- 1Department of Ophthalmology and Visual Sciences, Vanderbilt Eye Institute, Vanderbilt University Medical Center, Nashville, TN, United States
- 2Department of Cell and Developmental Biology, Vanderbilt University Medical School, Nashville, TN, United States
Microphthalmia, anophthalmia, and coloboma (MAC) are congenital ocular malformations causing 25% of childhood blindness. The X-linked disorder Focal Dermal Hypoplasia (FDH) is frequently associated with MAC and results from mutations in Porcn, a membrane bound O-acyl transferase required for palmitoylation of Wnts to activate multiple Wnt-dependent pathways. Wnt/β-catenin signaling is suppressed in the anterior neural plate for initiation of eye formation and is subsequently required during differentiation of the retinal pigment epithelium (RPE). Non-canonical Wnts are critical for early eye formation in frog and zebrafish. However, it is unclear whether this also applies to mammals. We performed ubiquitous conditional inactivation of Porcn in mouse around the eye field stage. In PorcnCKO, optic vesicles (OV) arrest in growth and fail to form an optic cup. Ventral proliferation is significantly decreased in the mutant OV, with a concomitant increase in apoptotic cell death. While pan-ocular transcription factors such as PAX6, SIX3, LHX2, and PAX2 are present, indicative of maintenance of OV identity, regional expression of VSX2, MITF, OTX2, and NR2F2 is downregulated. Failure of RPE differentiation in PorcnCKO is consistent with downregulation of the Wnt/β-catenin effector LEF1, starting around 2.5 days after inactivation. This suggests that Porcn inactivation affects signaling later than a potential requirement for Wnts to promote eye field formation. Altogether, our data shows a novel requirement for Porcn in regulating growth and morphogenesis of the OV, likely by controlling proliferation and survival. In FDH patients with ocular manifestations, growth deficiency during early ocular morphogenesis may be the underlying cause for microphthalmia.
Introduction
Congenital ocular malformations—microphthalmia (small eye), anophthalmia (absent eye, also called extreme microphthalmia), coloboma (optic fissure closure defect in the ventral optic cup) (collectively hereafter MAC)—originate from defective morphogenesis during early eye development and cause 25% of childhood blindness (Clementi et al., 1992; Morrison et al., 2002; Graw, 2019). Eye development is initiated during gastrulation in a single domain in the anterior neural plate, the eye field, characterized by expression of eye field transcription factors (EFTFs) that include Pax6, Six3, Six6, Rx, Tbx3, and Lhx2 (Oliver et al., 1995; Zuber et al., 2003; Bailey et al., 2004; Motahari et al., 2016; Liu and Cvekl, 2017). In combination with secreted factors inhibiting Nodal, BMP and Wnt/β-catenin signaling, EFTFs form a gene regulatory network to promote eye field specification (Zuber et al., 2003). Subsequentially, the eye field separates into two optic pits that evaginate laterally. Bilateral optic vesicles (OVs) are formed, comprised of neuroepithelial progenitor cells giving rise to optic stalk, retina and RPE. The distal domain of the OV contacts the overlying surface (lens) ectoderm, and both OV and ectoderm invaginate to form the optic cup (OC) and the lens vesicle, respectively. While the coordinated interactions regulating regionalization of OC and OV are well studied [for reviews, see (Fuhrmann, 2010; Viczian, 2014; Miesfeld and Brown, 2019)], regulation of the morphogenetic events during OV evagination and OC invagination are less understood.
Focal Dermal Hypoplasia (FDH; Goltz Syndrome) is an x-linked dominant disorder resulting from abnormal mesodermal and ectodermal development and is frequently associated with MAC (Goltz et al., 1962; Temple et al., 1990; Wang et al., 2014; Gisseman and Herce, 2016). FDH is caused by mutations in Porcn, a membrane bound O-acyl transferase localized to the endoplasmic reticulum (Grzeschik et al., 2007; Wang et al., 2007). Porcn is mostly dedicated to palmitoylate the Wnt family of cysteine-rich secreted glycoproteins, and this modification is necessary for trafficking and signaling (Tanaka et al., 2000; Galli et al., 2007). Porcn is expressed in the developing mouse eye (Biechele et al., 2011). We have shown that conditional Porcn disruption at the OV stage results in coloboma and RPE defects in most embryos, among other abnormalities (Bankhead et al., 2015).
Wnt ligands bind to surface receptors, including Frizzled transmembrane receptors (Fzd), and activate canonical (Wnt/β-catenin) and non-canonical pathways. Wnt/β-catenin activation prevents cytoplasmic degradation of β-catenin, thereby allowing its nuclear translocation to activate Tcf/Lef1 transcription factors. Wnt/β-catenin exerts many distinct functions during different phases of eye development [for reviews, see (Fuhrmann, 2008; Fujimura, 2016)]. During early OC morphogenesis, it is required for RPE differentiation (Fujimura et al., 2009; Westenskow et al., 2009; Hagglund et al., 2013) and dorsoventral patterning (Veien et al., 2008; Zhou et al., 2008). The pathway is tightly regulated; ectopic activation by disruption of the antagonists Dkk1 and Axin2 can result in microphthalmia and coloboma (Lieven and Ruther, 2011; Alldredge and Fuhrmann, 2016). During forebrain development, multiple antagonists maintain anterior neural fate. Hyperactive Wnt/β-catenin frequently leads to posterization and rostral truncation of the forebrain, with concomitant severe decrease of EFTF expression. The EFTF Six3 can directly suppress Wnt/β-catenin signaling (Lagutin et al., 2003), providing another mechanism for precise coordination of pathway activity.
Non-canonical, β-catenin-independent Wnt signaling occurs via multiple pathways, including intracellular Ca2+ release, activation of JNK and Planar Cell Polarity (PCP). It is essential for eye field formation in non-mammalian vertebrates by different mechanisms; it represses Wnt/β-catenin activity and promotes expression of EFTFs in frog and zebrafish (for review, see (Fuhrmann, 2008)). Close to the caudal border of the eye field, non-canonical Wnt11f2 (formerly Wnt11) and Wnt4 act through Fzd5 and Fzd3 to promote expression of the EFTFs Pax6 and Rx (Rasmussen et al., 2001; Cavodeassi et al., 2005; Maurus et al., 2005). In addition, Wnt11f2 and crosstalk between JNK/PCP and ephrinB1 are required for morphogenetic movements of ocular progenitor cells into the eye field (Heisenberg et al., 2000; Moore et al., 2004; Cavodeassi et al., 2005; Lee et al., 2006; Cavodeassi et al., 2013). However, it is unknown whether non-canonical Wnt signaling functions similarly in mammals; for example, germline disruption of Wnt4, Wnt11, Fzd3 does not cause obvious ocular phenotypes, suggesting either redundancy of pathway components and/or context- and species-dependent mechanisms.
To investigate the role of Porcn before OV morphogenesis, we performed temporally controlled inactivation before and during the eye field stage when Wnts are expressed in the cranial region (Parr et al., 1993; Kispert et al., 1996; Yamaguchi et al., 1999; Kemp et al., 2005). Our results show that Porcn inactivation around eye field formation leads to severely arrested OV 3 days later. Key regulatory genes for RPE differentiation OTX2, MITF, and NR2F2 are absent, proliferation and survival of ocular progenitors in the OV is decreased, and invagination during OC morphogenesis fails. Our studies reveal a novel role for Porcn during earliest stages of mouse eye development, recapitulating severe microphthalmia in FDH.
Materials and methods
Mouse lines were maintained on a mixed genetic C57BL/6 and CD-1 background. For temporally controlled Porcn inactivation, a conditional Porcn allele, tamoxifen-inducible, ubiquitous Gt(ROSA)26Sortm1(cre/ERT)Nat (hereafter ROSA26CreERT) and the recombination reporter RosaR26 were utilized, with established genotyping protocols or Transnetyx (Cordova, TN) using Taqman with custom-designed primers (Soriano, 1999; Badea et al., 2003; Barrott et al., 2011; Bankhead et al., 2015; Sun et al., 2020). Noon of the day with an observed vaginal plug was counted E0.5. Pregnant dams received tamoxifen (0.1 mg/g) by oral gavage between E6.5 and E7.5 (Park et al., 2008). To detect proliferating cells, pregnant dams received one intraperitoneal EdU injection 2 hours before sacrificing (30 μg/g; Thermofisher/Invitrogen; #E10187). Male embryos with conditional deletion of Porcn (hereafter PorcnCKO) and control littermates were processed as previously published (Sun et al., 2020). For antigen retrieval, cryostat sections were treated with 1% Triton X-100 or hot citrate buffer (pH 6). Detailed antibody information is provided in Supplementary Table S1. Filamentous actin was detected using Phalloidin (1:50; Thermo Fisher Scientific; #A12379). ApopTag Fluorescein In Situ Apoptosis Detection Kit (EMD Millipore, #S7110) was used to detect apoptotic cells. For EdU detection, the Click-iT® EdU Imaging Kit (Thermo Fisher Scientific; #C10637) was utilized. Cryostat sections were counter-labeled with DAPI and mounted in Prolong Gold Antifade. No obvious defects in morphology, proliferation or OV marker expression were observed in controls: conditional heterozygous female embryos (hereafter PorcnCHET) and embryos with or without Cre. Unless otherwise indicated, a minimum of 3 embryos from at least 2 individual litters were analyzed per genotype, time point and marker. For analyses of E9.5 embryos induced at E6.5, 3 embryos were analyzed and 2 showed indication of ocular development.
