- 1Haman Ranch, The Mannheimer Foundation, LaBelle, FL, United States
- 2Department of Clinical Sciences, College of Veterinary Medicine, Cornell University, Ithaca, NY, United States
- 3Toxicology Research Laboratory, Department of Pharmacology, College of Medicine, University of Illinois at Chicago, Chicago, IL, United States
Introduction: Cannabinoids are increasingly popular in human and veterinary medicine and have been studied as an alternative treatment for a wide range of disorders. The goal of this study was to perform a pharmacokinetic analysis of oral cannabidiol (CBD)-/cannabidiolic acid (CBDA)-rich hemp oil (CBD/ArHO) in juvenile cynomolgus macaques (Macaca fascicularis).
Methods: After a 2 mg/kg CBD/ArHO pilot study, 4 and 8 mg/kg direct-to-mouth CBD/ArHO were administered (n = 4 per dose) once daily for 14 days and blood was collected at 0-, 0.5-, 1-, 2-, 4-, 8-, 12-, and 24-h, and on Days 7 and 14, to quantify serum cannabinoid concentrations by high-performance liquid chromatography–tandem mass spectrometry. Serum biochemistries and complete blood counts were performed on Days 0, 1, and 14.
Results: The maximum mean serum concentration (Cmax) of CBDA was 28.6–36.2 times that of CBD at 4 and 8 mg/kg. At 8 mg/kg, the Cmax of CBD was 1.4 times higher (p = 0.0721), and CBDA was significantly 1.8 times higher (p = 0.0361), than at 4 mg/kg. The maximum mean serum concentration of ∆9-tetrahydrocannabinol (THC) was 4.80 ng/mL at 8 mg/kg. Changes in serum biochemistries and complete blood counts over time were not clinically significant.
Discussion: Given the low serum CBD concentrations, the doses and frequency used in this study may be insufficient for a therapeutic effect of CBD in particular; therefore, clinical studies are needed to determine the therapeutic dose of CBD and CBDA for macaques, which may differ based on the disorder targeted.
1 Introduction
The hemp plant (Cannabis sativa L.) is a source of a variety of cannabinoids, with the most notable abundant bioactive components being cannabidiol (CBD), ∆9-tetrahydrocannabiol (THC), and their acids (1). Temperature, humidity, precipitation, and genetic variety affect the phytocannabinoid profile of hemp plants (2), wherein cannabigerolic acid (CBGA) is converted to cannabidiolic acid (CBDA) by CBDA synthase or to ∆9-tetrahydrocannabinolic acid (THCA) by THCA synthase (3, 4). Then, light, heat, or oxygenation cause decarboxylation of these acids to cannabigerol (CBG), CBD, or THC during storage and processing (5, 6). Although CBD can be converted to THC under artificial gastric conditions in vitro (7), studies in humans and other species have failed to demonstrate this conversion when CBD is administered orally (8–10). The hemp plant also produces other phytochemicals, such as terpenes and hydrocarbons, that may potentiate cannabinoid activity—known as the entourage effect (11).
Due to its psychoactive properties, THC is currently a schedule I controlled drug in the United States except for a few synthetic prescription-only forms (12, 13); however, there is a lack of federal regulation regarding the sale and use of CBD-containing products. The Agricultural Improvement Act of 2018 resulted in federal legalization of cannabinoid products containing less than 0.3% THC (14). In addition, other commercially available products widely vary in CBD concentration, recommended dose, and routes of administration. Independent analyzes of numerous products have also demonstrated inconsistencies between the CBD concentrations provided on the label and their actual contents (15, 16).
While the only current FDA-approved use for CBD is as an anticonvulsant to treat seizures associated with Lennox-Gastaut syndrome, Dravet syndrome, or tuberous sclerosis complex (17), recent studies have indicated additional therapeutic benefits of CBD including anti-inflammatory (18), antioxidant (19, 20), analgesic (21), antiemetic (22), anxiolytic (23), anticarcinogenic (24, 25), antimicrobial (26, 27), and immunomodulatory (21, 28) properties. More specifically, research in various species has evaluated its efficacy in treating diseases such as diabetes mellitus (29–31), osteoarthritis (21, 28, 32), and neurologic diseases (e.g., Alzheimer’s, Parkinson’s) (33–37). In addition, CBD has been proposed to treat symptoms associated with stress (38), gastrointestinal disturbances (39, 40), orofacial pain (41, 42), hypertension (43–45), and neoplastic processes (46). Prior to cisplatin chemotherapy in the house musk shrew (Suncus murinus; n = 20), intraperitoneal CBD demonstrated a biphasic response—low doses (5 mg/kg) reduced emesis and higher doses (40 mg/kg) potentiated it (22); similarly, prior to lithium chloride administration (n = 36), intraperitoneal CBDA significantly reduced the incidence of emesis at low doses (0.1–0.5 mg/kg) but increased dosing (5 mg/kg) did not significantly reduce emesis compared to the control (47). Far fewer studies have been conducted regarding the other therapeutic benefits of CBDA but include anti-inflammatory (48), analgesic (49, 50), anticonvulsant (51), and anxiolytic (52–54) properties. Compared to CBD, CBDA was a more potent antihyperalgesic (49) but was less effective in reducing cancer cell proliferation in vitro (24). Unheated hemp extract contained higher concentrations of CBDA than CBD but resulted in a higher maximum serum CBD concentration compared to heated hemp extract (1).
Overall, CBD is reportedly safe with minimal side effects or signs of toxicity. Most negative effects have been anecdotal, inconsistent among studies, or occurred with high doses or extended use. Rarely reported potential side effects include sedation, mild diarrhea, inappetence, agitation, hypersensitivity, poor sleep quality, ataxia, pyrexia, infection, dry mouth, head-shaking, and excessive licking (55–59). Mildly elevated serum liver enzymes, including alanine transaminase, alkaline phosphatase (ALP), and aspartate aminotransferase (AST), were reported at typical daily CBD doses (0.5–2.8 mg/kg) in some human, canine, and feline subjects (28, 60, 61); although the increases were statistically significant, they were not of clinical concern. Conversely, higher CBD doses (≥ 20 mg/kg) in humans may lead to drug-induced liver injury (62, 63). There is also evidence that CBD may interfere with some drug metabolism by inhibition of cytochrome P450 enzymes (64, 65). Therefore, caution should be used prior to concurrent administration with other drugs, especially those with hepatic metabolism or affected by cytochrome P450 inhibition.
There are no current studies published about the use of CBD or CBDA as a potential therapeutic agent in nonhuman primates (NHP); however, oral high-dose CBD (30–300 mg/kg) for 90 days (n = 16) did not affect rhesus macaque growth rates but increased liver and kidney weights, decreased testicular weight and inhibited spermatogenesis, dose-dependently decreased red blood cell counts, and occasionally resulted in transient diarrhea (66). Based on evidence of the therapeutic benefits in humans and other species (32, 38, 40), low-dose CBD could be a candidate as an adjunctive therapy for some common NHP medical conditions, including osteoarthritis (67), environmental stress (68), and inflammatory diarrheal disease (69). As a first step, a pharmacokinetic (PK) analysis of CBD and CBDA would inform whether doses recently reported in other species would be appropriate in macaques and provide a baseline for future studies evaluating its PK or therapeutic potential.
Based on the therapeutic doses of CBD and CBDA reported in other species (28, 55, 61, 70, 71), we selected a commercially available oral veterinary CBD-/CBDA-rich hemp oil (CBD/ArHO; approximately 1:1 CBD:CBDA) with a guaranteed content analysis (Supplementary Table S1) that was previously evaluated in domestic dogs (70). We also performed a pilot study at 2 mg/kg CBD/ArHO in cynomolgus macaques and determined that serum CBD concentrations were lower than in some species, but serum CBDA concentrations were 3.8 times higher than CBD (72). The goal of this study was to perform a PK analysis of CBD/ArHO in juvenile cynomolgus macaques (Macaca fascicularis). Our objectives were to (1) perform a 24-h, single-dose PK study at 4 or 8 mg/kg CBD/ArHO; (2) determine any physical, biochemical, or hematological effects over time; (3) determine if any cannabinoids accumulate in the serum after 14 days of daily dosing, and (4) compare the results to reported findings in other species. We hypothesized that 8 mg/kg CBD/ArHO would provide higher serum concentrations of CBD and CBDA than 4 mg/kg, with negligible amounts of serum THC, and CBDA being significantly higher than CBD. We also hypothesized that there would be minimal negative physical effects, and no clinically significant serum biochemical or hematological changes.
2 Materials and methods
2.1 Humane animal care and use
All procedures were approved by The Mannheimer Foundation IACUC, an AAALAC-accredited facility, and adhered to all approved standard operating procedures. Animals were maintained according to Animal Welfare Act (73, 74) and Regulations (75), and the Guide for the Care and Use of Laboratory Animals (76).
2.2 Animals
Male [n = 4; weight (mean ± 1 SD), 3.70 ± 0.67 kg] and female (n = 4; weight, 3.63 ± 0.32 kg) juvenile cynomolgus macaques (aged 3.29 ± 0.13 years) were selected. Inclusion criteria were subjects born at The Mannheimer Foundation, open to positive human interactions (determined by colony manager), and naïve to experimental and medical interventions outside of the routine preventative medicine program. This program included semiannual physical examination, tuberculin skin testing (10 μL intradermally every 6 months; Tuberculin OT, Colorado Serum Company, Denver, CO), deworming with ivermectin [0.4 mg/kg intramuscularly (IM) every 6 months; Vetrimec 1%, MWI Animal Health, Boise, ID], and routine vaccination against Clostridium tetani (0.5 mL IM every 5 years; tetanus toxoid, Fort Dodge Animal Health, Fort Dodge, IA), Measles morbillivirus [1 mL subcutaneously (SC) every 6 months; Vanguard DM, Zoetis, Parsippany, NJ], and Rabies lyssavirus (1 mL SC every 3 years; Rabvac 3, Elanco US, Fort Dodge, IA). Animals were serologically negative for Macacine herpesvirus 1, Simian retrovirus 1, Simian T-lymphotrophic virus 1, and Simian immunodeficiency virus, and identified by a SC passive integrated transponder (PetLink™ Slim, Datamars, Inc., Woburn, MA) from birth and a chest tattoo of their unique identification number after 6 months old.
Subjects were individually caught in nets, anesthetized with ketamine hydrochloride (10–15 mg/kg IM), boxed (Prima-Carrier, Primate Products, Immokalee, FL), and transferred from outdoor, same-sex and -age social housing units. Animals were weighed, physically examined, collared (medium, aluminum; Primate Products, Immokalee, FL), and same-sex pair-housed in 2 stainless-steel squeeze-back cages with an open pass-through door (floor area, 0.4 m2 each; height, 76.2 cm) in indoor, climate-controlled rooms [70.3–80.2°F (21.3–26.8°C); relative humidity, 40–95%] on a 12:12-h light:dark cycle (07:00–19:00); cages were sanitized daily and disinfected at least every 15 days. Animals were fed a standard commercial primate diet (5049, Lab Diet, St Louis, MO) twice daily, and watered free-choice through an automated watering system. Each animal was environmentally enriched with a mirror, plastic ball, foraging board, and daily forage, including seeds, fruit, popcorn, multigrain fruit cereal (Fruit Spins, Great Value, Bentonville, Arkansas), and FiberBites (ClearH2O, Westbrook, ME). Animals were observed at least once daily to assess and document mentation, feed consumption, hydration status, stool quality, and any behavioral or health concerns.
Subjects were individually acclimated to human interactions and trained by positive reinforcement with handfed forage at least once daily after temporary separation by closing the mesh pass-through door. Soft classical music was played during handling. After the animals readily took forage directly from the trainers’ hand, they were acclimated to the pole (Primate Products, Immokalee, FL). Once the animal ignored the pole when held by the trainers, the pole was latched transversely through the cage mesh until it was accepted as a neutral object. Then, the pole was repeatedly held as close to the collar as the animal would allow until it could be latched to the collar. Once latching and unlatching of the pole to the collar was repeatedly successful, the animals were trained to allow two trainers to each latch their pole on opposite sides of the collar, climb from their cage to the floor, sit on the primate restraint chair (Primate Products, Immokalee, FL) in the same animal room, and allow their collar to be secured into the chair for 10–15 min intervals. After study completion, macaques were anesthetized again with ketamine hydrochloride for collar removal, weighed, boxed, and returned to their outdoor enclosures.
2.3 Study design
Eight subjects were selected to receive 4 (2 male, 2 female) or 8 (2 male, 2 female) mg/kg of CBD/ArHO (70 mg/mL). Then, a randomized block design was applied. Animals were grouped by sex before being randomly assigned to dose groups using a random sequence generator;1 the order of subject dosing was also randomized. The CBD/ArHO was administered orally with a 12-gauge curved gavage needle and 1-mL syringe after chairing. Subsequently, each animal was accessed by employing the squeeze-back mechanism on cage to allow for daily dosing for the study duration unless the animal was chaired for blood collection. All doses of CBD/ArHO (ElleVet Sciences, South Portland, ME) were administered between 07:00 and 09:00 on a given day prior to feeding. After a maximum chairing time of 4 h, animals were returned to their individual enclosures until the next timepoint for sample collection. Feed was provided immediately after the first 4 h of chairing. CBD/ArHO was administered for 14 days to determine any acute or subacute physiologic parameters over time, or if there was a cumulative effect of repeated administration. A procedural timeline is provided in Figure 1.
