- 1Department of Gastrointestinal Surgery, The First Affiliated Hospital, College of Clinical Medicine, Henan University of Science and Technology, Luoyang, Henan, China
- 2Department of Child Health Care, The Third Affiliated Hospital of Zhengzhou University, Zhengzhou, Henan, China
Colorectal cancer (CRC) remains one of the most prevalent and fatal malignancies worldwide, consistently ranking among the top three in terms of incidence and mortality. Despite notable advancements in early detection and therapeutic interventions, survival outcomes for advanced-stage CRC are still dismal, largely due to issues such as drug resistance and metastasis. Recent research has increasingly implicated the JAK-STAT signaling pathway as a pivotal contributor to CRC pathogenesis. This evolutionarily conserved pathway plays a key role in transmitting extracellular signals to the nucleus, thereby modulating gene expression involved in numerous fundamental biological processes. In CRC, dysregulation of the JAK-STAT pathway is frequently observed and is strongly associated with tumor progression, including processes such as cellular proliferation, apoptosis, metastasis, immune evasion, and the sustenance of cancer stem cells. Given its integral role in CRC advancement, the JAK-STAT pathway has gained recognition as a viable therapeutic target. Extensive evidence from preclinical and clinical models supports the efficacy and safety of targeting components of the JAK-STAT pathway, presenting new therapeutic possibilities for patients with CRC, particularly in addressing drug resistance and enhancing treatment outcomes. This review offers a detailed exploration of the JAK-STAT pathway, focusing on its regulatory mechanisms in CRC-related malignancies. Moreover, it examines the association between JAK-STAT protein expression, clinical features, prognosis, and its therapeutic potential in CRC management.
1 Introduction
Colorectal cancer (CRC) remains a highly prevalent and lethal malignancy globally (Midgley and Kerr, 1999; Siegel et al., 2020; Li N. et al., 2021; Ranasinghe et al., 2022). According to the latest Global Cancer Statistics 2022, CRC ranks third in incidence and second in cancer-related mortality in the United States (Rim et al., 2009; Biller and Schrag, 2021; Bray et al., 2024). In 2024, approximately 152,810 new cases were projected to be diagnosed, with an estimated 53,010 deaths resulting from the disease. Current CRC treatment approaches involve a combination of surgery, chemotherapy, radiotherapy, and targeted therapies, tailored to the tumor’s stage and molecular profile (Mármol et al., 2017; Wong and Yu, 2019; Liu Y. et al., 2023). For advanced and metastatic CRC, systemic chemotherapy regimens such as FOLFOX (5-fluorouracil, leucovorin, and oxaliplatin) or FOLFIRI (5-fluorouracil, leucovorin, and irinotecan) are commonly employed (Saltz, 2013; Tabernero et al., 2014; Venook et al., 2017; Stintzing et al., 2019; Guo et al., 2021). Prognosis is heavily influenced by the stage at diagnosis, with a significant decline in survival rates for advanced CRC, where the 5-year survival rate falls below 15% (Pickhardt et al., 2011; Nguyen and Weinberg, 2016; Rumpold et al., 2020; Shin et al., 2023). Despite advancements in screening and therapeutic strategies, the emergence of drug resistance and the limited availability of effective treatment options remain substantial challenges, particularly for patients with advanced disease (Andrei et al., 2022; Xing et al., 2023; Cañellas-Socias et al., 2024). Overcoming therapeutic resistance and developing novel treatment modalities for advanced and metastatic CRC are crucial for improving patient outcomes (Van Cutsem et al., 2013; Fessler and Medema, 2016; Zhang et al., 2023).
The Janus kinase (JAK)-signal transducer and activator of transcription (STAT) pathway plays a critical role in transmitting signals from extracellular cytokines and growth factors to the nucleus, modulating gene expression that governs cell proliferation, angiogenesis, immune responses, and survival (Hu et al., 2021; Chan et al., 2022; Hu et al., 2023; Xue et al., 2023). Beyond its role in maintaining normal physiological processes, the JAK-STAT pathway contributes to the pathogenesis of various disorders, including autoimmune diseases, hematologic malignancies, and solid tumors (O'Shea et al., 2015; Trivedi and Starz-Gaiano, 2018; Gutiérrez-Hoya and Soto-Cruz, 2020; Erdogan et al., 2022). In CRC, dysregulation of the JAK-STAT pathway has been widely reported, with abnormal signaling profoundly affecting critical biological processes, such as cellular proliferation, survival, migration, invasion, cancer stem cell maintenance, and immune evasion (Liang et al., 2019; Zheng et al., 2022; Ghasemian et al., 2023). Given its central role in CRC progression, targeting the JAK-STAT pathway has emerged as a promising therapeutic approach (Silva et al., 2021; Yang et al., 2022; Lu et al., 2023). Numerous studies have demonstrated the effectiveness and safety of JAK-STAT inhibitors in CRC models, showing reduced tumor growth, increased therapeutic sensitivity, and enhanced immune responses (Chalikonda et al., 2021; Bajpai et al., 2024; Wu et al., 2024).
This review offers an in-depth examination of the JAK-STAT pathway, with a focus on its regulatory mechanisms in CRC-related malignancies. Additionally, we discuss the clinical relevance of JAK-STAT protein expression, its prognostic value, and the potential of targeting this pathway as a therapeutic strategy in CRC management.
2 Overview of the JAK-STAT pathway
2.1 The JAK-STAT pathway composition
The JAK-STAT signaling pathway represents a highly efficient mechanism for transmitting extracellular signals directly to the nucleus (Vainchenker and Constantinescu, 2013; Xin et al., 2020; Park et al., 2023; Liang et al., 2024). The core components of this pathway include ligands, receptors, JAKs, STAT proteins (Signal Transducers and Activators of Transcription), and regulatory proteins such as Suppressors of Cytokine Signaling (SOCS).
In humans, JAKs belong to the non-receptor tyrosine kinase family and are composed of four members: JAK1, JAK2, JAK3, and TYK2 (Banerjee et al., 2017; Damerau et al., 2020; Pang et al., 2023; Liang et al., 2024). Each of these JAKs associates selectively with specific cytokine and hormone receptors, contributing to their distinct biological functions. JAK3, in particular, is predominantly expressed in the bone marrow, lymphatic system, endothelial cells, and vascular smooth muscle cells, whereas the other JAK members are typically present in most tissues. Structurally, JAK proteins are organized into several domains: the N-terminal FERM domain, which mediates interactions with receptors; the Src homology 2 (SH2)-like domain, which binds phosphorylated tyrosine residues; the pseudokinase domain, which exerts regulatory control; and the C-terminal tyrosine kinase domain, responsible for phosphorylating downstream targets.
The STAT family includes seven proteins: STAT1, STAT2, STAT3, STAT4, STAT5a, STAT5b, and STAT6 (Li, 2008; Yu et al., 2014; Verhoeven et al., 2020). In their inactive state, STAT proteins are localized in the cytoplasm, where they consist of six conserved domains that serve diverse functional roles. These domains include the N-terminal domain, which facilitates STAT dimerization; the coiled-coil domain, which regulates nuclear import and export; the DNA-binding domain, which interacts with gene promoters; the linker domain; the SH2 domain, which recognizes phosphorylated cytokine receptors; and the C-terminal transcriptional activation domain (TAD), which activates the transcription of target genes.
2.2 The JAK-STAT pathway activation and regulation
The JAK-STAT pathway orchestrates several critical biological functions, including cellular processes, immune regulation, hematopoiesis, angiogenesis, and oncogenesis (Hou et al., 2002; Amoyel and Bach, 2012). This pathway is activated when cytokines or growth factors bind to their respective receptors on the cell surface, inducing conformational changes that lead to the activation of JAK proteins (Müller et al., 2008; La Fortezza et al., 2016). Once activated, JAKs phosphorylate each other and tyrosine residues on the receptor’s cytoplasmic domain, creating docking sites for STAT proteins. The SH2 domains of STATs bind to these phosphorylated residues, positioning them for subsequent phosphorylation by JAKs. Phosphorylated STATs then dimerize and translocate to the nucleus, where they regulate the expression of target genes involved in diverse cellular processes. Cytokine or growth factor binding serves as the primary trigger for the JAK-STAT signaling cascade, ultimately resulting in changes to gene expression (Figure 1).
