- 1College of Veterinary Medicine, Sichaun Agricultural University, Chengdu, China
- 2Innovation Center of Guangdong Nuacid Biotechnology Co., Ltd., Qingyuan, China
- 3College of Animal Science and Technology, Jiangxi Agricultural University, Nanchang, China
Introduction: Surveillance of the Seneca Valley virus (SVV) shows a disproportionately higher incidence on Chinese pig farms. Currently, there are no vaccines or drugs to treat SVV infection effectively and effective treatment options are urgently needed.
Methods: In this study, we evaluated the antiviral activity of the following medium-chain fatty acids (MCFAs) or triglycerides (MCTs) against SVV: caprylic acid, caprylic monoglyceride, capric monoglyceride, and monolaurin.
Results: In vitro experiments showed that monolaurin inhibited viral replication by up to 80%, while in vivo studies showed that monolaurin reduced clinical manifestations, viral load, and organ damage in SVV-infected piglets. Monolaurin significantly reduced the release of inflammatory cytokines and promoted the release of interferon-γ, which enhanced the viral clearance activity of this type of MCFA.
Discussion: Therefore, monolaurin is a potentially effective candidate for the treatment of SVV infection in pigs.
Introduction
Seneca Valley virus (SVV) belongs to the genus Senecavirus in the family Picornaviridae. Phylogenetic analysis of the whole-genome sequence of Senecavirus A shows that it is closely related to members of the genus Cardiovirus (1). In 2015, Brazilian scientists isolated the complete genome of SVV from vesicular fluid and serum of pigs with vesicular disease and elucidated that SVV infection was associated with idiopathic vesicular disease in pigs (2). Subsequently, many other countries have also reported cases of pigs infected with SVV, where newborn piglets are more vulnerable to SVV infection. The main clinical manifestations of SVV infection in pigs are blisters and ulcers on the hoofs and snout (3, 4). Clinical symptoms can be similar to foot-and-mouth disease, swine vesicular disease, and vesicular stomatitis, with a potential impact on the immune system of pigs (5). The virus is shed through the oral cavity, nasal secretions, and feces with a viral shedding duration of ~28 days after infection (3). Presently, SVV is sporadically and locally prevalent, but its transmission mechanism is not completely clear. Currently, there is no vaccine or specific drug available for the prevention and treatment of SVV infection in pigs. Therefore, the control of SVV in pigs depends on the hygiene measures implemented on pig farms.
Replacing antibiotics in animal feed with biologically active substances has become a hot topic in China. Medium-chain fatty acids (MCFAs) are a class of saturated fatty acids containing 6–12 carbon atoms. Even-numbered carbon MCFAs, such as caproic acid (C6), caprylic acid (C8), capric acid (C10), and lauric acid (C12), are found in natural foods, such as coconut oil, palm kernel oil, and milk. MCFAs undergo esterification with glycerol to form triglycerides, known as medium-chain fatty acid triglycerides (MCTs). In addition to being a source of energy, MCFAs can also improve intestinal morphological structure and growth, prevent infection, regulate immunity, and act as an alternative to antibiotics (6, 7). Both MCFAs and MCTs exhibit strong bacteriostatic activity against a variety of pathogens, including gram-positive and gram-negative bacteria, viruses, fungi, algae, and protozoa (8, 9).
The antimicrobial properties of fatty acids have been reported extensively in the literature (10). Previously, studies have confirmed the antiviral activity of free MCFAs such as capric, lauric, myristic, and long-chain unsaturated oleic, linoleic and linolenic acids against vesicular stomatitis virus (VSV), herpes simplex virus (HSV) and visna virus (11). Other studies reported similar antiviral activity of MCFAs, together with their alcohol and monoglyceride derivatives, against HSV 1 and 2 (12). Research also showed that MCFAs (caprylic, capric, and lauric acids) and monolaurin can inhibit ASFV in liquid conditions and reduce Asfivirus (ASFV) infectivity, which may help to prevent disease progression and virus transmission (13). On the one hand, SVV is a small, non-enveloped picornavirus, unknown until 2002 when it was discovered incidentally as a cell culture contaminant, and the family Picornaviridae also contains foot-and-mouth disease virus (FMDV) and swine vesicular disease virus (SVDV). On the other hand, since the vesicular lesions caused by SVV infection are clinically similar from those caused by other vesicular disease viruses, such as FMDV, SVDV, VSV and vesicular exanthema of swine virus (VESV). Thus, we evaluated the antiviral activity of MCFAs or MCTs against SVV. In this study, the anti-SVV activity of selected MCFAs and MCTs was evaluated in vitro, and the most effective compound was selected and tested in vivo. The clinical symptoms, viral load, and proinflammatory cytokines were recorded and analyzed to evaluate the anti-SVV activity of monolaurin. Our results provide a reliable basis for the potential clinical use of monolaurin for the treatment of SVV infection in pigs.