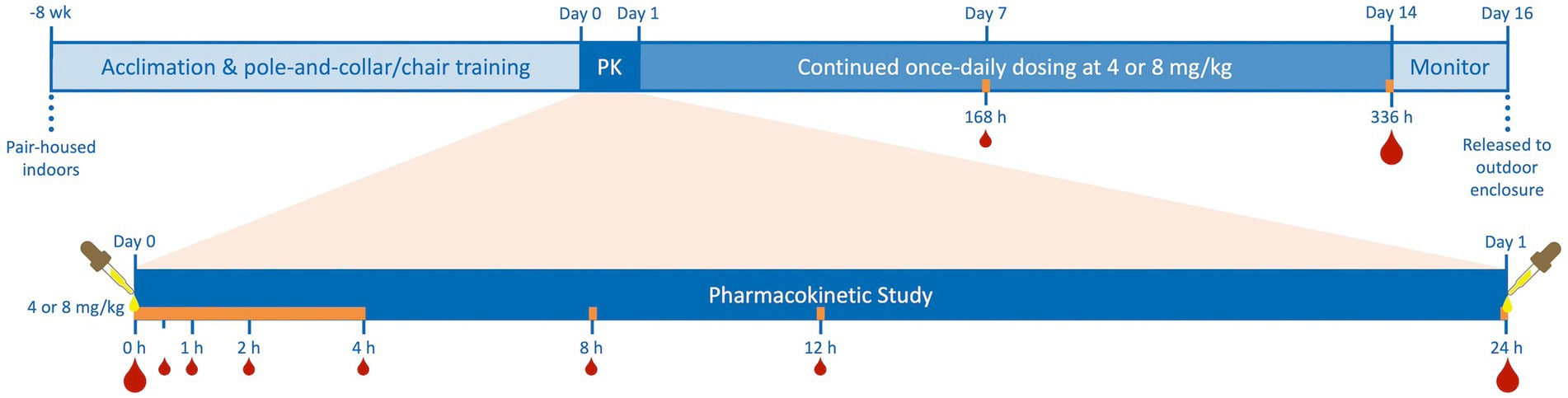
Figure 1. Timeline of juvenile cynomolgus macaque (4 males, 4 females) pair-housing, indoor acclimation, and pole-and-collar/chair training, 24-h pharmacokinetic study (PK) after single-dose oral administration of cannabidiol-/cannabidiolic acid-rich hemp oil (Day 0) at 4 or 8 mg/kg (2 males and 2 females per dose), continued once-daily dosing for an additional 13 days, and a two-day period of monitoring prior to release to their outdoor enclosures. Subjects were dosed daily at the same time as on their individual Day 0 and blood was always drawn immediately following the dose on Days 1, 7, and 14 as on Day 0. Blood was collected at 0-, 0.5-, 1-, 2-, 4-, 8-, 12-, 24-, 168-, and 336-h to quantify serum cannabinoid concentrations by high-performance liquid chromatography–tandem mass spectrometry. Additional blood was drawn for serum biochemistries and complete blood counts on Days 0, 1, and 14 (indicated by the larger blood drops). The orange bar represents the times of chair restraint for blood collection during the study, never exceeding 4 h.
Immediately following CBD/ArHO administration, blood was collected at Days 0 (0 h), 1 (24 h), and 14 (336 h) by femoral venipuncture using a 22-gauge vacuum phlebotomy system (Vacutainer, BD, Franklin Lakes, NJ), 4-mL serum-separator tube (8881302072, Medtronic, Minneapolis, MN), and 3-mL K2-EDTA tube (367856, Vacutainer, BD, Franklin Lakes, NJ). The serum tubes were held at 4°C for at least 30 min before centrifugation at 1,300 × g for 15 min at 4°C. The serum was divided into two 2-mL screw-top microtubes (72.694.406, Sarstedt, Nümbrecht, HR); one was stored at −80°C for PK analysis, while the other was refrigerated at 4°C with the K2-EDTA tube for serum biochemistry and complete blood count, respectively. Additional blood was collected at 0.5, 1, 2, 4, 8, and 12 h, and Day 7 (168 h) from alternating saphenous veins with a 25-gauge needle and 1-mL luer-lock syringe (ML12558, Air-Tite Products, Virginia Beach, VA); a cephalic or femoral vein was used if the saphenous collection failed. The blood was transferred to a 0.5-mL serum separator tube (365967, Becton Dickinson, Franklin Lakes, NJ) and held at 4°C for at least 30 min before centrifugation at 1,300 × g for 15 min at 4°C. Serum samples were transferred into sterile 2-mL screw-top microtubes and stored at −80°C until all samples were submitted for PK analysis.
2.4 Sample analysis
All samples were wrapped in an absorbable pad, placed in a 1-gallon Ziploc Freezer bag, and packaged in an insulating extruded polystyrene box within a cardboard box for overnight shipment according to the requirements of the International Air Transport Association Dangerous Goods Regulations for Category B biologic substances (UN 3373). Refrigerated serum and K2-EDTA samples were shipped with cold packs to VRL Laboratories (San Antonio, TX) for biochemistry and complete blood count (5506, Chem Profile II and CBC) within 48 h. Serum samples for cannabinoid analysis were shipped in dry ice (UN 1845) to the University of Illinois at Chicago Toxicology Research Laboratory.
Serum cannabinoid analyzes were performed using an exploratory (fit-for-purpose) method for fast measurement of 11 cannabinoids and their metabolites. The reference standards for CBD and CBDA were obtained from Restek Corporation (Bellefonte, PA); all other reference and internal standards were obtained from Cerilliant Corporation (Round Rock, TX). Cannabinoid serum concentration for CBD, CBDA, THC, THCA, cannabinol (CBN), cannabichromene, CBG, and CBGA and their metabolites 7-Nor-7-carboxycannabidiol (7-COOH-CBD), 11-nor-9-Carboxy-Δ9-tetrahydrocannabinol (COOH-THC), and 11-nor-9-Carboxy-Δ9-tetrahydrocannabinol-Glucuronide (COOH-THC-Glu) was determined using high-performance liquid chromatography–tandem mass spectrometry (Nexera X2 and LCMS 8050, Shimadzu Corp., Kyoto, Japan).
Each serum sample (40 μL) was mixed with internal standards (20 μL, 100 ng/mL each) in 1:1 water:methanol in a 96 well plate. Then, proteins were precipitated, and compounds were extracted by adding ice-cold acetonitrile (80 μL), vortexing for 1–2 min, and centrifuging at 4,000 rpm for 10 min at 4°C. Supernatants (70 μL) were diluted with deionized water (70 μL) in a another 96 well plate and centrifuged again. 10 μL of the processed samples were injected into a column (100 Å, 3 μm, 2.1 × 50 mm, Atlantis T3 column, Waters, Milford, MA) coupled to liquid chromatography–tandem mass spectrometry. A guard cartridge (100 Å, 3 μm, 2.1 × 5 mm, Atlantis T3 VanGuard, Waters, Milford, MA) was also used for columnar protection. The column was equilibrated with mobile phase A (0.1% formic acid in water) and mobile phase B (acetonitrile) at 50% B. The compounds were eluted by a linear gradient from 50% B to 95% B over 6 min, and then held at 95% B for 1 min. Subsequently, the column was reequilibrated at initial composition for 1 min at a flow rate of 0.3 mL/min. The autosampler and column temperature were set a 4°C and 30°C, respectively. The cannabinoids and their metabolites were detected in electrospray ionization positive or negative mode. Interface voltage was 4 kV or − 3.5 kV. Interface, desolvation line, and heat block temperatures were 300, 250, and 400°C, respectively. Nebulizing, heating, and drying gas flow were 2.7, 5, and 5 L/min, respectively. Serum cannabinoids concentrations were calculated by LabSolutions software (Shimadzu Corp., Kyoto, JP) using a quadratic calibration curve with 1/concentration2 weighing based on relative response (peak area of cannabinoids/peak area of internal standards). The reference standards, their multiple reaction monitoring, polarity, and retention time, internal standards and their multiple reaction monitoring and polarity, and calibration curve range are summarized in Table 1.
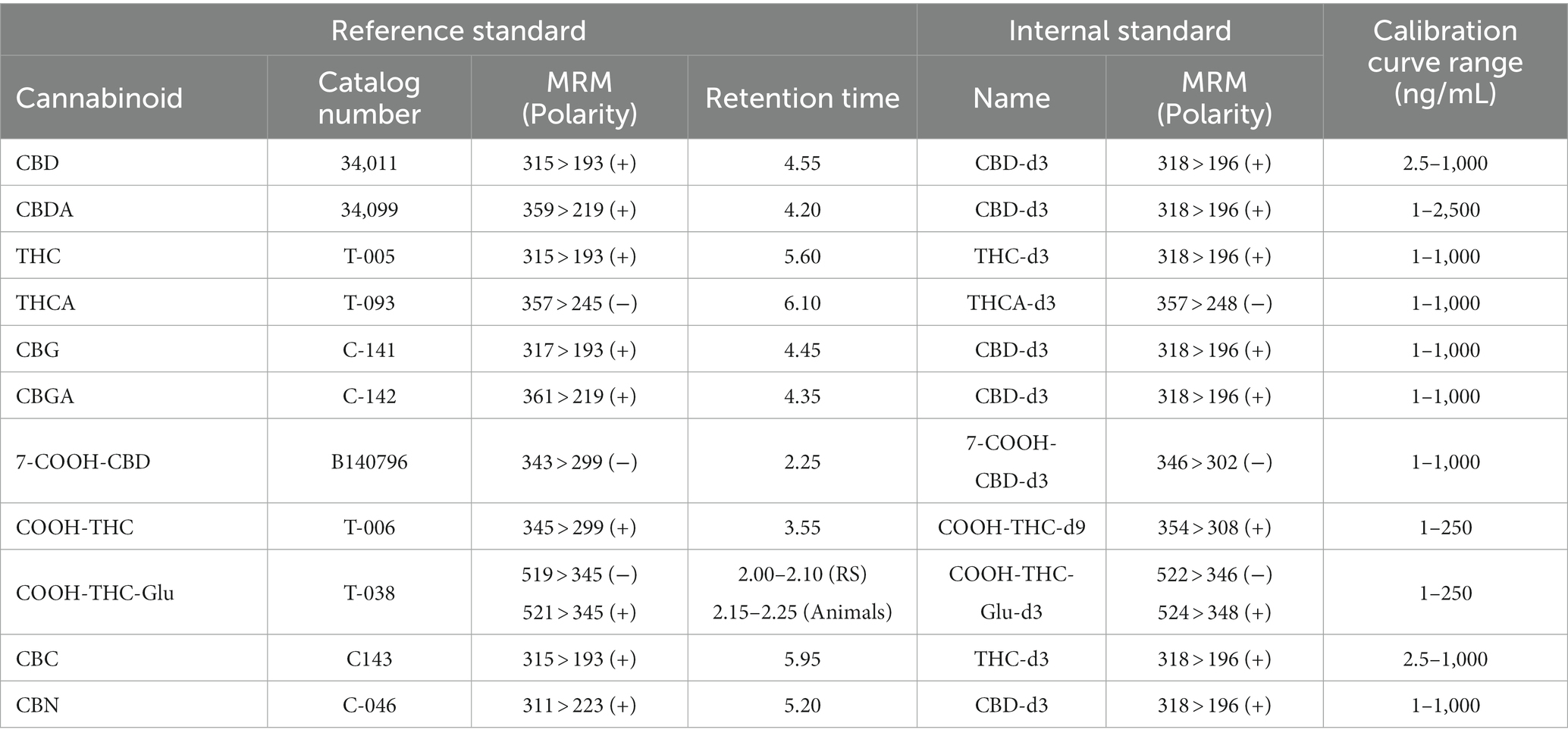
Table 1. High-performance liquid chromatography–tandem mass spectrometry reference standards (RS) for cannabidiol (CBD), cannabidiolic acid (CBDA), (−)-∆9-tetrahydrocannabinol (THC), ∆9-tetrahydrocannabinolic acid A (THCA), cannabigerol (CBG), cannabigerolic acid (CBGA), 7-Nor-7-carboxycannabidiol, (7-COOH-CBD), (+)-11-nor-9-Carboxy-Δ9-THC (COOH-THC), (+)-11-nor-9-Carboxy-Δ9-THC-Glucuronide (COOH-THC-Glu), cannabichromene (CBC), and cannabinol (CBN) with multiple reaction monitoring (MRM) and polarity, and retention time, as well as internal standards with MRM and polarity, and the overall calibration curve range (lower and upper limits of quantification) are presented.
2.5 Pharmacokinetic analysis
The PK analyzes were performed using Phoenix WinNonlin™ v8.3 (Certara, Princeton, NJ) by employing a plasma model (200–202) with extravascular dosing and the best fit method, which calculated the coefficient of determination (R2), maximum serum concentration (Cmax; ng/mL), time to maximal serum concentration (Tmax; h), and half-life of the terminal phase (t1/2–λz; h); a linear trapezoidal linear interpolation was used to calculate the area under the curve until the last measurement (AUClast; h·ng/mL), area under the moment curve until the last measurement (AUMClast; h2·ng/mL) and mean residence time until the last measurement (MRTlast; h). All values below the quantification level (BQL) before Cmax were set to zero. The first value BQL after Cmax was calculated as half of the lower limit of quantification (Table 1) for each cannabinoid; the subsequent values BQL were set to zero. Based on a limited sample size, lack of statistical difference by sex, and relatively low serum cannabinoid concentrations resulting in too few data points to calculate all non-compartmental analysis parameters by individual, the mean data were used for group analysis.