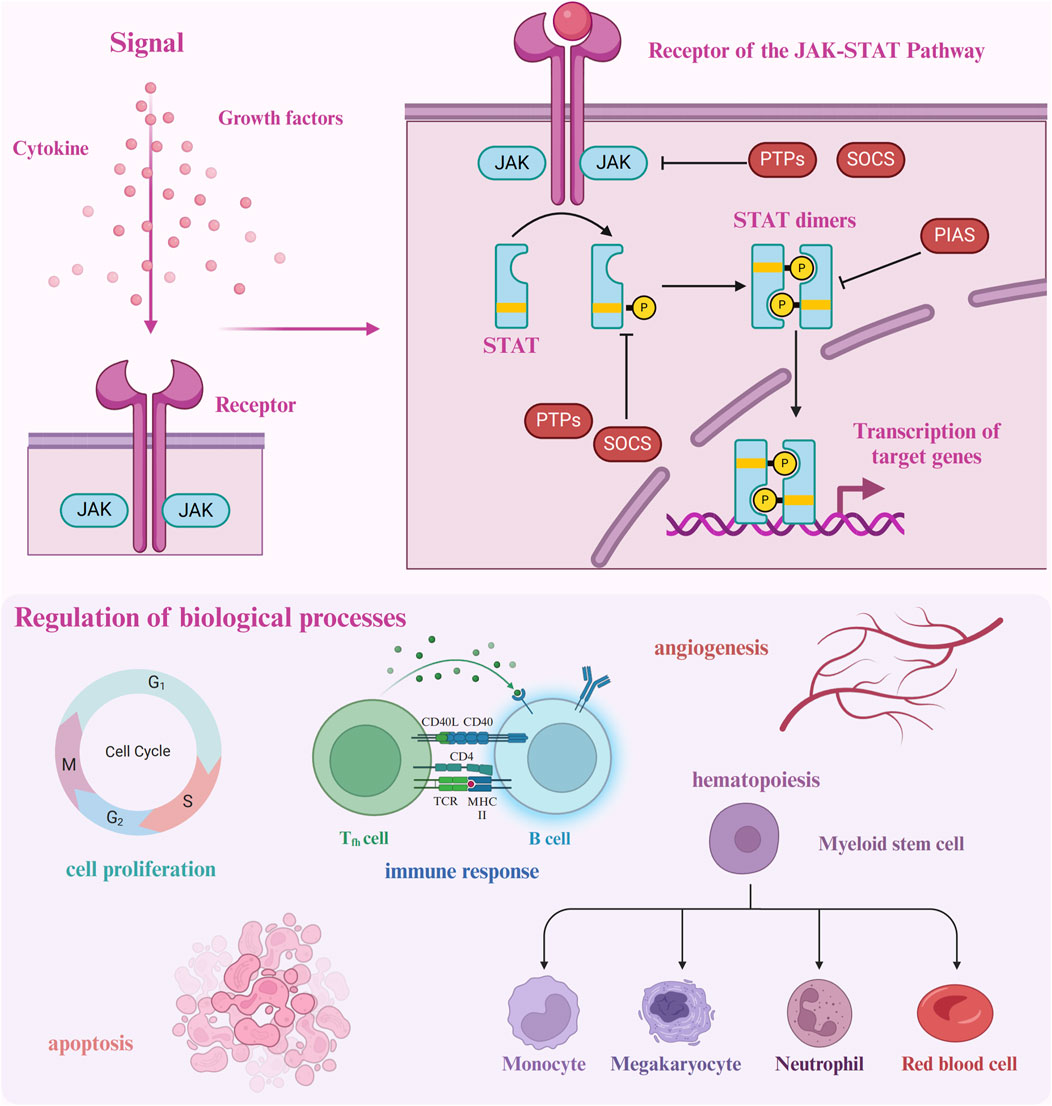
Figure 1. Upon ligand binding to its receptor, the JAK-STAT pathway is initiated by receptor dimerization, which facilitates the proximity of JAK proteins, enabling mutual phosphorylation as well as phosphorylation of the receptor. Phosphorylated tyrosine residues on the receptor serve as docking sites for STAT proteins, which are subsequently phosphorylated by JAKs. The activated STAT proteins dimerize and translocate to the nucleus, where they bind to DNA and regulate gene transcription. The pathway is tightly controlled by several regulatory mechanisms: SOCS proteins inhibit JAK activity, while PIAS and PTPs modulate and dephosphorylate STATs, respectively, ensuring precise signal termination.
The activation of the JAK-STAT pathway is tightly regulated through several negative feedback mechanisms to prevent excessive or prolonged signaling (Liu J. et al., 2023; López-Mejía et al., 2023). A primary regulatory mechanism involves the Suppressor of Cytokine Signaling (SOCS) proteins, which include SOCS1-7 and the cytokine-inducible SH2-containing protein (CIS) (Krebs and Hilton, 2001; Yoshimura et al., 2007; Kopalli et al., 2022; Pandey et al., 2023). Once JAK-STAT signaling is initiated, SOCS proteins bind to phosphorylated JAKs or receptors, targeting them for proteasomal degradation and thereby halting further activation of the pathway. Other key regulatory proteins include the protein inhibitors of activated STAT (PIAS) and protein tyrosine phosphatases (PTPs) (Tanuma et al., 2001; Xu and Qu, 2008; Luo et al., 2022; Tobin and Cooper, 2024). PIAS proteins inhibit STATs by either preventing their binding to DNA or promoting their sumoylation, which deactivates their transcriptional activity. PTPs, such as SHP-1 and SHP-2, dephosphorylate JAKs and STATs, reversing their activation and effectively terminating the signaling process (Niu et al., 2018; Taheri et al., 2018; Ghafouri-Fard et al., 2021).
Under normal physiological conditions, the JAK-STAT pathway is indispensable for transmitting signals from extracellular cytokines and growth factors to regulate essential biological functions. However, persistent activation of this pathway has been implicated in the pathogenesis of various diseases, particularly cancer and chronic inflammatory conditions (Banerjee et al., 2017; Xin et al., 2020; Sun et al., 2023).
3 Involvement of the JAK-STAT pathway in CRC
Abnormalities in the JAK-STAT pathway have been documented across various malignancies, including breast, colorectal, esophageal, liver, lung, ovarian, pancreatic, and prostate cancers (Xue et al., 2021; Fernández et al., 2023; Mahjoor et al., 2023; Jia et al., 2024). In recent years, growing evidence has underscored the pivotal role this pathway plays in CRC development. Dysregulated JAK-STAT signaling contributes to CRC progression by modulating essential processes such as cell proliferation, apoptosis, migration, invasion, stem cell phenotype maintenance, and immune evasion (Figure 2). Given the extensive involvement of this pathway in malignant behaviors, this section will focus on elucidating the molecular mechanisms and biological functions of the JAK-STAT pathway in key tumorigenic processes in CRC (Table 1).
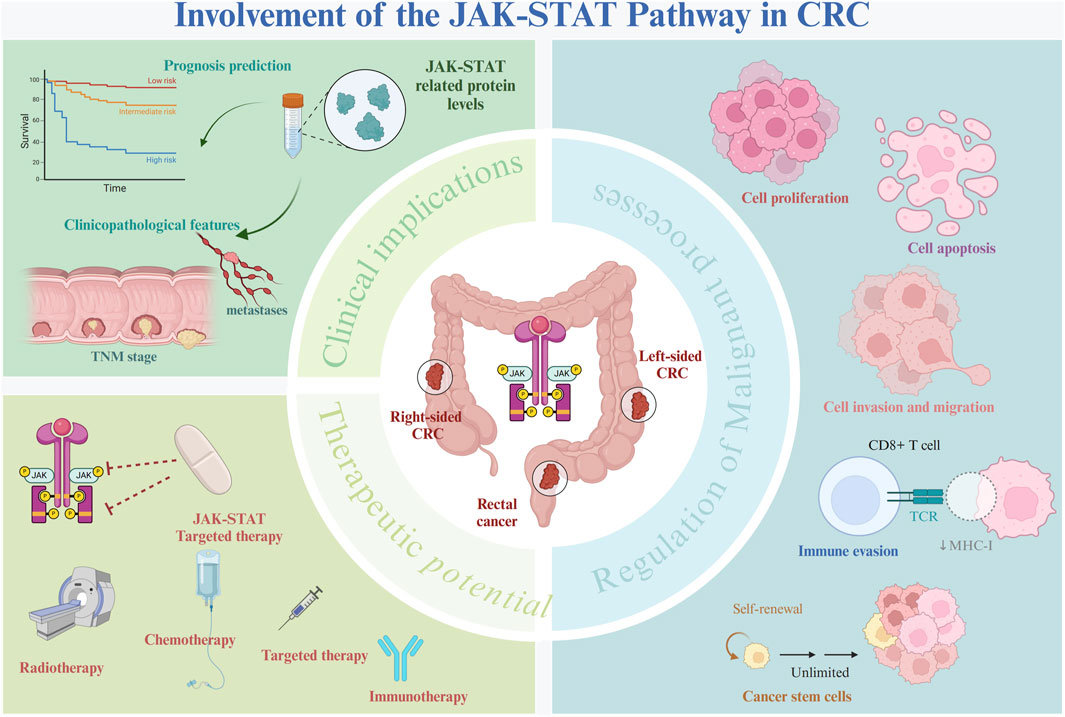
Figure 2. Role of the JAK-STAT pathway in CRC. The JAK-STAT pathway plays a pivotal role in the pathogenesis of CRC. Aberrant activation of the JAK-STAT pathway interacts with several oncogenic molecules and pathways, driving several key biological processes, including cell proliferation, migration, invasion, immune evasion, and the maintenance of cancer stem cell phenotypes. Dysregulation of the JAK-STAT pathway has been strongly linked to patient prognosis and various adverse clinical characteristics, making it a significant biomarker of CRC progression. Targeting the JAK-STAT pathway represents a promising therapeutic strategy that could potentially improve CRC treatment outcomes.
3.1 Cell proliferation, apoptosis, migration, and invasion
In CRC, upregulated circSPARC functions as a miR-485-3p sponge, which leads to increased JAK2 expression and the subsequent phosphorylation of STAT3. Moreover, circSPARC binds to FUS, promoting the nuclear translocation of phosphorylated STAT3 (p-STAT3). Activation of the JAK2-STAT3 signaling pathway enhances the viability, clonogenic potential, invasion, and proliferation of CRC cells, including HCT116 and DLD1, thus accelerating CRC progression (Wang et al., 2021). The IκB kinase (IKK) complex has also been frequently implicated in CRC initiation and advancement (Liu et al., 2020; Patel et al., 2022; Yu et al., 2022). Recent research has identified a functional interaction between IKK-α, BET family protein BRD4, and the JAK-STAT pathway. IKK-α phosphorylates BRD4 at serine 1,117, strengthening its interaction with STAT3 and activating the DNA damage response kinases ATM and Chk1 (Pecharromán et al., 2023). This process is mediated by NF-κB-driven induction of leukemia inhibitory factor (LIF), leading to enhanced STAT3 activation. As a result, BRD4-STAT3 complexes form, triggering the JAK-STAT3 pathway, which inhibits apoptosis and promotes DNA damage repair in human CRC cells (Pecharromán et al., 2023).
