- 1Department of Oral Medicine, Periodontology, and Diagnosis, Faculty of Dentistry, Suez Canal University, Ismailia, Egypt
- 2Department of Stem Cell and Regenerative Biotechnology, School of Advanced Biotechnology, Molecular & Cellular Reprogramming Center, Institute of Advanced Regenerative Science, and Institute of Health, Aging & Society, Konkuk University, Seoul, Republic of Korea
- 3Department of Basic and Clinical Medical Sciences, Faculty of Dentistry, Zarqa University, Zarqa, Jordan
- 4R&D Team, StemExOne Co., Ltd., Seoul, Republic of Korea
- 5Department of Basic Science, College of Science and Health Professions, King Saud bin Abdulaziz University for Health Sciences, Jeddah, Saudi Arabia
- 6National Guard- Health Affairs, King Abdullah International Medical Research Centre, Jeddah, Saudi Arabia
- 7Department of Biotechnology, Yeungnam University, Gyeongsan, Republic of Korea
- 8Molecular Therapeutics Program, Fox Chase Cancer Center, Temple University, Philadelphia, PA, United States
- 9Department of Biochemistry, Animal Health Research Institute, Cairo, Egypt
- 10Department of Substitutive Dental Sciences, College of Dentistry, Imam Abdulrahman Bin Faisal University, Dammam, Saudi Arabia
- 11College of Nursing, Prince Sattam bin Abdulaziz University, Al-Kharj, Saudi Arabia
- 12Center for Health Research, Northern Border University, Arar, Saudi Arabia
- 13Department of Natural Products and Alternative Medicine, Faculty of Pharmacy, University of Tabuk, Tabuk, Saudi Arabia
- 14Department of Basic Medical Sciences, College of Medicine, AlMaarefa University, Riyadh, Saudi Arabia
- 15Department of Physiology, Biochemistry, and Pharmacology, College of Veterinary Medicine, University of Kirkuk, Kirkuk, Iraq
- 16Department of Biomedical Sciences, Dubai Medical College for Girls, Dubai Medical University, Dubai, United Arab Emirates
- 17Department of Forensic Medicine and Toxicology, Faculty of Veterinary Medicine, Benha University, Toukh, Egypt
Urine-based therapy, an ancient practice, has been utilized across numerous civilizations to address a wide range of ailments. Urine was considered a priceless resource in numerous traditional therapeutic applications due to its reported medicinal capabilities. While the utilization of urine treatment is contentious and lacks significant support from modern healthcare, the discovery of urine-derived stem cells (UDSCs) has introduced a promising avenue for cell-based therapy. UDSCs offer a noninvasive and easily repeatable collection method, making them a practical and viable option for therapeutic applications. Research has shown that UDSCs contribute to organ preservation by promoting revascularization and decreasing inflammatory reactions in many diseases and conditions. This review will outline the contemporary status of UDSCs research and explore their potential applications in both fundamental science and medical practice.
1 Introduction
Regenerative medicine can restore damaged or incapacitated organs. Stem cells have made significant progress and are an increasingly popular approach in regenerative applications thanks to their tremendous multiplying ability and multi-differentiation potential (Augustine et al., 2021). Since all tissues include endogenous stem cells that are crucial for their equilibrium, they are usually recognized as a more accessible cell reservoir for use in therapy and remain an expanding and fascinating field with the promise to improve human healthcare (Liu et al., 2020a). Urine-derived stem cells (UDSCs) have developed as a potential cellular origin in the last decade due to their noninvasive acquiring process, robust proliferation capacity, and diverse medical applications and act as the starting point in order to convert them into disease-tailored induced pluripotent stem cells (IPSCs). UDSCs are now playing a significant part in adult stem cell biology (Barakat et al., 2020; Ji et al., 2017).
Sutherland and Bain announced the practical separation of live cells from urine utilizing specimens of newborns (Sutherland and Bain, 1972). Several teams repeated the process in subsequent years, and cells were taken from individuals with various illnesses. These urinary cells had a variety of topologies (polygonal or elongated), but the source was primarily epithelial (from the kidney tubules and urothelial) according to biomarker gene transcription (Kibschull et al., 2023; Zhuang et al., 2021; Sun et al., 2022b; Lone et al., 2022).
2 Extraction of UDSCs
As previously mentioned, Sutherland and Bain published the first research to describe the gathering of exfoliated urine cells in 1972. They obtained proliferating cells from the urine of four newborns under the age of 2 days (Sutherland and Bain, 1972). Zhang et al. identified renal progenitors in cultivated urine-derived cells from 15 healthy persons and eight patients with vesicoureteral reflux. There were around 2–7 progenitor-like cells per 100 mL of urine, capable of forming a homogeneous, concentrated colony from an individual cell in 2 weeks. These cells may develop in vitro for eight passages before differentiating into urothelial, smooth muscle, endothelial, and interstitial cells. Nevertheless, the fraction of cells that display stem/progenitor characteristics declined after every passage (Zhang et al., 2008).
Following gathering urine, it should be centrifuged to extract UDSCs, and then contaminated cells should be progressively eliminated. Urine cryopreservation from healthy young individuals is the most effective sample for extracting fresh UDSCs from samples taken from diseased persons, especially those suffering from diabetes (Zhao et al., 2018).
However, the most recent research demonstrates that they can be effectively obtained from these patients, but their regenerating capacity has been substantially decreased, making them unsuitable for treatment (Ouyang et al., 2019). After collecting the appropriate specimens, an antibiotic is applied to minimize contamination. After centrifuging, the remaining residue is eliminated, followed by two washes with phosphate buffer saline (PBS) and resuspension of the material. Because urine involves many types of cells, it is vital for identifying the different contents following extraction (Kim et al., 2020).
3 UDSCs culture
Separation of UDSC from fresh urine is more appropriate, but procedures for UDSC isolation could be accomplished within 24 h if urine is kept at 4°C in a storage medium with serum; prolonged preservation negatively affects the lifespan of cells (Lang et al., 2013). Investigations on urine storage for UDSCs separation are still limited, and it is a crucial subject to tackle as it would provide numerous benefits to the methodology of UDSCs separation (Zeng et al., 2022; Xie et al., 2023). Bharadwaj et al. reported an average of 3.7 × 108 UDSC cells at passage five after 27 days of culture. Nevertheless, amounts may vary depending on conditions (Bharadwaj et al., 2011). UDSCs may be separate from persons of both sexes, and the age range documented so far is 5–75 years (Kim et al., 2023). Telomerase activity in adult cells is mainly restricted to stem cells and is linked with strong proliferating ability. Bharadwaj et al. reported telomerase activity in 60% of the separate UDSC specimens (Bharadwaj et al., 2013), whereas standard genome sequencing was discovered at least until cell passage 15 (Kibschull et al., 2023).
Moreover, Chun et al. suggested implementing a 5% O2 hypoxic medium with collagen type I as a tailored technique for expanding collected UDSCs while preserving their chromosomal integrity and multipotent development capacity (Chun et al., 2016).
A new investigation found that flavonoids enriched with Matrigel. Flavonoids might improve UDSCs isolation, yield, colony-building capability, and differentiation capability (Kim et al., 2020). Nevertheless, to create a significant amount of developed cells before therapeutic administration, maximal growth of UDSCs is frequently necessary in vitro, resulting in cellular aging following several passages (Shi et al., 2022).
Liu et al. used undifferentiated UDSCs throughout the initial phases of in vitro passaging (≤p3) with a heparin hyaluronic-acid hydrogel (hp-HA) gel encapsulating growth factors. Following subcutaneous insertion in vivo, the cells demonstrated enhanced survival, integration, vascular, neuronal, and myogenic transformation capabilities (Liu et al., 2020b).
4 Properties of HUDSCs
Urine contains multiple cell types, generically categorized as UDSCs, containing numerous cellular groups and stem cells. UDSCs have MSC-like characteristics and possess the capacity to divide and multiply into diverse cell lines. The essential sources and particular mechanisms that underpin the formation and evacuation of UDSCs are yet unknown. In addition, they may be lost in urine due to the normal cycle of homeostatic tissue repair and renewal. Urine stem cells are proven to have several outstanding biological features (Figure 1) (Zhou et al., 2012).
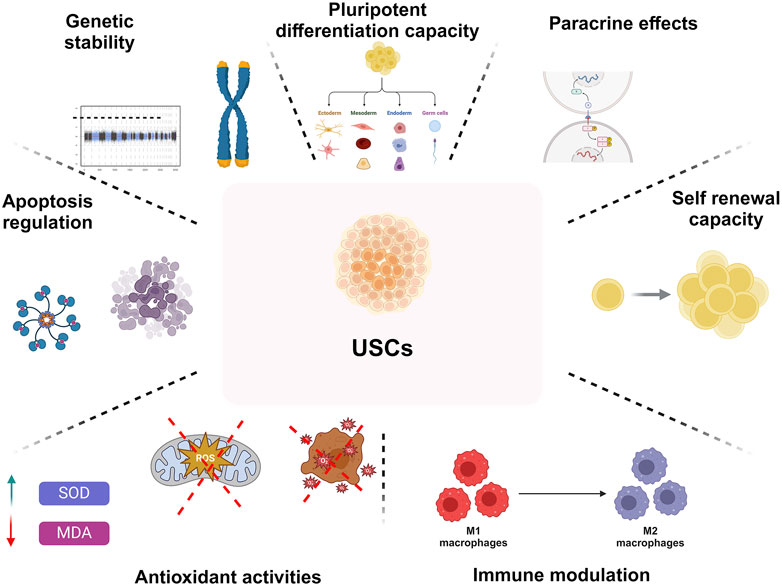
Figure 1. An overview on biological characteristics of UDSCs regrading pluripotent differentiation capacity, paracrine effects, self-renewing capacity, immune-modulating characteristics, antioxidant activities, modulation of apoptosis, and genetic stability.
4.1 Genomic durability
In vitro multiplication of UDSCs is required to generate a substantial number of cells for therapeutics because of their small quantity in human urine; however, this method enhances the danger of genetic changes and cellular mutations. Genetic integrity is an important safety issue for stem cell-based therapies since destabilization in the genome, including chromosomal changes, is linked to higher tumor formation (Wu et al., 2011). Telomere degradation during the proliferation of cells leads to cell death and mortality. Telomere length is critical for the continued or limitless multiplication of self-renewing cells. Cells’ telomerase transcription (low or undetectable) should be evaluated to exclude the potential of tumorigenicity. UDSCs’ powerful dividing capability is linked to their lengthy telomeres. Surprisingly, specific UDSCs clones exhibited apparent amounts of telomerase activity during the initial stages, and their dividing capacity was greater than that of telomerase-negative cells (Shi et al., 2022). Nonetheless, telomerase activity gradually dropped throughout cellular subculture. As a result, notwithstanding identifiable telomerase activity within specific UDSCs clones, in vivo investigations show that USCs did not become tumorigenic upon growth.
4.2 Differentiation capacity
Autologous somatic stem cells provide a unique benefit for upcoming therapeutic uses since they seldom cause immunological rejection (Jha et al., 2024; Selvido et al., 2023). Furthermore, the capacity to grow rapidly and be driven into multiple cell lineages provides a foundation for stem cell therapies. UDSCs may be grown to produce a vast population, and their adaptability has been extensively established via research (Figure 2; Table 1) (Shi et al., 2016). Initially, UDSCs were cultivated from newborn infants and showed low proliferation capacity (Sutherland and Bain, 1972). In 2008, urological tissue regeneration researchers effectively generated urine cells with remarkable division capacity (Zhang et al., 2008). Once extracted, UDSCs may be grown in vitro and divided into various cellular kinds by inducing lineage-specific differentiation under the right circumstances. UDSCs enhance PDLSC division, osteogenic and cementogenic transformation, and regrowth of fresh tissues in vivo in a ratio-dependent fashion via noncontact coculture. These findings point to its potential application as a unique strategy for clinical periodontal regeneration (Yang et al., 2020). Human urine-induced pluripotent stem cells (hU-iPSCs)-derived epithelium layers developed into dental-like frameworks, exhibiting physical qualities similar to those exhibited by regular human teeth (Cai et al., 2013).
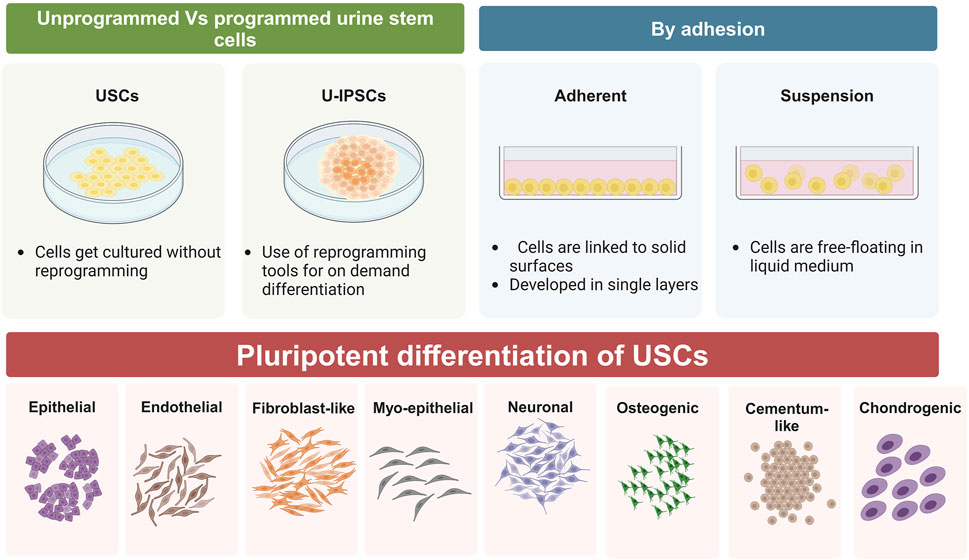
Figure 2. Schematic representation of differentiation capacity of UDSCs, whether they were used as programmed or unprogrammed cells, they have an outstanding tendency to form epithelial, endothelial, fibroblast-like, myo-epithelial, neurons-like, osteoblasts, cementoblasts, and chondrocytes.
When stimulated with proper media, UDSCs produce urothelial-specialized biomarkers, including uroplakin-III and cytokeratin. During the differentiation process, cell morphology changed dramatically, resulting in a cuboidal form (Wan et al., 2018). When grown in a myogenic media, UDSCs display muscular-associated biomarkers like myosin (Liu et al., 2013). Intradermal applications in nude mice and USCs with myogenic transformation could create an assortment of smooth muscle cells (Chen et al., 2017).
UDSCs can successfully transform into functional endothelial cells when incubated in an endothelial stimulating medium, exhibiting a cobblestone-like form, expressing endothelial biomarkers, forming tubules on Matrigel, and developing tight connections, analogous to natural endothelial cells (Liu et al., 2018).
Following osteogenic development, UDSCs can generate mineralized matrices. During osteogenic cell commitment, alkaline phosphatase activity and osteogenic biomarker transcription increase (Qin et al., 2014). UDSCs can alter shape to become a neuron-specific architecture with distinct neurogenic extensions (Wu et al., 2020). Additionally, the generated UDSCs exhibit neuronal biomarkers, including Nestin, and Sox2 (Sato et al., 2019).
