- 1Department of Biology and Chemistry, College of Liberal Arts and Sciences, National University of Defense Technology, Changsha, China
- 2State Key Laboratory of Freshwater Ecology and Biotechnology, Institute of Hydrobiology, Chinese Academy of Sciences, Wuhan, China
- 3Hunan Key Laboratory of Economic Crops, Genetic Improvement, and Integrated Utilization, School of Life Sciences, Hunan University of Science and Technology, Xiangtan, China
Metabolic engineering of cyanobacteria has received much attention as a sustainable strategy to convert CO2 to various longer carbon chain fuels. Pinene has become increasingly attractive since pinene dimers contain high volumetric energy and have been proposed to act as potential aircraft fuels. However, cyanobacteria cannot directly convert geranyl pyrophosphate into pinene due to the lack of endogenous pinene synthase. Herein, we integrated the gene encoding Abies grandis pinene synthase into the model cyanobacterium Synechococcus sp. PCC 7002 through homologous recombination. The genetically modified cyanobacteria achieved a pinene titer of 1.525 ± 0.l45 mg L−1 in the lab-scale tube photobioreactor with CO2 aeration. Specifically, the results showed a mixture of α- and β-pinene (∼33:67 ratio). The ratio of β-pinene in the product was significantly increased compared with that previously reported in the engineered Escherichia coli. Furthermore, we investigated the photoautotrophic growth performances of Synechococcus overlaid with different concentrations of dodecane. The work demonstrates that the engineered Synechococcus is a suitable potential platform for β-pinene production.
Introduction
Growing concerns about environmental pollution issues and demands for alternative energy have stimulated photosynthetic organisms emerging as powerful platforms converting CO2 to various bio-based products. As one of the oldest living phyla (Schopf and Packer, 1987), cyanobacteria are oxygenic photosynthetic autotrophic bacteria widely distributed in aquatic and terrestrial habitats (Díez and Ininbergs, 2014). They play a crucial role in the biogeochemical cycles of carbon and nitrogen with the capacity of fixing carbon dioxide and atmospheric nitrogen (Parmar et al., 2011). Moreover, cyanobacteria possess the dramatic property of photosynthetically converting CO2 to various beneficial organic compounds, including drugs (Simmons et al., 2005; Teruya et al., 2009), cosmetics (Conde et al., 2000; Rajneesh et al., 2017), food additives (Ma et al., 2019), and biofuels (Sarma et al., 2016; Singh et al., 2016; Choi et al., 2020), benefiting from high growth rates, ease of genetic engineering, and large-scale cultivation (Rajneesh et al., 2017). In addition, the increasingly abounding toolboxes for genetic manipulation impel cyanobacteria to become attractive platforms for bio-based production (Markley et al., 2015; Carroll et al., 2018; Sun et al., 2018; Xia et al., 2019).
Terpenoids are a large group of high-value compounds and have been successfully produced by multiple microbial cell factories (Davies et al., 2015; Wang et al., 2018; Moser and Pichler, 2019). Cyanobacteria synthesize terpenoid precursors isopentenyl diphosphate (IPP) and dimethylallyl diphosphate (DMAPP) with the endogenous methylerythritol phosphate (MEP) pathway (Lin and Pakrasi, 2019), which has been engineered to produce various terpenoids (Pattanaik and Lindberg, 2015; Betterle and Melis, 2019). Pinene is a plant natural monoterpene (C10) and has shown a wide range of commercial applications in flavorings (Gomes-Carneiro et al., 2005), fragrances (Kirby and Keasling, 2009), and pharmaceuticals (Zhao et al., 2018). Furthermore, pinene dimers’ high energy density is comparable to tactical fuels JP-10, and this has made pinene emerge as a potential bio-jet fuel used in aircraft and aircraft-launched missiles (Harvey et al., 2010).
Microbial factories have been employed to produce pinene with various genetic tools. Currently, the highest pinene production efficiency was achieved in the engineered Escherichia coli (E. coli) and yeast, reaching respective titers of 166.5 mg L−1 (Niu et al., 2018) and 36.1 mg L−1 (Wei et al., 2021). In order to build a photosynthetic microbial platform for pinene production, several efforts have been successfully carried in the photosynthetic microorganisms, including Rhodobacter sphaeroides and Synechocystis sp. PCC 6803 (Tashiro et al., 2016; Wu et al., 2021). The highest pinene production titer was achieved at 0.540 mg L−1 in the engineered R. sphaeroides by optimizing the expression of the critical enzymes in the MEP pathway (Wu et al., 2021). In contrast, only 0.08 mg L−1 pinene was harvested with the cold trap in the transgenic Synechocystis sp. PCC 6803 integrated with the mutant pinene synthase (PS) gene (Tashiro et al., 2016). Developing a superior cyanobacterial chassis for synthetic biology and metabolic engineering applications is supposed to be an effective effort to further improve the productivities of terpenoids for commercial production.
In cyanobacteria, the condensation reaction of IPP and DMAPP catalyzed by geranyl diphosphate synthase (GPPS) produces geranyl diphosphate (GPP), the substrate of PS. In the present study, we engineered Synechococcus sp. PCC 7002 (Synechococcus) to produce pinene by introducing a recombinant plasmid containing the Abies grandis PS (AgPS) gene. The AgPS gene was integrated into the cyanobacteria genome by homologous recombination and was overexpressed under the control of the cpcBA (phycocyanin) operon promoter (PcpcBA) from Synechocystis sp. PCC 6803 (Xu et al., 2011). The successful pinene production of transgenic Synechococcus was achieved at the highest rate of 1.525 ± 0.l45 mg L−1 under the condition of CO2 aeration and continuous light. Furthermore, the ratio of two pinene isomers, α- and β-pinene, was also determined in the final product.