Additionally, increasing evidence has demonstrated that Vitamin D confers a protective effect against CRC, acting through a vitamin D receptor (VDR)-dependent mechanism (Pálmer et al., 2003; Fernandez-Garcia et al., 2005; Chan and Giovannucci, 2010).
Notably, reduced vitamin D receptor (VDR) levels, in conjunction with increased bacterial colonization and elevated JAK2 and STAT3 expression, have been identified in both human CRC samples and azoxymethane/dextran sulfate sodium (AOM/DSS)-induced CRC models (Zhang et al., 2020). VDR deficiency in the gut contributes to the hyperactivation of the JAK2-STAT3 signaling pathway, leading to gut microbiota dysbiosis and heightened susceptibility to carcinogenesis (Zhang et al., 2020). Furthermore, nuclear factor κB (NF-κB)/p65 activation perpetuates the expression of the STAT3 cofactor progranulin in human CRC cells, which in turn augments STAT3 activation, promoting CRC cell proliferation and survival (Laudisi et al., 2019). The TP53 gene, which encodes 12 isoforms, exhibits notable dysregulation of the ∆133p53 isoform in human tumors, contributing to tumorigenesis (Fujita et al., 2009; Bernard et al., 2013; Gadea et al., 2016; Joruiz et al., 2020). The oncogenic function of ∆133p53 is reliant on Interleukin-6 (IL-6), which constitutively activates the JAK-STAT3 pathway, subsequently triggering GTPase RhoA and its associated kinase ROCK. This cascade activates NF-κB, promoting the secretion of pro-inflammatory chemokines and fostering an invasive CRC phenotype (Campbell et al., 2018). Triptolide, a diterpenoid triepoxide derived from traditional Chinese medicinal herbs, has demonstrated efficacy in disrupting the IL6R-JAK1-STAT3 pathway by inactivating small GTPase Rac1, thereby reducing CRC incidence, improving survival rates in colitis-associated CRC mouse models, and inhibiting proliferation in CRC cell lines such as SW480 and Caco-2 (Wang et al., 2009).
Aging-associated gene 8 protein (AAG8), a chaperone protein involved in endoplasmic reticulum (ER)-associated degradation, has emerged as an oncogenic factor in CRC. AAG8 promotes cancer cell proliferation by activating STAT3 independently of the IL6-JAK pathway (Sun et al., 2014). Additionally, mutations that inactivate the adenomatous polyposis coli (APC) gene, a key negative regulator of Wnt signaling, are major drivers of both sporadic and hereditary CRC (Ireland et al., 2004; Andreu et al., 2005; Hsu et al., 2007). The loss of APC triggers MYC upregulation and JAK-STAT3 pathway activation, fueling excessive intestinal stem cell (ISC) proliferation in the Drosophila midgut in response to heightened Wnt signaling (Cordero et al., 2012). Epidermal growth factor receptor (EGFR) signaling further establishes a positive feedback loop, linking Wnt/MYC activation with JAK-STAT3 pathway signaling in response to APC1 loss, amplifying this proliferative response (Cordero et al., 2012). The interplay between tumor cells and cancer-associated fibroblasts (CAFs) is also pivotal in driving cancer progression and metastasis (Hu et al., 2019; Gong et al., 2020; Kobayashi et al., 2022; Koncina et al., 2023). IL-1β and TGF-β1 are key factors that recruit fibroblasts and transform them into CAFs in CRC. Once activated, CAFs secrete pro-inflammatory mediators that activate the JAK-STAT3 and PI3KCA-AKT pathways, accelerating cancer progression (Guillén Díaz-Maroto et al., 2019). Furthermore, STAT5 and STAT6 are highly expressed in various CRC cell lines and tissues. The long non-coding RNA (lncRNA) RP11-468E2.5 interacts with STAT5 and STAT6, and its upregulation reduces phosphorylation levels of these proteins, leading to inhibited proliferation and the induction of G1/G0 phase arrest and apoptosis in CRC cells (Jiang et al., 2019). In contrast, unphosphorylated STAT5A functions as a tumor suppressor by binding to heterochromatin protein 1α (HP1α), stabilizing heterochromatin, and repressing oncogene expression, thereby inhibiting tumor growth in mouse CRC xenograft models (Figure 3) (Hu et al., 2013).
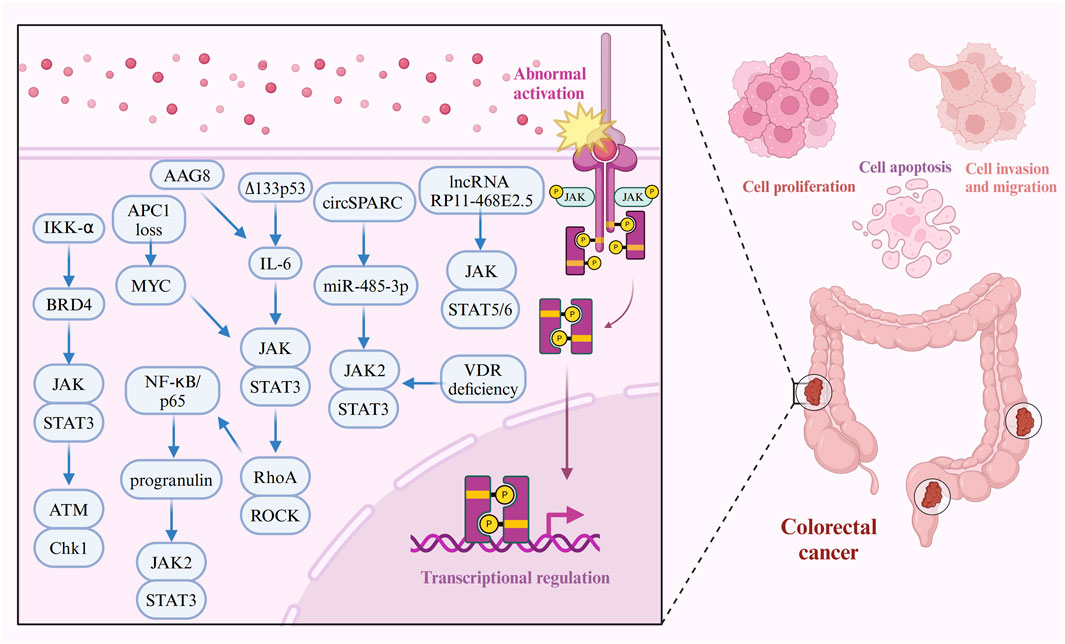
Figure 3. Molecular mechanisms of the JAK-STAT pathway in CRC cell proliferation, apoptosis, migration, and invasion. The JAK-STAT signaling pathway plays a pivotal role in promoting CRC cell proliferation, apoptosis resistance, migration, and invasion through interactions with various oncogenic molecules. For instance, circSPARC functions as a miR-485-3p sponge, leading to the upregulation of JAK2 and subsequent phosphorylation of STAT3, which enhances cell viability and invasiveness. BRD4, via IKK-α phosphorylation, interacts with STAT3 to facilitate DNA damage repair and tumor progression. Inflammatory cytokines such as IL-6 and NF-κB further activate STAT3, driving cancer cell survival and metastasis. Additionally, APC mutations fuel JAK-STAT signaling through the Wnt/MYC pathway, while VDR deficiency and gut microbiota dysbiosis amplify JAK2-STAT3 hyperactivation, further contributing to CRC development. Furthermore, molecules like progranulin, ∆133p53, and RhoA reinforce STAT3 signaling, driving CRC progression and promoting resistance to apoptosis.
3.2 Immune evasion
In CRC, the tumor microenvironment (TME) plays a pivotal role in promoting immune evasion (Chandra et al., 2021; Che et al., 2021; Kasprzak, 2021). One prominent factor is membrane-spanning four domains subfamily A member 4A (MS4A4A), which is significantly overexpressed in tumor-associated macrophages (TAMs). This overexpression activates JAK-STAT6 signaling, promoting M2 macrophage polarization and CD8+ T-cell dysfunction, thereby fostering an immunosuppressive TME that enables the tumor to escape immune surveillance (Li Y. et al., 2023). Metabolic alterations within the TME further exacerbate immune evasion. For example, lactate accumulation in the CRC TME has been shown to upregulate methyltransferase-like 3 (METTL3) in tumor-infiltrating myeloid cells (TIMs) (Li et al., 2024). In conjunction with YTH N6-methyladenosine RNA Binding Protein 1 (YTHDF1), METTL3 mediates m6A modification on JAK1 mRNA, enhancing JAK1 and STAT3 expression and promoting tumor immune escape (Xiong et al., 2022). Another mechanism involves cap methyltransferase 1 (CMTR1), a recently identified oncogene in CRC. CMTR1 binds strongly to the transcription start site (TSS) of STAT3, driving its expression and activation. Activated STAT3, in turn, promotes CMTR1-driven cell growth and immune evasion by suppressing the expression of chemokines and pro-inflammatory factors, thus further advancing tumor progression (You et al., 2023). Additionally, the transcription coactivator steroid receptor coactivator-1 (SRC-1) plays a pivotal role in regulating immune responses in CRC. SRC-1 suppresses SOCS1 expression, leading to the activation of JAK1-STAT3 signaling, which upregulates PD-L1 expression and reduces CD8+ T-cell infiltration, thereby diminishing antitumor immunity (Hong et al., 2024). Notably, CRC tumors with high microsatellite instability (MSI-H) exhibit increased responsiveness to PD-1 blockade therapy (Kim et al., 2016; Gong et al., 2017; Lee et al., 2017; Korehisa et al., 2018). Research suggests that low doses of interferon-gamma (IFN-γ) significantly elevate PD-L1 expression in MSI-H cell lines, such as HCT116, likely via the JAK-STAT pathway. Specifically, PD-L1 expression in these cells is primarily driven by the JAK3-STAT5A/5B pathway. Overexpression of PD-L1 not only enhances apoptosis resistance in CRC CT26 cells but also limits CD8+ T-cell infiltration, contributing to immune evasion and facilitating tumor growth (Yuan et al., 2019). Furthermore, interleukin-11 (IL-11) has emerged as a key player in the immunomodulatory landscape of CRC. By activating the JAK-STAT3 pathway, IL-11 inhibits the production of critical pro-inflammatory cytokines such as IFN-γ and TNF-α by tumor-infiltrating CD4+ T cells. This inhibition supports the creation of an immunosuppressive environment, which aids in CRC cell proliferation and survival (Huynh et al., 2021).