4.3 Paracrine effects
UDSCs have substantial paracrine impacts and could discharge various paracrine substances, including regulatory molecules and exosomes. The UDSCs secretory system has sparked considerable curiosity in tissue repair (Xiang et al., 2020). Human USCs were proven to decrease inflammatory reactions and fibrosis via paracrine mechanisms. When cultivated alongside PDLSCs, UDSCs can promote the osteogenic development of PDLSCs via paracrine mechanisms. Transfected genes have been used to modulate UDSCs synthesis. UDSCs could express the VEGF gene, showing more enhanced angiogenesis. Transplanting UDSCs engineered with the FGF2 gene significantly restored vascularity via paracrine actions in rats. As a crucial paracrine mediator, extracellular vesicles (EVs) carry numerous bioactive cargos (Zidan et al., 2021). Investigations on animals have highlighted the excellent prospects of UDSC-derived EVs (UDSC-EVs). For example, in ischemic mice, USC-EVs significantly improved angiogenesis. Furthermore, UDSCs-EVs can improve tissue repair, which could be due to the microRNAs they contain. UDSCs-exosomes (UDSCs-EXOs) can effectively inhibit osteonecrosis by delivering peptides having proangiogenic and antiapoptotic properties (Wan et al., 2022). UDSCs-EVs are capable of treating osteoporosis in mice by delivering functional proteins. UDSCs-EXOs are also helpful in shielding tissues from injuries (Chen et al., 2020).
4.4 Self-renewal potential
UDSCs could be obtained from urine, producing significant cellular quantities from just one copy. These cells produce homogeneous kinds of cells and are excessively proliferating since they have more vigorous telomerase activity and more considerable telomere lengths than other forms of MSCs(Supakul et al., 2023). The majority of UDSCs obtained from young adults displayed telomerase activity (TA+) and maintained extended telomeres sizes Telomerase activities, while UDSCs-TA + dropped to 50%–60% of the UDSCs among individuals 50 years old and beyond (Shi et al., 2012). Regardless of multiple passes, TA+ and TA-USCs maintain standard karyotyping in the growing environment. They did not develop teratomas 3 months following implantation (Shi et al., 2012). About 100–140 UDSC clones can be acquired every 24 h from every person (Zhang and Atala, 2022).
4.5 Immune-modulating activities
Recent investigations have revealed that UDSCs implantation can minimize localized inflammation and expedite tissue regeneration by encouraging macrophage polarization toward the M2 phenotype (Sun et al., 2021a; Pei et al., 2023). There was no evident immunological rejection or cancer detected in these investigations, indicating that USCs are low immunogenic and safe for xenotransplantation (Cehakova et al., 2023; Liu et al., 2022).
Host exposure to periodontitis controls not only the shift from microbial symbiosis to dysbiosis but also the onset of inflammation and the advancement of irreversible tissue degradation (Darby, 2022). Periodontitis advancement and severity are influenced by host-related factors, including immunoregulatory dysfunction, immunodeficiency, and systemic diseases associated with periodontitis (Hernández et al., 2021). Deficiencies or dysfunction of the host’s defenses lead to a failure to control dysbiotic microbial populations and the associated disease (Deng et al., 2022). Silk fibroin (SF)/nanohydroxyapatite (nHA) composite loaded with less than 0.5% GO were biocompatible and enhanced UDSCs multiplication and bone formation. It could stimulate M2-type differentiation while inhibiting M1-type conversion of macrophages. It displayed the most substantial potential for boosting the M2-type polarization of macrophages and promoting osteoinduction in vivo (Sun et al., 2021a).
4.6 Antioxidant activity
Oxidative stress is a hazardous mechanism that can cause malignancies, cardiac illness, neurological problems, respiratory diseases, renal disease, chronic inflammation, and premature aging (Deng et al., 2021). Li et al. discovered that UDSCs-EXOs had antioxidant potential since they were able to enhance SOD activity and decrease MDA levels (Li et al., 2020). Comparably, Zhang et al. discovered higher concentrations of SOD-1 in mice treated with UDSCs (Zhang et al., 2020a). Furthermore, UDSCs have been shown to have an antioxidant impact by significantly lowering the levels of oxidative stress-associated markers (Li et al., 2017a).
4.7 Apoptosis regulation
Apoptosis is a type of planned cell mortality that occurs when separate apoptotic bodies arise to preserve equilibrium and manage the normal cell cycle. However, severe apoptosis might degrade tissue function further, leading to serious diseases. As a result, blocking apoptosis is viewed as saving damaged tissue (Xu et al., 2019).
Sun et al. discovered that UDSCs inhibited the formation of Bax and Caspase three while increasing the production of the antiapoptotic protein Bcl-2 (Sun et al., 2019). An in vivo study proved that therapy with UDSCs decreased apoptotic levels by significantly lowering caspase-3 and Bax levels while significantly boosting Bcl-2 levels, resulting in fewer apoptosis nuclei (Li et al., 2017a).
TIMP1 is a naturally occurring matrix metalloproteinase (MMP) inhibitor. The PI3K and JNK signaling mechanisms were activated by the recombinant human TIMP1 protein, which prevented apoptosis. TIMP1 was discovered to be exceptionally abundant in UDSC-EVs by Chen et al. and might improve the anti-apoptotic properties of osteoblasts and endothelial cells, therefore playing a protective function (Chen et al., 2020).
5 UDSCs vs. U- IPSCs
UDSCs were previously classed as cells with multipotent characteristics, indicating that they may transform into several varieties of cells within the same lineage. Furthermore, they are capable of being turned into induced pluripotent stem cells., which have greater effectiveness (Guan et al., 2014b). However, a new study discovered that empty urine includes many functional epithelial cells that may be well cultivated and transformed into IPSCs (Kibschull et al., 2023).
There are two fundamental methods for creating a significant amount of different cell numbers: the first is cellular reprogramming using UIPSCs, and the second is immediate reconfiguration from baseline UDSCs (Table 5). Cell reprogramming, which is commonly performed through the viral or non-viral administration of reprogramming reagents, transforms UDSCs into proliferative U-IPSCs that can then be transformed into numerous cellular kinds utilizing established procedures. While this approach is quite efficient for creating vast quantities of cells, it is not without risk because of the reprogramming reagents. U-IPSCs may divide into various kinds of cells; U-IPSCs frequently develop into specific cell types by employing specialized induction procedures and triggers that mimic physiological phases of development. Here are some instances of how U-IPSCs can differentiate (Liu et al., 2021).
In preclinical research, developed u-iPSC-derived renal cells were used as an option for regenerative therapies. U-IPSCs have also demonstrated their ability to turn into various cellular lineages. The usefulness and productivity of techniques for development can change according to cellular species and techniques employed (Mulder et al., 2020). Continuing studies are concentrated on improving differentiation strategies to boost the efficiency and usability of U-IPSCs for regenerative therapies and disease models. The direct technique includes changing critical transcriptional genes. It aims to turn UDSCs directly into targeted kinds of cells without crossing via the iPSC stage, possibly preserving lineage-specific data while addressing safety issues. Numerous investigations proved that UDSCs can be directly transformed into multiple cell lines (Liu et al., 2021).
It is essential to stress that direct reprogramming investigations are just starting, and further investigations are essential for properly comprehending the possible uses of this technique for therapeutic purposes.
6 Employment of UDSCs in pathological simulation
U-IPSCs have been employed in disease models for researching an extensive spectrum of human illnesses, allowing researchers to understand the molecular processes, pathophysiology, and prospective treatment methods to address diverse ailments (Figure 3) (Li et al., 2017b). Furthermore, U-IPSCs obtained from those with certain genetic conditions can develop into illness-related cellular populations to simulate the condition in vitro. This strategy has been utilized to investigate pathological conditions (Li et al., 2017b; Shi and Cheung, 2021).
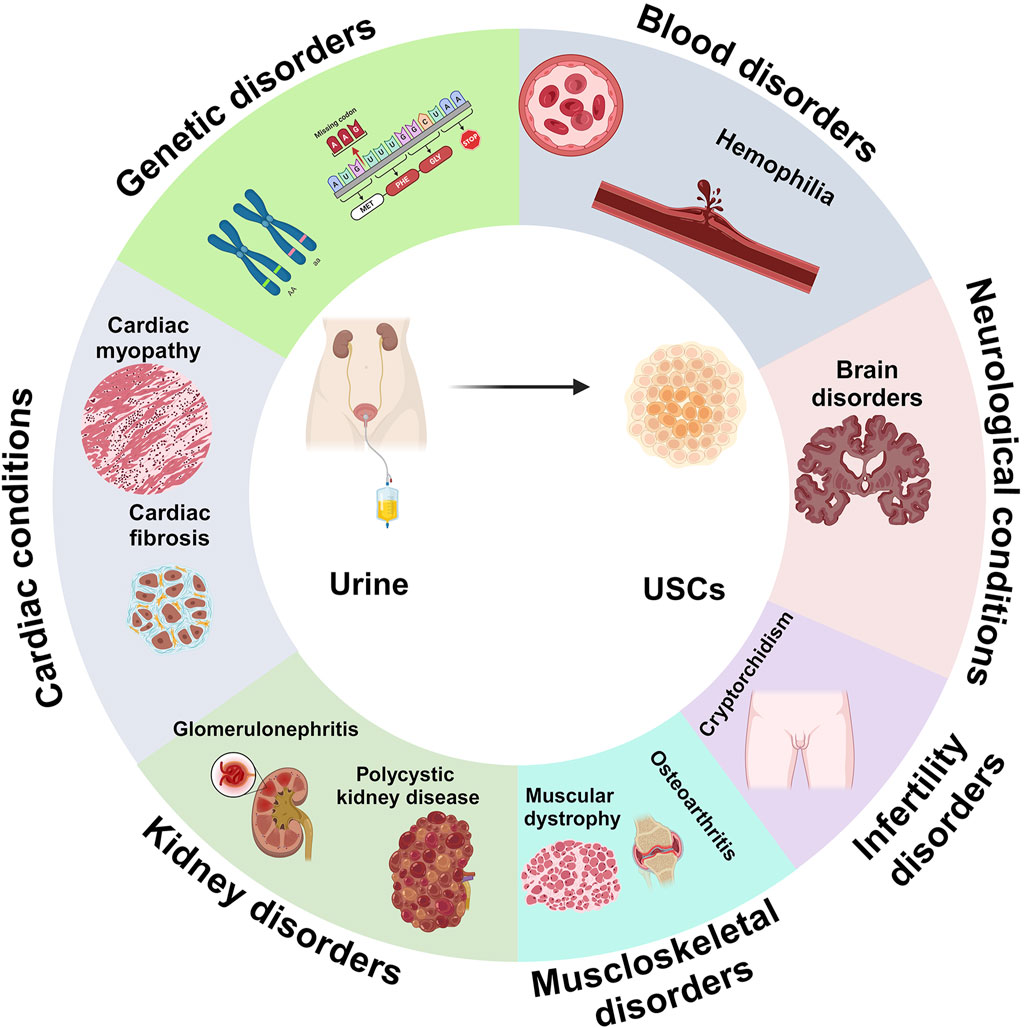
Figure 3. Adaptability of UDSCs as large-scale, ethical approach for disease modelling in numerous diseases and conditions, such as blood disorders, neurological conditions, infertility isorders, musculoskeletal disorders, kidney disorders, cadiac conditions, and genetic disorders.
Investigators can learn more about disease causes, evaluate possible medication candidates, and investigate personalized medicine techniques by examining u-iPSC-derived cells from afflicted patients. U-IPSCs have been employed to imitate several neurological illnesses; neurogenic transformation of U-IPSCs allows researchers to detect illness-specific cellular characteristics, evaluate disease development, and test novel treatments (Shi and Cheung, 2021). U-IPSCs have also been utilized to simulate cardiac illnesses (Huang et al., 2020). Differentiation of U-IPSCs into cardiomyocytes allows scientists to explore disease processes, assess aberrant cardiac cell function, and test prospective therapy techniques (Huang et al., 2020).
Furthermore, U-IPSCs have been used to imitate various nephrological conditions and acute renal damage. The transformation of U-IPSCs into kidney cellular lineages enables scientists to evaluate illness-specific cellular characteristics, track disease development, and test novel therapeutic approaches for kidney ailments (Bharadwaj et al., 2013).
Guo et al. obtained u-iPSC from a 5-year-old boy suffering from X-linked Alport Syndrome (X-LAS) and showed the practicality as a cellular-orchestrated illness model by validating the pluripotency capacity and proper karyotyping (Guo et al., 2020).
Hemophilia is a widespread inherited hemorrhaging disorder (Shah et al., 2023). Because of deficiencies in the way blood clots, those who have severe hemophilia could experience fatal episodes of bleeding during trauma or surgeries (Gualtierotti et al., 2024). The primary therapy is to replace the deficient factors (Koga et al., 2023). Lu et al. produced U-IPSCs from a Hemophilia A patient with an Inv22 mutation (Lu et al., 2020). The pathogenesis of neurological diseases is multifactorial, including intricate links between genetics and the environment that cannot be fully replicated in animal models (Yau et al., 2023).
Teles et al. developed human cerebral organoids from U-IPSCs obtained from Down syndrome (DS) patients, expressing evolutionary patterns of the early-stage forebrain (Teles e Silva et al., 2023). Musculoskeletal illnesses are the second most common cause of disability worldwide, inflicting a considerable cost on society (Giardullo et al., 2021). Autosomal dominant osteopetrosis type II (ADO2), a dominantly inherited musculoskeletal condition, causes fractures, joint discomfort, and bone morphological abnormalities (Lu et al., 2022). Ou et al. generated U-IPSCs from ADO2 patients and detected the identical CLCN7 polymorphism (R286W) in their specimens by juxtaposing them with ADO2-IPSCs (Ou et al., 2019).
Cryptorchidism is a frequent congenital condition in newborns, with the potential for infertility. Because human embryos are difficult to obtain for scientific research, in vitro models may be useful (Li et al., 2024). Zhou et al. used lentivirus to reprogram UDSCs from a cryptorchid patient, which co-cultured onto irradiated mouse embryonic fibroblasts and cultivated using human embryonic stem cells (ESCs) media. Their findings showed that these two cell lines resembled human ES cells in terms of morphology, biomarker transcription, and pluripotency-associated genes (Zhou et al., 2013).
7 Therapeutic and regenerative applications of UDSCs
Concerning the previously mentioned characteristics, UDSCs represent a promising option as a source for stem cells (Table 2). With the utilization of proper scaffolding material, the administration of UDSCs could be a promising therapeutic modality in addressing periodontal defects (Yang et al., 2020; Xing et al., 2022) (Figure 4).
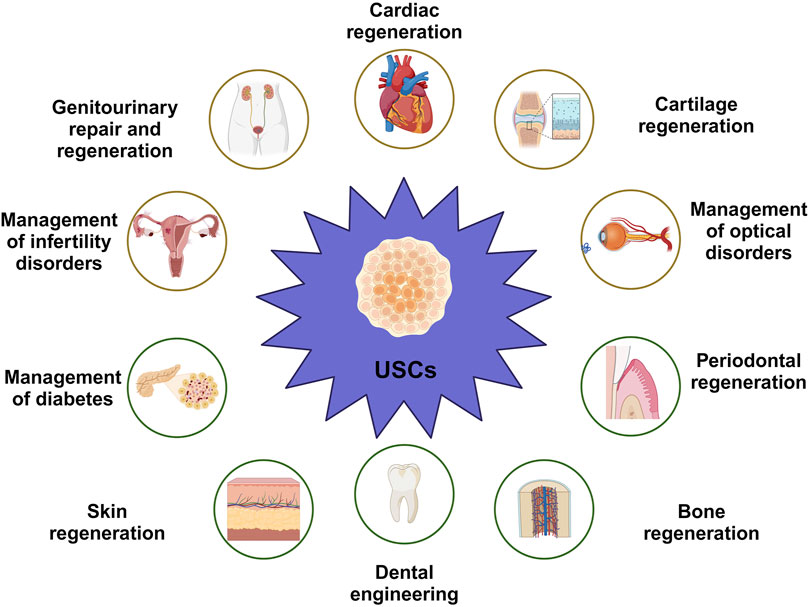
Figure 4. Versatility of UDSCs as economic, non-invasive therapeutic and regenerative approach in numerous applications, such as cartilage regeneration, management of cardiac conditions, management of optical disorders, periodontal regeneration, dental engineering, skin regeneration, management of diabetes, management of infertility disorders in both of males and females, and genitourinary repair and regeneration.
