sheep-pox live attenuated vaccines for the prevention of lumpy skin disease—the results of a randomized controlled field study. Vaccine. (2015) 33:4837–42. doi: 10.1016/j.vaccine.2015.07.071
12. Calistri, P, DeClercq, K, Gubbins, S, Klement, E, Stegeman, A, Cortiñas Abrahantes, J, et al. Lumpy skin disease: III. Data Coll Analy EFSA J. (2019) 17:e05638. doi: 10.2903/j.efsa.2019.5638
13. Wang, H, Kong, Y, Mei, L, Lv, J, Wu, S, Lin, X, et al. Multiplex real-time PCR method for simultaneous detection and differentiation of Goatpox virus, Sheeppox virus, and lumpy skin disease virus. J AOAC Int. (2021) 104:1389–93. doi: 10.1093/jaoacint/qsab040
14. Gelaye, E, Mach, L, Kolodziejek, J, Grabherr, R, Loitsch, A, Achenbach, JE, et al. A novel HRM assay for the simultaneous detection and differentiation of eight poxviruses of medical and veterinary importance. Sci Rep. (2017) 7:42892. doi: 10.1038/srep42892
15. Tulman, ER, Afonso, CL, Lu, Z, Zsak, L, Sur, JH, Sandybaev, NT, et al. The genomes of sheeppox and goatpox viruses. J Virol. (2002) 76:6054–61. doi: 10.1128/jvi.76.12.6054-6061.2002
16. Lamien, CE, le Goff, C, Silber, R, Wallace, DB, Gulyaz, V, Tuppurainen, E, et al. Use of the Capripoxvirus homologue of vaccinia virus 30kDa RNA polymerase subunit (RPO30) gene as a novel diagnostic and genotyping target: development of a classical PCR method to differentiate goat poxvirus from sheep poxvirus. Vet Microbiol. (2011) 149:30–9. doi: 10.1016/j.vetmic.2010.09.038
17. Agianniotaki, EI, Chaintoutis, SC, Haegeman, A, Tasioudi, KE, de Leeuw, I, Katsoulos, P, et al. Development and validation of a TaqMan probe-based real-time PCR method for the differentiation of wild type lumpy skin disease virus from vaccine virus strains. J Virol Methods. (2017) 249:48–57. doi: 10.1016/j.jviromet.2017.08.011
18. Wolff, J, Beer, M, and Hoffmann, B. Probe-based real-time qPCR assays for a reliable differentiation of Capripox virus species. Microorganisms. (2021) 9:765. doi: 10.3390/microorganisms9040765
19. Chibssa, TR, Settypalli, TBK, Berguido, FJ, Grabherr, R, Loitsch, A, Tuppurainen, E, et al. An HRM assay to differentiate Sheeppox virus vaccine strains from Sheeppox virus field isolates and other Capripoxvirus species. Sci Rep. (2019) 9:6646. doi: 10.1038/s41598-019-43158-x
20. Wittwer, CT . High-resolution DNA melting analysis: advancements and limitations. Hum Mutat. (2009) 30:857–9. doi: 10.1002/humu.20951
21. Herrmann, MG, Durtschi, JD, Bromley, LK, Wittwer, CT, and Voelkerding, KV. Amplicon DNA melting analysis for mutation scanning and genotyping: cross-platform comparison of instruments and dyes. Clin Chem. (2006) 52:494–503. doi: 10.1373/clinchem.2005.063438
22. Gao, Q, Feng, Y, Yang, Y, Luo, Y, Gong, T, Wang, H, et al. Establishment of a dual real-time PCR assay for the identification of African swine fever virus genotypes I and II in China. Front Vet Sci. (2022) 9:882824. doi: 10.3389/fvets.2022.882824
23. Yang, H, Peng, Z, Song, W, Zhang, C, Fan, J, Chen, H, et al. A triplex real-time PCR method to detect African swine fever virus gene-deleted and wild type strains. Front Vet Sci. (2022) 9:943099. doi: 10.3389/fvets.2022.943099
24. Wang, Y, Xu, L, Noll, L, Stoy, C, Porter, E, Fu, J, et al. Development of a real-time PCR assay for detection of African swine fever virus with an endogenous internal control. Transbound Emerg Dis. (2020) 67:2446–54. doi: 10.1111/tbed.13582
Keywords: Capripoxvirus
, detection, qPCR, differentiation,
Citation: Nan W, Gong M, Lu Y, Li J, Li L, Qu H, Liu C, Wang Y, Wu F, Wu X, Wang Z, Chen Y and Peng D (2023) A novel triplex real-time PCR assay for the differentiation of lumpy skin disease virus, goatpox virus, and sheeppox virus. Front. Vet. Sci. 10:1175391. doi: 10.3389/fvets.2023.1175391
Edited by:
Wei Wang, Wenzhou University, ChinaReviewed by:
Yidong Fei, Jilin Agricultural Science and Technology College, ChinaShawn Babiuk, Canadian Food Inspection Agency (CFIA), Canada
Xueyuan Hu, Qingdao Agricultural University, China
Copyright © 2023 Nan, Gong, Lu, Li, Li, Qu, Liu, Wang, Wu, Wu, Wang, Chen and Peng. This is an open-access article distributed under the terms of the Creative Commons Attribution License (CC BY). The use, distribution or reproduction in other forums is permitted, provided the original author(s) and the copyright owner(s) are credited and that the original publication in this journal is cited, in accordance with accepted academic practice. No use, distribution or reproduction is permitted which does not comply with these terms.
*Correspondence: Yiping Chen, Y3lwNzc3QDE2My5jb20=; Daxin Peng, cGVuZ2R4QHl6dS5lZHUuY24=
†These authors share first authorship