- 1Department of Obstetrics, Gynecology and Reproductive Sciences, University of California, San Diego, San Diego, CA, United States
- 2Glycobiology Research and Training Center, University of California, San Diego, San Diego, CA, United States
- 3Center for Predictive Medicine, University of Louisville, Louisville, KY, United States
- 4Department of Toxicology and Forensic Medicine, Cairo University, Faculty of Veterinary Medicine, Cairo, Egypt
- 5Center for Academic Research and Training in Anthropogeny, University of California, La Jolla, La Jolla, CA, United States
- 6Department of Pathology, University of California, San Diego, San Diego, CA, United States
- 7Department of Pediatrics, Division of Infectious Diseases, Washington University School of Medicine, St. Louis, MO, United States
- 8Center for Women’s Infectious Disease Research, Washington University School of Medicine, St. Louis, MO, United States
- 9Department of Bioengineering, University of Louisville Speed School of Engineering, Louisville, KY, United States
- 10Department of Pharmacology and Toxicology, University of Louisville School of Medicine, Louisville, KY, United States
- 11UofL Health – Brown Cancer Center, University of Louisville, Louisville, KY, United States
Introduction: Communities of bacteria collectively known as the vaginal microbiota reside in the human vagina. Bacterial vaginosis (BV) describes an imbalance of this microbiota, affecting more than 25% of women worldwide, and is linked to health problems such as infertility, cervical cancer, and preterm birth. Following antibiotic treatment, BV becomes recurrent in many individuals. Lactobacillus crispatus is widely believed to contribute to a healthy vaginal microbiome, and its therapeutic application has shown promise in early clinical trials investigating adjunct therapies for lasting treatment of conditions such as BV. There is a pressing need for therapeutic platforms that apply biologically active agents such as probiotic bacteria, to the vagina with little user effort but lasting effect.
Methods: Here, we use a mouse model to investigate the functional utility and potential harms of soft, slow-dissolving fibers made by electrospinning polyethylene oxide (PEO) and poly(lactic-co-glycolic acid) (PLGA). Blank electrospun fibers that passed quality control checkpoints were administered vaginally in a murine model and compared to animals receiving mock procedures.
Results: Fiber administration had no significant effects on mucus glycan markers, vaginal epithelial exfoliation, keratinization, tissue edema or neutrophil infiltration. L. crispatus-loaded fibers enabled L. crispatus colonization in most animals for more than one week. Mice receiving L. crispatus-loaded fibers had significantly higher measured concentrations of lactate in vaginal washes at 48 hrs compared to pre-colonization washes.
Discussion: These data provide pre-clinical proof of concept that vaginal administration of electrospun fibers can achieve viable delivery and vaginal colonization by metabolically active L. crispatus, without eliciting inflammation or injury.
Introduction
The microbiota of the human vagina has been linked with many health complications. Lactobacillus species are associated with better health outcomes when abundant in the vagina and produce multiple types of antimicrobial molecules, including lactic acid. In the condition bacterial vaginosis (BV), lactobacilli that typically act to thwart potential pathogens are in short supply and diverse potential pathogens can colonize the vagina. BV has been associated with preterm birth and low birth weight (Svare et al., 2006; Ralph et al., 1999), sexually transmitted infections (Wiesenfeld et al., 2003; Brotman et al., 2010; Wijgert, 2017), pelvic inflammatory disease (Ness et al., 2005), infertility (Spandorfer et al., 2001), cervical cancer (Di Paola et al., 2017; Brusselaers et al., 2019; Łaniewski et al., 2018), and other health complications. The health burden of BV and the substantial global cost of treatment is compounded by the fact that BV recurs in most individuals within months of standard antibiotic treatments such as metronidazole. In one study of bacterial dynamics during and following metronidazole treatment of BV, some bacterial taxa were reduced below the threshold of detection. However, other taxa implicated in BV were unstable, reduced only incrementally with treatment, and rebounded after antibiotic cessation (Mayer et al., 2015). In short, better treatment modalities are needed that extend the release of active agents to tip the balance in favor of lactobacilli.
In response to this need, probiotic Lactobacillus species have been administered vaginally to treat BV and reduce its recurrence, typically requiring many administrations and improving outcomes only modestly (Pendharkar et al., 2015; Mastromarino et al., 2013; Tomás et al., 2020; Mastromarino et al., 2009; Vivekanandan et al., 2024). L. acidophilus is one of the first and best characterized bacterial species used in fermented food products (Bull et al., 2013). In spite of its reputation as an intestinal species, L. acidophilus has also been isolated from the human vagina and has also been used in oral and vaginal administration in murine and clinical studies intended to treat BV (Drago et al., 2007; Ya et al., 2010; Lin et al., 2021; Jang et al, 2017). Lactobacillus crispatus is a more prevalent and abundant member of the human vaginal microbiota and may be a more relevant choice compared to L. acidophilus as a vaginal probiotic. When used aggressively following antibiotic treatment, individuals receiving vaginal L. crispatus after antibiotic treatment had significantly lower recurrence rates of recurrent BV and urinary tract infections (Stapleton et al., 2011; Armstrong et al., 2022; Cohen et al., 2020; Qi et al., 2023).
Our long-term goal is to use electrospinning technology to create soft, slowly dissolving platforms that release probiotic bacteria and other active agents. This work builds on several prior studies fabricating and testing electrospun fibers and other novel modalities in vitro and in preclinical models, from our group and others, for their abilities to incorporate and release antibiotics and probiotics (Herold et al., 2023; Kyser et al., 2023, 2023, 2024; Minooei et al., 2023, 2023). We have used initial formulations of rapidly dissolving fibers made of polyethylene oxide (PEO) as a vehicle for the vaginal administration of antibiotics and probiotics. These rapidly dissolving electrospun fibers could capably deliver the antibiotic metronidazole to the mouse vagina, discouraging Gardnerella colonization (Minooei et al., 2023). Additional proof of concept showed that these rapidly dissolving PEO electrospun fibers could yield viable recovery of L. acidophilus from the mouse vagina for up to 72 hours post-insertion (Minooei et al., 2023). In addition to the hydrophilic PEO rapid release formulations, we have aimed to develop more slowly dissolving platforms with hydrophobic components such as poly(lactic-co-glycolic acid) (PLGA). For example, we previously showed that ‘slow dissolve’ L. crispatus-loaded fibers containing PLGA could be repeatedly cultured for up to two weeks in vitro to yield robust numbers of metabolically active L. crispatus. Our team has also characterized several formulations of these fibers using in vitro assays that establish their abilities to encase and release viable bacteria whose metabolic products can inhibit Gardnerella without harming human cell monolayers (Minooei et al., 2023, 2023; Mahmoud et al., 2023).
Here we used an estrogenized mouse model in which β-estradiol-17-valerate induces a proestrus-like state known to encourage colonization by human vaginal bacteria. Our goal was to identify prototypes of PLGA-containing fibers to achieve Lactobacillus vaginal colonization in vivo over an extended period. Our preclinical studies investigated these novel vaginal probiotic delivery modalities in a total of N=85 mice. The potential harms of vaginal administration were examined, including histological changes in the vagina and cervix. We also measured the functional capacity of the fibers to deliver viable L. crispatus that can colonize the mouse vagina. Together, these studies establish proof of concept for the use of electrospun fibers to establish extended release of probiotic bacteria vaginally in vivo, with minimal apparent harm.
Methods
Bacterial strains and culture conditions
Lactobacillus crispatus MV-1A-US was purchased from American Type Culture Collection (ATCC). Mouse experiments used a spontaneous streptomycin-resistant isolate of MV-1A-US. Briefly, L. crispatus was cultured anaerobically overnight in deMan, Rogosa, and Sharpe (MRS) broth (BD™ Difco™) at 37°C, then plated on MRS agar with 1 mg/mL streptomycin sulfate to select for spontaneous streptomycin-resistant colonies. Colonies were cultured in MRS broth. Identity was confirmed by sequencing the rpsL gene using primers Fsequence 5’ TCAACCCAGCTCCACAAATG 3’ and RevSequence 5’ GTCCTTTACACGACCACCAC 3’. A missense mutation on the codon 175 of the rpsL gene led to K-56 → R substitution. The L. crispatus, prepared for fiber production, was grown using MRS (Sigma 69966) broth and agar plates, supplemented with 0.1% Tween 80. Bacterial cultures were initially prepared from frozen stocks using an inoculation loop to streak culture on the agar plates. Inoculated plates and maintained at 37°C under anaerobic conditions using 5% hydrogen, 5% carbon dioxide, and balanced with nitrogen using a Bactronez Anaerobic Workstation (Shel Lab). The incubation time to observe growth of L. crispatus was 48 h. A single bacteria colony was selected from the plate and cultured in 1 mL of MRS in a closed microcentrifuge tube for 48 h at 37°C. Bacteria were subsequently sub-cultured by diluting 200 µL of L. crispatus with 9.8 mL of MRS broth (1:50 dilution) and grown for 24 h before harvest. The method of determining the correlation between the OD600 and respective CFU counts was reported previously (Mahmoud et al., 2023). One absorbance unit at 600nm for L. crispatus, was found to be equivalent to 5.8 x 107 CFU/mL.