7.1 Genitourinary repair and regeneration
UDSCs, which originate in the urinary tract system, are an excellent alternative for repairing genitourinary organs (Table 3) (Liu et al., 2017). Application UDSCs have shown tremendous potential for treating acute kidney injury (AKI). For example, UDSCs or UDSCs-Exos can reduce cell death, control inflammatory reactions, and enhance renal functionality (Tian et al., 2017). UDSCs-Exos can protect kidney cell function during hypoxia/reoxygenation in vitro by transferring functional microRNAs (Zhang et al., 2020c). UDSCs could reduce fibrosis in the kidneys and inflammatory conditions and protect kidneys from damage through paracrine effects in rats (Xiong et al., 2020).
Bladder regeneration has been viewed as a viable approach to restoring functionality in individuals suffering from severe bladder disorders, including congenital abnormalities and malignancies (Sharma et al., 2013). Intravenous injection of UDSCs dramatically reduced oxidative stress, inflammatory reactions, and cell death in bladder tissues in mice. USCs can be accessible using a straightforward, noninvasive, and low-cost technique that does not need surgical operations. This finding might pave the way for a potential clinical investigation to evaluate the therapeutic effectiveness of USC in treating interstitial cystitis. (Li et al., 2017a).
Urethral defects are often treated with reconstructive surgery; however, this remains a considerable difficulty due to a variety of postoperative consequences (Hua et al., 2023). Because of the shortage of autogenic grafts for urethral repair, there has been much enthusiasm for bioengineered urethras. Given their multipotent capacity, UDSCs offer significant potential for urethral regeneration (Casarin et al., 2022).
UDSCs-loaded SIS scaffolds were found to promote urethral reconstruction, as demonstrated by substantial increases in urethral diameter, rejuvenation velocity, muscle content, and vascularization. There are several restrictions. First, scientists did not examine the bioactive substances produced by USCs that are thought to improve tissue regeneration. These include growth factors and cytokines. Second, in the current study, urethral defect models were produced in normal animals, that can’t adequately imitate the clinical state of urethral strictures, which are distinguished by a fibrotic urethral bed. Finally, just a few test subjects were examined at each time point. Additional studies are required to transfer such technology into the clinic (Liu et al., 2017).
7.2 Cartilage regeneration
Due to cartilage’s weak intrinsic healing capabilities, stem cells have sparked interest in articular cartilage repair. Autogenic stem cells are the golden standard in tissue engineering; however, most conventional stem cells need invasive and complex steps to use (Zha et al., 2021). UDSCs are an innovative stem cell reservoir that may be obtained non-invasively and repeatedly from the same person (Table 4).
Chen et al. initially verified the potential of UDSCs for cartilage healing in 2018 (Chen et al., 2018). They found that induced hUDSCs produced chondrogenic biomarkers such as aggrecan and collagen II, and their transcript levels were elevated in vitro. In addition, they mixed hUDSCs with hyaluronic acid (HA) and administered in joint defect in rabbits. Twelve weeks following treatment could encourage cartilage regeneration. These results indicated that hUSCs might be another potential medicinal cell origin for cartilage tissue engineering, particularly if coupled with HA. Despite the outcomes of this investigation seem intriguing, numerous constraints must be tackled before therapeutic applications, and more research is necessary to demonstrate: 1) how to boost hUSCs’ chondrogenic capability since the expression levels of all chondrogenic genes were comparatively low; 2) the benefits of hUSCs over other MSCs by comparing their chondrogenic ability to that of other MSC types, including hBMSCs and hASCs; and 3) the biological processes that govern the chemical reactions between hUSCs and HA (Chen et al., 2018).
Sun et al. tested the chondrogenic biological activities of hUDSCs and hBMSCs from the same person. In vitro tests showed that hUDSCs had a higher potential for division, colony formation, and migration than hBMSCs in the same passage. At 12 weeks after placement, scaffolds loaded with hUDSCs or hBMSCs considerably stimulated cartilage defect healing in the rabbit knee model, with the regenerated tissue primarily being hyaline cartilage. Nevertheless, there was not a substantial distinction in cartilage repair efficacy between hUDSCs and hBMSCs. However, this investigation has certain drawbacks. First, since various media can sustain the steady and fast proliferation of hUSCs and hBMSCs, respectively, the micro-environment supplied by the media may influence cellular activities in vitro. Moreover, there are differences in cellular activities across stem cells from various donors. Further investigations should also investigate interindividual differences.
In general, hUSCs can be regarded as an alternative to typical stem cells for cartilage repair; however, differences in the capability of hUSCs and hBMSCs to stimulate cartilage regeneration in vivo and in vitro should be further examined utilizing cell surveillance, genomic assessments, and other research (Sun et al., 2022a).
The tendon-bone interface (TBI) is critical in the transmission of mechanical stresses, yet it is vulnerable to injuries, which usually contribute to fibrous scar tissue development (Chae et al., 2021). As a result, rejuvenation of the fibrocartilaginous zone has become a critical topic of study. Currently, the utilization of stem cells offers an auspicious method for TBI therapy (Yea et al., 2020).
Small intestine submucosa (SIS) hydrogel loaded with hUDSCs that excessively express bFGF was utilized to treat TBI damage, correct inflammatory response abnormalities, and promote the polarizing of macrophages towards regenerative characteristics, establishing a favorable immunological milieu. The amalgamated impact of essential fibroblast growth factor (bFGF) and an optimal immunological environment are expected to boost hUDSCs’ chondrogenic capacity, assisting in the functional recovery of TBI injuries. There are several drawbacks to this research. First, while macrophages are the subject matter, other immune cells like T and B cells may potentially have a substantial impact on TBI repair, but their functions have not been investigated in this study. Second, the specific processes via which SIS hydrogel modifies macrophage morphologies have yet to be identified. Third, despite the multiplicity of macrophage phenotypes characterized by functioning, this study simplifies them into M1 and M2 categories. As a result, future research must thoroughly examine macrophage-produced growth factors, describe the interaction of diverse inflammatory mediators, and document the temporal-spatial patterns of macrophage phenotypic development during TBI repair. Notwithstanding these limits, previous data indicate that SIS hydrogel, especially when paired with hUSCs-bFGF, is a viable approach for enhancing TBI recovery (Chen et al., 2024).
In an investigation conducted by Liu et al.,, chondrocytes injected with IL-1β via hUDSCs-EXOs showed increased proliferation and migration, while apoptosis was prevented. However, extracellular matrix (ECM) secretion diminished as an undesirable effect. HUDSCs-140-Exos preserved the benefits of hUDSCs-EXOs while further increasing ECM secretion by targeting VEGFA, such as collagen II and aggrecan. In vivo testing proved they could promote osteochondral regeneration. While IL-1β activation and surgical initiation are commonly utilized to simulate OA-like changes, the pathophysiology of OA is far more complicated. Second, they employed female rats to imitate in vivo OA in their investigation, because estrogen has been shown to boost miR-140 expression, protecting cartilage from degeneration. As a result, they must confirm their findings using male rats (Liu et al., 2022).
As verified by proteomics data, UDSCS decellularized ECM (dECM) improved rabbit IPFSC cartilage reconstruction and functionality restoration, especially for UDSCs-deposited dECM. RNA-Seq research revealed that inflammatory triggering of macrophages and polarization, as well as mesenchymal-epithelial transition (MET), may be implicated in the C-dECM-mediated increase of IPFSCs’ chondrogenic ability, requiring additional investigation (Pei et al., 2023).
Furthermore, Zeng et al. found that UDSCs in dECM hydrogel survived, multiplied, and formed a large amount of cartilaginous ECM comprising collagen II and aggrecan. In addition, This bioactive platform could stimulate extracellular matrix production, regulate the immune system’s reaction, and enhance cartilage repair in rats (Zeng et al., 2022).
7.3 Skin wound healing
Healthy skin is critical for sustaining the physiological equilibrium because it defends against infection, loss of minerals, mechanical stresses, and temperature imbalance. It also plays an important role in dynamic processes, including hydration, vitamin D production, and excretion (Kim et al., 2021).
Consequently, any interruption in skin integrity may lead to tissue breakdown, leading to acute or chronic lesions. Acute wounds are injuries caused by injuries such as burns or surgically created wounds that heal in a reasonable amount of time, while chronic wounds like ulcers or post-surgical wounds do not progress via the standard healing process promptly, leading to an absence of substantial rehabilitation over an extended period (Zhong et al., 2022). UDSCs could perform an important part in the promotion of skin wound regeneration (Table 5). For example, UDSCs combined with a nanofibrous membrane composed of polycaprolactone/gelatin (PCL/GT) significantly improved revascularization and wound recuperation in rabbits. These findings also show that adding gelatin significantly enhances the hydrophilicity and mechanical characteristics of PCL/GT fibrous membranes, obtaining values equivalent to those of human skin tissue (Fu et al., 2014).
Bacterial cellulose (BC) has good physical and chemical characteristics, and it has a strong influence on wound healing (Liu et al., 2025). Given the superior qualities of bacterial cellulose, the surface structured BC is built using a modified version of the “contact guidance” hypothesis, with the goal of influencing cell activity via the arrangement of the material surface to accomplish what is needed for therapy (Liu et al., 2024; Don et al., 2025). Cao et al. discovered that combining the bacterial cellulose and UDSCs improved revascularization and wound rejuvenation in comparison with treating with scaffold or UDSCs alone (Cao et al., 2019). When activated by bioglass, UDSCs may upregulate growth factor expression, resulting in more favorable wound healing results (Zhang et al., 2018).
7.4 Diabetes
Diabetes mellitus type 2, the ninth most significant cause of mortality, causes insulin resistance and loss of pancreatic β-cells (El-Sherbiny et al., 2020). Isolated UDSCs have been shown to develop into insulin-producing β cells and enhance pancreatic islet angiogenesis, making them appropriate for diabetes therapy (Zhao et al., 2018; Hwang et al., 2019). Hwang et al. discovered that UDSCs can be transformed into insulin-generating cells, which makes reestablishing functioning insulin-releasing cells derived from UDSCs preferable to organ transplantation simply because of their relative abundance. Though additional research is required, their findings indicate that hUDSCs might be a promising source for cell treatment in type 1 diabetes (Hwang et al., 2019).
Given that previous research on UDSCs showed better glucose tolerance in diabetic mice, these findings appear inconsistent. Intrapancreatic administrations of UDSCs could represent the most efficient technique, but intravenous administration had an insufficient impact on reducing blood glucose due to the possibility of entrapment in unwanted tissues. Dong et al. found that USCs repaired and protected pancreatic islets in diabetic rats. Therapy with USCs dramatically reduced histological damage and functional deterioration.
While the USC therapy failed to significantly lower fasting blood glucose levels, it did considerably suppress fibrosis and apoptosis. The present research suggested that administering USCs might be beneficial in the treatment of diabetic problems. Regional administration, intra-arterial delivery, or increasing paracrine activity might all be helpful therapy options for wounded tissues. Additional studies should look at the therapeutic benefits of USCs in T2D and its consequences (Dong et al., 2016).
7.5 Dental engineering
The tooth is generated by a mutually beneficial interplay between the epithelium and mesenchymal cells (Olaru et al., 2021). The epithelium then divides into ameloblasts, eventually becoming the enamel, whereas the mesenchyme develops into other dental structures. Nevertheless, certain critical difficulties must be addressed before these emerging ideas may be used in dentistry clinics. The paucity of persistent avenues for epithelial stem cells with odontogenic capacity in adult humans is likely the most significant restricting issue (Shen et al., 2020; Lee et al., 2022). In this context, Cai et al. differentiated U-IPSCs into epithelial sheets, which were reorganized with E14.5 mouse dental mesenchyme. Dental-like constructs have been isolated within 3 weeks, with yields of up to 30% for eight distinct iPSC lines, similar to H1 hESC. They also discovered that UiPSC-derived epithelial sheets developed into ameloblasts in dental-like structures, which had physical attributes similar to those observed in regular human teeth, like elastic modulus and hardness.
Of course, further work is needed to generate an iPSC-derived epithelial sheet with odontogenic capability through a deep examination of molecular processes linked with epithelial-mesenchymal reactions, which is a recurring theme throughout development. However, future research ought to concentrate on the derivation of the odontogenic capacity of integration-free human urine induced pluripotent stem cells (ifhU-iPSCs), which will be beneficial for the ultimate objective of the entire regeneration of human teeth for therapeutic therapy (Cai et al., 2013).
7.6 Periodontal regeneration
Over the past 150 years, significant scientific discoveries in periodontology have profoundly revolutionized how doctors diagnose and treat periodontal illnesses. Nevertheless, there is still no optimum treatment method today to treat periodontitis or accomplish reliable and efficient tissue regeneration (Karobari et al., 2022; Marya et al., 2022). In many situations, contemporary periodontal therapy fails to regain functionality in various tissues (El-Nablaway et al., 2024b; Atia et al., 2023). Traditional mechanical or anti-infective periodontal therapy has aimed to eliminate inflammatory reactions and slow disease development (Rokaya et al., 2022; Heboyan et al., 2022). In the last 20 years, many regeneration techniques have been devised, tried, and reviewed to repair missing tooth-supporting components (Banakar et al., 2022; Moonla et al., 2022).
Human periodontal ligament stem cells (PDLSCs) have been used extensively as seeding cells and cellular sheets in periodontal tissue regeneration (Atia et al., 2025). Despite substantial advances in PDLSC application, promoting cell proliferation and numerous differentiations of PDLSCs remains a key problem due to low cell numbers at the time of acquiring (Alkandari et al., 2025; El-Nablaway et al., 2024a). Yang et al. investigated the paracrine impacts of UDSCs on cell division and bone-forming transformation in PDLSCs. When combined with UDSCs, PDLSCs could exhibit enhanced division and cementogenic/osteogenic transformation. In vivo implantation revealed that PDLSC sheets cocultured with UDSCs generated more fresh and dense tissues while also expressing greater quantities of osteogenic and cementogenic proteins.
These findings point to a potential new technique for clinical periodontal tissue restoration. While the cellular process of this experiment deserves additional investigation, the noninvasive collecting approach to USCs and their steady growth render USCs a potential novel method for utilization in clinical periodontal tissue restoration (Yang et al., 2020). Furthermore, Xiong et al. investigated the biological impacts of the hUSC-derived ECM on the division, attachment, dispersing, and transformation of hPDLSCs. They discovered that UDSCs-derived ECM (UECM) increased PDLSC division, osteogenic transformation capacity, and angiogenesis in comparison with native PDLSCs. The drawbacks of this study stem from the inability to precisely quantify the protein content of the ECM. Equivalent quantities of cells underwent stimulation for 8 days to form ECMs.