Materials and Methods
Strains and Construction of Plasmids
Strains and plasmids used in this study are listed in Table 1. The AgPS gene was amplified from pAgGPPS-(GSG)2-AgPS by PCR with specific primers AgPS_F: 5′-CAGCATATGCGTCGTG-GTAAATCTATCAC-3′ and AgPS_R: 5′-GCGGGATCCTTA-CAGCGGAACAGATTCCAG-3′. The PCR product was purified and ligated into the cloning vector pBZ (TransGen Biotech, China) to perform sequencing. The AgPS gene with the correct sequence was inserted into pAQ1EX-PcpcBA to generate pAQ1EX-AgPS plasmid via NdeI and BamHI (Thermo Scientific) digestion. The plasmid pAQ1EX-AgPS was aiming at integrating the functional genes into the endogenous high-copy plasmid pAQ1 through homologous recombination. A modified protocol following that described previously (Stevens and Porter, 1980; Frigaard et al., 2004) was used to transform pAQ1EX-AgPS into Synechococcus. To select the transformant strain, 100 μg ml−1 streptomycin was applied, and the successful transgene incorporation was confirmed by colony PCR and sequencing using the primers PAQIN_F: 5′-GGAATTGTGCGTGTGGTTTC-3′ and PAQIN_R: 5′-CTAACGATCAGCGCGAAAAG-3′.
Growth Experiments
Synechococcus wild-type (WT) and AgPS transformant (7002-PS) strains were grown in liquid medium A+ containing the following: 18 g L−1 NaCl, 5 g L−1 MgSO4 7H2O, 1 g L−1 NaNO3, 0.6 g L−1 KCl, 0.050 g L−1 KH2PO4, 0.270 g L−1 CaCl2, 0.020 g L−1 Na2CO3, 0.030 g L−1 Na2EDTA·2H2O, 3.890 mg L−1 FeCl3·6H2O, 1 g L−1 Tris HCl (pH 8.2), 2.860 mg L−1 H3BO3, 1.810 mg L−1 MnCl2·4H2O, 0.220 mg L−1 ZnSO4·7H2O, 0.390 mg L−1 Na2MoO4·2H2O, 0.100 mg L−1 CuSO4·5H2O, 0.050 mg L−1 Co(NO3)2·6H2O, and 4 μg L−1 vitamin B12 (Stevens and Porter, 1980; Ludwig and Bryant, 2011). The 7002-PS strain containing SmR cassette was selected on solid A+ medium, adding 1.2% (w/v) Bacto Agar (BD) and streptomycin with the required concentration. Seed cultures were performed in 50-ml Erlenmeyer flasks using an orbital shaker at 100 r min−1. The flasks contained 30 ml of liquid A+ medium in the presence of antibiotic. All Synechococcus strains were grown at 30°C under continuous illumination of 100 μmol photons m−2 s−1, and optical density (OD) was measured to monitor cell growth using a spectrophotometer (Varian) at 730 nm.
Western Blotting
Synechococcus cultures used for western blotting were inoculated into 50 ml fresh A+ medium with an initial OD730 = 0.1 and grown for 24 h under continuous aeration supplement with 1% (v/v) CO2. Cells were harvested by centrifugation and disrupted with a motor-driven tissue grinder (Sangon Biotech). Equal amounts of total proteins in crude cell lysates were subjected to western blotting analysis. AgPS was identified with anti-His mouse monoclonal antibody (TransGen Biotech, China) followed by goat anti-mouse IgG (H + L) (TransGen Biotech, China) and visualized using a DAB horseradish peroxidase color development kit (Beyotime, China).
Pulse Amplitude Modulation Fluorometry
The Water-PAM (Walz) was used to measure the variable chlorophyll fluorescence with a Water-S stirring device capable of keeping the samples homogenous and preventing sedimentation of the cells. Light-emitting diodes supplied red measuring light (spectral peak at 650 nm), actinic light, and saturation pulses (spectral peak at 660 nm). After 20 min of dark adaption, a 2-ml sample was used to analyze fluorescence parameters Fv/Fm by measuring Fo and Fm. Light treatment strategy and stirrer operation in this study were according to the previous reports (Campbell et al., 1998; Cosgrove and Borowitzka, 2006).
Pinene Production and Analysis
Synechococcus cultures used for pinene production were inoculated into 60 ml fresh A+ medium without antibiotic with an initial OD730 = 1.0. The cultures were grown for 72 h under continuous aeration supplement with 1% (v/v) CO2, and a 6-ml dodecane (Aladdin) overlay was applied at the beginning to trap the pinene excreted from the cells. Samples of 500 μl dodecane overlay were harvested at the end of the production period. The procedure of sample pretreatment was following a modified method as described previously (Sarria et al., 2014), adding (R)-(+)-limonene (Aladdin) as an internal standard. These samples were analyzed on a gas chromatography–mass spectrometer (GC-MS) (Agilent 7890A with Agilent 5975C MS detector) by a standard curve of (−)-α- and β-pinene (Aladdin). The GC-MS was equipped with a DB-5MS column (30 m × 0.25 mm × 0.25 µm) to separate hydrophobic molecules. The analysis conditions were as follows: He (1 ml min−1) as a carrier gas, split ratio of 5:1; an injector temperature of 300°C; and an oven program of 50°C for 5 min, ramp at 10°C min−1 to 150°C, ramp at 30°C min−1 to 280°C and held for 5 min.
