- Guangdong Key Laboratory for Veterinary Drug Development and Safety Evaluation, College of Veterinary Medicine, South China Agricultural University, Guangzhou, China
Tilmicosin is a semi-synthetic macrolide for veterinary use with strong antibacterial effect on respiratory bacteria. In this study, the pharmacokinetic/pharmacodynamic (PK/PD) integration of tilmicosin against Pasteurella multocida (P. multocida) was evaluated by establishing a piglet tissue cage infection model. Concentration of tilmicosin and bacterial numbers of P. multocida in the tissue-cage fluid were monitered. After the population of P. multocida was equal to or greater than 107 CFU/mL in a tissue cage, piglets received an oral administration of tilmicosin at a dose of 30, 40, 50, and 60 mg/kg b.w., once daily for 3 days, respectively. Bacteria were counted every 24 h after drug administration and at 48 and 72 h after the last administration. A sigmoidal Emax model was used to fit the relationship between PK/PD parameters and the antibacterial effect. AUC24h/MIC was the best PK/PD index that correlated with effectiveness of tilmicosin against P. multocida. The magnitude of AUC24h/MIC required for continuous 1/3-log, 1/2-log, and 3/4-log reductions were 19.65 h, 23.86 h, and 35.77 h, respectively, during each 24 h treatment period. In this study, when the dosage was >50 mg/kg, the AUC24h/MIC was still >35.77 h in the period of 24–48 h after the last administration due to the slow elimination, that is, tilmicosin exhibited a potent antibacterial effect against P. multocida after three successive daily administrations. The data provide meaningful guidance to optimize regimens of tilmicosin to treat respiratory tract infections caused by P. multocida.
1. Introduction
Pasteurella multocida (P. multocida) caused acute infectious diseases such as hemorrhagic septicemia or infectious pneumonia in various animals. The infection was often accompanied by the risk of other bacteria, causing porcine respiratory disease complex (PRDC). Multiple serotypes and complex typing make it difficult to prevent and control by vaccine (1). Tilmicosin is a macrolide synthesized from tylosin, which showed strong antibacterial activity against Actinobacillus pleuropneumoniae, P. multocida, and Mycoplasma (2–4).
The pharmacokinetics of tilmicosin was characterized by rapid absorption, slow elimination, and large apparent volume of distribution (Vd). Futhermore, the higher concentration in lungs relative to plasma makes it a valuable treatment option for respiratory infections. According to Zhang et al. (5), the Cmax of tilmicosin in lung tissues was 14.28 times higher than in plasma in chickens. In rabbits, the concentrations attained in lung tissue exceeded that in plasma by 7-fold after the second day of treatment (6). Foster et al. (7) determined that in calves, the concentration of tilmicosin in bronchoalveolar lavage samples exceeded plasma concentration by 776%. The absorption half-time (T1/ka) and elimination half-life (T1/2kel) of tilmicosin in healthy pigs were 2.27 h and 43.53 h after oral administration, respectively, and 1.64 h and 20.69 h in another study (8, 9). The Vd in pigs was 48.36 L/kg after a single oral administration and the Vd in both sheep and cattle were 25.0 L/kg when normalized for animal body weight, indicating the extensive tissue distribution of tilmicosin (10, 11).
Studies indicated that tilmicosin exhibited higher efficacy in treating bovine respiratory tract infection compared to oxytetracycline (12). Tilmicosin mixed feed was often used to treat and prevent respiratory diseases in pigs. However, irrational use of antimicrobials leads to severe acquired resistance of bacteria, resulting in treatment failure (13). PK/PD analysis could describe the relationship between the kinetics and the effect to establish rational dosage regimens of antimicrobial agents. PK/PD modeling in veterinary medicine can be used to define the relationship among concentrations, time, and therapeutic outcomes to more truly describe the dynamic relationship among drugs, hosts, and pathogens. Tissue cage model is a common method to study PK/PD in vivo. A tissue cage made of silicone tube, small volume, is suitable for animals with tight skin, mainly pigs. The model has been used to study the PK/PD integration of cefquinome and tulathromycin on P. multocida in vivo (14, 15). Therefore, it is of great significance to study the pharmacokinetics and pharmacodynamics of tilmicosin against P. multocida in pigs to ensure the rational use of drugs.
For pigs, it is difficult to obtain the drug concentrations and bacterial load at the target tissue in lungs of P. multocida in fection. The tissue cage model is an ideal model to study the relationship between PK/PD parameters and the antibacterial effect, which considers the interaction of the drug, host, and pathogen. The objective of the study was to determine the relationship between pharmacokinetic/pharmacodynamic indices and the antibacterial efficacy of tilmicosin against P. multocida. Additionally, the findings could inform the development of tilmicosin dosage regimens for treating respiratory illnesses caused by P. multocida infection.
2. Materials and methods
2.1. Bacterial strain, antimicrobials, and chemicals
Strain C44-15 (serovar D:7) of P. multocida was obtained from the China Institute of Veterinary Drug Control (Beijing, China). Timicosin phosphate with a purity of 80.4% (Tilmicosin) was provided by Guangdong Dahuanong Animal Health Products (Guangdong, China). Pentobarbital sodium was purchased from Xiangbo Biotechnology Co., Ltd. Procainamide hydrochloride was supplied by Shangdong Fangming pharmaceutical Co., Ltd. Tryptic soy broth (TSB) and tryptic soy agar (TSA) were purchased from Guangdong Huankai Microbial Technology (Guangzhou, China). Newborn bovine serum was supplied by Guangzhou Ruite Biotechnology (Guangzhou, China).
2.2. Animals and tissue-cage infection model
The study used 10 healthy crossbred piglets (Duroc × Landrace × Yorkshire) weighing 25–30 kg acquired from the Guangzhou Fine Breed Swine Farm. The piglets were given antibiotic-free feed and water over the course of the experiment. All the experimental procedures involving the animals were approved by the Committee on the Ethics of Animals of South China Agricultural University (approval number: 2018A001).
The specifications and surgical implantation procedures for the tissue cages (TCs) matched those described previously (16). The TCs were constructed from food-grade silicone tubes measuring 65 mm in length, 13 mm inner diameter, and 18 mm outer diameter. Twelve holes were made at each end of the tubes. The TCs were surgically implanted under general anesthesia induced by pentobarbital sodium and local anesthesia from procainamide hydrochloride. Sterile TCs were inserted subcutaneously on both sides of the pigs’ necks, positioned equidistantly between the jugular vein and spinal cord. Following surgery, the incision sites were treated with penicillin and tetracycline ointment for 3–5 days to prevent infection. After a 4-week recovery period, the TCs contained clear, yellow tissue cage fluid (TCF). The TCF was collected for bacteriological testing, and bacteria-free TCs were used for the experiment.