2.6 Statistical analysis
Mixed-effects models with the Geisser–Greenhouse correction were performed using Prism v10.0.0 for macOS X (GraphPad, Boston, MA) to determine statistical significance (p ≤ 0.05). Random effects zero or less were removed to simplify the model. The independent variables included CBD/ArHO dose (mg/mL), sex (male, female), and time (h). Dependent variables included serum cannabinoid concentrations (ng/mL), serum biochemical concentrations, and complete blood count parameters, which were square-root transformed to improve normality. Residual plots were used to confirm model correctness and Quantile-Quantile plots of predicted versus actual residuals were used to confirm distribution normality.
3 Results
3.1 Pharmacokinetic study
All animals were bright, alert, and responsive throughout the study. During daily observations, they consistently maintained normal hydration, appetites, and stool quality. Most animals were reluctant to take the CBD/ArHO, many of whom turned their heads away from the gavage needle and syringe, and actively pushed them away during administration. Immediately following administration of the CBD/ArHO, some animals had mild hypersalivation, which resolved prior to the next blood collection timepoint.
3.1.1 Serum cannabinoids
Each cannabinoid significantly differed over time (p ≤ 0.0017). There were no significant differences between males and females for any of the cannabinoids. CBDA was the only cannabinoid that significantly differed by dose over time (p = 0.0361); the 8 mg/kg dose was significantly higher than the 4 mg/kg dose. The PK curves for CBD, CBDA, THC, THCA, and 7-COOH-CBD are displayed in Figure 2. The PK results for all detectable cannabinoids are summarized in Table 2 and the serum cannabinoid concentrations on Days 1, 7, and 14 are summarized in Table 3.
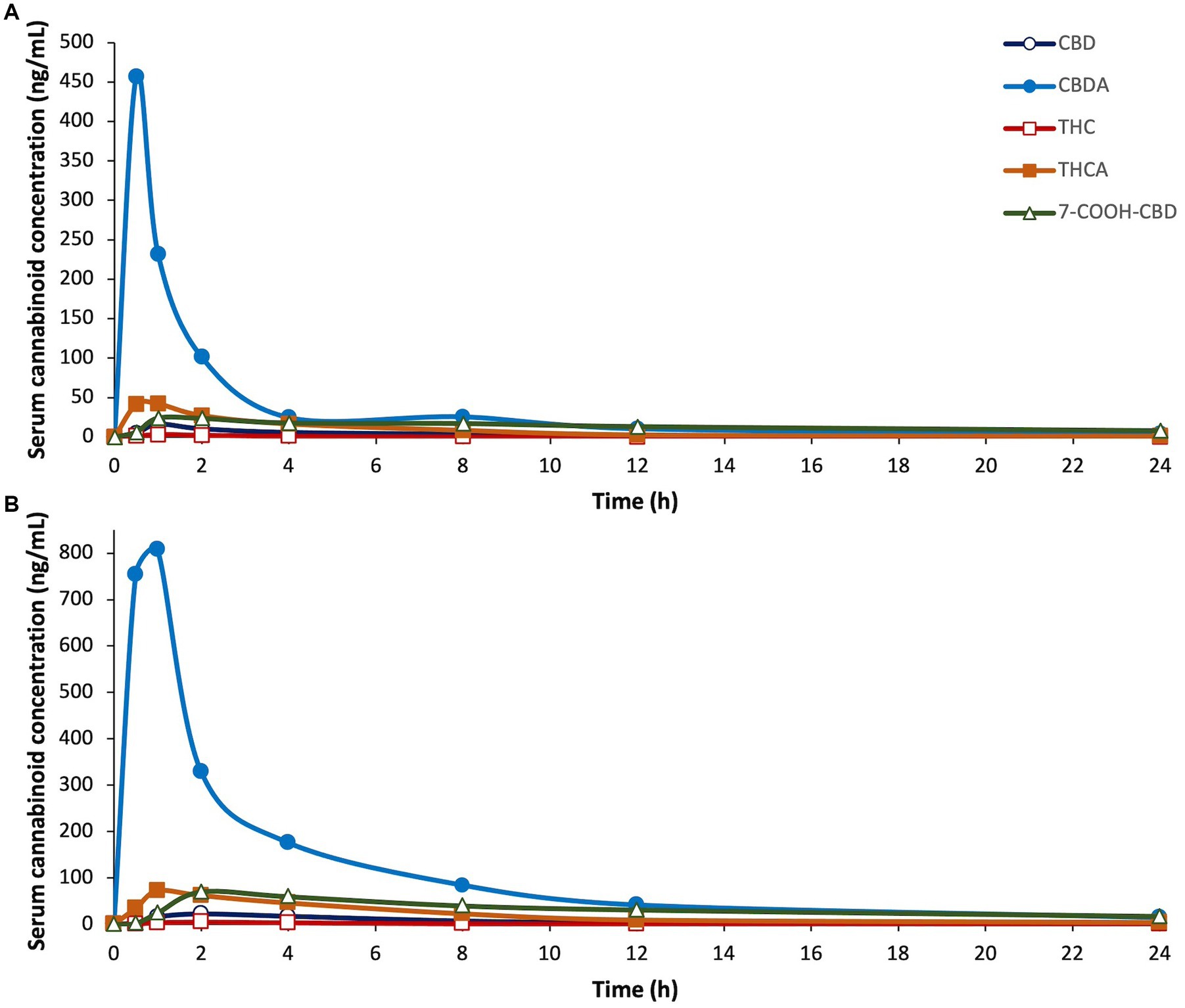
Figure 2. Serum concentrations of cannabidiol (CBD), cannabidiolic acid (CBDA), ∆9-tetrahydrocannabinol (THC), tetrahydrocannabinolic acid (THCA), and 7-carboxy cannabidiol, (7-COOH-CBD), after a single dose of oral administration of cannabidiol-/cannabidiolic acid-rich hemp oil at (A) 4 or (B) 8 mg/kg to juvenile cynomolgus macaques (n = 4 per dose) over 24 h. Note differences in the X-axes due to the sizeable increases in serum cannabinoid concentrations between doses. Each cannabinoid significantly differed over time (p ≤ 0.0017).
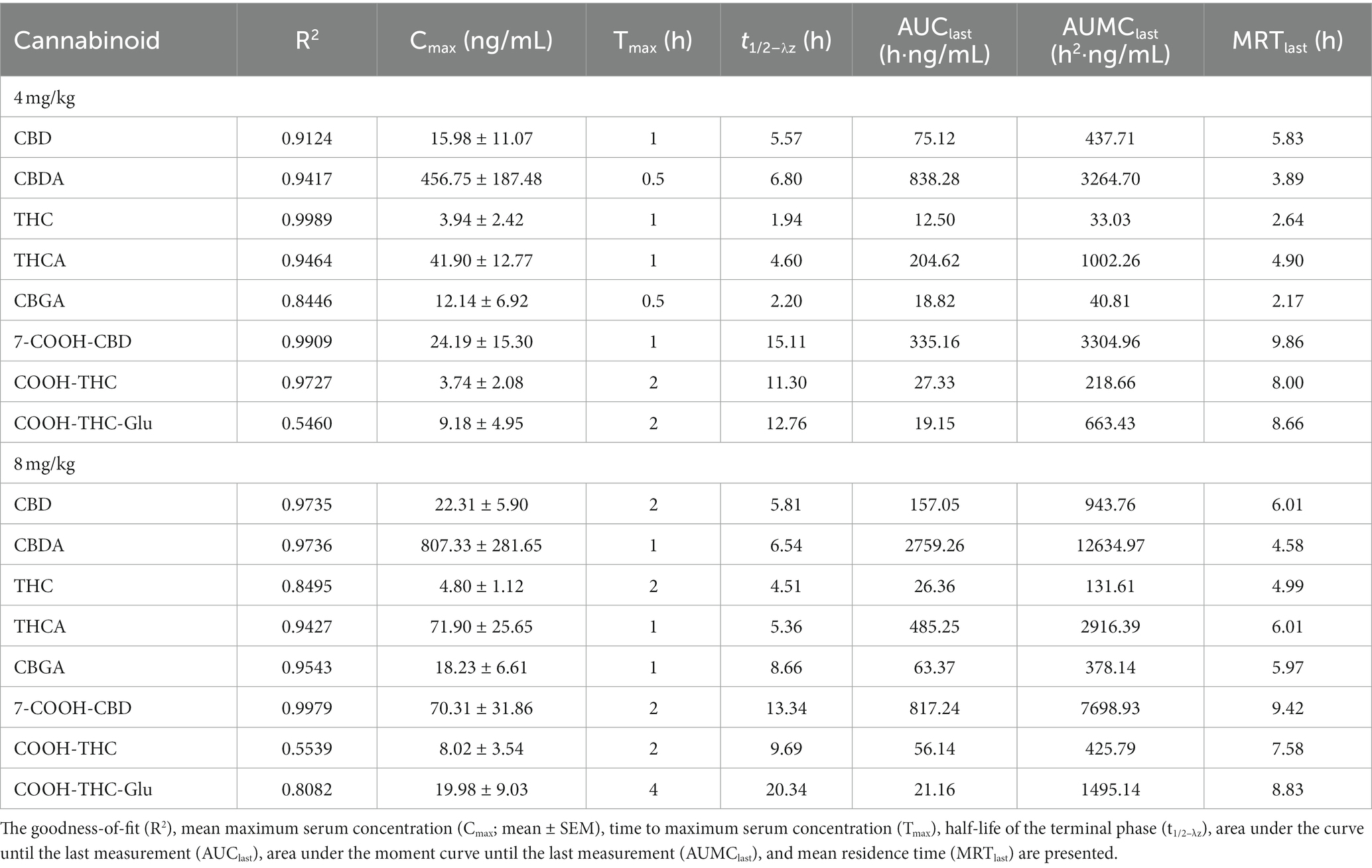
Table 2. Pharmacokinetic summary of cannabidiol (CBD), cannabidiolic acid (CBDA), ∆9-tetrahydrocannabinol (THC), ∆9-tetrahydrocannabinolic acid (THCA), cannabigerolic acid (CBGA), 7-Nor-7-carboxycannabidiol (7-COOH-CBD), 11-nor-9-Carboxy-Δ9-tetrahydrocannabinol (COOH-THC), and 11-nor-9-Carboxy-Δ9-tetrahydrocannabinol-Glucuronide (COOH-THC-Glu) after a single dose of oral administration of cannabidiol-/cannabidiolic acid-rich hemp oil at 4 or 8 mg/kg to juvenile cynomolgus macaques (n = 4 per dose) over 24 h.
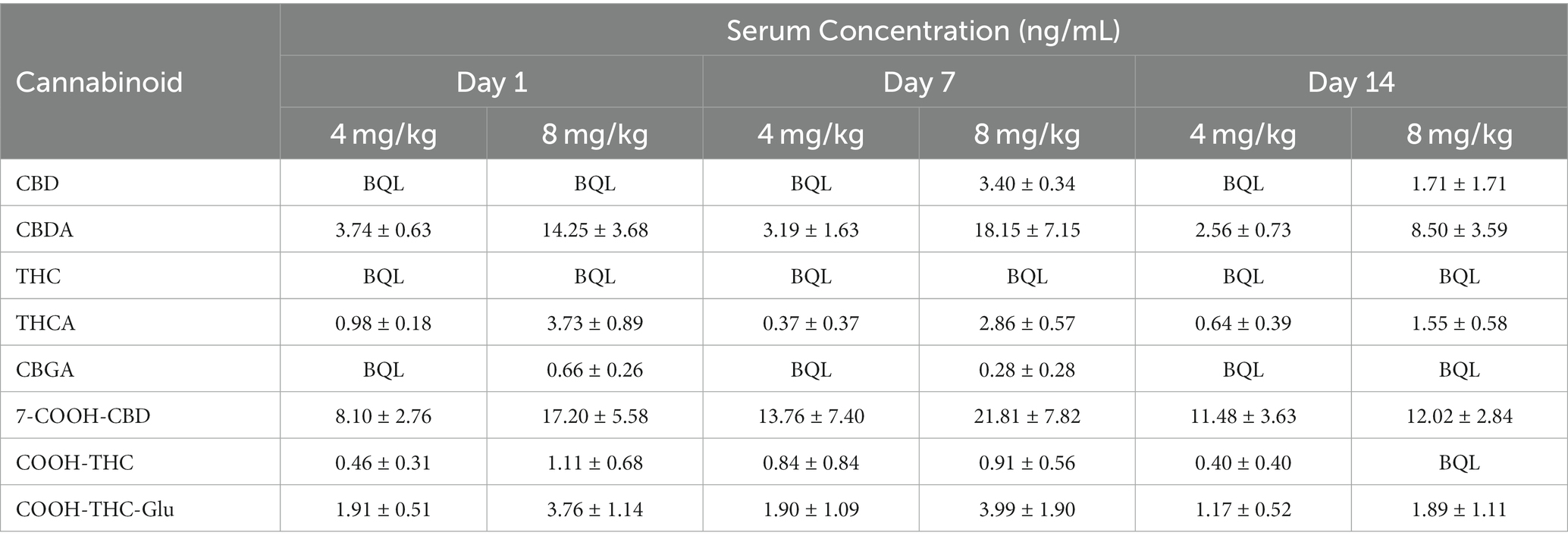
Table 3. Mean serum cannabinoid concentration (mean ± SEM) of cannabidiol (CBD), cannabidiolic acid (CBDA), ∆9-tetrahydrocannabinol (THC), ∆9-tetrahydrocannabinolic acid (THCA), cannabigerolic acid (CBGA), 7-Nor-7-carboxycannabidiol (7-COOH-CBD), 11-nor-9-Carboxy-Δ9-tetrahydrocannabinol (COOH-THC), and 11-nor-9-Carboxy-Δ9-tetrahydrocannabinol-Glucuronide (COOH-THC-Glu) after once daily oral administration of cannabidiol-/cannabidiolic acid-rich hemp oil at 4 or 8 mg/kg to juvenile cynomolgus macaques (n = 4 per dose) on Days 1, 7, and 14 at 24, 168, and 336 h, respectively.