Electrospun fiber design and fabrication
Methods for synthesis of probiotic-loaded fibers have been previously described (Minooei et al., 2023, 2023). Briefly, PLGA (50:50, 0.55–0.75 dL/g, 31-57 kDa MW) and PEO (600,000 MW) were purchased from Lactel Absorbable Polymers (Cupertino, CA) and Sigma Aldrich (St. Louis, MO), respectively. To fabricate blank fibers, PLGA (15% w/w) and PEO (5% w/w) were dissolved in 3 mL of hexafluoro-2-propanol (HFIP, Fisher Scientific, Waltham, MA, USA) and MRS broth, respectively and were incubated at 37°C overnight. For probiotic-containing formulations, 150 mg of PEO w/w was added to 2.5 mL MRS broth and immediately before electrospinning, 0.5 mL of MRS incorporating 5 x 107 CFU L. crispatus/mg PEO was added to the PEO solution. To minimize the risk of fiber contamination, polymer solutions were passed through a 0.45 µm syringe filter (VWR, PA, USA) before probiotic incorporation, and electrospinning box and all materials in contact with fibers were sterilized prior to electrospinning. During electrospinning, a positive voltage of 18 and 25 kV was applied at the tip of a needle connected to two syringes containing PLGA and PEO (for ‘slowdissolve ‘ formulation), respectively. The electrospun fibers were collected on a rotating, 8 mm outer diameter stainless steel mandrel, positioned 18 cm from the blunt needle tip of the PLGA syringe and 15 cm from the needle tip of PEO syringe. Syringe flow rates were maintained at 0.3 mL/h for both PLGA and PEO to fabricate PLGA: PEO (1:1) fibers, while flow rates of 0.1 and 0.3 mL/h were used to fabricate PLGA: PEO (1:3) fibers. The collector was placed between the PLGA and PEO syringes at a distance of 18 and 15 cm, respectively, and fibers were collected simultaneously on the collector.
Electrospun fibers quality control
Blank fibers were assessed for bacterial contamination by incubating the fiber in Tryptic Soy Broth and Brain Heart Infusion Broth (BD Difco™) for up to 5 days. Contamination of L. crispatus-loaded fibers was assessed by chromogenic agar (CHROMagar™ Orientation). Lipopolysaccharide (LPS) contamination for both blank and loaded fibers was assessed by a chromogenic Limulus Amoebocyte Assay (LAL) (Associates of Cape Cod, Inc.). All fibers used in this study had endotoxin levels less than 5 EU/disk. A companion paper will be published to fully describe our quality control pipeline.
Estrogenized mouse model
6–8-week-old conventional female C57Bl/6J mice (Jackson Labs) were used for in vivo experiments in accordance with our approved IACUC protocol (#S20057) from the University of California San Diego. Mice were rested for at least four days after their arrival before experiments commenced. Vaginal washes from mice carried out pre-infection did not reveal any streptomycin resistance within the endogenous microbiota cultured on MRS agar media with 1 mg/ml streptomycin. During the course of this experiment, animals were housed using a 12:12 light:dark cycle in disposable plastic cages with bed o’cobb bedding in a BSL-2-approved room under negative pressure. The mice received two 0.1 cc intraperitoneal injections of 5 mg/mL β-estradiol-17-valerate (Sigma-AldrichⓇ) diluted in National Formulary grade sesame oil (SpectrumⓇ Chemical). The first injection was delivered 2-3 days prior to fiber insertion, while the second was delivered on the day of insertion.
Mouse vaginal fiber insertion
Discs were inserted with metal forceps that were previously baked at 200°C for 2 h and autoclaved at 121°C for 20 minutes (min). A sterile P200 pipette tip was used to push the disk closer to the cervix. Mice undergoing the mock procedure were treated with forceps inserted into the vagina for 20 seconds and a pipette tip inserted for 10 seconds.
Vaginal lavage (wash)
Vaginal wash tubes were prepared by pipetting 70 µL of sterile Dulbecco’s PBS (-Mg, -Cl) (Gibco™) in a microcentrifuge tube in anaerobic conditions. 50 L of PBS were pipetted into the mouse vagina with a P200 pipette. The pipette tip was inserted 2-5 mm into the mouse vagina. For probiotic fibers, washes were returned to the anaerobic chamber and 10 µL were diluted into 90 L of dPBS. The wash was serially diluted, and dilutions were spot plated on Lactobacillus MRS agar with 1 mg/mL streptomycin sulfate. An additional 10 L of undiluted wash were plated for 48 h and later washes. Plates were incubated anaerobically at 37°C for 24 hours (h).
Fixation and histology
After the mice were euthanized, the vagina and cervix were removed, placed in cassettes, and fixed in 10% formalin for 24 h. After 24 h, the cassettes were transferred to 70% ethanol. The vagina and cervix were paraffin-embedded, sectioned onto slides, and H&E (hematoxylin and eosin) stained.
Histological scoring and analysis
A pathologist made observations about keratin, edema, and polymorphonuclear cells (surface and stromal) for the vagina and cervix using a Keyence microscope. The pathologist was blinded from the individual groups’ treatments. Observations were ranked from the most severe case to the least severe case and given a numeric value in 1 point increments. Keratin was assessed by presence and thickness on the epithelium on a scale of 0-2. Edema was assessed by the presence and severity on a scale of 0-2. Polymorphonuclear cells were assessed by the presence and quantity on a scale of 0-4. Scoring system is described in Figure 1C.
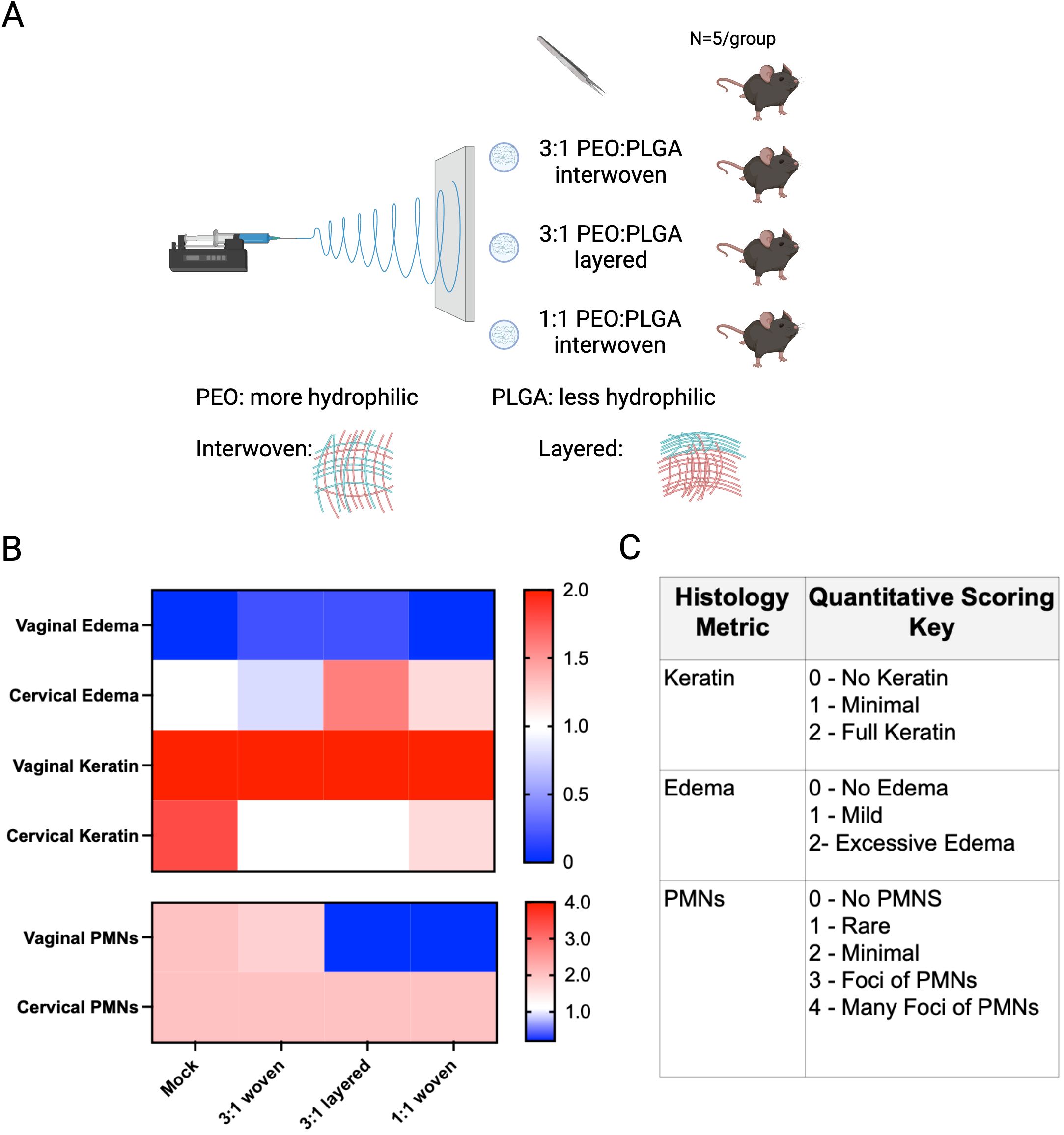
Figure 1. Histopathology of vaginal and cervical tissues, 24 h after insertion of electrospun fibers made of PEO-PLGA in β-estradiol-valerate-treated C57Bl/6J mice, compared to a group that received the mock procedure. (A) Experimental schematic of the initial in vivo experiment. (B) Heat map depicting average histological scores for each group of mice. Keratin and edema were scored on a scale of 0 to 2, where polymorphonuclear cells (PMNs) were scored on a scale of 0 to 4. (C) Scoring method used to score histological samples. Observers were blinded to the experimental groups. These experiments were only powered to observe phenotypes with a large effect size.
Epithelial exfoliation
10 µL of vaginal wash were resuspended and placed on a microscope slide, and a coverslip was added to make a wet mount that was visualized via phase contrast microscopy (Leica). Under 100x magnification, five fields of view were photographed. Epithelial cells were counted in each field of view, and the five fields were averaged for each mouse. Although the numbers of shed epithelial cells vary widely between animals, we calculated that we had 90% power to detect a 2-fold difference between the mock and treated groups (20 mice per group, alpha = 0.05). Previous experiments detected exfoliation in murine (Gilbert et al., 2013, 2019) and human (Amegashie et al., 2017) contexts.