Materials and methods
Samples and reagents
BHK-21 cells and the Seneca virus A strain SVV-SC-MS (complete genome GenBank: MN700930.1) were obtained from the Animal Biotechnology Center (ABTC) at Sichuan Agricultural University School of Veterinary Medicine. Fetal bovine serum, cell culture medium (DMEM), trypsin, and PBS buffer were purchased from Solarbio (Beijing Solarbio Science and Technology Co., Ltd., Beijing, China); DMSO was purchased from Sigma (USA); the CCK8 kit (code: Beyotime. C0038) used in this study was purchased from Beyotime Biotechnology Co., Ltd. Caprylic, caprylic monoglyceride, capric monoglyceride, and monolaurin were prepared by Guangdong Nuacid Biotechnology Co., Ltd. The PrimeScript™ RT reagent Kit (Perfect Real Time), DNA/RNA extraction kit, and TB Green® Premix Ex Taq™ (Tli RNaseH Plus) were purchased from Takara (Dalian) Engineering Co., Ltd.
Maximum nontoxic dose
BHK-21 cells were cultured in a 96-well plate at 37°C under 5% CO2 for 24–36 h until the cells grew into a monolayer. Caprylic, caprylic monoglyceride, capric monoglyceride, and monolaurin were dissolved in DMSO independently to prepare a 10 mg/mL stock solution. A cell maintenance solution of 100 μg/mL was prepared from the stock solution for these four test MCFA, whereafter a total of 11 concentration gradients were prepared from the cell maintenance solution following 2-fold gradient dilution. The concentrations of the cell maintenance solution for these four test MCFA were 100, 50, 25, 12.5, 6.25, 3.125, 1.563, 0.781, 0.391, 0.195, 0.098, and 0.049 μg/mL. Supernatant from all wells of a 96-well-plate with monolayer BHK-21 cells was discarded, and the MCFA sample solution (100 μL/well) was added. Two percent DMEM and one precebt DMSO controls were also set at the same time. The cells were cultured in an incubator for 48 h at 37°C and 5% CO2. A cytotoxicity assay was performed according to the instructions of the CCK8 kit, and the cell viability was calculated. The concentration corresponding to a cell viability >90% was recorded as the maximum non-toxic dose (MNTD), which was used as the working dose for subsequent experiments.
In vitro calculation of viral inhibition rate
A mixture of 1 MNTD and 100 TCID50 viral suspension was prepared by mixing the virus solution with the MCFA solution. BHK-21 cells were seeded in a 96-well-plate and grown to monolayers at 37°C in a 5% CO2 incubator. The supernatants were discarded, and an equal volume of the viral suspension was added to the wells (100 μL/well) of the experimental group. The 2% DMEM (A) and 1% DMSO (B) controls were also set. The plates were incubated for 1 h at 37°C with 5% CO2 in a cell incubator. After incubation, the supernatant was discarded, and 100 μL of the sample solution with a concentration of 1 MNTD or 100 μL of the maintenance solution was added to the corresponding wells and then incubated at 37°C in a 5% CO2 incubator. Cell infection was stopped when complete CPE was developed in virus-only control wells (for ~36–48 h post-infection). The supernatants were collected and measured with the CCK8 method, and the virus inhibition rate was calculated.
In vivo evaluation of anti-SVV activity
A total of 25 weaned piglets at 21 days old were obtained from a pig farm (Sichuan gistar group) in Sichuan Province, China. All the piglets were SVV negative for the antigen and antibody test by PCR or ELISA kits (detection methods were established by ATBC). Before the experiment, the animal lab was sterilized with formaldehyde and pasteurizer. All the pigs were cared for according to original farm procedures to prevent stress and bacterial infection in the pigs. The piglets were fed common complete feed. Piglets were first observed for 3 days and then subjected to viral challenge and drug administration. The 25 weaned piglets were divided into 5 groups (n = 5), as shown in Table 1.
Clinical symptoms
The clinical symptoms of piglets in each group were observed daily, and scores were assigned as follows: fever at 2 points; lethargy at 2 points; decreased feed intake at 1 point; anorexia at 2 points; blisters or ulcers at 2 points; and death as 5 points. Additionally, for every piglet in each group, morning feces and 0.5 mL jugular blood were collected daily for the determination of SVV load. Whenever blisters and crusts appeared or the piglets were on the verge of death, they were immediately sacrificed and necropsied. On day 14, all the remaining piglets were sacrificed, and the lungs, spleens, kidneys, and livers were aseptically collected and fixed in 4% paraformaldehyde.
Quantitative detection of SVV load in stool and blood by RT–qPCR
The feces and blood samples were thoroughly mixed with 3.0 mL of PBS and then centrifuged at 12,000 r/min for 3 min. Supernatants were collected for RNA extraction. Extracted RNA was reverse transcribed to obtain cDNA, which was added to the PCR master mix as detailed in Table 2, and loaded into a fluorescent quantitative PCR machine to detect the SVV viral load under the following thermocycling conditions: 40 cycles of denaturation at 95°C for 30 s, annealing at 95°C for 5 s, and elongation at 58°C for 30 s. At the end of the amplification, melting curve analysis was performed from 65 to 95°C with 0.5°C per second.
Histopathology
Briefly, the Lung, spleen, liver, and kidney tissues were fixed in 4% paraformaldehyde for 36 h and then embedded in paraffin. According to standard method, tissue sections (4 μm), were stained with Hematoxylin and Eosin (H&E) for histopathological examination. Finally, histological lesions were recorded with a light microscope (OLYMPUS, Japan) at 400× magnifications.