For CBD, the model’s goodness-of-fit was R2 ≥ 0.91 at both doses. The Cmax was 1.4 times higher at 8 mg/kg than at 4 mg/kg. The Tmax occurred at 1 h at 4 mg/kg, and 2 h at 8 mg/kg. At 8 mg/kg, the AUClast was 2.1 times, and the AUMClast was 2.2 times, higher than at 4 mg/kg. At 4 mg/kg, the t1/2–λz was 0.24 h less, and the MRT was 0.18 h less, than at 8 mg/kg; the t1/2–λz was 0.26 h less than the MRT at 4 mg/kg and 0.20 h less than the MRT at 8 mg/kg. At 4 mg/kg, the Cmax for one animal was at least 5.0 times higher than any other individual Cmax, and 8.0 times higher than any other animal at the 1-h timepoint. At 8 mg/kg, the Cmax for one animal was at least 1.9 times lower, and its Tmax was at least 6 h later, than any other individual. By the 24-h timepoint, all serum CBD concentrations were BQL and remained BQL at 4 mg/kg. On Day 7 at 8 mg/kg, all serum CBD concentrations were 2.73–4.23 ng/mL; however, on Day 14 at 8 mg/kg, the serum CBD concentration was BQL for all but one animal (less than 6.85 ng/mL).
For CBDA, the model’s goodness-of-fit was R2 ≥ 0.94 at both doses. The Cmax was 1.8 times higher at 8 mg/kg than at 4 mg/kg. The Tmax occurred at 0.5 h at 4 mg/kg, and 1 h at 8 mg/kg. At 8 mg/kg, the AUClast was 3.3 times, and the AUMClast was 3.9 times, higher than at 4 mg/kg. At 4 mg/kg, the t1/2–λz was 0.26 h greater, and the MRT was 0.69 h less, than at 8 mg/kg; the t1/2–λz was 2.91 h greater than MRT at 4 mg/kg and 1.96 h greater than MRT at 8 mg/kg. At 4 mg/kg, the Cmax for one animal was at least 2.5 times higher than any other individual Cmax, while another was at least 3.8 times lower than any other individual Cmax. At 8 mg/kg, the individual Cmax values ranged from 223.54 ng/mL at 1 h to 1391.74 ng/mL at 0.5 h. By the 24-h timepoint, all serum CBDA concentrations ranged from 1.92–4.73 ng/mL at 4 mg/kg and 5.47–23.34 ng/mL at 8 mg/kg. On Day 7, the serum CBDA concentration for only one individual (at 4 mg/kg) was BQL; similarly, on Day 14, the serum CBDA concentration for only one individual (at 8 mg/kg) was BQL.
For THC, the model’s goodness-of-fit was R2 = 0.9989 at 4 mg/kg and R2 = 0.8395 at 8 mg/kg. The Cmax was 1.2 times higher at 8 mg/kg than at 4 mg/kg. The Tmax occurred at 1 h at 4 mg/kg, and 2 h at 8 mg/kg. At 8 mg/kg, the AUClast was 2.1 times, and the AUMClast was 4.0 times, higher than at 4 mg/kg. At 4 mg/kg, the t1/2–λz was 2.57 h less, and the MRT was 2.35 h less, than at 8 mg/kg; the t1/2–λz was 0.70 h less than the MRT at 4 mg/kg and 0.48 h less than the MRT at 8 mg/kg. The Cmax for one animal that received 4 mg/kg was BQL at all timepoints, while another that received 8 mg/kg was the only animal with detectable serum THC concentrations by the 8-h timepoint (less than 2.99 ng/mL). All serum THC concentrations were BQL for all animals at the 24-h, 7-day, and 14-day timepoints.
For THCA, the model’s goodness-of-fit was R2 ≥ 0.94 at both doses. The Cmax was 1.7 times higher at 8 mg/kg than at 4 mg/kg. The Tmax occurred at 1 h at both doses. At 8 mg/kg, the AUClast was 2.4 times, and the AUMClast was 2.9 times, higher than at 4 mg/kg. At 4 mg/kg, the t1/2–λz was 0.76 h less, and the MRT was 1.11 h less, than at 8 mg/kg; the t1/2–λz was 0.30 h less than the MRT at 4 mg/kg and 0.65 h less than the MRT at 8 mg/kg. At 4 mg/kg, the Cmax for one animal was at least 2.5 times higher than any other individual Cmax and 5.8 times higher than any other animal at the 0.5-h timepoint; by the 2-h timepoint, the serum THCA concentration of that animal was less than the mean. The Cmax for another animal at 4 mg/kg was 5.3 times lower than any other Cmax. On Day 7, the serum THCA concentration was BQL for all but one animal (less than 1.50 ng/mL) at 4 mg/kg and none of the animals at 8 mg/kg; however, on Day 14, all but one individual at 4 mg/kg, and only one animal 8 mg/kg, had serum THCA concentrations less than 1.00 ng/mL.
For 7-COOH-CBD, the model’s goodness-of-fit was R2 ≥ 0.99 at both doses. The Cmax was 2.9 times higher at 8 mg/kg than at 4 mg/kg. The Tmax occurred at 1 h at 4 mg/kg and 2 h at 8 mg/kg. At 8 mg/kg, the AUClast was 2.4 times, and the AUMClast was 2.3 times, higher than at 4 mg/kg. At 4 mg/kg, the t1/2–λz was 1.77 h greater, and the MRT was 0.44 h greater, than at 8 mg/kg; the t1/2–λz was 5.25 h greater than the MRT at 4 mg/kg and 3.92 h greater than the MRT at 8 mg/kg. At 4 mg/kg, the Cmax for one animal was at least 2.3 times higher than any other individual Cmax and 4.6 times higher than any other animal at the 1-h timepoint. The Cmax and Tmax results at 8 mg/kg ranged widely by individual: 151.02 ng/mL at 2 h, 103.05 ng/mL at 4 h, 31.99 ng/mL at 2 h, and 16.70 ng/mL at 8 h. Once the serum 7-COOH-CBD concentration was above the BQL, it did not fall below BQL in any subsequent measurements.
3.1.2 Serum biochemistry and complete blood count
A total of 22 biochemistry analytes were evaluated on Days 0, 1, and 14 and summarized in Supplementary Table S2. Of the liver parameters, alanine transaminase and total bilirubin did not significantly differ by time or sex. ALP (p = 0.0218) and gamma-glutamyl transferase (GGT; p = 0.0087) significantly differed by sex. The mean ALP was consistently higher in males than females. One male at 4 mg/kg (898 U/L) and another at 8 mg/kg (954 U/L) were higher than the recommended ALP reference range at Day 0, and the other male at 8 mg/kg (1,392 U/L) was higher than the recommended ALP reference range on Day 1. ALP was within the recommended reference range for all individuals on Day 14. GGT was consistently higher in all males than females, but all values were still within the recommended reference range. AST significantly differed over time (p = 0.0094). The mean AST was 1.9–2.5 times higher at Day 1 compared to Day 0 and Day 14; one individual at 4 mg/kg and two individuals at 8 mg/kg were higher than the recommended reference range at Day 1. AST was within the recommended reference range for all individuals by Day 14. All liver parameters are summarized in Table 4.
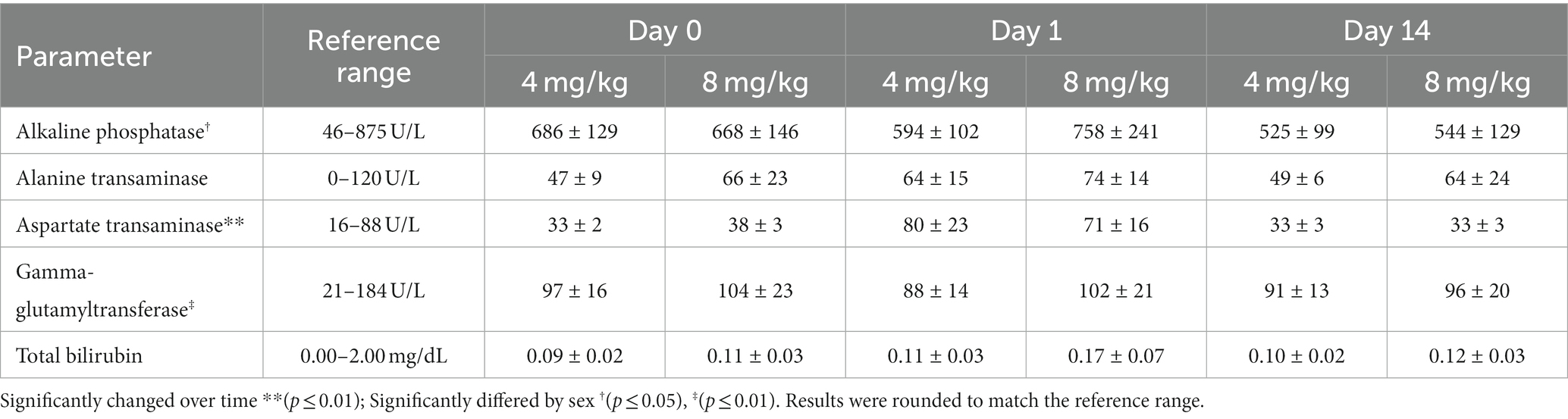
Table 4. Serum liver biochemistry analytes (mean ± SEM) after once daily oral administration of cannabidiol-/cannabidiolic acid-rich hemp oil at 4 or 8 mg/kg to juvenile cynomolgus macaques (n = 4 per dose) on Days 0, 1, and 14.
The complete blood count parameters are summarized in Supplementary Table S3. Three Day 14 samples (2 females at 4 mg/kg, 1 female at 8 mg/kg) submitted for hematologic analysis were reported as frozen by the laboratory, invalidating the results, and excluding them from analysis. No parameters significantly differed by dose.
4 Discussion
Few studies have been published regarding the PK or use of CBD in NHP. The PK of intravenous (1.4 mg/kg; n = 2) and oral (114 mg/kg; n = 1) CBD administration in rhesus macaques (Macaca mulatta) were reported while evaluating if electron-capture gas chromatography could be used to analyze serum CBD concentrations (77). The PK of a combination product, CBD (3 mg/kg) and THC (1 mg/kg), administered IM daily for 4 months were determined in common squirrel monkeys (Saimiri sciureus; n = 4) (78). High-dose CBD (150–300 mg/kg) administered intravenously to rhesus macaques (n = 12) resulted in a median lethal dose of 212 mg/kg, and side effects included tremors, emesis, and abnormal respiratory rate (66). Finally, multiple NHP studies have demonstrated that CBD can attenuate the behavioral and neurological (cognition, memory, task performance) effects of THC (78–82).
In addition to NHP, PK studies of CBD or CBDA have been conducted in dogs (28, 55, 70, 71), cats (55, 61, 83), humans (84–86), horses (87, 88), cows (89–91), rabbits (92), guinea pigs (93), rats (94), mice (51), and parrots (95). The CBD doses examined have been highly variable, ranging from 0.5–300 mg/kg in humans (96). Except for Epidiolex, an oral CBD oil, CBD and CBDA products are not FDA approved or regulated, which can affect quality and cannabinoid concentrations. Other products vary in form and administration route, including oral chews (70), oral pastes (61), oral soft gels (71), transmucosal sprays (21), transdermal gels (97), inhalational powders (98), and SC (94), IM (80), intraperitoneal (49), or intravenous (77) injections, which affect serum cannabinoid concentrations and bioavailability.
Based on the CBD:CBDA ratio of the CBD/ArHO used in this study, administration at a 4 mg/kg dose equated to approximately 2 mg/kg of each CBD and CBDA; these doses must be considered when comparing our findings with other studies, especially those that utilized pure CBD isolates. There is conflicting evidence of the pharmacokinetic interactions of CBD and CBDA when administered in a multi-cannabinoid product compared to a CBD isolate (1, 99).