Results and Discussion
Determination of Candidate PS Genes
Synechococcus is capable of utilizing the endogenous MEP pathway to synthesize GPP. In order to build Synechococcus a platform for pinene production, we need to integrate an exogenous pinene synthase into the genome of Synechococcus to convert GPP into α- or β-pinene with high fidelity. Although both pinene isomers can be found in turpentine (Behr and Johnen, 2009), the β-pinene isomer is considered preferable for higher economic value (Sarria et al., 2014) and dimerization efficiency (Walls and Rios-Solis, 2020). Thus, the AgPS gene was selected to produce pinene with more proportion of β-pinene (Bohlmann et al., 1997) and had shown the highest activity for pinene synthesis in E. coli among the high-fidelity pinene synthases (Sarria et al., 2014).
Construction of Synechococcus Strain Integrated With AgPS Transgene
The nucleotide sequence of the AgPS gene from Sarria et al. (2014) had been modified and expressed according to the codon usage preference of E. coli. A comparison of the modified AgPS gene sequence with the codon usage of Synechococcus was predicted with the Graphical Codon Usage Analyser (Fuhrmann et al., 2004), and the results showed that the relative adaptiveness values are all higher than 30% (Supplementary Material S1). We therefore directly amplified the modified AgPS gene from plasmid pAgGPPS-(GSG)2-AgPS and therewith integrated it into the pAQ1EX vector between the NdeI and BamHI sites. The insertion of AgPS gene into the neutral region of pAQ1 between two open-reading frames encoding hypothetical proteins (Figure 1A) was confirmed by colony PCR and sequencing in numerous transformants of the 7002-PS strain (Figure 1B). Interestingly, although the 7002-PS strain was isolated using the streak plate method three times, as the colony PCR results showed, the neutral region of pAQ1 between two open-reading frames had still not been entirely replaced by the integrated sequence. We supposed that the region replaced by the transgenes in pAQ1EX-PS was essential for the growth of Synechococcus (Xu et al., 2011), and the complete integration of the exogenous genes in pAQ1EX was hard to realize. The potential different amount of the transgenic pAQ1 might eventually lead to the differences in pinene production performance among the independent transformants of the 7002-PS strain. The expression of AgPS was confirmed by western blotting (Figure 1C).
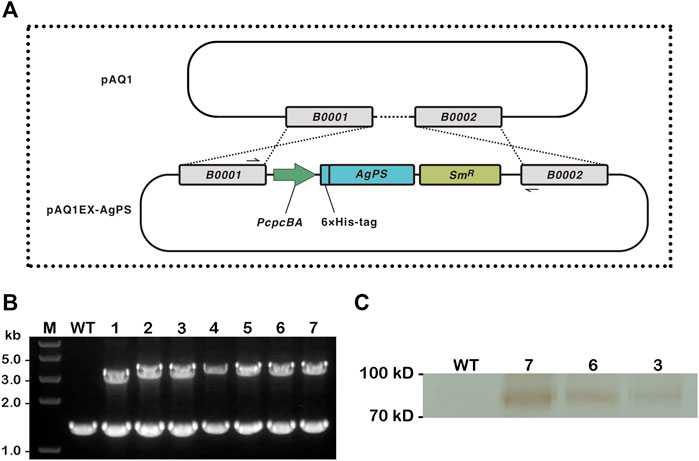
FIGURE 1. Construction of Synechococcus sp. PCC 7002 overexpressing Abies grandis pinene synthase. (A) Illustrations of the integration of endogenous plasmid pAQ1 in wild-type Synechococcus with the inserted A. grandis pinene synthase (AgPS) cassette in the recombinant plasmid pAQ1EX-AgPS. The AgPS cassette contained PcpcBA to drive transgene expression, and a spectinomycin resistance (SmR) selectable marker. The specific primers PAQIN_F and PAQIN_R shown in black solid half arrows are used for demonstrating the successful integration of AgPS cassette into pAQ1 (between open-reading frames B0001 and B0002) via double homologous recombination. (B) Confirmation of transgene integration by colony PCR using the above-mentioned primers. The wild-type (WT) strain and seven independent transformants of the 7002-PS strain (1–7) were verified. Lane M: Trans2K® Plus II DNA Marker (TransGen Biotech, China). Each number above the image of the agarose gel correlated with PCR product. (C) Western blotting analysis of the total protein extract isolated from WT and three independent transformants of the 7002-PS strain (3, 6, and 7). Sample from the WT strain was used as a control. Proteins were detected using anti-His mouse monoclonal antibody (TransGen Biotech, China) followed by the incubation with anti-mouse secondary antibody conjugated with HRP. Reactions were visualized using a DAB horseradish peroxidase color development kit (Beyotime, China).