The sterile TCs were inoculated with 1 mL of a P. multocida suspension containing approximately 1.5 × 108 CFU/mL. Following 48 h of incubation, 0.5 mL of TCF was extracted from each tissue cage to quantify the bacteria. TCs with bacterial counts around 107 CFU/mL were selected for use in the subsequent experiment.
2.3. Minimum inhibitory concentration determination
The MIC of tilmicosin against P. multocida was ascertained using a microdilution technique following the guidelines of the Clinical and Laboratory Standards Institute (CLSI, 2013). The MIC value was derived from TSB supplemented with 5% calf serum and TCF cultures.
2.4. Experimental design and sample collection
Ten piglets were randomly divided into four treatment groups (two piglets and four tissues cages each group) and one control group (infected but not treatment). One male and one female pig were contained in each group. Each treatment group was administered orally with tilmicosin at the dosages of 30, 40, 50, and 60 mg/kg respectively, once a day for 3 days. The control group was treated with the same amount of normal saline.
For pharmacokinetics, around 0.5 mL of TCF was obtained at 1, 3, 6, 9, 12, and 24 h following each administration, and additional samples were collected at 48 and 72 h after the final dose. The samples underwent centrifugation at 3000 × g for 10 min to remove particulate matter and were kept frozen at −20°C until analyzed.
For pharmacodynamics, about 0.1 mL of TCF was collected for bacterial counting at 24 h after each administration and at 48 and 72 h after the last administration. The suspension was serially diluted 10-fold in saline, with 100 μL used initially. Subsequently, 20 μL of the dilutions were dispersed onto agar plates and incubated for 12 h to enumerate the bacterial population.
2.5. Quantification of tilmicosin in tissue cage fluid
The tilmicosin concentration was measured by high-performance liquid chromatography-tandem masss pectrometry (HPLC-MS/MS) using a system from Agilent Technologies (Santa Clara, CA, United States). Separation was achieved on a C18 column (150 mm × 2.0 mm, 5 μm; Phenomenex, Torrance, CA, United States). The mobile phase consisted of solution A (water with 0.1% formic acid, V/V) and solution B (acetonitrile) at a flow rate of 0.25 mL/min. The elution gradient was: 0–1.5 min, 10% B; 1.5–6 min, 95% B; 6–6.5 min, 5% B; 6.5–12.5 min, 5% B. The injection volume was 5 μL.
The samples were allowed to thaw to ambient temperature prior to analysis. Aliquots of 200 μL TCF were added to a 1.5 mL micro centrifuge tube and then equal volume acetonitrile was added to precipitate protein. After vortexing for 2 min, and centrifuging at 12000 × g for 10 min, the clear supernatant was filtered through a 0.22-μm nylon syringe filter (JinTeng Experiment Equipment Company) and then injected into an auto sampler vial. A calibration curve was established with tilmicosin concentrations (0.005–0.5 μg/mL). PK parameters were calculated using WinNonlin software version 6.1 (Pharsight, Mountain View, CA, United States).
2.6. Intergration and modeling of PK/PD
The PK/PD parameters were calculated based on the individual pharmacokinetics of each treated infected TCs: AUC24h/MIC (AUC24h, area under the concentration–time curve between 0 to 24 h after each dose), Cmax/MIC (Cmax, maximum concentration of tilmicosin after each dose), and %T > MIC (the percentage of time that tilmicosin concentration above MIC after each dosing interval). The relationship between the PK/PD indices and efficacy were evaluated using the sigmoidal maximum effect (Emax) PD model. The formula was as follows:
Where E represents the antibacterial effect, defined as the change in bacterial count (log10CFU/mL) over a 24 h dosing interval; E0 denotes the change of bacterial count in the control sample; Emax is the maximum antibacterial effect during the dosing interval; Ce signifies the value of a PK/PD index (Cmax/MIC, AUC24h/MIC, and %T > MIC); EC50 represents the PK/PD index value producing 50% of the maximal effect; and N is the Hill coefficient defining the slope of the effect curve.
3. Results
3.1. Minimum inhibitory concentration
The MIC values of tilmicosin against P. multocida (D:7) in both TCF and TSB broth were 0.25 μg/mL.
3.2. Pharmacokinetics of tilmicosin in the piglet tissue-cage infection model
The concentration-time profiles of tilmicosin in TCF following multiple dosing are depicted in Figure 1. The mean values for AUC24h, Cmax, and %T > MIC are presented in Table 1. Pharmacokinetic analysis was performed using non-compartmental methods in WinNonlin software. The determined AUC24h ranged from 3.57 ± 0.20 to 13.65 ± 0.38 μg h/mL. Cmax values were obtained directly from the concentration-time curves, spanning 0.20 ± 0.03 to 0.63 ± 0.03 μg/mL. The %T > MIC values covered a range of 0 to 100%. Specifically, the AUC values from 24–48 h after the final dose at 30, 40, 50 and 60 mg/kg were 6.31 ± 0.51, 8.35 ± 0.36, 11.69 ± 0.33, and 12.87 ± 0.22 μg h/mL, respectively.