4.1 Pharmacokinetic study
Based on our pilot study (72), low-dose human recommendations (84–86, 100), and other studies (61, 70), increased doses of 4 and 8 mg/kg/day were selected for our PK study. Throughout this study, the animals actively avoided direct-to-mouth CBD/ArHO administration and mildly hypersalivated post-administration, a reported sign of unpalatability in macaques (101). Similarly, cats were reported with signs associated with unpalatability (i.e., lip-licking, head-shaking, and drooling) post-CBD/ArHO administration (55). In addition, use of the squeeze-back cage mechanism was necessary for dosing and likely resulted in increased environmental stress compared to more passive drug delivery systems; however, no additional adverse effects were observed, supporting the relative tolerance of CBD/ArHO.
Due to the number of animals available of similar age and weight meeting inclusion criteria, sample size in this study was low; this, the need to group individuals for the PK analysis due to the frequency of cannabinoid BQL values, and drastic differences in individual Cmax or Tmax skewed the overall means, limited statistical power, and prevented identification and exclusion of outliers. High inter-subject variability in cannabinoid concentration has been reported previously in humans (86).
4.1.1 Serum cannabinoids
While all serum cannabinoid concentrations were higher at 8 mg/kg than 4 mg/kg, serum CBDA concentration over time was the only statistically significant difference detected between doses (p = 0.0361). At 8 mg/kg, the Cmax of CBD, CBDA, THC, THCA, and CBGA were less than twice those at 4 mg/kg, while the Cmax for 7-COOH-CBD, COOH-THC, and COOH-THC-Glu were more than twice those at 4 mg/kg. While a linear relationship between dose and AUC of cannabinoids has been reported (86), other studies have reported a less than dose-proportional increase in Cmax and AUC as dose increased, suggesting the potential of dose-based difference in bioavailability or metabolism (102). Interestingly, at twice the dose, our results found that the Cmax for CBD and CBDA less than doubled, while the AUC was 2.1–3.3 times higher.
One PK analysis in dogs at 8 mg/kg had a Cmax for CBD of 591 ng/mL (28), 26.5 times higher than in our subjects, indicating a higher absorption and bioavailability, or differences in metabolism, distribution, or elimination, compared to cynomolgus macaques. Cats have a lower serum concentration of cannabinoids compared to dogs but varied by study. The Cmax of an orally administered pure CBD isolate in oil was 17.8 ng/mL at 2.5 mg/kg, 61.1 ng/mL at 5 mg/kg, and 132.6 ng/mL at 10 mg/kg (83). In another study administering CBD/ArHO at 2 mg/kg, the Cmax of CBD was 43 ng/mL in cats, 6 times lower than dogs in the same study (55); however, this Cmax was twice that of the Cmax in our subjects at 8 mg/kg. In a third study administering an oral paste at approximately 2.5 mg/kg (1.37 mg/kg CBD + 1.13 mg/kg CBDA), the Cmax of CBD was 6.6 times higher than cats receiving 2 mg/kg CBD/ArHO (55, 61). In humans, pure CBD at 1.25 mg/kg resulted in a serum CBD concentration of 37.6 ng/mL at the 2.5-h timepoint, closer to our subjects, albeit 1.7 times higher than our 8 mg/kg CBD/ArHO (86). At a 200 mg/subject dose, the Cmax was 153 ng/mL in healthy patients (85), similar to dogs dosed with CBD/ArHO at 2 mg/kg (28, 70).
The Cmax of CBDA in cats at 2.5 mg/kg (1.37 mg/kg CBD + 1.13 mg/kg CBDA) of oral paste was higher (1011.3 ng/mL) than for our subjects (807.33 ng/mL) at 8 mg/kg CBD/ArHO, although the AUClast in our subjects was minimally higher (2759.26 ng/mL) than in cats (2638.7 ng/mL) (61). This suggests that a 4 times higher dose would be required to reach the same CBDA absorption. The Cmax for CBDA was at more than 28.6 times that of CBD, indicating a higher oral absorption and bioavailability of CBDA, consistent with cannabis extract administration in humans (103); however, few studies have determined the therapeutic dose for CBDA. In dogs, the Cmax for CBDA was 2–6 times higher than CBD when administered at 2 mg/kg (70, 71).
In our study, the Tmax was 1–2 h for CBD, and 0.5–1 h for CBDA while the t1/2–λz was 5.57–5.81 h for CBD and 6.54–6.80 h CBDA. While no animal fell BQL for CBDA by the 24-h timepoint, for CBD, two individuals at 4 mg/kg were BQL at the 8-h timepoint, and one animal at 8 mg/kg was BQL at the 12-h timepoint; thus, dosing every 6–12 h would provide better coverage. Twice daily dosing was most reported in dogs (28), cats (55), and humans (102), was therapeutically efficacious in dogs and humans, and may be a more appropriate dosing regimen for NHP.
With the exception of THCA at 4 mg/kg, the Tmax of the cannabinoid acids (CBDA, THCA, and CBGA) were earlier than the other cannabinoids detected, indicating rapid serum absorption as also reported in mice (51); however, in our study, the t1/2–λz of cannabinoid acids was longer than the corresponding neutral cannabinoid. In this study, the serum CBDA concentration was 10.9–11.2 times higher than the second highest cannabinoid, THCA, and 28.6–36.2 times higher than CBD, despite approximately equal concentrations of CBD and CBDA in the CBD/ArHO. Like CBDA, the THCA Cmax was higher than THC, consistent with a similar study in dogs and indicating better absorption (70). As in other studies using CBD/ArHO (70, 71), serum THC concentrations were considerably lower than other metabolites, reaching less than 4.81 ng/mL even at 8 mg/kg; given the low THC concentration (0.15 weight percent) in the CBD/ArHO, this was expected.
In humans, 7-COOH-CBD is the major circulating cannabinoid (85, 86, 102). At 1.25 mg/kg of a pure CBD isolate, the serum 7-COOH-CBD (157 ng/mL) concentration was 4.2 times higher than circulating CBD (37.6 ng/mL) (86). Conversely, at 2 mg/kg CBD/ArHO, the 7-COOH-CBD Cmax (13 ng/mL) in dogs was 9.5 times lower than that of CBD (124 ng/mL) (70), and, at 2.5 mg/kg (1.37 mg/kg CBD + 1.13 mg/kg CBDA), the 7-COOH-CBD Cmax (41.4 ng/mL) in cats was 6.8 times lower than CBD (282 ng/mL) (61). More similarly to humans but at much lower serum concentrations, the 7-COOH-CBD Cmax (24.19 ng/mL) in our study was 1.5 times the CBD Cmax (15.98 ng/mL) at 4 mg/kg and 3.2 times (70.31 ng/mL) the CBD Cmax (22.31 ng/mL CBD) at 8 mg/kg. On Days 1, 7, and 14, 7-COOH-CBD was higher than serum CBD and CBDA concentrations, potentially due to conversion to or reduced elimination of 7-COOH-CBD. Despite its persistent serum concentration, 7-COOH-CBD was not responsible for the anticonvulsant effects of CBD in animals (104).
4.1.2 Serum biochemistry and complete blood count
Of the liver parameters, males had significantly higher ALP and GGT levels compared to females. Male cynomolgus macaques (after 36 months of age) and humans have also been reported to have higher ALP and GGT than females; ALP elevations also occur in young animals due to bone growth (105–107). AST was the only analyte that significantly differed over time (p = 0.0094). While AST is a biomarker of hepatocellular injury, elevations may also be due to normal variation, hemolysis, exercise, or muscle injury or disease. Concurrently elevated CK, as seen in 2 of the 3 animals at Day 0, often occurs in myopathies or issues with phlebotomy technique. Without elevations in alanine transaminase, high AST is less likely related to hepatopathy (108). After 2-week administration of CBD/ArHO, our results indicated that no clinically significant biochemical changes occurred over time.
Three complete blood count samples collected on Day 14 (all female, two at 4 mg/kg, one at 8 mg/kg) froze between shipment and analysis. This resulted in artificially low hematologic parameters, especially for the white blood cells. Similarly, whole-blood storage at −70°C for 15–30 days prior to a complete blood cell count significantly lowered all mean parameters other than hemoglobin and platelet count compared to fresh whole blood (109). After 2-week administration of CBD/ArHO, our overall results indicated that no clinically significant hematological changes occurred over time.
4.2 Other considerations
As terpenes are likely responsible for the smell and flavor of cannabinoid products, contribute to their bitter and unpleasant taste, and reduce patient compliance, the removal of terpenes from the formulation could improve palatability; however, given that terpenes may increase cannabinoid efficacy due to the entourage effect (11), this could reduce dose potency. Due to these concerns and to reduce stress associated with direct-to-mouth administration, we attempted CBD/ArHO administration in a 10-mL gelatin-based gummy vehicle; however, we encountered additional compliance issues including wide-ranging consumption times or refusal to consume the gummy at all. Alternatively, combining the CBD/ArHO with other, more palatable or aromatic substances such as peppermint oil may mask its bitter taste and improve palatability (110).
The pharmacokinetics of cannabinoids vary based on diet and feeding schedule. Feeding a high-fat diet increased serum CBD concentrations in dogs (70) and humans (102, 111). Conversely, feeding rabbits immediately after CBD/ArHO administration resulted in decreased serum CBD and CBDA concentrations (92). Unlike dogs and humans, rabbits are hindgut fermenters and consume a high-fiber diet, which may absorb the oil. Based on the results in non-hindgut fermenters, feeding our cynomolgus macaques could improve the Cmax of cannabinoids, as in dogs and humans. On collection days, feed was provided after the 4-h collection; however, feed consumption varied among individuals over the course of each day, so some animals may have had more feed in their gastrointestinal tract than others. Providing a high-fat meal prior to dosing could decrease inter-individual variability and increase absorption.
Although cannabinoid serum accumulation did not appear to occur in our study, the once daily dosing may have been insufficient for this effect. Given that CBD was BQL by the 24-h timepoint, increased CBD/ArHO dosing frequency and duration would be necessary to demonstrate any cumulative effects on serum cannabinoid concentration. Human studies suggest a minimal to moderate CBD accumulation over time (102, 112), but metabolites such as 7-COOH-CBD have greater accumulation (86).
Therapeutic doses and serum concentrations of cannabinoids have not been determined in most species, including macaques. For CBD, the recommended therapeutic doses and serum concentrations vary in reports in other species depending on its intended use. In humans, plasma CBD concentrations of 100 ng/mL were effective in reducing seizures and doses up to 50 mg/kg had a linear increase in plasma concentration and efficacy (100). In dogs, 2 mg/kg of CBD resulted in a median Cmax of 102 ng/mL and twice daily dosing effectively reduced osteoarthritis-related discomfort (28). In our subjects at 8 mg/kg, the Cmax of CBD only reached 22 ng/mL, 4.5 times less, suggesting that it would be a subtherapeutic dose if efficacy is similar to dogs and humans.
A biphasic or inverted U-shaped curve effect of CBD and other cannabinoids has been reported in multiple species. While anxiolytic or anti-emetic at certain doses, at some point increasing or decreasing the dose had no effect or even exacerbated the condition (22, 113). Doses of 0.1 and 0.5 mg/kg CBDA were effective in reducing vomiting in house shrews; however, vomiting was comparable to the control at 5 mg/kg (47). Similarly, 0.01 mg/kg of oral CBDA sufficiently reduced hyperalgesia in rats but 1 mg/kg did not (49). Little additional information is currently available regarding this effect; therefore, developing the therapeutic dose of CBD and CBDA in macaques should account for these potential issues.
As they are lipid soluble, cannabinoid concentrations should also be quantified in excreta, adipose, and target tissues (114). Cannabinoids concentrations in target tissues, such as the brain (51), joints (93), or gastrointestinal tract could affect efficacy. Histopathology could help evaluate potential beneficial or deleterious effects to healthy or disease-affected tissues after CBD/ArHO administration and determine therapeutic doses (115).
Other studies have shown anatomical and physiological side effects (66), and biochemical changes (28), after 30 days of dosing. Although our study did not detect any clinically significant changes in liver parameters, changes could be seen after long-term administration; therefore, it should be used cautiously in animals with hepatopathies, prone to liver failure, or receiving other treatments that involve cytochrome P450 mechanisms. Additionally, its use in NHP breeding colonies at lower doses should be evaluated, as high doses were shown to reduce spermatogenesis (66).
4.3 Conclusion
Due to poor regulation of commercially available products, which are frequently mislabeled and often widely variable in cannabinoid composition, products which provide a guaranteed analysis by an independent laboratory are most reliable. Clinical studies are needed to determine the therapeutic dose of CBD and CBDA for macaques, which may differ based on the disorder targeted. Additionally, CBD/ArHO should be evaluated as an adjunctive therapy in non-human primates. Given the low serum CBD concentrations, the doses and frequency used in this study may be insufficient for a therapeutic effect; however, if CBDA has similar therapeutic benefit to CBD, then CBD/ArHO has promise. During a clinical pilot study, we observed that accessing animals for multiple daily dosing may have increased environmental stress, which may limit the usefulness of CBD/ArHO unless an alternative, palatable vehicle is developed. In addition, once daily dosing would be more convenient and increase compliance of use as some facilities do not have 24-h personnel able to medicate the animals every 6–12 h; however, our PK data suggested that once daily dosing was insufficient in maintaining serum CBD concentrations. Given the considerable inter-subject variability and differences of our results compared to other species, CBD/ArHO should be evaluated for reproducibility in other cynomolgus macaques and other NHP to determine if results are similar to our findings.