In vivo detection of inflammatory cytokines
Piglet venous blood was collected at 0 and 3 dpi. The expression of IL-6, IL-8, IL-10, IL-1β, IFN-γ, and TNF-α in the supernatant was detected using ELISA kits according to the manufacturer's instructions (Multisciences (Lianke) Biotech Co., Ltd., Hangzhou, China). The absorbance was measured using a microplate reader at 450 nm. In brief, the samples were added to the wells, followed by the antigen in the samples bounding to the capture antibody, the microplate was washed, the detection antibody was added, and the microplate was washed again. After the substrate was added, the microplate reader detected the colored reaction products and calculated light density (OD) values, which were used to calculate and analyze the amount of antigen in each sample.
Statistical analysis
Statistical results were expressed as means and standard deviation (SD). Significant differences were determined with one-way analysis of variance (ANOVA), followed by Duncan's multiple range test in SPSS 20.0 (IBM Corp., Armonk, NY, USA). Significance was set at P < 0.05.
Results
Maximum non-toxic concentration determination
After adding different concentrations of drugs and culturing for 96 h, the OD450 value or cell viability was determined using a CCK-8 kit following the manufacturer's instructions. If the cell viability was >90%, the dose was recorded as MBTD. BHK-21 cells showed good tolerance to glycerol caprylate and monoglycery laurate with an MNTD of 50 μg/mL. Caprylic and capric monoglycerides showed little toxicity to these cells with an MNTD of 25 μg/mL (Figure 1).
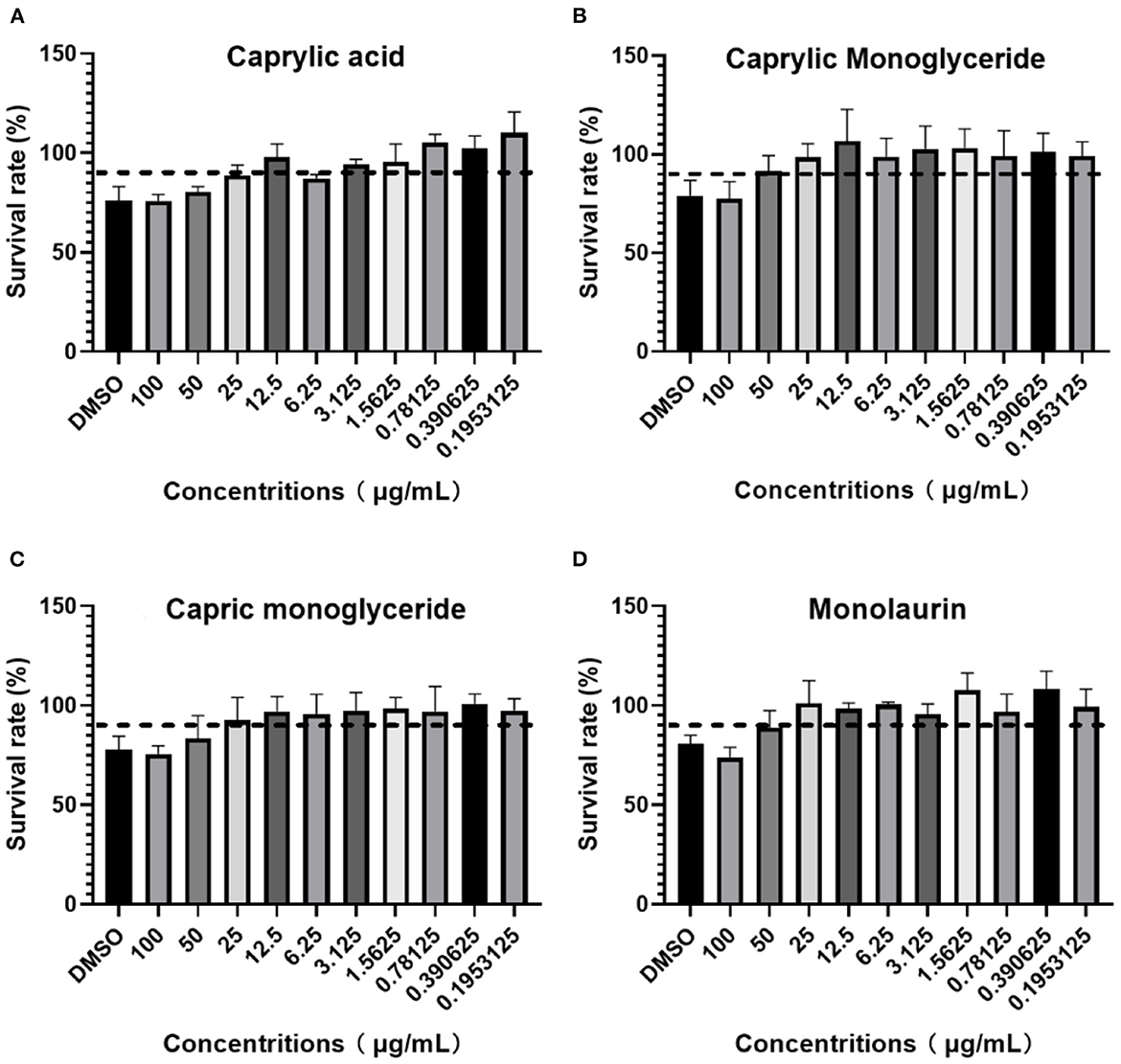
Figure 1. BHK-21 cell survival rate in: (A) Caprylic acid; (B) Caprylic monoglyceride; (C) Carpric monoglyceride; (D) Monolaurin.