Images were captured using a U-CMAD3 camera, mounted on an Olympus SZX12 stereomicroscope, and a XM10 camera, mounted on an Olympus BX51 epifluorescence system. For confocal imaging, the Olympus FV100 or ZEISS LSM 880 systems were used. Images were processed using ImageJ (NIH) and Adobe Photoshop software (versions CS6, 23.5.1).
Quantification of EdU-labeled and Tunel-labeled cells was performed on cryostat sections midway through the OV, with separation of dorsal and ventral subdivisions. The percentage of labeled cells was calculated by determining the number of total cells using DAPI-labeled nuclei. Statistical analysis was performed using Prism version 9 (Graphpad) for One-Way ANOVA.
Results
Conditional Porcn inactivation before eye field induction (E6.5) causes abnormal OV morphogenesis
We observed that Porcn needs to be depleted in multiple ocular and extraocular tissues at the OV stage to recapitulate consistently the ocular abnormalities found in FDH patients (Bankhead et al., 2015). In mouse, the eye field becomes established at E7.5, and Wnts are expressed in tissues adjacent to the eye field. To target both cell autonomous and non-cell autonomous Wnt production, we disrupted Porcn using the ubiquitous ROSA26CreERT, allowing temporally controlled recombination. To account for delay of pathway inactivation in responding cells due to cell non-autonomous Wnt ligand production, extracellular release and downstream receptor activation, we started inactivating Porcn at E6.5. RosaR26 recombination confirmed ubiquitous Cre activity, as expected (Supplementary Figure S1) (Soriano, 1999). At E8.5–8.75, PorcnCKO show severe posterior truncation (Barrott et al., 2011; Biechele et al., 2013). However, anterior regions do continue to develop, with reduced size of OVs (Figures 1B–H; 8–13 somites). In PorcnCKO, expression of the Wnt/β-catenin readout LEF1 can be slightly decreased in the dorsal OV, consistent with decreased Wnt signaling (Figure 1D). Lhx2 is required for the transition from eye field to OV and is robustly expressed in PorcnCKO (Figure 1F). Otx2 is critical for rostral brain regionalization and early ocular development. In PorcnCKO, OTX2 expression is present in the dorsal OV (Figure 1H). To determine whether the observed alterations could be due to a developmental delay, we examined PorcnCKO embryos 1–2 days later. We observed major developmental abnormalities on E10.5 PorcnCKO embryos, not suitable for further analysis (Supplementary Figure S2). Thus, we continued to examine embryos at E9.5 (20–23 somites); PorcnCKO exhibit more defects compared to E8.5–8.75, including abnormalities in the head region (Figures 1J,K). Morphogenesis of the OVs does not proceed properly; they are arrested and fail to contact the surface ectoderm (Figure 1M,O,Q,S). Expression of LEF1 is absent in the dorsal OV, indicating loss of Wnt/β-catenin signaling (Figure 1M). Phalloidin labeling reveals that apicobasal polarity is generally preserved (Figure 1O). Thus, it is possible that non-canonical Wnt pathways such as planar cell polarity are largely maintained. Robust LHX2 expression is maintained in PorcnCKO (Figure 1Q). In contrast, OTX2 is downregulated in the dorsal OV, while weak expression is detectable in the adjacent forebrain (Figure 1S). Overall, our results demonstrate that Porcn inactivation starts to affect eye development after approx. 2.5 days, with slightly decreased Wnt/β-catenin signaling in the dorsal OV. Evagination can occur, suggesting that OV identity is maintained, and morphogenesis initiated. However, with ongoing loss of Porcn at E9.5, dorsal regionalization and expansion of the OV is severely affected.
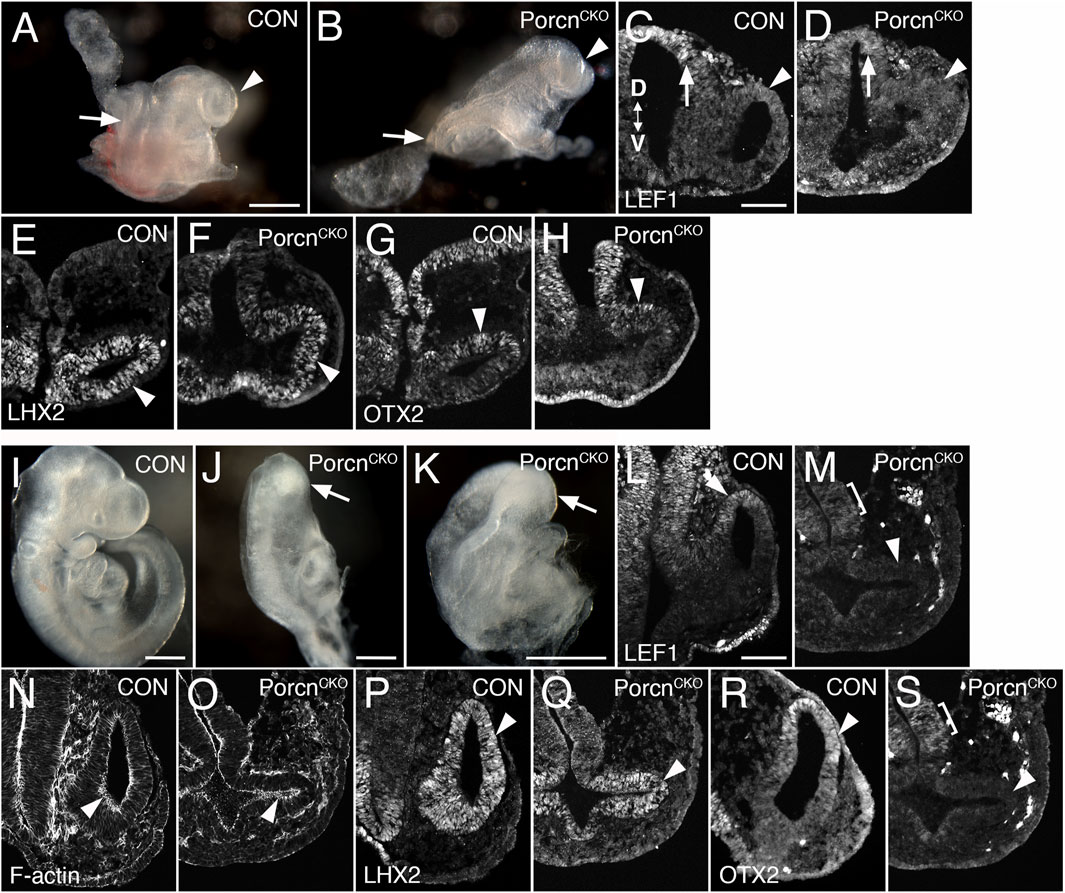
FIGURE 1. Conditional Porcn inactivation before eye field formation (E6.5) interferes with early OV morphogenesis. (A–H) Embryos at E8.75-E9.0. (A) Control embryo (8 somites) with OVs (arrowhead). (B) PorcnCKO with caudal truncation (arrow; somites not detectable). Head development proceeds at this stage and OV-like structures are present (arrowhead). (C and D) LEF1 expression in 13 somite embryos. Coronal cryostat sections show slightly decreased LEF1 expression in the dorsal OV of PorcnCKO (D; arrowhead), expression in the dorsal and ventral forebrain is largely maintained (D; arrow). (E–H): 7-8 somite embryos. (F) LHX2 is robustly expressed in the mutant OV (arrowhead). (H) In PorcnCKO, OTX2 expression is present in the dorsal OV (arrowhead). (I–S) Embryos at E9.5 (20–23 somites); 2 of 3 embryos showed distinct OVs, shown here in (J–S). (J–K) Examples of PorcnCKO showing severe abnormalities throughout the body, including the heads (arrows). (M) LEF1 expression is absent in PorcnCKO OV (arrowhead), low expression is detectable in the adjacent diencephalon (bracket). (O) Phalloidin labeling shows that apicobasal polarity is largely maintained in PorcnCKO (arrowhead). (Q) In the mutant OV, LHX2 is robustly expressed, similar to controls (arrowhead). (S) OTX2 is absent in the OV of PorcnCKO (arrowhead). Weak expression is detectable in the forebrain neuroepithelium (bracket). Controls: PorcnCHET. OV: optic vesicle. D < - > V: Dorsoventral orientation. Scale bars A, B, I–K: 0.5 mm; C, L: 0.1 mm.
