- 1Departamento de Ingeniería Mecánica, Universidad de Santiago de Chile, Santiago, Chile
- 2Departamento de Física, Universidad de Santiago de Chile, Santiago, Chile
- 3Department of Mechanical and Metallurgical Engineering, Pontificia Universidad Católica de Chile, Santiago, Chile
- 4Institute of Health Sciences, Universidad de O’Higgins, Rancagua, Chile
- 5Pathophysiology Program, Faculty of Medicine, Institute of Biomedical Sciences (ICBM), Universidad de Chile, Santiago, Chile
- 6International Center for Andean Studies (INCAS), Universidad de Chile, Santiago, Chile
This study aims to assess the efficacy of melatonin in mitigating the adverse effects of hypobaric hypoxia on the cardiovascular system of neonatal lambs (30 days old). Two groups were considered for this purpose: (i) Melatonin-treated group (N = 5) and (ii) Control group (N = 6) without treatment. All subjects were exposed to hypobaric hypoxia during gestation and perinatal periods, with melatonin administered after birth. The study focused on the carotid artery, a known predictor of cardiovascular risk. Biomechanical tests, morphometric, and histological measurements were conducted, and a numerical model was developed based on the biomechanical data. Key findings showed remodeling effects: Firstly, a realignment of collagen fibers towards a longitudinal direction was observed with melatonin treatment, similar to non-hypoxic arteries. Second, changes in residual stress and ex-vivo luminal radius were noted, aiming to reduce wall stress and increase vascular resistance. These changes indicate an antihypertensive response, reducing the effects of increased blood pressure and flow due to hypobaric hypoxia. This study demonstrates that biomechanical and histomorphometric methodologies effectively assess the beneficial effects of melatonin treatment under hypobaric hypoxia exposure.
1 Introduction
Vasculature is particularly affected by changes in the physiological environment, manifested in adaptative/maladaptative processes (Sehgal et al., 2019). Accordingly, biomechanics has been proven as a successful tool in assessing vascular impairments, particularly related to the aging process (Haskett et al., 2010), along with the development and progression of cardiovascular diseases (Lasheras, 2007; Walsh et al., 2014; Murtada et al., 2021; Tong et al., 2023).
Focusing attention on the arteries, their characteristic microstructural configuration plays a key role in the biomechanical response under physiological conditions. The mechanical behavior is mainly governed by the action of elastin and collagen fibers (extracellular matrix (ECM)), along with the smooth muscle cells (SMC) (Pukaluk et al., 2024; Holzapfel and Ogden, 2018). Kochova et al., 2012 (Kochová et al., 2012) found out the passive biomechanical effect of each one of these components by its respective degradation, through the inflation-deflation testing. They discovered that elastin is related to an increased diameter at physiological pressure levels without determining stiffness changes, which is attributed to collagen influence. In contrast, SMC content induces both diameter change and arterial wall stiffening at supra-physiological pressures.
Hypoxia, characterized by low oxygen levels at the cellular and tissue levels, is detrimental to maintaining normal physiological processes (Ream et al., 2008). In the gestational stage, hypoxia induced by a high-altitude environment (commonly referred to as hypobaric hypoxia, HH) is classified as a kind of preplacental hypoxia, due to both mother and fetus being subjected to this condition (Hutter et al., 2010). Besides the geographic conditions, fetal hypoxia can also develop as a result of biological abnormalities, namely, impaired development of the placenta during the early pregnancy period (Eskild et al., 2016), umbilical cord occlusion (Kawagoe et al., 1999), and maternal diabetes (Klemetti et al., 2021). In particular, this condition triggers an alteration in normal development in the perinatal period, where chronic exposure to HH during pregnancy has revealed high incidence of intrauterine growth restriction (IUGR) (Parraguez et al., 2005; brown and GiussanI, 2024). IUGR has been closely related to cardiopulmonary complications during life (Rueda-Clausen et al., 2009), including premature pulmonary hypertension (Herrera et al., 2010; Papamatheakis et al., 2013; Ding et al., 2020; Sigaeva et al., 2019; Steinhorn, 2017) and reduced cardiac performance (Patterson and Zhang, 2010) which leads in turn, to higher risks of adult cardiovascular disease (Giussani and Davidge, 2013; Ream et al., 2008).
Aiming to mitigate the pulmonary hypertension effects, the performance of vasodilator treatments has been assessed. In the physiological context, melatonin is naturally released by the pineal gland to the body at night, aiming to regulate the circadian and seasonal rhythms (Xu et al., 2018; Nelson and Drazen, 2000; Zisapel, 2018), along with pubertal development (Pandi-Perumal et al., 2008; Olcese, 2020). Different research has shown that when used as a treatment, melatonin exhibits antioxidant properties (Figueroa et al., 2021). This is particularly crucial in different types of hypoxia exposure conditions (Debevec et al., 2017; Farías et al., 2012; González-Candia et al., 2019). In addition, this drug has successfully mitigated effects linked to pulmonary arterial hypertension (Torres et al., 2015; Maarman and Lecour, 2021; Hung et al., 2017; Yildiz and Balcioğlu, 2024; Astorga et al., 2018). Simultaneously, several alternative treatments have been proposed for these same goals, i.e., atrial natriuretic peptide (Wiedemann et al., 2001; Werner et al., 2016; Hussain et al., 2019), cinaciguat (Beñaldo et al., 2022; Chester et al., 2011; Laubrie et al., 2023), and allopurinol (Liu-Shiu-Cheong et al., 2020; Gokcen et al., 2022).
Physiologically, the common carotid artery (CCA) plays a crucial role in the cardiovascular system, by perfusing oxygenated blood from the heart to the brain territory (Sethi et al., 2023). Structurally, it is classified as a conductive or elastic-type artery, denoted by a higher content of elastic fibers than smooth muscle cells, unlike in the peripheral muscular arteries (Brown et al., 2018). This distinctive feature impacts a high level of vascular compliance, essential for responding to the significant blood pressure fluctuations this kind of artery is subjected to (Peace et al., 2018). Different authors have shown the impact of intrauterine growth restriction (IUGR) on the carotid arteries of various animal models along perinatal development. Kucukbas and Doğan (2023) evaluated hemodynamics parameters (i.e., pulsatility and resistance indexes, along with peak systolic velocity) via ultrasound Doppler technique in the common carotid artery of fetuses with IUGR, determining an abnormal blood flow under this condition to the difference of those subjected to normal pregnancy. Paz et al. (2019) assessed the changes in carotid morphology under IUGR conditions, specifically noting a reduction in luminal diameter, while observing no alterations in vascular reactivity, neither in contractile nor dilation ex-vivo function. Cañas et al., (2018) determined the passive mechanical properties and residual stress quantification via ring tensile and ring opening tests in the aorta, carotid, and femoral arteries of guinea pigs fetuses, in pregnancies subjected to progressive uterine artery occlusion. The main results did not find conclusive evidence about changes in the mechanical behavior and residual stress in carotid arteries. Dodson et al. (2013a) studied the effect of placental insufficiency-induced IUGR in umbilical and carotid arteries of sheep near-term fetuses, determining a decrement in compliance for both studied arteries, measured through the inflation-extension test. In addition, histological observations reveal arterial remodeling, denoted by an increment in the content of elastic and collagen fibers in the carotid.
Focusing on the biomechanical aspects of perinatal development under chronic HH-induced IUGR, there is limited research (Rivera et al., 2020; Rivera et al., 2021; Navarrete et al., 2024), and even less on those of the carotid artery (Navarrete et al., 2020). On the other hand, there is little information about the drug-based treatment effects. Melatonin has been found to have a vasodilator effect in pulmonary circulation, but its impact on systemic circulation arteries is yet to be established. Based on the fact that there is evidence of alteration in the normal characteristics of the carotid artery regarding biomechanics and morphological parameters, under the mentioned condition, we hypothesize that administration of melatonin treatment in newborn lambs exposed to chronic HH during both pregnancy and postnatal periods alter the carotid artery mechanical response and its morphology.