Monolaurin inhibits virus proliferation
Although all four treatments had an inhibitory effect on SVV, the inhibitory rate of monolaurin was the highest, with values up to 80% (Figure 2). The anti-SVV activity of caprylic monoglycerides was better than that of capric monoglycerides. Monolaurin was selected for the subsequent in vivo tests.
Clinical symptoms and scores
After the piglets were challenged with the virus and treated with monolaurin, the development and progression of their clinical symptoms were observed and monitored continuously for 14 days (Figure 3). One piglet from the low-dose group died at 2 dpi. There were no other piglet deaths recorded in this group on the subsequent days. One piglet from the model group died at 3 dpi. No piglets died in the middle-dose, high-dose, or control groups. The piglets in the low-dose and model groups showed decreased feed intake and symptoms such as anorexia, lethargy, and fever after the virus challenge. These piglets had blisters and ulcers on their snouts and hooves at 7 dpi. Except that erosions, ulcerations, and vesicular lesions of the snout, oral mucosa, and distal limbs, especially around the coronary band, as well as more general symptoms of illness such as fever, lethargy, and anorexia, may be observed from the model group. Hoof sloughing and lameness can also occur. By contrast the clinical symptoms, mental status, and feed intake of the monolaurin-treated piglets were better than those of piglets in the model group. The piglets in the middle-dose and high-dose groups had similar clinical symptoms, with a lower post-challenge score than that of the model group.
Post-challenge effects of monolaurin on SVV load
Piglet viral load peaked at 3 dpi and then continued to decline (Figure 3). Monolaurin reduced the viral load in the feces and blood of piglets infected with SVV in a dose-dependent manner (Figure 4). The treatment with high-dose Monolaurin was the most effective for viral load in fecal and blood of SVV-infected piglets. Compared with fecal viral load of SVV infected piglets, blood viral load decreased more significantly after 3 dpi (Figure 4B).
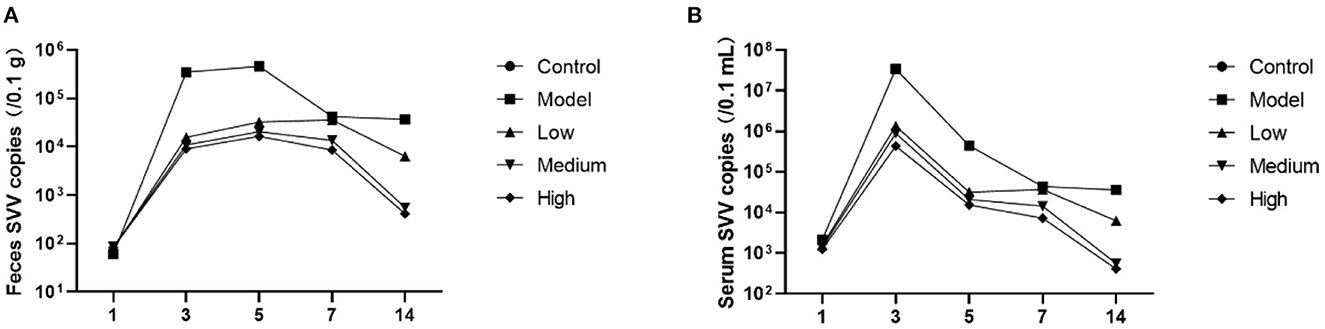
Figure 4. Viral load in the feces and blood of piglets infected with SVV. (A) Viral load in feces; (B) Viral load in blood.
Histopathological examination
Blisters and ulcers manifested on the snout and hoofs at 7 dpi. Pigs in the model and low-dose groups exhibited the following clinical manifestations: parts of the lung were atrophied, the alveolar septum was thickened, and the rest of the lung tissues had compensatory emphysema. In the model group, spleens showed diffuse hemorrhage, severe swelling of hepatocytes, partial cell necrosis, glomerular atrophy, and partial shedding of the renal tubular epithelium. There were no significant changes in the spleens, livers, and kidneys of pigs in the low-dose group. The pigs in the middle-dose and high-dose groups had alveoli without obvious lesions, while their spleens, kidneys, and livers had clear structures but no obvious lesions (Figure 5).