Sialic acid quantification
25 µL of vaginal washes were used to quantify total sialic acid (N-acetyl neuraminic acid) concentration using previously published methods (Gilbert et al., 2013). Sialic acids from glycans in vaginal washes were first released using 2M acetic acid for 3 h at 80°C. Hydrolyzed vaginal washes were DMB-derivatized for 2 h at 50°C. Derivatized vaginal washes were analyzed using HPLC with fluorescence detection (excitation: 373 nm, detection: 448 nm) using pure N-acetyl neuraminic acid as a standard.
Lactate quantification
10 µL of vaginal washes were used to quantify total lactate concentration in mice that were administered the L. crispatus-loaded fibers. Lactic acid was measured using a modified protocol for the D-L-Lactic Acid Assay Kit (Megazyme). 10 µL of vaginal wash were added to a master mix consisting of 20 µL of the provided buffer, 8 µL of NAD+, 0.8 L of D-GPT, and 37.36 L milliQ water in a half-area flat bottom UV-transparent plate (Greiner). A standard curve was generated using D- and L- lactic acid, ranging from 3.12 mM to 0.195 mM. Plates were read initially at 340 nm for 2 minutes to establish stability. 0.8 µL of the provided L-LDH and D-LDH suspensions were added to each well. Plates were read at 340 nm for 20 minutes.
Statistics and data analysis
Data were processed using GraphPad Prism Version 10.2.3. Histological scores, sialic acid concentration, and epithelial cell shedding were analyzed using the Mann Whitney U-test. The correlation analysis between L. crispatus titers and lactic acid concentration used the Spearman correlation coefficient. Friedman’s test was used to analyze matched pairs of the L. crispatus titers over time, as well as the lactic acid concentration. Fisher’s exact was used to analyze the changes in the number of mice with detectable lactic acid. Each test is described in figure legends.
Results
Pilot studies in mice to examine epithelial and inflammatory potential of vaginally administered electrospun fibers of different composition
To investigate the utility of electrospun fibers in the vagina in vivo, we used a murine model (Figure 1). Briefly, 6–8-week-old mice were estrogenized with long-acting β-estradiol-17-valerate prior to vaginal inoculation with bacteria. Pilot studies in a total of n=35 estrogenized mice were performed to investigate epithelial changes that may indicate an adverse response to fibers. A variety of initial prototype designs were tested, and these individuals were compared to animals that received a mock procedure in parallel. Initial studies used n=5 mice, distributed amongst seven experimental groups to look for large effect sizes. Fibers were created by electrospinning hydrophilic PEO with varying the rate of poly(lactic-co-glycolic acid) (PLGA) incorporation (1:3 or 1:1). Fiber architectures were also varied by spinning the two components simultaneously or, alternately, by creating interwoven or layered fibers respectively (Figures 1A-C). In the first experiments, histopathological features were scored 24 h after insertion. This approach was intended to identify and exclude prototypes with potentially large effect sizes that skewed away from the normal phenotypes. Among the 35 mice initially examined, several groups of mice were tested with each electrospun fiber prototype and compared to mock procedures. The goal was to select a fiber composition whose in vivo effects most resembled mice receiving mock procedures.
In general, the insertion of electrospun fibers did not appear to have major effects on the tissues. In all of the mice, the appearance of vaginal epithelial keratinization remained uniform. In contrast to the vaginal epithelium, epithelial cells of the ectocervix were somewhat less keratinized in experimental groups in which fibers were placed (Figure 1B, compared to mock procedure). Enumeration of epithelial cells present in vaginal washes due to exfoliation did not reveal a difference between mock and fiber-exposed groups. There was little if any vaginal edema in mice receiving devices or mock procedures. Polymorphonuclear cells (PMNs) were evident at low levels in tissues of the vagina and uterine cervix in the control mice. Although our pilot studies were not powered to detect small differences in individual designs, initial testing in n=25 mice led us to choose the PEO: PLGA (3:1) fiber composition with a woven architecture as likely to be the most biocompatible. Higher levels of PLGA, as in the PEO: PLGA (1:1) fiber, resulted in fewer immune cells than in the normal control mice (Figure 1B). While such an effect could theoretically contribute to benefiting some clinical settings, the goal was to select the most inert formulation that best represented the control group. Edema was comparable in the 3:1 group and the mock group, while the other fibers were more likely to result in moderate to severe cervical edema (Figure 1B). Thus, the 3:1 PEO: PLGA woven fiber was chosen for the next iteration of experiments, designed with larger sample sizes that are better powered to detect epithelial or inflammatory changes.
3:1 PEO: PLGA interwoven fibers did not trigger histological changes in the vagina or cervix in a mouse model
Next, we tested the PEO: PLGA (3:1) blank fibers selected from our early experiments, in a larger number of animals, comparing blank fibers to mock procedures and looking at a relatively longer post insertion endpoint of 72 h. Fibers were inserted into the vaginas of female C57Bl/6J mice, according to the schematic in Figure 2A. Electrospun fibers with high levels of lipopolysaccharide (< 2.0 Endotoxin Units, see methods) were excluded from in vivo experiments. Animals were compared to a mock group where forceps were inserted without a fiber using the same maneuver (n=40 total using n=10 per group in two independent experiments). At 72 h post insertion of the fibers, each mouse underwent vaginal washing for enumeration of exfoliated epithelial cells and changes in mucus characteristics (Figures 2B–D). Mice were then euthanized, followed by removal of the vagina and cervix and fixation of tissues in buffered formalin. The tissues were paraffin-embedded, sectioned, and stained with hematoxylin and eosin (H&E). Observers blinded to the experimental groups provided scores for several structured metrics, such as presence of keratinized epithelial layers and edema (Figures 3, 4A).
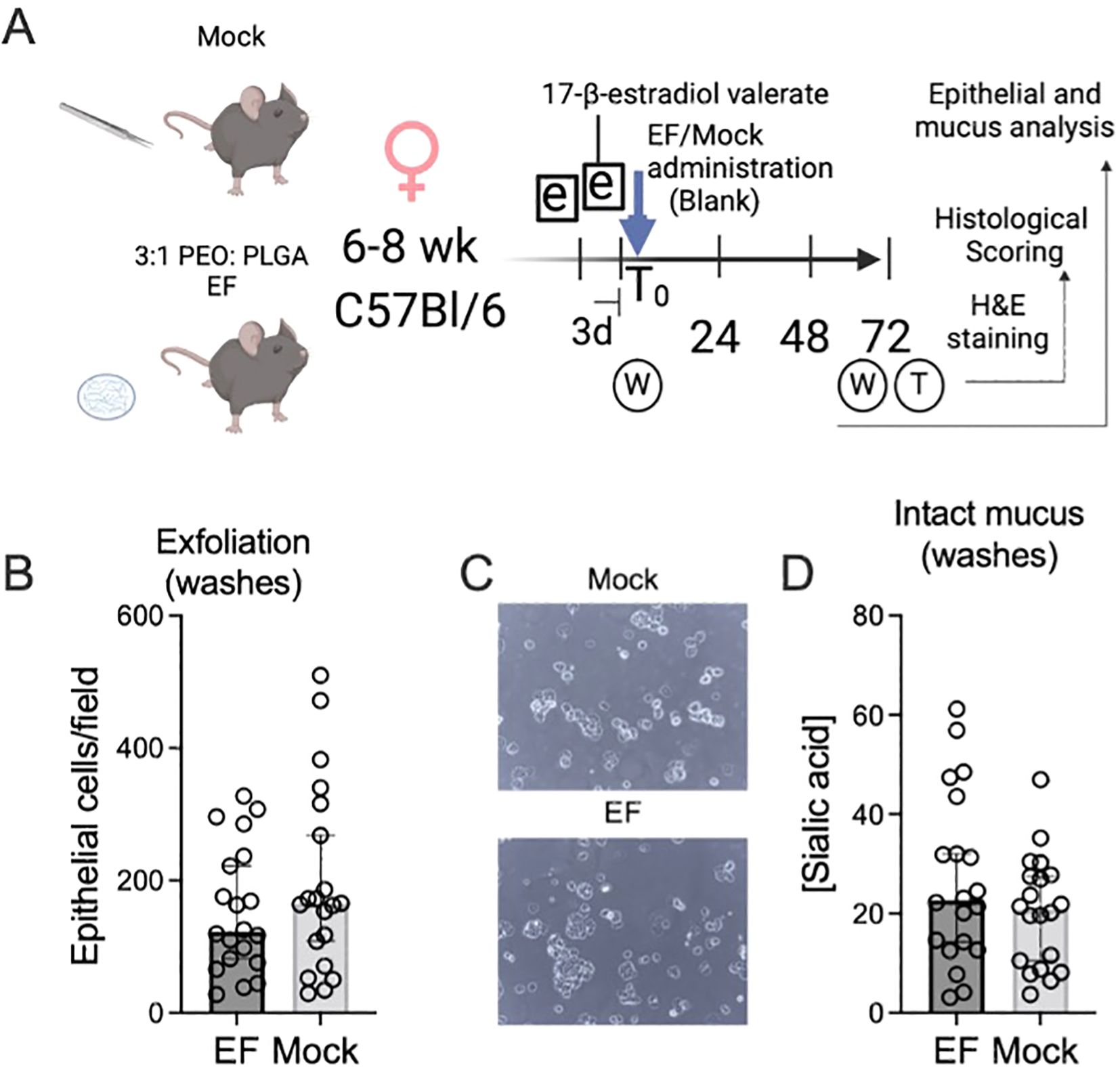
Figure 2. Epithelial exfoliation and mucus sialic acid content were indistinguishable in mice receiving vaginal electrospun fibers. (A) Experimental schematic for data in Figures 2-4. (B) Vaginal epithelial cells were enumerated in 72 h vaginal washes using phase contrast microscopy of mouse vaginal washes. An average of 5 fields was used for each data point. (C) Representative images of two mouse vaginal washes under phase contrast. (D) Sialic acid (N-acetylneuraminic acid) concentrations, a proxy for intact mucosal secretions in mouse vaginal washes, were determined by high performance liquid chromatography as described in the methods. Sialic acid concentrations are measured in picomoles per 10 µL of vaginal wash. The Mann Whitney U-test did not detect statistically significant differences in epithelial exfoliation or mucus sialic acid content between animals receiving mock-administrations versus electrospun fibers.