To verify the research hypothesis, a numerical-experimental study of preclinical nature was carried out in the common carotid artery of newborn lambs gestated and bred in high altitude conditions. The passive mechanical properties were determined through biomechanical tests (explained in Section 2.2), and the corresponding characterization was performed using a suitable constitutive model (detailed in Section 2.3.1). Once the model has been characterized, a well-established numerical simulation procedure (detailed in Section 2.3.2) determines the residual stress field in the arterial wall. In addition, histological and ultrastructural assessments were conducted to determine the microstructure and morphology of the artery wall (Section 3.3).
2 Materials and methods
2.1 Materials
The study was conducted on the carotid artery of lambs whose conception, gestation, and neonatal period took place in an environment of hypobaric hypoxia at the International Center for Andean Studies (INCAS) of Universidad de Chile, located in the town of Putre at 3,600 m above sea level (m.a.s.l.).
The number of animals required for the study was determined following the 3 R s of good practices in animal experimentation (Replacement, Reduction, and Refinement of animals, from “The principles of human experimental techniques”, 1959). The samples were divided into two experimental groups.
• Control group (CN), consisting of six specimens without pharmacological treatment.
• Melatonin group (MN), consisting of five specimens treated with melatonin medication.
The pharmacological treatment consists of the oral administration of 1 mg/kg of melatonin at approximately 8 p.m. for 20 days, between four and 23 days of age. After delivery, neonates are left without interventions for 2–3 days to ensure maternal-newborn attachment and proper recognition of their mothers, which ensures good lactation. It has been done with the purpose of increasing melatonin levels at a steady rate without altering the circadian rhythm of the lambs. Euthanasia occurs at 30 days of age, through the intravenous administration of 100 mg/kg of sodium thiopental. Subsequently, the dissection and extraction of the carotid artery were carried out, and within a period not exceeding 24 h, the corresponding biomechanical tests were performed. All animal procedures for this study were carried out with the approval of the Bioethics Committee on animal research of the Faculty of Medicine of Universidad de Chile (CBA #0761 FMUCH).
2.2 Experimental methods
2.2.1 Biomechanical tests
Different biomechanical tests were conducted in the carotid artery. They were perfomed inmedialty after disection procedure. During the entire process (both extraction and testing), the sample was immersed in physiological saline solution. Moreover, after extraction, the arterial segment was preserved at 4
2.2.1.1 Uniaxial tensile test
Figure 1a, schematizes the main aspects of the experimental procedure involved in the uniaxial tensile test. For each sample, two uniaxial tensile tests were performed: one in longitudinal and the other in circumferential directions (denoted by the directions
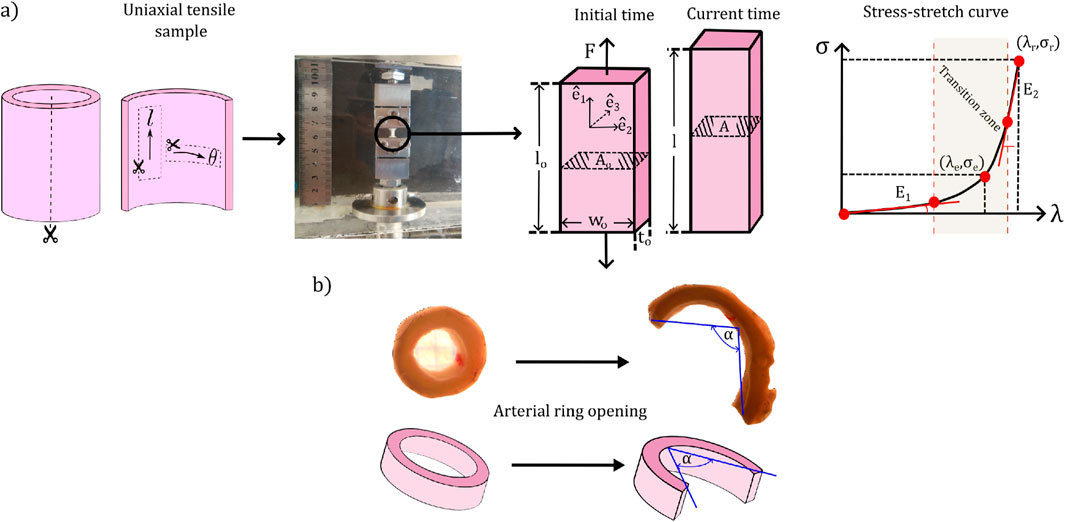
Figure 1. Set-up of biomechanical tests and representative measurements. (a) Uniaxial tensile test. (b) Ring opening test.
The test was conducted by stretching the sample in the direction
From the experimental dates, stress-stretch curves were generated, which consider the dataset of force from the beginning of the test, until when it reaches its maximum value (right side of the Figure 1a). From them, the following characteristic parameters were quantified: the slope at low strain levels
2.2.1.2 Ring opening test
In this research, a 4-mm arterial ring segment was extracted from the carotid artery, which was radially cut to measure its opening angle
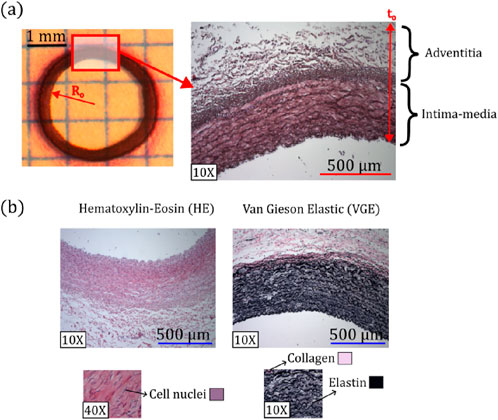
Figure 2. (a) Representative parameters of morphometric measurements. (b) Representative histological images of Hematoxylin-Eosin (HE) and van Gieson Elastic (VGE) staining, along with colors and zones where elastic fibers, collagen, and cell nuclei were quantified.
2.2.2 Histomorphometry
Figure 2a schematizes the protocol to obtain the morphometric measurements of samples, corresponding to the external radius
To determine the microstructural composition, a well-known histological procedure was applied to the artery under study (Carson and Hladik, 2009). In particular, two stains were used for this purpose (see Figure 2b). On the one hand, the Hematoxylin-Eosin (HE) staining was used to identify the cell nuclei (visualized with purple color), determining the cell nuclei density (number of cells per unit surface). Each cell nucleus was identified through a color thresholding process. The overall number of nuclei per image, was then quantified using a particle analyzer, which counts these regions based on a minimum size of 50
2.2.3 Statistical procedure
All data of the samples were expressed as mean
2.3 Numerical methods
2.3.1 Constitutive modelling
Continuum mechanics models soft tissue’s purely elastic mechanical response as those of hyperelastic materials (Dwivedi et al., 2022). According to the intrinsic characteristics of the material under study, a suitable hyperelastic constitutive model must be considered for this purpose. In particular, several authors have considered the artery wall to be an isotropic ground matrix fiber-reinforced (Huh et al., 2019; Lee et al., 2019; Pagoulatou et al., 2021). This idea is supported by observations of its microstructure, where the isotropic matrix represents the non-preferential direction of the elastic fibers, whereas the fibrous part in the model reflects the preferential orientation of the collagen fibers along the arterial wall. Thus, we opted for the transversely isotropic Gasser-Holzapfel-Ogden (GHO) model in this study (Gasser et al., 2006).
In general, for hyperelastic materials, the stress-strain relationship is determined based on the strain energy function
which involves the following terms: (i) The first invariant of the right Cauchy-Green strain tensor
A particular consideration about arterial tissue is its incompressibility, where the volume does not change in the face of any characteristic deformation state (García-Herrera et al., 2016). In this case, the definition of the stress state is not only deformation-dependent, since added hydrostatic pressure
where
where
where the tensor
Particular case: uniaxial tensile test.