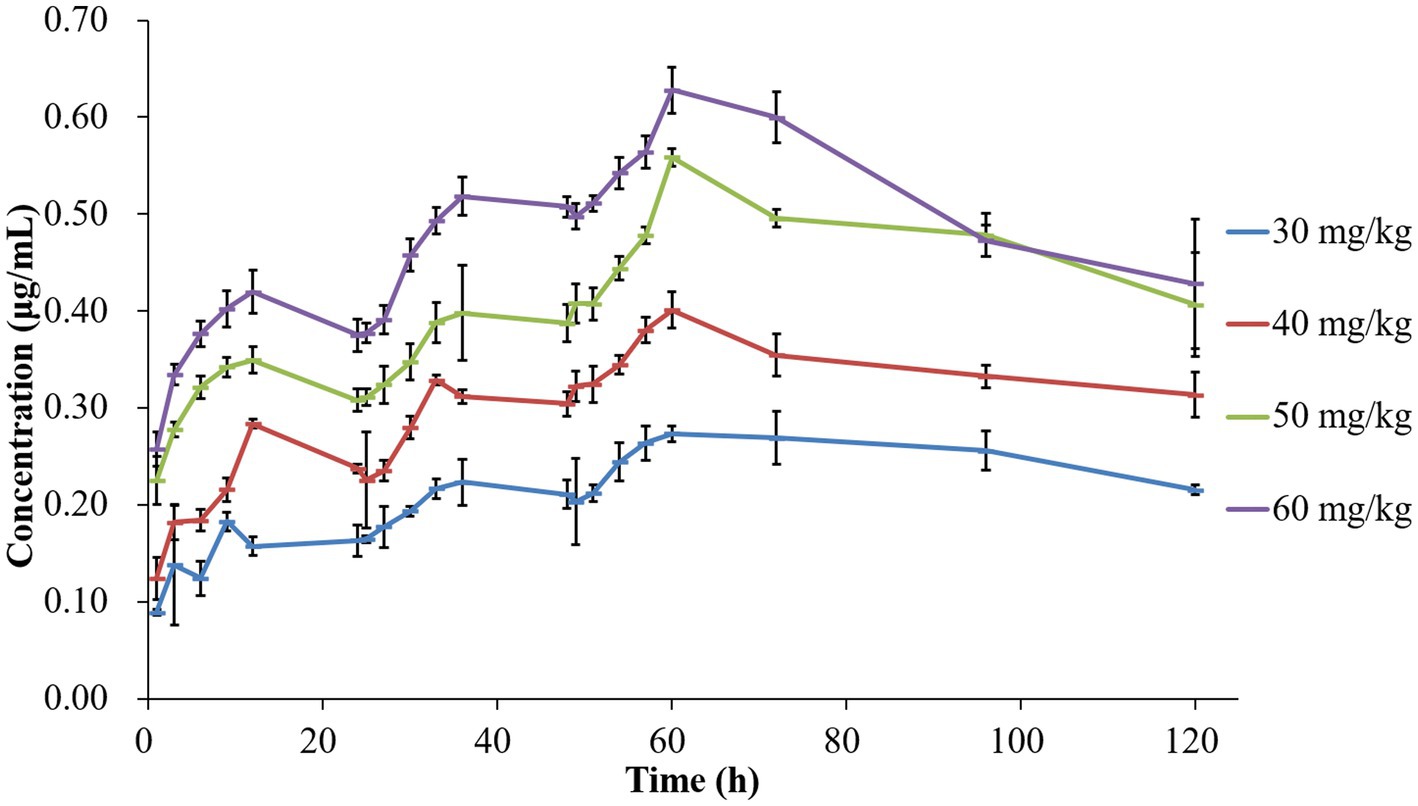
Figure 1. Concentration-time curves of tilmicosin in infected tissue cages following different multiple dosage administration.
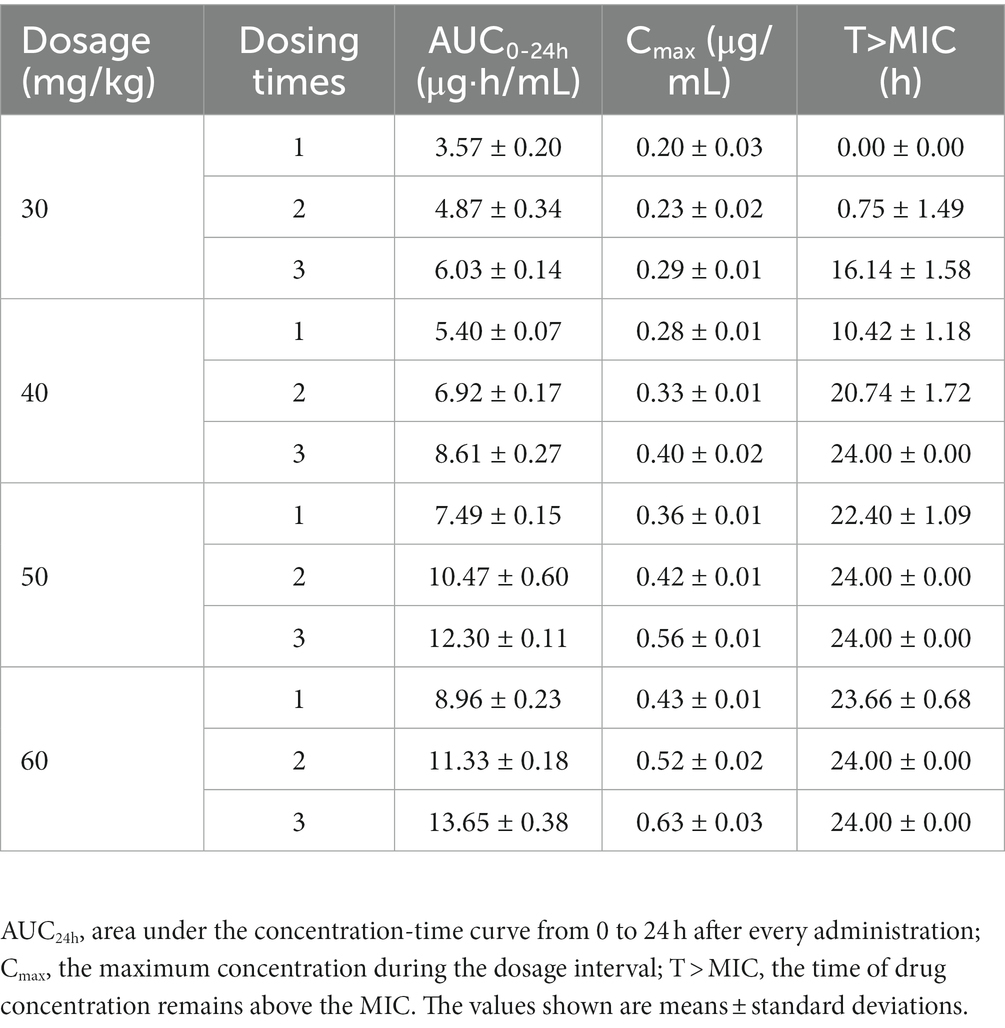
Table 1. Pharmacokinetic parameters of tilmicosin in infected tissue cage fluid after multiple administration.