Porcn deletion during eye field induction (E7.5) variably affects brain and eye formation
To bypass major developmental defects before OV morphogenesis (Figure 1), we administered tamoxifen 1 day later (E7.4–7.5). At E10.5, PorcnCKO embryos were recovered with a range of ocular abnormalities, possibly due to developmental variability between litters and embryos at the time of tamoxifen administration (Figure 2; 9 litters with 26 PorcnCKO embryos). In 5 PorcnCKO, no detectable eye phenotype was observed (19%; not shown). In mildly affected PorcnCKO, telencephalic vesicles are slightly smaller (Figure 2F). Eye size can be reduced, associated with incomplete OC morphogenesis, failure of lens vesicle formation and accumulation of POM between distal OV and surface ectoderm (Figures 2G–J; 27%, n = 7). In mildly affected PorcnCKO, OVs can be more closely associated with lens ectoderm, occasionally with further advanced invagination (not shown). We also observed PorcnCKO embryos with a more severe developmental and ocular phenotype (Figures 2K–S; 54%, n = 14). These PorcnCKO embryos exhibit defects in mid- and forebrain development; the mid-hindbrain border is missing and telencephalic vesicles are underdeveloped (Figure 2K). Ocular morphogenesis is consistently arrested with small OVs that fail to expand, with abnormal accumulation of anterior POM preventing close contact between distal OV and lens ectoderm (Figure 2L–O). Consequently, neither the OV nor the surface ectoderm show any signs of invagination (Figure 2M–O). Some thickening of the surface ectoderm is occasionally detectable, suggesting that early aspects of lens morphogenesis can be initiated (Figure 2M). In PorcnCKO embryos with a severe phenotype, overall LEF1 expression is decreased, specifically in the dorsal forebrain, dorsal OV and facial primordia, indicating widespread downregulation of the Wnt/β-catenin pathway (Figures 2Q,S).
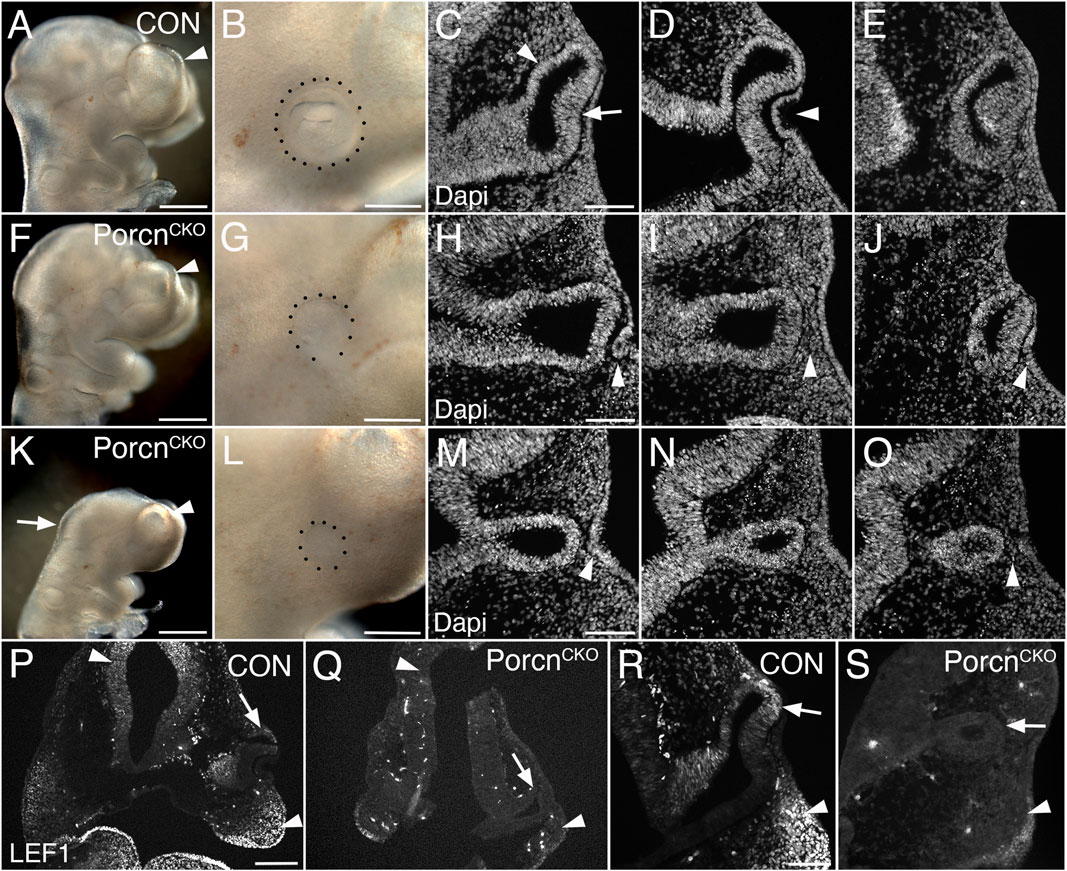
FIGURE 2. Conditional Porcn inactivation during eye field formation (E7.4-7.5) causes a range of brain and ocular abnormalities at E10.5. (A–E) Control PorcnCHET embryos showing telencephalic vesicle (A; arrowhead), early OC with lens vesicle (B; dotted outline), presumptive RPE and neural retina (C; arrowhead and arrow, respectively) and invaginating lens vesicle (D; arrowhead) in sequential Dapi-labeled coronal sections (C–E). (F–J) PorcnCKO with mild abnormalities; reduced telencephalic vesicle size (F; arrowhead), reduced eye size (G), incomplete invagination of OV and lens ectoderm (H, J; arrowheads) and accumulation of POM between distal OV and lens ectoderm (I; arrowhead). (K–O) PorcnCKO with major defects showing loss of the mid-hindbrain border and further reduction of telencephalic vesicles (K; arrow and arrowhead, respectively), microphthalmia (L; dotted outline, compare B, G, L), arrested OV (M–O) and accumulation of POM (O; arrowhead). Some thickening of the surface can occur (M; arrowhead). (P–S) LEF1 immunolabeling of coronal control PorcnCHET sections shows expression in the dorsal forebrain (P, left arrowhead), facial primordia mesenchyme (P; right arrowhead, R; arrowhead) and dorsal OC (P, R; arrows). In PorcnCKO embryos, LEF1 is severely reduced in the dorsal forebrain (Q; left arrowhead), facial primordia (Q; right arrowhead, S; arrowhead) and dorsal OC (Q, S; arrows). Scale bars A, F, K: 0.5 mm; B, G, L, P: 0.2 mm; C, H, M, R: 0.1 mm.
Eye field transcription factors are robustly expressed in the arrested OV of severely affected E10.5 PorcnCKO
Since OC morphogenesis is consistently and impaired the most, we proceeded with analyzing severely affected E10.5 PorcnCKO (Figures 2F–J). The pan-ocular transcription factor PAX6 is expressed in the arrested PorcnCKO OV and also present in the surface ectoderm (Figure 3B). The eye field transcription factor Six3 is required for neuroretinal specification the mammalian eye (Liu et al., 2010; Liu and Cvekl, 2017). SIX3 expression is not altered in the OV and surface ectoderm of PorcnCKO (Figure 3D). LHX2 is not altered (Figure 3F), confirming that general ocular specification including OV identity are maintained at later stages.
