- 1Division of Molecular and Experimental Surgery, Department of Surgery, Universitätsklinikum Erlangen, Friedrich-Alexander-Universität Erlangen-Nürnberg (FAU), Erlangen, Germany
- 2Department of Medicine I, Universitätsklinikum Erlangen, Friedrich-Alexander-Universität Erlangen-Nürnberg (FAU), Erlangen, Germany
- 3Biognosys AG, Schlieren, Switzerland
- 4Department of Pediatric Surgery, University Medicine Greifswald, Greifswald, Germany
- 5Division of Surgical Research, Department of Surgery, Universitätsklinikum Erlangen, Friedrich-Alexander-Universität Erlangen-Nürnberg (FAU), Erlangen, Germany
- 6Comprehensive Cancer Center Erlangen-EMN, Universitätsklinikum Erlangen, Erlangen, Germany
The development of inflammatory bowel diseases (IBD) involves the breakdown of two barriers: the epithelial barrier and the gut-vascular barrier (GVB). The destabilization of each barrier can promote initiation and progression of the disease. Interestingly, first evidence is available that both barriers are communicating through secreted factors that may accordingly serve as targets for therapeutic modulation of barrier functions. Interferon (IFN)-γ is among the major pathogenesis factors in IBD and can severely impair both barriers. In order to identify factors transmitting signals from the GVB to the epithelial cell barrier, we analyzed the secretome of IFN-γ-treated human intestinal endothelial cells (HIEC). To this goal, HIEC were isolated in high purity from normal colon tissues. HIEC were either untreated or stimulated with IFN-γ (10 U/mL). After 48 h, conditioned media (CM) were harvested and subjected to comparative hyper reaction monitoring mass spectrometry (HRM™ MS). In total, 1,084 human proteins were detected in the HIEC-CM. Among these, 43 proteins were present in significantly different concentrations between the CM of IFN-γ- and control-stimulated HIEC. Several of these proteins were also differentially expressed in various murine colitis models as compared to healthy animals supporting the relevance of these proteins secreted by inflammatory activated HIEC in the inter-barrier communication in IBD. The angiocrine pathogenic impact of these differentially secreted HIEC proteins on the epithelial cell barrier and their perspectives as targets to treat IBD by modulation of trans-barrier communication is discussed in detail.
Introduction
Inflammatory bowel diseases (IBD) affect several million individuals worldwide, with Crohn’s disease (CD) and ulcerative colitis (UC) being the clinically predominant forms. IBD similarities are based on their common presentation as intestinal chronic inflammatory disorders characterized by cyclic flares of destructive inflammation resulting in severe impact on the intestinal barrier functions (Zhang and Li, 2014). Heterogeneity is present at the levels of clinical presentation, immune reactions, molecular-genetic components and microbial players involved (Lloyd-Price et al., 2019).
The intestinal barrier serves manifold tasks, which is also evident from its complex structure composed of two sequential physical barriers. The first barrier from the intestinal lumen is established by the epithelial barrier that consists of a single cell layer of epithelial cells overlaid by a mucus layer, which physically separates the microbiota in the gut lumen from epithelial cells (Stürzl et al., 2021). Directly below this epithelial barrier lies the gut-vascular barrier (GVB) controlling the entry of molecules and cells into the portal circulation and their subsequent delivery to the liver (Spadoni et al., 2015; Spadoni et al., 2017).
The structure and functions of the epithelial barrier have been comprehensively described in previous work (López-Posadas et al., 2017). In contrast, the existence and significant contribution of the GVB to IBD has been recognized only recently. Clinical evidence for a role of the GVB in IBD was obtained by the observation that the vasculature in patients exhibits increased permeability during acute phases of the disease, which is decreasing or absent in remission phases (Langer et al., 2019). In addition, studies in preclinical mouse models revealed that a breakdown of the GVB in the colon allows the permeation of bacteria into the blood with access to distant organs, including the liver, with significant impact on IBD pathogenesis (Spadoni et al., 2015). In own studies, we detected that IFN-γ, an immune-modulatory cytokine with driver activity in IBD pathogenesis, increases vascular permeability in the dextran sodium sulfate (DSS)-induced colitis model (Langer et al., 2019). Increased intestinal blood vessel permeability was associated with structural and functional perturbations of the adherens junction protein vascular endothelial (VE)-cadherin and significant worsening of the disease. An endothelial specific knock-out of the IFN-γ-receptor 2 (IFNγR2) as well as pharmacological vessel stabilization in mouse models suppressed vascular permeability and the development of acute and chronic DSS-colitis (Langer et al., 2019). These results provided clear evidence for the importance of the vascular barrier in IBD.
Effective cooperation of two different barriers requires coordinated action and communication. Well-established communication pathways between the epithelial barrier and the GVB are indicated by the observation that nutrient composition in the gut can affect the blood flow (Stan et al., 2012; Gentile and King, 2018). In addition, epithelial cells can secrete factors in response to pathogens such as cytokines, chemokines, reactive oxygen species, and lipid mediators, which can activate endothelial cells (Boueiz and Hassoun, 2009; Franze et al., 2016; Ferrari et al., 2017; Gentile and King, 2018).
However, the endothelium is not only a passive tube system transporting blood and receiving signals from surrounding cells, but exerts perfusion-independent functions, which actively contribute to the tissue microenvironment in organ development and diseases. In IBD, the intestinal microvasculature is notably involved in immune cell recruitment through expression of cell adhesion molecules (CAMs), such as VCAM1 or MadCAM1 (Binion et al., 1998). The inhibition of T-cell recruitment by targeting the binding of α4β7 integrins to endothelial MadCAM1 represents a new therapeutic axis in IBD (Neurath, 2017).
The first hint for an active paracrine function of the endothelium within the tissue microenvironment was derived from cancer research (Butler et al., 2010). Subsequent studies identified tumor repressive molecules that are expressed and released from endothelial cells, including the slit homolog 2 protein (Slit 2), perlecan, thrombospondin and SPARCL1 (Butler et al., 2010; Franses et al., 2011; Naschberger et al., 2016; Hinshaw and Shevde, 2019). Now, it is generally accepted that endothelial cells can actively trigger the microenvironment via so called “angiocrine factors” -a term that. includes secreted and membrane-bound inhibitory or stimulatory growth factors, trophogens, chemokines, cytokines, extracellular matrix components, exosomes, and other cellular products expressed by endothelial cells (Rafii et al., 2016).
Angiocrine functions in IBD have not been extensively investigated as yet. Only recently, we performed a meta-analysis to investigate whether angiocrine signaling in the colon may impact epithelial barrier functions (Stürzl et al., 2021). This approach yielded six putative candidates that are secreted from endothelial cells and may contribute to IBD pathogenesis, including proteins of the von Willebrand factor domain superfamily (VWA1, vWF), tissue inhibitor of metalloproteinases (TIMP)-1, matrix metalloproteinase (MMP)-14, the chemokine CXCL10, and the matricellular protein SPARCL1 (Stürzl et al., 2021). The expression and known functions of these proteins supported the hypothesis that they may be active in IBD. However, the bioinformatical analysis also showed that the overlap of genes retrieved from the different studies was very low, which was well in agreement with the high variation of activation and organ-dependent plasticity of endothelial cells (Stürzl et al., 2021).
Analysis of the IFN-γ-induced secretome in HIEC
Here we aimed to determine putative angiocrine factors released from cultivated primary human intestinal endothelial cells (HIEC) under pathogenically relevant stimulation in an experimental approach. Based on own previous results we used IFN-γ as a model cytokine for stimulation (Langer et al., 2019). In order to reduce pathogenesis-related heterogeneity we refrained from using patient-derived human HIEC but focused on highly pure cultures of healthy HIEC instead. To this goal, HIEC were isolated from healthy colon areas of five patients who underwent surgical therapy for colorectal cancer (CRC) (see Supplementary Methods). Endothelial cells were isolated by FACS-based cell sorting following previously established protocols (Naschberger et al., 2016; Naschberger et al., 2018). A purity above 98% of all five cultures was determined with reverse transcription quantitative polymerase chain reaction (RT-qPCR) and cytochemistry as described previously (Naschberger et al., 2016; Naschberger et al., 2018) and is exemplarily shown here by the uniform expression of the endothelial marker CD31 in all HIEC of the different patients but not in the CRC tumor cell line DLD1 (Figure 1A, Supplementary Figures S1A).