3.3. In vivo antibacterial studies tilmicosin against Pasteurella multocida
The time-kill curves depicted in Figure 2 demonstrate the antibacterial efficacy of tilmicosin against P. multocida in the piglet tissue-cage infection model across varying doses. Bacterial counts for the untreated control group remained around 107 CFU/mL. Following tilmicosin administration at 30, 40, 50, and 60 mg/kg for 3 days, the total reductions in bacteria (log10 CFU/mL) were 1.48 ± 0.13, 2.82 ± 0.10, 3.39 ± 0.11, and 3.52 ± 0.15, respectively. Tilmicosin exhibited bactericidal activity at doses of 50 and 60 mg/kg after multiple administrations.
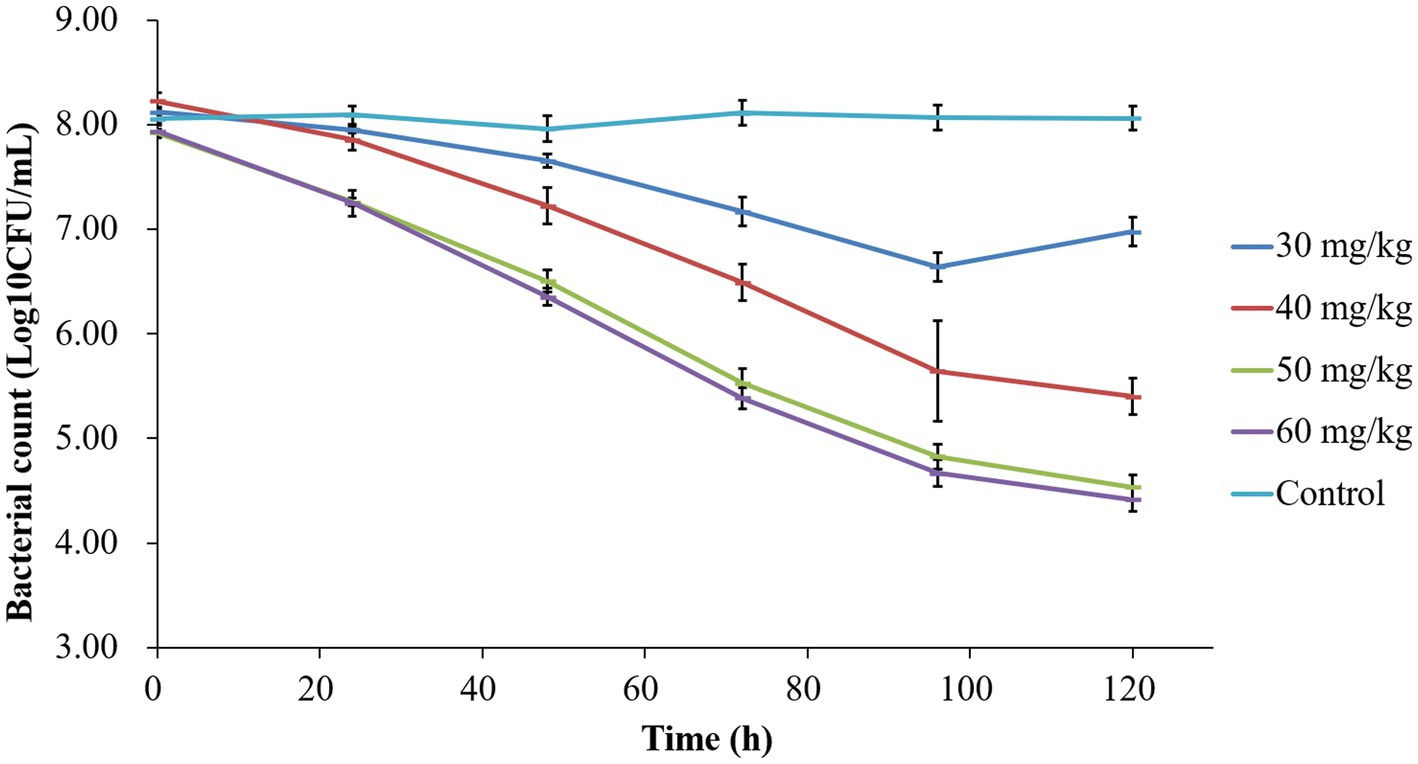
Figure 2. Time-killing curves of tilmicosin against P. multocida at different dosages in the tissue-cage model.
3.4. In vivo pharmacokinetic/pharmacodynamic integration and modeling
The correlation between three PK/PD parameters (AUC24h/MIC, Cmax/MIC, and %T > MIC) and antibacterial activity of tilmicosin against P. multocida in TCF derived from Sigmoid Emax model was shown in Figure 3. The R2 of AUC24h/MIC, Cmax/MIC, and %T > MIC with an antibacterial effect was 0.92, 0.90, and 0.83, respectively. The obtained parameters of E0, Emax, EC50, and the Hill coefficient are listed in Table 2. The estimated AUC24h/MIC values for a 1/3 log10CFU/mL, 1/2 log10CFU/mL, and 3/4 log10CFU/mL reduction were 19.64 h, 33.45 h, and 35.64 h, respectively, during the 24 h administration of tilmicosin. Figure 3 illustrates the correlation between three PK/PD indices (AUC24h/MIC, Cmax/MIC, and %T > MIC) and the antibacterial activity of tilmicosin against P. multocida in tissue cage fluid, as modeled using the Sigmoid Emax model. The coefficients of determination (R2) for AUC24h/MIC, Cmax/MIC, and %T > MIC with antibacterial effect were 0.92, 0.90, and 0.83, respectively. The derived E0, Emax, EC50, and Hill coefficient parameters are tabulated in Table 2. Based on the model, the estimated AUC24h/MIC values required for a 1/3 log10CFU/mL, 1/2 log10CFU/mL, and 3/4 log10CFU/mL bacterial reduction over 24 h were 19.64 h, 33.45 h, and 35.64 h, respectively.