The Effect of Dodecane on the Photoautotrophic Growth of Synechococcus
Dodecane overlay has been turned out as an effective method for terpenes harvesting (Davies et al., 2014; Sarria et al., 2014; Niu et al., 2018; Dienst et al., 2020). This article utilized 1% (v/v) CO2 bubbles to supply inorganic carbon for pinene production in the 7002-PS strain. Photoautotrophic cultivation of cyanobacteria with sodium bicarbonate as an inorganic carbon source and using orbital shakers or magnetic stirrers seem adequate for terpenes production (Silva et al., 2016). However, CO2 used as the inorganic carbon source has environmentally friendly advantages in integrating atmospheric CO2 into biomass. Although filling the upper space of the medium with CO2 could maintain continuous production of terpene (Davies et al., 2014), we need to develop deeper insight into the production performance of engineered strains grown with CO2 bubble aeration.
To investigate whether dodecane overlay limits the photoautotrophic growth of cyanobacteria ventilated with CO2, we inspected the photoautotrophic growth of the WT strain overlaid with different dodecane concentrations over a 72-h time course at various aeration rates. Cell growth rates were measured by OD730, and the photosynthetic performance was evaluated by the chlorophyll fluorescence parameter Fv/Fm. The chlorophyll fluorescence parameter Fv/Fm and OD730 of the WT cells were compared when growing with 0%, 5%, 10%, and 15% (v/v) dodecane overlay. As the organic phase could not maintain stability with an aeration rate higher than 0.12 vvm (volume of gas per volume of liquid per minute) in this work, the aeration rates were initially set to 0.02, 0.03, and 0.12 vvm. Apparently, the limited CO2 provision caused by the lower aeration rates (0.02 and 0.03 vvm) had an adverse effect on the photoautotrophic growth of Synechococcus. Meanwhile, the OD730 of the WT cells cultivated with aeration rate at 0.12 vvm exhibited obvious variation (Figure 2A). The chlorophyll fluorescence parameter Fv/Fm of the cells without dodecane overlay was higher than the that of the cells overlaid with dodecane after 2 days, and the cells aerating at 0.12 vvm presented the greatest gap, which was supposed to aggravate the difference of OD730 (Figure 2B). However, neither the Fv/Fm nor OD730 of the WT cells showed apparent difference whenever the aeration rate was 0.02 or 0.03 vvm. The results indicated that the dodecane overlay led to a slight restriction to the photosynthetic growth of Synechococcus cultivated with CO2 bubble aeration at a high rate (0.12 vvm), and the three concentrations (5%, 10%, and 15%) of the dodecane overlay had no significant difference in the limitation effect. However, the dramatic variance of Fv/Fm between the WT cells overlaid with and without dodecane demonstrated that cultivation with dodecane overlay at a high aeration rate enables to impair the photosystem II efficiency of Synechococcus, which suggests that dodecane overlay is not the best choice for long-term product harvest in the condition of high gas aeration rate for Synechococcus.
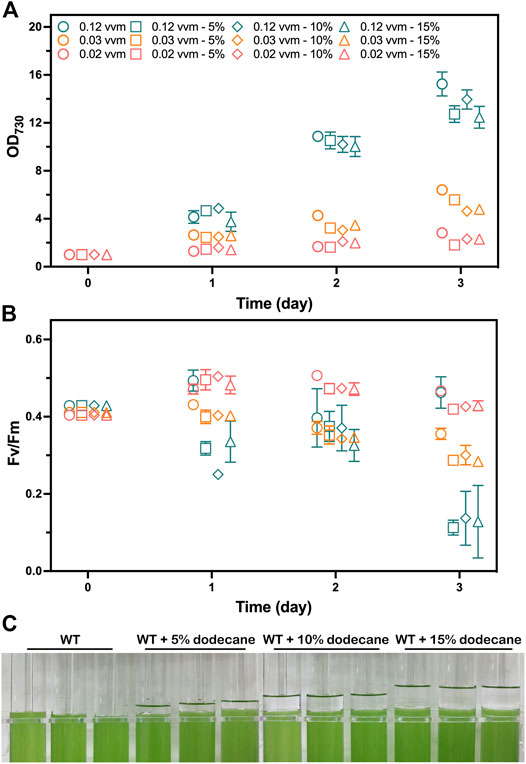
FIGURE 2. Photoautotrophic performance analysis of the WT strain grown with 1% (v/v) CO2 aeration at 0.12, 0.03, and 0.02 vvm for 3 days. The mediums were overlaid with 0%, 5%, 10%, and 15% (v/v) of dodecane, respectively. Samples were collected for the analysis at 1-day intervals. (A) The photoautotrophic growth parameters monitored by OD730. (B) Photosynthetic efficiency evaluated by chlorophyll fluorescence parameter Fv/Fm. The works on the WT strain grown with 0%, 5%, 10%, and 15% (v/v) of dodecane overlay are shown as circles, squares, diamonds, and triangles, respectively. The works on the WT strain grown with 1% (v/v) CO2 aeration at 0.12, 0.03, and 0.02 vvm are shown as teal, tangerine, and salmon colors, respectively. (C) Image of the WT strain cultivated in A+ medium with 0%, 5%, 10%, and 15% (v/v) of dedocane overlay, respectively. The error bars represent standard deviations of means (mean ± SD, n = 3).