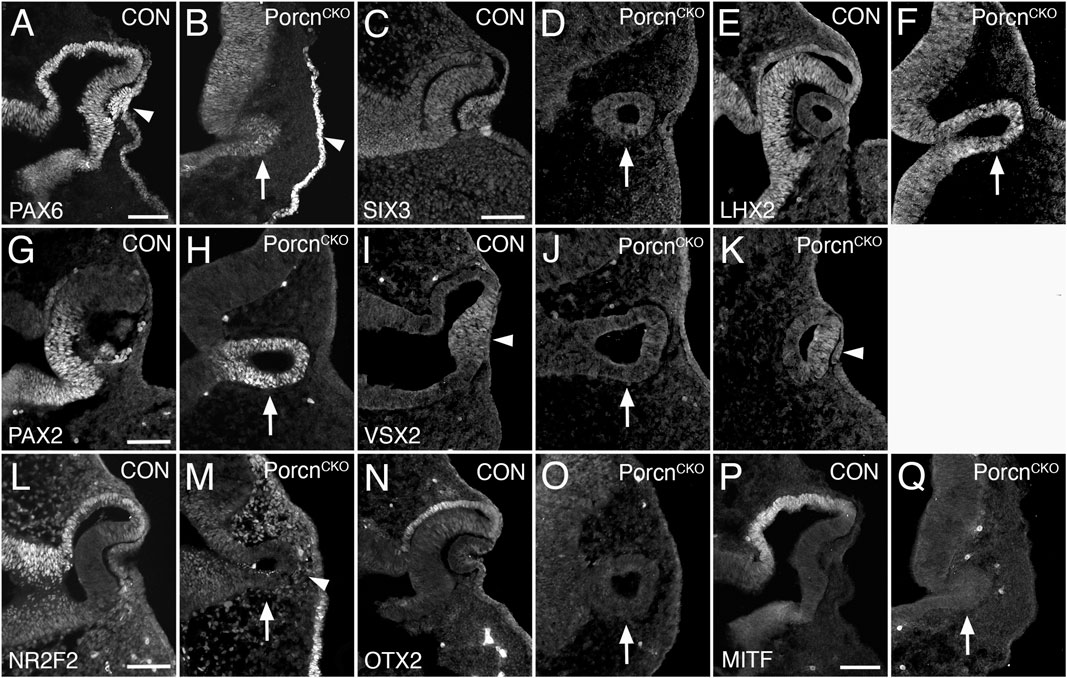
FIGURE 3. Porcn mutant embryos display defective regionalization at E10.5. Coronal view of control embryos (A,C,E,G,I,L,N,P) and PorcnCKO embryos that were treated with tamoxifen at E7.4 (B,D,F,H,J,K,M,O,Q). (A) During normal eye development, PAX6 is expressed in ocular tissues and in the overlying surface ectoderm (arrowhead). (B) In the mutant OV, PAX6 expression is expressed (arrow) and is present in the surface ectoderm (arrowhead). (C and D) SIX3 expression is not altered in the OV and surface ectoderm of PorcnCKO (D; arrow). (E and F) In control (E) and in PorcnCKO (F; arrow) LHX2 is expressed throughout the OC and OV, respectively. (G) In control OC, PAX2 is restricted to the ventral OC. (H) In PorcnCKO, PAX2 expression is maintained throughout the OV (arrow). (I) In controls, the distal OC is tightly attached to the overlying lens ectoderm and expresses VSX2 (arrowhead). (J) In PorcnCKO with loss of close contact, VSX2 is not detectable (arrow). (K) When distal OV and lens ectoderm are closely associated, some cells in the distal OV in PorcnCKO can express VSX2 (arrowhead). (L) NR2F2 expression shown in control embryos. (M) In the OV of PorcnCKO, NR2F2 expression is missing (arrow). NRF2 expressing POM cells are abnormally present between distal OV and surface ectoderm (arrowhead). (N,O) OTX2 is normally robustly expressed in the presumptive RPE of the OC but is absent in the mutant OV (O; arrow). (P) MITF is an early differentiation marker restricted to the presumptive RPE. (Q) In the OV of PorcnCKO, MITF expression is not detectable (arrow). Scale bars A, C, G, L, P: 0.1 mm.
Porcn mutant embryos display defective regionalization of the arrested OV at E10.5
The paired homeobox transcription factor PAX2 is initially expressed throughout the OV and is later confined to the ventral OC and optic stalk (Nornes et al., 1990; Burns et al., 2008). In PorcnCKO, PAX2 expression is maintained throughout the OV (Figure 3H), consistent with failure of OC formation and dorsoventral regionalization. During normal eye development, proximodistal regionalization into RPE and retina occurs in the advanced OV (Fuhrmann, 2010; Fuhrmann et al., 2014; Miesfeld and Brown, 2019). In PorcnCKO with loss of close contact due to POM accumulation between the distal OV and surface ectoderm (Figure 2), the strictly retina-specific CVC homeodomain transcription factor VSX2 is not expressed (Figure 3J) (Burmeister et al., 1996; Green et al., 2003). However, if close contact occurs in PorcnCKO, some cells in the presumptive retina can express VSX2 (Figure 3K), consistent with the requirement of lens-derived FGF for retina specification (Cai et al., 2013). Concerning proximal regionalization, we observed a complete loss of the key regulatory transcription factors NR2F2, OTX2, and MITF (Figure 3M,O,Q) that are required for RPE differentiation (Hodgkinson et al., 1993; Bumsted and Barnstable, 2000; Nguyen and Arnheiter, 2000; Martinez-Morales et al., 2001; Tang et al., 2010). NR2F2 expression confirms abnormal abundance of POM between distal OV and lens ectoderm (Figure 3M). Together, our results demonstrate that dorsoventral and proximodistal regionalization is impaired in the arrested OV of PorcnCKO at the time when OC morphogenesis normally commences.
Decrease of proliferation, survival and local NR2F2 and OTX2 expression in the PorcnCKO OV at E9.5
To elucidate a potential mechanism underlying abnormal ocular growth obvious in E10.5 PorcnCKO, we analyzed embryos 1 day earlier, following tamoxifen induction at E7.4-E7.5. At E9.5, PorcnCKO show slightly decreased telencephalic vesicles and mid-hindbrain regions (Figure 4B). LEF1 expression in the dorsal OV and adjacent dorsal forebrain neuroepithelium in PorcnCKO embryos is unaltered, suggesting that Wnt/β−catenin signaling is intact at this age (Figure 4D). MITF expression is not affected in the presumptive RPE of PorcnCKO (Figure 4F). However, other RPE markers NR2F2 and OTX2 start to be reduced dorsally in the distal OV domain (Figures 4H,J) suggesting that some aspects of regionalization can be affected at this age.
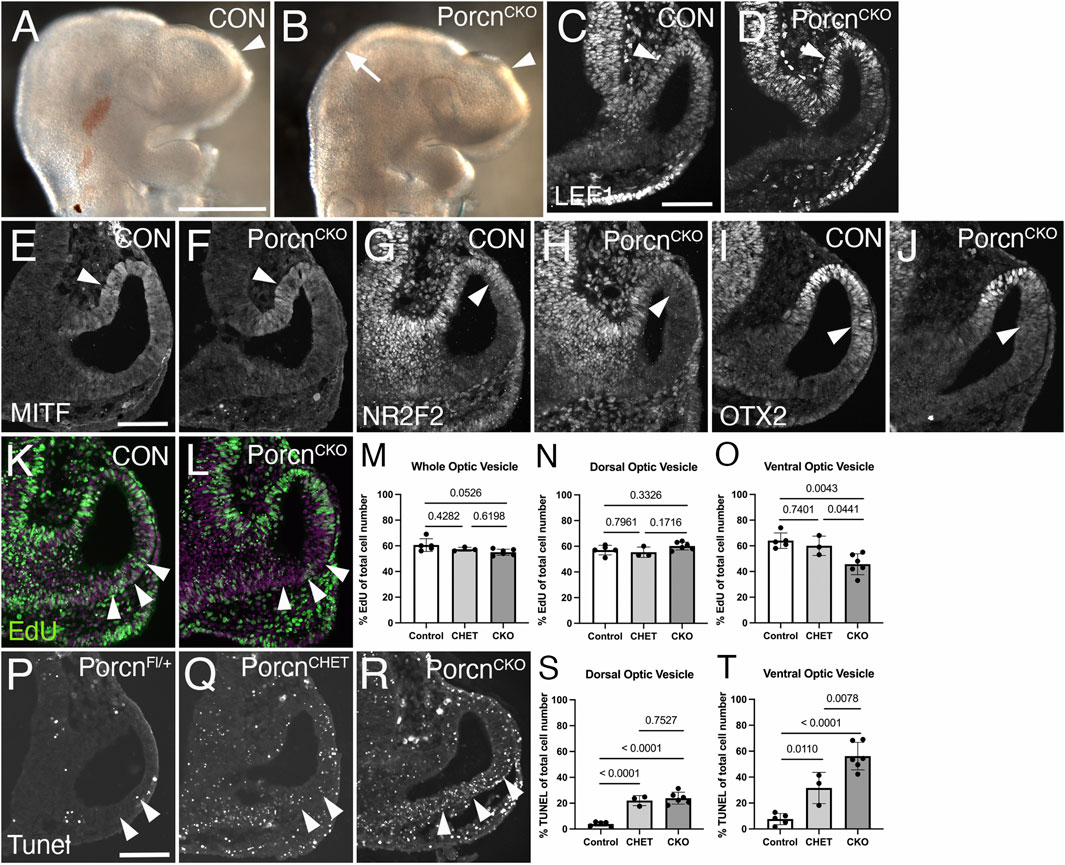
FIGURE 4. Decrease of proliferation, survival and local NR2F2 and OTX2 expression in the PorcnCKO OV at E9.5. Coronal view of control embryos (A,C,E,G,I,K; PorcnCHET) and PorcnCKO embryos, induced at E7.4–7.5 (B,D,F,H,J,L). (A) Telencephalic vesicle in E9.5 control embryo (arrowhead). (B) PorcnCKO show slightly smaller telencephalic vesicles (arrowhead) and mid-hindbrain regions (arrow). (C) In controls, LEF1 is expressed in the dorsal OV (arrowhead). (D) In PorcnCKO embryos, LEF1 is unaltered (arrowhead). (E and F) MITF expression appears unaffected in the presumptive RPE of PorcnCKO (F; arrowhead). (G and H) In the mutant RPE, NR2F2 can be slightly downregulated in the dorsal OV (H; arrowhead). (I and J) OTX2 can start to be reduced dorsally in the distal OV domain in PorcnCKO (J; arrowhead). (K,L) Edu incorporation (green) in control (K) and in PorcnCKO OV (L), showing reduced number of EdU-labeled cells in the ventral OV (L; arrowheads). Magenta: Dapi. (M–O) Quantification of EdU labeled cells in the entire OV (M), dorsal OV (N) and ventral OV (O). In PorcnCKO, the number of EdU-labeled cells is significantly reduced in the ventral OV (O). (P–R) Coronal view of representative images of Tunel-labeled E9.5 sections induced with tamoxifen at E7.4. Arrowheads mark the Tunel-labeled region in the ventral OV. Compared to Cre-negative controls (P), overall Tunel signal is increased throughout the tissues in PorcnCHET and PorcnCKO embryos (Q). In the ventral OV of PorcnCKO embryos, more Tunel-labeled cells are detectable (R), compared to PorcnCHET (Q). (S,T) Quantification of Tunel-labeled cells in the ventral OV shows a significant increase in PorcnCKO (T). Quantitative data are means ± s.d. One-way ANOVA with Tukey’s posthoc analysis was applied for statistical analysis, and p-values are indicated on the horizontal lines in each graph (significance <0.05). Scale bars A: 0.5 mm; C, E, P: 0.1 mm.