Yet, the ultimate amount of ECM was challenging to determine since the process of producing ECM is influenced by a variety of circumstances. With the features listed above, UECM might be a potential agent for utilization in 3D bioink applications or biological constructs. Nevertheless, other biological experiments to identify the biological basis of the interaction between ECM and hPDLSCs are deserving of further investigation (Xiong et al., 2019).
7.7 Ophthalmological disorders
Retinal ganglion cells (RGCs) are neurons in the retina’s last segment. Their axons are dispersed on the surface of the omentum and gathered in the papilla of the optic tract (optic nerve) (Goetz et al., 2022). UDSCs-derived exosomes have therapeutic value by suppressing cell apoptosis, increasing cellular survival, and promoting the division of aged RGCs. The foundational process consists of various genetic variations as well as modifications in communication signaling. Exosome-targeted therapy is a fresh strategy for cell-free therapy and medication delivery for the management of age-related illnesses.
Despite exosome treatment being a novel method for combating aging, translating preclinical outcomes to the clinic requires more detailed research into exosome biology and associated approaches. In general, attention to the influence of aging on USCs and vice versa is crucial for devising innovative therapy employing USCs with an emphasis on the treatment of older adults (Dan et al., 2023).
7.8 Infertility disorders
Infertility has developed as a global health concern that can be caused by male, female, or both (Volarevic et al., 2014; Vander Borght and Wyns, 2018). Intrauterine adhesion (IUA), an important factor of recurrent abortions and secondary infertility, is defined as partial or complete obliteration of the uterus owing to injuries to the endometrium. While many therapies have been explored to prevent IUA, they have demonstrated poor therapeutic efficacy. Tissue engineering technological innovation, a promising bioengineering tool, can potentially improve endometrial regeneration.
Small intestine submucosa (SIS) loaded with UDSCs has been used to regenerate endometrial. In vitro, the SIS scaffolds showed good mechanical characteristics and biocompatibility while promoting epithelial migration and revascularization. Transplantation of SIS/UDSCs preserved standard luminal structure, increased endometrial and glandular rejuvenation, revascularization, reduced fibrosis, and enhanced endometrium responsiveness. This has resulted in a new technique for boosting endometrial shape and functioning, which could prove useful for IUA avoidance and management in clinics. The biological process behind the unique role of the USCs in endometrial and gland regeneration is inadequately known, although it deserves rigorous and comprehensive research (Song et al., 2023).
Nonobstructive azoospermia (NOA) is a severe condition of male infertility with few viable treatment options (Zhankina et al., 2021). UDSCs have multipotent differentiation potential and paracrine actions and are involved in tissue reconstruction and rejuvenation. Deng et al. demonstrated that both UDSCs and UDSCs-EXOs recovered spermatogenesis in NOA mice 36 days following busulfan administration, but they failed to shield the mouse testicles from early busulfan damage. This investigation offers a unique perspective on the therapy of NOA (Deng et al., 2019).
7.9 Cardiac regeneration
Cardiomyocyte loss is connected with a reduction in the heart’s pumping ability. Since cardiomyocytes have an exceedingly poor self-renewal capability (Bergmann et al., 2015). Human genetically acquired heart disorders have been researched regardless of patient genetic origin. Because human cardiomyocytes (CMs) are in short supply, using urine specimens to make U-IPSCs-derived CMs would be a noninvasive way to uncover cardiac disorders that cause diseases in individuals with specific genetic backgrounds.
Jouni et al. modified cells from the urine of a patient with long QT syndrome who had the HERG A561P gene mutation utilizing an episomal-based approach.
UhiPS cells were subsequently differentiated into CMs by the matrix sandwich technique. UhiPS-CMs properly expressed atrial and ventricular myofilament proteins, as well as ion channels. These results suggest the application of CMs derived from iPS cells rather than noncardiac heterologous transcription methods to gain a true understanding of the mechanism behind cardiac channelopathy. Currently, they are the most analogous model to human CMs derived directly from heart tissue, enabling exact knowledge of the molecular foundation that underlies disease phenotypes (Jouni et al., 2015).
Ventricular septal defects (VSDs) are among the most prevalent congenital cardiac abnormalities. Cao et al. used a Sendai virus vector for successfully generating integration-free iPSCs from urine specimens of a patient with VSD and HF who carried an L2483R mutation in the RyR2 gene. U-IPSCs exhibited characteristics of cardiomyocytes, such as spontaneously induced contractions and high levels of cardiac biomarkers. Nevertheless, as compared to cardiomyocytes produced from H9 cells, UDSCs showed a more significant amount of autophagy, indicating that autophagy may play a crucial part in the emergence of VSD with heart failure (HF). The approach reported here enables the affordable and repeatable production of human cardiomyocytes in entirely chemically controlled circumstances, facilitating the implementation of iPSCs into large-scale testing applications or regenerative therapies (Cao et al., 2018). In vivo, the application of UDSCs could alleviate fibrosis and apoptosis of the myocardium in rats. Injection of USCs restored the poor functioning of the left ventricle. A viable therapy strategy for problems might involve reducing fibrosis and preventing cell death. Additional studies should look at the mechanism and therapeutic applications (Dong et al., 2016).
7.10 Bone engineering
Bone regeneration is important in orthopedic and dental therapy, particularly in traumatic and congenital abnormalities and bone strengthening for biomedical implants. Regardless of the wide range of procedures used in healthcare settings, efficient and functional bone regeneration represents a substantial issue (Gu et al., 2022).
While autogenic bone-based surgeries are regarded as the most effective approach, their practical application remains restricted because of comorbidity at the donation site and a paucity of appropriate bone volume. As a result, these constraints have prompted researchers to investigate artificial substitutes as potential scaffolds or alternatives to bone transplantation (He et al., 2022).
Numerous materials have been created and assessed for their suitability for bone regeneration. Additionally, artificial implantable biomaterials designed to replace natural bone should have bone-like architecture and mechanical characteristics for maximum performance. Stem cell transplantation, which uses the body’s regenerative capacities, offers a cutting-edge way to modify conventional therapies and potentially give more individualized and successful therapies for bone defects. MSCs also produce bioactive chemicals that can modulate immunity system activity and stimulate tissue renewal. As a result, MSCs are widely recognized as a promising tissue regeneration tool (Lau et al., 2022). Autogenous MSCs have shown inadequate efficacy in addressing osteoporosis and bone abnormalities due to diminished functionality and reduced regeneration capacity (Feroz and Dias, 2021; Liu et al., 2020c). Numerous materials have been created and assessed for their suitability for bone regeneration. Additionally, artificial implantable biomaterials designed to replace natural bone should have bone-like architecture and mechanical characteristics for maximum performance. Stem cell transplantation, which uses the body’s regenerative capacities, offers a cutting-edge way to modify conventional therapies and potentially give more individualized and successful therapies for bone defects. MSCs also produce bioactive chemicals that modulate immunity system activity and stimulate tissue renewal. As a result, MSCs are widely recognized as a promising tissue regeneration tool (Anderson et al., 2022).
Bone substitutes could be utilized as an efficient bone regeneration method. A substantial human supply of autologous cells that can develop or be transformed into osteoblasts is vital for designing human bone transplants (Amini et al., 2012). Nevertheless, extensive bone injury necessitates the supply of osteogenic cells as a 3D platform to facilitate bone repair. ESCs and IPSCs are now employed for bone transplants (Guan et al., 2015b).
Furthermore, because of UDSC’s excellent proliferative, self-regeneration, and transformation potential when transformed into osteoblasts, urine stem cells might be an excellent alternative for bone regeneration. Guan et al. used Calcium silicate (CS) particulates and mixed with poly (lactic-co-glycolic acid) (PLGA), to generate PLGA/CS composite. UDSCs were then sown onto these frameworks, and they were then transplanted into naked mice. The findings demonstrated that CS ion extracts boosted cell division, ALP action, calcium deposits, and the synthesis of new bones significantly and strongly stimulated the bone-forming transformation of UDSCs in vivo. As a result, PLGA +10% CS scaffolds may have the optimal aforementioned features for USCs. These findings can help guide future research on biomaterial development. Nevertheless, the precise mechanism behind this phenomenon remains obscure and requires additional exploration (Guan et al., 2015a).
8 Limitations and concerns
As previously mentioned, gathering urine before the UDSCs separation is straightforward, reliable., innocuous, and inexpensive in comparison to surgical procedures used to collect other stem cell types. UDSCs can be extracted from healthy and diseased people, preserving their ability to grow and divide and allowing for large-scale benign sampling and storage. Moreover, there are no substantial ethical issues related to UDSCs collection, and they can be used for both customized and substantial scientific or therapeutic purposes (Ferreiro et al., 2024).
These changes can be attributed to transforming culturing circumstances, particularly the chemical composition of the medium used for cultivation, or to a natural property of the separated cells that may differ among individuals. Additional investigations are necessary to understand further surface marker levels of transcription and their importance in UDSC development (Wang et al., 2024).
Several essential biological concerns, including immunoregulatory actions and carcinogenic potential hazards, have not been thoroughly investigated to gain additional insight into the usefulness and safety of UDSCs. UDSCs’ immune-modulating impacts must be explored because they are most likely significant (Rao et al., 2024). To produce proof for UDSCs-based therapy, the distinctive characteristics of UDSCs subpopulations must be identified. Furthermore, a fundamental study on the origin of UDSCs is important since it may answer questions including who is best suited to provide UDSCs, the way to collect UDSCs appropriately, and if there are any unique indicators of UDSCs. We hope that single-cell sequencing paired with lineage tracking can provide a few clues.
Clinical-grade stem cell synthesis should adhere strictly to quality assurance (QA) criteria. The reliability and efficacy of the UDSCs must be evaluated (Zhao et al., 2022). Generally, producing UDSCs includes donor selection, cell harvesting, medium composition, cell amplifying process, inspection for quality standards, and so on. First, donor factors, including age, disease, and medications, could impact the biological properties of the UDSCs collected. Gao et al. discovered that UDSCs from younger donors had greater proliferating capacity, less death, and more excellent osteogenic differentiation capability despite UDSCs of all ages having the capacity for bone regeneration (Pizzuti et al., 2024).
Schosserer et al. discovered a more significant successful percentage separating UDSCs from males than from females (70% vs. 42%) (Schosserer et al., 2015). Given the differences between male and female sexual organs, caution should be exercised when gathering specimens of urine from females to avoid the menstrual period and the initial urination of the day, as well as washing the genitalia. Second, the manner of cell collection could impact the final treatment effect; thus it is critical to optimize the UDSCs culture process (Liu et al., 2021; Kibschull et al., 2023).
Third, because medium composition differs by laboratory, the variances are primarily associated with serum levels and nutritional factors. Clinical uses typically demand many cells; thus, the rapid and widespread multiplication of UDSCs for widespread use has presented a significant obstacle. Microcarrier-based suspension cultivation could offer a solution (Hao et al., 2023).
Nevertheless, it is unclear if UDSCs cultivated using this approach stay the same or if the changes between UDSCs cultivated using numerous ways result in various medicinal properties. An alternative way to solve the same challenge is to reconstitute the settings for the cultivation of the UDSCs by including certain nutrients, implanting the UDSCs on frameworks, and controlling oxygen levels to enhance UDSCs separation and division (Kibschull et al., 2023; Liu et al., 2021).
Meeting the high-quality assurance standards is a precondition for UDSCs-based cyto-therapeutics and may require significant efforts. An assay matrix is proposed that includes RNA identification of specific genes, transcriptional evaluation of essential cell surface indicators, and secretome protein identification (Dayati et al., 2024). When using UDSCs in the field of regenerative therapy, the scaffold materials should be evaluated alongside the cellular-scaffolding complexes based on the clinical requirements and the methods via which stem cells can perform biological tasks (Yang et al., 2020; Lu et al., 2023).
Indeed, it is unclear how UDSCs might contribute to tissue restoration. As a result, whether cellular stimulation or other modifications are required remains unclear (Yang et al., 2018). Moreover, the use of UDSCs discharges, including exosomes and ECM, may avoid the possible risks associated with employing UDSCs to treat the condition. It will require an extended period to develop sufficient cells for autologous treatment. As a result, it is greatly practicable to use UDSCs for managing chronic wounds and surgical operations rather than acute burns until allogeneic UDSCs treatments are shown to be safe, successful, and cost-efficient (Yang et al., 2024).
Although U-IPSCs show tremendous prospects for personalized healthcare, various obstacles, and issues should be tackled to realize their capacity completely. IPSCs have significant pluripotency, but their reconfiguration responsiveness varies based on the cellular source, as do their growth courses and development characteristics (Mulder et al., 2020). Furthermore, changes in reprogramming component quantities, mechanisms of transfections, cell cultivation circumstances, and timings among numerous research settings may result in decreased iPSC initiation efficacy and even the creation of off-target cells (Al Abbar et al., 2020). As a result, developing standardized reprogramming techniques is critical to ensuring repeatability and comparability of experiments among various investigation groups, which is essential for enhancing research reliability outcomes. Standardization approaches can involve using a single cell origin, adopting an array of commonly used classical reprogramming factors, ensuring uniformity in experimental circumstances, and developing consistent iPSC recognition standards. Although IPSCs can proliferate indefinitely, the mutation rates vary amongst cell lines. Specific genetic alterations could have been generated during IPSCs processing, resulting in tumorigenesis (De Masi et al., 2020). As a result, preserving genetic integrity in IPSCs throughout long-term proliferation is critical for their safety. To remove potential variances, researchers evaluate the differentiation status, sequence the IPSCs, and analyze the karyotypes. Other options involve employing non-integrative reprogramming strategies, applying genetic modification approaches to fix putative tumor-causing abnormalities in IPSCs, and Pre-differentiation of IPSCs into particular types of cells (Poetsch et al., 2022). To summarize, IPSCs must undergo severe quality assurance and security precautions before being used in therapy to eradicate their cancer-causing capacity.
In several contemporary findings from experiments, IPSCs display standard indicators and have distinct morphological characteristics. Nevertheless, they may not operate properly in vivo (Du et al., 2015). Nevertheless, IPSCs can abnormally develop into teratomas, resulting in immunological rejection. Furthermore, longevity and transplantation of IPSCs in vivo necessitate adequate criteria, and straightforward cellular injections might not offer the necessary milieu that encourages their differentiation and maturity (Kim et al., 2024). As a result, researchers can examine a variety of approaches, such as selecting the appropriate treatment schedule, sufficient quantities of cells, and employing biomaterials as scaffolding for IPSCs.
After overcoming several laboratory difficulties, the objective is to establish massive iPSC generation to satisfy clinical requirements. First, the choice of a suitable cell origin, like UDSCs, which can be acquired in enormous amounts non-invasively, is critical for wide-scale development (Citterio et al., 2020). Non-integrating reconfiguration approaches for editing genes and optimized Cellular development techniques and the construction of adaptable, computerized culture platforms must be used to increase cell cultivation effectiveness. Furthermore, periodic examination and evaluation of IPSCs, early excision of aberrant cells, and cell quality assurance are required (Citterio et al., 2020). Moreover, reliable filtration and the acquisition of the intended cell types, as well as the construction of proper storage techniques, are required for IPSCs to be used quickly on demand (Jamal et al., 2021).