Data availability statement
The raw data supporting the conclusions of this article will be made available by the authors, without undue reservation.
Ethics statement
The animal study was approved by the Mannheimer Foundation’s Institutional Animal Care and Use Committee. The study was conducted in accordance with the local legislation and institutional requirements.
Author contributions
TJ: Conceptualization, Data curation, Formal analysis, Investigation, Methodology, Project administration, Supervision, Writing – original draft, Writing – review & editing, Funding acquisition, Resources. JW: Conceptualization, Funding acquisition, Resources, Writing – review & editing. AL: Data curation, Formal analysis, Methodology, Validation, Writing – original draft. AZ: Data curation, Formal analysis, Methodology, Validation, Writing – review & editing. WB: Conceptualization, Data curation, Formal analysis, Funding acquisition, Investigation, Methodology, Project administration, Resources, Supervision, Visualization, Writing – original draft, Writing – review & editing.
Funding
The author(s) declare financial support was received for the research, authorship, and/or publication of this article. The Mannheimer Foundation internally funded the animal use, staff support, equipment, clinical pathology, and disposable materials. ElleVet Sciences provided the CBD/ArHO at no cost and financially supported the independent serum cannabinoid analysis by the Toxicology Research Laboratory of the University of Illinois at Chicago and publication costs.
Acknowledgments
We thank Pablo Morales and The Mannheimer Foundation Board of Trustees for supporting this project, and our colony manager, Olivia Forsthay-Moreno, and veterinary assistant, Carlos Moreno, for assisting with the subject selection and training, and sample collection. We thank ElleVet Sciences for providing the CBD/ArHO at no costand financially supporting the independent serum cannabinoid analysis by the Toxicology Research Laboratory of the University of Illinois at Chicago and publication costs. We thank Beatriz Gomez for performing the cannabinoid analysis at the Toxicology Research Laboratory of the University of Illinois at Chicago.
Conflict of interest
JW is a paid consultant of ElleVet Sciences. Although the CBD/ArHO and serum cannabinoid analysis and publication costs were financially supported by ElleVet, the study was conducted by the staff at The Mannheimer Foundation (TJ and WB) and independently analyzed by the Toxicology Research Laboratory of the University of Illinois at Chicago (AL and AZ).
Publisher’s note
All claims expressed in this article are solely those of the authors and do not necessarily represent those of their affiliated organizations, or those of the publisher, the editors and the reviewers. Any product that may be evaluated in this article, or claim that may be made by its manufacturer, is not guaranteed or endorsed by the publisher.
Supplementary material
The Supplementary material for this article can be found online at: https://www.frontiersin.org/articles/10.3389/fvets.2023.1286158/full#supplementary-material
Footnotes
References
1. Eichler, M, Spinedi, L, Unfer-Grauwiler, S, Bodmer, M, Surber, C, Luedi, M, et al. Heat exposure of Cannabis sativa extracts affects the pharmacokinetic and metabolic profile in healthy male subjects. Planta Med. (2012) 78:686–91. doi: 10.1055/s-0031-1298334
2. Sikora, V, Berenji, J, and Latković, D. Influence of agroclimatic conditions on content of main cannabinoids in industrial hemp (Cannabis sativa L.). Genetika-Belgrade. (2011) 43:449–56. doi: 10.2298/GENSR1103449S
3. Taura, F, Morimoto, S, and Shoyama, Y. Purification and characterization of cannabidiolic-acid synthase from Cannabis sativa L.: biochemical analysis of a novel enzyme that catalyzes the oxidocyclization of cannabigerolic acid to cannabidiolic acid. J Biol Chem. (1996) 271:17411–6. doi: 10.1074/jbc.271.29.17411
4. Taura, F, Sirikantaramas, S, Shoyama, Y, Yoshikai, K, Shoyama, Y, and Morimoto, S. Cannabidiolic-acid synthase, the chemotype-determining enzyme in the fiber-type Cannabis sativa. FEBS Lett. (2007) 16:2929–34. doi: 10.1016/j.febslet.2007.05.043
5. Marks, MD, Tian, L, Wenger, JP, Omburo, SN, Soto-Fuentes, W, He, J, et al. Identification of candidate genes affecting Δ9-tetrahydrocannabinol biosynthesis in Cannabis sativa. J Exp Bot. (2009) 60:3715–26. doi: 10.1093/jxb/erp210
6. Russo, EB. Cannabidiol claims and misconceptions. Trends Pharmacol Sci. (2017) 38:198–201. doi: 10.1016/j.tips.2016.12.004
7. Merrick, J, Lane, B, Sebree, T, Yaksh, T, O’Neill, C, and Banks, SL. Identification of psychoactive degradants of cannabidiol in simulated gastric and physiological fluid. Cannabis Cannabinoid Res. (2016) 1:102–12. doi: 10.1089/can.2015.0004
8. Crippa, JAS, Zuardi, AW, Hallak, JEC, Miyazawa, B, Bernardo, SA, Donaduzzi, CM, et al. Oral cannabidiol does not convert to Δ8-THC or Δ9-THC in humans: a pharmacokinetic study in healthy subjects. Cannabis Cannabinoid Res. (2020) 5:89–98. doi: 10.1089/can.2019.0024
9. Wray, L, Stott, C, Jones, N, and Wright, S. Cannabidiol does not convert to Δ9-tetrahydrocannabinol in an in vivo animal model. Cannabis Cannabinoid Res. (2017) 2:282–7. doi: 10.1089/can.2017.0032
10. Palazzoli, F, Citti, C, Licata, M, Vilella, A, Manca, L, Zoli, M, et al. Development of a simple and sensitive liquid chromatography triple quadrupole mass spectrometry (LC–MS/MS) method for the determination of cannabidiol (CBD), Δ9-tetrahydrocannabinol (THC) and its metabolites in rat whole blood after oral administration of a single high dose of CBD. J Pharm Biomed Anal. (2018) 150:25–32. doi: 10.1016/j.jpba.2017.11.054
11. Nahler, G, Jones, TM, and Russo, EB. Cannabidiol and contributions of major hemp phytocompounds to the “entourage effect”; possible mechanisms. Altern Complement Integr Med. (2019) 5:1–16. doi: 10.24966/ACIM-7562/100066
12. CFR. Schedules of controlled substance–schedule I. 21 CFR § 1308.11. (2022). Available at: https://www.govinfo.gov/app/details/CFR-2022-title21-vol9/CFR-2022-title21-vol9-sec1308-11 (Accessed August 21, 2023).
13. USC. Schedule of controlled substances. 21 USC § 812. (2021). Available at: https://www.govinfo.gov/content/pkg/USCODE-2021-title21/pdf/USCODE-2021-title21-chap13-subchapI-partB-sec812.pdf (Accessed August 21, 2023).
14. PubL. Agriculture improvement act of 2018. PubL 115–334. (2018). Available at: https://www.govinfo.gov/content/pkg/PLAW-115publ334/pdf/PLAW-115publ334.pdf (Accessed August 21, 2023).
15. Wakshlag, JJ, Cital, S, Eaton, SJ, Prussin, R, and Hudalla, C. Cannabinoid, terpene, and heavy metal analysis of 29 over-the-counter commercial veterinary hemp supplements. Vet Med (Auckl). (2020) 11:45–55. doi: 10.2147/VMRR.S248712
16. Bonn-Miller, MO, Loflin, MJE, Thomas, BF, Marcu, JP, Hyke, T, and Vandrey, R. Labeling accuracy of cannabidiol extracts sold online. JAMA. (2017) 318:1708–9. doi: 10.1001/jama.2017.11909
17. Mazurkiewicz-Bełdzińska, M, and Zawadzka, M. Use of cannabidiol in the treatment of epilepsy. Neurol Neurochir Pol. (2022) 56:14–20. doi: 10.5603/PJNNS.a2022.0020
18. Li, K, Yan, FJ, Yu, LY, Yuece, B, Hong, LX, Ying, YL, et al. Anti-inflammatory role of cannabidiol and O-1602 in cerulein-induced acute pancreatitis in mice. Pancreas. (2013) 42:123–9. doi: 10.1097/MPA.0b013e318259f6f0
19. Jastrząb, A, Jarocka-Karpowicz, I, Markowska, A, Wroński, A, Gęgotek, A, and Skrzydlewska, E. Antioxidant and anti-inflammatory effect of cannabidiol contributes to the decreased lipid peroxidation of keratinocytes of rat skin exposed to UV radiation. Oxidative Med Cell Longev. (2021) 2021:e6647222:1–13. doi: 10.1155/2021/6647222
20. Pan, H, Mukhopadhyay, P, Rajesh, M, Patel, V, Mukhopadhyay, B, Gao, B, et al. Cannabidiol attenuates cisplatin-induced nephrotoxicity by decreasing oxidative/nitrosative stress, inflammation, and cell death. J Pharmacol Exp Ther. (2009) 328:708–14. doi: 10.1124/jpet.108.147181
21. Brioschi, FA, Di Cesare, F, Gioeni, D, Rabbogliatti, V, Ferrari, F, D’Urso, ES, et al. Oral transmucosal cannabidiol oil formulation as part of a multimodal analgesic regimen: effects on pain relief and quality of life improvement in dogs affected by spontaneous osteoarthritis. Animals. (2020) 10:1505. doi: 10.3390/ani10091505
22. Kwiatkowska, M, Parker, LA, Burton, P, and Mechoulam, R. A comparative analysis of the potential of cannabinoids and ondansetron to suppress cisplatin-induced emesis in the Suncus murinus (house musk shrew). Psychopharmacology. (2004) 174:254–9. doi: 10.1007/s00213-003-1739-9
23. Guimarães, FS, de Aguiar, JC, Mechoulam, R, and Breuer, A. Anxiolytic effect of cannabidiol derivatives in the elevated plus-maze. Gen Pharmacol Vasc Syst. (1994) 25:161–4. doi: 10.1016/0306-3623(94)90027-2
24. Henry, JG, Shoemaker, G, Prieto, JM, Hannon, MB, and Wakshlag, JJ. The effect of cannabidiol on canine neoplastic cell proliferation and mitogen-activated protein kinase activation during autophagy and apoptosis. Vet Comp Oncol. (2021) 19:253–65. doi: 10.1111/vco.12669
25. Ligresti, A, Moriello, A, Starowicz, K, Matias, I, Pisanti, S, De Petrocellis, L, et al. Antitumor activity of plant cannabinoids with emphasis on the effect of cannabidiol on human breast carcinoma. J Pharmacol Exp Ther. (2006) 318:1375–87. doi: 10.1124/jpet.106.105247
26. Luz-Veiga, M, Amorim, M, Pinto-Ribeiro, I, Oliveira, ALS, Silva, S, Pimentel, LL, et al. Cannabidiol and cannabigerol exert antimicrobial activity without compromising skin microbiota. Int J Mol Sci. (2023) 24:2389. doi: 10.3390/ijms24032389
27. Abichabki, N, Zacharias, LV, Moreira, NC, Bellissimo-Rodrigues, F, Moreira, FL, Benzi, JRL, et al. Potential cannabidiol (CBD) repurposing as antibacterial and promising therapy of CBD plus polymyxin B (PB) against PB-resistant gram-negative bacilli. Sci Rep. (2022) 12:6454. doi: 10.1038/s41598-022-10393-8
28. Gamble, LJ, Boesch, JM, Frye, CW, Schwark, WS, Mann, S, Wolfe, L, et al. Pharmacokinetics, safety, and clinical efficacy of cannabidiol treatment in osteoarthritic dogs. Front Vet Sci. (2018) 5:165. doi: 10.3389/fvets.2018.00165
29. Weiss, L, Zeira, M, Reich, S, Har-Noy, M, Mechoulam, R, Slavin, S, et al. Cannabidiol lowers incidence of diabetes in non-obese diabetic mice. Autoimmunity. (2006) 39:143–51. doi: 10.1080/08916930500356674
30. Weiss, L, Zeira, M, Reich, S, Slavin, S, Raz, I, Mechoulam, R, et al. Cannabidiol arrests onset of autoimmune diabetes in NOD mice. Neuropharmacology. (2008) 54:244–9. doi: 10.1016/j.neuropharm.2007.06.029
31. El-Remessy, AB, Al-Shabrawey, M, Khalifa, Y, Tsai, NT, Caldwell, RB, and Liou, GI. Neuroprotective and blood-retinal barrier-preserving effects of cannabidiol in experimental diabetes. Am J Pathol. (2006) 168:235–44. doi: 10.2353/ajpath.2006.050500
32. Halpert, M, Verrico, C, Wesson, S, Konduri, V, Hofferek, C, Vazquez-Perez, J, et al. A randomized, double-blind, placebo-controlled study of daily cannabidiol for the treatment of canine osteoarthritis. J Immunol. (2020) 204:67.11. doi: 10.4049/jimmunol.204.Supp.67.11