To determine effects on proliferation, we quantified the number of E9.5 OV cells incorporating EdU (Figures 4K–O). In PorcnCKO, the number of EdU-labeled cells show a trend in decrease in the entire OV (Figures 4L,M). This effect is specifically due to a significant reduction in the ventral OV by 24% and 29%, compared to PorcnCHET and PorcnFl/+/y, respectively (Figure 4O). We also examined apoptotic cell death in ocular progenitors. Compared to PorcnCHET, the percentage of Tunel-labeled cells is significantly upregulated by 78% in the ventral OV of PorcnCKO (Figures 4R,T). Unexpectedly, we observed a considerable effect of Porcn gene reduction on survival of ocular progenitors; PorcnCHET OVs show an overall significant increase in Tunel-labeled cells, compared to PorcnFl/+/y (Figures 4Q,S,T). These results suggest that already 2 days after tamoxifen administration loss of Porcn negatively impacts both survival and proliferation of ocular progenitors in the ventral OV. These changes occur before major defects in expression of the Wnt/β−catenin readout LEF1, regionalization markers and abnormal morphogenesis become obvious.
Discussion
Our results show that Porcn inactivation either before or during eye field formation leads to severely arrested OVs, likely due to an increase in cell death and downregulation of proliferation. In PorcnCKO, the RPE key regulatory transcription factors OTX2, MITF and NR2F2 are downregulated. POM accumulates between distal OV and adjacent surface ectoderm, preventing tight association, and the retina-specific gene VSX2 is not properly expressed. Arrested eye vesicles in PorcnCKO do not invaginate, resulting in failed invagination of the OV into an OC. Our studies reveal a novel role for Porcn in the OV as the earliest obvious morphological stage of eye development and a continued requirement during OC morphogenesis, recapitulating severe microphthalmia in FHD.
Effect of Porcn disruption on wnt signaling
We first determined when potential effectors of Wnt signaling are affected by Porcn inactivation at E6.5. Wnt/β−catenin signaling is normally not active until OV invagination starts; the pathway readouts Axin2 and BATgal reporter are absent in the OV around E8.75 (8 somites) (Liu et al., 2010). Here, we observed that another readout, LEF1, starts to become weakly expressed around E9.0 in the dorsal OV of controls (13 somites; Figure 1C). In PorcnCKO embryos, LEF1 is slightly downregulated in the dorsal OV (Figure 1D) suggesting commencement of a pathway response. Indeed, in the E9.5 OV, LEF1 is absent, demonstrating complete shutdown of the pathway, and OV morphogenesis is abnormal (Figure 1M). Thus, it takes at least 2.5 days after tamoxifen treatment to detect initial effects of Porcn inactivation on Wnt/β−catenin signaling and approximately 3 days to observe defects in gene expression and eye morphogenesis.
Since Wnt/β-catenin signaling needs to be suppressed in the anterior neural plate, we reasoned that any requirement for Porcn would be likely due to a need for the non-canonical Wnt pathway. Inactivation of Porcn also prevents potentially confounding, concomitant upregulation of Wnt/β-catenin signaling. Consistent with this, arrested growth of the OV has not been observed by early Wnt/β−catenin pathway inactivation in other studies (Hagglund et al., 2013). We observed that the putative non-canonical Wnt pathway readout pJUN is normally not robustly expressed in the E9.5 OV (not shown), and F-actin shows normal apicobasal localization in PorcnCKO (Figure 1). However, we cannot exclude effects on other potential non-canonical Wnt targets in PorcnCKO. In addition, Porcn can exert Wnt-independent roles in regulating cancer growth and AMPA receptor assembly and function in rate hippocampal neurons (Covey et al., 2012; Erlenhardt et al., 2016). While we cannot exclude Wnt-independent roles of Porcn during early eye development, it needs to be shown whether these functions are critical for early forebrain development.
Porcn is not required for maintenance of EFTF expression in the OV and OC
Eye field formation with expression of EFTFs in mouse starts around E7.5. To determine Porcn’s role during the earliest stages of eye morphogenesis, we induced inactivation at E6.5. Analysis of E8.75–9.5 PorcnCKO embryos revealed that expression of the EFTFs LHX2 and OTX2 is unaffected at E9.0, and that LHX2 is still present 3 days after tamoxifen induction (Figures 1F,H,Q). This also applies to PorcnCKO induced at E7.5 and analyzed at E10.5; the EFTFs PAX6, LHX2 and SIX3 are expressed (Figures 3B,D,F). Therefore, our data demonstrates that Porcn is not required to maintain EFTF expression in the OV.
Overall, our strategy of Porcn inactivation may be not quick enough to affect EFTF expression in the eye field between E7.5 and E8.5. However, it could be challenging to perform Porcn inactivation before E6.5 without causing most severe developmental defects and allowing to determine whether Porcn promotes EFTF expression and eye field formation.
Porcn is required for initiation of RPE differentiation in the OV
Our results are consistent with previous studies showing that Porcn inactivation prevents maintenance of RPE differentiation in the OC, most likely due to loss of Wnt/β−catenin signaling. RPE-specific inactivation of the pathway effector β−catenin in the early OC interferes with further RPE differentiation (Fujimura et al., 2009; Westenskow et al., 2009; Hagglund et al., 2013). Loss of RPE fate is likely caused by a failure to transactivate RPE gene expression, therefore, the mutant RPE transdifferentiates into retina (Fujimura et al., 2009; Westenskow et al., 2009). Here we show that RPE differentiation fails to initiate in PorcnCKO embryos induced at E6.5, since the early RPE marker OTX2 is absent in the dorsal E9.5 OV (Figure 1S). The POM is essential for RPE differentiation and a source for Wnt ligands, in addition to ocular tissues (Gage et al., 1999; Fuhrmann et al., 2000; Bassett et al., 2010; Bankhead et al., 2015; Carpenter et al., 2015). Thus, ubiquitous depletion of Porcn may interfere with RPE differentiation cell and non-cell autonomously. To examine a cell autonomous requirement, we attempted to disrupt Porcn using a more restricted Cre line, HesCreERT2 (Yun et al., 2009; Kopinke et al., 2011). We administered up to 0.12 mg/g tamoxifen around E6.8 and observed no obvious phenotype in Porcn mutant embryos (n = 4; not shown). We detected mosaic RosaR26 reporter expression in only one E10.5 embryo (n = 3; not shown) suggesting that HesCreERT2 may not be sufficiently activated at this time point. However, we showed in an earlier study that Porcn disruption causes consistent RPE differentiation defects only when performed simultaneously in ocular and extraocular tissues in the OV (Bankhead et al., 2015). We propose that Wnts are also redundantly produced and available within the eye field and adjacent tissues.
Porcn is required for ocular growth by promoting proliferation and survival of ocular progenitors
Our study demonstrates that Porcn inactivation results in severe morphogenesis defects 3 days later, either at the OV (E9.5) or OC stage (E10.5). The PorcnCKO OV or OC is small and OV expansion or OC invagination fails, respectively. Analysis of cell death (TUNEL) and proliferation (EdU incorporation) showed robustly downregulated proliferation and survival of ocular progenitors in the ventral OV 1 day earlier when morphology and expression of the Wnt/β−catenin readout appear normal (Figure 4K–T). Wnt signaling can directly regulate proliferation and survival of ocular progenitors (Burns et al., 2008; Hagglund et al., 2013). Interestingly, during embryonic morphogenesis, Wnt signaling may support metabolic demands by preventing a cellular stress response, which could affect proliferation and survival (Poncet et al., 2020). A reduction of Porcn gene dosage in the embryo may impact the overall stress response and affect general survival as observed in the dorsal PorcnCHET OV and surrounding tissues (Figure 4Q).