Although various cell culture settings have been documented, whether these variables have a substantial influence on the medicinal value of USCs is mainly unclear (Xie et al., 2023). The absence of criteria for the classification of UDSCs, in particular, makes direct comparisons between research difficult, which should be tackled in the future. Along with freshly discharged urine, samples for UDSCs separation can be acquired by ureteral catheterization and kept in various conditions (Wu et al., 2011). In addition to healthy donors, USCs from patients with various disorders (e.g., bladder cancer) have been defined (Ooki et al., 2018; Jiang et al., 2016; Zhou et al., 2012). In initial cultivation, UDSCs revealed a rice grain or spindle-like architecture (Chen et al., 2017). Due to long telomeres, USCs can multiply extensively, even after 60–70 cell doublings (Garbe et al., 2009). From a therapeutic standpoint, it is crucial to evaluate the variations in USC biology throughout serial cultures, which is a precondition for determining optimal cell passages for treatment (Sun et al., 2021b; Liu et al., 2022). Donor age, sex, and specimen harvesting techniques also affect the separation and development of UDSCs (Gao et al., 2017). In comparison with older donors, young people demonstrated better functioning, more significant division, and reduced aging in their UDSCs (Ferreiro et al., 2024; Strässler et al., 2018). UDSCs separation was more efficient in male volunteers than with female ones (Klapper-Goldstein et al., 2022; Gutiérrez-Aguirre et al., 2022). Catheterization-collected urine contained more UDSCs than freely voided urine, possibly due to the extraction of cells from the inner bladder wall (Coelho et al., 2023; Yu et al., 2021).
Recommendations on the methodology of urine collection, such as undesirable conditions (e.g., contaminations and cancers) to be utilized in regenerative therapies, have already been published; however, the coming years will bring developments in the automated retrieval of cells from the urine.
9 Concluding remarks
UDSCs-dependent periodontal tissue engineering has shown promise in creating a milieu favorable to periodontal regeneration via well-constructed controlled scaffolding constructions that mimic periodontal multilayer frameworks. However, the application of UDSCs continues to encounter various obstacles, notably risks associated with the proliferation of cells in vitro, standardized manufacturing processes for biological substances, intrinsic antigenicity, selectivity of scaffold components, biological compatibility, and material breakdown. Cell-free therapy, which incorporates biological compounds to stimulate regeneration in organisms, focused on a number of the drawbacks of cell therapy and provided a better understanding of how UDSCs work. Nevertheless, the setting and conditions of exosome cultivation significantly impact exosome attributes. Further research is needed to define exosome activity, dosage, effectiveness, and appropriate collection and preservation techniques. Finally, pharmacological therapy that targets signaling molecules promotes functioning periodontal regeneration.
Author contributions
GA: Conceptualization, Data curation, Formal Analysis, Resources, Software, Supervision, Validation, Visualization, Writing – original draft, Writing – review and editing. AAD: Conceptualization, Data curation, Formal Analysis, Funding acquisition, Resources, Software, Validation, Visualization, Writing – original draft, Writing – review and editing. ET: Conceptualization, Data curation, Formal Analysis, Resources, Software, Validation, Visualization, Writing – original draft, Writing – review and editing. WA: Conceptualization, Data curation, Formal Analysis, Software, Validation, Visualization, Writing – original draft, Writing – review and editing. S-GC: Conceptualization, Data curation, Formal Analysis, Funding acquisition, Resources, Software, Validation, Visualization, Writing – original draft, Writing – review and editing. AAA: Conceptualization, Data curation, Software, Validation, Visualization, Writing – original draft, Writing – review and editing. MH: Conceptualization, Data curation, Formal Analysis, Software, Supervision, Validation, Visualization, Writing – original draft, Writing – review and editing. AbA: Conceptualization, Data curation, Formal Analysis, Software, Validation, Visualization, Writing – original draft, Writing – review and editing. NT: Conceptualization, Data curation, Formal Analysis, Software, Validation, Visualization, Writing – original draft, Writing – review and editing. AI: Conceptualization, Data curation, Formal Analysis, Resources, Software, Validation, Visualization, Writing – original draft, Writing – review and editing. DZ: Conceptualization, Data curation, Formal Analysis, Software, Validation, Visualization, Writing – original draft, Writing – review and editing. EE: Conceptualization, Data curation, Formal Analysis, Software, Validation, Visualization, Writing – original draft, Writing – review and editing. HH: Conceptualization, Data curation, Formal Analysis, Software, Validation, Visualization, Writing – original draft, Writing – review and editing. MM: Conceptualization, Data curation, Formal Analysis, Software, Validation, Visualization, Writing – original draft, Writing – review and editing. KA: Data curation, Methodology, Software, Validation, Visualization, Writing – review and editing. SK: Data curation, Funding acquisition, Software, Validation, Visualization, Writing – review and editing. AhA: Conceptualization, Formal Analysis, Resources, Software, Supervision, Validation, Visualization, Writing – original draft, Writing – review and editing.
Funding
The author(s) declare that financial support was received for the research and/or publication of this article. This research was supported by the Korean Fund for Regenerative Medicine (KFRM) grant funded by the Korean government (the Ministry of Science and ICT and the Ministry of Health & Welfare) Grant numbers: 24A0203L1 (S-GC). This paper was also supported by the KU-Research Professor Program of Konkuk University, Seoul, South Korea (AAD) and Zarqa University-Jordan. This work is also supported via funding from Prince Sattam bin Abudlaziz University project number (PSAU/2025/R/1446) and Researchers Supporting Project number (MHIRSP2025006), AlMaarefa University, Riyadh, Saudi Arabia.
Conflict of interest
Author S-GC was employed by StemExOne Co., Ltd.
The remaining authors declare that the research was conducted in the absence of any commercial or financial relationships that could be construed as a potential conflict of interest.
Generative AI statement
The author(s) declare that no Generative AI was used in the creation of this manuscript.
Publisher’s note
All claims expressed in this article are solely those of the authors and do not necessarily represent those of their affiliated organizations, or those of the publisher, the editors and the reviewers. Any product that may be evaluated in this article, or claim that may be made by its manufacturer, is not guaranteed or endorsed by the publisher.
References
Al Abbar, A., Ngai, S. C., Nograles, N., Alhaji, S. Y., and Abdullah, S. J. B. O. A. (2020). Induced pluripotent stem cells: reprogramming platforms and applications in cell replacement therapy. Biores. Open Access 9, 121–136. doi:10.1089/biores.2019.0046
Alkandari, M., Barai, P., Atia, G. A. N., Mohamed, S. Z., Ghobashy, M. M., Shalaby, H. K., et al. (2025). Bioactive functionalized chitosan thermo-responsive hydrogels as promising platforms for therapeutic, regenerative oral, and maxillofacial applications. Regen. Oral, Maxillofac. Appl. 20, e202400653. doi:10.1002/biot.202400653
Amini, A. R., Laurencin, C. T., and Nukavarapu, S. P. (2012). Bone tissue engineering: recent advances and challenges. Crit. Reviews™ Biomed. Eng. 40, 363–408. doi:10.1615/critrevbiomedeng.v40.i5.10
Anderson, M., Dubey, N., Bogie, K., Cao, C., Li, J., Lerchbacker, J., et al. (2022). Three-dimensional printing of clinical scale and personalized calcium phosphate scaffolds for alveolar bone reconstruction. Dent. Mat. 38, 529–539. doi:10.1016/j.dental.2021.12.141
Atia, G. A., Shalaby, H. K., Roomi, A. B., Ghobashy, M. M., Attia, H. A., Mohamed, S. Z., et al. (2023). Macro, micro, and nano-inspired bioactive polymeric biomaterials in therapeutic, and regenerative orofacial applications. Dev. and Ther. 17, 2985–3021. doi:10.2147/dddt.s419361
Atia, G. A. N., Mohamed, S. Z., Taymour, N., Soliman, M. M., Halim, H. A., Shalaby, H. K., et al. (2025). Carbon dots as promising carbon nanomaterials for diagnostics, therapeutics, and regenerative orofacial applications. J. Drug Deliv. Sci. Technol. 106808. doi:10.1016/j.jddst.2025.106808
Augustine, R., Dan, P., Hasan, A., Khalaf, I. M., Prasad, P., Ghosal, K., et al. (2021). Stem cell-based approaches in cardiac tissue engineering: controlling the microenvironment for autologous cells. PHARMACOTHERAPY 138, 111425. doi:10.1016/j.biopha.2021.111425
Banakar, M., Moayedi, S., Shamsoddin, E., Vahedi, Z., Banakar, M. H., Mousavi, S. M., et al. (2022). Chewing gums as a drug delivery approach for oral health. Int. J. Dent. 2022, 1–10. doi:10.1155/2022/9430988
Barakat, B., Franke, K., Schakaki, S., Hijazi, S., Hasselhof, V., and Vögeli, T.-A. J. A. J. O. U. (2020). Stem cell applications in regenerative medicine for stress urinary incontinence: a review of effectiveness based on clinical trials. Arab. J. Urol. 18, 194–205. doi:10.1080/2090598X.2020.1750864
Bergmann, O., Zdunek, S., Felker, A., Salehpour, M., Alkass, K., Bernard, S., et al. (2015). Dynamics of cell generation and turnover in the human heart. Cell 161, 1566–1575. doi:10.1016/j.cell.2015.05.026
Bharadwaj, S., Liu, G., Shi, Y., Markert, C., Andersson, K.-E., Atala, A., et al. (2011). Characterization of urine-derived stem cells obtained from upper urinary tract for use in cell-based urological tissue engineering. Tissue Eng. Part A 17, 2123–2132. doi:10.1089/ten.tea.2010.0637
Bharadwaj, S., Liu, G., Shi, Y., Wu, R., Yang, B., He, T., et al. (2013). Multipotential differentiation of human urine-derived stem cells: potential for therapeutic applications in urology. Stem Cells 31, 1840–1856. doi:10.1002/stem.1424
Cai, J., Zhang, Y., Liu, P., Chen, S., Wu, X., Sun, Y., et al. (2013). Generation of tooth-like structures from integration-free human urine induced pluripotent. stem cells 2, 1–8. doi:10.1186/2045-9769-2-6
Cao, Y.-M., Liu, M.-Y., Xue, Z.-W., Qiu, Y., Li, J., Wang, Y., et al. (2019). Surface-structured bacterial cellulose loaded with hUSCs accelerate skin wound healing by promoting angiogenesis in rats. Biochem. Biophys. Res. Commun. 516, 1167–1174. doi:10.1016/j.bbrc.2019.06.161
Cao, Y., Xu, J., Wen, J., Ma, X., Liu, F., Li, Y., et al. (2018). Generation of a urine-derived Ips cell line from a patient with a ventricular septal defect and heart failure and the robust differentiation of these cells to cardiomyocytes via small molecules. Cell. Physiol. biochem. 50, 538–551. doi:10.1159/000494167
Casarin, M., Morlacco, A., and Dal Moro, F. J. I. J. O. M. S. (2022). Tissue engineering and regenerative medicine in pediatric urology: urethral and urinary bladder reconstruction. Int. J. Mol. Sci. 23, 6360. doi:10.3390/ijms23126360
Cehakova, M., Ivanisova, D., Strecanska, M., Plava, J., Varchulova Novakova, Z., Nicodemou, A., et al. (2023). Rheumatoid synovial fluid and acidic extracellular pH modulate the immunomodulatory activity of urine-derived stem cells. stem cells 24, 15856. doi:10.3390/ijms242115856
Chae, S., Sun, Y., Choi, Y.-J., Ha, D.-H., Jeon, I., and Cho, D.-W. J. B. (2021). 3D cell-printing of tendon-bone interface using tissue-derived extracellular matrix bioinks for chronic rotator cuff repair. Biofabrication 13, 035005. doi:10.1088/1758-5090/abd159
Chen, C.-Y., Du, W., Rao, S.-S., Tan, Y.-J., Hu, X.-K., Luo, M.-J., et al. (2020). Extracellular vesicles from human urine-derived stem cells inhibit glucocorticoid-induced osteonecrosis of the femoral head by transporting and releasing pro-angiogenic DMBT1 and anti-apoptotic TIMP1. Acta Biomater. 111, 208–220. doi:10.1016/j.actbio.2020.05.020
Chen, J., Zhang, Q.-Y., Tan, J., He, T., Qin, B.-Q., Sheng, N., et al. (2024). Enhanced fibrocartilage regeneration at the tendon-bone interface injury through extracellular matrix hydrogel laden with bFGF-overexpressing human urine-derived stem cells. stem cells 497, 154333. doi:10.1016/j.cej.2024.154333
Chen, L., Li, L., Xing, F., Peng, J., Peng, K., Wang, Y., et al. (2018). Human urine-derived stem cells: potential for cell-based therapy of cartilage defects. Stem Cells Int. 2018, 1–14. doi:10.1155/2018/4686259
Chen, W., Xie, M., Yang, B., Bharadwaj, S., Song, L., Liu, G., et al. (2017). Skeletal myogenic differentiation of human urine-derived cells as a potential source for skeletal muscle regeneration. J. Tissue Eng. Regen. Med. 11, 334–341. doi:10.1002/term.1914
Choi, J. Y., Chun, S. Y., Ha, Y.-S., Kim, D. H., Kim, J., Song, P. H., et al. (2017). Potency of human urine-derived stem cells for renal lineage differentiation. Tissue Eng. Regen. Med. 14, 775–785. doi:10.1007/s13770-017-0081-y
Chun, S. Y., Kim, H. T., Kwon, S. Y., Kim, J., Kim, B. S., Yoo, E. S., et al. (2016). The efficacy and safety of Collagen-I and hypoxic conditions in urine-derived stem cell ex vivo culture. Tissue Eng. Regen. Med. 13, 403–415. doi:10.1007/s13770-016-9073-6
Citterio, F., Gualini, G., Fierravanti, L., Aimetti, M. J. P., and Research, A. (2020). Stem cells and periodontal regeneration: present and future. Plast. Aesthet. Res. 7, 41. doi:10.20517/2347-9264.2020.29
Coelho, H., Neves, S., Menezes, J., Antoniolli-Silva, A., and Oliveira, R. J. B. J. O. B. (2023). Cell therapy with adipose tissue-derived human stem cells in the urinary bladder improves detrusor contractility and reduces voiding residue. Braz. J. Biol. 83, e268540. doi:10.1590/1519-6984.268540