33. Cheng, D, Spiro, AS, Jenner, AM, Garner, B, and Karl, T. Long-term cannabidiol treatment prevents the development of social recognition memory deficits in Alzheimer’s disease transgenic mice. J Alzheimers Dis. (2014) 42:1383–96. doi: 10.3233/JAD-140921
34. Khodadadi, H, Salles, ÉL, Jarrahi, A, Costigliola, V, Khan, MB, Yu, JC, et al. Cannabidiol ameliorates cognitive function via regulation of IL-33 and TREM2 upregulation in a murine model of Alzheimer’s disease. J Alzheimers Dis. (2021) 80:973–7. doi: 10.3233/JAD-210026
35. Crivelaro Do Nascimento, G, Ferrari, DP, Guimaraes, FS, Del Bel, EA, Bortolanza, M, and Ferreira, NC. Cannabidiol increases the nociceptive threshold in a preclinical model of Parkinson’s disease. Neuropharmacology. (2020) 163:107808. doi: 10.1016/j.neuropharm.2019.107808
36. Zuardi, AW. Cannabidiol: from an inactive cannabinoid to a drug with wide spectrum of action. Braz J Psychiatry. (2008) 30:271–80. doi: 10.1590/S1516-44462008000300015
37. Chagas, MHN, Zuardi, AW, Tumas, V, Pena-Pereira, MA, Sobreira, ET, Bergamaschi, MM, et al. Effects of cannabidiol in the treatment of patients with Parkinson’s disease: an exploratory double-blind trial. J Psychopharmacol (Oxf). (2014) 28:1088–98. doi: 10.1177/0269881114550355
38. Hunt, ABG, Flint, HE, Logan, DW, and King, T. A single dose of cannabidiol (CBD) positively influences measures of stress in dogs during separation and car travel. Front Vet Sci. (2023) 10:1112604. doi: 10.3389/fvets.2023.1112604
39. Scicluna, RL, Wilson, BB, Thelaus, SH, Arnold, JC, McGregor, IS, and Bowen, MT. Cannabidiol reduced the severity of gastrointestinal symptoms of opioid withdrawal in male and female mice. Cannabis Cannabinoid Res. (2022) 2022:36. doi: 10.1089/can.2022.0036
40. Schicho, R, and Storr, M. Topical and systemic cannabidiol improves trinitrobenzene sulfonic acid colitis in mice. Pharmacology. (2012) 89:149–55. doi: 10.1159/000336871
41. Vivanco-Estela, AN, Dos-Santos-Pereira, M, Guimaraes, FS, Del-Bel, E, and Nascimento, GC. Cannabidiol has therapeutic potential for myofascial pain in female and male parkinsonian rats. Neuropharmacology. (2021) 196:108700. doi: 10.1016/j.neuropharm.2021.108700
42. Nitecka-Buchta, A, Nowak-Wachol, A, Wachol, K, Walczyńska-Dragon, K, Olczyk, P, Batoryna, O, et al. Myorelaxant effect of transdermal cannabidiol application in patients with TMD: a randomized, double-blind trial. J Clin Med. (2019) 8:1886. doi: 10.3390/jcm8111886
43. Krzyżewska, A, Baranowska-Kuczko, M, Kasacka, I, and Kozłowska, H. Cannabidiol inhibits lung proliferation in monocrotaline-induced pulmonary hypertension in rats. Biomed Pharmacother. (2023) 159:114234. doi: 10.1016/j.biopha.2023.114234
44. Kumric, M, Dujic, G, Vrdoljak, J, Svagusa, K, Kurir, TT, Supe-Domic, D, et al. CBD supplementation reduces arterial blood pressure via modulation of the sympatho-chromaffin system: a substudy from the HYPER-H21-4 trial. Biomed Pharmacother. (2023) 160:114387. doi: 10.1016/j.biopha.2023.114387
45. Batinic, A, Sutlović, D, Kuret, S, Matana, A, Kumric, M, Bozic, J, et al. Trial of a novel oral cannabinoid formulation in patients with hypertension: a double-blind, placebo-controlled pharmacogenetic study. Pharmaceuticals. (2023) 16:645. doi: 10.3390/ph16050645
46. Rossignol, J, Hatton, S, Ridley, A, Hermine, O, and Greco, C. The effectiveness and safety of pharmaceutical-grade cannabidiol in the treatment of mastocytosis-associated pain: a pilot study. Biomedicine. (2023) 11:520. doi: 10.3390/biomedicines11020520
47. Bolognini, D, Rock, E, Cluny, N, Cascio, M, Limebeer, C, Duncan, M, et al. Cannabidiolic acid prevents vomiting in Suncus murinus and nausea-induced behaviour in rats by enhancing 5-HT1A receptor activation. Br J Pharmacol. (2013) 168:1456–70. doi: 10.1111/bph.12043
48. Takeda, S, Misawa, K, Yamamoto, I, and Watanabe, K. Cannabidiolic acid as a selective cyclooxygenase-2 inhibitory component in cannabis. Drug Metab Dispos. (2008) 36:1917–21. doi: 10.1124/dmd.108.020909
49. Rock, EM, Limebeer, CL, and Parker, LA. Effect of cannabidiolic acid and ∆9-tetrahydrocannabinol on carrageenan-induced hyperalgesia and edema in a rodent model of inflammatory pain. Psychopharmacology. (2018) 235:3259–71. doi: 10.1007/s00213-018-5034-1
50. Vigli, D, Cosentino, L, Pellas, M, and De Filippis, B. Chronic treatment with cannabidiolic acid (CBDA) reduces thermal pain sensitivity in male mice and rescues the hyperalgesia in a mouse model of Rett syndrome. Neuroscience. (2020) 453:113–23. doi: 10.1016/j.neuroscience.2020.09.041
51. Anderson, LL, Low, IK, Banister, SD, McGregor, IS, and Arnold, JC. Pharmacokinetics of phytocannabinoid acids and anticonvulsant effect of cannabidiolic acid in a mouse model of Dravet syndrome. J Nat Prod. (2019) 82:3047–55. doi: 10.1021/acs.jnatprod.9b00600
52. Calapai, F, Cardia, L, Calapai, G, Di Mauro, D, Trimarchi, F, Ammendolia, I, et al. Effects of cannabidiol on locomotor activity. Life. (2022) 12:652. doi: 10.3390/life12050652
53. Kleinhenz, MD, Weeder, M, Montgomery, S, Martin, M, Curtis, A, Magnin, G, et al. Short term feeding of industrial hemp with a high cannabidiolic acid (CBDA) content increases lying behavior and reduces biomarkers of stress and inflammation in Holstein steers. Sci Rep. (2022) 12:3683. doi: 10.1038/s41598-022-07795-z
54. Assareh, N, Gururajan, A, Zhou, C, Luo, JL, Kevin, RC, and Arnold, JC. Cannabidiol disrupts conditioned fear expression and cannabidiolic acid reduces trauma-induced anxiety-related behaviour in mice. Behav Pharmacol. (2020) 31:591–6. doi: 10.1097/FBP.0000000000000565
55. Deabold, KA, Schwark, WS, Wolf, L, and Wakshlag, JJ. Single-dose pharmacokinetics and preliminary safety assessment with use of CBD-rich hemp nutraceutical in healthy dogs and cats. Animals. (2019) 9:832. doi: 10.3390/ani9100832
56. Devinsky, O, Marsh, E, Friedman, D, Thiele, E, Laux, L, Sullivan, J, et al. Cannabidiol in patients with treatment-resistant epilepsy: an open-label interventional trial. Lancet Neurol. (2016) 15:270–8. doi: 10.1016/S1474-4422(15)00379-8
57. Contin, M, Mohamed, S, Santucci, M, Lodi, MAM, Russo, E, Mecarelli, O, et al. Cannabidiol in pharmacoresistant epilepsy: clinical pharmacokinetic data from an expanded access program. Front Pharmacol. (2021) 12:179. doi: 10.3389/fphar.2021.637801
58. McGrath, S, Bartner, LR, Rao, S, Packer, RA, and Gustafson, DL. Randomized blinded controlled clinical trial to assess the effect of oral cannabidiol administration in addition to conventional antiepileptic treatment on seizure frequency in dogs with intractable idiopathic epilepsy. J Am Vet Med Assoc. (2019) 254:1301–8. doi: 10.2460/javma.254.11.1301
59. McGrath, S, Bartner, LR, Rao, S, Kogan, LR, and Hellyer, PW. A report of adverse effects associated with the administration of cannabidiol in healthy dogs. Am Holistic Vet Med Assoc. (2018) 52:34–8.
60. Kaufmann, R, Aqua, K, Lombardo, J, and Lee, M. Observed impact of long-term consumption of oral cannabidiol on liver function in healthy adults. Cannabis Cannabinoid Res. (2021) 8:148–54. doi: 10.1089/can.2021.0114
61. Wang, T, Zakharov, A, Gomez, B, Lyubimov, A, Trottier, NL, Schwark, WS, et al. Serum cannabinoid 24 h and 1 week steady state pharmacokinetic assessment in cats using a CBD/CBDA rich hemp paste. Front Vet Sci. (2022) 9:895368. doi: 10.3389/fvets.2022.895368
62. Devinsky, O, Cross, JH, Laux, L, Marsh, E, Miller, I, Nabbout, R, et al. Trial of cannabidiol for drug-resistant seizures in the Dravet syndrome. N Engl J Med. (2017) 376:2011–20. doi: 10.1056/NEJMoa1611618
63. Watkins, PB, Church, RJ, Li, J, and Knappertz, V. Cannabidiol and abnormal liver chemistries in healthy adults: results of a phase I clinical trial. Clin Pharmacol Ther. (2021) 109:1224–31. doi: 10.1002/cpt.2071
64. Doohan, PT, Oldfield, LD, Arnold, JC, and Anderson, LL. Cannabinoid interactions with cytochrome P450 drug metabolism: a full-spectrum characterization. AAPS J. (2021) 23:91. doi: 10.1208/s12248-021-00616-7
65. Nasrin, S, Watson, CJW, Perez-Paramo, YX, and Lazarus, P. Cannabinoid metabolites as inhibitors of major hepatic CYP450 enzymes, with implications for cannabis-drug interactions. Drug Metab Dispos Biol Fate Chem. (2021) 49:1070–80. doi: 10.1124/dmd.121.000442
66. Rosenkrantz, H, Fleischman, RW, and Grant, RJ. Toxicity of short-term administration of cannabinoids to rhesus monkeys. Toxicol Appl Pharmacol. (1981) 58:118–31. doi: 10.1016/0041-008X(81)90122-8
67. Carlson, CS, Loeser, RF, Jayo, MJ, Weaver, DS, Adams, MR, and Jerome, CP. Osteoarthritis in cynomolgus macaques: a primate model of naturally occurring disease. J Orthop Res. (1994) 12:331–9. doi: 10.1002/jor.1100120305
68. Gottlieb, DH, Capitanio, JP, and McCowan, B. Risk factors for stereotypic behavior and self-biting in rhesus macaques (Macaca mulatta): Animal’s history, current environment, and personality. Am J Primatol. (2013) 75:995–1008. doi: 10.1002/ajp.22161
69. Hird, DW, Anderson, JH, and Bielitzki, JT. Diarrhea in nonhuman primates: a survey of primate colonies for incidence rates and clinical opinion. Lab Anim Sci. (1984) 34:465–70.
70. Wakshlag, JJ, Schwark, WS, Deabold, KA, Talsma, BN, Cital, S, Lyubimov, A, et al. Pharmacokinetics of cannabidiol, cannabidiolic acid, ∆9-tetrahydrocannabinol, tetrahydrocannabinolic acid and related metabolites in canine serum after dosing with three oral forms of hemp extract. Front Vet Sci. (2020) 7:7. doi: 10.3389/fvets.2020.00505
71. Tittle, DJ, Wakshlag, JJ, Schwark, WS, Lyubimov, A, Zakharov, A, and Gomez, B. Twenty-four hour and one-week steady state pharmacokinetics of cannabinoids in two formulations of cannabidiol and cannabidiolic acid rich hemp in dogs. Med Res Arch. (2022) 10:2907. doi: 10.18103/mra.v10i7.2907
72. Johns, TN, Cameron, AI, and Burnside, WM. Cannabidiol and cannabidiolic acid-rich hemp oil for the treatment of diarrheal disease in cynomolgus macaques (Macaca fascicularis). Abstracts of scientific presentations: 2020 AALAS virtual national meeting. J Am Assoc Lab Anim Sci. (2020) 59:659.
73. PubL. Animal welfare act as amended. PubL 89–544. (2018). Available at: https://www.govinfo.gov/content/pkg/COMPS-10262/pdf/COMPS-10262.pdf (Accessed August 21, 2023).
74. USC. Transportation, Sale, and handling of certain animals. 7 USC § 2131–2160. (2022). Available at: https://www.govinfo.gov/content/pkg/USCODE-2021-title7/pdf/USCODE-2021-title7-chap54.pdf (Accessed August 21, 2023).
75. CFR. Animal welfare regulations. 9 CFR § 1–12. (2022). https://www.govinfo.gov/content/pkg/CFR-2022-title9-vol1/pdf/CFR-2022-title9-vol1-chapI-subchapA.pdf (Accessed August 21, 2023).
76. National Research Council (US) Committee for the Update of the Guide for the Care and Use of Laboratory Animals. Guide for the care and use of laboratory animals. 8th ed. Washington, DC: National Academies Press (2011). 220 p.