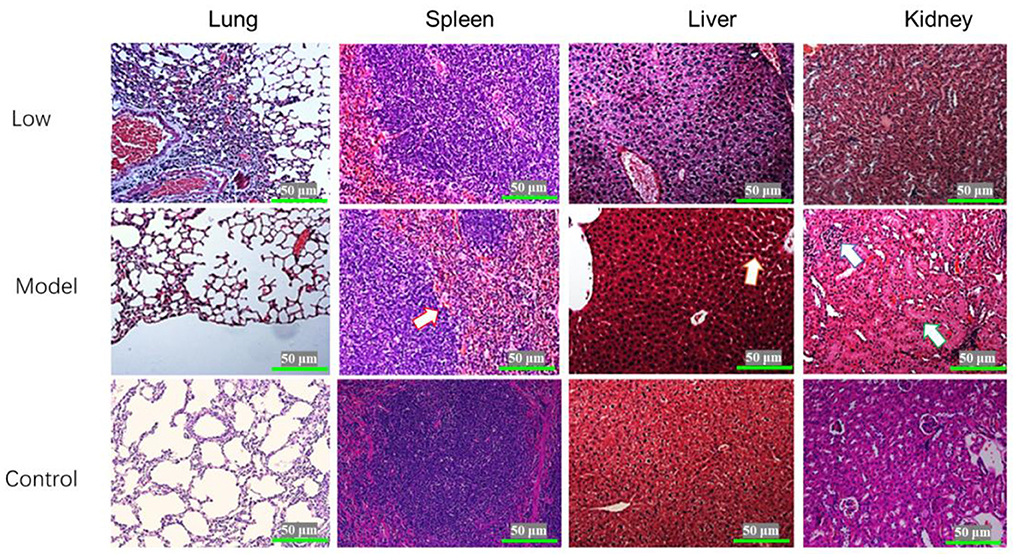
Figure 5. Histopathological examination of the lungs, spleen, liver, and kidneys of piglets infected with SVV (HE×400). The arrow on Model-spleen indicated the hemorrhage diffuse; the arrow on Model-liver indicated the cell swelling; and the arrows on Model-kidney indicated the glomerular atrophy and tubular swelling.
In vivo detection of inflammatory cytokines
Proinflammatory cytokines were significantly increased by SVV infection, and treatment with monolaurin showed some degree of anti-inflammatory activity (Figure 6). High doses of monolaurin significantly decreased the levels of IL-1β, IL-10, and TNF-α to levels (p < 0.05), without a significant difference compared with the corresponding control groups (p > 0.05). However, with the increase of the monolaurin dose, the trend of the increase of IFN-γ level is more obvious. So, high doses of monolaurin significantly increased the amount of IFN-γ in a dose-dependent manner.
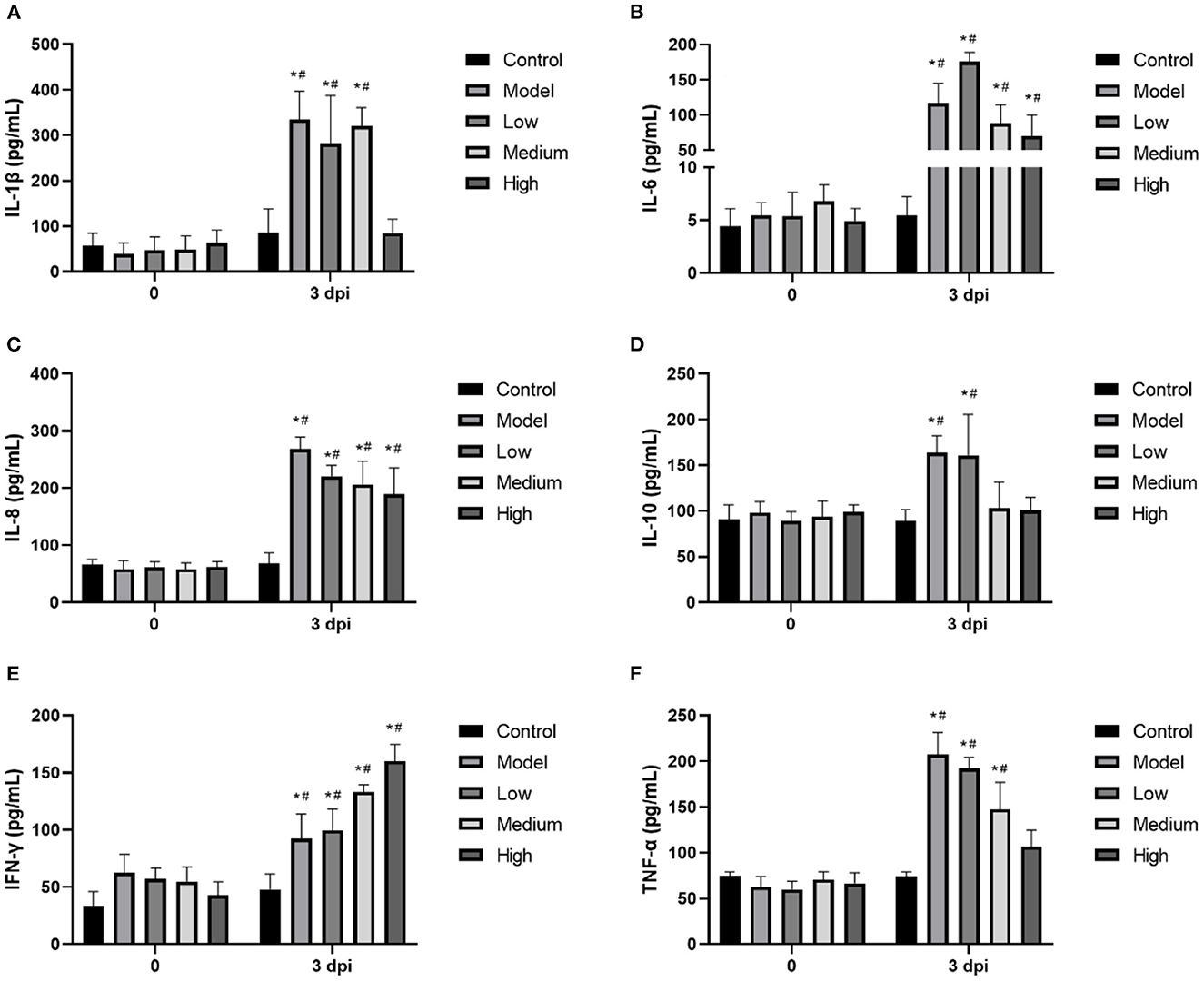
Figure 6. (A–F) The relative mRNA expressions of cellular inflammatory factors IL-1β, IL-6, IL-8, IL-10, IFN-γ. and TNF-α in piglet blood. Effects of monolaurin on cytokine release in piglet blood infected with SVV: *p < 0.05 vs. 0 dpi, #p < 0.05 vs. control.