3.3 Stem cell characteristics
Cancer stem cells (CSCs) play a pivotal role in tumor growth, metastasis, drug resistance, and recurrence (Frank et al., 2021; Zheng et al., 2022; Zhu et al., 2022; Zhao et al., 2024). Napabucasin (BBI608), an orally administered STAT3 inhibitor, has undergone clinical evaluation for treating various cancers (Hubbard and Grothey, 2017; Froeling et al., 2019; Bitsch et al., 2022; Bekaii-Saab et al., 2023; Jing et al., 2023). In vitro studies have revealed that Napabucasin exerts potent tumor-suppressive effects by specifically targeting CSCs in CRC. It achieves this by inhibiting STAT3 and STAT1 signaling, leading to a reduction in CD44 expression, a key marker linked to CSCs (Jing et al., 2023). Additionally, laminin 521 (LN521) has been shown to promote the self-renewal and invasion of CRC cells by enhancing STAT3 phosphorylation, an effect that can be counteracted by Napabucasin (Qin et al., 2020). In radioresistant CRC tissues, the JAK/STAT signaling pathway is hyperactivated, which is associated with both local and distant metastasis. Research indicates that CRC stem cell subpopulations exposed to radiotherapy (RT) tend to overexpress JAK2, resulting in increased STAT3 phosphorylation. This heightened activation drives the transcription of cyclin D2 (CCND2), promoting CSC self-renewal and contributing to the persistence of radioresistance, further complicating treatment outcomes (Park et al., 2019).
4 Clinical implications and treatment potentials of the JAK-STAT pathway in CRC
4.1 Clinical implications of the JAK-STAT pathway in CRC
Emerging research increasingly links abnormal JAK-STAT signaling to CRC prognosis and clinicopathological characteristics, including tumor invasion depth, size, and metastasis (Table 2) (Amoyel et al., 2014; Jia et al., 2024). Multiple studies have demonstrated a significant association between elevated JAK-STAT3 expression in various CRC cell lines and poorer survival in patients with high stromal invasion (TSPhigh). This correlation has been observed across the TransSCOT study and two large retrospective CRC cohorts (Glasgow combined array and Glasgow Royal Infirmary array) (Pennel et al., 2024). Additionally, population-based case-control studies have highlighted the importance of the JAK-STAT-SOCS signaling pathway in CRC development, where genetic variants in this pathway are linked to an increased risk of both colon and rectal cancers. Furthermore, several single nucleotide polymorphisms (SNPs) in JAK-STAT pathway genes have been associated with survival outcomes in patients with CRC, underscoring their potential as prognostic markers (Slattery et al., 2013). Whole exome sequencing of tissues from metachronous CRC liver metastases (mCLM) and matched non-malignant liver samples has revealed that genetic alterations within the JAK-STAT pathway may serve as indicators of longer overall survival (OS) in patients with mCLM (Heczko et al., 2023). Additionally, phosphorylated STAT5 (p-STAT5) and phosphorylated STAT6 (p-STAT6) are expressed at significantly higher levels in CRC tissues compared to adjacent normal tissues. Higher expression levels of these phosphorylated proteins correlate with deeper tumor invasion, suggesting a more aggressive tumor phenotype (Jiang et al., 2019). Recent findings have also identified lymphocyte antigen 6 complex locus G6D (LY6G6D) as a carcinogenic antigen in microsatellite stable (MSS) CRC. In an analysis of CRC subtypes stratified by mismatch repair (MMR) status (TCGA, n = 276), STAT5 and LY6G6D were more highly expressed in MSS tumors compared to microsatellite instability (MSI) tumors. Metastatic cell lines, such as SW620, characterized by elevated LY6G6D expression, also demonstrated the highest levels of p-STAT5. Importantly, positive staining of p-STAT5 and LY6G6D in normal mucosa and CRC samples was directly linked to shorter survival times for patients with CRC (Giordano et al., 2019). Further analysis of The Cancer Genome Atlas (TCGA) data revealed a strong positive correlation between mRNA expression levels of STAT1, STAT2, and STAT3 with CD44, suggesting that JAK-STAT signaling is closely associated with the maintenance of stemness in CRC stem cells (Jing et al., 2023). Additionally, both TCGA data and immunohistochemical (IHC) analysis of 32 CRC primary tumors, metastases, and normal intestinal mucosa from patients with lung metastases have suggested a key role for SOCS3 in CRC progression. SOCS3 expression is closely linked to macrophage infiltration and CRC lung metastasis, with patients presenting lymph node and distant metastases at diagnosis often showing elevated SOCS3 expression in lung metastasis sites (Li X. et al., 2023). Lung metastases also exhibit higher levels of both CD163 and SOCS3 compared to primary tumors, indicating SOCS3’s potential role in promoting CRC metastasis and influencing patient prognosis (Li X. et al., 2023).
4.2 Targeting the JAK-STAT pathway as a therapeutic strategy in CRC
A range of agents targeting the dysregulated JAK-STAT pathway has gained notable attention as therapeutic options for precision treatment in CRC. Inhibiting JAK-STAT3 signaling has emerged as a promising strategy for patients with CRC. JAK1/2 inhibitors have demonstrated significant efficacy in reducing cell viability and tumor growth across multiple CRC models, including cell lines, mouse models, and patient-derived organoids (PDOs) (Seyfinejad and Jouyban, 2022; Pennel et al., 2024). However, despite these encouraging preclinical outcomes, a 2018 multicenter, randomized, double-blind phase II clinical trial involving 396 patients revealed that combining the potent JAK1/2 inhibitor Ruxolitinib with regorafenib did not improve OS or progression-free survival (PFS) in patients with relapsed or refractory metastatic CRC when compared to regorafenib plus placebo (Fogelman et al., 2018). Additionally, NSC13626, discovered via structure-based virtual screening of the National Cancer Institute (NCI) database, has shown potential as a JAK2 inhibitor. Cellular studies indicate that NSC13626 targets JAK2, leading to reduced STAT3 phosphorylation, arrest of the CRC cell cycle, and suppression of cell growth (Lin et al., 2018). Tumor-derived microparticles (TMPs) from apoptotic tumor cells have also been investigated as potential drug delivery vehicles (Fremder et al., 2014; Phuengkham et al., 2019; Zhao et al., 2019; Akhtar et al., 2023; Chen Y. et al., 2024).
Growing preclinical and clinical evidence suggests that Napabucasin (BBI608), a STAT3 inhibitor, shows considerable promise for CRC treatment (Shao et al., 2023). In a Phase III clinical trial (NCT01830621) across 68 centers with 282 patients with advanced CRC, those with pSTAT3-positive tumors demonstrated a notable survival advantage when treated with Napabucasin and best supportive care compared to the placebo group (median OS 5.1 months vs. 3.0 months) (Jonker et al., 2018). Additionally, a Phase I study in Japan involving four patients with advanced CRC treated with Napabucasin in combination with leucovorin, 5-fluorouracil, irinotecan (FOLFIRI), and bevacizumab exhibited a manageable and tolerable safety profile (Taniguchi et al., 2021). However, in the Phase III CanStem303C trial (NCT02753127) with 1,255 patients with metastatic CRC, Napabucasin combined with FOLFIRI, with or without bevacizumab, failed to improve OS in the general population, though it maintained an acceptable safety profile (Shah et al., 2023). Further supporting Napabucasin’s potential, a recent study introduced the nanoplatform N3-TMPs@NAP, which integrates diagnostic and therapeutic capabilities. PET/CT imaging studies showed that N3-TMPs@NAP exhibited strong antitumor effects by inhibiting STAT1 and STAT3 activation, suppressing CRC CSCs, stimulating T-cell-mediated immune responses, and preventing liver metastasis (Jing et al., 2023). Beyond Napabucasin, other STAT3 inhibitors have shown potential in CRC treatment. The naphthoquinothiazole derivative SZ6 has emerged as a potent STAT3 inhibitor, effectively blocking STAT3 phosphorylation and binding directly to STAT3 (Fan et al., 2024). Moreover, two novel SHP-1 agonists, SC-43 and SC-78, have been identified for their ability to inactivate STAT3 and demonstrate significant antitumor activity by targeting CSC properties (Chung et al., 2018). These SHP-1 agonists have proven more effective than regorafenib in reducing active STAT3 levels. When combined with first-line metastatic CRC therapies, such as oxaliplatin or irinotecan, SHP-1 agonists synergistically diminish CSC subpopulations, further enhancing their therapeutic efficacy.