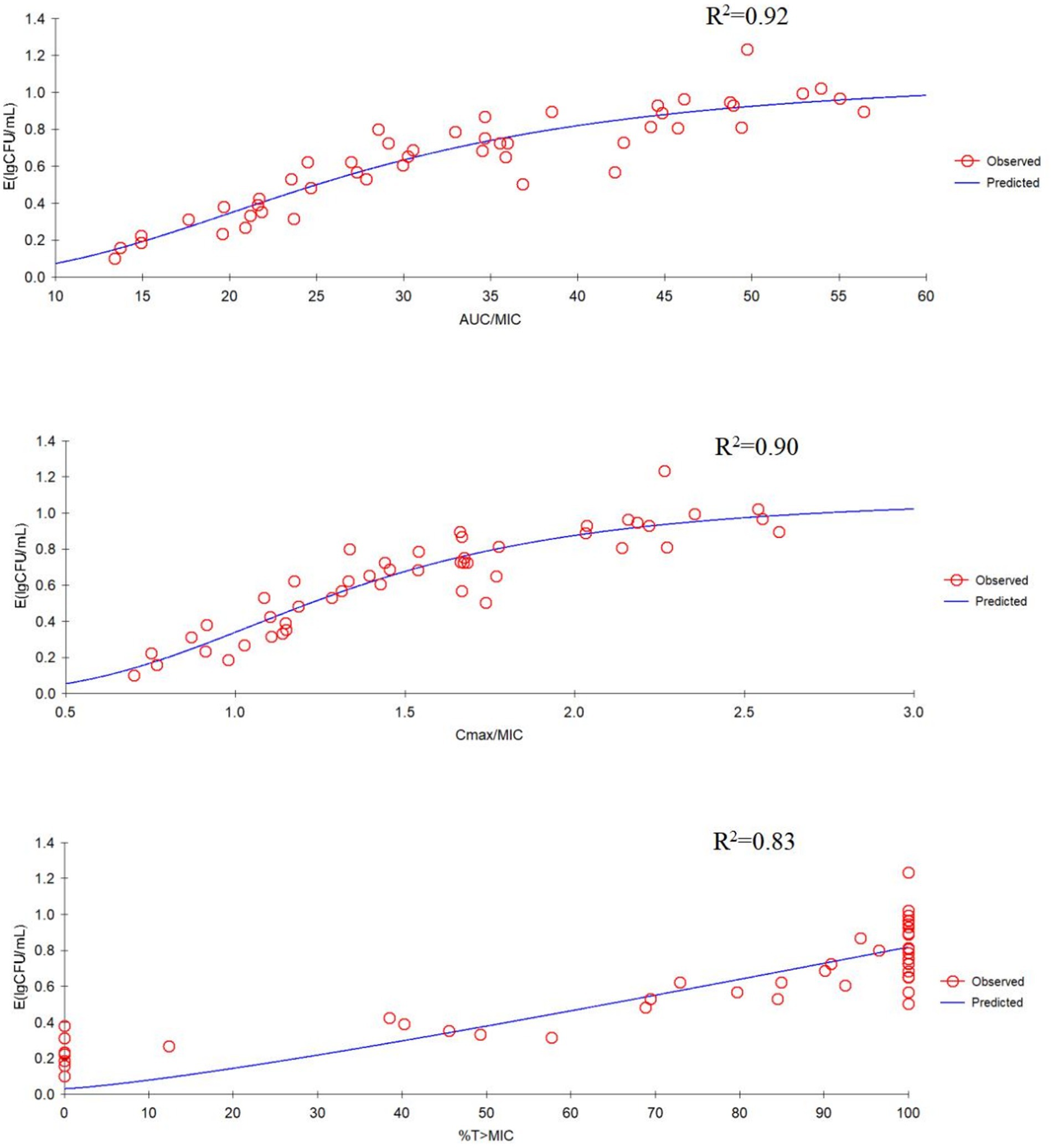
Figure 3. Emax relationships for the three pharmacokinetic/pharmacodynamic (PK/PD) parameters versus antibacterial effect.
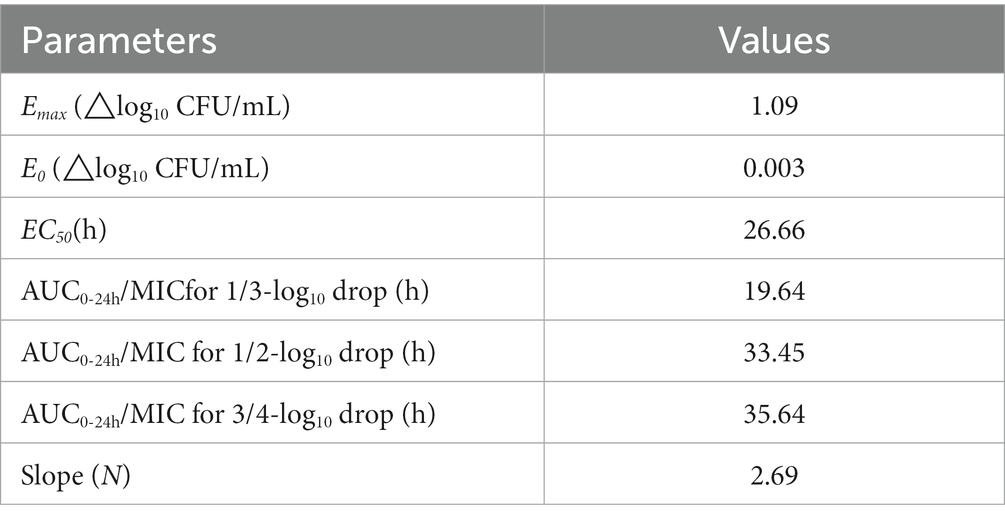
Table 2. The values of PK/PD parameters and AUC24h/MIC required for the indicated antibacterial effects.
4. Discussion
P. multocida is one of the most common bacterial species among porcine respiratory diseases, which greatly affects the production and health of swine and results in high economic losses. PK/PD modeling provides a chance for dosage optimization of antimicrobial therapy. Tissue cage model has been used to study the PK/PD integration of tulathromycin, marbofloxacin, and cefquinome on P. multicida (14, 15, 17). The dosage of other drugs commonly used in veterinary clinic, such as danofloxacin, florfenicol, ceftiofur, and oxytetracycline, have also been studied using PK/PD model (18–21). AUC24h/MIC was recognized as an important PK/PD parameter of macrolide antibiotic tulathromycin for antibacterial efficiency (14). As a semi-synthetic macrolide antibiotic with broad-spectrum activity, tilmicosin demonstrates potent efficacy against respiratory tract pathogens including Actinobacillus pleuropneumoniae, Haemophilus parasuis, and even Mycoplasma pneumonia (3, 22, 23). The study on the effect of tilmicosin on P. multocida will be helpful to the treatment of respiratory infection in pigs.