Three-dimensional spatial region designated for the tensile test (Figure 1a) is defined by the orthogonal unit vector triad
In particular, in the case of uniaxial tensile test, the definition of right Cauchy-Green strain tensor corresponds to the diagonal matrix
The set of material parameters defined by the GHO model (i.e.,
2.3.2 Numerical simulation of the ring closure
Aiming to determine the residual stress field across the artery wall, the numerical simulation of the inverse process to the experimental ring opening test is described below. Experimental information of the ring opening angle
Figure 3a, outlines the numerical procedure in the simulation, where material homogeneity and geometrical symmetry were considered (García-Herrera et al., 2016). The referential configuration (region ABCD) corresponds to a stress-free, open and stabilized arterial ring. The numerical ring-closure was achieved by imposing displacements on the line AB, which moves via A’B’ and finishes in the closed ring configuration (A″B″ position).
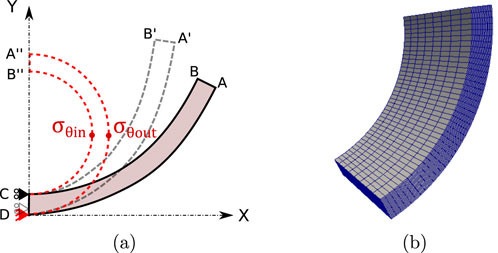
Figure 3. (a) Representation 2D of the boundary conditions for the computational simulation of the closure of the rings, (b) 3D finite element mesh [generated in Gmsh (Geuzaine and Remacle, 2009) and visualized in ParaView software].
To achieve the adequate reconstruction of the closed geometry, the mean perimeter of the closed arterial ring is related to the arc formed by its respective open configuration. An analytical expression (Equation 6) is used to relate the mean radius of the open configuration
where
Simulations were performed using the Finite Element Method (FEM) via an in-house code (Celentano et al., 1996; Celentano, 2001). In the FEM context, incompressibility in hyperelastic models (as GHO) was implemented through a nearly incompressible condition, by adding an appropriate penalty parameter
From simulation, two characteristic values were considered: circumferential residual Cauchy stress in the inner
3 Results
From the experimental and numerical procedure described in Section 2, the biomechanical, structural, and morphometric results are listed in detail below.
3.1 Uniaxial tensile test
Table 1 exhibits the mechanical parameters directly determined from tensile curves for both study groups, as displayed in the procedure stated in Section 2.2.1.
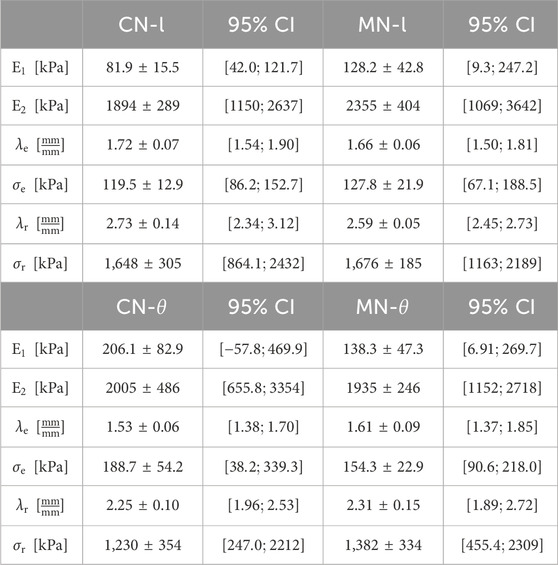
Table 1. Average
From the Table 1, material stiffening at low and high deformation levels, (
According to the fitting procedure carried out for the hyperelastic model (GHO) in this study (Section 2.3.1), the corresponding material parameters have been displayed in Table 2.
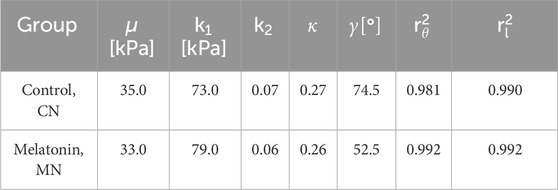
Table 2. Values for the GHO model parameters were optimized to fit the experimental data obtained from uniaxial tension tests. The coefficients
When comparing the GHO parameters, fitted from the mean stress-stretch curves obtained from control and melatonin groups,
Figures 4a, b, depict the experimental (
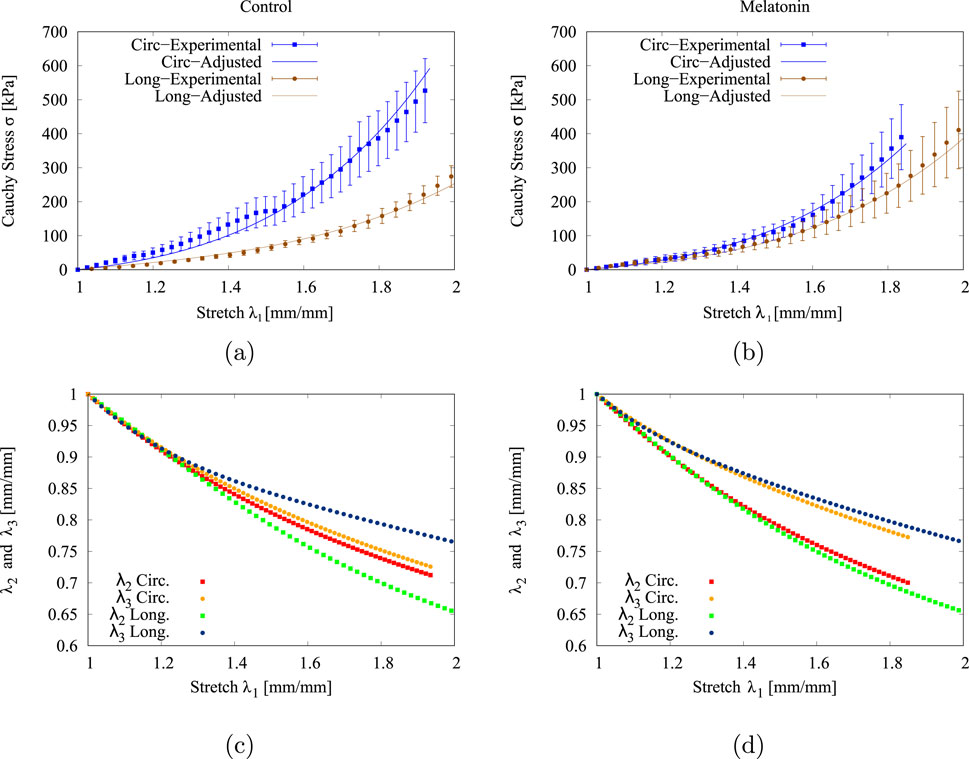
Figure 4. Experimental (
3.2 Ring-opening test and residual stress
Following the procedure described in Section 2.2.1, the opening angle
The mean
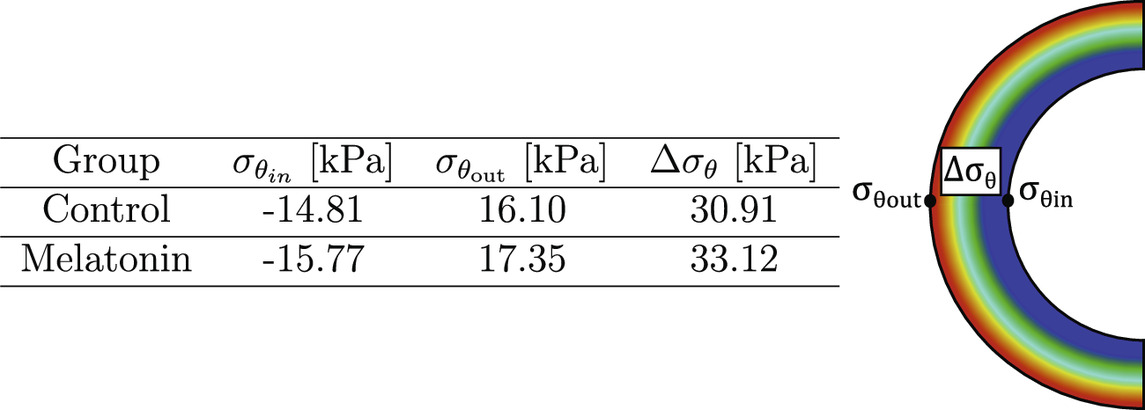
Table 3. Circumferential residual stress in inner and outer arterial radius (
3.3 Histomorphometry
The different characteristics that describe the morphometric and microstructural composition of the carotid artery wall are detailed in Table 4.