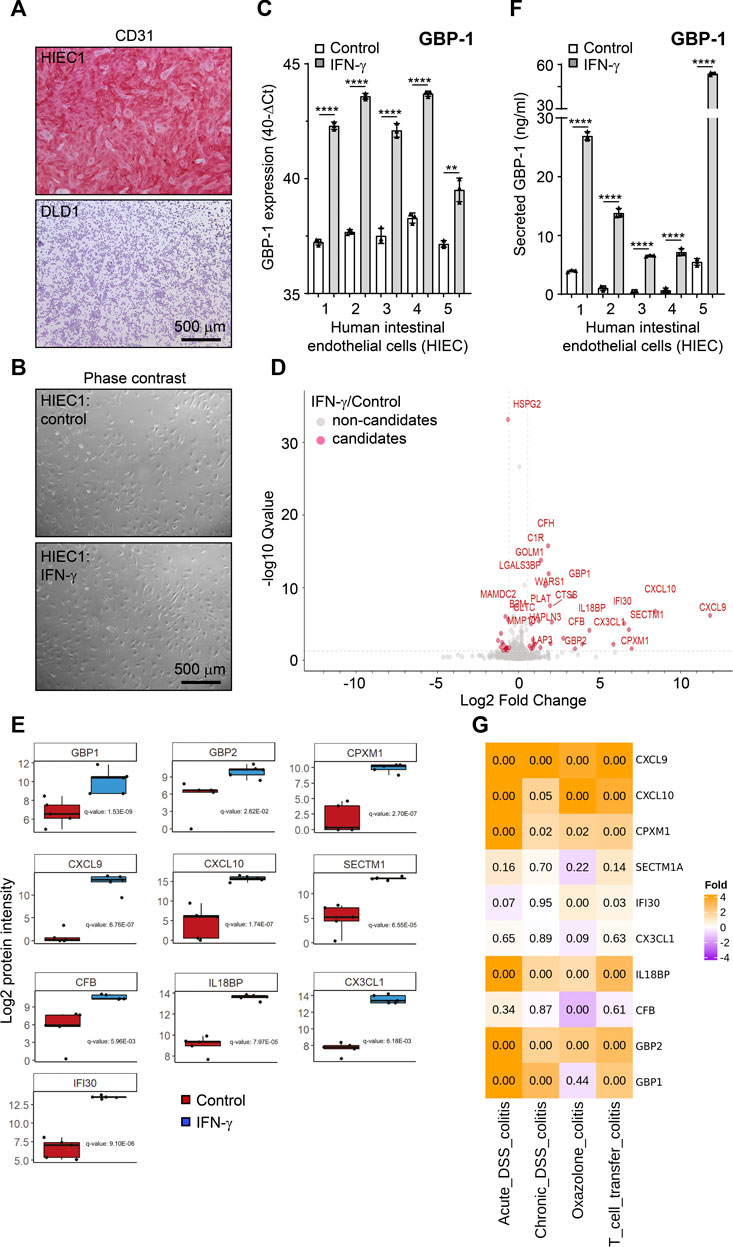
FIGURE 1. The secretome of IFN-γ-treated human intestinal endothelial cells. (A) Cultivated human intestinal endothelial cells (HIEC) uniformly express the endothelial cell-specific CD31 antigen whereas the epithelial colorectal cancer cell line DLD1 is negative. (B) No difference in the cell phenotype is detected in untreated and IFN-γ-treated HIEC. (C) IFN-γ treatment (10 U/mL, 48 h) induces expression of GBP-1 in all HIEC cultures as determined by RT-qPCR. (D) Volcano blot of the secretome of IFN-γ-treated HIEC. Proteins present in significantly different concentrations in the cell culture supernatants of IFN-γ-treated and untreated HIEC are indicated in red. (E) Box blots showing differential secretion of the different factors in all HIEC cultures (n = 5) in response to IFN-γ. p-values were calculated with the one sample t-test (μ = 0) and were corrected for overall FDR using the q-value approach (Storey and Tibshirani, 2003). (F) IFN-γ treatment (10 U/mL, 48 h) induces secretion of GBP-1 in all HIEC cultures as determined by GBP-1-specific ELISA. (G) Expression of genes encoding the top ten secreted proteins from IFN-γ-treated HIEC in different experimentally induced murine colitis models. Expression relative to healthy control mice is indicated by color code. Numbers are representing adjusted p-values of statistical differences. (A,B) Scale bars correspond to 500 µm. (C,F) p values: *** = p <0.001, ** = p <0.01, and * = p <0.05, paired t-test.
The five HIEC cultures were treated with IFN-γ which did not induce different morphology in untreated as compared to treated HIEC (Figure 1B, quantitative evaluation Supplementary Figures S1B). However, successful stimulation of all five cultures was indicated by the expression of IFN-γ-induced guanylate binding protein-1 (GBP-1), a well-established marker for IFN-γ stimulation of eukaryotic cells (Guenzi et al., 2001; Lubeseder-Martellato et al., 2002), which was highly increased in all stimulated HIEC (Figure 1C).
Next, cell culture supernatants were harvested from the IFN-γ-treated and untreated HIEC and subjected to hyper reaction monitoring mass spectrometry (HRM™ MS). Comparison of stimulated and unstimulated cultures identified 1,713 proteins detected by a mean of 5.79 peptides per protein. From all proteins identified, 1,084 were of human origin (629 from medium FBS) with 43 proteins differentially secreted between the CM of IFN-γ-stimulated and unstimulated HIEC (Figure 1D, red; Table 1). The top ten differentially secreted proteins included the chemokines CXCL9, CXCL10 and fractalkine as well as the IFN-γ-induced secreted proteins secreted and transmembrane protein 1 (SECTM1 or K12), gamma-interferon-inducible lysosomal thiol reductase (IFI30) and GBP-1 (Figure 1E). Of note, increased secretion of GBP-1 by IFN-γ-treated HIECs as detected by mass spectrometry was confirmed by independent ELISA (Figure 1F).
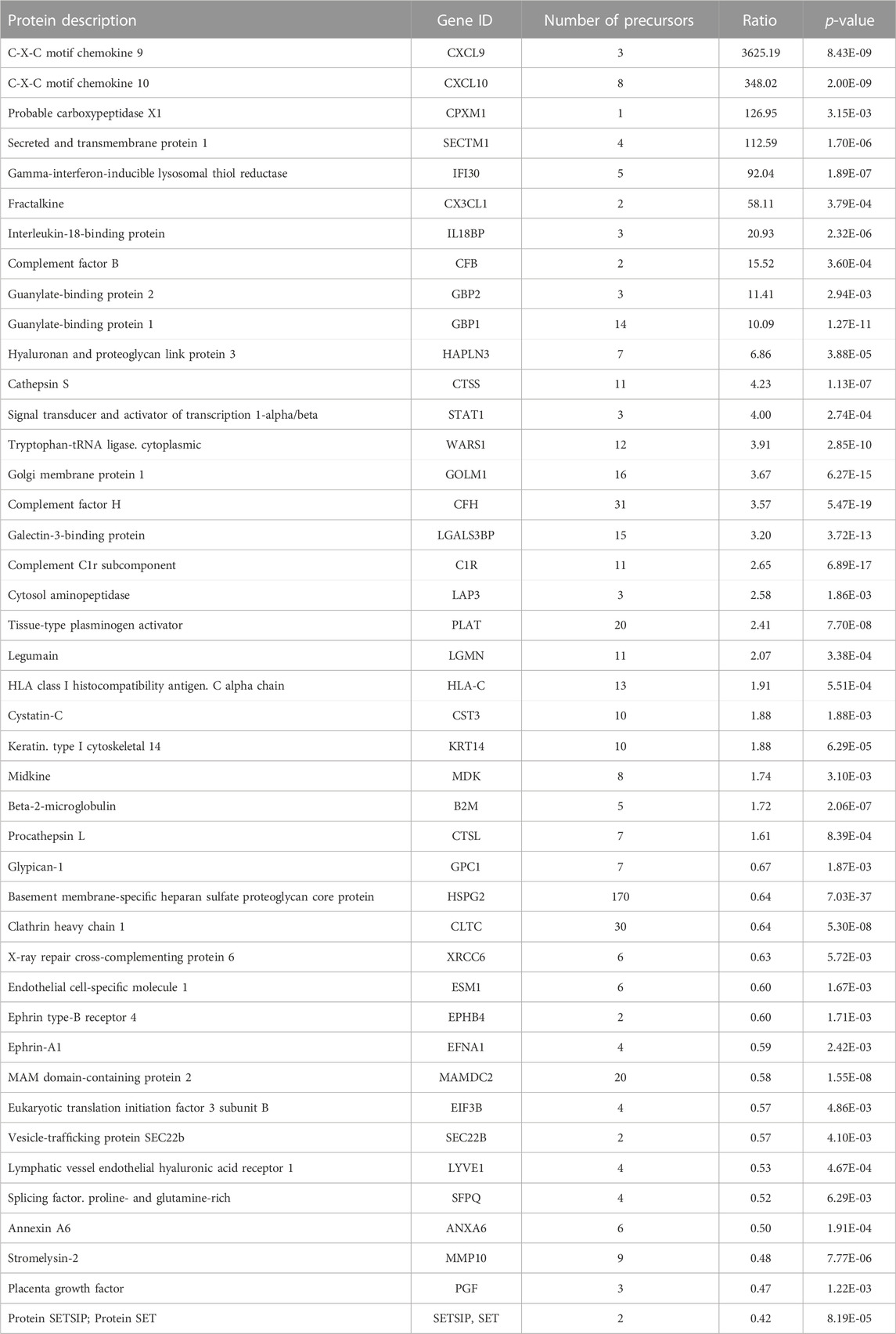
TABLE 1. Significantly changed proteins between the supernatants of IFN-γ treated and untreated HIEC.