Discussion
Monolaurin, a monoglyceride formed from 12-carbon saturated fatty acids and glycerol, is naturally found in coconut oil, palm oil, and breast milk, and is a safe and highly effective monoglyceride with bacteriostatic activity (14). Monolaurin has broad antibacterial activities, including inhibiting bacterial growth, reducing the production of exotoxin, and forming biofilms (15). As a lipid, monolaurin can bind to the phospholipid bilayer of bacteria and disrupt the normal physiological processes of the bacteria, thereby inducing a bacteriostatic effect. Monolaurin is also reported to have a strong inhibitory effect on the growth and reproduction of gram-positive bacteria such as Staphylococcus aureus, Listeria monocytogenes, Helicobacter pylori, Bacillus, and Campylobacter jejuni, among others (16). Furthermore, monolaurin can block the release of gram-positive bacterial exotoxins (such as enterotoxins and streptococcal pyrogenic exotoxins, etc.) (17). Monolaurin can also bind to the lipid bilayer membrane enveloped viruses and inhibit viral activity by compromising viral integrity and infectivity. Monolaurin has shown a good inhibitory effect on some enveloped viruses, such as the HSV, influenza virus, PRRS virus, and porcine epidemic diarrhea virus (18, 19). In this study, we show that monolaurin has a strong inhibitory effect on SVV even though it is a non-enveloped virus. However, the anti-SVV mechanism of monolaurin needs further research.
Even though SVV causes blisters and ulcers on the snout and hoofs of pigs, there are only a few reports on autopsy symptoms and microscopic pathogenesis of this viral infection. Pathological experiments in this study showed lesions in the lungs, livers, spleens, and kidneys of infected piglets. Preventing SVV from destroying the integrity of the intestinal barrier helps to reduce the damage of the virus. Monolaurin has great potential for application in animal health, as it promotes growth and gut health. For example, some studies have found that monolaurin can significantly improve the growth performance of weaned piglets (20, 21). Additionally, dose-related monolaurin has been found to improve body weight, regulation of gut microbiota, and systemic inflammation in mice fed a low-fat diet (22). These studies show a significant positive correlation between monolaurin and the increased abundance of probiotics, such as Lactobacillus reuteri and Ruminococcus gnavus (22). Normal intestinal flora is necessary for the integrity of the tight junctions of the intestinal tract. Here, monolaurin significantly improves the health of the intestinal tract, which reduces the chance of viruses invading the intestinal epithelial cells and the bloodstream. Our findings corroborate the findings of these previous studies, as we found that monolaurin-treated pigs had significantly reduced viral loads in their blood and feces as well as reduced clinical symptoms associated with SVV infection. During the trial, one piglet from the low-dose group died at 2 dpi, one piglet from the model group died at 3 dpi. No piglets died in the middle-dose, high-dose.
Many viruses can induce inflammatory responses and even cause an inflammatory factor storm (23). The mechanism is the excessive activation of immune cells by increasing intracellular inflammatory factors, including interleukin, TNF-α, and complement protein molecules (24). The storm-like suicide attack induced by pathogenic microorganisms in infected cells can cause bystander damage to other tissues by increasing vascular permeability and circulatory disorders, which can even result in multiple organ functional failure (MOF) (25). Usually, inflammation is a protective immune response that is conducive to clearing pathogenic microorganisms. However, uncontrollable excessive inflammation can cause autoimmune damage (26). In this study, we observed that SVV infection induced the release of many inflammatory cytokines, including IL-1β, IL-6, IL-8, IL-10, and TNF-α, triggering an inflammatory cytokine storm. Previous studies have found that monolaurin affects the lipid dynamics of human T cells and regulates T-cell signaling and the release of functional factors. It also inhibits the immune response that is overactivated by the virus, thereby reducing the amount of SVV-induced inflammatory cells (27). Seneca Valley virus infection can reduce the level of IFN-γ in the serum, hence reducing the antiviral activity of the body. On the other hand, monolaurin can increase the level of IFN-γ, possibly explaining one of the anti-viral mechanisms of MCFAs.