The different concentrations of dodecane overlay resulted in the liquid level differences of the organic phase in the tube photobioreactor (Figure 2C). Considering the disturbance of the interface between two phases caused by bubble breakage, the moderate thickness of the organic phase is supposed to be of great significance for reducing pinene loss and the inhibition effect of dodecane overlay on cell growth. Besides, a slight loss of dodecane volume caused by gas aeration was observed on the tube wall (data not shown), which demanded an appropriate volume to reduce the calculation error caused by the volume change. Thus, we determined that a 10% (v/v) dodecane overlay was suitable for pinene harvesting from the culture medium in our tube photobioreactor.
Growth and Pinene Production of the 7002-PS Strain
To investigate the influence of genetic modification on the photoautotrophic growth of Synechococcus, we compared the photoautotrophic growth rates measured by daily OD730 between the WT strain and three transformants of the 7002-PS strain grown in tube photobioreactor for 6 days (Figure 3A). No significant variation was observed among the examined strains under culture conditions of 30°C, 100 μmol photons m−2 s−1, and 1% (v/v) CO2 aeration. Obviously, metabolic stress caused by the overexpression of transgene and the engineered flux of GPP towards pinene production has no adverse effect on the growth of cyanobacteria grown under the conditions described above.
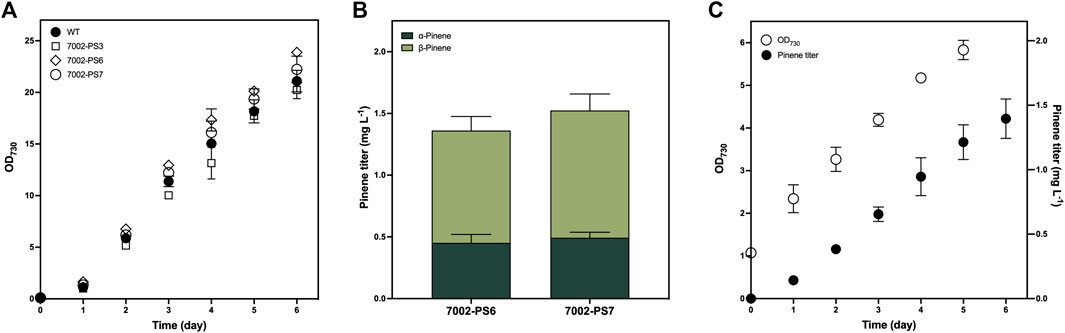
FIGURE 3. Growth and pinene production of the 7002-PS strain. (A) Photoautotrophic growth of the WT strain and three independent transformants of the 7002-PS strain grown with 1% (v/v) CO2 aeration at 0.12 vvm for 6 days. Samples were collected for the analysis at 1-day intervals. (B) Pinene production of 7002-PS6 and 7002-PS7 after a 72-h cultivation with 10% (v/v) dodecane overlay and 1% (v/v) CO2 aeration at 0.12 vvm. (C) Photoautotrophic growth and pinene production of 7002-PS7 grown with 10% (v/v) dodecane overlay and 1% (v/v) CO2 aeration at 0.03 vvm for 6 days. Samples were collected for the analysis at 1-day intervals. The growth parameters were monitored by OD730, and the pinene titers were analyzed on a gas chromatography–mass spectrometer (GC-MS). The error bars represent standard deviations of means (mean ± SD, n = 3).
As the colony PCR results confirmed that the integrated sequence had not entirely replaced the neutral region of pAQ1 between two open-reading frames (Figure 1B), numerous transformants of the 7002-PS strain were supposed to exhibit discrepant production performance. In this work, aeration rates at 0.12 and 0.03 vvm were applied to realize a 72-h and a 6-day period of pinene production, respectively. Two independent transformants of the 7002-PS strain were selected for 72-h pinene production with 10% (v/v) dodecane and 1% (v/v) CO2 aeration at 0.12 vvm, resulting in the pinene titer at 1.362 ± 0.148 mg L−1 and 1.525 ± 0.l45 mg L−1 (pinene productivity at 0.110 ± 0.018 mg L−1 OD730−1 and 0.114 ± 0.013 mg L−1 OD730−1), respectively (Figure 3B). The transformant with higher pinene-producing performance, named 7002-PS7, showed relatively stable pinene productivity of 0.246 ± 0.05 mg L−1 day−1 for a 6-day period of pinene production with 10% (v/v) dodecane and 1% (v/v) CO2 aeration at 0.03 vvm (Figure 3C). Besides, the α/β isomer ratios exhibited by the 7002-PS strain were also investigated (Figure 3B). Interestingly, AgPS expressed in Synechococcus resulted in a ∼33:67 mixture of α- to β-pinene, in which a significant increase in β-pinene production was realized compared to that produced in E. coli (Sarria et al., 2014). We hypothesized that the cellular metabolic environment of Synechococcus is suitable for forming the β-pinene isomer.
Conclusion
Pinene is of particular interest due to the excellent potential for its dimer to be aircraft fuel. This study described the construction of pinene-producing strain harboring AgPS-overexpressing cassette in Synechococcus and investigated its performance of photoautotrophic growth and pinene production using CO2 bubble aeration. Moreover, we determined the concentration of dodecane overlay suitable for collecting volatile pinene under aeration conditions. As a result, we successfully realized the photosynthetic production of pinene directly from CO2 with a productivity of up to 1.525 ± 0.l45 mg l−1 (α/β isomer ratios at ∼33:67) after 72 h of cultivation. However, the cultivation condition and the pinene-producing pathway could be further optimized, and the gas-trapping system (Kiyota et al., 2014) is more likely suitable for long-term production. Therefore, there still exists a significant opportunity for higher productivity of pinene in Synechococcus.