Interestingly, with the exception of CXCL10 no further top candidate of our previous meta-analysis was detected in the present study confirming that endothelial cells exhibit high tissue-related heterogeneity and suggesting that HIEC should be preferentially used in order to obtain data of relevance for IBD.
Pathogenic impact of the vascular IFN-γ-secretome in IBD
In order to determine the pathogenic impact of the intestinal vascular IFN-γ-induced secretome, the expression of the top ten secreted proteins was examined in different murine models of experimentally induced colitis, including acute and chronic DSS-colitis as well as oxazolone-induced colitis and T-cell transfer colitis in a next step. The expression of the genes encoding CXCL9, CXCL10, CPMX1, IL18BP and GBP2 was highly significantly increased in each of the different models (Figure 1G). Moreover, GBP-1 and IFI30 showed an increased expression in three and two of the models, respectively (Figure 1G). Only SECTM1, fractalkine and CFB did not show a significant increase of expression in any of the different colitis models (Figure 1G). Altogether, seven of the ten genes, encoding for the most differentially secreted proteins from HIEC in the presence of IFN-γ, also showed significantly increased expression in experimentally induced colitis models, supporting their function in pathogenesis. In the following, the most relevant top candidates involved in IBD pathogenesis retrieved by our screening are discussed in detail.
Discussion
CXCL10
CXCL10 is an 8.7 kDa non-glutamic acid leucine-arginine (ELR)-CXC chemokine, which acts as a ligand for the CXCR3 receptor (Singh et al., 2007). CXCL10 is secreted by several cell types, including endothelial cells, in response to IFN-γ to induce the recruitment and activation of CXCR3+ cells (Singh et al., 2007). CXCL10 is upregulated in colonic tissues of patients with UC and CD compared to control non-IBD tissues (Uguccioni et al., 1999; Zahn et al., 2009; Schroepf et al., 2010; Hosomi et al., 2011; Ostvik et al., 2013). Accordingly, the number of CXCR3-expressing immune cells is increased in the lamina propria of IBD patients (Singh et al., 2007). Expression of CXCL10 in colon biopsies correlates with secondary loss of response to anti-TNF-α therapy after achieving an initial response (Luther et al., 2018). Elevated CXCL10 serum levels correlate with extra-intestinal manifestations indicating that CXCL10 is released into the circulation during IBD (Martinez-Fierro et al., 2019). Furthermore, CXCL10 serum levels are increased in IBD patients with unstable remission compared to patients with stable remission (Kessel et al., 2021). Based on these findings, several clinical trials were performed to test the efficacy of eldelumab, a human monoclonal antibody against CXCL10, as treatment for UC (Trivedi and Adams, 2018). Despite trends towards clinical response and remission, the primary and secondary end points were not met and further dose-response or combination studies are warranted (Danese and Panés, 2014; Mayer et al., 2014; Sandborn et al., 2016). In murine colitis models, inhibition of CXCL10 reduces intestinal inflammation (Sasaki et al., 2002; Singh et al., 2003; Hyun et al., 2005; Suzuki et al., 2007; Zhao et al., 2017) but also had unexpected effects on intestinal epithelial cells (Sasaki et al., 2002; Singh et al., 2003; Hyun et al., 2005; Suzuki et al., 2007; Zhao et al., 2017). Neutralization of CXCL10 resulted in increased epithelial cell proliferation and decreased apoptosis, which resulted in reduced epithelial ulceration and longer colon crypts (Sasaki et al., 2002; Suzuki et al., 2007). In addition, CD patients with the highest levels of the IFN-γ-induced chemokines CXCL9, CXCL10 and CXCL11 showed hypertrophied epithelial layers at multiple sites (Singh et al., 2007). These findings suggest that CXCL10 secreted by endothelial cells during intestinal inflammation is not only involved in immune cell recruitment but also crypt cell growth regulation and extra-intestinal manifestations.
CXCL9
Similarly to CXCL10, CXCL9 is a CXC-chemokine induced by IFN-γ in numerous cell types. It also binds to the CXCR3 receptor, and is involved in the recruitment of granulocytes and mononuclear cells. CXCL9 expression is increased in mucosal samples of UC and CD patients (Hosomi et al., 2011; Elia and Guglielmi, 2018; Caruso, 2019) and positively correlates with disease activity and negatively with response to treatment using corticosteroids in UC or anti-TNF-α in CD (Egesten et al., 2007; Lacher et al., 2007; Luther et al., 2018; Zhong et al., 2022). Serum CXCL9 levels also reflect disease activity in both UC and CD (Caruso, 2019; Bergemalm et al., 2021; Boucher et al., 2022; Chen et al., 2022) and circulating CXCL9 was identified in preclinical CD and UC as an IBD-risk biomarker (Bergemalm et al., 2021; Leibovitzh et al., 2023) that predicts relapse in UC and CD (Kessel et al., 2021; Walshe et al., 2022). At the molecular level, CXCL9 has been shown to inhibit the reconstitution of the intestinal mucosa after injury (Lu et al., 2015) and to control E. coli overgrowth through the pyruvate dehydrogenase-encoding aceE gene in a DSS-induced colitis model (Wei et al., 2022). Hence, CXCL9 released by endothelial cells might not only increase immune cell recruitment but also may compromise the epithelial barrier and alter the microbiota in intestinal inflammation.
Fractalkine/CX3CL1
Fractalkine (FKN/CX3CL1) is a transmembrane protein which mediates leukocyte adhesion to endothelial cells (Sans et al., 2007). In addition, a soluble form of fractalkine with chemoattractive properties is secreted by cleavage. Its receptor, CX3CR1, is expressed primarily on the surface of monocytes, natural killer cells, and CD8+ T cells and mediates both adhesive and chemoattractive functions (Sans et al., 2007). Fractalkine expression is upregulated by inflammatory cytokines (IFN-γ, IL-1β and TNF-α) or by direct leukocyte contact (Muehlhoefer et al., 2000; Sans et al., 2007), and has been detected in intestinal epithelial cells and endothelial cells both in normal small intestine and in active Crohn’s disease mucosa (Muehlhoefer et al., 2000). However, significantly higher levels of fractalkine mRNA were found in the intestine during active CD and UC (Muehlhoefer et al., 2000; Brand et al., 2006; Kobayashi et al., 2007). Similarly, HIECs isolated from IBD patients exhibited significantly stronger fractalkine expression as compared to control HIECs (Sans et al., 2007). This correlated with significantly higher numbers of mucosal circulating CX3CR1+ T cells in active IBD compared to inactive IBD or healthy subjects (Kobayashi et al., 2007; Sans et al., 2007). The presence of two CX3CR1 polymorphisms (T280M and V249I) has been associated with intestinal stenosis in CD patients (Brand et al., 2006; Sabate et al., 2008). The knockout/blockade of fractalkine attenuated mucosal inflammation in murine colitis models and showed a moderate clinical response in CD patients (Wakita et al., 2017; Kuboi et al., 2019; Tabuchi et al., 2019; Matsuoka et al., 2021). Targeting endothelial fractalkine might be particularly important to block leukocyte adhesion and migration, platelet adhesion and even angiogenesis (Scaldaferri et al., 2009; Rutella et al., 2011).
GBP-1 and GBP-2
Two members of the guanylate binding protein family, GBP-1 and GBP-2, were detected in our analysis. GBPs are large GTPases, which are expressed in response to stimulation by inflammatory cytokines (Britzen-Laurent et al., 2016). GBP-1 is among the most highly induced proteins by IFN-γ in eukaryotic cells. In vivo, a strong expression of GBP-1 is associated with the presence of inflammation and was detected in inflamed tissues during autoimmune diseases or IBD, where it is mostly associated with blood vessels (Lubeseder-Martellato et al., 2002; Haep et al., 2015; Ning et al., 2023). In pediatric patients with IBD, a high expression of GBP-1 was associated with an absence of early response to anti-TNF treatment (Salvador-Martín et al., 2021). Murine GBP-1/GBP-2b is also upregulated during experimental colitis (de Buhr et al., 2006). Intracellular expression of GBP-1 inhibits angiogenesis in endothelial cells (Guenzi et al., 2001), and inhibits proliferation and migration in tumor cells and intestinal epithelial cells, while preventing cell apoptosis (Schnoor et al., 2009; Britzen-Laurent et al., 2013; Ostler et al., 2014). GBP-1 is also able to regulate T-cell receptor signaling (Forster et al., 2014). Interestingly, GBP-1 is specifically and efficiently secreted from endothelial cells by a non-classical, likely ABC transporter-dependent, pathway (Naschberger et al., 2006; Naschberger et al., 2017; Carbotti et al., 2020). GBP-1 has been detected in the serum or cerebrospinal fluid during infectious and inflammatory diseases including bacterial meningitis, systemic lupus erythematosus, rheumatoid arthritis and systemic sclerosis (Naschberger et al., 2006; Hammon et al., 2011; Naschberger et al., 2017). The functions of secreted GBP-1 and GBP-2 remain unknown and further studies are warranted to investigate their potential as blood biomarkers in IBD, as well as their function on the intestinal epithelial barrier.