The results from this study support the efficacy of Monolaurin against SVV. Data suggest that monolaurin block virus proliferation and systemic inflammation response. Despite the promising results, our sample sizes were small and it is not clear whether other mechanisms are involved in the regulation of the efficacy of antiviral therapies. A large-scale evaluation of the anti-viral potential and mechanism of monolaurin should be the subject of future studies.
Data availability statement
The original contributions presented in the study are included in the article/supplementary material, further inquiries can be directed to the corresponding authors.
Ethics statement
The animal study was reviewed and approved by the Animal Ethical Committee of Sichuan Agricultural University.
Author contributions
BS, YW, and SJ conceived of the presented idea. HT and HD developed the theory and performed the computations. All authors contributed to the article and approved the submitted version.
Conflict of interest
XZ, JL, HC, and YH were employed by Innovation Center of Guangdong Nuacid Biotechnology Co., Ltd.
The remaining authors declare that the research was conducted in the absence of any commercial or financial relationships that could be construed as a potential conflict of interest.
Publisher's note
All claims expressed in this article are solely those of the authors and do not necessarily represent those of their affiliated organizations, or those of the publisher, the editors and the reviewers. Any product that may be evaluated in this article, or claim that may be made by its manufacturer, is not guaranteed or endorsed by the publisher.
References
1. Venkataraman S, Reddy SP, Loo J, Idamakanti N, Hallenbeck PL, Reddy VS. Structure of Seneca Valley virus-001: an oncolytic picornavirus representing a new genus. Structure. (2008) 16:1555–61. doi: 10.1016/j.str.2008.07.013
2. Vannucci FA, Linhares D, Barcellos D, Lam H, Collins J, Marthaler D. Identification and complete genome of Seneca Valley virus in vesicular fluid and sera of pigs affected with idiopathic vesicular disease, Brazil. Transbound Emerg Dis. (2015) 62:589–93. doi: 10.1111/tbed.12410
3. Joshi LR, Fernandes MH, Clement T, Lawson S, Pillatzki A, Resende TP, et al. Pathogenesis of Senecavirus A infection in finishing pigs. J Gen Virol. (2016) 97:3267–79. doi: 10.1099/jgv.0.000631
4. Montiel N, Buckley A, Guo B, Kulshreshtha V, VanGeelen A, Hoang H, et al. Vesicular disease in 9-week-old pigs experimentally infected with Senecavirus A. Emerg Infect Dis. (2016) 22:1246. doi: 10.3201/eid2207.151863
5. Xue Q, Liu H, Zhu Z, Yang F, Ma L, Cai X, et al. Seneca Valley virus 3Cpro abrogates the IRF3-and IRF7-mediated innate immune response by degrading IRF3 and IRF7. Virology. (2018) 518:1–7. doi: 10.1016/j.virol.2018.01.028
6. Dahmer PL, Leubcke GE, Lerner AB, Jones CK. Effects of medium-chain fatty acids as alternatives to ZnO or antibiotics in nursery pig diets. Transl Anim Sci. (2020) 4:txaa151. doi: 10.1093/tas/txaa151
7. Lawrence JM, Lerner AB, Jones CK. The Effect of Feeding Medium Chain Fatty Acids (MCFA) on Nursery Pig Growth in an Attempt to Replace ZnO or Carbadox, Animal Sciences and Industry Undergraduate Research Symposium. Spring (2019).
8. Hanczakowska E, Swiatkiewicz M, Natonek-Wiśniewska M, Okoń K. Medium chain fatty acids (MCFA) and/or probiotic Enterococcus faecium as a feed supplement for piglets. Livest Sci. (2016) 192:1–7. doi: 10.1016/j.livsci.2016.08.002
9. Huang CB, Alimova Y, Myers TM, Ebersole JL. Short-and medium-chain fatty acids exhibit antimicrobial activity for oral microorganisms. Arch Oral Biol. (2011) 56:650–4. doi: 10.1016/j.archoralbio.2011.01.011
10. Desbois AP. Potential applications of antimicrobial fatty acids in medicine, agriculture and other industries. Recent Pat Antiinfect Drug Discov. (2012) 7:111–22. doi: 10.2174/157489112801619728
11. Thormar H, Isaacs CE, Brown HR, Barshatzky MR, Pessolano T. Inactivation of enveloped viruses and killing of cells by fatty acids and monoglycerides. Antimicrob Agents Chemother. (1987) 31:27–31. doi: 10.1128/AAC.31.1.27
12. Hilmarsson H, Kristmundsdottir T, Thormar H. Virucidal activities of medium-and long-chain fatty alcohols, fatty acids and monoglycerides against herpes simplex virus types 1 and 2: comparison at different pH levels. APMI. (2005) 113:58–65. doi: 10.1111/j.1600-0463.2005.apm1130109.x