There may be differences in the antibacterial effect in artificial culture medium or in serum or tissue fluid. The MICs of tiamulin against several strains of Actinobacillus pleuropneumoniae in culture medium and pig serum were 12 and 24, 14 and 24, 12 and 32, and 12 and 24 μg/mL, respectively (24). Marbofloxacin has the same antibacterial effect on Mannheimia haemolytica in broth medium and sheep serum, MIC 0.035 μg/mL and MBC 0.045 μg/mL (25). For cefquinome against P. multocida, the MIC was similar in broth medium and body fluid, 0.03 μg/mL in medium and 0.04 μg/mL both in serum and tissue fluid (26). The MICs of tulathromycin against P. multocida CVCC430 in serum, transudate, and exudate were all found to be 0.03 μg/mL whereas in MHB medium it was 0.25 μg/mL, and the concentrations of tulathromycin in transudate and exudate were lower than those in serum (14). The determination of MIC in different media is necessary to study the antibacterial activity of drugs. In this study, the MICs of tilmicosin against P. multocida (D:7) in both TCF and TSB were 0.25 μg/mL, which was also used for in vivo PK/PD analysis. In this study, the data derived from tissue fluid in tissue cage model can better integrate pharmacokinetics and pharmacodynamics.
Oral administration of tilmicosin was adopted in this study. Study indicated subcutaneous injection of tilmicosin caused inflammation at the injection site (27, 28), and the side effects such as accelerated respiration, vomiting, convulsions, or even death may be produced after its intravenous and intramuscular administration or repeated high-dose administration (29). The potential application for an injectable formulation of tilmicosin for the treatment or control of porcine respiratory disease was contraindicated due to a low margin of safety. In clinical practice, the mixed feeding administration of tilmicosin at 200–400 mg/kg demonstrated a good effect on the prevention and treatment of pneumonia caused by Actinobacillus pleuropneumoniae and P. multocida (30, 31). Previous studies have found that most macrolide drugs exhibited time-dependent antibacterial activity, and two PK/PD parameters used to illustrate the antibacterial effect are AUC24h/MIC and %T > MIC. Before the present study, we had a preliminary study to design dose regimen. When the dose is below 20 mg/kg, the drug concentration is always lower than MIC. The ideal PK/PD parameters of T > MIC cannot be obtained, so the dose range for this study is 30 mg/kg to 60 mg/kg.
It is sometimes confusing to select the best PK/PD parameters to evaluate the efficacy of drugs based on the antibacterial characteristics (time-dependent or concentration-dependent), because the characteristics of the drug itself have a lot to do with it. The classification of drugs is not the only reference for selecting parameters to describe antibacterial effect. For drugs with long elimination half-life, PK/PD parameter AUC24h/MIC is usually appropriate to describe the effect. The t1/2β of tilmicosin likely enables T > MIC to adequately suppress regrowth of the predominant bacterial population. This suggests that the AUC24h/MIC is the optimal PK/PD index to predict antibacterial activity. Azithromycin, time-dependent, because of its long elimination half-life, the best parameter for predicting the efficacy is AUC/MIC (32). The PK/PD parameters describing the effect of florfenicol is AUC24h/MIC for animals with long elimination half-life and %T > MIC could also be adopted for animals with short elimination half-life (33, 34). In vitro time-kill assays demonstrated a concentration-dependent antibacterial effect of tilmicosin against Haemophilus parasuis (22). The effect of tilmicosin against Lawsonia intracellularis was concentration-dependent, thus, AUC/MIC was used to formulate the dosage regimen (35). In the present study, the correlation between the PK/PD index of AUC24h/MIC and antibacterial effect was the highest (R2 = 0.92), which may be due to the slow elimination of tilmicosin in pigs. This is also an important reason why it is inappropriate to use% T > MIC to guide the administration of tilmicosin.
The relationship between AUC24h/MIC and bacterial change was fitted by Sigmoid model, and the magnitude of AUC24h/MIC required for a 3/4 log10CFU/mL of bacterial killing during 24 h was 35.64 h. Bactericidal activity was defined as >3-log10 reduction in CFU/mL. In this study, tilmicosin was administered once a day for 3 days. When the dosage was >50 mg/kg, the AUC24h/MIC was still >35.64 h in the period of 24–48 h after the last administration due to the slow elimination of tilmicosin, that is, the number of bacteria could be reduced by 3 log10CFU/mL within 96 h after administration and bactericidal effects can be achieved. In this study, the counts of bacteria under 50 and 60 mg/kg were no significantly difference (Figure 3). The possible reason is that for concentration-dependent drugs, the effect is enhanced with the increase of drug concentration within a certain range. When the dose is 50 mg/kg, the maximum effect has been achieved. Moreover, tilmicosin belongs to macrolide drugs, which are antibacterial drugs, and cannot completely eliminate the P. multocida.
Although some useful in vivo PK/PD data were obtained by establishing tissue cage infection model in the study, there are some limitations of this model. After all, the lung and not the tissue cage was the site of the infection. Compare with convention PK/PD model, physiological-based pharmacokinetic (PBPK) and hollow fiber infection model (HFIM) showed the power in flexibility and prediction. For instance, the concentrations under different doses of tilmicosin in different tissues even in pigs with different ages can be predicted by applying a PBPK model. In a HFIM, except the total counts of bacteria, the counts of resistant subpopulation and non-inherited resistant subpopulation (persistent cells and biofilms) can be detected. In future studies, PBPK model and HFIM model should be considered.
5. Conclusion
The current study investigated the PK/PD integration of tilmicosin against P. multocida strain D:7 was studied by establishing a tissue cage infection model in pigs. The results demonstrated that the AUC24h/MIC (R2 = 0.92) was the optimal PK/PD index for describing antibacterial activity. Further analysis indicated that an AUC24h/MIC value of 35.64 h was necessary for tilmicosin to achieve a 3/4 log10CFU/mL reduction in TCF. The bactericidal efficacy of tilmicosin against P. multocida was attained at doses of 50 mg/kg or higher, administered once daily for 3 days. These findings will facilitate guidance and optimization of clinical dosing regimens for treatment of P. multocida infections in piglets.
Data availability statement
The original contributions presented in the study are included in the article/supplementary material, further inquiries can be directed to the corresponding author.
Ethics statement
The animal study was approved by Committee on the Ethics of Animals of South China Agricultural University. The study was conducted in accordance with the local legislation and institutional requirements.