4.3 Combination of JAK-STAT-targeting therapies with existing therapies
Chemotherapy remains a cornerstone in CRC treatment, although its efficacy is often hampered by the emergence of drug resistance and severe side effects. Genistein has gained recognition as a potent chemopreventive agent in CRC (Chen et al., 2020; Rendón et al., 2022; Liao et al., 2023), with molecular docking studies showing its effective interaction with STAT proteins, resulting in inhibited cell proliferation and reduced STAT3 expression in CRC cell lines, including HCT116 and HT-29 (Dariya et al., 2021). Furthermore, combining chemotherapy with inhibitors of IKK-α, STAT3, and BRD4 has shown promise in overcoming chemoresistance in CRC. Specifically, inhibiting STAT3 with Ruxolitinib or an anti-LIF antibody, along with the BRD4 inhibitor JQ1 and the BRAF inhibitor AZ628, has been shown to prevent IKK-α (p45) activation, significantly inducing cell death in CRC cells and patient-derived organoids treated with 5-fluorouracil (5-FU) and irinotecan (Pecharromán et al., 2023). Additionally, the combination of BRAF and JAK-STAT inhibitors has enhanced the long-term efficacy of chemotherapy in CRC xenografts, even in tumors with various mutational profiles, including those resistant to first-line treatments (Pecharromán et al., 2023). Furthermore, progranulin knockdown to downregulate STAT3 sensitizes CRC cells to chemotherapeutic agents like 5-FU, oxaliplatin, and CPT-11, significantly increasing CRC cell death (Laudisi et al., 2019).
In parallel, targeted therapies, such as mitogen-activated protein kinase kinase/extracellular regulated protein kinase (MEK/ERK) inhibitors, have garnered attention for their potential to enhance antitumor immunity through complex immunomodulatory effects on tumor cells and tumor-infiltrating lymphocytes (TILs) (Li H. et al., 2021; Nalli et al., 2021; Tian et al., 2023). Trametinib, a specific inhibitor of MEK1 and MEK2, targets key enzymes in the MAPK/ERK pathway, which are essential for cell division and survival. In CRC tumors expressing LY6G6D and CD15, particularly within the MSS CRC subgroup, combining trametinib with the JAK1/2 inhibitor momelotinib has been found to significantly enhance growth inhibition and apoptosis (Giordano et al., 2019). In mouse models with CT26 MEK1 knockout tumors, marked upregulation of the JAK1-STAT1 pathway, increased MHCI expression, and a higher proportion of activated CD8+ T cells led to immune-mediated suppression of CRC tumor growth (Dennison et al., 2021). The JAK-STAT pathway has been identified as a key mediator of immunomodulatory functions in these tumors. However, while cobimetinib, another MEK inhibitor, has been shown to enhance antitumor immunity in tumor cells, it simultaneously impairs T-cell activation, a limitation that can be reversed by treatment with the T-cell agonist anti-4-1BB. The combination of cobimetinib, anti-PD-1, and anti-4-1BB has demonstrated synergistic antitumor activity, significantly improving survival rates and offering a promising therapeutic strategy for CRC (Dennison et al., 2021).
In the past decade, immunotherapy has revolutionized the treatment landscape for a subset of patients with CRC, becoming an increasingly vital option in CRC management (Chen and Wang, 2024). The STAT6 inhibitor AS1517499 has shown significant efficacy in inhibiting M2 macrophage polarization in cells overexpressing MS4A4A. Additionally, siRNA targeting the MS4A4A gene (siMS4A4A) and an anti-MS4A4A monoclonal antibody not only slow tumor growth and reshape the TME but also enhance the efficacy of anti-PD-1 therapy (Li Y. et al., 2023). In C57BL/6J mice implanted with MC38 cells, the combination of CMTR1 knockdown with anti-PD-1 treatment has been shown to significantly increase CD8+ T-cell infiltration into the TME and suppress tumor growth, along with increased expression of chemokines and pro-inflammatory factors (You et al., 2023). Moreover, genetic deletion or pharmacological inhibition of SRC-1 using the small-molecule inhibitor bufalin, when combined with PD-L1 blockade, effectively suppresses JAK1-STAT3 signaling, thereby boosting immune responses and producing synergistic antitumor effects (Hong et al., 2024). Currently, pembrolizumab and nivolumab are approved for treating adults with mismatch repair-deficient (dMMR) and MSI-H metastatic or progressive CRC (Riley et al., 2018; Modest et al., 2019; Payandeh et al., 2020; Kanani et al., 2021; Chen X. et al., 2024). However, responses to PD-1 inhibitors among patients with MSI-H CRC can vary (Morse et al., 2020; Bai et al., 2021; Zhang et al., 2022). Recent studies suggest that the MSI-H cell line HCT116 is highly responsive to the IFN-γ-JAK3-STAT5A/5B signaling pathway. The interaction between intrinsic PD-L1 expression and exogenous IFN-γ exposure enhances PD-1 therapy’s effectiveness in CRC by increasing CD8+ T-cell infiltration into the TME through modulation of the JAK-STAT pathway (Yuan et al., 2019).
Beyond pharmacological approaches, RT remains an effective nonsurgical option for patients with advanced CRC (Parikh et al., 2021; Hsieh et al., 2022; Shi et al., 2023). In a CRC xenograft mouse model, combining JAK2 inhibition with RT significantly reduces tumor growth. Targeting the JAK2-STAT3 pathway promotes apoptosis and reduces the clonogenic potential of RT-exposed CRC cells, such as HCT116 and LoVo, thereby improving therapeutic outcomes following radiotherapy (Park et al., 2019).
4.4 Challenges of targeting the JAK-STAT pathway in CRC
Despite extensive research into the mechanisms and therapeutic potential of targeting the JAK-STAT pathway in CRC carcinogenesis, no clinical drugs specifically aimed at this pathway have been developed for CRC treatment. A key challenge is the intricate crosstalk between the JAK-STAT pathway and other critical signaling cascades, such as PI3K-AKT and Wnt (Bose et al., 2020; Singh and Singh, 2020; Verhoeven et al., 2020). This interaction can activate compensatory mechanisms that undermine the effectiveness of JAK-STAT inhibitors, allowing tumor cells to circumvent therapeutic interventions. Furthermore, non-canonical JAK-STAT signaling represents a significant obstacle, as this alternative pathway can continue to promote CRC progression even in the presence of inhibitors designed to block canonical signaling, thus diminishing the overall treatment efficacy (Majoros et al., 2017; La Manna et al., 2021; Rah et al., 2022; Park et al., 2023). Additional challenges, such as issues related to drug bioavailability, tissue penetration, and specificity, further complicate the clinical application of JAK-STAT inhibitors (Bose et al., 2020; Favoino et al., 2021; Wang et al., 2024). Although numerous agents have shown promise in preclinical studies and animal models, their translation to clinical practice has been limited by concerns regarding definitive efficacy and the lack of sustained long-term benefits for patients (Yu et al., 2014; O’Shea et al., 2015; Trivedi and Starz-Gaiano, 2018; McLornan et al., 2021). To address these limitations, more comprehensive, large-scale clinical trials, alongside detailed animal studies, are essential to unravel the complexities of JAK-STAT signaling in CRC and to develop more effective therapeutic strategies.
5 Conclusion
The abnormal activation of the JAK-STAT pathway has been extensively documented in CRC and is closely linked to prognosis and various clinical features. A growing body of research underscores the pivotal role of JAK-STAT signaling in CRC progression, influencing critical processes such as tumor growth, metastasis, immune evasion, and the maintenance of cancer stem cell phenotypes. As the underlying mechanisms of JAK-STAT signaling in CRC are further elucidated, this pathway has emerged as a promising therapeutic target. Modulating the JAK-STAT pathway holds significant potential to enhance the efficacy of existing CRC treatments, including chemotherapy, immunotherapy, targeted therapies, and radiotherapy. However, several challenges continue to hinder the clinical translation of therapies targeting the JAK-STAT pathway in CRC. Issues such as bioavailability and pathway-specific selectivity complicate drug development. Addressing these limitations requires more rigorous research efforts, including multicenter clinical trials and comprehensive experiments, to better understand the molecular mechanisms driving JAK-STAT signaling in CRC and to evaluate the potential therapeutic benefits of targeting this pathway.
Author contributions
PL: Conceptualization, Visualization, Writing–original draft, Writing–review and editing. DH: Visualization, Writing–original draft, Writing–review and editing.
Funding
The author(s) declare that no financial support was received for the research, authorship, and/or publication of this article.
Acknowledgments
The figures were created using Biorender (https://biorender.com/).
Conflict of interest
The authors declare that the research was conducted in the absence of any commercial or financial relationships that could be construed as a potential conflict of interest.
Generative AI statement
The author(s) declare that no Generative AI was used in the creation of this manuscript.
Publisher’s note
All claims expressed in this article are solely those of the authors and do not necessarily represent those of their affiliated organizations, or those of the publisher, the editors and the reviewers. Any product that may be evaluated in this article, or claim that may be made by its manufacturer, is not guaranteed or endorsed by the publisher.