77. Jones, AB, Elsohly, MA, Bedford, JA, and Turner, CE. Determination of cannabidiol in plasma by electron-capture gas chromatography. J Chromatogr B Biomed Sci App. (1981) 226:99–105. doi: 10.1016/S0378-4347(00)84210-3
78. Withey, SL, Eisold, JE, Chelini, G, Kangas, BD, Bergman, J, and Madras, BK. Behavioral and molecular effects of THC and CBD in adolescent nonhuman primates. FASEB J. (2020) 34:1. doi: 10.1096/fasebj.2020.34.s1.09462
79. Brady, KT, and Balster, RL. The effects of Δ9-tetrahydrocannabinol alone and in combination with cannabidiol on fixed-interval performance in rhesus monkeys. Psychopharmacology. (1980) 72:21–6. doi: 10.1007/BF00433803
80. Withey, SL, Kangas, BD, Charles, S, Gumbert, AB, Eisold, JE, George, S, et al. Effects of daily Δ9-tetrahydrocannabinol (THC) alone or combined with cannabidiol (CBD) on cognition-based behavior and activity in adolescent nonhuman primates. Drug Alcohol Depend. (2021) 221:108629. doi: 10.1016/j.drugalcdep.2021.108629
81. Wright, MJ Jr, Vandewater, SA, and Taffe, MA. Cannabidiol attenuates deficits of visuospatial associative memory induced by Δ9tetrahydrocannabinol. Br J Pharmacol. (2013) 170:1365–73. doi: 10.1111/bph.12199
82. Jacobs, DS, Kohut, SJ, Jiang, S, Nikas, SP, Makriyannis, A, and Bergman, J. Acute and chronic effects of cannabidiol on Δ9-tetrahydrocannabinol (Δ9-THC)-induced disruption in stop signal task performance. Exp Clin Psychopharmacol. (2016) 24:320–30. doi: 10.1037/pha0000081
83. Rozental, AJ, Gustafson, DL, Kusick, BR, Bartner, LR, Castro, SC, and McGrath, S. Pharmacokinetics of escalating single-dose administration of cannabidiol to cats. J Vet Pharmacol Ther. (2023) 46:25–33. doi: 10.1111/jvp.13100
84. Taylor, L, Crockett, J, Tayo, B, and Morrison, G. A phase I, open-label, parallel-group, single-dose trial of the pharmacokinetics and safety of cannabidiol (CBD) in subjects with mild to severe hepatic impairment. J Clin Pharmacol. (2019) 59:1110–9. doi: 10.1002/jcph.1412
85. Tayo, B, Taylor, L, Sahebkar, F, and Morrison, G. A phase I, open-label, parallel-group, single-dose trial of the pharmacokinetics, safety, and tolerability of cannabidiol in subjects with mild to severe renal impairment. Clin Pharmacokinet. (2020) 59:747–55. doi: 10.1007/s40262-019-00841-6
86. Devinsky, O, Patel, AD, Thiele, EA, Wong, MH, Appleton, R, Harden, CL, et al. Randomized, dose-ranging safety trial of cannabidiol in Dravet syndrome. Neurology. (2018) 90:1204–11. doi: 10.1212/WNL.0000000000005254
87. Turner, SE, Knych, HK, and Adams, AA. Pharmacokinetics of cannabidiol in a randomized crossover trial in senior horses. Am J Vet Res. (2022) 83:28. doi: 10.2460/ajvr.22.02.0028
88. Declan, R, McKemie, DS, Kass, PH, Puschner, B, and Knych, HK. Pharmacokinetics and effects on arachidonic acid metabolism of low doses of cannabidiol following oral administration to horses. Drug Test Anal. (2021) 13:1305–17. doi: 10.1002/dta.3028
89. Meyer, K, Hayman, K, Baumgartner, J, and Gorden, PJ. Plasma pharmacokinetics of cannabidiol following oral administration of cannabidiol oil to dairy calves. Front Vet Sci. (2022) 9:789495. doi: 10.3389/fvets.2022.789495
90. Kleinhenz, MD, Magnin, G, Lin, Z, Griffin, J, Kleinhenz, KE, Montgomery, S, et al. Plasma concentrations of eleven cannabinoids in cattle following oral administration of industrial hemp (Cannabis sativa). Sci Rep. (2020) 10:12753. doi: 10.1038/s41598-020-69768-4
91. Kleinhenz, MD, Magnin, G, Lin, J, Griffin, J, Kleinhenz, KE, Curtis, A, et al. Pharmacokinetics of cannabidiolic acid in cattle following oral dosing of industrial hemp (Cannabis sativa). Am Assoc Bov Pract Conf Proc. (2020) 53:391. doi: 10.21423/aabppro20208136
92. Rooney, TA, Carpenter, JW, KuKanich, B, Gardhouse, SM, Magnin, GC, and Tully, TN. Feeding decreases the oral bioavailability of cannabidiol and cannabidiolic acid in hemp oil in New Zealand white rabbits (Oryctolagus cuniculus). Am J Vet Res. (2022) 83:6. doi: 10.2460/ajvr.22.01.0006
93. Spittler, AP, Helbling, JE, McGrath, S, Gustafson, DL, Santangelo, KS, and Sadar, MJ. Plasma and joint tissue pharmacokinetics of two doses of oral cannabidiol oil in guinea pigs (Cavia porcellus). J Vet Pharmacol Ther. (2021) 44:967–74. doi: 10.1111/jvp.13026
94. Hložek, T, Uttl, L, Kadeřábek, L, Balíková, M, Lhotková, E, Horsley, RR, et al. Pharmacokinetic and behavioural profile of THC, CBD, and THC+CBD combination after pulmonary, oral, and subcutaneous administration in rats and confirmation of conversion in vivo of CBD to THC. Eur Neuropsychopharmacol. (2017) 27:1223–37. doi: 10.1016/j.euroneuro.2017.10.037
95. Carpenter, JW, Tulley, TN, Rockwell, K, and KuKanich, B. Pharmacokinetics of cannabidiol in the Hispaniolan Amazon parrot (Amazona ventralis). J Avian Med Surg. (2022) 36:121–7. doi: 10.1647/20-00076
96. Leo, A, Russo, E, and Elia, M. Cannabidiol and epilepsy: rationale and therapeutic potential. Pharmacol Res. (2016) 107:85–92. doi: 10.1016/j.phrs.2016.03.005
97. Hammell, DC, Zhang, LP, Ma, F, Abshire, SM, McIlwrath, SL, Stinchcomb, AL, et al. Transdermal cannabidiol reduces inflammation and pain-related behaviours in a rat model of arthritis. Eur J Pain. (2016) 20:936–48. doi: 10.1002/ejp.818
98. Devinsky, O, Kraft, K, Rusch, L, Fein, M, and Leone-Bay, A. Improved bioavailability with dry powder cannabidiol inhalation: a phase I clinical study. J Pharm Sci. (2021) 110:3946–52. doi: 10.1016/j.xphs.2021.08.012
99. Anderson, LL, Etchart, MG, Bahceci, D, Golembiewski, TA, and Arnold, JC. Cannabis constituents interact at the drug efflux pump BCRP to markedly increase plasma cannabidiolic acid concentrations. Sci Rep. (2021) 11:14948. doi: 10.1038/s41598-021-94212-6
100. Szaflarski, JP, Hernando, K, Bebin, EM, Gaston, TE, Grayson, LE, Ampah, SB, et al. Higher cannabidiol plasma levels are associated with better seizure response following treatment with a pharmaceutical grade cannabidiol. Epilepsy Behav. (2019) 95:131–6. doi: 10.1016/j.yebeh.2019.03.042
101. Pulley, ACS, Roberts, JA, and Lerche, NW. Four preanesthetic oral sedation protocols for rhesus macaques (Macaca mulatta). J Zoo Wildl Med. (2004) 35:497–502. doi: 10.1638/03-092
102. Taylor, L, Gidal, B, Blakey, G, Tayo, B, and Morrison, G. A phase I, randomized, double-blind, placebo-controlled, single ascending dose, multiple dose, and food effect trial of the safety, tolerability and pharmacokinetics of highly purified cannabidiol in healthy subjects. CNS Drugs. (2018) 32:1053–67. doi: 10.1007/s40263-018-0578-5
103. Pellesi, L, Licata, M, Verri, P, Vandelli, D, Palazzoli, F, Marchesi, F, et al. Pharmacokinetics and tolerability of oral cannabis preparations in patients with medication overuse headache (MOH)—a pilot study. Eur J Clin Pharmacol. (2018) 74:1427–36. doi: 10.1007/s00228-018-2516-3
104. Whalley, BJ, Stott, C, Gray, RA, and Jones, NA. Default. The human metabolite of cannabidiol, 7-hydroxy cannabidiol, but not 7-carboxy cannabidiol, is anticonvulsant in the maximal electroshock seizure threshold test (MEST) in mouse. AES Annual Meeting 2017. (2017). Available at: https://aesnet.org/abstractslisting/the-human-metabolite-of-cannabidiol--7-hydroxy-cannabidiol--but-not-7-carboxy-cannabidiol--is-anticonvulsant-in-the-maximal-electroshock-seizure-threshold-test-(mest)-in-mouse.
105. Koo, BS, Lee, DH, Kang, P, Jeong, KJ, Lee, S, Kim, K, et al. Reference values of hematological and biochemical parameters in young-adult cynomolgus monkey (Macaca fascicularis) and rhesus monkey (Macaca mulatta) anesthetized with ketamine hydrochloride. Lab Anim Res. (2019) 5:7. doi: 10.1186/s42826-019-0006-0
106. Xie, L, Xu, F, Liu, S, Ji, Y, Zhou, Q, Wu, Q, et al. Age- and sex-based hematological and biochemical parameters for Macaca fascicularis. PLoS One. (2013) 8:64892. doi: 10.1371/journal.pone.0064892
107. Manolio, TA, Burke, GL, Savage, PJ, Jacobs, DR, Sidney, S, Wagenknecht, LE, et al. Sex- and race-related differences in liver-associated serum chemistry tests in young adults in the cardia study. Clin Chem. (1992) 38:1853–9. doi: 10.1093/clinchem/38.9.1853
108. Giboney, PT. Mildly elevated liver transaminase levels in the asymptomatic patient. Am Fam Physician. (2005) 71:1105–10.
109. Tang, O, Selvin, E, Arends, V, and Saenger, A. Short-term stability of hematologic parameters in frozen whole blood. J Appl Lab Med. (2019) 4:410–4. doi: 10.1373/jalm.2018.028357
110. Limpongsa, E, Tabboon, P, Pongjanyakul, T, and Jaipakdee, N. Preparation and evaluation of directly compressible orally disintegrating tablets of cannabidiol formulated using liquisolid technique. Pharmaceutics. (2022) 14:2407. doi: 10.3390/pharmaceutics14112407
111. Abbotts, KSS, Ewell, TR, Butterklee, HM, Bomar, MC, Akagi, N, Dooley, GP, et al. Cannabidiol and cannabidiol metabolites: pharmacokinetics, interaction with food, and influence on liver function. Nutrients. (2022) 14:2152. doi: 10.3390/nu14102152
112. Stott, CG, White, L, Wright, S, Wilbraham, D, and Guy, GW. A phase I study to assess the single and multiple dose pharmacokinetics of THC/CBD oromucosal spray. Eur J Clin Pharmacol. (2013) 69:1135–47. doi: 10.1007/s00228-012-1441-0
113. Zuardi, AW, Rodrigues, NP, Silva, AL, Bernardo, SA, Hallak, JEC, Guimarães, FS, et al. Inverted U-shaped dose-response curve of the anxiolytic effect of cannabidiol during public speaking in real life. Front Pharmacol. (2017) 8:259. doi: 10.3389/fphar.2017.00259
114. Child, RB, and Tallon, MJ. Cannabidiol (CBD) dosing: plasma pharmacokinetics and effects on accumulation in skeletal muscle, liver and adipose tissue. Nutrients. (2022) 14:2101. doi: 10.3390/nu14102101
115. Malfait, AM, Gallily, R, Sumariwalla, PF, Malik, AS, Andreakos, E, Mechoulam, R, et al. The nonpsychoactive cannabis constituent cannabidiol is an oral anti-arthritic therapeutic in murine collagen-induced arthritis. Proc Natl Acad Sci. (2000) 97:9561–6. doi: 10.1073/pnas.160105897
Glossary
Keywords: pharmacokinetics, monkey, nonhuman primate, cannabidiol, cannabidiolic acid, cannabinoids, hemp (Cannabis sativa L.), noncompartmental analysis
Citation: Johns TN, Wakshlag JJ, Lyubimov AV, Zakharov A and Burnside WM (2023) Pharmacokinetics of cannabidiol-/cannabidiolic acid-rich hemp oil in juvenile cynomolgus macaques (Macaca fascicularis). Front. Vet. Sci. 10:1286158. doi: 10.3389/fvets.2023.1286158
Edited by:
Robin Temmerman, European College of Veterinary Pharmacology and Toxicology, BelgiumReviewed by:
Catarina Jota Baptista, Universidade de Trás os Montes e Alto Douro, PortugalAna Isabel Fraguas, Complutense University, Spain
Copyright © 2023 Johns, Wakshlag, Lyubimov, Zakharov and Burnside. This is an open-access article distributed under the terms of the Creative Commons Attribution License (CC BY). The use, distribution or reproduction in other forums is permitted, provided the original author(s) and the copyright owner(s) are credited and that the original publication in this journal is cited, in accordance with accepted academic practice. No use, distribution or reproduction is permitted which does not comply with these terms.
*Correspondence: Tinika N. Johns, dGpvaG5zQGhhbWFucmFuY2gub3Jn