Author contributions
YC: Writing – original draft, Data curation, Software. XJ: Validation, Writing – original draft. SZ: Validation, Writing – original draft. WW: Writing – review & editing. HZ: Writing – original draft. HD: Conceptualization, Funding acquisition, Project administration, Writing – original draft, Writing – review & editing.
Funding
This work was supported by the National Natural Science Foundation of China (grant no. 31972733).
Conflict of interest
The authors declare that the research was conducted in the absence of any commercial or financial relationships that could be construed as a potential conflict of interest.
Publisher’s note
All claims expressed in this article are solely those of the authors and do not necessarily represent those of their affiliated organizations, or those of the publisher, the editors and the reviewers. Any product that may be evaluated in this article, or claim that may be made by its manufacturer, is not guaranteed or endorsed by the publisher.
References
1. Smith, E, Miller, E, Aguayo, JM, Figueroa, CF, Nezworski, J, Studniski, M, et al. Genomic diversity and molecular epidemiology of Pasteurella Multocida. PLoS One. (2021) 16:e0249138. doi: 10.1371/journal.pone.0249138
2. Garmyn, A, Vereecken, M, De Gussem, K, Depondt, W, Haesebrouck, F, and Martel, A. Efficacy of Tylosin and Tilmicosin against experimental Mycoplasma Gallisepticum infection in chickens. Avian Dis. (2019) 63:359–65. doi: 10.1637/11991-110818-Reg.1
3. Dong, Z, Zhou, XZ, Sun, JC, Meng, XB, Li, HS, Cheng, FS, et al. Efficacy of enteric-coated Tilmicosin granules in pigs artificially infected with Actinobacillus Pleuropneumoniae serotype 2. Vet Med Sci. (2020) 6:105–13. doi: 10.1002/vms3.198
4. Moore, GM, Basson, RP, and Tonkinson, LV. Clinical field trials with Tilmicosin phosphate in feed for the control of naturally acquired pneumonia caused by Actinobacillus Pleuropneumoniae and Pasteurella Multocida in swine. Am J Vet Res. (1996) 57:224–8.
5. Zhang, N, Liu, Z, Wei, Y, Zhang, C, Mao, C, Cai, Q, et al. Comparison of the pharmacokinetics of Tilmicosin in plasma and lung tissue in healthy chickens and chickens experimentally infected with Mycoplasma Gallisepticum. J Vet Pharmacol Ther. (2020) 43:347–54. doi: 10.1111/jvp.12847
6. Gallina, G, Lucatello, L, Drigo, I, Cocchi, M, Scandurra, S, Agnoletti, F, et al. Kinetics and intrapulmonary disposition of Tilmicosin after single and repeated Oral bolus administrations to rabbits. Vet Res Commun. (2010) 34 Suppl 1:S69–72. doi: 10.1007/s11259-010-9385-2
7. Foster, DM, Sylvester, HJ, and Papich, MG. Comparison of direct sampling and Brochoalveolar lavage for determining active drug concentrations in the pulmonary epithelial lining fluid of calves injected with Enrofloxacin or Tilmicosin. J Vet Pharmacol Ther. (2017) 40:e45–53. doi: 10.1111/jvp.12412
8. Shen, J, Li, C, Jiang, H, Zhang, S, Guo, P, Ding, S, et al. Pharmacokinetics of Tilmicosin after Oral Administration in Swine. Am J Vet Res. (2005) 66:1071–4. doi: 10.2460/ajvr.2005.66.1071
9. Zhang, L, Zhao, L, Liu, Y, Liu, J, and Li, X. Pharmacokinetics of Tilmicosin in healthy pigs and in pigs experimentally infected with Haemophilus Parasuis. J Vet Sci. (2017) 18:431–7. doi: 10.4142/jvs.2017.18.4.431
10. Zhang, N, Ba, J, Wang, S, Xu, Z, Wu, F, Li, Z, et al. Pharmacokinetics and bioavailability of solid dispersion formulation of Tilmicosin in pigs. J Vet Pharmacol Ther. (2021) 44:359–66. doi: 10.1111/jvp.12929
11. Modric, S, Webb, AI, and Derendorf, H. Pharmacokinetics and pharmacodynamics of Tilmicosin in sheep and cattle. J Vet Pharmacol Ther. (1998) 21:444–52. doi: 10.1046/j.1365-2885.1998.00177.x
12. Musser, J, Mechor, GD, Gröhn, YT, Dubovi, EJ, and Shin, S. Comparison of Tilmicosin with long-acting Oxytetracycline for treatment of respiratory tract disease in calves. J Am Vet Med Assoc. (1996) 208:102–6.