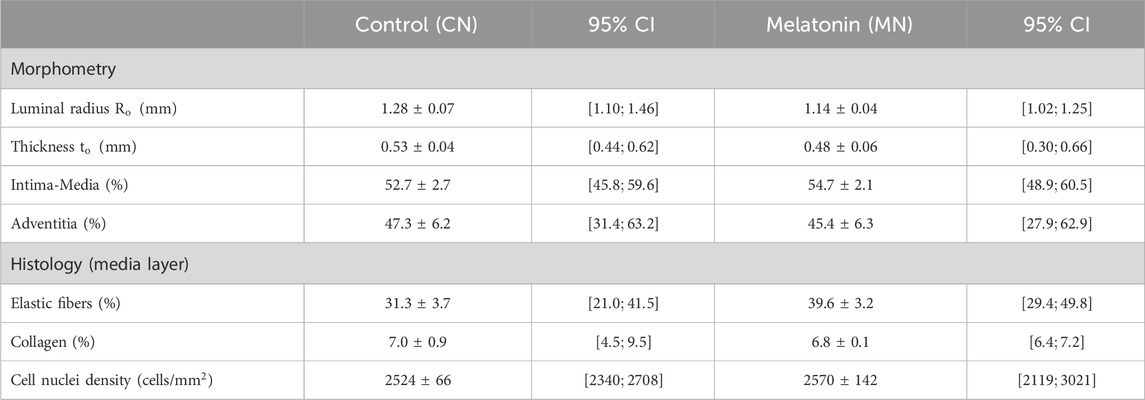
Table 4. Average
Within structural measures, luminal radius
In the same way, changes in both mean values exhibited below are related to an increase in the wall percentage covered by the intima-media (CN
Histological results, which include the percentage of collagen and elastic fibers, along with the density of the cell nuclei (Table 4), were performed following the protocol stated in Section 2.2. Elastic fibers exhibit a tendency of higher content in arteries treated with melatonin (CN
4 Discussion
This preclinical study has been conducted to identify the main aspects related to HH exposure and the effects of drug-based treatment on biomechanics, morphometric and histology measurements.
Physiologically, the study on the carotid artery is of clinical relevance because alteration of its properties and structure has been widely identified as an indicator of cardiovascular risks, triggering adverse consequences mainly on the cerebral territory (Moghadasi et al., 2024; Hirata et al., 2006). Morphological, changes in intima-media and adventitia thickness have been assessed as indicators of atherosclerosis and coronary heart disease (Mohamed et al., 2023; Skilton et al., 2011; Ebrahimi, 2009). Furthermore, alteration of the microstructural components of the arterial wall, such as elastin degradation (Fonck et al., 2007) and density decrement (Kamenskiy et al., 2015), have been directly associated with atherosclerosis and hypertension. Likewise, the assessment of biomechanical metrics, such as 2D strain, stress, energy storage, and arterial stiffness, among others, have been linked to both cardiovascular complications (Kim et al., 2012; Lanne et al., 1994; Forsblad-D’Elia et al., 2021; Olver et al., 2016) and aging (Gkousioudi et al., 2022; Sherman et al., 2022). Therefore, the biomechanical, morphological, and structural characteristics assessed in the current work are relevant to determine potential adverse cardiovascular effects in the face of hypobaric hypoxia exposure.
From Table 1, no biomechanical parameter obtained exhibits any statistical differences between groups. However, the results obtained via numerical analysis, expressed in terms of the material parameters of the GHO hyperelastic model, show that the phenomenological parameter
Residual stress plays a key role in arteries, which has been closely related to blood vessel remodeling (Cheng and Zhang, 2019). Biomechanically, the effect of residual stress arises intending to diminish the peaks or gradients in pressure on the artery wall, which is generated from blood pressure (Zahn and Balzani, 2018; Cañas et al., 2018). The residual stress field obtained from the numerical simulation procedure detailed in Section 2.3 is represented through the parameters shown in Table 3, whose values are dependent on the circumferential residual strain (quantified by the opening angle), and the material parameters from GHO model. In this sense, according to Equation 1, the first term of the GHO model
Within the scope of this study, the effects of melatonin were examined only under HH conditions. The impact of the treatment at sea-level conditions was not addressed in this work. Precisely, this aspect would be interesting to address in future studies to evaluate the baseline parameters that the melatonin group exposed to HH should reach. In addition, an increase in the number of specimens in each group could lead to more conclusive results on the outcomes.
5 Conclusion
Through a coupled experimental-numerical approach, the influence of melatonin treatment on the biomechanical response in carotid arteries subjected to hypoxia hypobaric condition has been assessed. Aiming to this goal, several relevant biomechanical parameters have been determined for this goal, i.e., material stiffness, stress-stretch rupture levels, and residual stress. In addition, morphometric and histological measurements arise as further insights to give explanation to the mechanism that governs the characteristic mechanical response on the artery.
Melatonin treatment in animals exposed to gestational and perinatal hypobaric hypoxia primarily induces collagen fiber reorientation, rather than changes in extracellular matrix proliferation, degradation, or cell nuclei density. This is supported by biomechanical responses from uniaxial tensile tests and alterations in the hyperelastic model parameter
Future studies should focus on investigating the impact of different melatonin doses in distinct developmental stages, including long-term effects. New biomechanical analysis can be conducted on the artery wall, determining the effect of the active response, viscoelastic effects, and damage phenomenon, among others. Furthermore, the exploration of alternative biomechanical models encompassing growth and remodeling phenomena, as well as the impact of fiber degradation (Humphrey, 2021) can be explored. Moreover, novel ultrastructural techniques can be explored to obtain additional information regarding the microstructural phenomena carried out during the remodeling process on the arterial wall (i.e., second harmonic generation, atomic force microscopy, electron microscopy, and multiphoton imaging), which cannot be obtained through conventional histological techniques.
Data availability statement
The raw data supporting the conclusions of this article will be made available by the authors, without undue reservation.
Ethics statement
The animal study was approved by the Bioethics Committee of Universidad de Chile (Protocol CBA 761 FMUCH). The study was conducted in accordance with the local legislation and institutional requirements.
Author contributions
ER: Conceptualization, Data curation, Formal Analysis, Funding acquisition, Investigation, Methodology, Project administration, Supervision, Validation, Writing – original draft, Writing – review and editing. AN: Conceptualization, Data curation, Formal Analysis, Methodology, Software, Validation, Visualization, Writing – original draft, Writing – review and editing. CG-H: Conceptualization, Formal Analysis, Funding acquisition, Investigation, Methodology, Supervision, Validation, Writing – original draft, Writing – review and editing. LG: Formal Analysis, Funding acquisition, Investigation, Methodology, Visualization, Writing – original draft, Writing – review and editing. EC: Formal Analysis, Funding acquisition, Investigation, Methodology, Visualization, Writing – original draft, Writing – review and editing. DC: Conceptualization, Formal Analysis, Methodology, Software, Validation, Writing – original draft, Writing – review and editing. AG-C: Data curation, Formal Analysis, Investigation, Methodology, Visualization, Writing – original draft, Writing – review and editing. EH: Conceptualization, Funding acquisition, Investigation, Methodology, Project administration, Resources, Supervision, Visualization, Writing – original draft, Writing – review and editing.