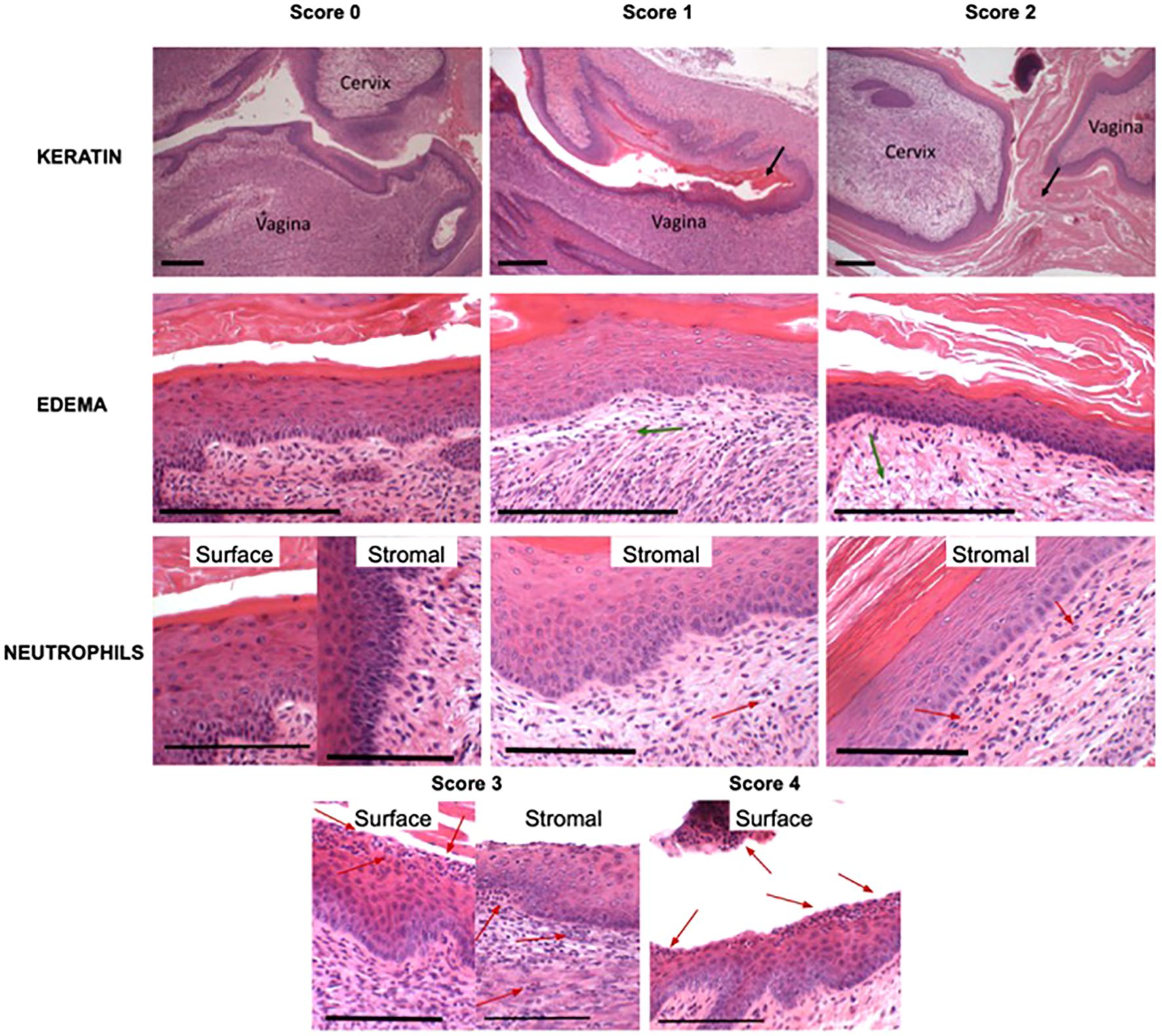
Figure 3. Scoring of histological images of the vaginal epithelium. Representative images of scores are shown for each histological metric as defined in Figure 1C. Keratin and edema scale bars represent 200 µm, while neutrophil scale bars represent 100 µm. Black arrows indicate presence of keratin, green arrows indicate presence of edema, and red arrows indicate presence of polymorphonuclear cells (PMNs).
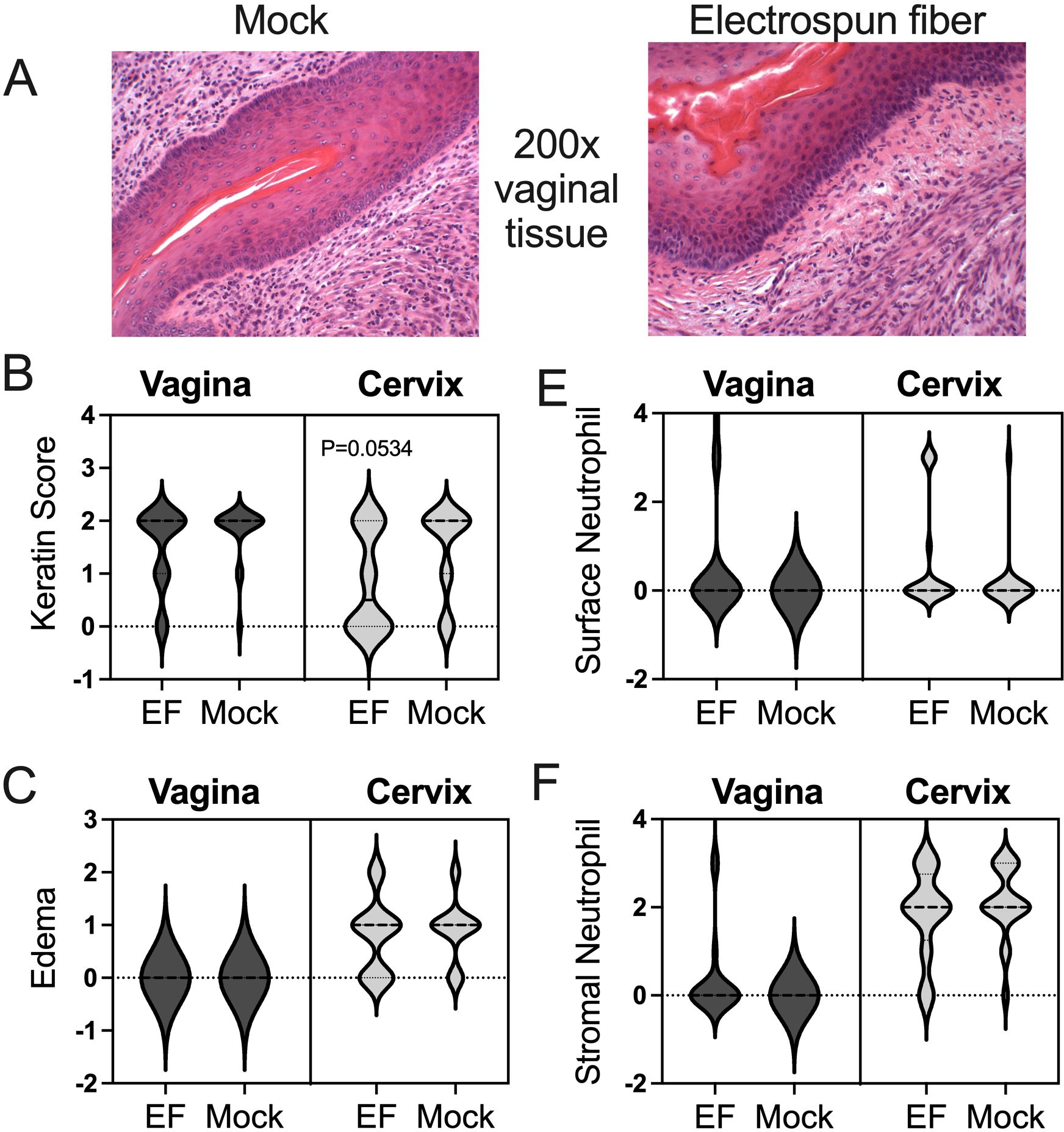
Figure 4. Vaginal and cervical histopathology in mock- and electrospun fiber-treated mice. (A) Images of vaginal tissue from mice from the mock group and electrospun fiber group. Both show similar levels of keratinization, edema, and neutrophil infiltration. (B, C, E, F) Blinded scoring of histology metrics was performed based on the rubric described in Figure 1C. The Mann Whitney U-test showedno significant differences between experimental groups for the vaginal or cervical tissues. Less keratinization of the cervix was more likely in the electrospun fiber group, but not statistically significant(p=0.0534 using nonparametric Mann-Whitney analysis). These data represent a total of n=40 mice, with n=10 per group in two independent experiments.