IL-18BP
IL-18 binding protein (IL-18BP) is a natural circulating high-affinity antagonist of interleukin-18 (IL-18), which belongs to the IL-1 superfamily. While IL-18 is produced by a range of immune and non-immune cells including macrophages, dendritic cells (DCs), fibroblasts and intestinal epithelial cells, its receptor (IL-18R) is expressed by T cells, macrophages, NK-cells or endothelial cells (Kaplanski, 2018). IL-18BP blocks the binding of IL-18 to IL-18R, thereby dampening IFN-γ production. In children and adult CD patients, elevated expression of both IL-18 and IL-18BP has been detected in mucosal samples, with intestinal endothelial cells and macrophages being the major sources of IL-18BP (Corbaz et al., 2002). Higher IL-18 and IL-18BP levels have also been observed in the serum of IBD patients as compared to controls, which might be attributed to secretion by endothelial cells (Corbaz et al., 2002; Ludwiczek et al., 2005; Naftali et al., 2007; Leach et al., 2008). In particular in CD, circulating levels of both IL-18 and IL-18BP correlated with disease activity, which is well in agreement with the exacerbated Th1 immune response characteristic of the disease (Corbaz et al., 2002; Ludwiczek et al., 2005; Naftali et al., 2007; Leach et al., 2008). However, high levels of free unbound IL-18 are still detectable in CD patients, suggesting that IL-18BP is not produced in sufficient amounts to compensate the effects of IL-18 (Corbaz et al., 2002; Ludwiczek et al., 2005; Naftali et al., 2007; Leach et al., 2008). In DSS-induced experimental colitis the administration of IL-18BP or the neutralization of IL-18 was able to attenuate intestinal inflammation and weight loss (Siegmund et al., 2001; Sivakumar et al., 2002; Siegmund et al., 2004). IL-18BP may act anti-inflammatory not only by inhibition of immune cell recruitment but also through inhibition of IL-18-induced intestinal epithelial permeability (Allam et al., 2018). This is supported by the fact that, the knock-out of IL-18 in endothelial cells, hematopoietic cells or in intestinal epithelial cells was found to abrogate DSS-induced colitis, while the knock-out of IL-18R was only protective when present in intestinal epithelial cells (Nowarski et al., 2015). Overall, IL-18BP is produced and released during IBD, notably by endothelial cells, where it exerts protective effects by dampening the pro-inflammatory effects of IL-18.
Complement factors
Our analysis has revealed an increased secretion of three complement system members in IFN-γ-stimulated intestinal endothelial cells: the complement C1r subcomponent (C1R) from the classical pathway and the complement factor B (CFB) and H (CFH) from the alternative pathway (Lubbers et al., 2017). Complement proteins are produced and secreted mostly by hepatocytes but also by endothelial cells, epithelial cells and leukocytes (Morgan and Gasque, 1997; Lubbers et al., 2017). IBD patients exhibit increased levels of circulating CFB (Nielsen et al., 1978; Campbell et al., 1982; Adinolfi and Lehner, 1988) and a similar increase of serum CFB has been observed in DSS-induced and bisphenol A (BPA)-induced experimental colitis in mice (Huang et al., 2022). More recently, genome-wide association studies (GWAS) have identified one SNP (rs4151657) at the CFB locus, which represents a risk variant for UC susceptibility (Juyal et al., 2015; Gupta et al., 2016; Shi et al., 2020; Mortlock et al., 2023). The presence of the rs4151651 SNP was associated with increased CFB expression, and CFB expression was shown to correlate with disease activity (Shi et al., 2020; Mortlock et al., 2023). CFB expression can be induced in human glomerular endothelial cells and intestinal epithelial cells by different inflammatory cytokines and is found in increased concentrations in the jejunal fluid of IBD patients (Ahrenstedt et al., 1990; Ostvik et al., 2014; Sartain et al., 2016). In contrast to CFB, very little is known about the role of CFH and C1r in IBD. C1r concentration was significantly increased in the serum of CD patients in clinical and serological remission in response to treatment with the anti-TNF-α antibody infliximab, suggesting an inverse correlation between C1r production and disease activity (Gazouli et al., 2013).
Potential new angiocrine factors in IBD
Little is known about the role of CPXM1, IFI30 and SECTM1 in IBD, which were also among the top ten candidates of our screening. SECTM1 is an IFN-γ-regulated molecule acting as a co-stimulatory molecule in T and NK cells, where it binds CD7 (Lyman et al., 2000; Wang et al., 2012; Hubel et al., 2019). SECTM1 is expressed by antigen-presenting cells and epithelial cells that may also secrete a soluble form (Lam et al., 2005; Kamata et al., 2016). Carboxypeptidase X-1 (CPX-1), an inactive member of the metallocarboxypeptidase family encoded by the CPXM1 gene, is also a secreted protein (Reznik and Fricker, 2001; Kim et al., 2015). CPXM1 expression is upregulated in the inflamed intestinal mucosa of CD patients (Hong et al., 2017). Finally, IFN-γ-inducible lysosomal thiol reductase (IFI30/GILT) is a thiol reductase involved in the processing of antigenic proteins for antigen presentation by MHC class II molecules (Barjaktarević et al., 2006). Upregulation of IFI30 has been observed in uterine microvascular endothelial cells in response to IFN-γ (Kitaya et al., 2007).
In conclusion, the important pathogenic role of the vasculature in IBD has been appreciated only recently. Here, we identified and discussed factors secreted from HIEC in the presence of IFN-γ stimulation. Among these factors, CXCL9, CXCL10 and fractalkine have been already described to be closely associated with IBD pathogenesis either in preclinical murine models or in patients. Moreover, we identified novel factors secreted from IFN-γ-activated HIEC including GBP-1, GBP-2, CPXM1, IFI30 and SECTM1. These factors may warrant further studies on their role in IBD pathogenesis and as target for disease monitoring.
Data availability statement
Datasets are available on request: The raw data supporting the conclusions of this article will be made available by the authors, without undue reservation.
Ethics statement
The study involving humans was approved by the Ethics committee of the Friedrich-Alexander-Universität Erlangen- Nürnberg (AZ 159_15B, TuMiC Study) and written informed consent was provided by the patients. The studies were conducted in accordance with the local legislation and institutional requirements. The human samples used in this study were acquired from primarily isolated as part of your previous study for which ethical approval was obtained. Written informed consent for participation was not required from the participants or the participants’ legal guardians/next of kin. The animal study was approved by the Institutional Animal Care and Use Committee of the University of Erlangen and the Animal Experiment Committee of the State Government of Lower Franconia, Würzburg, Germany (55.2 2532–2-1137 and -1178). The studies were conducted in accordance with the local legislation and institutional requirements.
Author contributions
Concept of manuscript: MS and NB-L. Experimental work: CB, CF, EN, LE, JH, MB, MG-A, RG-B, and VSS. Data analyses: CB, EN, LE, MG-A, MB, MM, MS, RG-B, and NB-L. Writing of manuscript: EN, MS, and NB-L. All authors contributed to the article and approved the submitted version.
Funding
This work was funded by the Deutsche Forschungsgemeinschaft (DFG, German Research Foundation) Project-ID 375876048—TRR 241 subproject A03 to CB and A06 to NB-L and MS, Project-ID 429280966—TRR 305 subproject B07 to NB-L and B08 to EN, Project-ID 437201724—STU 238/10-1 to MS, Project-ID 280163318—FOR 2438-2 to EN and MS, Project-ID 292410854—BR 5196/2-1 to NB-L; by the W. Lutz Stiftung, the Forschungsstiftung Medizin am Universitätsklinikum Erlangen and a start-up funding from the TRR 241 to NB-L and MS.
Conflict of interest
Authors MB and MM were employed by Biognosys AG.
The remaining authors declare that the research was conducted in the absence of any commercial or financial relationships that could be construed as a potential conflict of interest.
Publisher’s note
All claims expressed in this article are solely those of the authors and do not necessarily represent those of their affiliated organizations, or those of the publisher, the editors and the reviewers. Any product that may be evaluated in this article, or claim that may be made by its manufacturer, is not guaranteed or endorsed by the publisher.
Supplementary material
The Supplementary Material for this article can be found online at: https://www.frontiersin.org/articles/10.3389/fcell.2023.1213383/full#supplementary-material
References
Adinolfi, M., and Lehner, T. (1988). C9 and factor B as acute phase proteins and their diagnostic and prognostic value in disease. Exp. Clin. Immunogenet 5 (2-3), 123–132.