Data Availability Statement
The original contributions presented in the study are included in the article/Supplementary Material, further inquiries can be directed to the corresponding authors.
Author Contributions
RY and LZ designed the experiments. RY and ZY performed the experiments. LZ, TL, and DZ directed the research. L-YZ participated in the research. RY and LZ wrote and revised the manuscript. All authors contributed to the article and approved the submitted version.
Funding
This work was financially supported by grants from the National Key Research and Development Program of China (2021YFA0910700), the “Huxiang Young Talents Plan” Project of Hunan Province (2019RS2030), the National Natural Science Foundation of China (31870855 and 32171429), and the Fund for NUDT Young Innovator Awards (20190104).
Conflict of Interest
The authors declare that the research was conducted in the absence of any commercial or financial relationships that could be construed as a potential conflict of interest.
Publisher’s Note
All claims expressed in this article are solely those of the authors and do not necessarily represent those of their affiliated organizations, or those of the publisher, the editors, and the reviewers. Any product that may be evaluated in this article, or claim that may be made by its manufacturer, is not guaranteed or endorsed by the publisher.
Acknowledgments
The authors acknowledge Ding He, Hui Wang, and Tianzhong Liu for the support of experimental conditions.
Supplementary Material
The Supplementary Material for this article can be found online at: https://www.frontiersin.org/articles/10.3389/fbioe.2021.779437/full#supplementary-material
References
Behr, A., and Johnen, L. (2009). Myrcene as a Natural Base Chemical in Sustainable Chemistry: A Critical Review. Chemsuschem 2 (12), 1072–1095. doi:10.1002/cssc.200900186
Betterle, N., and Melis, A. (2019). Photosynthetic Generation of Heterologous Terpenoids in Cyanobacteria. Biotechnol. Bioeng. 116 (8), 2041–2051. doi:10.1002/bit.26988
Bohlmann, J., Steele, C. L., and Croteau, R. (1997). Monoterpene Synthases from Grand Fir (Abies Grandis). J. Biol. Chem. 272 (35), 21784–21792. doi:10.1074/jbc.272.35.21784
Campbell, D., Hurry, V., Clarke, A. K., Gustafsson, P., and Öquist, G. (1998). Chlorophyll Fluorescence Analysis of Cyanobacterial Photosynthesis and Acclimation. Microbiol. Mol. Biol. Rev. 62(3), 667–683. doi:10.1128/mmbr.62.3.667-683.1998
Carroll, A. L., Case, A. E., Zhang, A., and Atsumi, S. (2018). Metabolic Engineering Tools in Model Cyanobacteria. Metab. Eng. 50, 47–56. doi:10.1016/j.ymben.2018.03.014
Choi, Y.-N., Lee, J. W., Kim, J. W., and Park, J. M. (2020). Acetyl-CoA-derived Biofuel and Biochemical Production in Cyanobacteria: a Mini Review. J. Appl. Phycol 32 (3), 1643–1653. doi:10.1007/s10811-020-02128-x
Conde, F. R., Churio, M. S., and Previtali, C. M. (2000). The Photoprotector Mechanism of Mycosporine-like Amino Acids. Excited-State Properties and Photostability of Porphyra-334 in Aqueous Solution. J. Photochem. Photobiol. B 56 (2-3), 139–144. doi:10.1016/s1011-1344(00)00066-x
Cosgrove, J., and Borowitzka, M. (2006). Applying Pulse Amplitude Modulation (PAM) Fluorometry to Microalgae Suspensions: Stirring Potentially Impacts Fluorescence. Photosynth Res. 88 (3), 343–350. doi:10.1007/s11120-006-9063-y
Davies, F. K., Jinkerson, R. E., and Posewitz, M. C. (2015). Toward a Photosynthetic Microbial Platform for Terpenoid Engineering. Photosynth Res. 123 (3), 265–284. doi:10.1007/s11120-014-9979-6
Davies, F. K., Work, V. H., Beliaev, A. S., and Posewitz, M. C. (2014). Engineering Limonene and Bisabolene Production in Wild Type and a Glycogen-Deficient Mutant of Synechococcus Sp. PCC 7002. Front. Bioeng. Biotechnol. 2, 21. doi:10.3389/fbioe.2014.00021
Dienst, D., Wichmann, J., Mantovani, O., Rodrigues, J. S., and Lindberg, P. (2020). High Density Cultivation for Efficient Sesquiterpenoid Biosynthesis in Synechocystis Sp. PCC 6803. Sci. Rep. 10 (1), 5932. doi:10.1038/s41598-020-62681-w
Díez, B., and Ininbergs, K. (2014). “Ecological Importance of Cyanobacteria,” in Cyanobacteria (Hoboken, NJ: John Wiley & Sons, Ltd), 41–63. doi:10.1002/9781118402238.ch3
Frigaard, N.-U., Sakuragi, Y., and Bryant, D. A. (2004). “Using In Vitro-Made DNA Constructs and Natural Transformation,” in Gene Inactivation in the Cyanobacterium Synechococcus Sp. PCC 7002 and the Green Sulfur Bacterium Chlorobium tepidum Photosynthesis Research Protocols. Editor R. Carpentier (Totowa, NJ: Humana Press), 325–340.