Administration of the blank devices did not result in significant vaginal epithelial changes (Figures 2B, C) or mucus sialic acid content (Figure 2D). Likewise, vaginal administration of blank fibers did not result in statistically significant changes in epithelial keratinization (Figure 4B), the severity of tissue edema (Figure 4A, C) or neutrophil presence at the epithelial surface or in the stroma (Figures 4D, E) in this model.
Between the mock and fiber (EF) groups, there was no significant change in epithelial keratinization in the vagina or cervix (Figures 4A, B). There was also no significant difference in inflammatory phenotypes such as edema (Figures 4A, C). H&E-stained samples were also assessed for neutrophil recruitment on the surface and in the stroma of the vagina and cervix. There was no significant difference between the number of surface neutrophils (Figures 4A, D) nor stromal neutrophils (Figures 4A, E) in mock versus fiber-administered mice.
Dissolving fibers established vaginal colonization by L. crispatus in mice
Having established that the PEO: PLGA (3:1) fiber design had little negative impact on its own in the mouse vaginal model, we set out to incorporate L. crispatus into the new design of a less hydrophilic fiber. To test if PEO-PLGA enable the successful colonization by L. crispatus, fibers containing a probiotic load of 105-106 colony forming units (CFU) of streptomycin-resistant L. crispatus strain MV-1A-US were administered to mice (total of n=20). Viable L. crispatus were recovered from all of the animals at 24 h post infection, reaching a median of approximately 105 cfu/mL. At the seven-day timepoint, 55% of the animals remained colonized (Figure 5A). To establish if the lactobacilli are metabolically active, we measured lactate in the vaginal washes. Not all vaginal washes contained detectable levels of lactate. However, there was significantly higher lactate concentrations in vaginal washes two days after fiber insertion compared to vaginal washes taken prior to fiber insertion (p=0.0145) (Figure 5B). Moreover, there were a higher number of individuals with detectable lactate at 24 h and 48 h compared to pre-washes (0 h) (Figure 5C). When the bacterial colonization began to wane by about 100-fold from initial colonization (~103cfu/mL) by 72 h, there were correspondingly lower lactate concentrations and significantly fewer animals had detectable lactate. There was a weak but positive correlation between L. crispatus titers and lactic acid concentration in the vaginal washes recovered from mice (Pearson r=0.2615; P=0.0191) (Figure 5C). In conclusion, viable and metabolically active L. crispatus released from 3:1 PEO: PLGA was able to successfully colonize the mouse vagina leading to detectable levels of lactic acid.
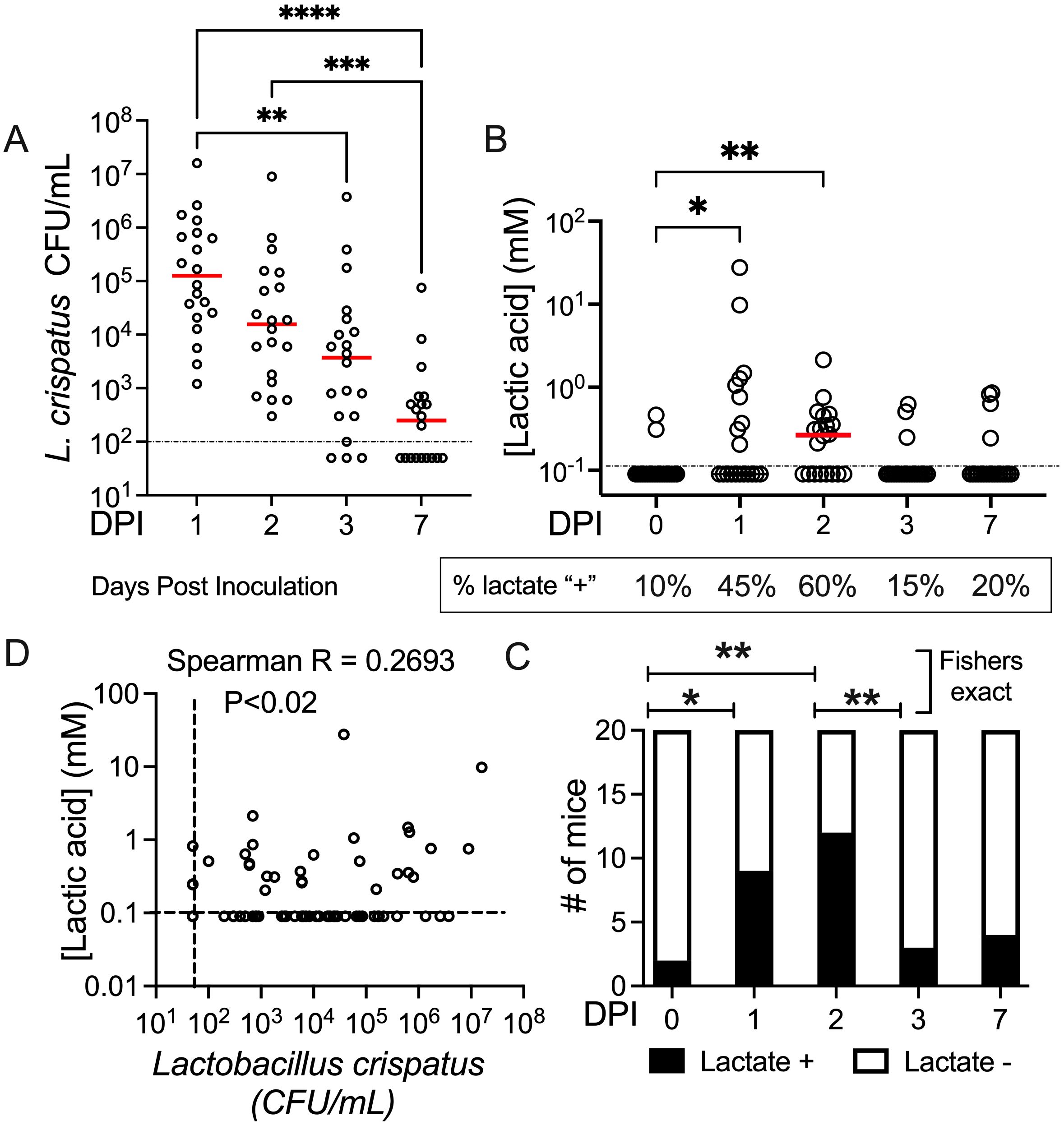
Figure 5. L. crispatus-loaded PEO: PLGA fibers initiate colonization of the mouse vagina. (A) Vaginal wash titers of streptomycin-resistant L. crispatus strain MV-1A-US over the course of 7 days from mice treated with L. crispatus-loaded PEO: PLGA fibers (105-106 CFU/fiber). Statistical analysis assumed nonparametric data and matched data (Friedman’s test) reflecting the same subjects over time with Dunns post-hoc test to enable multiple comparisons. (B) Lactic acid concentration in mouse vaginal washes before and after treatment with PEO: PLGA fibers. The Friedman test was used to compare each timepoint to a control timepoint (T0), comparing them as matched pairs from each individual animal (C) Categorical analysis comparing the proportion of animals at each time point with undetectable versus measurable levels of lactate in vaginal washes, according to data in panel (B) Results of the Fisher’s Exact test are shown. (D) Correlation between lactate concentration and Lactobacillus crispatus titers in vaginal washes for all data points collected (n=100 paired datapoints, including T0). There was a weak correlation between L. crispatus titers and lactate concentration (p=0.0103, r=.2693) using Spearman correlation coefficient. DPI, Days Post Inoculation *P<0.05 **P<0.01***P<0.001.
Discussion
New modalities for delivering active therapies to the vagina are needed. Therapeutic delivery platforms must be administered without causing injury, swelling, immune cell infiltration, infection or other adverse tissue reactions. Previously, we have shown that dissolvable electrospun fibers, formulated from PEO and PLGA, did not cause cytotoxic effects on vaginal epithelial cells in vitro (Minooei et al., 2023). In this study, we expanded testing to a mouse model. This was achieved by testing fibers in a mouse model of vaginal administration. The experimental model in (C57Bl/6J) mice is a strength as it allows the investigation of prototypes in living animals capable of mounting responses to externally administered agents. A small animal model affords a larger number of individuals that can be studied compared to larger mammals such as rabbits or sheep. Another strength of the study was a blinded design for histological scoring, based on systematic histological observations. Some previous reports have used preclinical models from rabbits to pigs to macaques to examine the impact of devices relevant to gynecological applications, employing histological changes and PMN recruitment into the analysis (Moss et al., 2014; D’Cruz and Uckun, 2007). Here we adapted methods for the mouse model, including blinded scoring for presence and severity of PMN recruitment, the amount of fluid in the tissue (edema), and the extent of keratinization of epithelial surfaces in the vagina and cervix. Using the scoring system, the experiments show that the administration of blank 3:1 PEO: PLGA woven fibers did not cause evident damage or increased edema in the vaginal or cervical tissues. Likewise, fibers did not influence epithelial shedding evident in vaginal washes, mucus production measured in the form of sialic acid content, or neutrophil recruitment in this model. Together the data indicate that electrospun fibers composed of 3:1 PEO and PLGA are retained and appear to be well-tolerated in the mouse vaginal model.
Microbes and the content of the microbiota can be consequential for mouse models of infection and colonization (Mejia et al., 2023; Mercado-Evans et al., 2024; Patras et al., 2015; Vrbanac et al., 2018; Gilbert et al., 2022; Lewis and Gilbert, 2020; Morrill et al., 2020; Agarwal et al., 2020; Gilbert et al., 2021; Gilbert and Lewis, 2019; Gilbert et al., 2017) However, robust germ-free/gnotobiotic mouse models that represent the human vaginal microbiome research are still in their infancy (Mejia et al., 2023). The model described here used commercially raised mice (Jackson Labs) and conventional housing conditions as detailed in the methods.