Dan, Q.-Q., Chen, L., Shi, L.-L., Zhou, X., Wang, T.-H., Liu, H. J. B. M., et al. (2023). Urine-derived mesenchymal stem cells-derived exosomes enhances survival and proliferation of aging retinal ganglion cells. BMC Mol. Cell Biol. 24, 8. doi:10.1186/s12860-023-00467-4
Darby, I. J. P. (2022). Risk factors for periodontitis and peri-implantitis. Periodontol. 2000. 90, 9–12. doi:10.1111/prd.12447
Dayati, P., Shakhssalim, N., and Allameh, A. J. C. B. (2024). Over-expression of KRT17 and MDK genes at mRNA levels in urine-exfoliated cells is associated with early non-invasive diagnosis of non-muscle-invasive bladder cancer. cancer 131-132, 110808. doi:10.1016/j.clinbiochem.2024.110808
De Masi, C., Spitalieri, P., Murdocca, M., Novelli, G., and Sangiuolo, F. J. H. G. (2020). Application of CRISPR/Cas9 to human-induced pluripotent stem cells: from gene editing to drug discovery. Hum. Genomics 14, 25–12. doi:10.1186/s40246-020-00276-2
Deng, C., Xie, Y., Zhang, C., Ouyang, B., Chen, H., Lv, L., et al. (2019). Urine-derived stem cells facilitate endogenous spermatogenesis restoration of busulfan-induced nonobstructive azoospermic mice by paracrine exosomes. Stem Cells Dev. 28, 1322–1333. doi:10.1089/scd.2019.0026
Deng, J., Lu, C., Zhao, Q., Chen, K., Ma, S., and Li, Z. J. J. O. P. R. (2022). The Th17/Treg cell balance: crosstalk among the immune system, bone and microbes in periodontitis. J. Periodontal Res. 57, 246–255. doi:10.1111/jre.12958
Deng, L., Du, C., Song, P., Chen, T., Rui, S., Armstrong, D. G., et al. (2021). The role of oxidative stress and antioxidants in diabetic wound healing. Oxid. Med. Cell. Longev. 2021, 8852759. doi:10.1155/2021/8852759
Don, T.-M., Lee, K.-T., Chen, B.-Y., Tang, S., Huang, Y.-C., and Chuang, A. E.-Y. J. I. J. O. B. M. (2025). Physicochemical properties of bacterial cellulose/phototherapeutic polypyrrole/antibacterial chitosan composite membranes and their evaluation as chronic wound dressings. Int. J. Biol. Macromol. 308, 142183. doi:10.1016/j.ijbiomac.2025.142183
Dong, X., Zhang, T., Liu, Q., Zhu, J., Zhao, J., Li, J., et al. (2016). Beneficial effects of urine-derived stem cells on fibrosis and apoptosis of myocardial, glomerular and bladder cells. Mol. Cell. Endocrinol. 427, 21–32. doi:10.1016/j.mce.2016.03.001
Du, M., Duan, X., and Yang, P. J. C. O. H. R. (2015). Induced pluripotent stem cells and periodontal regeneration. Curr. Oral Health Rep. 2, 257–265. doi:10.1007/s40496-015-0065-8
El-Nablaway, M., Rashed, F., Taher, E. S., Abdeen, A., Taymour, N., Soliman, M. M., et al. (2024a). Prospective and challenges of locally applied repurposed pharmaceuticals for periodontal tissue regeneration. Front. Bioeng. Biotechnol. 12, 1400472. doi:10.3389/fbioe.2024.1400472
El-Nablaway, M., Rashed, F., Taher, E. S., Foda, T., Abdeen, A., Abdo, M., et al. (2024b). Prospectives and challenges of nano-tailored biomaterials-assisted biological molecules delivery for tissue engineering purposes. Life Sci. 349, 122671. doi:10.1016/j.lfs.2024.122671
El-Sherbiny, M., Eladl, M. A., Ranade, A. V., Guimei, M., and Gabr, H. J. S. M. J. (2020). Functional beta-cells derived from umbilical cord blood mesenchymal stem cells for curing rats with streptozotocin-induced diabetes mellitus. Singap. Med. J. 61, 39–45. doi:10.11622/smedj.2019120
Feroz, S., and Dias, G. J. H. (2021). Hydroxypropylmethyl cellulose (HPMC) crosslinked keratin/hydroxyapatite (HA) scaffold fabrication, characterization and in vitro biocompatibility assessment as a bone graft for alveolar bone regeneration. Charact. vitro Biocompat. Assess. as a bone graft alveolar bone Regen. 7, e08294. doi:10.1016/j.heliyon.2021.e08294
Ferreiro, E., Monteiro, M., Pereira, F., Barroso, C., Egas, C., Macedo, P., et al. (2024). Age-dependent energy metabolism and transcriptome changes in urine-derived stem cells. Stem cells 218, 111912. doi:10.1016/j.mad.2024.111912
Fu, Y., Guan, J., Guo, S., Guo, F., Niu, X., Liu, Q., et al. (2014). Human urine-derived stem cells in combination with polycaprolactone/gelatin nanofibrous membranes enhance wound healing by promoting angiogenesis. J. Transl. Med. 12, 274–314. doi:10.1186/s12967-014-0274-2
Gao, P., Han, P., Jiang, D., Yang, S., Cui, Q., and Li, Z. J. C. (2017). Effects of the donor age on proliferation, senescence and osteogenic capacity of human urine-derived stem cells. stem cells 69, 751–763. doi:10.1007/s10616-017-0084-5
Garbe, J. C., Bhattacharya, S., Merchant, B., Bassett, E., Swisshelm, K., Feiler, H. S., et al. (2009). Molecular distinctions between stasis and telomere attrition senescence barriers shown by long-term culture of normal human mammary epithelial cells. Cancer Res. 69, 7557–7568. doi:10.1158/0008-5472.can-09-0270
Giardullo, L., Corrado, A., Maruotti, N., Cici, D., Mansueto, N., and Cantatore, F. P. J. I. J. O. I. (2021). Adipokine role in physiopathology of inflammatory and degenerative musculoskeletal diseases. Int. J. Immunopathol. Pharmacol. 35, 20587384211015034. doi:10.1177/20587384211015034
Goetz, J., Jessen, Z. F., Jacobi, A., Mani, A., Cooler, S., Greer, D., et al. (2022). Unified classification of mouse retinal ganglion cells using function, morphology, and gene expression. Morphol. gene Expr. 40, 111040. doi:10.1016/j.celrep.2022.111040
Gu, J.-T., Jiao, K., Li, J., Yan, J.-F., Wang, K.-Y., Wang, F., et al. (2022). Polyphosphate-crosslinked collagen scaffolds for hemostasis and alveolar bone regeneration after tooth extraction. Bioact. Mat. 15, 68–81. doi:10.1016/j.bioactmat.2021.12.019
Gualtierotti, R., Giachi, A., Suffritti, C., Bedogni, L., Franco, F., Poggi, F., et al. (2024). Optimizing long-term joint health in the treatment of hemophilia. Expert Rev. Hematol. 17, 713–721. doi:10.1080/17474086.2024.2396617
Guan, J.-J., Niu, X., Gong, F.-X., Hu, B., Guo, S.-C., Lou, Y.-L., et al. (2014a). Biological characteristics of human-urine-derived stem cells: potential for cell-based therapy in neurology. Tissue Eng. Part A 20, 1794–1806. doi:10.1089/ten.tea.2013.0584
Guan, J., Zhang, J., Guo, S., Zhu, H., Zhu, Z., Li, H., et al. (2015a). Human urine-derived stem cells can be induced into osteogenic lineage by silicate bioceramics via activation of the Wnt/β-catenin signaling pathway. Biomaterials 55, 1–11. doi:10.1016/j.biomaterials.2015.03.029
Guan, J., Zhang, J., Li, H., Zhu, Z., Guo, S., Niu, X., et al. (2015b). Human urine derived stem cells in combination with β-TCP can be applied for bone regeneration. PLoS One 10, e0125253. doi:10.1371/journal.pone.0125253
Guan, X., Mack, D. L., Moreno, C. M., Strande, J. L., Mathieu, J., Shi, Y., et al. (2014b). Dystrophin-deficient cardiomyocytes derived from human urine: new biologic reagents for drug discovery. Stem Cell Res. 12, 467–480. doi:10.1016/j.scr.2013.12.004
Guo, X., Ji, W., Niu, C., Ding, Y., Chen, Z., Chen, C., et al. (2020). Generation of an urine-derived induced pluripotent stem cell line from a 5-year old X-linked Alport syndrome (X-LAS) patient. Stem Cell Res. 49, 102085. doi:10.1016/j.scr.2020.102085
Gutiérrez-Aguirre, C. H., Esparza-Sandoval, A. C., Palomares-Leal, A., Jaime-Pérez, J. C., Gómez-Almaguer, D., Cantú-Rodríguez, O. G. J. H., et al. (2022). Outpatient haploidentical hematopoietic stem cell transplant using post-transplant cyclophosphamide and incidence of hemorrhagic cystitis. Hematol. Transfus. Cell Ther. 44, 163–168. doi:10.1016/j.htct.2020.09.149
Hao, C., Chu, S., Quan, X., Zhou, T., Shi, J., Huang, X., et al. (2023). Establishing extended pluripotent stem cells from human urine cells. Cell Biosci. 13, 88. doi:10.1186/s13578-023-01051-1
Heboyan, A., Avetisyan, A., Karobari, M. I., Marya, A., Khurshid, Z., Rokaya, D., et al. (2022). Tooth root resorption: a review. Sci. Prog. 105, 00368504221109217. doi:10.1177/00368504221109217
Hernández, H. G., Hernández-Castañeda, A. A., Pieschacón, M. P., and Arboleda, H. J. J. O. P. R. (2021). ZNF718, HOXA4, and ZFP57 are differentially methylated in periodontitis in comparison with periodontal health: epigenome-wide DNA methylation pilot study. J. Periodontal Res. 56, 710–725. doi:10.1111/jre.12868
He, Y., Tian, M., Li, X., Hou, J., Chen, S., Yang, G., et al. (2022). A hierarchical-structured mineralized nanofiber scaffold with osteoimmunomodulatory and osteoinductive functions for enhanced alveolar bone regeneration. Adv. Healthc. Mat. 11, 2102236. doi:10.1002/adhm.202102236
Huang, P., Li, Y., Nasser, M., Guo, H., Huang, H., Zhao, M., et al. (2020). Urine-derived induced pluripotent stem cells in cardiovascular disease. Cardiol. Res. Pract. 2020, 1–8. doi:10.1155/2020/3563519
Hua, Y., Wang, K., Huo, Y., Zhuang, Y., Wang, Y., Fang, W., et al. (2023). Four-dimensional hydrogel dressing adaptable to the urethral microenvironment for scarless urethral reconstruction. Nat. Commun. 14, 7632. doi:10.1038/s41467-023-43421-w
Hu, C., He, Y., Fang, S., Tian, N., Gong, M., Xu, X., et al. (2020). Urine-derived stem cells accelerate the recovery of injured mouse hepatic tissue. Am. J. Transl. Res. 12, 5131–5150.
Hwang, Y., Cha, S.-H., Hong, Y., Jung, A. R., and Jun, H.-S. J. I. J. O. M. S. (2019). Direct differentiation of insulin-producing cells from human urine-derived stem cells. stem cells 16, 1668–1676. doi:10.7150/ijms.36011
Jamal, M., Bashir, A., Al-Sayegh, M., and Huang, G. T.-J. (2021). “Oral tissues as sources for induced pluripotent stem cell derivation and their applications for neural, craniofacial, and dental tissue regeneration,” in Cell sources for iPSCs (Elsevier).
Jha, P. K., Jaidumrong, T., Rokaya, D., and Ovatlarnporn, C. J. R. A. (2024). Callistemon viminalis leaf extract phytochemicals modified silver–ruthenium bimetallic zinc oxide nanocomposite biosynthesis: application on nanocoating photocatalytic Escherichia coli disinfection. RSC Adv. 14, 11017–11026. doi:10.1039/d4ra01355g
Jiang, Y.-F., Chen, M., Zhang, N.-N., Yang, H.-J., Rui, Q., and Zhou, Y.-F. J. B. O. (2018). In vitro and in vivo differentiation of induced pluripotent stem cells generated from urine-derived cells into cardiomyocytes. Biol. Open 7, bio029157. doi:10.1242/bio.029157
Jiang, Z.-Z., Liu, Y.-M., Niu, X., Yin, J.-Y., Hu, B., Guo, S.-C., et al. (2016). Exosomes secreted by human urine-derived stem cells could prevent kidney complications from type I diabetes in rats. Stem Cell Res. Ther. 7, 24–13. doi:10.1186/s13287-016-0287-2
Ji, X., Wang, M., Chen, F., and Zhou, J. J. S. C. I. (2017). Urine-derived stem cells: the present and the future. Stem Cells Int. 2017, 1–8. doi:10.1155/2017/4378947
Jouni, M., Si-Tayeb, K., Es-Salah-Lamoureux, Z., Latypova, X., Champon, B., Caillaud, A., et al. (2015). Toward personalized medicine: using cardiomyocytes differentiated from urine-derived pluripotent stem cells to recapitulate electrophysiological characteristics of type 2 long QT syndrome. J. Am. Heart Assoc. 4, e002159. doi:10.1161/jaha.115.002159
Karobari, M. I., Siddharthan, S., Adil, A. H., Khan, M. M., Venugopal, A., Rokaya, D., et al. (2022). Modifiable and non-modifiable risk factors affecting oral and periodontal health and quality of life in south asia. Open Dent. J. 16. doi:10.2174/18742106-v16-e2209270
Kibschull, M., Nguyen, T., Chow, T., Alarab, M., Lye, S., Rogers, I., et al. (2023). Differentiation of patient-specific void urine-derived human induced pluripotent stem cells to fibroblasts and skeletal muscle myocytes. Sci. Rep. 13, 4746. doi:10.1038/s41598-023-31780-9
Kim, E.-J., Kim, K.-H., Kim, H.-Y., Lee, D.-J., Li, S., Han, M. N., et al. (2024). Harnessing the dental cells derived from human induced pluripotent stem cells for hard tissue engineering. J. Adv. Res., 61, 119–131. doi:10.1016/j.jare.2023.08.012
Kim, S.-H., Lee, S.-H., Jin, J.-A., So, H.-J., Lee, J.-U., Ji, M.-J., et al. (2023). In vivo safety and biodistribution profile of Klotho-enhanced human urine-derived stem cells for clinical application. Stem Cell Res. Ther. 14, 355. doi:10.1186/s13287-023-03595-y
Kim, D., Lee, A. E., Xu, Q., Zhang, Q., and Le, A. D. J. F. I. I. (2021). Gingiva-derived mesenchymal stem cells: potential application in tissue engineering and regenerative medicine-a comprehensive review. Front. Immunol. 12, 667221. doi:10.3389/fimmu.2021.667221
Kim, K., Gil, M., Dayem, A. A., Choi, S., Kang, G.-H., Yang, G.-M., et al. (2020). Improved isolation and culture of urine-derived stem cells (USCs) and enhanced production of immune cells from the USC-derived induced pluripotent stem cells. stem cells 9, 827. doi:10.3390/jcm9030827
Klapper Goldstein, H., Tamam, S., Sade, S., and Weintraub, A. Y. J. I. J. O. G. (2022). A systematic review of stem cell therapy treatment for women suffering from stress urinary incontinence. Intl. J. Gynecol. and Obste. 157, 19–30. doi:10.1002/ijgo.13769
Koga, H., Yamano, T., Betancur, J., Nagatomo, S., Ikeda, Y., Yamaguchi, K., et al. (2023). Efficient production of bispecific antibody by FAST-IgTM and its application to NXT007 for the treatment of hemophilia A. Taylor and Francis 15, 2222441. doi:10.1080/19420862.2023.2222441
Lang, R., Liu, G., Shi, Y., Bharadwaj, S., Leng, X., Zhou, X., et al. (2013). Self-renewal and differentiation capacity of urine-derived stem cells after urine preservation for 24 hours. PLoS One 8, e53980. doi:10.1371/journal.pone.0053980
Lau, C. S., Chua, J., Pena, E. M., Lim, J., Saigo, L., and Goh, B. T. (2022). A porcine model using adipose stem cell-loaded scaffolds for alveolar ridge augmentation. Tissue Eng. Part C Methods 28, 228–237. doi:10.1089/ten.tec.2022.0062
Lee, D.-S., Song, Y. J., Gug, H. R., Lee, J.-H., Bae, H. S., and Park, J.-C. J. S. C. I. (2022). Nuclear factor I-C regulates stemness genes and proliferation of stem cells in various mineralized tissue through epithelial-mesenchymal interactions in dental epithelial stem cells. Stem Cells 2022, 1092184. doi:10.1155/2022/1092184
Li, J., Luo, H., Dong, X., Liu, Q., Wu, C., Zhang, T., et al. (2017a). Therapeutic effect of urine-derived stem cells for protamine/lipopolysaccharide-induced interstitial cystitis in a rat model. Stem Cell Res. Ther. 8, 107–111. doi:10.1186/s13287-017-0547-9
Li, P., Lu, X., Deng, J., Peloso, A., and Zhang, Y. (2017b). “Urine progenitor cells for potential application in renal tissue repair,” in Kidney transplantation, bioengineering and regeneration (Elsevier).