Funding
The author(s) declare that financial support was received for the research and/or publication of this article. Authors want to thank to: DICYT associative project, code No 052316RM_DAS, provided by “Vicerrectoría de Investigación, Innovación y Creación” from Universidad de Santiago de Chile. Support from “Agencia Nacional de Investigación y Desarrollo” (ANID) through project FONDECYT No 1151119.
Conflict of interest
The authors declare that the research was conducted in the absence of any commercial or financial relationships that could be construed as a potential conflict of interest.
Generative AI statement
The author(s) declare that no Generative AI was used in the creation of this manuscript.
Publisher’s note
All claims expressed in this article are solely those of the authors and do not necessarily represent those of their affiliated organizations, or those of the publisher, the editors and the reviewers. Any product that may be evaluated in this article, or claim that may be made by its manufacturer, is not guaranteed or endorsed by the publisher.
References
Astorga, C. R., González-Candia, A., Candia, A. A., Figueroa, E. G., Cañas, D., Ebensperger, G., et al. (2018). Melatonin decreases pulmonary vascular remodeling and oxygen sensitivity in pulmonary hypertensive newborn lambs. Front. physiology 9, 185. doi:10.3389/FPHYS.2018.00185
Avril, S., Badel, P., Gabr, M., Sutton, M. A., and Lessner, S. M. (2013). Biomechanics of porcine renal arteries and role of axial stretch. J. Biomechanical Eng. 135, 0810071. issn 01480731. Available from. doi:10.1115/1.4024685
Beñaldo, F. A., Araya-Quijada, C., Ebensperger, G., Herrera, E. A., Reyes, R. V., Moraga, F. A., et al. (2022). Cinaciguat (BAY-582667) modifies cardiopulmonary and systemic circulation in chronically hypoxic and pulmonary hypertensive neonatal lambs in the alto andino. Front. Physiology 13, 1. issn 1664042X. Available from. doi:10.3389/fphys.2022.864010
Brown, E. R., and Giussani, D. A. (2024). Cause of fetal growth restriction during high-altitude pregnancy. iScience 27, 109702. Available from. doi:10.1016/J.ISCI.2024.109702
Brown, I. A. M., Diederich, L., Good, M. E., DeLalio, L. J., Murphy, S. A., Cortese-Krott, M. M., et al. (2018). Vascular smooth muscle remodeling in conductive and resistance arteries in hypertension. Arteriosclerosis, Thrombosis, Vasc. Biol. 38, 1969–1985. issn 15244636. Available from. doi:10.1161/ATVBAHA.118.311229
Cañas, D., García-Herrera, C. M., Herrera, E. A., Celentano, D. J., and Krause, B. J. (2018). Mechanical characterization of arteries affected by fetal growth restriction in Guinea pigs (Cavia porcellus). J. Mech. Behav. Biomed. Mater. 88, 92–101. issn 18780180. Available from. doi:10.1016/j.jmbbm.2018.08.010
Cardamone, L., Valentín, A., Eberth, J. F., and Humphrey, J. D. (2009). Origin of axial prestretch and residual stress in arteries. Biomechanics Model. Mechanobiol. 8 (6), 431–446. Available from. doi:10.1007/S10237-008-0146-X
Carson, F., and Hladik, C. (2009). Histotechnology: a self-instructional. 3ed. Am. Soc. Clin. Pathology.
Celentano, D. (2001). A large strain thermoviscoplastic formulation for the solidification of S.G. cast iron in a green sand mould. Int. J. Plasticity 17, 1623–1658. Available from. doi:10.1016/S0749-6419(00)00095-4
Celentano, D., Oller, S., and Oñate, E. (1996). A coupled thermomechanical model for the solidification of cast metals. Int. J. Solids Struct. 33 (5), 647–673. Available from. doi:10.1016/0020-7683(95)00056-G
Cheng, J., and Zhang, L. T. (2019). Simulation of vessel tissue remodeling with residual stress: an application to in-stent restenosis. Int. J. Smart Nano Mater. 10, 11–27. issn 1947542X. Available from. doi:10.1080/19475411.2018.1529002
Chester, M., Seedorf, G., Tourneux, P., Gien, J., Tseng, N., Grover, T., et al. (2011). Cinaciguat, a soluble guanylate cyclase activator, augments cGMP after oxidative stress and causes pulmonary vasodilation in neonatal pulmonary hypertension. Am. J. Physiology - Lung Cell. Mol. Physiology 301, L755–L764. doi:10.1152/AJPLUNG.00138.2010
Debevec, T., Millet, G. P., and Pialoux, V. (2017). Hypoxia-induced oxidative stress modulation with physical activity. Front. Physiology 8, 84. issn 1664042X. Available from. doi:10.3389/FPHYS.2017.00084
Ding, H., Luo, Y., Hu, K., Huang, H., Liu, P., Xiong, M., et al. (2020). Hypoxia in utero increases the risk of pulmonary hypertension in rat offspring and is associated with vasopressin type-2 receptor upregulation. Mol. Med. Rep. 22, 4173–4182. issn 17913004. Available from. doi:10.3892/MMR.2020.11533
Dodson, R. B., Rozance, P. J., Fleenor, B. S., Petrash, C. C., Shoemaker, L. G., Hunter, K. S., et al. (2013a). Increased arterial stiffness and extracellular matrix reorganization in intrauterine growth-restricted fetal sheep. Pediatr. Res. 73, 147–154. doi:10.1038/PR.2012.156
Dodson, R. B., Rozance, P. J., Reina-Romo, E., Ferguson, V. L., and Hunter, K. S. (2013b). Hyperelastic remodeling in the intrauterine growth restricted (IUGR) carotid artery in the near-term fetus. J. biomechanics 46, 956–963. issn 00219290. Available from. doi:10.1016/J.JBIOMECH.2012.12.013
Dwivedi, K.Kr, Lakhani, P., Kumar, S., and Kumar, N. (2022). A hyperelastic model to capture the mechanical behaviour and histological aspects of the soft tissues. J. Mech. Behav. Biomed. Mater. 126, 105013. Available from. doi:10.1016/j.jmbbm.2021.105013
Ebrahimi, A. P. (2009). Mechanical properties of normal and diseased cerebrovascular system. J. Vasc. Interventional Neurology 2, 155–162. doi:10.5281/zenodo.10319619
Eskild, A., Strøm-Roum, , Marie, E., and Haavaldsen, C. (2016). Does the biological response to fetal hypoxia involve angiogenesis, placental enlargement and preeclampsia? Paediatr. Perinat. Epidemiol. 30, 305–309. issn 13653016. Available from. doi:10.1111/PPE.12283
Farías, J. G., Zepeda, A. B., and Calaf, G. M. (2012). Melatonin protects the heart, lungs and kidneys from oxidative stress under intermittent hypobaric hypoxia in rats. Biol. Res. 45, 81–85. issn 0716-9760. Available from. doi:10.4067/S0716-97602012000100011
Figueroa, E. G., Gonzaléz-Candia, A., Villanueva, C. A., Ebensperger, G., Reyes, R. V., Llanos, A. J., et al. (2021). Beneficial effects of melatonin on prostanoids pathways in pulmonary hypertensive neonates. Vasc. Pharmacol. 138, 106853. issn 1537-1891. Available from. doi:10.1016/J.VPH.2021.106853
Fonck, E., Prod'hom, G., Roy, S., Augsburger, L., Rüfenacht, D. A., and Stergiopulos, N. (2007). Effect of elastin degradation on carotid wall mechanics as assessed by a constituent-based biomechanical model. Am. J. Physiology - Heart Circulatory Physiology 292, H2754–H2763. issn 03636135. Available from. doi:10.1152/ajpheart.01108.2006
Forsblad-D’Elia, H., Law, L., Bengtsson, K., Smeds, J., Ketonen, M., Sundström, B., et al. (2021). Biomechanical properties of common carotid arteries assessed by circumferential 2D strain and β stiffness index in patients with ankylosing spondylitis. J. rheumatology 48, 352–360. issn 1499-2752. Available from. doi:10.3899/JRHEUM.200146
García-Herrera, C. M., Bustos, C. A., Celentano, D. J., and Ortega, R. (2016). Mechanical analysis of the ring opening test applied to human ascending aortas. vol. 5842, no. May. Available from doi:10.1080/10255842.2016.1183125