References
Akhtar, M. J., Kumar, B., Paul, J., Singh, K., Pannu, S., Pal, R., et al. (2023). An update on recently developed analytical and bio-analytical methods for some anticancer drugs. Curr. Pharm. Anal. 19 (2), 117–135. doi:10.2174/1573412919666221123110420
Amoyel, M., Anderson, A. M., and Bach, E. A. (2014). JAK/STAT pathway dysregulation in tumors: a Drosophila perspective. Semin. Cell Dev. Biol. 28, 96–103. doi:10.1016/j.semcdb.2014.03.023
Amoyel, M., and Bach, E. A. (2012). Functions of the Drosophila JAK-STAT pathway: lessons from stem cells. Jakstat 1 (3), 176–183. doi:10.4161/jkst.21621
Andrei, P., Battuello, P., Grasso, G., Rovera, E., Tesio, N., and Bardelli, A. (2022). Integrated approaches for precision oncology in colorectal cancer: the more you know, the better. Semin. Cancer Biol. 84, 199–213. doi:10.1016/j.semcancer.2021.04.007
Andreu, P., Colnot, S., Godard, C., Gad, S., Chafey, P., Niwa-Kawakita, M., et al. (2005). Crypt-restricted proliferation and commitment to the Paneth cell lineage following Apc loss in the mouse intestine. Development 132 (6), 1443–1451. doi:10.1242/dev.01700
Bai, Z., Zhou, Y., Ye, Z., Xiong, J., Lan, H., and Wang, F. (2021). Tumor-infiltrating lymphocytes in colorectal cancer: the fundamental indication and application on immunotherapy. Front. Immunol. 12, 808964. doi:10.3389/fimmu.2021.808964
Bajpai, P., Agarwal, S., Afaq, F., Al Diffalha, S., Chandrashekar, D. S., Kim, H. G., et al. (2024). Combination of dual JAK/HDAC inhibitor with regorafenib synergistically reduces tumor growth, metastasis, and regorafenib-induced toxicity in colorectal cancer. J. Exp. Clin. Cancer Res. 43 (1), 192. doi:10.1186/s13046-024-03106-8
Banerjee, S., Biehl, A., Gadina, M., Hasni, S., and Schwartz, D. M. (2017). JAK-STAT signaling as a target for inflammatory and autoimmune diseases: current and future prospects. Drugs 77 (5), 521–546. doi:10.1007/s40265-017-0701-9
Bekaii-Saab, T., Okusaka, T., Goldstein, D., Oh, D. Y., Ueno, M., Ioka, T., et al. (2023). Napabucasin plus nab-paclitaxel with gemcitabine versus nab-paclitaxel with gemcitabine in previously untreated metastatic pancreatic adenocarcinoma: an adaptive multicentre, randomised, open-label, phase 3, superiority trial. EClinicalMedicine 58, 101897. doi:10.1016/j.eclinm.2023.101897
Bernard, H., Garmy-Susini, B., Ainaoui, N., Van Den Berghe, L., Peurichard, A., Javerzat, S., et al. (2013). The p53 isoform, Δ133p53α, stimulates angiogenesis and tumour progression. Oncogene 32 (17), 2150–2160. doi:10.1038/onc.2012.242
Biller, L. H., and Schrag, D. (2021). Diagnosis and treatment of metastatic colorectal cancer: a review. Jama 325 (7), 669–685. doi:10.1001/jama.2021.0106
Bitsch, R., Kurzay, A., Özbay, K. F., De La, T. C., Lasser, S., Lepper, A., et al. (2022). STAT3 inhibitor Napabucasin abrogates MDSC immunosuppressive capacity and prolongs survival of melanoma-bearing mice. J. Immunother. Cancer 10 (3), e004384. doi:10.1136/jitc-2021-004384
Bose, S., Banerjee, S., Mondal, A., Chakraborty, U., Pumarol, J., Croley, C. R., et al. (2020). Targeting the JAK/STAT signaling pathway using phytocompounds for cancer prevention and therapy. Cells 9 (6), 1451. doi:10.3390/cells9061451
Bray, F., Laversanne, M., Sung, H., Ferlay, J., Siegel, R. L., Soerjomataram, I., et al. (2024). Global cancer statistics 2022: GLOBOCAN estimates of incidence and mortality worldwide for 36 cancers in 185 countries. CA Cancer J. Clin. 74 (3), 229–263. doi:10.3322/caac.21834
Campbell, H., Fleming, N., Roth, I., Mehta, S., Wiles, A., Williams, G., et al. (2018). ∆133p53 isoform promotes tumour invasion and metastasis via interleukin-6 activation of JAK-STAT and RhoA-ROCK signalling. Nat. Commun. 9 (1), 254. doi:10.1038/s41467-017-02408-0
Cañellas-Socias, A., Sancho, E., and Batlle, E. (2024). Mechanisms of metastatic colorectal cancer. Nat. Rev. Gastroenterol. Hepatol. 21 (9), 609–625. doi:10.1038/s41575-024-00934-z
Chalikonda, G., Lee, H., Sheik, A., and Huh, Y. S. (2021). Targeting key transcriptional factor STAT3 in colorectal cancer. Mol. Cell Biochem. 476 (9), 3219–3228. doi:10.1007/s11010-021-04156-8
Chan, A. T., and Giovannucci, E. L. (2010). Primary prevention of colorectal cancer. Gastroenterology 138 (6), 2029–2043. doi:10.1053/j.gastro.2010.01.057
Chan, J. M., Zaidi, S., Love, J. R., Zhao, J. L., Setty, M., Wadosky, K. M., et al. (2022). Lineage plasticity in prostate cancer depends on JAK/STAT inflammatory signaling. Science 377 (6611), 1180–1191. doi:10.1126/science.abn0478
Chandra, R., Karalis, J. D., Liu, C., Murimwa, G. Z., Voth Park, J., Heid, C. A., et al. (2021). The colorectal cancer tumor microenvironment and its impact on liver and lung metastasis. Cancers (Basel) 13 (24), 6206. doi:10.3390/cancers13246206
Che, L. H., Liu, J. W., Huo, J. P., Luo, R., Xu, R. M., He, C., et al. (2021). A single-cell atlas of liver metastases of colorectal cancer reveals reprogramming of the tumor microenvironment in response to preoperative chemotherapy. Cell Discov. 7 (1), 80. doi:10.1038/s41421-021-00312-y
Chen, M., and Wang, S. (2024). Preclinical development and clinical studies of targeted JAK/STAT combined Anti-PD-1/PD-L1 therapy. Int. Immunopharmacol. 130, 111717. doi:10.1016/j.intimp.2024.111717
Chen, X., Chen, L. J., Peng, X. F., Deng, L., Wang, Y., Li, J. J., et al. (2024). Anti-PD-1/PD-L1 therapy for colorectal cancer: clinical implications and future considerations. Transl. Oncol. 40, 101851. doi:10.1016/j.tranon.2023.101851
Chen, X., Gu, J., Wu, Y., Liang, P., Shen, M., Xi, J., et al. (2020). Clinical characteristics of colorectal cancer patients and anti-neoplasm activity of genistein. Biomed. Pharmacother. 124, 109835. doi:10.1016/j.biopha.2020.109835
Chen, Y., Zhang, Y., Wang, J., Cai, X., Chen, J., Min, X., et al. (2024). Drug-loaded tumor-derived microparticles elicit CD8+ T cell-mediated anti-tumor response in hepatocellular carcinoma. Int. J. Nanomedicine 19, 2227–2239. doi:10.2147/ijn.S449694
Chung, S. Y., Chen, Y. H., Lin, P. R., Chao, T. C., Su, J. C., Shiau, C. W., et al. (2018). Two novel SHP-1 agonists, SC-43 and SC-78, are more potent than regorafenib in suppressing the in vitro stemness of human colorectal cancer cells. Cell Death Discov. 4, 25. doi:10.1038/s41420-018-0084-z
Cordero, J. B., Stefanatos, R. K., Myant, K., Vidal, M., and Sansom, O. J. (2012). Non-autonomous crosstalk between the Jak/Stat and Egfr pathways mediates Apc1-driven intestinal stem cell hyperplasia in the Drosophila adult midgut. Development 139 (24), 4524–4535. doi:10.1242/dev.078261
Damerau, A., Gaber, T., Ohrndorf, S., and Hoff, P. (2020). JAK/STAT activation: a general mechanism for bone development, homeostasis, and regeneration. Int. J. Mol. Sci. 21 (23), 9004. doi:10.3390/ijms21239004
Dariya, B., Muppala, S., Srivani, G., Momin, S., Alam, A., and Saddala, M. S. (2021). Targeting STAT proteins via computational analysis in colorectal cancer. Mol. Cell Biochem. 476 (1), 165–174. doi:10.1007/s11010-020-03893-6
Dennison, L., Ruggieri, A., Mohan, A., Leatherman, J., Cruz, K., Woolman, S., et al. (2021). Context-dependent immunomodulatory effects of MEK inhibition are enhanced with T-cell agonist therapy. Cancer Immunol. Res. 9 (10), 1187–1201. doi:10.1158/2326-6066.Cir-21-0147
Erdogan, F., Radu, T. B., Orlova, A., Qadree, A. K., De Araujo, E. D., Israelian, J., et al. (2022). JAK-STAT core cancer pathway: an integrative cancer interactome analysis. J. Cell Mol. Med. 26 (7), 2049–2062. doi:10.1111/jcmm.17228