Liu, Z.-W., Wang, X.-L., Xian, H.-J., Zhong, J.-H., Ye, X.-G., Yang, Y.-X., et al. (2024). Highly efficient malachite green adsorption by bacterial cellulose and bacterial cellulose/locust bean gum composite. Int. J. Biol. Macromol. 279, 134991. doi:10.1016/j.ijbiomac.2024.134991
Liu, G., David, B. T., Trawczynski, M., and Fessler, R. G. J. S. C. R. (2020a). Advances in pluripotent stem cells: history, mechanisms, technologies, and applications. Stem Cell Rev. Rep. 16, 3–32. doi:10.1007/s12015-019-09935-x
Liu, G., Pareta, R. A., Wu, R., Shi, Y., Zhou, X., Liu, H., et al. (2013). Skeletal myogenic differentiation of urine-derived stem cells and angiogenesis using microbeads loaded with growth factors. Biomaterials 34, 1311–1326. doi:10.1016/j.biomaterials.2012.10.038
Liu, G., Wu, R., Yang, B., Deng, C., Lu, X., Walker, S. J., et al. (2018). Human urine-derived stem cell differentiation to endothelial cells with barrier function and nitric oxide production. Stem Cells Transl. Med. 7, 686–698. doi:10.1002/sctm.18-0040
Liu, G., Wu, R., Yang, B., Shi, Y., Deng, C., Atala, A., et al. (2020b). A cocktail of growth factors released from a heparin hyaluronic-acid hydrogel promotes the myogenic potential of human urine-derived stem cells in vivo. stem cells vivo 107, 50–64. doi:10.1016/j.actbio.2020.02.005
Liu, X., Hu, H., Ma, J., and Wang, B. J. C. P. (2025). Mineralized cellulose nanofibers reinforced bioactive hydrogel remodels the osteogenic and angiogenic microenvironment for enhancing bone regeneration. Carbohydr. Polym. 357, 123480. doi:10.1016/j.carbpol.2025.123480
Liu, Y., Li, X., Fu, W., Liu, M., Wang, M., Hu, L., et al. (2021). An induced pluripotent stem cells line (ZZUNEUi022-A) derived from urine cells of healthy male human. Stem Cell Res. 51, 102191. doi:10.1016/j.scr.2021.102191
Liu, Y., Ma, W., Liu, B., Wang, Y., Chu, J., Xiong, G., et al. (2017). Urethral reconstruction with autologous urine-derived stem cells seeded in three-dimensional porous small intestinal submucosa in a rabbit model. Stem Cell Res. Ther. 8, 63–14. doi:10.1186/s13287-017-0500-y
Liu, Y., Wang, H., Dou, H., Tian, B., Li, L., Jin, L., et al. (2020c). Bone regeneration capacities of alveolar bone mesenchymal stem cells sheet in rabbit calvarial bone defect. J. Tissue Eng. 11, 2041731420930379. doi:10.1177/2041731420930379
Liu, Y., Zeng, Y., Si, H.-B., Tang, L., Xie, H.-Q., and Shen, B. J. T. A. J. O. S. M. (2022). Exosomes derived from human urine–derived stem cells overexpressing miR-140-5p alleviate knee osteoarthritis through downregulation of VEGFA in a rat model. Am. J. Sports Med. 50, 1088–1105. doi:10.1177/03635465221073991
Li, W., Chen, Z., Xu, W., Gao, Y., Liu, Z., Li, Q., et al. (2024). Prevalence of congenital cryptorchidism in China: a nationwide population-based surveillance study. Andrology 13 (3):577–586. doi:10.1111/andr.13686
Li, X., Liao, J., Su, X., Li, W., Bi, Z., Wang, J., et al. (2020). Human urine-derived stem cells protect against renal ischemia/reperfusion injury in a rat model via exosomal miR-146a-5p which targets IRAK1. Theranostics 10, 9561–9578. doi:10.7150/thno.42153
Lone, S. N., Nisar, S., Masoodi, T., Singh, M., Rizwan, A., Hashem, S., et al. (2022). Liquid biopsy: a step closer to transform diagnosis, prognosis and future of cancer treatments. Mol. Cancer 21, 79. doi:10.1186/s12943-022-01543-7
Lu, D., Xue, Y., Song, B., Liu, N., Xie, Y., Cheng, Y., et al. (2020). Generation of induced pluripotent stem cell GZLSL-i001-A derived from urine-derived cells of Hemophilia A patient with Inv22 mutation. Stem Cell Res. 49, 102053. doi:10.1016/j.scr.2020.102053
Lu, K., Cheng, B., Shi, Q., Gao, X.-J., and Li, C. J. B. M. D. (2022). Anterior cruciate ligament rupture in a patient with Albers-Schonberg disease. BMC Musculoskelet. Disord. 23, 719. doi:10.1186/s12891-022-05687-x
Lu, W., Zeng, M., Liu, W., Ma, T., Fan, X., Li, H., et al. (2023). Human urine-derived stem cell exosomes delivered via injectable GelMA templated hydrogel accelerate bone regeneration. Mat. Today Bio 19, 100569. doi:10.1016/j.mtbio.2023.100569
Marya, A., Rokaya, D., Heboyan, A., and Fernandes, G. V. O. (2022). Biomolecular and biochemical aspects of the oral cavity. MDPI 27, 8676. doi:10.3390/molecules27248676
Moonla, C., Lee, D. H., Rokaya, D., Rasitanon, N., Kathayat, G., Lee, W.-Y., et al. (2022). Review—lab-in-a-Mouth and advanced point-of-care sensing systems: detecting bioinformation from the oral cavity and saliva. ECS Sens. Plus 1, 021603. doi:10.1149/2754-2726/ac7533
Mulder, J., Sharmin, S., Chow, T., Rodrigues, D. C., Hildebrandt, M. R., D’Cruz, R., et al. (2020). Generation of infant-and pediatric-derived urinary induced pluripotent stem cells competent to form kidney organoids. Pediatr. Res. 87, 647–655. doi:10.1038/s41390-019-0618-y
Olaru, M., Sachelarie, L., and Calin, G. J. M. (2021). Hard dental tissues regeneration—approaches and challenges. Mater. (Basel) 14, 2558. doi:10.3390/ma14102558
Ooki, A., Vandenbussche, C. J., Kates, M., Hahn, N. M., Matoso, A., Mcconkey, D. J., et al. (2018). CD24 regulates cancer stem cell (CSC)-like traits and a panel of CSC-related molecules serves as a non-invasive urinary biomarker for the detection of bladder cancer. Br. J. Cancer 119, 961–970. doi:10.1038/s41416-018-0291-7
Ou, M., Li, C., Tang, D., Xue, W., Xu, Y., Zhu, P., et al. (2019). Genotyping, generation and proteomic profiling of the first human autosomal dominant osteopetrosis type II-specific induced pluripotent. Stem cells 10, 1–17. doi:10.1186/s13287-019-1369-8
Ouyang, B., Xie, Y., Zhang, C., Deng, C., Lv, L., Yao, J., et al. (2019). Extracellular vesicles from human urine-derived stem cells ameliorate erectile dysfunction in a diabetic rat model by delivering proangiogenic MicroRNA. microRNA 7, 241–250. doi:10.1016/j.esxm.2019.02.001
Park, J., Jeong, K., Kim, M., Kim, W., and Park, J. H. J. F. I. B. (2023). Enhanced osteogenesis of human urine-derived stem cells by direct delivery of 30Kc19α–Lin28A protein. Front. Bioeng. Biotechnol. 11, 1215087. doi:10.3389/fbioe.2023.1215087
Pei, M., Pei, Y. A., Zhou, S., Mikaeiliagah, E., Erickson, C., Giertych, B., et al. (2023). Matrix from urine stem cells boosts tissue-specific stem cell mediated functional cartilage reconstruction. Bioact. Mat. 23, 353–367. doi:10.1016/j.bioactmat.2022.11.012
Pizzuti, V., Balducelli, E., Di Nunzio, M., Conte, D., Gessaroli, E., Demetri, M., et al. (2024). Urine-derived Renal epithelial cells isolated after kidney transplant are sensitive to Neutrophil gelatinase-associated lipocalin exposure during in vitro culture. Eur. J. Cell Biol. 103, 151442. doi:10.1016/j.ejcb.2024.151442
Poetsch, M. S., Strano, A., and Guan, K. J. S. C. (2022). Human induced pluripotent stem cells: from cell origin, genomic stability, and epigenetic memory to translational medicine. Stem Cells 40, 546–555. doi:10.1093/stmcls/sxac020
Qin, H., Zhu, C., An, Z., Jiang, Y., Zhao, Y., Wang, J., et al. (2014). Silver nanoparticles promote osteogenic differentiation of human urine-derived stem cells at noncytotoxic concentrations. Int. J. Nanomedicine 9, 2469–2478. doi:10.2147/ijn.s59753
Rao, S., He, Z., Wang, Z., Yin, H., Hu, X., Tan, Y., et al. (2024). Extracellular vesicles from human urine-derived stem cells delay aging through the transfer of PLAU and TIMP1. Acta Pharm. Sin. B 14, 1166–1186. doi:10.1016/j.apsb.2023.12.009
Rokaya, D., Rokaya, N., Patel, J. N., Sah, A., Yadav, R. K., and Suttagul, K. (2022). Health problems and challenges for optimal health-care delivery in humla district, Nepal. J. Datta Meghe Inst. Med. Sci. Univ. 17, 616–619. doi:10.4103/jdmimsu.jdmimsu_85_18
Sato, M., Takizawa, H., Nakamura, A., Turner, B. J., Shabanpoor, F., and Aoki, Y. J. F. I. M. N. (2019). Application of urine-derived stem cells to cellular modeling in neuromuscular and neurodegenerative diseases. Front. Mol. Neurosci. 12, 297. doi:10.3389/fnmol.2019.00297
Schosserer, M., Reynoso, R., Wally, V., Jug, B., Kantner, V., Weilner, S., et al. (2015). Urine is a novel source of autologous mesenchymal stem cells for patients with epidermolysis bullosa. BMC Res. Notes 8, 767–812. doi:10.1186/s13104-015-1686-7
Selvido, D. I., Skallevold, H. E., Bhattarai, B. P., Dashaputra, R., Syed, A. U. Y., and Rokaya, D. J. P. S. I. N. O. C. (2023). Alveolar bone protective effects of natural products, 299–310.