13. Klima, CL, Holman, DB, Cook, SR, Conrad, CC, Ralston, BJ, Allan, N, et al. Multidrug resistance in Pasteurellaceae associated with bovine respiratory disease mortalities in North America from 2011 to 2016. Front Microbiol. (2020) 11:606438. doi: 10.3389/fmicb.2020.606438
14. Zhou, Q, Zhang, G, Wang, Q, Liu, W, Huang, Y, Yu, P, et al. Pharmacokinetic/pharmacodynamic modeling of Tulathromycin against Pasteurella Multocida in a porcine tissue cage model. Front Pharmacol. (2017) 8:392. doi: 10.3389/fphar.2017.00392
15. Zhang, L, Wu, X, Huang, Z, Kang, Z, Chen, Y, Shen, X, et al. Pharmacokinetic/pharmacodynamic integration of Cefquinome against Pasteurella Multocida in a piglet tissue cage model. J Vet Pharmacol Ther. (2019) 42:60–6. doi: 10.1111/jvp.12705
16. Zhang, BX, Lu, XX, Gu, XY, Li, XH, Gu, MX, Zhang, N, et al. Pharmacokinetics and ex vivo pharmacodynamics of Cefquinome in porcine serum and tissue cage fluids. Vet J. (2014) 199:399–405. doi: 10.1016/j.tvjl.2013.12.015
17. Zeng, QL, Mei, X, Su, J, Li, XH, Xiong, WG, Lu, Y, et al. Integrated pharmacokinetic-Pharmacodynamic (Pk/Pd) model to evaluate the in vivo antimicrobial activity of Marbofloxacin against Pasteurella Multocida in piglets. BMC Vet Res. (2017) 13:178. doi: 10.1186/s12917-017-1099-z
18. Zhou, YF, Sun, Z, Wang, RL, Li, JG, Niu, CY, Li, XA, et al. Comparison of Pk/Pd targets and cutoff values for Danofloxacin against Pasteurella Multocida and Haemophilus Parasuis in piglets. Front Vet Sci. (2022) 9:811967. doi: 10.3389/fvets.2022.811967
19. Dorey, L, Pelligand, L, Cheng, Z, and Lees, P. Pharmacokinetic/pharmacodynamic integration and modelling of Florfenicol for the pig pneumonia pathogens Actinobacillus Pleuropneumoniae and Pasteurella Multocida. PLoS One. (2017) 12:e0177568. doi: 10.1371/journal.pone.0177568
20. Mi, K, Pu, S, Hou, Y, Sun, L, Zhou, K, Ma, W, et al. Optimization and validation of dosage regimen for Ceftiofur against Pasteurella Multocida in swine by physiological based pharmacokinetic-Pharmacodynamic model. Int J Mol Sci. (2022) 23:3722. doi: 10.3390/ijms23073722
21. Dorey, L, Pelligand, L, Cheng, Z, and Lees, P. Pharmacokinetic/pharmacodynamic integration and modelling of Oxytetracycline for the porcine pneumonia pathogens Actinobacillus Pleuropneumoniae and Pasteurella Multocida. J Vet Pharmacol Ther. (2017) 40:505–16. doi: 10.1111/jvp.12385
22. Zhang, P, Hao, H, Li, J, Ahmad, I, Cheng, G, Chen, D, et al. The epidemiologic and Pharmacodynamic cutoff values of Tilmicosin against Haemophilus Parasuis. Front Microbiol. (2016) 7:385. doi: 10.3389/fmicb.2016.00385
23. Huang, Z, Hu, Z, Zheng, H, Xia, X, Gu, X, Shen, X, et al. The Pk/Pd integration and resistance of Tilmicosin against Mycoplasma Hyopneumoniae. Pathogens. (2020) 9:487. doi: 10.3390/pathogens9060487
24. Pridmore, A, Burch, D, and Lees, P. Determination of minimum inhibitory and minimum bactericidal concentrations of Tiamulin against field isolates of Actinobacillus Pleuropneumoniae. Vet Microbiol. (2011) 151:409–12. doi: 10.1016/j.vetmic.2011.03.016
25. Sidhu, PK, Landoni, MF, Aliabadi, FS, and Lees, P. Pharmacokinetic and Pharmacodynamic modelling of Marbofloxacin administered alone and in combination with Tolfenamic acid in goats. Vet J. (2010) 184:219–29. doi: 10.1016/j.tvjl.2009.02.009
26. Shan, Q, Yang, F, Wang, J, Ding, H, He, L, and Zeng, Z. Pharmacokinetic/pharmacodynamic relationship of Cefquinome against Pasteurella Multocida in a tissue-cage model in yellow cattle. J Vet Pharmacol Ther. (2014) 37:178–85. doi: 10.1111/jvp.12076
27. Van Donkersgoed, J, Dubeski, PL, VanderKop, M, Aalhus, JL, Bygrove, S, and Starr, WN. The effect of animal health products on the formation of injection site lesions in Subprimals of experimentally injected beef calves. Can Vet J. (2000) 41:617–22.
28. Clark, C, Dowling, PM, Ross, S, Woodbury, M, and Boison, JO. Pharmacokinetics of Tilmicosin in equine tissues and plasma. J Vet Pharmacol Ther. (2008) 31:66–70. doi: 10.1111/j.1365-2885.2007.00909.x
29. Jordan, WH, Byrd, RA, Cochrane, RL, Hanasono, GK, Hoyt, JA, Main, BW, et al. A review of the toxicology of the antibiotic Micotil 300. Vet Hum Toxicol. (1993) 35:151–8.
30. Paradis, MA, Vessie, GH, Merrill, JK, Dick, CP, Moore, C, Charbonneau, G, et al. Efficacy of Tilmicosin in the control of experimentally induced Actinobacillus Pleuropneumoniae infection in swine. Can J Vet Res. (2004) 68:7–11.
31. Nerland, EM, LeBlanc, JM, Fedwick, JP, Morck, DW, Merrill, JK, Dick, P, et al. Effects of Oral Administration of Tilmicosin on pulmonary inflammation in piglets experimentally infected with Actinobacillus Pleuropneumoniae. Am J Vet Res. (2005) 66:100–7. doi: 10.2460/ajvr.2005.66.100
32. Van Bambeke, F, and Tulkens, PM. Macrolides: pharmacokinetics and pharmacodynamics. Int J Antimicrob Agents. (2001) 18:17–23. doi: 10.1016/s0924-8579(01)00406-x
33. Lei, Z, Liu, Q, Yang, S, Yang, B, Khaliq, H, Li, K, et al. Pk-Pd integration modeling and cutoff value of Florfenicol against Streptococcus Suis in pigs. Front Pharmacol. (2018) 9:2. doi: 10.3389/fphar.2018.00002
34. Zhang, H, Huang, Y, Yu, J, Liu, X, and Ding, H. Pk/Pd integration of Florfenicol alone and in combination with doxycycline against Riemerella Anatipestifer. Front Vet Sci. (2022) 9:975673. doi: 10.3389/fvets.2022.975673
Keywords: Pasteurella multocida, Tilmicosin, PK/PD relationship, tissue cage infection model, in vivo
Citation: Chen Y, Ji X, Zhang S, Wang W, Zhang H and Ding H (2023) Pharmacokinetic/pharmacodynamic integration of tilmicosin against Pasteurella multocida in a piglet tissue cage model. Front. Vet. Sci. 10:1260990. doi: 10.3389/fvets.2023.1260990
Edited by:
Fan Yang, Henan University of Science and Technology, ChinaReviewed by:
Yingchun Liu, Chinese Academy of Agricultural Sciences, ChinaKaixiang Zhou, China Agricultural University, China
Copyright © 2023 Chen, Ji, Zhang, Wang, Zhang and Ding. This is an open-access article distributed under the terms of the Creative Commons Attribution License (CC BY). The use, distribution or reproduction in other forums is permitted, provided the original author(s) and the copyright owner(s) are credited and that the original publication in this journal is cited, in accordance with accepted academic practice. No use, distribution or reproduction is permitted which does not comply with these terms.
*Correspondence: Huanzhong Ding, aHpkaW5nQHNjYXUuZWR1LmNu