Most of the phenotypes that we investigated were unaffected by the administration of blank electrospun fibers. In the cervix, fiber administration may lead to a reduction in cervical keratinization (P=0.0534). In this model, β-estradiol-17-valerate treatment leads to a proestrus-like state in which a keratinized stratified squamous epithelium predominates. It has been observed that keratinized mucosal epithelial cells can become less keratinized and more cornified during hormonal or mechanical stimulation (Bragulla and Homberger, 2009). A closer look at the two independent experiments revealed that two cages of mice in the first experiment had more animals with low cervical keratinization than the second set of cages in the second experiment. This could be an effect of the cervix responding to a mechanical stimulus caused by our (initially) less experienced insertion practices. Future studies should keep this in mind.
One potential limitation to the β-estradiol-17-valerate treated mouse model is that it may impact the nature of proinflammatory responses. Prior studies show that conditional ablation of estrogen receptor alpha in vaginal epithelial cells led to higher levels of the neutrophil chemoattractant, IL-8, and unregulated immune cell infiltration into vaginal tissues (at all stages of the estrus cycle), supporting a role for epithelial estrogen responsiveness in regulating access of immune cells to the vaginal lumen in cycling mice (Collins et al., 2022; Li et al., 2018). Estrogenized mice have been used to model vaginal colonization using bacterial and fungal isolates from humans, starting with Neisseria gonorrhoeae (Jerse, 1999) and more recently including multiple types of Streptococcus, Gardnerella, Prevotella, Fusobacterium, and Candida (Yano and Fidel, 2011; Watson et al., 2013; Patras and Doran, 2016; Morrill et al., 2022). It has been postulated that the success of bacterial colonization in some of these mouse models is owed to the limitation of PMN influx that normally occurs in mice during diestrus. However, estrogen does not render mice incapable of mounting PMN recruitment responses to pathogens. For example, estrogenized mice infected with N. gonorrhoeae developed significant PMN responses (Soler-García and Jerse, 2007), similar to the infection in humans (Rest and Shafer, 1989). Streptococcus pyogenes, a common cause of vulvovaginitis in prepubescent girls (Jones, 1996), was also shown to provoke dose-dependent leukocyte responses evident in vaginal fluids (Watson et al., 2013). In contrast, vaginal administration with Gardnerella and/or Prevotella in estrogenized mice did not provoke the accumulation of neutrophils, natural killer cells, or macrophages in vaginal tissues compared to uninfected mice (Gilbert et al., 2019). This is consistent with the abundance of Gardnerella and Prevotella in BV, a condition characterized by a lack of PMN recruitment or other hallmarks of gross inflammation (Roselletti et al., 2020). Together the data suggest that estrogenized mouse models can yield PMN responses that are context-specific and may reflect host-microbe relationships in the human vagina. Nevertheless, we acknowledge that electrospun fibers could have different effects in mice treated with medroxyprogesterone acetate or at other stages of the mouse estrus cycle.
In BV, the epithelium is believed to host an unhealthy bacterial biofilm. One of the clinical diagnostic features (Amsel criteria) is the presence of a high number (>20%) of exfoliated epithelial cells with damaged-looking surfaces in wet mount (Amsel et al., 1983). Previously, we found that vaginal inoculation of estrogenized mice with Gardnerella led to higher numbers of exfoliated epithelial cells in mouse vaginal washes and other qualitative features of BV, such as the presence of clue-like cells and the appearance of Gardnerella adhering to epithelium (Gilbert et al., 2013, 2019). In mice that received bladder inoculations of Gardnerella, we previously observed that damage to the epithelium was accompanied by cleavage of caspase-3, the so-called executioner caspase that controls apoptosis (Gilbert et al., 2017; Gilbert and Lewis, 2019; O’Brien et al., 2020). Studies comparing human vaginal specimens suggest that outermost vaginal epithelial cells in BV are likewise undergoing caspase-3-dependent apoptosis, a noninflammatory form of cell death (Roselletti et al., 2020). In the current study, vaginal application of electrospun-fibers did not lead to higher numbers of exfoliated epithelial cells compared to mock-treated mice. These data suggest that 3:1 PEO: PLGA electrospun fibers will have a low risk of further contributing to the epithelial exfoliation responses already seen in the setting of BV.
One of the major objectives of this study was to develop an inert probiotic vaginal delivery platform incorporating PLGA to enable probiotic colonization of the vagina. Here, we show that mouse vaginal colonization is possible with a human isolate of L. crispatus. Despite only being able to detect lactic acid levels in 60% of the mice, there was a significant increase in lactic acid production 48 h post inoculation. While the increase upon inoculation and correlation between L. crispatus titers and lactate levels is consistent with a Lactobacillus source, other sources are possible.
Other investigators used a different mouse model to examine the relationship of L. crispatus colonization in the mouse, ultimately concluding that they could not distinguish differences between groups in lactate levels. However, the control group did not receive Lactobacillus or estradiol treatment, and there was no prewash timepoint to compare to for each individual (Muench et al., 2009). Thus, compared to the prior data, the current experiments may be better controlled and better powered to detect differences. Unfortunately, lactate did not achieve concentrations relevant to human vaginal specimens or L. crispatus cultured in vitro. As the number of animals colonized by L. crispatus decreased over time, so did the lactate concentrations. By 72 hrs the lactate levels were below our detection threshold. Future experiments will need to optimize conditions to achieve better L. crispatus colonization in mice. We expect that higher lactic acid concentrations may be required to achieve antimicrobial properties. Ingredients that promotes the growth of beneficial bacteria (prebiotics) or non-living byproducts of probiotic bacteria (postbiotics), or combinations of different species of lactobacilli may be worthwhile areas of investigation. The experiments show, in principle, that electrospun fibers with L. crispatus can achieve colonization of the mouse vagina with detectable lactate levels in most mice by 24-48 h.
Many additional questions can be asked based on the model developed here. For example, future studies could examine changes in the endogenous mouse vaginal microbiome as a result of administering the L. crispatus-loaded electrospun fibers. Whether L. crispatus administration enables knock-down of potential pathogens or a BV-like consortia introduced into the mouse vagina is another area of research worth pursuing.
Data availability statement
The raw data supporting the conclusions of this article will be made available by the authors, without undue reservation.
Ethics statement
The animal study was approved by IACUC-University of California San Diego and Washington University St. Louis. The study was conducted in accordance with the local legislation and institutional requirements.
Author contributions
CA: Data curation, Formal Analysis, Investigation, Methodology, Visualization, Writing – original draft, Writing – review & editing. SS: Investigation, Writing – review & editing. MA: Investigation, Writing – review & editing. MM: Investigation, Methodology, Writing – review & editing. NV: Data curation, Methodology, Visualization, Writing – review & editing. NG: Methodology, Supervision, Writing – review & editing. HF: Funding acquisition, Methodology, Project administration, Resources, Supervision, Writing – review & editing. WL: Funding acquisition, Methodology, Project administration, Resources, Writing – review & editing. AL: Conceptualization, Data curation, Formal Analysis, Funding acquisition, Methodology, Project administration, Resources, Supervision, Visualization, Writing – original draft, Writing – review & editing.
Funding
The author(s) declare that financial support was received for the research and/or publication of this article. This work was partially supported by the National Institutes of Health/National Institute of Allergy and Infectious Diseases grant R01AI139671 (A Lewis & H. Frieboes).
Acknowledgments
The authors gratefully acknowledge the technical efforts of Lynne Foster and Andrea Verhagen. All schematics were generated using BioRender.com.
Conflict of interest
The authors declare that the research was conducted in the absence of any commercial or financial relationships that could be construed as a potential conflict of interest.
Generative AI statement
The author(s) declare that no Generative AI was used in the creation of this manuscript.
Publisher’s note
All claims expressed in this article are solely those of the authors and do not necessarily represent those of their affiliated organizations, or those of the publisher, the editors and the reviewers. Any product that may be evaluated in this article, or claim that may be made by its manufacturer, is not guaranteed or endorsed by the publisher.