Ahrenstedt, O., Knutson, L., Nilsson, B., Nilsson-Ekdahl, K., Odlind, B., and Hällgren, R. (1990). Enhanced local production of complement components in the small intestines of patients with Crohn's disease. N. Engl. J. Med. 322 (19), 1345–1349. doi:10.1056/NEJM199005103221903
Allam, O., Samarani, S., Mehraj, V., Jenabian, M. A., Tremblay, C., Routy, J. P., et al. (2018). HIV induces production of IL-18 from intestinal epithelial cells that increases intestinal permeability and microbial translocation. PLoS One 13 (3), 0194185. doi:10.1371/journal.pone.0194185
Barjaktarević, I., Rahman, A., Radoja, S., Bogunović, B., Vollmer, A., Vukmanović, S., et al. (2006). Inhibitory role of IFN-gamma-inducible lysosomal thiol reductase in T cell activation. J. Immunol. 177 (7), 4369–4375. doi:10.4049/jimmunol.177.7.4369
Bergemalm, D., Andersson, E., Hultdin, J., Eriksson, C., Rush, S. T., Kalla, R., et al. (2021). Systemic inflammation in preclinical ulcerative colitis. Gastroenterology 161 (5), 1526–1539.e9. doi:10.1053/j.gastro.2021.07.026
Binion, D. G., West, G. A., Volk, E. E., Drazba, J. A., Ziats, N. P., Petras, R. E., et al. (1998). Acquired increase in leucocyte binding by intestinal microvascular endothelium in inflammatory bowel disease. Lancet 352 (9142), 1742–1746. doi:10.1016/S0140-6736(98)05050-8
Boucher, G., Paradis, A., Chabot-Roy, G., Coderre, L., Hillhouse, E. E., Bitton, A., et al. (2022). Serum analyte profiles associated with Crohn's disease and disease location. Inflamm. Bowel Dis. 28 (1), 9–20. doi:10.1093/ibd/izab123
Boueiz, A., and Hassoun, P. M. (2009). Regulation of endothelial barrier function by reactive oxygen and nitrogen species. Microvasc. Res. 77 (1), 26–34. doi:10.1016/j.mvr.2008.10.005
Brand, S., Hofbauer, K., Dambacher, J., Schnitzler, F., Staudinger, T., Pfennig, S., et al. (2006). Increased expression of the chemokine fractalkine in Crohn's disease and association of the fractalkine receptor T280M polymorphism with a fibrostenosing disease Phenotype. Am. J. Gastroenterol. 101 (1), 99–106. doi:10.1111/j.1572-0241.2005.00361.x
Britzen-Laurent, N., Herrmann, C., Naschberger, E., Croner, R. S., and Stürzl, M. (2016). Pathophysiological role of guanylate-binding proteins in gastrointestinal diseases. World J. Gastroenterol. 22 (28), 6434–6443. doi:10.3748/wjg.v22.i28.6434
Britzen-Laurent, N., Lipnik, K., Ocker, M., Naschberger, E., Schellerer, V. S., Croner, R. S., et al. (2013). GBP-1 acts as a tumor suppressor in colorectal cancer cells. Carcinogenesis 34 (1), 153–162. doi:10.1093/carcin/bgs310
Butler, J. M., Kobayashi, H., and Rafii, S. (2010). Instructive role of the vascular niche in promoting tumour growth and tissue repair by angiocrine factors. Nat. Rev. Cancer 10 (2), 138–146. doi:10.1038/nrc2791
Campbell, C. A., Walker-Smith, J. A., Hindocha, P., and Adinolfi, M. (1982). Acute phase proteins in chronic inflammatory bowel disease in childhood. J. Pediatr. Gastroenterol. Nutr. 1 (2), 193–200. doi:10.1097/00005176-198201020-00007
Carbotti, G., Petretto, A., Naschberger, E., Stürzl, M., Martini, S., Mingari, M. C., et al. (2020). Cytokine-induced guanylate binding protein 1 (GBP1) release from human ovarian cancer cells. Cancers (Basel) 12 (2), 488. doi:10.3390/cancers12020488
Chen, J., Zhou, Y., Sun, Y., Yuan, S., Kalla, R., Sun, J., et al. (2022). Bi-directional Mendelian randomization analysis provides evidence for the causal involvement of dysregulation of CXCL9, CCL11 and CASP8 in the pathogenesis of ulcerative colitis. J. Crohns Colitis 17 (5), 777–785. doi:10.1093/ecco-jcc/jjac191
Corbaz, A., ten Hove, T., Herren, S., Graber, P., Schwartsburd, B., Belzer, I., et al. (2002). IL-18-binding protein expression by endothelial cells and macrophages is up-regulated during active Crohn's disease. J. Immunol. 168 (7), 3608–3616. doi:10.4049/jimmunol.168.7.3608
Danese, S., and Panés, J. (2014). Development of drugs to target interactions between leukocytes and endothelial cells and treatment algorithms for inflammatory bowel diseases. Gastroenterology 147 (5), 981–989. doi:10.1053/j.gastro.2014.08.044
de Buhr, M. F., Mähler, M., Geffers, R., Hansen, W., Westendorf, A. M., Lauber, J., et al. (2006). Cd14, Gbp1, and Pla2g2a: three major candidate genes for experimental IBD identified by combining QTL and microarray analyses. Physiol. Genomics 25 (3), 426–434. doi:10.1152/physiolgenomics.00022.2005
Egesten, A., Eliasson, M., Olin, A. I., Erjefält, J. S., Bjartell, A., Sangfelt, P., et al. (2007). The proinflammatory CXC-chemokines GRO-alpha/CXCL1 and MIG/CXCL9 are concomitantly expressed in ulcerative colitis and decrease during treatment with topical corticosteroids. Int. J. Colorectal Dis. 22 (12), 1421–1427. doi:10.1007/s00384-007-0370-3
Elia, G., and Guglielmi, G. (2018). CXCL9 chemokine in ulcerative colitis. Clin. Ter. 169 (5), e235–e241. doi:10.7417/CT.2018.2085
Ferrari, D., Cimino, F., Fratantonio, D., Molonia, M. S., Bashllari, R., Busà, R., et al. (2017). Cyanidin-3-O-Glucoside modulates the in vitro inflammatory crosstalk between intestinal epithelial and endothelial cells. Mediat. Inflamm. 2017, 3454023. doi:10.1155/2017/3454023
Forster, F., Paster, W., Supper, V., Schatzlmaier, P., Sunzenauer, S., Ostler, N., et al. (2014). Guanylate binding protein 1-mediated interaction of T cell antigen receptor signaling with the cytoskeleton. J. Immunol. 192 (2), 771–781. doi:10.4049/jimmunol.1300377
Franses, J. W., Baker, A. B., Chitalia, V. C., and Edelman, E. R. (2011). Stromal endothelial cells directly influence cancer progression. Sci. Transl. Med. 3 (66), 66ra5. doi:10.1126/scitranslmed.3001542
Franze, E., Marafini, I., De Simone, V., Monteleone, I., Caprioli, F., Colantoni, A., et al. (2016). Interleukin-34 induces cc-chemokine ligand 20 in gut epithelial cells. J. Crohns Colitis 10 (1), 87–94. doi:10.1093/ecco-jcc/jjv181
Gazouli, M., Anagnostopoulos, A. K., Papadopoulou, A., Vaiopoulou, A., Papamichael, K., Mantzaris, G., et al. (2013). Serum protein profile of Crohn's disease treated with infliximab. J. Crohns Colitis 7 (10), e461–e470. doi:10.1016/j.crohns.2013.02.021
Gentile, M. E., and King, I. L. (2018). Blood and guts: the intestinal vasculature during health and helminth infection. PLoS Pathog. 14 (7), 1007045. doi:10.1371/journal.ppat.1007045
Guenzi, E., Töpolt, K., Cornali, E., Lubeseder-Martellato, C., Jörg, A., Matzen, K., et al. (2001). The helical domain of GBP-1 mediates the inhibition of endothelial cell proliferation by inflammatory cytokines. EMBO J. 20 (20), 5568–5577. doi:10.1093/emboj/20.20.5568
Gupta, A., Juyal, G., Sood, A., Midha, V., Yamazaki, K., Vich Vila, A., et al. (2016). A cross-ethnic survey of CFB and SLC44A4, Indian ulcerative colitis GWAS hits, underscores their potential role in disease susceptibility. Eur. J. Hum. Genet. 25 (1), 111–122. doi:10.1038/ejhg.2016.131