Fuhrmann, M., Hausherr, A., Ferbitz, L., Schödl, T., Heitzer, M., and Hegemann, P. (2004). Monitoring Dynamic Expression of Nuclear Genes in Chlamydomonas Reinhardtii by Using a Synthetic Luciferase Reporter Gene. Plant Mol. Biol. 55 (6), 869–881. doi:10.1007/s11103-004-2150-610.1007/s11103-005-2150-1
Gomes-Carneiro, M. R., Viana, M. E. S., Felzenszwalb, I., and Paumgartten, F. J. R. (2005). Evaluation of β-myrcene, α-terpinene and (+)- and (−)-α-Pinene in the Salmonella/microsome Assay. Food Chem. Toxicol. 43 (2), 247–252. doi:10.1016/j.fct.2004.09.011
Harvey, B. G., Wright, M. E., and Quintana, R. L. (2010). High-Density Renewable Fuels Based on the Selective Dimerization of Pinenes. Energy Fuels 24 (1), 267–273. doi:10.1021/ef900799c
Kirby, J., and Keasling, J. D. (2009). Biosynthesis of Plant Isoprenoids: Perspectives for Microbial Engineering. Annu. Rev. Plant Biol. 60, 335–355. doi:10.1146/annurev.arplant.043008.091955
Kiyota, H., Okuda, Y., Ito, M., Hirai, M. Y., and Ikeuchi, M. (2014). Engineering of Cyanobacteria for the Photosynthetic Production of Limonene from CO2. J. Biotechnol. 185, 1–7. doi:10.1016/j.jbiotec.2014.05.025
Lin, P.-C., and Pakrasi, H. B. (2019). Engineering Cyanobacteria for Production of Terpenoids. Planta 249 (1), 145–154. doi:10.1007/s00425-018-3047-y
Ludwig, M., and Bryant, D. A. (2011). Transcription Profiling of the Model Cyanobacterium Synechococcus Sp. Strain PCC 7002 by Next-Gen (SOLiD) Sequencing of cDNA. Front. Microbio. 2, 41. doi:10.3389/fmicb.2011.00041
Ma, Z., Ahmed, F., Yuan, B., and Zhang, W. (2019). Fresh Living Arthrospira as Dietary Supplements: Current Status and Challenges. Trends Food Sci. Tech. 88, 439–444. doi:10.1016/j.tifs.2019.04.010
Markley, A. L., Begemann, M. B., Clarke, R. E., Gordon, G. C., and Pfleger, B. F. (2015). Synthetic Biology Toolbox for Controlling Gene Expression in the Cyanobacterium Synechococcus Sp. Strain PCC 7002. ACS Synth. Biol. 4 (5), 595–603. doi:10.1021/sb500260k
Moser, S., and Pichler, H. (2019). Identifying and Engineering the Ideal Microbial Terpenoid Production Host. Appl. Microbiol. Biotechnol. 103 (14), 5501–5516. doi:10.1007/s00253-019-09892-y
Niu, F.-X., He, X., Wu, Y.-Q., and Liu, J.-Z. (2018). Enhancing Production of Pinene in Escherichia coli by Using a Combination of Tolerance, Evolution, and Modular Co-culture Engineering. Front. Microbiol. 9, 1623. doi:10.3389/fmicb.2018.01623
Parmar, A., Singh, N. K., Pandey, A., Gnansounou, E., and Madamwar, D. (2011). Cyanobacteria and Microalgae: a Positive prospect for Biofuels. Bioresour. Tech. 102 (22), 10163–10172. doi:10.1016/j.biortech.2011.08.030
Pattanaik, B., and Lindberg, P. (2015). Terpenoids and Their Biosynthesis in Cyanobacteria. Life 5 (1), 269–293. doi:10.3390/life5010269
Rajneesh, , , Singh, S. P., Pathak, J., and Sinha, R. P. (2017). Cyanobacterial Factories for the Production of green Energy and Value-Added Products: An Integrated Approach for Economic Viability. Renew. Sust. Energ. Rev. 69, 578–595. doi:10.1016/j.rser.2016.11.110
Sarma, M. K., Kaushik, S., and Goswami, P. (2016). Cyanobacteria: A Metabolic Power House for Harvesting Solar Energy to Produce Bio-Electricity and Biofuels. Biomass and Bioenergy 90, 187–201. doi:10.1016/j.biombioe.2016.03.043
Sarria, S., Wong, B., Martín, H. G., Keasling, J. D., and Peralta-Yahya, P. (2014). Microbial Synthesis of Pinene. ACS Synth. Biol. 3 (7), 466–475. doi:10.1021/sb4001382
Schopf, J. W., and Packer, B. M. (1987). Early Archean (3.3-billion to 3.5-Billion-Year-Old) Microfossils from Warrawoona Group, Australia. Science 237, 70–73. doi:10.1126/science.11539686
Silva, C. E. D., Gris, B., Sforza, E., La Rocca, N., and Bertucco, A. (2016). “Effects of Sodium Bicarbonate on Biomass and Carbohydrate Production in Synechococcus PCC 7002,” in 5th International Symposium on Industrial Biotechnology (Ibic 2016), Bologna, Italy, April 10–11, 2016, 49, 241–246. doi:10.3303/Cet1649041
Simmons, T. L., Andrianasolo, E., McPhail, K., Flatt, P., and Gerwick, W. H. (2005). Marine Natural Products as Anticancer Drugs. Mol. Cancer Ther. 4 (2), 333–342.