Shah, J., Kim, H., Sivamurthy, K., Monahan, P. E., and Fries, M. J. C. M. R. (2023). Comprehensive analysis and prediction of long-term durability of factor IX activity following etranacogene dezaparvovec gene therapy in the treatment of hemophilia B. Curr. Med. Res. Opin. 39, 227–237. doi:10.1080/03007995.2022.2133492
Sharma, A. K., Bury, M. I., Fuller, N. J., Marks, A. J., Kollhoff, D. M., Rao, M. V., et al. (2013). Cotransplantation with specific populations of spina bifida bone marrow stem/progenitor cells enhances urinary bladder regeneration. Proc. Natl. Acad. Sci. U. S. A. 110, 4003–4008. doi:10.1073/pnas.1220764110
Shen, Z., Kuang, S., Zhang, Y., Yang, M., Qin, W., Shi, X., et al. (2020). Chitosan hydrogel incorporated with dental pulp stem cell-derived exosomes alleviates periodontitis in mice via a macrophage-dependent mechanism. Bioact. Mat. 5, 1113–1126. doi:10.1016/j.bioactmat.2020.07.002
Shi, L., Cui, Y., Luan, J., Zhou, X., Han, J. J. I., and Research, R. D. (2016). Urine-derived induced pluripotent stem cells as a modeling tool to study rare human diseases. Intractable Rare Dis. Res. 5, 192–201. doi:10.5582/irdr.2016.01062
Shi, T., and Cheung, M. J. C. (2021). Urine-derived induced pluripotent/neural stem cells for modeling neurological diseases. BIOSCIENCE 11, 85. doi:10.1186/s13578-021-00594-5
Shi, Y., Liu, G., Shantaram, B., Atala, A., and Zhang, Y. J. T. J. O. U. (2012). 736 Urine derived stem cells with high telomerase activity for cell based therapy in urology. J. Urology, 187, e302. doi:10.1016/j.juro.2012.02.821
Shi, Y., Liu, G., Wu, R., Mack, D. L., Sun, X. S., Maxwell, J., et al. (2022). Differentiation capacity of human urine-derived stem cells to retain telomerase activity. Front. Cell Dev. Biol. 10, 890574. doi:10.3389/fcell.2022.890574
Song, Y.-T., Dong, L., Hu, J.-G., Liu, P.-C., Jiang, Y.-L., Zhou, L., et al. (2023). Application of genipin-crosslinked small intestine submucosa and urine-derived stem cells for the prevention of intrauterine adhesion in a rat model. Compos. Part B Eng., 250, 110461. doi:10.1016/j.compositesb.2022.110461
Strässler, E. T., Aalto-Setälä, K., Kiamehr, M., Landmesser, U., and Kränkel, N. J. F. I. C. M. (2018). Age is relative—impact of donor age on induced pluripotent stem cell-derived cell functionality. Front. Cardiovasc. Med. 5, 4. doi:10.3389/fcvm.2018.00004
Sun, B., Luo, X., Yang, C., Liu, P., Yang, Y., Dong, X., et al. (2019). Therapeutic effects of human urine-derived stem cells in a rat model of cisplatin-induced acute kidney injury in vivo and in vitro. Stem Cells Int. 2019, 1–13. doi:10.1155/2019/8035076
Sun, J., Li, L., Xing, F., Yang, Y., Gong, M., Liu, G., et al. (2021a). Graphene oxide-modified silk fibroin/nanohydroxyapatite scaffold loaded with urine-derived stem cells for immunomodulation and bone regeneration. Stem Cell Res. Ther. 12, 591–620. doi:10.1186/s13287-021-02634-w
Sun, J., Xing, F., Zou, M., Gong, M., Li, L., and Xiang, Z. J. S. C. R. (2021b). Comparison of chondrogenesis-related biological behaviors between human urine-derived stem cells and human bone marrow mesenchymal stem cells from the same individual. Stem Cell Res. Ther. 12, 366–419. doi:10.1186/s13287-021-02370-1
Sun, J., Xing, F., Zou, M., Gong, M., Li, L., and Xiang, Z. J. S. C. R. (2022a). Correction: comparison of chondrogenesis-related biological behaviors between human urine-derived stem cells and human bone marrow mesenchymal stem cells from the same individual. Stem Cell Res. Ther. 13, 514. doi:10.1186/s13287-022-03193-4
Sun, Z., Wu, J., Bi, Q., and Wang, W. J. S. C. R. (2022b). Exosomal lncRNA TUG1 derived from human urine-derived stem cells attenuates renal ischemia/reperfusion injury by interacting with SRSF1 to regulate ASCL4-mediated ferroptosis. Stem Cell Res. Ther. 13, 297. doi:10.1186/s13287-022-02986-x
Supakul, S., Hatakeyama, Y., Leventoux, N., Itsuno, M., Numata, N., Hiramine, H., et al. (2023). Urine-derived cells from the aged donor for the 2D/3D modeling of neural cells via iPSCs. Aging Brain 4, 100101. doi:10.1016/j.nbas.2023.100101
Sutherland, G. R., and Bain, A. D. J. N. (1972). Culture of cells from the urine of newborn children. Nature 239, 231. doi:10.1038/239231a0
Teles E Silva, A. L., Yokota, B. Y., Sertié, A. L., and Zampieri, B. L. J. S. C. R. (2023). Generation of urine-derived induced pluripotent stem cells and cerebral organoids for modeling down syndrome. Stem Cell Rev. Rep. 19, 1116–1123. doi:10.1007/s12015-022-10497-8
Tian, S. F., Jiang, Z. Z., Liu, Y. M., Niu, X., Hu, B., Guo, S. C., et al. (2017). Human urine-derived stem cells contribute to the repair of ischemic acute kidney injury in rats. Mol. Med. Rep. 16, 5541–5548. doi:10.3892/mmr.2017.7240
Vander Borght, M., and Wyns, C. J. C. B. (2018). Fertility and infertility: definition and epidemiology. Clin. Biochem. 62, 2–10. doi:10.1016/j.clinbiochem.2018.03.012
Volarevic, V., Bojic, S., Nurkovic, J., Volarevic, A., Ljujic, B., Arsenijevic, N., et al. (2014). Stem cells as new agents for the treatment of infertility: current and future perspectives and challenges. Biomed. Res. Int. 2014, 1–8. doi:10.1155/2014/507234
Wang, C., Hei, F., Ju, Z., Yu, J., Yang, S., and Chen, M. J. C. R. (2016). Differentiation of urine-derived human induced pluripotent stem cells to alveolar type II epithelial cells. Cell. Reprogr. 18, 30–36. doi:10.1089/cell.2015.0015
Wang, L., Wang, L., Huang, W., Su, H., Xue, Y., Su, Z., et al. (2013). Generation of integration-free neural progenitor cells from cells in human urine. Nat. Methods 10, 84–89. doi:10.1038/nmeth.2283
Wang, Y., Li, H., Fan, X., Ma, T., Lu, W., Hu, Y., et al. (2024). Role of miR-21-5p in the protective effects of PLGA-encapsulated extracellular vesicles from urine-derived stem cells on periprosthetic osteolysis. Appl. Mat. Today 38, 102205. doi:10.1016/j.apmt.2024.102205
Wan, Q., Xiong, G., Liu, G., Shupe, T. D., Wei, G., Zhang, D., et al. (2018). Urothelium with barrier function differentiated from human urine-derived stem cells for potential use in urinary tract reconstruction. Stem Cell Res. Ther. 9, 304–313. doi:10.1186/s13287-018-1035-6
Wan, S., Bao, D., Li, J., Lin, K., Huang, Q., Li, Q., et al. (2022). Extracellular vesicles from hypoxic pretreated urine-derived stem cells enhance the proliferation and migration of chondrocytes by delivering miR-26a-5p. Cartilage 13, 19476035221077401. doi:10.1177/19476035221077401
Wu, R., Luo, S., Yang, H., Hu, X., Lin, A., Pan, G., et al. (2020). Transplantation of neural progenitor cells generated from human urine epithelial cell-derived induced pluripotent stem cells improves neurological functions in rats with stroke. Discov. Med. 29, 53–64. doi:10.1186/s13578-021-00594-5
Wu, S., Liu, Y., Bharadwaj, S., Atala, A., and Zhang, Y. J. B. (2011). Human urine-derived stem cells seeded in a modified 3D porous small intestinal submucosa scaffold for urethral tissue engineering. Biomaterials 32, 1317–1326. doi:10.1016/j.biomaterials.2010.10.006
Xiang, H., Su, W., Wu, X., Chen, W., Cong, W., Yang, S., et al. (2020). Exosomes derived from human urine-derived stem cells inhibit intervertebral disc degeneration by ameliorating endoplasmic reticulum stress. Oxid. Med. Cell. Longev. 2020, 1–21. doi:10.1155/2020/6697577
Xie, J., Hu, Y., Li, H., Wang, Y., Fan, X., Lu, W., et al. (2023). Targeted therapy for peri-prosthetic osteolysis using macrophage membrane-encapsulated human urine-derived stem cell extracellular vesicles. Acta Biomater. 160, 297–310. doi:10.1016/j.actbio.2023.02.003
Xing, F., Yin, H.-M., Zhe, M., Xie, J.-C., Duan, X., Xu, J.-Z., et al. (2022). Nanotopographical 3D-Printed poly (ε-caprolactone) scaffolds enhance proliferation and osteogenic differentiation of urine-derived stem cells for bone regeneration. Pharmaceutics 14, 1437. doi:10.3390/pharmaceutics14071437
Xiong, G., Tao, L., Ma, W.-J., Gong, M.-J., Zhao, L., Shen, L.-J., et al. (2020). Urine-derived stem cells for the therapy of diabetic nephropathy mouse model. Eur. Rev. Med. Pharmacol. Sci. 24, 1316–1324. doi:10.26355/eurrev_202002_20189
Xiong, X., Yang, X., Dai, H., Feng, G., Zhang, Y., Zhou, J., et al. (2019). Extracellular matrix derived from human urine-derived stem cells enhances the expansion, adhesion, spreading, and differentiation of human periodontal ligament stem cells. Stem Cell Res. Ther. 10, 1–16. doi:10.1186/s13287-019-1483-7
Xu, X., Lai, Y., and Hua, Z.-C. J. B. R. (2019). Apoptosis and apoptotic body: disease message and therapeutic target potentials. Biosci. Rep. 39, BSR20180992. doi:10.1042/BSR20180992
Yang, B., Wang, J., Qiao, J., Zhang, Q., Liu, Q., Tan, Y., et al. (2024). Circ DENND4C inhibits pyroptosis and alleviates ischemia-reperfusion acute kidney injury by exosomes secreted from human urine-derived stem cells. stem cells 391, 110922. doi:10.1016/j.cbi.2024.110922
Yang, H., Chen, B., Deng, J., Zhuang, G., Wu, S., Liu, G., et al. (2018). Characterization of rabbit urine-derived stem cells for potential application in lower urinary tract tissue regeneration. Cell Tissue Res. 374, 303–315. doi:10.1007/s00441-018-2885-z
Yang, X., Xiong, X., Zhou, W., Feng, G., Zhang, Y., Dai, H., et al. (2020). Effects of human urine-derived stem cells on the cementogenic differentiation of indirectly-cocultured periodontal ligament stem cells. stem cells 12, 361–378.
Yau, G. T. Y., Tai, W., Arnold, J. C., Chan, H.-K., and Kwok, P. C. L. J. P. R. (2023). Cannabidiol for the treatment of brain disorders: therapeutic potential and routes of administration. Pharm. Res. 40, 1087–1114. doi:10.1007/s11095-023-03469-1
Yea, J.-H., Bae, T. S., Kim, B. J., Cho, Y. W., and Jo, C. H. J. A. B. (2020). Regeneration of the rotator cuff tendon-to-bone interface using umbilical cord-derived mesenchymal stem cells and gradient extracellular matrix scaffolds from adipose tissue in a rat model. Acta Biomater. 114, 104–116. doi:10.1016/j.actbio.2020.07.020
Yi, H., Xie, B., Liu, B., Wang, X., Xu, L., Liu, J., et al. (2018). Derivation and identification of motor neurons from human urine-derived induced pluripotent stem cells. Stem Cells Int. 2018, 3628578. doi:10.1155/2018/3628578
Yu, H. Y., Shin, J. H., Yun, H., Ryu, C.-M., Lee, S., Heo, J., et al. (2021). A preclinical study of human embryonic stem cell-derived mesenchymal stem cells for treating detrusor underactivity by chronic bladder ischemia. Stem Cell Rev. Rep. 17, 2139–2152. doi:10.1007/s12015-021-10204-z
Zeng, J., Huang, L., Xiong, H., Li, Q., Wu, C., Huang, Y., et al. (2022). Injectable decellularized cartilage matrix hydrogel encapsulating urine-derived stem cells for immunomodulatory and cartilage defect regeneration. npj Regen. Med. 7, 75. doi:10.1038/s41536-022-00269-w
Zha, K., Li, X., Yang, Z., Tian, G., Sun, Z., Sui, X., et al. (2021). Heterogeneity of mesenchymal stem cells in cartilage regeneration: from characterization to application. npj Regen. Med. 6, 14. doi:10.1038/s41536-021-00122-6
Zhang, X.-R., Huang, Y.-Z., Gao, H.-W., Jiang, Y.-L., Hu, J.-G., Pi, J.-K., et al. (2020b). Hypoxic preconditioning of human urine-derived stem cell-laden small intestinal submucosa enhances wound healing potential. Stem Cell Res. Ther. 11, 150–213. doi:10.1186/s13287-020-01662-2
Zhang, C., George, S. K., Wu, R., Thakker, P. U., Abolbashari, M., Kim, T.-H., et al. (2020a). Reno-protection of urine-derived stem cells in a chronic kidney disease rat model induced by renal ischemia and nephrotoxicity. Int. J. Biol. Sci. 16, 435–446. doi:10.7150/ijbs.37550
Zhang, N., Zhao, L., Liu, D., Hu, C., Wang, Y., He, T., et al. (2021). Characterization of urine-derived stem cells from patients with end-stage liver diseases and application to induced acute and chronic liver injury of nude mice model. Stem Cells Dev. 30, 1126–1138. doi:10.1089/scd.2021.0137
Zhang, Y., and Atala, A. (2022). “Nephroprotective effect of urine-derived stem cells for renal injury,” in Regenerative nephrology (Elsevier).
Zhang, Y., Mcneill, E., Tian, H., Soker, S., Andersson, K.-E., Yoo, J. J., et al. (2008). Urine derived cells are a potential source for urological tissue reconstruction. J. Urol. 180, 2226–2233. doi:10.1016/j.juro.2008.07.023
Zhang, Y., Niu, X., Dong, X., Wang, Y., Li, H. J. J. O. T. E., and Medicine, R. (2018). Bioglass enhanced wound healing ability of urine-derived stem cells through promoting paracrine effects between stem cells and recipient cells. J. Tissue Eng. Regen. Med. 12, e1609–e1622. doi:10.1002/term.2587
Zhang, Y., Wang, J., Yang, B., Qiao, R., Li, A., Guo, H., et al. (2020c). Transfer of MicroRNA-216a-5p from exosomes secreted by human urine-derived stem cells reduces renal ischemia/reperfusion injury. Reperfus. Inj. 8, 610587. doi:10.3389/fcell.2020.610587
Zhankina, R., Baghban, N., Askarov, M., Saipiyeva, D., Ibragimov, A., Kadirova, B., et al. (2021). Mesenchymal stromal/stem cells and their exosomes for restoration of spermatogenesis in non-obstructive azoospermia: a systemic review. Stem Cell Res. Ther. 12, 229–312. doi:10.1186/s13287-021-02295-9
Zhao, A. G., Shah, K., Cromer, B., and Sumer, H. J. E. C. R. (2022). Comparative analysis of extracellular vesicles isolated from human mesenchymal stem cells by different isolation methods and visualisation of their uptake. Exp. Cell Res. 414, 113097. doi:10.1016/j.yexcr.2022.113097
Zhao, T., Luo, D., Sun, Y., Niu, X., Wang, Y., Wang, C., et al. (2018). Human urine-derived stem cells play a novel role in the treatment of STZ-induced diabetic mice. J. Mol. Histol. 49, 419–428. doi:10.1007/s10735-018-9772-5
Zhong, J., Wang, H., Yang, K., Wang, H., Duan, C., Ni, N., et al. (2022). Reversibly immortalized keratinocytes (iKera) facilitate re-epithelization and skin wound healing: potential applications in cell-based skin tissue engineering. Bioact. Mat. 9, 523–540. doi:10.1016/j.bioactmat.2021.07.022
Zhou, J., Wang, X., Zhang, S., Gu, Y., Yu, L., Wu, J., et al. (2013). Generation and characterization of human cryptorchid-specific induced pluripotent stem cells from urine. Stem Cells Dev. 22, 717–725. doi:10.1089/scd.2012.0260
Zhou, M., Shen, L., Qiao, Y., and Sun, Z. J. J. O. C. B. (2020). Inducing differentiation of human urine-derived stem cells into hepatocyte-like cells by coculturing with human hepatocyte L02 cells. J. Cell. Biochem. 121, 566–573. doi:10.1002/jcb.29301
Zhou, T., Benda, C., Dunzinger, S., Huang, Y., Ho, J. C., Yang, J., et al. (2012). Generation of human induced pluripotent stem cells from urine samples. Nat. Protoc. 7, 2080–2089. doi:10.1038/nprot.2012.115
Zhuang, G., Wen, Y., Briggs, M., Shao, Q., Tran, D., Wang, H., et al. (2021). Secretomes of human pluripotent stem cell-derived smooth muscle cell progenitors upregulate extracellular matrix metabolism in the lower urinary tract and vagina. Stem Cell Res. Ther. 12, 228–315. doi:10.1186/s13287-021-02292-y
Keywords: regenerative medicine, non-invasive cell therapy, disease modeling, cartilage repair, exosomes, biomaterials, tissue engineering
Citation: Atia GA, Abdal Dayem A, Taher ES, Alghonemy WY, Cho S-G, Aldarmahi AA, Haque MA, Alshambky A, Taymour N, Ibrahim AM, Zaghamir DE, Elmorsy EM, Hetta HF, Mohamed ME, Abass KS, Khanday S and Abdeen A (2025) Urine-derived stem cells: a sustainable resource for advancing personalized medicine and dental regeneration. Front. Bioeng. Biotechnol. 13:1571066. doi: 10.3389/fbioe.2025.1571066
Received: 05 February 2025; Accepted: 07 April 2025;
Published: 28 April 2025.
Edited by:
Maria Angelica Miglino, Universidade de Marília, BrazilReviewed by:
Vinod Reddy Lekkala, University of California, San Francisco, United StatesHao Tian, Army Medical University, China
Copyright © 2025 Atia, Abdal Dayem, Taher, Alghonemy, Cho, Aldarmahi, Haque, Alshambky, Taymour, Ibrahim, Zaghamir, Elmorsy, Hetta, Mohamed, Abass, Khanday and Abdeen. This is an open-access article distributed under the terms of the Creative Commons Attribution License (CC BY). The use, distribution or reproduction in other forums is permitted, provided the original author(s) and the copyright owner(s) are credited and that the original publication in this journal is cited, in accordance with accepted academic practice. No use, distribution or reproduction is permitted which does not comply with these terms.
*Correspondence: Ssang-Goo Cho, c3Nhbmdvb0Brb25rdWsuYWMua3I=; Md Azizul Haque, YXppenVsQHludS5hYy5rcg==; Shifan Khanday, ZHIuc2hpZmFuQGRtY2cuYWU=
†These authors have contributed equally to this work