Fan, D., Liu, P., Li, Z., He, X., Zhang, L., Jiang, W., et al. (2024). Design, synthesis and biological evaluation of novel naphthoquinothiazole derivatives as potent antitumor agents through inhibiting STAT3. Bioorg Chem. 150, 107565. doi:10.1016/j.bioorg.2024.107565
Favoino, E., Prete, M., Catacchio, G., Ruscitti, P., Navarini, L., Giacomelli, R., et al. (2021). Working and safety profiles of JAK/STAT signaling inhibitors. Are these small molecules also smart? Autoimmun. Rev. 20 (3), 102750. doi:10.1016/j.autrev.2021.102750
Fernández, S., Solórzano, J. L., Díaz, E., Menéndez, V., Maestre, L., Palacios, S., et al. (2023). JAK/STAT blockade reverses the malignant phenotype of Hodgkin and Reed-Sternberg cells. Blood Adv. 7 (15), 4135–4147. doi:10.1182/bloodadvances.2021006336
Fernandez-Garcia, N. I., Palmer, H. G., Garcia, M., Gonzalez-Martin, A., Del Rio, M., Barettino, D., et al. (2005). 1alpha,25-Dihydroxyvitamin D3 regulates the expression of Id1 and Id2 genes and the angiogenic phenotype of human colon carcinoma cells. Oncogene 24 (43), 6533–6544. doi:10.1038/sj.onc.1208801
Fessler, E., and Medema, J. P. (2016). Colorectal cancer subtypes: developmental origin and microenvironmental regulation. Trends Cancer 2 (9), 505–518. doi:10.1016/j.trecan.2016.07.008
Fogelman, D., Cubillo, A., García-Alfonso, P., Mirón, M. L. L., Nemunaitis, J., Flora, D., et al. (2018). Randomized, double-blind, phase two study of ruxolitinib plus regorafenib in patients with relapsed/refractory metastatic colorectal cancer. Cancer Med. 7 (11), 5382–5393. doi:10.1002/cam4.1703
Frank, M. H., Wilson, B. J., Gold, J. S., and Frank, N. Y. (2021). Clinical implications of colorectal cancer stem cells in the age of single-cell omics and targeted therapies. Gastroenterology 160 (6), 1947–1960. doi:10.1053/j.gastro.2020.12.080
Fremder, E., Munster, M., Aharon, A., Miller, V., Gingis-Velitski, S., Voloshin, T., et al. (2014). Tumor-derived microparticles induce bone marrow-derived cell mobilization and tumor homing: a process regulated by osteopontin. Int. J. Cancer 135 (2), 270–281. doi:10.1002/ijc.28678
Froeling, F. E. M., Swamynathan, M. M., Deschênes, A., Chio, I. I. C., Brosnan, E., Yao, M. A., et al. (2019). Bioactivation of napabucasin triggers reactive oxygen species-mediated cancer cell death. Clin. Cancer Res. 25 (23), 7162–7174. doi:10.1158/1078-0432.Ccr-19-0302
Fujita, K., Mondal, A. M., Horikawa, I., Nguyen, G. H., Kumamoto, K., Sohn, J. J., et al. (2009). p53 isoforms Delta133p53 and p53beta are endogenous regulators of replicative cellular senescence. Nat. Cell Biol. 11 (9), 1135–1142. doi:10.1038/ncb1928
Gadea, G., Arsic, N., Fernandes, K., Diot, A., Joruiz, S. M., Abdallah, S., et al. (2016). TP53 drives invasion through expression of its Δ133p53β variant. Elife 5, e14734. doi:10.7554/eLife.14734
Ghafouri-Fard, S., Hussen, B. M., Nicknafs, F., Nazer, N., Sayad, A., and Taheri, M. (2021). Expression analysis of protein inhibitor of activated STAT in inflammatory demyelinating polyradiculoneuropathy. Front. Immunol. 12, 659038. doi:10.3389/fimmu.2021.659038
Ghasemian, A., Omear, H. A., Mansoori, Y., Mansouri, P., Deng, X., Darbeheshti, F., et al. (2023). Long non-coding RNAs and JAK/STAT signaling pathway regulation in colorectal cancer development. Front. Genet. 14, 1297093. doi:10.3389/fgene.2023.1297093
Giordano, G., Parcesepe, P., D'andrea, M. R., Coppola, L., Di Raimo, T., Remo, A., et al. (2019). JAK/Stat5-mediated subtype-specific lymphocyte antigen 6 complex, locus G6D (LY6G6D) expression drives mismatch repair proficient colorectal cancer. J. Exp. Clin. Cancer Res. 38 (1), 28. doi:10.1186/s13046-018-1019-5
Gong, J., Lin, Y., Zhang, H., Liu, C., Cheng, Z., Yang, X., et al. (2020). Reprogramming of lipid metabolism in cancer-associated fibroblasts potentiates migration of colorectal cancer cells. Cell Death Dis. 11 (4), 267. doi:10.1038/s41419-020-2434-z
Gong, J., Wang, C., Lee, P. P., Chu, P., and Fakih, M. (2017). Response to PD-1 blockade in microsatellite stable metastatic colorectal cancer harboring a POLE mutation. J. Natl. Compr. Canc Netw. 15 (2), 142–147. doi:10.6004/jnccn.2017.0016
Guillén Díaz-Maroto, N., Sanz-Pamplona, R., Berdiel-Acer, M., Cimas, F. J., García, E., Gonçalves-Ribeiro, S., et al. (2019). Noncanonical TGFβ pathway relieves the blockade of IL1β/TGFβ-mediated crosstalk between tumor and stroma: TGFBR1 and TAK1 inhibition in colorectal cancer. Clin. Cancer Res. 25 (14), 4466–4479. doi:10.1158/1078-0432.Ccr-18-3957
Guo, J., Yu, Z., Sun, D., Zou, Y., Liu, Y., and Huang, L. (2021). Two nanoformulations induce reactive oxygen species and immunogenetic cell death for synergistic chemo-immunotherapy eradicating colorectal cancer and hepatocellular carcinoma. Mol. Cancer 20 (1), 10. doi:10.1186/s12943-020-01297-0
Gutiérrez-Hoya, A., and Soto-Cruz, I. (2020). Role of the JAK/STAT pathway in cervical cancer: its relationship with HPV E6/E7 oncoproteins. Cells 9 (10), 2297. doi:10.3390/cells9102297
Heczko, L., Hlaváč, V., Holý, P., Dvořák, P., Liška, V., Vyčítal, O., et al. (2023). Prognostic potential of whole exome sequencing in the clinical management of metachronous colorectal cancer liver metastases. Cancer Cell Int. 23 (1), 295. doi:10.1186/s12935-023-03135-x
Hong, Y., Chen, Q., Wang, Z., Zhang, Y., Li, B., Guo, H., et al. (2024). Targeting nuclear receptor coactivator SRC-1 prevents colorectal cancer immune escape by reducing transcription and protein stability of PD-L1. Adv. Sci. (Weinh) 11, e2310037. doi:10.1002/advs.202310037
Hou, S. X., Zheng, Z., Chen, X., and Perrimon, N. (2002). The Jak/STAT pathway in model organisms: emerging roles in cell movement. Dev. Cell 3 (6), 765–778. doi:10.1016/s1534-5807(02)00376-3
Hsieh, R. C., Krishnan, S., Wu, R. C., Boda, A. R., Liu, A., Winkler, M., et al. (2022). ATR-mediated CD47 and PD-L1 up-regulation restricts radiotherapy-induced immune priming and abscopal responses in colorectal cancer. Sci. Immunol. 7 (72), eabl9330. doi:10.1126/sciimmunol.abl9330
Hsu, M. C., Chang, H. C., and Hung, W. C. (2007). HER-2/neu transcriptionally activates Jab1 expression via the AKT/beta-catenin pathway in breast cancer cells. Endocr. Relat. Cancer 14 (3), 655–667. doi:10.1677/erc-07-0077
Hu, J. L., Wang, W., Lan, X. L., Zeng, Z. C., Liang, Y. S., Yan, Y. R., et al. (2019). CAFs secreted exosomes promote metastasis and chemotherapy resistance by enhancing cell stemness and epithelial-mesenchymal transition in colorectal cancer. Mol. Cancer 18 (1), 91. doi:10.1186/s12943-019-1019-x
Hu, W. M., Liu, S. Q., Zhu, K. F., Li, W., Yang, Z. J., Yang, Q., et al. (2023). The ALOX5 inhibitor Zileuton regulates tumor-associated macrophage M2 polarization by JAK/STAT and inhibits pancreatic cancer invasion and metastasis. Int. Immunopharmacol. 121, 110505. doi:10.1016/j.intimp.2023.110505
Hu, X., Dutta, P., Tsurumi, A., Li, J., Wang, J., Land, H., et al. (2013). Unphosphorylated STAT5A stabilizes heterochromatin and suppresses tumor growth. Proc. Natl. Acad. Sci. U S A. 110 (25), 10213–10218. doi:10.1073/pnas.1221243110
Hu, X., Li, J., Fu, M., Zhao, X., and Wang, W. (2021). The JAK/STAT signaling pathway: from bench to clinic. Signal Transduct. Target Ther. 6 (1), 402. doi:10.1038/s41392-021-00791-1
Hubbard, J. M., and Grothey, A. (2017). Napabucasin: an update on the first-in-class cancer stemness inhibitor. Drugs 77 (10), 1091–1103. doi:10.1007/s40265-017-0759-4
Huynh, J., Baloyan, D., Chisanga, D., Shi, W., O'brien, M., Afshar-Sterle, S., et al. (2021). Host IL11 signaling suppresses CD4(+) T cell-mediated antitumor responses to colon cancer in mice. Cancer Immunol. Res. 9 (7), 735–747. doi:10.1158/2326-6066.Cir-19-1023