Significantly reduced proliferation during OC morphogenesis has been observed in embryos with germ line mutation of early ocular regulatory genes, for example Pax6sey/sey, Lhx2, BMP7, Hes1 and Mab21L2 (Grindley et al., 1995; Porter et al., 1997; Yamada et al., 2004; Lee et al., 2005; Morcillo et al., 2006; Yun et al., 2009). However, PAX6 and LHX2 are expressed in the OV of PorcnCKO, and Pax6, Bmp7, Hes1 and Mab21L2 mutants do not show a robust morphogenesis defect until E10.5. Therefore, to our knowledge, the ocular phenotype in PorcnCKO is unique because it shows an earlier requirement for proliferation and survival of ocular progenitors.
Porcn inactivation around the eye field stage recapitulates microphthalmia and anophthalmia in FDH patients
PorcnCKO display severe microphthalmia 3 days after tamoxifen treatment before or during the eye field stage. PorcnCKO embryos induced at E6.5 and harvested at E10.5 showed severe developmental abnormalities not feasible for further analysis at later time points (Supplementary Figure S2). Lhx2 mutants exhibit a similar OV morphogenesis defect leading to anophthalmia subsequently (Porter et al., 1997). Thus, we hypothesize that Porcn inactivation before the OV stage ultimately results in anophthalmia, consistent for an early role of Porcn in FDH. A case report for 18 FDH patients revealed a high incidence of ophthalmologic abnormalities (77%), including microphthalmia (44%) and anophthalmia (11%) (Gisseman and Herce, 2016). We propose that Porcn inactivation around the eye field stage represents a novel mouse model recapitulating severe microphthalmia and anophthalmia in humans. PorcnCHET female embryos do not display an apparent ocular phenotype compared to few embryos with germline inactivation of one Porcn allele (Bankhead et al., 2015). The late dosage reduction of Porcn, combined with variable mosaic X inactivation, may cause a failure to induce ocular defects in PorcnCHET at this critical developmental stage.
Conclusion
Using temporally controlled, conditional inactivation in mouse, our studies reveal a novel role for Porcn in regulating growth and morphogenesis of the optic vesicle and optic cup, via a requirement in proliferation, survival, and regionalization of ocular gene expression, recapitulating severe microphthalmia in FDH.
Data availability statement
The original contributions presented in the study are included in the article/Supplementary Materials, further inquiries can be directed to the corresponding author.
Ethics statement
The animal study was reviewed and approved by Institutional Animal Care and Use Committee at Vanderbilt University Medical Center.
Author contributions
SF designed experiments, supervised the research, carried out experiments presented in the manuscript, analyzed the data, and wrote the manuscript. SR carried out experiments, analyzed data and edited the manuscript. MMA carried out experiments, analyzed data and edited the manuscript. CC carried out experiments, analyzed data and edited the manuscript.
Funding
This work was supported by the National Institutes of Health (R01 EY024373 to SF, T32 HD007502 Training Grant in Stem Cell and Regenerative Developmental Biology to SR, Core Grant P30 EY14800); a Catalyst Award to SF from Research to Prevent Blindness Inc./American Macular Degeneration Foundation, an unrestricted award to the Department of Ophthalmology and Visual Sciences from Research to Prevent Blindness, Inc. Data presentation was performed in part through the use of the Vanderbilt Cell Imaging Shared Resource (supported by NIH grants CA68485, DK20593, DK58404, DK59637 and EY08126). Zeiss LSM880 Airyscan Confocal Microscope is obtained through the NIH S10 award (1 S10 OD021630 1, PI Sam Wells).
Acknowledgments
Special thanks to Ethan Lee and Yukio Saijoh for expert advice. Many thanks to Maria Elias and Katrina Hofstetter for excellent technical support. We thank Ed Levine and his team for helpful comments and discussions. Special thanks to Charles Murtaugh (University of Utah, Salt Lake City, UT) for kindly providing the conditional Porcn allele and the Cre line Hes1CreERT2.
Conflict of interest
The authors declare that the research was conducted in the absence of any commercial or financial relationships that could be construed as a potential conflict of interest.
Publisher’s note
All claims expressed in this article are solely those of the authors and do not necessarily represent those of their affiliated organizations, or those of the publisher, the editors and the reviewers. Any product that may be evaluated in this article, or claim that may be made by its manufacturer, is not guaranteed or endorsed by the publisher.
Supplementary material
The Supplementary Material for this article can be found online at: https://www.frontiersin.org/articles/10.3389/fcell.2022.1016182/full#supplementary-material
Supplementary Figure 1 | RosaR26 reporter activation. Coronal view of E9.0 embryonic heads, induced with tamoxifen at E6.5 (A, B) and E9.5 embryos (C, D), induced at E7.4. (A, B, D) PorcnCHET and PorcnCKO embryos show widespread expression of β-galactosidase protein, in contrast to controls without Cre (C). Arrows point to OVs in each image. Scale bar: 0.1 mm.
Supplementary Figure 2 | Developmental defects of PorcnCKO embryos 4 days after tamoxifen administration. (A-C) Embryos at E10.5, treated with tamoxifen at E6.5. (A) Control embryo (PorcnCHET; 37 somites). (B, C) PorcnCKO littermates with severe developmental defects (somites not detectable). Scale bar: 0.5 mm.
References
Alldredge, A., and Fuhrmann, S. (2016). Loss of Axin2 causes ocular defects during mouse eye development. Investigative Ophthalmol. Vis. Sci. 57, 5253–5262. doi:10.1167/iovs.15-18599
Badea, T. C., Wang, Y., and Nathans, J. (2003). A noninvasive genetic/pharmacologic strategy for visualizing cell morphology and clonal relationships in the mouse. J. Soc. Neurosci. 23, 2314–2322. doi:10.1523/JNEUROSCI.23-06-02314.2003
Bailey, T. J., El-Hodiri, H., Zhang, L., Shah, R., Mathers, P. H., and Jamrich, M. (2004). Regulation of vertebrate eye development by Rx genes. Int. J. Dev. Biol. 48, 761–770. doi:10.1387/ijdb.041878tb
Bankhead, E. J., Colasanto, M. P., Dyorich, K. M., Jamrich, M., Murtaugh, L. C., and Fuhrmann, S. (2015). Multiple requirements of the focal dermal hypoplasia gene porcupine during ocular morphogenesis. Am. J. Pathol. 185, 197–213. doi:10.1016/j.ajpath.2014.09.002
Barrott, J. J., Cash, G. M., Smith, A. P., Barrow, J. R., and Murtaugh, L. C. (2011). Deletion of mouse Porcn blocks Wnt ligand secretion and reveals an ectodermal etiology of human focal dermal hypoplasia/Goltz syndrome. Proc. Natl. Acad. Sci. U. S. A. 108, 12752–12757. doi:10.1073/pnas.1006437108
Bassett, E. A., Williams, T., Zacharias, A. L., Gage, P. J., Fuhrmann, S., and West-Mays, J. A. (2010). AP-2alpha knockout mice exhibit optic cup patterning defects and failure of optic stalk morphogenesis. Hum. Mol. Genet. 19, 1791–1804. doi:10.1093/hmg/ddq060
Biechele, S., Cox, B. J., and Rossant, J. (2011). Porcupine homolog is required for canonical Wnt signaling and gastrulation in mouse embryos. Dev. Biol. 355, 275–285. doi:10.1016/j.ydbio.2011.04.029
Biechele, S., Adissu, H. A., Cox, B. J., and Rossant, J. (2013). Zygotic Porcn paternal allele deletion in mice to model human focal dermal hypoplasia. PloS one 8, e79139. doi:10.1371/journal.pone.0079139
Bumsted, K. M., and Barnstable, C. J. (2000). Dorsal retinal pigment epithelium differentiates as neural retina in the microphthalmia (mi/mi) mouse. Investigative Ophthalmol. Vis. Sci. 41, 903–908.
Burmeister, M., Novak, J., Liang, M. Y., Basu, S., Ploder, L., and Hawes, N. L., (1996). Ocular retardation mouse caused by Chx10 homeobox null allele: Impaired retinal progenitor proliferation and bipolar cell differentiation. Nat. Genet. 12, 376–384.