Singh, V., Chaudhary, D. K., Mani, I., and Dhar, P. K. (2016). Recent Advances and Challenges of the Use of Cyanobacteria towards the Production of Biofuels. Renew. Sust. Energ. Rev. 60, 1–10. doi:10.1016/j.rser.2016.01.099
Stevens, S. E., and Porter, R. D. (1980). Transformation in Agmenellum Quadruplicatum. Proc. Natl. Acad. Sci. 77 (10), 6052–6056. doi:10.1073/pnas.77.10.6052
Sun, T., Li, S., Song, X., Diao, J., Chen, L., and Zhang, W. (2018). Toolboxes for Cyanobacteria: Recent Advances and Future Direction. Biotechnol. Adv. 36, 1293–1307. doi:10.1016/j.biotechadv.2018.04.007
Tashiro, M., Kiyota, H., Kawai-Noma, S., Saito, K., Ikeuchi, M., Iijima, Y., et al. (2016). Bacterial Production of Pinene by a Laboratory-Evolved Pinene-Synthase. ACS Synth. Biol. 5 (9), 1011–1020. doi:10.1021/acssynbio.6b00140
Teruya, T., Sasaki, H., Fukazawa, H., and Suenaga, K. (2009). Bisebromoamide, a Potent Cytotoxic Peptide from the marine Cyanobacterium Lyngbya sp.: Isolation, Stereostructure, and Biological Activity. Org. Lett. 11 (21), 5062–5065. doi:10.1021/ol9020546
Walls, L. E., and Rios-Solis, L. (2020). Sustainable Production of Microbial Isoprenoid Derived Advanced Biojet Fuels Using Different Generation Feedstocks: A Review. Front. Bioeng. Biotechnol. 8, 599560. doi:10.3389/fbioe.2020.599560
Wang, C., Liwei, M., Park, J.-B., Jeong, S.-H., Wei, G., Wang, Y., et al. (2018). Microbial Platform for Terpenoid Production: Escherichia coli and Yeast. Front. Microbiol. 9, 2460. doi:10.3389/fmicb.2018.02460
Wei, L.-J., Zhong, Y.-T., Nie, M.-Y., Liu, S.-C., and Hua, Q. (2021). Biosynthesis of α-Pinene by Genetically Engineered Yarrowia Lipolytica from Low-Cost Renewable Feedstocks. J. Agric. Food Chem. 69 (1), 275–285. doi:10.1021/acs.jafc.0c06504
Wu, X., Ma, G., Liu, C., Qiu, X.-y., Min, L., Kuang, J., et al. (2021). Biosynthesis of Pinene in Purple Non-sulfur Photosynthetic Bacteria. Microb. Cel Fact 20 (1), 101. doi:10.1186/s12934-021-01591-6
Xia, P. F., Ling, H., Foo, J. L., and Chang, M. W. (2019). Synthetic Biology Toolkits for Metabolic Engineering of Cyanobacteria. Biotechnol. J. 14 (6), 1800496. doi:10.1002/biot.201800496
Xu, Y., Alvey, R. M., Byrne, P. O., Graham, J. E., Shen, G., and Bryant, D. A. (2011). Expression of Genes in Cyanobacteria: Adaptation of Endogenous Plasmids as Platforms for High-Level Gene Expression in Synechococcus Sp. PCC 7002. Methods Mol. Biol. (Clifton, N.J.) 684, 273–293. doi:10.1007/978-1-60761-925-3_21
Yang, M.-k., Qiao, Z.-x., Zhang, W.-y., Xiong, Q., Zhang, J., Li, T., et al. (2013). Global Phosphoproteomic Analysis Reveals Diverse Functions of Serine/Threonine/Tyrosine Phosphorylation in the Model Cyanobacterium Synechococcus Sp. Strain PCC 7002. J. Proteome Res. 12 (4), 1909–1923. doi:10.1021/pr4000043
Keywords: terpenoids, pinene, cyanobacteria, pinene synthase, dodecane
Citation: Yang R, Zhu L, Li T, Zhu L-y, Ye Z and Zhang D (2021) Photosynthetic Conversion of CO2 Into Pinene Using Engineered Synechococcus sp. PCC 7002. Front. Bioeng. Biotechnol. 9:779437. doi: 10.3389/fbioe.2021.779437
Received: 18 September 2021; Accepted: 22 November 2021;
Published: 17 December 2021.
Edited by:
Shuobo Shi, Beijing University of Chemical Technology, ChinaReviewed by:
Michael Jahn, Royal Institute of Technology, SwedenRajib Saha, University of Nebraska-Lincoln, United States
Copyright © 2021 Yang, Zhu, Li, Zhu, Ye and Zhang. This is an open-access article distributed under the terms of the Creative Commons Attribution License (CC BY). The use, distribution or reproduction in other forums is permitted, provided the original author(s) and the copyright owner(s) are credited and that the original publication in this journal is cited, in accordance with accepted academic practice. No use, distribution or reproduction is permitted which does not comply with these terms.
*Correspondence: Zi Ye, eWV6aUBpaGIuYWMuY24=; Dongyi Zhang, ZG9uZ3lpemhhbmdAbnVkdC5lZHUuY24=
†These authors have contributed equally to this work and share first authorship