García-Herrera, C., Atienza, J. M., Rojo, F. J., Claes, E., Guinea, G. V., Celentano, D. J., et al. (2012). Mechanical behaviour and rupture of normal and pathological human ascending aortic wall. Med. Biol. Eng. Comput. 50 (6), 559–566. doi:10.1007/s11517-012-0876-x
Gasser, T., Christian, O, Ray, W., and Holzapfel, G. A. (2006). Hyperelastic modelling of arterial layers with distributed collagen fibre orientations. J. R. Soc. Interface 3 (6), 15–35. issn 17425662. Available from. doi:10.1098/rsif.2005.0073
Geuzaine, C., and Remacle, J. F. (2009). Gmsh: a 3-D finite element mesh generator with built-in pre- and post-processing facilities. Int. J. Numer. Methods Eng. 79, 1309–1331. issn 00295981. Available from. doi:10.1002/NME.2579
Giussani, D. A., and Davidge, S. T. (2013). Developmental programming of cardiovascular disease by prenatal hypoxia. J. Dev. Orig. health Dis. 4, 328–337. issn 2040-1752. Available from. doi:10.1017/S204017441300010X
Gkousioudi, A., Yu, X., Ferruzzi, J., Qian, J., Wainford, R. D., Seta, F., et al. (2022). Biomechanical properties of mouse carotid arteries with diet-induced metabolic syndrome and aging. Front. Bioeng. Biotechnol. 10, 862996. issn 22964185. Available from. doi:10.3389/FBIOE.2022.862996
Gokcen, T., Inci, K., Inci, E. E., Sevgen, O., and Serdar, U. (2022). Allopurinol treatment reduced vascular remodeling and improved vascular functions in monocrotaline-induced pulmonary hypertensive rats. Pulm. Pharmacol. Ther. 77, 102166. issn 15229629. Available from. doi:10.1016/j.pupt.2022.102166
Gonzalez-Candia, A., Veliz, M., Carrasco-Pozo, C., Castillo, R. L., Cárdenas, J. C., Ebensperger, G., et al. (2019). Antenatal melatonin modulates an enhanced antioxidant/pro-oxidant ratio in pulmonary hypertensive newborn sheep. Redox Biol. 22, 101128. Available from. doi:10.1016/j.redox.2019.101128
Greenwald, S. E., Moore, J. E., Rachev, A., Kane, T. P. C., and Meister, J. J. (1997). Experimental investigation of the distribution of residual strains in the artery wall. J. Biomechanical Eng. 119, 438–444. doi:10.1115/1.2798291
Gundiah, N., Ratcliffe, M. B., and Pruitt, L. A. (2009). The biomechanics of arterial elastin. J. Mech. Behav. Biomed. Mater. 2, 288–296. issn 17516161. Available from. doi:10.1016/j.jmbbm.2008.10.007
Guo, X., Gong, C., Zhai, Y., Yu, H., Li, J., Sun, H., et al. (2023). Biomechanical characterization of normal and pathological human ascending aortic tissues via biaxial testing Experiment, constitutive modeling and finite element analysis. Comput. Biol. Med. 166, 107561. Available from. doi:10.1016/J.COMPBIOMED.2023.107561
Haskett, D., Johnson, G., Zhou, A., Utzinger, U., and Vande Geest, J. (2010). Microstructural and biomechanical alterations of the human aorta as a function of age and location. Biomechanics Model. Mechanobiol. 9, 725–736. doi:10.1007/S10237-010-0209-7
Herrera, E. A., Riquelme, R. A., Ebensperger, G., Reyes, R. V., Ulloa, C. E., Cabello, G., et al. (2010). Long-term exposure to high-altitude chronic hypoxia during gestation induces neonatal pulmonary hypertension at sea level. Am. J. Physiol. Regul. Integr. Comp. Physiol. 299, 1676–1684. Available from. doi:10.1152/ajpregu.00123.2010
Hirata, K., Yaginuma, T., O’Rourke, M. F., and Kawakami, M. (2006). Age-related changes in carotid artery flow and pressure pulses: possible implications for cerebral microvascular disease. Stroke 37, 2552–2556. issn 1524-4628. Available from. doi:10.1161/01.STR.0000242289.20381.F4
Holzapfel, G. A., and Ogden, R. W. (2018). Biomechanical relevance of the microstructure in artery walls with a focus on passive and active components. Am. J. physiology. Heart circulatory physiology 315, H540–H549. doi:10.1152/AJPHEART.00117.2018
Huh, U., Lee, C. W., You, J. H., Song, C. H., Lee, C. S., and Ryu, D. M. (2019). Determination of the material parameters in the holzapfel-gasser-ogden constitutive model for simulation of age-dependent material nonlinear behavior for aortic wall tissue under uniaxial tension. Appl. Sci. 9, 2851. doi:10.3390/APP9142851
Humphrey, J. D. (2021). Constrained mixture models of soft tissue growth and remodeling – twenty years after. J. Elast. 145, 49–75. issn 15732681. Available from. doi:10.1007/S10659-020-09809-1
Hung, M. W., Yeung, H., Lau, C., Poon, A., Tipoe, G., and Fung, M. (2017). Melatonin attenuates pulmonary hypertension in chronically hypoxic rats. Int. J. Mol. Sci. 18, 1125. doi:10.3390/IJMS18061125
Hussain, A., Bennett, R. T., Tahir, Z., Isaac, E., Chaudhry, M. A., Qadri, S. S., et al. (2019). Differential effects of atrial and brain natriuretic peptides on human pulmonary artery: an in vitro study. World J. Cardiol. 11, 236–243. issn 1949-8462. Available from. doi:10.4330/WJC.V11.I10.236
Hutter, D., Kingdom, J., and Jaeggi, E. (2010). Causes and mechanisms of intrauterine hypoxia and its impact on the fetal cardiovascular system: a review. Int. J. Pediatr. 2010, 1–9. doi:10.1155/2010/401323
Kamenskiy, A. V., Pipinos, I. I., Carson, J. S., MacTaggart, J. N., and Baxter, B. T. (2015). Age and disease-related geometric and structural remodeling of the carotid artery. J. Vasc. Surg. 62, 1521–1528. issn 0741-5214. Available from. doi:10.1016/J.JVS.2014.10.041
Kawagoe, Y., Green, L., White, S., and Richardson, B. (1999). Intermittent umbilical cord occlusion in the ovine fetus near term: effects on behavioral state activity. Am. J. obstetrics Gynecol. 181, 1520–1529. issn 0002-9378. Available from. doi:10.1016/S0002-9378(99)70399-6
Kim, Su A., Park, S. M., Kim, M. N., Kim, Y. H., Cho, D. H., Ahn, C. M., et al. (2012). The relationship between mechanical properties of carotid artery and coronary artery disease. Eur. J. Echocardiogr. 13, 568–573. doi:10.1093/EJECHOCARD/JER259
Klemetti, M. M., Teramo, K., Kautiainen, H., Wasenius, N., Eriksson, J. G., and Laine, M. K. (2021). Late-pregnancy fetal hypoxia is associated with altered glucose metabolism and adiposity in young adult offspring of women with type 1 diabetes. Front. Endocrinol. 12, 1. issn 16642392. Available from. doi:10.3389/fendo.2021.738570
Kochová, P., Kuncová, J., Švíglerová, J., Cimrman, R., Miklíková, M., Liška, V., et al. (2012). The contribution of vascular smooth muscle, elastin and collagen on the passive mechanics of porcine carotid arteries. Physiol. Meas. 33, 1335–1351. issn 1361-6579. Available from. doi:10.1088/0967-3334/33/8/1335
Kucukbas, G. N., and Doğan, Y. (2023). Evaluation of carotid artery Doppler measurements in late-onset fetal growth restriction: a cross-sectional study. J. Surg. Med. 7, 673–677. issn 2602-2079. Available from. doi:10.28982/JOSAM.7953
Lanne, T., Hansen, F., Mangell, P., and Sonesson, B. (1994). Differences in mechanical properties of the common carotid artery and abdominal aorta in healthy males. J. Vasc. Surg. 20, 218–225. issn 0741-5214. Available from. doi:10.1016/0741-5214(94)90009-4
Lasheras, J. C. (2007). The biomechanics of arterial aneurysms. Annu. Rev. Fluid Mech. 39, 293–319. isbn 0824307399. issn 00664189. Available from. doi:10.1146/annurev.fluid.39.050905.110128