References
Agarwal K., Robinson L. S., Aggarwal S., Foster L. R., Hernandez-Leyva A., Lin H., et al. (2020). Glycan cross-feeding supports mutualism between fusobacterium and the vaginal microbiota. PloS Biol. 18, e3000788. doi: 10.1371/journal.pbio.3000788
Amegashie C. P., Gilbert N. M., Peipert J. F., Allsworth J. E., Lewis W. G., Lewis a. A. L. (2017). Relationship between nugent score and vaginal epithelial exfoliation. Edited by caroline mitchell. PloS One 12, e01777975. doi: 10.1371/journal.pone.0177797
Amsel R., Totten P. A., Spiegel C. A., Chen K. C., Eschenbach D., Holmes K. K. (1983). Nonspecific vaginitis. Diagnostic criteria and microbial and epidemiologic associations. Am. J. Med. 74, 14–22. doi: 10.1016/0002-9343(83)91112-9
Armstrong E., Hemmerling A., Miller S., Burke K. E., Newmann S. J., Morris S. R., et al. (2022). Sustained effect of LACTIN-V (Lactobacillus crispatus CTV-05) on genital immunology following standard bacterial vaginosis treatment: results from a randomised, placebo-controlled trial. Lancet Microbe 3, e435–e442. doi: 10.1016/S2666-5247(22)00043-X
Bragulla H. H., Homberger D. G. (2009). Structure and functions of keratin proteins in simple, stratified, keratinized and cornified epithelia. J. Anat. 214, 516–595. doi: 10.1111/j.1469-7580.2009.01066.x
Brotman R. M., Klebanoff M. A., Nansel T. R., Yu K. F., Andrews W. W., Zhang J., et al. (2010). Bacterial vaginosis assessed by gram stain and diminished colonization resistance to incident gonococcal, chlamydial, and trichomonal genital infection. J. Infect. Dis. 202, 1907–1155. doi: 10.1086/657320
Brusselaers N., Shrestha S., Wijgert J. V. D., Verstraelen H. (2019). Vaginal dysbiosis and the risk of human papillomavirus and cervical cancer: systematic review and meta-analysis. Am. J. Obstetrics Gynecology 221, 9–185. doi: 10.1016/j.ajog.2018.12.011
Bull M., Plummer S., Marchesi J., Mahenthiralingam E. (2013). The life history of lactobacillus acidophilus as a probiotic: A tale of revisionary taxonomy, misidentification and commercial success. FEMS Microbiol. Lett. 349, 77–875. doi: 10.1111/1574-6968.12293
Cohen C. R., Wierzbicki M. R., French A. L., Morris S., Newmann S., Reno H., et al. (2020). Randomized trial of lactin-V to prevent recurrence of bacterial vaginosis. New Engl. J. Med. 382, 1906–1915. doi: 10.1056/NEJMoa1915254
Collins M. K., McCutcheon C. R., Petroff M. G. (2022). Impact of estrogen and progesterone on immune cells and host-pathogen interactions in the lower female reproductive tract. J. Immunol. (Baltimore Md.: 1950) 209, 1437–1495. doi: 10.4049/jimmunol.2200454
D’Cruz O. J., Uckun F. M. (2007). Preclinical evaluation of a dual-acting microbicidal prodrug WHI-07 in combination with vanadocene dithiocarbamate in the female reproductive tract of rabbit, pig, and cat. Toxicologic Pathol. 35, 910–275. doi: 10.1080/01926230701748115
Di Paola M., Sani C., Clemente A. M., Iossa A., Perissi E., Castronovo G., et al. (2017). Characterization of cervico-vaginal microbiota in women developing persistent high-risk human papillomavirus infection. Sci. Rep. 7, 10200. doi: 10.1038/s41598-017-09842-6
Drago L., Vecchi E. D., Nicola L., Zucchetti E., Gismondo M. R., Vicariotto F. (2007). Activity of a lactobacillus acidophilus –based douche for the treatment of bacterial vaginosis. J. Altern. Complementary Med. 13, 435–385. doi: 10.1089/acm.2006.6040
Gilbert N. M., Ackerman A.L., Lewis A. L. (2022). Editorial: the urogenital microbiota in urinary tract diseases. Front. Cell. Infection Microbiol. 12. doi: 10.3389/fcimb.2022.1096168
Gilbert N. M., Foster L. R., Cao B., Yin Y., Mysorekar I. U., Lewis A. L. (2021). Gardnerella vaginalis promotes group B streptococcus vaginal colonization, enabling ascending uteroplacental infection in pregnant mice. Am. J. Obstetrics Gynecology 224, 5305.e1–530.e17. doi: 10.1016/j.ajog.2020.11.032
Gilbert N. M., Lewis A. L. (2019). Covert pathogenesis: transient exposures to microbes as triggers of disease. PloS Pathog. 15, e10075865. doi: 10.1371/journal.ppat.1007586
Gilbert N. M., Lewis W. G., Lewis A. L. (2013). Clinical features of bacterial vaginosis in a murine model of vaginal infection with gardnerella vaginalis. PloS One 8, e59539. doi: 10.1371/journal.pone.0059539
Gilbert N. M., Lewis W. G., Li G., Sojka D. K., Lubin J. B., Lewis A. L. (2019). Gardnerella vaginalis and Prevotella bivia Trigger Distinct and Overlapping Phenotypes in a Mouse Model of Bacterial Vaginosis. J. Infect. Dis. 220, 1099–11085. doi: 10.1093/infdis/jiy704
Gilbert N. M., O’Brien V. P., Lewis A. L. (2017). Transient microbiota exposures activate dormant escherichia coli infection in the bladder and drive severe outcomes of recurrent disease. PloS Pathog. 13, e10062385. doi: 10.1371/journal.ppat.1006238
Herold S. E., Kyser A. J., Orr M. G., Mahmoud M. Y., Lewis W. G., Lewis A. L., et al. (2023). Release kinetics of metronidazole from 3D printed silicone scaffolds for sustained application to the female reproductive tract. Biomed. Eng. Adv. 5, 100078. doi: 10.1016/j.bea.2023.100078
Jang S.-E., Jeong J.-J., Choi S.-Y., Kim H., Han M. J., Kim D.-H. (2017). Lactobacillus rhamnosus HN001 and Lactobacillus acidophilus La-14 Attenuate Gardnerella vaginalis-Infected Bacterial Vaginosis in Mice. Nutrients 9, 5315. doi: 10.3390/nu9060531
Jerse A. E. (1999). Experimental gonococcal genital tract infection and opacity protein expression in estradiol-treated mice. Infection Immun. 67, 5699–5708. doi: 10.1128/IAI.67.11.5699-5708.1999
Jones R. (1996). Childhood vulvovaginitis and vaginal discharge in general practice. Family Pract. 13, 369–372. doi: 10.1093/fampra/13.4.369
Kyser A. J., Mahmoud M. Y., Fotouh B., Patel R., Armstrong C., Aagard M., et al. (2024). Sustained dual delivery of metronidazole and viable lactobacillus crispatus from 3D-printed silicone shells. Biomaterials Adv. 165, 214005. doi: 10.1016/j.bioadv.2024.214005
Kyser A. J., Mahmoud M. Y., Herold S. E., Lewis W. G., Lewis A. L., Steinbach-Rankins J. M., et al. (2023a). Formulation and characterization of pressure-assisted microsyringe 3D-printed scaffolds for controlled intravaginal antibiotic release. Int. J. Pharmaceutics 641, 123054. doi: 10.1016/j.ijpharm.2023.123054
Kyser A. J., Masigol M., Mahmoud M. Y., Ryan M., Lewis W. G., Lewis A. L., et al. (2023b). Fabrication and characterization of bioprints with lactobacillus crispatus for vaginal application. J. Controlled Release: Off. J. Controlled Release Soc. 357, 545–560. doi: 10.1016/j.jconrel.2023.04.023
Łaniewski P., Barnes D., Goulder A., Cui H., Roe D. J., Chase D. M., et al. (2018). Linking cervicovaginal immune signatures, HPV and microbiota composition in cervical carcinogenesis in non-hispanic and hispanic women. Sci. Rep. 8, 75935. doi: 10.1038/s41598-018-25879-7
Lewis A. L., Gilbert N. M. (2020). Roles of the vagina and the vaginal microbiota in urinary tract infection: evidence from clinical correlations and experimental models. GMS Infect. Dis. 8, Doc02. doi: 10.3205/id000046
Li S., Herrera G. G., Tam K. K., Lizarraga J. S., Beedle M.-T., Winuthayanon W. (2018). Estrogen action in the epithelial cells of the mouse vagina regulates neutrophil infiltration and vaginal tissue integrity. Sci. Rep. 8, 112475. doi: 10.1038/s41598-018-29423-5
Lin T.-C., Hsu I.-L., Tsai W.-H., Chu Y.-C., Kuan L.-C., Huang M.-S., et al. (2021). Improvement of bacterial vaginosis by oral lactobacillus supplement: A randomized, double-blinded trial. Appl. Sci. 11, 9025. doi: 10.3390/app11030902
Mahmoud M. Y., Wesley M., Kyser A., Lewis W. G., Lewis A. L., Steinbach-Rankins J. M., et al. (2023). Lactobacillus crispatus-Loaded electrospun fibers yield viable and metabolically active bacteria that kill gardnerella in vitro. Eur. J. Pharmaceutics Biopharmaceutics: Off. J. Arbeitsgemeinschaft Fur Pharmazeutische Verfahrenstechnik e. V 187, 68–75. doi: 10.1016/j.ejpb.2023.04.011
Mastromarino P., Macchia S., Meggiorini L., Trinchieri V., Mosca L., Perluigi M., et al. (2009). Effectiveness of lactobacillus-containing vaginal tablets in the treatment of symptomatic bacterial vaginosis. Clin. Microbiol. Infection: Off. Publ. Eur. Soc. Clin. Microbiol. Infect. Dis. 15, 67–74. doi: 10.1111/j.1469-0691.2008.02112.x
Mastromarino P., Vitali B., Mosca L. (2013). Bacterial vaginosis: A review on clinical trials with probiotics. New Microbiologica 36, 229–238.