Burns, C. J., Zhang, J., Brown, E. C., Van Bibber, A. M., Van Es, J., and Clevers, H., (2008). Investigation of Frizzled-5 during embryonic neural development in mouse. Dev. Dyn. official Publ. Am. Assoc. Anatomists 237, 1614–1626. doi:10.1002/dvdy.21565
Cai, Z., Tao, C., Li, H., Ladher, R., Gotoh, N., and Feng, G. S., (2013). Deficient FGF signaling causes optic nerve dysgenesis and ocular coloboma. Development 140, 2711–2723. doi:10.1242/dev.089987
Carpenter, A. C., Smith, A. N., Wagner, H., Cohen-Tayar, Y., Rao, S., and Wallace, V., (2015). Wnt ligands from the embryonic surface ectoderm regulate 'bimetallic strip' optic cup morphogenesis in mouse. Development 142, 972–982. doi:10.1242/dev.120022
Cavodeassi, F., Carreira-Barbosa, F., Young, R. M., Concha, M. L., Allende, M. L., and Houart, C., (2005). Early stages of zebrafish eye formation require the coordinated activity of Wnt11, Fz5, and the Wnt/beta-catenin pathway. Neuron 47, 43–56. doi:10.1016/j.neuron.2005.05.026
Cavodeassi, F., Ivanovitch, K., and Wilson, S. W. (2013). Eph/Ephrin signalling maintains eye field segregation from adjacent neural plate territories during forebrain morphogenesis. Development 140, 4193–4202. doi:10.1242/dev.097048
Clementi, M., Turolla, L., Mammi, I., and Tenconi, R. (1992). Clinical anophthalmia: An epidemiological study in northeast Italy based on 368, 256 consecutive births. Teratology 46, 551–553. doi:10.1002/tera.1420460604
Covey, T. M., Kaur, S., Tan Ong, T., Proffitt, K. D., Wu, Y., and Tan, P., (2012). PORCN moonlights in a Wnt-independent pathway that regulates cancer cell proliferation. PloS one 7, e34532. doi:10.1371/journal.pone.0034532
Erlenhardt, N., Yu, H., Abiraman, K., Yamasaki, T., Wadiche, J. I., and Tomita, S., (2016). Porcupine controls hippocampal AMPAR levels, composition, and synaptic transmission. Cell Rep. 14, 782–794. doi:10.1016/j.celrep.2015.12.078
Fuhrmann, S., Levine, E. M., and Reh, T. A. (2000). Extraocular mesenchyme patterns the optic vesicle during early eye development in the embryonic chick. Development 127, 4599–4609. doi:10.1242/dev.127.21.4599
Fuhrmann, S., Zou, C., and Levine, E. M. (2014). Retinal pigment epithelium development, plasticity, and tissue homeostasis. Exp. eye Res. 123, 141–150. doi:10.1016/j.exer.2013.09.003
Fuhrmann, S. (2008). Wnt signaling in eye organogenesis. Organogenesis 4, 60–67. doi:10.4161/org.4.2.5850
Fuhrmann, S. (2010). Eye morphogenesis and patterning of the optic vesicle. Curr. Top. Dev. Biol. 93, 61–84. doi:10.1016/B978-0-12-385044-7.00003-5
Fujimura, N., Taketo, M. M., Mori, M., Korinek, V., and Kozmik, Z. (2009). Spatial and temporal regulation of Wnt/beta-catenin signaling is essential for development of the retinal pigment epithelium. Dev. Biol. 334, 31–45. doi:10.1016/j.ydbio.2009.07.002
Fujimura, N. (2016). WNT/beta-Catenin signaling in vertebrate eye development. Front. Cell Dev. Biol. 4, 138. doi:10.3389/fcell.2016.00138
Gage, P. J., Suh, H., and Camper, S. A. (1999). Dosage requirement of Pitx2 for development of multiple organs. Development 126, 4643–4651. doi:10.1242/dev.126.20.4643
Galli, L. M., Barnes, T. L., Secrest, S. S., Kadowaki, T., and Burrus, L. W. (2007). Porcupine-mediated lipid-modification regulates the activity and distribution of Wnt proteins in the chick neural tube. Development 134, 3339–3348. doi:10.1242/dev.02881
Gisseman, J. D., and Herce, H. H. (2016). Ophthalmologic manifestations of focal dermal hypoplasia (Goltz syndrome): A case series of 18 patients. Am. J. Med. Genet. C Semin. Med. Genet. 172C, 59–63. doi:10.1002/ajmg.c.31480
Goltz, R. W., Peterson, W. C., Gorlin, R. J., and Ravits, H. G. (1962). Focal dermal hypoplasia. Archives dermatology 86, 708–717. doi:10.1001/archderm.1962.01590120006002
Graw, J. (2019). Mouse models for microphthalmia, anophthalmia and cataracts. Hum. Genet. 138, 1007–1018. doi:10.1007/s00439-019-01995-w
Green, E. S., Stubbs, J. L., and Levine, E. M. (2003). Genetic rescue of cell number in a mouse model of microphthalmia: Interactions between Chx10 and G1-phase cell cycle regulators. Development 130, 539–552. doi:10.1242/dev.00275
Grindley, J. C., Davidson, D. R., and Hill, R. E. (1995). The role of Pax-6 in eye and nasal development. Development 121, 1433–1442. doi:10.1242/dev.121.5.1433
Grzeschik, K. H., Bornholdt, D., Oeffner, F., Konig, A., del Carmen Boente, M., and Enders, H., (2007). Deficiency of PORCN, a regulator of Wnt signaling, is associated with focal dermal hypoplasia. Nat. Genet. 39, 833–835. doi:10.1038/ng2052
Hagglund, A. C., Berghard, A., and Carlsson, L. (2013). Canonical Wnt/beta-catenin signalling is essential for optic cup formation. PloS one 8, e81158. doi:10.1371/journal.pone.0081158
Heisenberg, C. P., Tada, M., Rauch, G. J., Saude, L., Concha, M. L., and Geisler, R., (2000). Silberblick/Wnt11 mediates convergent extension movements during zebrafish gastrulation. Nature 405, 76–81. doi:10.1038/35011068
Hodgkinson, C. A., Moore, K. J., Nakayama, A., Steingrimsson, E., Copeland, N. G., and Jenkins, N. A., (1993). Mutations at the mouse microphthalmia locus are associated with defects in a gene encoding a novel basic-helix-loop-helix-zipper protein. Cell 74, 395–404. doi:10.1016/0092-8674(93)90429-t
Kemp, C., Willems, E., Abdo, S., Lambiv, L., and Leyns, L. (2005). Expression of all Wnt genes and their secreted antagonists during mouse blastocyst and postimplantation development. Dev. Dyn. official Publ. Am. Assoc. Anatomists 233, 1064–1075. doi:10.1002/dvdy.20408
Kispert, A., Vainio, S., Shen, L., Rowitch, D. H., and McMahon, A. P. (1996). Proteoglycans are required for maintenance of Wnt-11 expression in the ureter tips. Development 122, 3627–3637. doi:10.1242/dev.122.11.3627
Kopinke, D., Brailsford, M., Shea, J. E., Leavitt, R., Scaife, C. L., and Murtaugh, L. C. (2011). Lineage tracing reveals the dynamic contribution of Hes1+ cells to the developing and adult pancreas. Development 138, 431–441. doi:10.1242/dev.053843
Lagutin, O. V., Zhu, C. C., Kobayashi, D., Topczewski, J., Shimamura, K., and Puelles, L., (2003). Six3 repression of Wnt signaling in the anterior neuroectoderm is essential for vertebrate forebrain development. Genes & Dev. 17, 368–379. doi:10.1101/gad.1059403
Lee, H. Y., Wroblewski, E., Philips, G. T., Stair, C. N., Conley, K., and Reedy, M., (2005). Multiple requirements for Hes 1 during early eye formation. Dev. Biol. 284, 464–478. doi:10.1016/j.ydbio.2005.06.010
Lee, H. S., Bong, Y. S., Moore, K. B., Soria, K., Moody, S. A., and Daar, I. O. (2006). Dishevelled mediates ephrinB1 signalling in the eye field through the planar cell polarity pathway. Nat. Cell Biol. 8, 55–63. doi:10.1038/ncb1344
Lieven, O., and Ruther, U. (2011). The Dkk1 dose is critical for eye development. Dev. Biol. 355, 124–137. doi:10.1016/j.ydbio.2011.04.023
Liu, W., and Cvekl, A. (2017). Six3 in a small population of progenitors at E8.5 is required for neuroretinal specification via regulating cell signaling and survival in mice. Dev. Biol. 428, 164–175. doi:10.1016/j.ydbio.2017.05.026
Liu, W., Lagutin, O., Swindell, E., Jamrich, M., and Oliver, G. (2010). Neuroretina specification in mouse embryos requires Six3-mediated suppression of Wnt8b in the anterior neural plate. J. Clin. investigation 120, 3568–3577. doi:10.1172/JCI43219
Martinez-Morales, J. R., Signore, M., Acampora, D., Simeone, A., and Bovolenta, P. (2001). Otx genes are required for tissue specification in the developing eye. Development 128, 2019–2030. doi:10.1242/dev.128.11.2019