Haep, L., Britzen-Laurent, N., Weber, T. G., Naschberger, E., Schaefer, A., Kremmer, E., et al. (2015). Interferon gamma counteracts the angiogenic switch and induces vascular permeability in dextran sulfate sodium colitis in mice. Inflamm. Bowel Dis. 21 (10), 2360–2371. doi:10.1097/MIB.0000000000000490
Hammon, M., Herrmann, M., Bleiziffer, O., Pryymachuk, G., Andreoli, L., Munoz, L. E., et al. (2011). Role of guanylate binding protein-1 in vascular defects associated with chronic inflammatory diseases. J. Cell Mol. Med. 15 (7), 1582–1592. doi:10.1111/j.1582-4934.2010.01146.x
Hinshaw, D. C., and Shevde, L. A. (2019). The tumor microenvironment innately modulates cancer progression. Cancer Res. 79 (18), 4557–4566. doi:10.1158/0008-5472.CAN-18-3962
Hong, S. N., Joung, J. G., Bae, J. S., Lee, C. S., Koo, J. S., Park, S. J., et al. (2017). RNA-Seq reveals transcriptomic differences in inflamed and noninflamed intestinal mucosa of Crohn's disease patients compared with normal mucosa of healthy controls. Inflamm. Bowel Dis. 23 (7), 1098–1108. doi:10.1097/MIB.0000000000001066
Hosomi, S., Oshitani, N., Kamata, N., Sogawa, M., Okazaki, H., Tanigawa, T., et al. (2011). Increased numbers of immature plasma cells in peripheral blood specifically overexpress chemokine receptor CXCR3 and CXCR4 in patients with ulcerative colitis. Clin. Exp. Immunol. 163 (2), 215–224. doi:10.1111/j.1365-2249.2010.04290.x
Huang, C., Wang, Y., Lin, X., Chan, T. F., Lai, K. P., and Li, R. (2022). Uncovering the functions of plasma proteins in ulcerative colitis and identifying biomarkers for BPA-induced severe ulcerative colitis: a plasma proteome analysis. Ecotoxicol. Environ. Saf. 242, 113897. doi:10.1016/j.ecoenv.2022.113897
Hubel, P., Urban, C., Bergant, V., Schneider, W. M., Knauer, B., Stukalov, A., et al. (2019). A protein-interaction network of interferon-stimulated genes extends the innate immune system landscape. Nat. Immunol. 20 (4), 493–502. doi:10.1038/s41590-019-0323-3
Hyun, J. G., Lee, G., Brown, J. B., Grimm, G. R., Tang, Y., Mittal, N., et al. (2005). Anti-interferon-inducible chemokine, CXCL10, reduces colitis by impairing T helper-1 induction and recruitment in mice. Inflamm. Bowel Dis. 11 (9), 799–805. doi:10.1097/01.mib.0000178263.34099.89
Juyal, G., Negi, S., Sood, A., Gupta, A., Prasad, P., Senapati, S., et al. (2015). Genome-wide association scan in north Indians reveals three novel HLA-independent risk loci for ulcerative colitis. Gut 64 (4), 571–579. doi:10.1136/gutjnl-2013-306625
Kamata, H., Yamamoto, K., Wasserman, G. A., Zabinski, M. C., Yuen, C. K., Lung, W. Y., et al. (2016). Epithelial cell-derived secreted and transmembrane 1a signals to activated neutrophils during pneumococcal pneumonia. Am. J. Respir. Cell Mol. Biol. 55 (3), 407–418. doi:10.1165/rcmb.2015-0261OC
Kaplanski, G. (2018). Interleukin-18: biological properties and role in disease pathogenesis. Immunol. Rev. 281 (1), 138–153. doi:10.1111/imr.12616
Kessel, C., Lavric, M., Weinhage, T., Brueckner, M., de Roock, S., Däbritz, J., et al. (2021). Serum biomarkers confirming stable remission in inflammatory bowel disease. Sci. Rep. 11 (1), 6690. doi:10.1038/s41598-021-86251-w
Kim, Y. H., O'Neill, H. M., and Whitehead, J. P. (2015). Carboxypeptidase X-1 (CPX-1) is a secreted collagen-binding glycoprotein. Biochem. Biophys. Res. Commun. 468 (4), 894–899. doi:10.1016/j.bbrc.2015.11.053
Kitaya, K., Yasuo, T., Yamaguchi, T., Fushiki, S., and Honjo, H. (2007). Genes regulated by interferon-gamma in human uterine microvascular endothelial cells. Int. J. Mol. Med. 20 (5), 689–697.
Kobayashi, T., Okamoto, S., Iwakami, Y., Nakazawa, A., Hisamatsu, T., Chinen, H., et al. (2007). Exclusive increase of CX3CR1+CD28-CD4+ T cells in inflammatory bowel disease and their recruitment as intraepithelial lymphocytes. Inflamm. Bowel Dis. 13 (7), 837–846. doi:10.1002/ibd.20113
Kuboi, Y., Nishimura, M., Ikeda, W., Nakatani, T., Seki, Y., Yamaura, Y., et al. (2019). Blockade of the fractalkine-CX3CR1 axis ameliorates experimental colitis by dislodging venous crawling monocytes. Int. Immunol. 31 (5), 287–302. doi:10.1093/intimm/dxz006
Lacher, M., Kappler, R., Berkholz, S., Baurecht, H., von Schweinitz, D., and Koletzko, S. (2007). Association of a CXCL9 polymorphism with pediatric Crohn's disease. Biochem. Biophys. Res. Commun. 363 (3), 701–707. doi:10.1016/j.bbrc.2007.09.020
Lam, G. K., Liao, H. X., Xue, Y., Alam, S. M., Scearce, R. M., Kaufman, R. E., et al. (2005). Expression of the CD7 ligand K-12 in human thymic epithelial cells: regulation by IFN-gamma. J. Clin. Immunol. 25 (1), 41–49. doi:10.1007/s10875-005-0356-5
Langer, V., Vivi, E., Regensburger, D., Winkler, T. H., Waldner, M. J., Rath, T., et al. (2019). IFN-gamma drives inflammatory bowel disease pathogenesis through VE-cadherin-directed vascular barrier disruption. J. Clin. Invest. 129 (11), 4691–4707. doi:10.1172/JCI124884
Leach, S. T., Messina, I., Lemberg, D. A., Novick, D., Rubenstein, M., and Day, A. S. (2008). Local and systemic interleukin-18 and interleukin-18-binding protein in children with inflammatory bowel disease. Inflamm. Bowel Dis. 14 (1), 68–74. doi:10.1002/ibd.20272
Leibovitzh, H., Lee, S. H., Raygoza Garay, J. A., Espin-Garcia, O., Xue, M., Neustaeter, A., et al. (2023). Immune response and barrier dysfunction-related proteomic signatures in preclinical phase of Crohn's disease highlight earliest events of pathogenesis. Gut 72, 1462–1471. doi:10.1136/gutjnl-2022-328421
Lloyd-Price, J., Arze, C., Ananthakrishnan, A. N., Schirmer, M., Avila-Pacheco, J., Poon, T. W., et al. (2019). Multi-omics of the gut microbial ecosystem in inflammatory bowel diseases. Nature 569 (7758), 655–662. doi:10.1038/s41586-019-1237-9
López-Posadas, R., Stürzl, M., Atreya, I., Neurath, M. F., and Britzen-Laurent, N. (2017). Interplay of GTPases and cytoskeleton in cellular barrier defects during gut inflammation. Front. Immunol. 8, 1240. doi:10.3389/fimmu.2017.01240
Lu, H., Liu, H., Wang, J., Shen, J., Weng, S., Han, L., et al. (2015). The chemokine CXCL9 exacerbates chemotherapy-induced acute intestinal damage through inhibition of mucosal restitution. J. Cancer Res. Clin. Oncol. 141 (6), 983–992. doi:10.1007/s00432-014-1869-y
Lubbers, R., van Essen, M. F., van Kooten, C., and Trouw, L. A. (2017). Production of complement components by cells of the immune system. Clin. Exp. Immunol. 188 (2), 183–194. doi:10.1111/cei.12952
Lubeseder-Martellato, C., Guenzi, E., Jörg, A., Töpolt, K., Naschberger, E., Kremmer, E., et al. (2002). Guanylate-binding protein-1 expression is selectively induced by inflammatory cytokines and is an activation marker of endothelial cells during inflammatory diseases. Am. J. Pathol. 161 (5), 1749–1759. doi:10.1016/S0002-9440(10)64452-5
Ludwiczek, O., Kaser, A., Novick, D., Dinarello, C. A., Rubinstein, M., and Tilg, H. (2005). Elevated systemic levels of free interleukin-18 (IL-18) in patients with Crohn's disease. Eur. Cytokine Netw. 16 (1), 27–33.