Mayer B. T., Srinivasan S., Fiedler T. L., Marrazzo J. M., Fredricks D. N., Schiffer J. T. (2015). Rapid and profound shifts in the vaginal microbiota following antibiotic treatment for bacterial vaginosis. J. Infect. Dis. 212, 793–8025. doi: 10.1093/infdis/jiv079
Mejia M. E., Mercado-Evans V., Zulk J. J., Ottinger S., Ruiz K., Ballard M. B., et al. (2023). Vaginal microbial dynamics and pathogen colonization in a humanized microbiota mouse model. NPJ Biofilms Microbiomes 9, 875. doi: 10.1038/s41522-023-00454-9
Mercado-Evans V., Mejia M. E., Zulk J. J., Ottinger S., Hameed Z. A., Serchejian C., et al. (20241035). Gestational diabetes augments group B streptococcus infection by disrupting maternal immunity and the vaginal microbiota. Nat. Commun. 15. doi: 10.1038/s41467-024-45336-6
Minooei F., Gilbert N. M., Zhang L., NeCamp M. S., Mahmoud M. Y., Kyser A. J., et al. (2023a). Rapid-dissolving electrospun nanofibers for intra-vaginal antibiotic or probiotic delivery. Eur. J. Pharmaceutics Biopharmaceutics: Off. J. Arbeitsgemeinschaft Fur Pharmazeutische Verfahrenstechnik e.V 190 , 81–93. doi: 10.1016/j.ejpb.2023.07.009
Minooei F., Kanukunta A. R., Mahmoud M. Y., Gilbert N. M., Lewis W. G., Lewis A. L., et al. (2023b). Mesh and Layered Electrospun Fiber Architectures as Vehicles for Lactobacillus acidophilus and Lactobacillus crispatus Intended for Vaginal Delivery. Biomaterials Adv. 154, 213614. doi: 10.1016/j.bioadv.2023.213614
Morrill S. R., Agarwal K., Saha S., Lewis W. G., Gilbert N. M., Lewis A. L. (2022). Models of murine vaginal colonization by anaerobically grown bacteria. J. Visualized Experiments: JoVE 183. doi: 10.3791/64032
Morrill S., Gilbert N. M., Lewis A. L. (2020). Gardnerella vaginalis as a cause of bacterial vaginosis: appraisal of the evidence from in vivo models. Front. Cell. Infection Microbiol. 10. doi: 10.3389/fcimb.2020.00168
Moss J. A., Srinivasan P., Thomas J., Butkyavichene I., Lopez G., et al. (2014). Pharmacokinetics and preliminary safety study of pod-intravaginal rings delivering antiretroviral combinations for HIV prophylaxis in a macaque model. Antimicrobial Agents Chemotherapy 58, 5125–5355. doi: 10.1128/AAC.02871-14
Muench D. F., Kuch D. J., Wu H., Begum A. A., Veit S. J., Pelletier M.-E., et al. (2009). Hydrogen Peroxide-Producing Lactobacilli Inhibit Gonococci in Vitro but Not during Experimental Genital Tract Infection. J. Infect. Dis. 199, 1369–1785. doi: 10.1086/597390
Ness R. B., Kip K. E., Hillier S. L., Soper D. E., Stamm C. A., Sweet R. L., et al. (2005). A cluster analysis of bacterial vaginosis–associated microflora and pelvic inflammatory disease. Am. J. Epidemiol. 162, 585–905. doi: 10.1093/aje/kwi243
O’Brien V. P., Joens M. S., Lewis A. L., Gilbert. N. M. (2020). Recurrent Escherichia coli Urinary Tract Infection Triggered by Gardnerella vaginalis Bladder Exposure in Mice. J. Visualized Experiments: JoVE 166. doi: 10.3791/61967
Patras K. A., Doran K. S. (2016). A murine model of group B streptococcus vaginal colonization. J. Visualized Experiments: JoVE 117, 54708. doi: 10.3791/54708
Patras K. A., Wescombe P. A., Rösler B., Hale J. D., Tagg J. R., Doran K. S. (2015). Streptococcus salivarius K12 limits group B streptococcus vaginal colonization. Infection Immun. 83, 3438–3445. doi: 10.1128/IAI.00409-15
Pendharkar S., Brandsborg E., Hammarström L., Marcotte H., Larsson P. (2015). Vaginal colonisation by probiotic lactobacilli and clinical outcome in women conventionally treated for bacterial vaginosis and yeast infection. BMC Infect. Dis. 15, 2555. doi: 10.1186/s12879-015-0971-3
Qi F., Fan S., Fang C., Ge L., Lyu J., Huang Z., et al. (2023). Orally Administrated Lactobacillus gasseri TM13 and Lactobacillus crispatus LG55 Can Restore the Vaginal Health of Patients Recovering from Bacterial Vaginosis. Front. Immunol. 14. doi: 10.3389/fimmu.2023.1125239
Ralph S. G., Rutherford A. J., Wilson J. D. (1999). Influence of bacterial vaginosis on conception and miscarriage in the first trimester: cohort study. BMJ 319, 220–223. doi: 10.1136/bmj.319.7204.220
Rest R. F., Shafer W. M. (1989). Interactions of neisseria gonorrhoeae with human neutrophils. Clin. Microbiol. Rev. 2 Suppl, S83–S91. doi: 10.1128/CMR.2.Suppl.S83
Roselletti E., Sabbatini S., Perito S., Mencacci A., Vecchiarelli A., Monari C. (2020). Apoptosis of vaginal epithelial cells in clinical samples from women with diagnosed bacterial vaginosis. Sci. Rep. 10, 19785. doi: 10.1038/s41598-020-58862-2
Soler-García A. A., Jerse A. E. (2007). Neisseria gonorrhoeae Catalase Is Not Required for Experimental Genital Tract Infection despite the Induction of a Localized Neutrophil Response. Infection Immun. 75, 2225–2335. doi: 10.1128/IAI.01513-06
Spandorfer S. D., Neuer A., Giraldo P. C., Rosenwaks Z., Witkin S. S. (2001). Relationship of abnormal vaginal flora, proinflammatory cytokines and idiopathic infertility in women undergoing IVF. J. Reprod. Med. 46, 806–810.
Stapleton A. E., Au-Yeung M., Hooton T. M., Fredricks D. N., Roberts P. L., Czaja C. A., et al. (2011). Randomized, Placebo-Controlled Phase 2 Trial of a Lactobacillus crispatus Probiotic given Intravaginally for Prevention of Recurrent Urinary Tract Infection. Clin. Infect. Diseases: Off. Publ. Infect. Dis. Soc. America 52, 1212–1175. doi: 10.1093/cid/cir183
Svare J., Schmidt H., Hansen Bb, Lose G. (2006). Bacterial vaginosis in a cohort of danish pregnant women: prevalence and relationship with preterm delivery, low birthweight and perinatal infections. BJOG: Int. J. Obstetrics Gynaecology 113, 1419–1255. doi: 10.1111/j.1471-0528.2006.01087.x
Tomás M., Palmeira-de-Oliveira A., Simões S., Martinez-de-Oliveira J., Palmeira-de-Oliveira R. (2020). Bacterial vaginosis: standard treatments and alternative strategies. Int. J. Pharmaceutics 587, 119659. doi: 10.1016/j.ijpharm.2020.119659
Vivekanandan V., Khan Z. H., Venugopal G., Musunuru B., Mishra P., Srivastava S., et al. (2024). VagiBIOM lactobacillus suppository improves vaginal health index in perimenopausal women with bacterial vaginosis: A randomized control trial. Sci. Rep. 14, 33175. doi: 10.1038/s41598-024-53770-1
Vrbanac A., Riestra A. M., Coady A., Knight R., Nizet V., Patras K. A. (2018). The murine vaginal microbiota and its perturbation by the human pathogen group B streptococcus. BMC Microbiol. 18, 1975. doi: 10.1186/s12866-018-1341-2
Watson M. E., Nielsen H. V., Hultgren S. J., Caparon M. G. (2013). Murine vaginal colonization model for investigating asymptomatic mucosal carriage of. Streptococcus pyogenes. Infection Immun. 81, 1606–1175. doi: 10.1128/IAI.00021-13
Wiesenfeld H. C., Hillier S. L., Krohn M. A., Landers D. V., Sweet R. L. (2003). Bacterial Vaginosis Is a Strong Predictor of Neisseria gonorrhoeae and Chlamydia trachomatis Infection. Clin. Infect. Diseases: Off. Publ. Infect. Dis. Soc. America 36, 663–685. doi: 10.1086/367658
Wijgert J. H.H.M. (2017). The vaginal microbiome and sexually transmitted infections are interlinked: consequences for treatment and prevention. PloS Med. 14, e1002478. doi: 10.1371/journal.pmed.1002478
Ya W., Reifer C., Miller L. E. (2010). Efficacy of vaginal probiotic capsules for recurrent bacterial vaginosis: A double-blind, randomized, placebo-controlled study. Am. J. Obstetrics Gynecology 203, 1205.e1–120.e6. doi: 10.1016/j.ajog.2010.05.023
Keywords: bacterial vaginosis, Lactobacillus crispatus, electrospun fibers, vaginal colonization, histopathology, lactate, vaginal microbiome, probiotics
Citation: Armstrong CN, Saha S, Aagard MA, Mahmoud MY, Varki NM, Gilbert NM, Frieboes HB, Lewis WG and Lewis AL (2025) Preclinical validation of electrospun fibers to achieve vaginal colonization by Lactobacillus crispatus. Front. Bacteriol. 4:1562077. doi: 10.3389/fbrio.2025.1562077
Received: 16 January 2025; Accepted: 25 March 2025;
Published: 16 April 2025.
Edited by:
Nadia Andrea Andreani, Max Planck Institute for Evolutionary Biology, GermanyReviewed by:
Padhmanand Sudhakar, Kumaraguru College of Technology, IndiaMeghna Basu, Max Planck Institute for Evolutionary Biology, Germany
Copyright © 2025 Armstrong, Saha, Aagard, Mahmoud, Varki, Gilbert, Frieboes, Lewis and Lewis. This is an open-access article distributed under the terms of the Creative Commons Attribution License (CC BY). The use, distribution or reproduction in other forums is permitted, provided the original author(s) and the copyright owner(s) are credited and that the original publication in this journal is cited, in accordance with accepted academic practice. No use, distribution or reproduction is permitted which does not comply with these terms.
*Correspondence: Amanda L. Lewis, YTFsZXdpc0BoZWFsdGgudWNzZC5lZHU=
†Present address: Sudeshna Saha, Department of Biological Sciences, SRM University-AP, Amaravati, Andhra Pradesh, India
Mohamed Y. Mahmoud, Northeastern University, Boston, MA, United States