Maurus, D., Heligon, C., Burger-Schwarzler, A., Brandli, A. W., and Kuhl, M. (2005). Noncanonical Wnt-4 signaling and EAF2 are required for eye development in Xenopus laevis. EMBO J. 24, 1181–1191. doi:10.1038/sj.emboj.7600603
Miesfeld, J. B., and Brown, N. L. (2019). Eye organogenesis: A hierarchical view of ocular development. Curr. Top. Dev. Biol. 132, 351–393. doi:10.1016/bs.ctdb.2018.12.008
Moore, K. B., Mood, K., Daar, I. O., and Moody, S. A. (2004). Morphogenetic movements underlying eye field formation require interactions between the FGF and ephrinB1 signaling pathways. Dev. Cell 6, 55–67. doi:10.1016/s1534-5807(03)00395-2
Morcillo, J., Martinez-Morales, J. R., Trousse, F., Fermin, Y., Sowden, J. C., and Bovolenta, P. (2006). Proper patterning of the optic fissure requires the sequential activity of BMP7 and SHH. Development 133, 3179–3190. doi:10.1242/dev.02493
Morrison, D., FitzPatrick, D., Hanson, I., Williamson, K., van Heyningen, V., and Fleck, B., (2002). National study of microphthalmia, anophthalmia, and coloboma (MAC) in scotland: Investigation of genetic aetiology. J. Med. Genet. 39, 16–22. doi:10.1136/jmg.39.1.16
Motahari, Z., Martinez-De Luna, R. I., Viczian, A. S., and Zuber, M. E. (2016). Tbx3 represses bmp4 expression and, with Pax6, is required and sufficient for retina formation. Development 143, 3560–3572. doi:10.1242/dev.130955
Nguyen, M., and Arnheiter, H. (2000). Signaling and transcriptional regulation in early mammalian eye development: A link between FGF and MITF. Development 127, 3581–3591. doi:10.1242/dev.127.16.3581
Nornes, H. O., Dressler, G. R., Knapik, E. W., Deutsch, U., and Gruss, P. (1990). Spatially and temporally restricted expression of Pax2 during murine neurogenesis. Development 109, 797–809. doi:10.1242/dev.109.4.797
Oliver, G., Mailhos, A., Wehr, R., Copeland, N. G., Jenkins, N. A., and Gruss, P. (1995). Six3, a murine homologue of the sine oculis gene, demarcates the most anterior border of the developing neural plate and is expressed during eye development. Development 121, 4045–4055. doi:10.1242/dev.121.12.4045
Park, E. J., Sun, X., Nichol, P., Saijoh, Y., Martin, J. F., and Moon, A. M. (2008). System for tamoxifen-inducible expression of cre-recombinase from the Foxa2 locus in mice. Dev. Dyn. official Publ. Am. Assoc. Anatomists 237, 447–453. doi:10.1002/dvdy.21415
Parr, B. A., Shea, M. J., Vassileva, G., and McMahon, A. P. (1993). Mouse Wnt genes exhibit discrete domains of expression in the early embryonic CNS and limb buds. Development 119, 247–261. doi:10.1242/dev.119.1.247
Poncet, N., Halley, P. A., Lipina, C., Gierlinski, M., Dady, A., and Singer, G. A., (2020). Wnt regulates amino acid transporter Slc7a5 and so constrains the integrated stress response in mouse embryos. EMBO Rep. 21, e48469. doi:10.15252/embr.201948469
Porter, F. D., Drago, J., Xu, Y., Cheema, S. S., Wassif, C., and Huang, S. P., (1997). Lhx2, a LIM homeobox gene, is required for eye, forebrain, and definitive erythrocyte development. Development 124, 2935–2944. doi:10.1242/dev.124.15.2935
Rasmussen, J. T., Deardorff, M. A., Tan, C., Rao, M. S., Klein, P. S., and Vetter, M. L. (2001). Regulation of eye development by frizzled signaling in Xenopus. Proc. Natl. Acad. Sci. U. S. A. 98, 3861–3866. doi:10.1073/pnas.071586298
Soriano, P. (1999). Generalized lacZ expression with the ROSA26 Cre reporter strain. Nat. Genet. 21, 70–71. doi:10.1038/5007
Sun, W. R., Ramirez, S., Spiller, K. E., Zhao, Y., and Fuhrmann, S. (2020). Nf2 fine-tunes proliferation and tissue alignment during closure of the optic fissure in the embryonic mouse eye. Hum. Mol. Genet. 29, 3373–3387. doi:10.1093/hmg/ddaa228
Tanaka, K., Okabayashi, K., Asashima, M., Perrimon, N., and Kadowaki, T. (2000). The evolutionarily conserved porcupine gene family is involved in the processing of the Wnt family. Eur. J. Biochem./FEBS 267, 4300–4311. doi:10.1046/j.1432-1033.2000.01478.x
Tang, K., Xie, X., Park, J. I., Jamrich, M., Tsai, S., and Tsai, M. J. (2010). COUP-TFs regulate eye development by controlling factors essential for optic vesicle morphogenesis. Development 137, 725–734. doi:10.1242/dev.040568
Temple, I. K., MacDowall, P., Baraitser, M., and Atherton, D. J. (1990). Focal dermal hypoplasia (Goltz syndrome). J. Med. Genet. 27, 180–187. doi:10.1136/jmg.27.3.180
Veien, E. S., Rosenthal, J. S., Kruse-Bend, R. C., Chien, C. B., and Dorsky, R. I. (2008). Canonical Wnt signaling is required for the maintenance of dorsal retinal identity. Development 135, 4101–4111. doi:10.1242/dev.027367
Viczian, A. S. Z. (2014). “Retinal development,” in Principles of developmental genetics. Editor S. A. Moody. second ed. (Cambridge, MA, U.S.A: Academic Press), 297–313.
Wang, X., Reid Sutton, V., Omar Peraza-Llanes, J., Yu, Z., Rosetta, R., Kou, Y. C., et al. (2007). Mutations in X-linked PORCN, a putative regulator of Wnt signaling, cause focal dermal hypoplasia. Nat. Genet. 39, 836–838. doi:10.1038/ng2057
Wang, L., Jin, X., Zhao, X., Liu, D., Hu, T., and Li, W., (2014). Focal dermal hypoplasia: Updates. Oral Dis. 20, 17–24. doi:10.1111/odi.12083
Westenskow, P., Piccolo, S., and Fuhrmann, S. (2009). Beta-catenin controls differentiation of the retinal pigment epithelium in the mouse optic cup by regulating Mitf and Otx2 expression. Development 136, 2505–2510. doi:10.1242/dev.032136
Yamada, R., Mizutani-Koseki, Y., Koseki, H., and Takahashi, N. (2004). Requirement for Mab21l2 during development of murine retina and ventral body wall. Dev. Biol. 274, 295–307. doi:10.1016/j.ydbio.2004.07.016
Yamaguchi, T. P., Bradley, A., McMahon, A. P., and Jones, S. (1999). A Wnt5a pathway underlies outgrowth of multiple structures in the vertebrate embryo. Development 126, 1211–1223. doi:10.1242/dev.126.6.1211
Yun, S., Saijoh, Y., Hirokawa, K. E., Kopinke, D., Murtaugh, L. C., and Monuki, E. S., (2009). Lhx2 links the intrinsic and extrinsic factors that control optic cup formation. Development 136, 3895–3906. doi:10.1242/dev.041202
Zhou, C. J., Molotkov, A., Song, L., Li, Y., Pleasure, D. E., and Pleasure, S. J., (2008). Ocular coloboma and dorsoventral neuroretinal patterning defects in Lrp6 mutant eyes. Dev. Dyn. official Publ. Am. Assoc. Anatomists 237, 3681–3689. doi:10.1002/dvdy.21770
Keywords: microphthalmia, Focal Dermal Hypoplasia, Goltz Syndrome, Porcn, Wnt, optic vesicle, optic cup, mouse
Citation: Fuhrmann S, Ramirez S, Mina Abouda M and Campbell CD (2022) Porcn is essential for growth and invagination of the mammalian optic cup. Front. Cell Dev. Biol. 10:1016182. doi: 10.3389/fcell.2022.1016182
Received: 11 August 2022; Accepted: 04 October 2022;
Published: 31 October 2022.
Edited by:
Rajalekshmy Shyam, Indiana University Bloomington, United StatesReviewed by:
Wei Liu, Albert Einstein College of Medicine, United StatesFei Liu, Institute of Hydrobiology (CAS), China
Ala Moshiri, UC Davis Medical Center, United States
Copyright © 2022 Fuhrmann, Ramirez, Mina Abouda and Campbell. This is an open-access article distributed under the terms of the Creative Commons Attribution License (CC BY). The use, distribution or reproduction in other forums is permitted, provided the original author(s) and the copyright owner(s) are credited and that the original publication in this journal is cited, in accordance with accepted academic practice. No use, distribution or reproduction is permitted which does not comply with these terms.
*Correspondence: Sabine Fuhrmann, c2FiaW5lLmZ1aHJtYW5uQHZhbmRlcmJpbHQuZWR1