Luther, J., Gala, M., Patel, S. J., Dave, M., Borren, N., Xavier, R. J., et al. (2018). Loss of response to anti-tumor necrosis factor alpha therapy in Crohn's disease is not associated with emergence of novel inflammatory pathways. Dig. Dis. Sci. 63 (3), 738–745. doi:10.1007/s10620-018-4932-8
Lyman, S. D., Escobar, S., Rousseau, A. M., Armstrong, A., and Fanslow, W. C. (2000). Identification of CD7 as a cognate of the human K12 (SECTM1) protein. J. Biol. Chem. 275 (5), 3431–3437. doi:10.1074/jbc.275.5.3431
Martinez-Fierro, M. L., Garza-Veloz, I., Rocha-Pizaña, M. R., Cardenas-Vargas, E., Cid-Baez, M. A., Trejo-Vazquez, F., et al. (2019). Serum cytokine, chemokine, and growth factor profiles and their modulation in inflammatory bowel disease. Med. Baltim. 98 (38), e17208. doi:10.1097/MD.0000000000017208
Matsuoka, K., Naganuma, M., Hibi, T., Tsubouchi, H., Oketani, K., Katsurabara, T., et al. (2021). Phase 1 study on the safety and efficacy of E6011, antifractalkine antibody, in patients with Crohn's disease. J. Gastroenterol. Hepatol. 36 (8), 2180–2186. doi:10.1111/jgh.15463
Mayer, L., Sandborn, W. J., Stepanov, Y., Geboes, K., Hardi, R., Yellin, M., et al. (2014). Anti-IP-10 antibody (BMS-936557) for ulcerative colitis: a phase II randomised study. Gut 63 (3), 442–450. doi:10.1136/gutjnl-2012-303424
Morgan, B. P., and Gasque, P. (1997). Extrahepatic complement biosynthesis: where, when and why? Clin. Exp. Immunol. 107 (1), 1–7. doi:10.1046/j.1365-2249.1997.d01-890.x
Mortlock, S., Lord, A., Montgomery, G., Zakrzewski, M., Simms, L. A., Krishnaprasad, K., et al. (2023). An extremes of phenotype approach confirms significant genetic heterogeneity in patients with ulcerative colitis. J. Crohns Colitis 17 (2), 277–288. doi:10.1093/ecco-jcc/jjac121
Muehlhoefer, A., Saubermann, L. J., Gu, X., Luedtke-Heckenkamp, K., Xavier, R., Blumberg, R. S., et al. (2000). Fractalkine is an epithelial and endothelial cell-derived chemoattractant for intraepithelial lymphocytes in the small intestinal mucosa. J. Immunol. 164 (6), 3368–3376. doi:10.4049/jimmunol.164.6.3368
Naftali, T., Novick, D., Gabay, G., Rubinstein, M., and Novis, B. (2007). Interleukin-18 and its binding protein in patients with inflammatory bowel disease during remission and exacerbation. Isr. Med. Assoc. J. 9 (7), 504–508.
Naschberger, E., Geißdörfer, W., Bogdan, C., Tripal, P., Kremmer, E., Stürzl, M., et al. (2017). Processing and secretion of guanylate binding protein-1 depend on inflammatory caspase activity. J. Cell Mol. Med. 21 (9), 1954–1966. doi:10.1111/jcmm.13116
Naschberger, E., Liebl, A., Schellerer, V. S., Schütz, M., Britzen-Laurent, N., Kölbel, P., et al. (2016). Matricellular protein SPARCL1 regulates tumor microenvironment-dependent endothelial cell heterogeneity in colorectal carcinoma. J. Clin. Invest. 126 (11), 4187–4204. doi:10.1172/JCI78260
Naschberger, E., Lubeseder-Martellato, C., Meyer, N., Gessner, R., Kremmer, E., Gessner, A., et al. (2006). Human guanylate binding protein-1 is a secreted GTPase present in increased concentrations in the cerebrospinal fluid of patients with bacterial meningitis. Am. J. Pathol. 169 (3), 1088–1099. doi:10.2353/ajpath.2006.060244
Naschberger, E., Regensburger, D., Tenkerian, C., Langheinrich, M., Engel, F. B., Geppert, C., et al. (2018). Isolation of human endothelial cells from normal colon and colorectal carcinoma - an improved protocol. J. Vis. Exp. 4 (134), 57400. doi:10.3791/57400
Neurath, M. F. (2017). Current and emerging therapeutic targets for IBD. Nat. Rev. Gastroenterol. Hepatol. 14 (5), 269–278. doi:10.1038/nrgastro.2016.208
Nielsen, H., Petersen, P. H., and Svehag, S. E. (1978). Circulating immune complexes in ulcerative colitis--II Correlation with serum protein concentrations and complement conversion products. Clin. Exp. Immunol. 31 (1), 81–91.
Ning, Y., Lin, K., Fang, J., Chen, X., Hu, X., Liu, L., et al. (2023). Pyroptosis-related signature predicts the progression of ulcerative colitis and colitis-associated colorectal cancer as well as the anti-TNF therapeutic response. J. Immunol. Res. 2023, 7040113. doi:10.1155/2023/7040113
Nowarski, R., Jackson, R., Gagliani, N., de Zoete, M. R., Palm, N. W., Bailis, W., et al. (2015). Epithelial IL-18 equilibrium controls barrier function in colitis. Cell 163 (6), 1444–1456. doi:10.1016/j.cell.2015.10.072
Ostler, N., Britzen-Laurent, N., Liebl, A., Naschberger, E., Lochnit, G., Ostler, M., et al. (2014). Gamma interferon-induced guanylate binding protein 1 is a novel actin cytoskeleton remodeling factor. Mol. Cell Biol. 34 (2), 196–209. doi:10.1128/MCB.00664-13
Ostvik, A. E., Granlund, A. V. B., Bugge, M., Nilsen, N. J., Torp, S. H., Waldum, H. L., et al. (2013). Enhanced expression of CXCL10 in inflammatory bowel disease: potential role of mucosal toll-like receptor 3 stimulation. Inflamm. Bowel Dis. 19 (2), 265–274. doi:10.1002/ibd.23034
Ostvik, A. E., Granlund, A. v., Gustafsson, B. I., Torp, S. H., Espevik, T., Mollnes, T. E., et al. (2014). Mucosal toll-like receptor 3-dependent synthesis of complement factor B and systemic complement activation in inflammatory bowel disease. Inflamm. Bowel Dis. 20 (6), 995–1003. doi:10.1097/MIB.0000000000000035
Rafii, S., Butler, J. M., and Ding, B. S. (2016). Angiocrine functions of organ-specific endothelial cells. Nature 529 (7586), 316–325. doi:10.1038/nature17040
Reznik, S. E., and Fricker, L. D. (2001). Carboxypeptidases from A to z: implications in embryonic development and wnt binding. Cell Mol. Life Sci. 58 (12-13), 1790–1804. doi:10.1007/PL00000819
Rutella, S., Vetrano, S., Correale, C., Graziani, C., Sturm, A., Spinelli, A., et al. (2011). Enhanced platelet adhesion induces angiogenesis in intestinal inflammation and inflammatory bowel disease microvasculature. J. Cell Mol. Med. 15 (3), 625–634. doi:10.1111/j.1582-4934.2010.01033.x
Sabate, J. M., Ameziane, N., Lamoril, J., Jouet, P., Farmachidi, J. P., Soulé, J. C., et al. (2008). The V249I polymorphism of the CX3CR1 gene is associated with fibrostenotic disease behavior in patients with Crohn's disease. Eur. J. Gastroenterol. Hepatol. 20 (8), 748–755. doi:10.1097/MEG.0b013e3282f824c9
Salvador-Martín, S., Kaczmarczyk, B., Álvarez, R., Navas-López, V. M., Gallego-Fernández, C., Moreno-Álvarez, A., et al. (2021). Whole transcription profile of responders to anti-TNF drugs in pediatric inflammatory bowel disease. Pharmaceutics 13 (1), 77. doi:10.3390/pharmaceutics13010077
Sandborn, W. J., Colombel, J. F., Ghosh, S., Sands, B. E., Dryden, G., Hébuterne, X., et al. (2016). Eldelumab [Anti-IP-10] induction therapy for ulcerative colitis: a randomised, placebo-controlled, phase 2b study. J. Crohns Colitis 10 (4), 418–428. doi:10.1093/ecco-jcc/jjv224
Sans, M., Danese, S., de la Motte, C., de Souza, H. S. P., Rivera-Reyes, B. M., West, G. A., et al. (2007). Enhanced recruitment of CX3CR1+ T cells by mucosal endothelial cell-derived fractalkine in inflammatory bowel disease. Gastroenterology 132 (1), 139–153. doi:10.1053/j.gastro.2006.10.010
Sartain, S. E., Turner, N. A., and Moake, J. L. (2016). TNF regulates essential alternative complement pathway components and impairs activation of protein C in human glomerular endothelial cells. J. Immunol. 196 (2), 832–845. doi:10.4049/jimmunol.1500960
Sasaki, S., Yoneyama, H., Suzuki, K., Suriki, H., Aiba, T., Watanabe, S., et al. (2002). Blockade of CXCL10 protects mice from acute colitis and enhances crypt cell survival. Eur. J. Immunol. 32 (11), 3197–3205. doi:10.1002/1521-4141(200211)32:11<3197::AID-IMMU3197>3.0.CO;2-1