Laubrie, J. D., Bezmalinovic, A., García-Herrera, C. M., Celentano, D. J., Herrera, E. A., Avril, S., et al. (2023). Hyperelastic and damage properties of the hypoxic aorta treated with Cinaciguat. J. Biomechanics 147, 111457. issn 18732380. Available from. doi:10.1016/j.jbiomech.2023.111457
Lee, C.-W., Huh, U., You, J.-H., Lee, C.-S., Kim, K.-H., Song, C.-H., et al. (2018). Computational evaluation for age-dependent material nonlinear behavior of aortic wall tissue on abdominal aortic aneurysms. Appl. Sci. 9, 101. doi:10.3390/APP9010101
Liu-Shiu-Cheong, , Patrick, S. K., Weir-McCall, J. R., Houston, J. G., and Struthers, A. D. (2020). Allopurinol in patients with pulmonary hypertension associated with chronic lung disease. Int. J. Chronic Obstr. Pulm. Dis. 15, 2015–2024. issn 11782005. Available from. doi:10.2147/COPD.S260917
Maarman, G. J., and Lecour, S. (2021). Melatonin against pulmonary arterial hypertension: is it ready for testing in patients? Cardiovasc. J. Afr. 32, 57–58. issn 16800745. Available from. doi:10.5830/CVJA-2021-008
Moghadasi, K., Ghayesh, M. H., Hu, E., and Li, J. (2024). Nonlinear biomechanics of diseased carotid arteries. Int. J. Eng. Sci. 199, 104070. Available from. doi:10.1016/J.IJENGSCI.2024.104070
Mohamed, S. F., Khayeka-Wandabwa, C., Muthuri, S., Ngomi, N., Kyobutungi, C., and Haregu, T. (2023). Carotid intima media thickness (CIMT) in adults in the AWI-Gen Nairobi site study: profiles and predictors. Hipertens. Riesgo Vasc. vol. 40, no. 1, pp. 5–15. doi:10.1016/j.hipert.2022.08.001
Murtada, S. I., Kawamura, Y., Weiss, D., and Humphrey, J. (2021). Differential biomechanical responses of elastic and muscular arteries to angiotensin II-induced hypertension. J. Biomechanics 119, 110297. issn 18732380. Available from. doi:10.1016/j.jbiomech.2021.110297
Navarrete, A., Chen, Z., Aranda, P., Poblete, D., Utrera, A., García-Herrera, C. M., et al. (2020). Study of the effect of treatment with atrial natriuretic peptide (ANP) and cinaciguat in chronic hypoxic neonatal lambs on residual strain and microstructure of the arteries. Front. Bioeng. Biotechnol. 8, 590488. issn 22964185. Available from. doi:10.3389/fbioe.2020.590488
Navarrete, A., Inostroza, M., Utrera, A., Bezmalinovic, A., González-Candia, A., Rivera, E., et al. (2024). Biomechanical effects of hemin and sildenafil treatments on the aortic wall of chronic-hypoxic lambs. Front. Bioeng. Biotechnol. 12, 1406214. doi:10.3389/FBIOE.2024.1406214
Nelson, R. J., and Drazen, D. L. (2000). Melatonin mediates seasonal changes in immune function. Ann. N. Y. Acad. Sci. 917, 404–415. issn 00778923. Available from. doi:10.1111/J.1749-6632.2000.TB05405.X
Olcese, J. M. (2020). Melatonin and female reproduction: an expanding universe. Front. Endocrinol. 11, 85. issn 16642392. Available from. doi:10.3389/fendo.2020.00085
Olver, T. D., Klakotskaia, D., Ferguson, B. S., Hiemstra, J. A., Schachtman, T. R., Laughlin, M. H., et al. (2016). Carotid artery vascular mechanics serve as biomarkers of cognitive dysfunction in aortic-banded miniature swine that can Be treated with an exercise intervention. J. Am. Heart Assoc. 5 (issn), 2047–9980. Available from. doi:10.1161/JAHA.116.003248
Pagoulatou, S. Z., Ferraro, M., Trachet, B., Bikia, V., Rovas, G., Crowe, L. A., et al. (2021). The effect of the elongation of the proximal aorta on the estimation of the aortic wall distensibility. Biomechanics Model. Mechanobiol. 20, 107–119. issn 16177940. Available from. doi:10.1007/s10237-020-01371-y
Pandi-Perumal, S. R., Trakht, I., Srinivasan, V., Spence, D., Maestroni, G., Zisapel, N., et al. (2008). Physiological effects of melatonin: role of melatonin receptors and signal transduction pathways. Prog. Neurobiol. 85, 335–353. issn 0301-0082. Available from. doi:10.1016/J.PNEUROBIO.2008.04.001
Papamatheakis, D. G., Blood, A., Kim, J., and Wilson, S. (2013). Antenatal hypoxia and pulmonary vascular function and remodeling. Curr. Vasc. Pharmacol. 11, 616–640. issn 15701611. Available from. doi:10.2174/1570161111311050006
Parraguez, V. H., Atlagich, M., Díaz, R., Bruzzone, M. E., Behn, C., and Raggi, L. A. (2005). Effect of hypobaric hypoxia on lamb intrauterine growth: comparison between high- and low-altitude native ewes. Reproduction, Fertil. Dev. 17, 497–505. issn 1448-5990. Available from. doi:10.1071/RD04060
Patterson, A. J., and Zhang, L. (2010). Hypoxia and fetal heart development. Curr. Mol. Med. 10, 653–666. issn 15665240. Available from. doi:10.2174/156652410792630643
Paz, A. A., Arenas, G. A., Castillo-Galán, S., Peñaloza, E., Cáceres-Rojas, G., Suazo, J., et al. (2019). Premature vascular aging in Guinea pigs affected by fetal growth restriction. Int. J. Mol. Sci. 20, 3474. issn 14220067. Available from. doi:10.3390/IJMS20143474
Peace, A., Van Mil, A., Jones, H., and Thijssen, D. H. (2018). Similarities and differences between carotid artery and coronary artery function. Curr. Cardiol. Rev. 14, 254–263. issn 1573403X. Available from. doi:10.2174/1573403X14666180910125638
Pukaluk, A., Sommer, G., and Holzapfel, G. A. (2024). Multimodal experimental studies of the passive mechanical behavior of human aortas: current approaches and future directions. Acta Biomater. 178, 1–12. issn 1742-7061. Available from. doi:10.1016/J.ACTBIO.2024.02.026
Ream, M., Ray, A. M., Chandra, R., and Chikaraishi, D. M. (2008). Early fetal hypoxia leads to growth restriction and myocardial thinning. Am. J. Physiology - Regul. Integr. Comp. Physiology 295, R583–R595. issn 03636119. Available from. doi:10.1152/AJPREGU.00771.2007
Rivera, E., Canales, C., Pacheco, M., García-Herrera, C., Macías, D., Celentano, D. J., et al. (2021). Biomechanical characterization of the passive response of the thoracic aorta in chronic hypoxic newborn lambs using an evolutionary strategy. Sci. Rep. 11 (1), 13875–13911. isbn 0123456789. issn 20452322. Available from. doi:10.1038/s41598-021-93267-9
Rivera, E., García-Herrera, C., González-Candia, A., Celentano, D. J., and Herrera, E. A. (2020). Effects of melatonin on the passive mechanical response of arteries in chronic hypoxic newborn lambs. J. Mech. Behav. Biomed. Mater. 112, 104013. January. issn 18780180. Available from. doi:10.1016/j.jmbbm.2020.104013
Rueda-Clausen, C. F., Morton, J. S., and Davidge, S. T. (2009). Effects of hypoxia-induced intrauterine growth restriction on cardiopulmonary structure and function during adulthood. Cardiovasc. Res. 81, 713–722. issn 0008-6363. Available from. doi:10.1093/CVR/CVN341
Saw, S. N., Tay, J. J. H., Poh, Y. W., Yang, L., Tan, W. C., Tan, L. K., et al. (2018). Altered placental chorionic arterial biomechanical properties during intrauterine growth restriction. Sci. Rep. 8, 16526–16612. issn 2045-2322. Available from. doi:10.1038/s41598-018-34834-5
Schneider, C. A., Rasband, W. S., and Eliceiri, K. W. (2012). NIH Image to ImageJ: 25 years of image analysis. Nat. Methods 9, 671–675. Available from. doi:10.1038/nmeth.2089
Sehgal, A., Murthi, P., and Dahlstrom, J. E. (2019). Vascular changes in fetal growth restriction: clinical relevance and future therapeutics. J. perinatology official J. Calif. Perinat. Assoc. 39, 366–374. issn 1476-5543. Available from. doi:10.1038/S41372-018-0287-4
Sethi, D., Gofur, E. M., and Munakomi, S. (2023). Anatomy, head and neck: carotid arteries. StatPearls. Available online at: https://www.ncbi.nlm.nih.gov/books/NBK545238/.