13. Jackman JA, Hakobyan A, Zakaryan H, Elrod CC. Inhibition of African swine fever virus in liquid and feed by medium-chain fatty acids and glycerol monolaurate. J Anim Sci Biotechnol. (2020) 11:1–10. doi: 10.1186/s40104-020-00517-3
14. Jackman JA, Boyd RD, Elrod CC. Medium-chain fatty acids and monoglycerides as feed additives for pig production: towards gut health improvement and feed pathogen mitigation. J Anim Sci Biotechnol. (2020) 11:1–15. doi: 10.1186/s40104-020-00446-1
15. Rozenbaum RT, Su L, Umerska A, Eveillard M, Håkansson J, Mahlapuu M, et al. Antimicrobial synergy of monolaurin lipid nanocapsules with adsorbed antimicrobial peptides against Staphylococcus aureus biofilms in vitro is absent in vivo. J Control Release. (2019) 293:73–83. doi: 10.1016/j.jconrel.2018.11.018
16. Yoon BK, Jackman JA, Valle-González ER, Cho NJ. Antibacterial free fatty acids and monoglycerides: biological activities, experimental testing, and therapeutic applications. Int J Mol Sci. (2018) 19:1114. doi: 10.3390/ijms19041114
17. Preuss HG, Echard B, Dadgar A, Talpur N, Manohar V, Enig M, et al. Effects of essential oils and monolaurin on Staphylococcus aureus: in vitro and in vivo studies. Toxicol Mech Methods. (2005) 15:279–85. doi: 10.1080/15376520590968833
18. Hierholzer JC, Kabara JJ. In vitro effects of monolaurin compounds on enveloped RNA and DNA viruses 1. J Food Saf. (1982) 4:1–12. doi: 10.1111/j.1745-4565.1982.tb00429.x
19. Lieberman S, Enig MG, Preuss HG. A review of monolaurin and lauric acid: natural virucidal and bactericidal agents. Altern Complemen Ther. (2006) 12:310–4. doi: 10.1089/act.2006.12.310
20. Ren C, Wang Y, Lin X, Song H, Zhou Q, Xu W, et al. A combination of formic acid and monolaurin attenuates enterotoxigenic Escherichia coli induced intestinal inflammation in piglets by inhibiting the NF-κB/MAPK pathways with modulation of gut microbiota. J Agric Food Chem. (2020) 68:4155–65. doi: 10.1021/acs.jafc.0c01414
21. Thomas LL, Woodworth JC, Tokach MD, Dritz SS, DeRouchey JM, Goodband RD, et al. Evaluation of different blends of medium-chain fatty acids, lactic acid, and monolaurin on nursery pig growth performance. Transl Anim Sci. (2020) 4:548–57. doi: 10.1093/tas/txaa024
22. Li Y, Liu T, Zhang X, Zhao M, Zhang H, Feng F. Lactobacillus plantarum helps to suppress body weight gain, improve serum lipid profile and ameliorate low-grade inflammation in mice administered with glycerol monolaurate. J Funct Foods. (2019) 53:54–61. doi: 10.1016/j.jff.2018.12.015
23. Gupta KK, Khan MA, Singh SK. Constitutive inflammatory cytokine storm: a major threat to human health. J Interferon Cytokine Res. (2020) 40:19–23. doi: 10.1089/jir.2019.0085
24. Pan P, Shen M, Yu Z, Ge W, Chen K, Tian M, et al. SARS-CoV-2 N protein promotes NLRP3 inflammasome activation to induce hyperinflammation. Nat Commun. (2021) 12:4664. doi: 10.1038/s41467-021-25015-6
25. Iwasaki M, Saito J, Zhao HL, Sakamoto A, Hirota K, Ma DQ. Inflammation triggered by SARS-CoV-2 and ACE2 augment drives multiple organ failure of severe COVID-19: molecular mechanisms and implications. Inflammation. (2021) 44:13–34. doi: 10.1007/s10753-020-01337-3
26. Deretic V. Autophagy in inflammation, infection, and immunometabolism. Immunity. (2021) 54:437–53. doi: 10.1016/j.immuni.2021.01.018
Keywords: MCFA, antiviral agent, monolaurin, SVV, inflammatory response
Citation: Su B, Wang Y, Jian S, Tang H, Deng H, Zhu L, Zhao X, Liu J, Cheng H, Zhang L, Hu Y and Xu Z (2023) In vitro and in vivo antiviral activity of monolaurin against Seneca Valley virus. Front. Vet. Sci. 10:980187. doi: 10.3389/fvets.2023.980187
Received: 28 June 2022; Accepted: 10 January 2023;
Published: 27 January 2023.
Edited by:
Dayou Shi, South China Agricultural University, ChinaReviewed by:
Hongbin Si, Guangxi University, ChinaAhmed Nisar, Lasbela University of Agriculture, Water and Marine Sciences, Pakistan
Yi Wu, Nanjing Agricultural University, China
Copyright © 2023 Su, Wang, Jian, Tang, Deng, Zhu, Zhao, Liu, Cheng, Zhang, Hu and Xu. This is an open-access article distributed under the terms of the Creative Commons Attribution License (CC BY). The use, distribution or reproduction in other forums is permitted, provided the original author(s) and the copyright owner(s) are credited and that the original publication in this journal is cited, in accordance with accepted academic practice. No use, distribution or reproduction is permitted which does not comply with these terms.
*Correspondence: Youjun Hu, NzQ4ODEwMzk5QHFxLmNvbQ==; Zhiwen Xu,
YWJ0Y3h6d0AxMjYuY29t
†These authors share first authorship