Scaldaferri, F., Sans, M., Vetrano, S., Correale, C., Arena, V., Pagano, N., et al. (2009). The role of MAPK in governing lymphocyte adhesion to and migration across the microvasculature in inflammatory bowel disease. Eur. J. Immunol. 39 (1), 290–300. doi:10.1002/eji.200838316
Schnoor, M., Betanzos, A., Weber, D. A., and Parkos, C. A. (2009). Guanylate-binding protein-1 is expressed at tight junctions of intestinal epithelial cells in response to interferon-gamma and regulates barrier function through effects on apoptosis. Mucosal Immunol. 2 (1), 33–42. doi:10.1038/mi.2008.62
Schroepf, S., Kappler, R., Brand, S., Prell, C., Lohse, P., Glas, J., et al. (2010). Strong overexpression of CXCR3 axis components in childhood inflammatory bowel disease. Inflamm. Bowel Dis. 16 (11), 1882–1890. doi:10.1002/ibd.21312
Shi, D., Zhong, Z., Wang, M., Cai, L., Fu, D., Peng, Y., et al. (2020). Identification of susceptibility locus shared by IgA nephropathy and inflammatory bowel disease in a Chinese Han population. J. Hum. Genet. 65 (3), 241–249. doi:10.1038/s10038-019-0699-9
Siegmund, B., Fantuzzi, G., Rieder, F., Gamboni-Robertson, F., Lehr, H. A., Hartmann, G., et al. (2001). Neutralization of interleukin-18 reduces severity in murine colitis and intestinal IFN-gamma and TNF-alpha production. Am. J. Physiol. Regul. Integr. Comp. Physiol. 281 (4), R1264–R1273. doi:10.1152/ajpregu.2001.281.4.R1264
Siegmund, B., Sennello, J. A., Lehr, H. A., Senaldi, G., Dinarello, C. A., and Fantuzzi, G. (2004). Frontline: interferon regulatory factor-1 as a protective gene in intestinal inflammation: role of TCR gamma delta T cells and interleukin-18-binding protein. Eur. J. Immunol. 34 (9), 2356–2364. doi:10.1002/eji.200425124
Singh, U. P., Singh, S., Taub, D. D., and Lillard, J. W. (2003). Inhibition of IFN-gamma-inducible protein-10 abrogates colitis in IL-10-/- mice. J. Immunol. 171 (3), 1401–1406. doi:10.4049/jimmunol.171.3.1401
Singh, U. P., Venkataraman, C., Singh, R., and Lillard, J. W. (2007). CXCR3 axis: role in inflammatory bowel disease and its therapeutic implication. Endocr. Metab. Immune Disord. Drug Targets 7 (2), 111–123. doi:10.2174/187153007780832109
Sivakumar, P. V., Westrich, G. M., Kanaly, S., Garka, K., Born, T. L., Derry, J. M. J., et al. (2002). Interleukin 18 is a primary mediator of the inflammation associated with dextran sulphate sodium induced colitis: blocking interleukin 18 attenuates intestinal damage. Gut 50 (6), 812–820. doi:10.1136/gut.50.6.812
Spadoni, I., Fornasa, G., and Rescigno, M. (2017). Organ-specific protection mediated by cooperation between vascular and epithelial barriers. Nat. Rev. Immunol. 17 (12), 761–773. doi:10.1038/nri.2017.100
Spadoni, I., Zagato, E., Bertocchi, A., Paolinelli, R., Hot, E., Di Sabatino, A., et al. (2015). A gut-vascular barrier controls the systemic dissemination of bacteria. Science 350 (6262), 830–834. doi:10.1126/science.aad0135
Stan, R. V., Tse, D., Deharvengt, S. J., Smits, N. C., Xu, Y., Luciano, M. R., et al. (2012). The diaphragms of fenestrated endothelia: gatekeepers of vascular permeability and blood composition. Dev. Cell 23 (6), 1203–1218. doi:10.1016/j.devcel.2012.11.003
Storey, J. D., and Tibshirani, R. (2003). Statistical significance for genomewide studies. Proc. Natl. Acad. Sci. U. S. A. 100 (16), 9440–9445. doi:10.1073/pnas.1530509100
Stürzl, M., Kunz, M., Krug, S. M., and Naschberger, E. (2021). Angiocrine regulation of epithelial barrier integrity in inflammatory bowel disease. Front. Med. (Lausanne) 8, 643607. doi:10.3389/fmed.2021.643607
Suzuki, K., Kawauchi, Y., Palaniyandi, S. S., Veeraveedu, P. T., Fujii, M., Yamagiwa, S., et al. (2007). Blockade of interferon-gamma-inducible protein-10 attenuates chronic experimental colitis by blocking cellular trafficking and protecting intestinal epithelial cells. Pathol. Int. 57 (7), 413–420. doi:10.1111/j.1440-1827.2007.02117.x
Tabuchi, H., Katsurabara, T., Mori, M., Aoyama, M., Obara, T., Yasuda, N., et al. (2019). Pharmacokinetics, pharmacodynamics, and safety of E6011, a novel humanized antifractalkine (CX3CL1) monoclonal antibody: a randomized, double-blind, placebo-controlled single-ascending-dose study. J. Clin. Pharmacol. 59 (5), 688–701. doi:10.1002/jcph.1361
Trivedi, P. J., and Adams, D. H. (2018). Chemokines and chemokine receptors as therapeutic targets in inflammatory bowel disease; pitfalls and promise. J. Crohns Colitis 12 (2), 1508–s652. doi:10.1093/ecco-jcc/jjy130
Uguccioni, M., Gionchetti, P., Robbiani, D. F., Rizzello, F., Peruzzo, S., Campieri, M., et al. (1999). Increased expression of IP-10, IL-8, MCP-1, and MCP-3 in ulcerative colitis. Am. J. Pathol. 155 (2), 331–336. doi:10.1016/S0002-9440(10)65128-0
Wakita, H., Yanagawa, T., Kuboi, Y., and Imai, T. (2017). E6130, a novel CX3C chemokine receptor 1 (CX3CR1) modulator, attenuates mucosal inflammation and reduces CX3CR1(+) leukocyte trafficking in mice with colitis. Mol. Pharmacol. 92 (5), 502–509. doi:10.1124/mol.117.108381
Walshe, M., Nayeri, S., Ji, J., Hernandez-Rocha, C., Sabic, K., Hu, L., et al. (2022). A role for CXCR3 ligands as biomarkers of post-operative Crohn's disease recurrence. J. Crohns Colitis 16 (6), 900–910. doi:10.1093/ecco-jcc/jjab186
Wang, T., Huang, C., Lopez-Coral, A., Slentz-Kesler, K. A., Xiao, M., Wherry, E. J., et al. (2012). K12/SECTM1, an interferon-γ regulated molecule, synergizes with CD28 to costimulate human T cell proliferation. J. Leukoc. Biol. 91 (3), 449–459. doi:10.1189/jlb.1011498
Wei, J., Zhang, C., Gao, Y., Li, Y., Zhang, Q., Qi, H., et al. (2022). Gut epithelial-derived CXCL9 maintains gut homeostasis through preventing overgrown E. coli. J. Crohns Colitis 16 (6), 963–977. doi:10.1093/ecco-jcc/jjab234
Zahn, A., Giese, T., Karner, M., Braun, A., Hinz, U., Stremmel, W., et al. (2009). Transcript levels of different cytokines and chemokines correlate with clinical and endoscopic activity in ulcerative colitis. BMC Gastroenterol. 9, 13. doi:10.1186/1471-230X-9-13
Zhang, Y. Z., and Li, Y. Y. (2014). Inflammatory bowel disease: pathogenesis. World J. Gastroenterol. 20 (1), 91–99. doi:10.3748/wjg.v20.i1.91
Zhao, Q., Kim, T., Pang, J., Sun, W., Yang, X., Wang, J., et al. (2017). A novel function of CXCL10 in mediating monocyte production of proinflammatory cytokines. J. Leukoc. Biol. 102 (5), 1271–1280. doi:10.1189/jlb.5A0717-302
Keywords: IBD-inflammatory bowel disease, cytokines, interferon, secretion, angiocrine, paracrine, barrier, endothelial
Citation: Naschberger E, Flierl C, Huang J, Erkert L, Gamez-Belmonte R, Gonzalez-Acera M, Bober M, Mehnert M, Becker C, Schellerer VS, Britzen-Laurent N and Stürzl M (2023) Analysis of the interferon-γ-induced secretome of intestinal endothelial cells: putative impact on epithelial barrier dysfunction in IBD. Front. Cell Dev. Biol. 11:1213383. doi: 10.3389/fcell.2023.1213383
Received: 22 May 2023; Accepted: 31 July 2023;
Published: 14 August 2023.
Edited by:
Carlos Pardo-Pastor, King’s College London, United KingdomReviewed by:
Triet M. Bui, Dana–Farber Cancer Institute, United StatesCopyright © 2023 Naschberger, Flierl, Huang, Erkert, Gamez-Belmonte, Gonzalez-Acera, Bober, Mehnert, Becker, Schellerer, Britzen-Laurent and Stürzl. This is an open-access article distributed under the terms of the Creative Commons Attribution License (CC BY). The use, distribution or reproduction in other forums is permitted, provided the original author(s) and the copyright owner(s) are credited and that the original publication in this journal is cited, in accordance with accepted academic practice. No use, distribution or reproduction is permitted which does not comply with these terms.
*Correspondence: Michael Stürzl, bWljaGFlbC5zdHVlcnpsQHVrLWVybGFuZ2VuLmRl
†These authors have contributed equally to this work