Sherman, S. R., Lefferts, W. K., Lefferts, E. C., Grigoriadis, G., Lima, N. S., Fernhall, B., et al. (2022). The effect of aging on carotid artery wall mechanics during maximal resistance exercise. Eur. J. Appl. Physiology 122, 2477–2488. issn 14396327. Available from. doi:10.1007/S00421-022-05016-Z
Sigaeva, T., Destrade, M., Martino, D. I., and Elena, S. (2019). Multi-sector approximation method for arteries: the residual stresses of circumferential rings with non-trivial openings. J. R. Soc. Interface 16 (156), 20190023. isbn 0000000167. issn 17425662. Available from. doi:10.1098/rsif.2019.0023
Skilton, M. R., Boussel, L., Bonnet, F., Bernard, S., Douek, P. C., Moulin, P., et al. (2011). Carotid intima–media and adventitial thickening: comparison of new and established ultrasound and magnetic resonance imaging techniques. Atherosclerosis 215 (2), 405–410. issn 0021-9150. Available from. doi:10.1016/j.atherosclerosis.2010.12.036
Steinhorn, R. H. (2017). Persistent pulmonary hypertension of the newborn. Fetal Neonatal Brain Inj., 566–582. Available from. doi:10.1017/9781316275498.037
Tong, J., Xin, Y. F., Zhang, Z., Xu, X., and Li, T. (2023). Effect of hypertension on the delamination and tensile strength of ascending thoracic aortic aneurysm with a focus on right lateral region. J. Biomechanics 154, 111615. issn 18732380. Available from. doi:10.1016/j.jbiomech.2023.111615
Torres, F., González-Candia, A., Montt, C., Ebensperger, G., Chubretovic, M., Serón-Ferré, M., et al. (2015). Melatonin reduces oxidative stress and improves vascular function in pulmonary hypertensive newborn sheep. J. Pineal Res. 58, 362–373. issn 1600079X. Available from. doi:10.1111/JPI.12222
Walsh, M. T., Cunnane, E., Mulvihill, J., Akyildiz, A., Gijsen, F., and Holzapfel, G. (2014). Uniaxial tensile testing approaches for characterisation of atherosclerotic plaques. J. Biomechanics 47, 793–804. issn 18732380. Available from. doi:10.1016/j.jbiomech.2014.01.017
Werner, F., Kojonazarov, B., Gaßner, B., Abeßer, M., Schuh, K., Völker, K., et al. (2016). Endothelial actions of atrial natriuretic peptide prevent pulmonary hypertension in mice. Basic Res. Cardiol. 111, 22–16. issn 14351803. Available from. doi:10.1007/S00395-016-0541-X
Wiedemann, R., Ghofrani, H., Weissmann, N., Schermuly, R., Quanz, K., Grimminger, F., et al. (2001). Atrial natriuretic peptide in severe primary and nonprimary pulmonary hypertension: response to iloprost inhalation. J. Am. Coll. Cardiol. 38, 1130–1136. issn 07351097. Available from. doi:10.1016/S0735-1097(01)01490-5
Xu, X., Liu, X., Ma, S., Xu, Y., Xu, Y., Guo, X., et al. (2018). Association of melatonin production with seasonal changes, low temperature, and immuno-responses in hamsters. Mol. A J. Synthetic Chem. Nat. Prod. Chem. 23, 703. issn 14203049. Available from. doi:10.3390/MOLECULES23030703
Yildiz, M., and Balcioğlu, S. A. (2024). Current position and future perspectives of melatonin and its supplements in pulmonary hypertension. Koşuyolu Heart J. 27, 37–39. doi:10.51645/KHJ.2024.429
Yosibash, Z., and Priel, E. (2011). P-FEMs for hyperelastic anisotropic nearly incompressible materials under finite deformations with applications to arteries simulation. Int. J. Numer. METHODS Eng. Int. J. Numer. Meth. Engng. 88, 1152–1174. Available from. doi:10.1002/nme.3213
Zahn, A., and Balzani, D. (2018). A combined growth and remodeling framework for the approximation of residual stresses in arterial walls. ZAMM Z. fur Angew. Math. Mech. 98, 2072–2100. issn 15214001. Available from. doi:10.1002/ZAMM.201700273
Keywords: biomechanics, carotid artery, hypobaric hypoxia, melatonin, biomechanical tests, finite element method
Citation: Rivera E, Navarrete A, Garcia-Herrera CM, Gordillo L, Cerda E, Celentano DJ, Gonzalez-Candia A and Herrera EA (2025) Biomechanical and histomorphometric characterization of the melatonin treatment effect in the carotid artery subjected to hypobaric hypoxia. Front. Bioeng. Biotechnol. 13:1554004. doi: 10.3389/fbioe.2025.1554004
Received: 31 December 2024; Accepted: 02 April 2025;
Published: 16 April 2025.
Edited by:
Yang Liu, Hong Kong Polytechnic University, Hong Kong SAR, ChinaReviewed by:
Mitchell C. Lock, The University of Manchester, United KingdomIryna Oliynyk, National Academy of Agrarian Sciences of Ukraine, Ukraine
Charmaine R. Rock, Monash University, Australia
Copyright © 2025 Rivera, Navarrete, Garcia-Herrera, Gordillo, Cerda, Celentano, Gonzalez-Candia and Herrera. This is an open-access article distributed under the terms of the Creative Commons Attribution License (CC BY). The use, distribution or reproduction in other forums is permitted, provided the original author(s) and the copyright owner(s) are credited and that the original publication in this journal is cited, in accordance with accepted academic practice. No use, distribution or reproduction is permitted which does not comply with these terms.
*Correspondence: Eugenio Rivera, ZXVnZW5pby5yaXZlcmFAdXNhY2guY2w=