Ireland, H., Kemp, R., Houghton, C., Howard, L., Clarke, A. R., Sansom, O. J., et al. (2004). Inducible Cre-mediated control of gene expression in the murine gastrointestinal tract: effect of loss of beta-catenin. Gastroenterology 126 (5), 1236–1246. doi:10.1053/j.gastro.2004.03.020
Jia, J., Zhou, X., and Chu, Q. (2024). Mechanisms and therapeutic prospect of the JAK-STAT signaling pathway in liver cancer. Mol. Cell Biochem. doi:10.1007/s11010-024-04983-5
Jiang, L., Zhao, X. H., Mao, Y. L., Wang, J. F., Zheng, H. J., and You, Q. S. (2019). Long non-coding RNA RP11-468E2.5 curtails colorectal cancer cell proliferation and stimulates apoptosis via the JAK/STAT signaling pathway by targeting STAT5 and STAT6. J. Exp. Clin. Cancer Res. 38 (1), 465. doi:10.1186/s13046-019-1428-0
Jing, B., Guo, F., An, R., Gao, Y., Li, Y., Xie, Y., et al. (2023). Apoptotic tumor cell-derived microparticles loading Napabucasin inhibit CSCs and synergistic immune therapy. J. Nanobiotechnology 21 (1), 37. doi:10.1186/s12951-023-01792-8
Jonker, D. J., Nott, L., Yoshino, T., Gill, S., Shapiro, J., Ohtsu, A., et al. (2018). Napabucasin versus placebo in refractory advanced colorectal cancer: a randomised phase 3 trial. Lancet Gastroenterol. Hepatol. 3 (4), 263–270. doi:10.1016/s2468-1253(18)30009-8
Joruiz, S. M., Beck, J. A., Horikawa, I., and Harris, C. C. (2020). The Δ133p53 isoforms, tuners of the p53 pathway. Cancers (Basel) 12 (11), 3422. doi:10.3390/cancers12113422
Kanani, A., Veen, T., and Søreide, K. (2021). Neoadjuvant immunotherapy in primary and metastatic colorectal cancer. Br. J. Surg. 108 (12), 1417–1425. doi:10.1093/bjs/znab342
Kasprzak, A. (2021). The role of tumor microenvironment cells in colorectal cancer (CRC) cachexia. Int. J. Mol. Sci. 22 (4), 1565. doi:10.3390/ijms22041565
Kim, J. H., Park, H. E., Cho, N. Y., Lee, H. S., and Kang, G. H. (2016). Characterisation of PD-L1-positive subsets of microsatellite-unstable colorectal cancers. Br. J. Cancer 115 (4), 490–496. doi:10.1038/bjc.2016.211
Kobayashi, H., Gieniec, K. A., Lannagan, T. R. M., Wang, T., Asai, N., Mizutani, Y., et al. (2022). The origin and contribution of cancer-associated fibroblasts in colorectal carcinogenesis. Gastroenterology 162 (3), 890–906. doi:10.1053/j.gastro.2021.11.037
Koncina, E., Nurmik, M., Pozdeev, V. I., Gilson, C., Tsenkova, M., Begaj, R., et al. (2023). IL1R1(+) cancer-associated fibroblasts drive tumor development and immunosuppression in colorectal cancer. Nat. Commun. 14 (1), 4251. doi:10.1038/s41467-023-39953-w
Kopalli, S. R., Annamneedi, V. P., and Koppula, S. (2022). Potential natural biomolecules targeting JAK/STAT/SOCS signaling in the management of atopic dermatitis. Molecules 27 (14), 4660. doi:10.3390/molecules27144660
Korehisa, S., Oki, E., Iimori, M., Nakaji, Y., Shimokawa, M., Saeki, H., et al. (2018). Clinical significance of programmed cell death-ligand 1 expression and the immune microenvironment at the invasive front of colorectal cancers with high microsatellite instability. Int. J. Cancer 142 (4), 822–832. doi:10.1002/ijc.31107
Krebs, D. L., and Hilton, D. J. (2001). SOCS proteins: negative regulators of cytokine signaling. Stem Cells 19 (5), 378–387. doi:10.1634/stemcells.19-5-378
La Fortezza, M., Schenk, M., Cosolo, A., Kolybaba, A., Grass, I., and Classen, A. K. (2016). JAK/STAT signalling mediates cell survival in response to tissue stress. Development 143 (16), 2907–2919. doi:10.1242/dev.132340
La Manna, S., De Benedictis, I., and Marasco, D. (2021). Proteomimetics of natural regulators of JAK-STAT pathway: novel therapeutic perspectives. Front. Mol. Biosci. 8, 792546. doi:10.3389/fmolb.2021.792546
Laudisi, F., Cherubini, F., Di Grazia, A., Dinallo, V., Di Fusco, D., Franzè, E., et al. (2019). Progranulin sustains STAT3 hyper-activation and oncogenic function in colorectal cancer cells. Mol. Oncol. 13 (10), 2142–2159. doi:10.1002/1878-0261.12552
Lee, K. S., Kwak, Y., Ahn, S., Shin, E., Oh, H. K., Kim, D. W., et al. (2017). Prognostic implication of CD274 (PD-L1) protein expression in tumor-infiltrating immune cells for microsatellite unstable and stable colorectal cancer. Cancer Immunol. Immunother. 66 (7), 927–939. doi:10.1007/s00262-017-1999-6
Li, G., Yao, Q., Liu, P., Zhang, H., Liu, Y., Li, S., et al. (2024). Critical roles and clinical perspectives of RNA methylation in cancer. MedComm (2020) 5 (5), e559. doi:10.1002/mco2.559
Li, H., Liang, J., Wang, J., Han, J., Li, S., Huang, K., et al. (2021). Mex3a promotes oncogenesis through the RAP1/MAPK signaling pathway in colorectal cancer and is inhibited by hsa-miR-6887-3p. Cancer Commun. (Lond). 41 (6), 472–491. doi:10.1002/cac2.12149
Li, N., Lu, B., Luo, C., Cai, J., Lu, M., Zhang, Y., et al. (2021). Incidence, mortality, survival, risk factor and screening of colorectal cancer: a comparison among China, Europe, and northern America. Cancer Lett. 522, 255–268. doi:10.1016/j.canlet.2021.09.034
Li, W. X. (2008). Canonical and non-canonical JAK-STAT signaling. Trends Cell Biol. 18 (11), 545–551. doi:10.1016/j.tcb.2008.08.008
Li, X., Yang, Z., Chen, B., Gu, L., Tian, G., and Sui, X. (2023). SOCS3 as a potential driver of lung metastasis in colon cancer patients. Front. Immunol. 14, 1088542. doi:10.3389/fimmu.2023.1088542
Li, Y., Shen, Z., Chai, Z., Zhan, Y., Zhang, Y., Liu, Z., et al. (2023). Targeting MS4A4A on tumour-associated macrophages restores CD8+ T-cell-mediated antitumour immunity. Gut 72 (12), 2307–2320. doi:10.1136/gutjnl-2022-329147
Liang, C., Zhao, T., Li, H., He, F., Zhao, X., Zhang, Y., et al. (2019). Long non-coding RNA ITIH4-AS1 accelerates the proliferation and metastasis of colorectal cancer by activating JAK/STAT3 signaling. Mol. Ther. Nucleic Acids 18, 183–193. doi:10.1016/j.omtn.2019.08.009
Liang, D., Wang, Q., Zhang, W., Tang, H., Song, C., Yan, Z., et al. (2024). JAK/STAT in leukemia: a clinical update. Mol. Cancer 23 (1), 25. doi:10.1186/s12943-023-01929-1
Liao, W., Zhang, L., Chen, X., Xiang, J., Zheng, Q., Chen, N., et al. (2023). Targeting cancer stem cells and signalling pathways through phytochemicals: a promising approach against colorectal cancer. Phytomedicine 108, 154524. doi:10.1016/j.phymed.2022.154524
Lin, T. E., Huangfu, W. C., Chao, M. W., Sung, T. Y., Chang, C. D., Chen, Y. Y., et al. (2018). A novel selective JAK2 inhibitor identified using pharmacological interactions. Front. Pharmacol. 9, 1379. doi:10.3389/fphar.2018.01379
Liu, G., Bao, Y., Liu, C., Zhu, Q., Zhao, L., Lu, X., et al. (2020). IKKε phosphorylates kindlin-2 to induce invadopodia formation and promote colorectal cancer metastasis. Theranostics 10 (5), 2358–2373. doi:10.7150/thno.40397
Liu, J., Wang, F., and Luo, F. (2023). The role of JAK/STAT pathway in fibrotic diseases: molecular and cellular mechanisms. Biomolecules 13 (1), 119. doi:10.3390/biom13010119
Liu, Y., Lau, H. C., Cheng, W. Y., and Yu, J. (2023). Gut microbiome in colorectal cancer: clinical diagnosis and treatment. Genomics Proteomics Bioinforma. 21 (1), 84–96. doi:10.1016/j.gpb.2022.07.002
López-Mejía, J. A., Mantilla-Ollarves, J. C., and Rocha-Zavaleta, L. (2023). Modulation of JAK-STAT signaling by LNK: a forgotten oncogenic pathway in hormone receptor-positive breast cancer. Int. J. Mol. Sci. 24 (19), 14777. doi:10.3390/ijms241914777
Lu, Y., Chen, Y., Li, Y., Xu, S., Lian, D., Liang, J., et al. (2023). Monotropein inhibits colitis associated cancer through VDR/JAK1/STAT1 regulation of macrophage polarization. Int. Immunopharmacol. 124 (Pt A), 110838. doi:10.1016/j.intimp.2023.110838
Luo, M., Xu, X., Liu, X., Shen, W., Yang, L., Zhu, Z., et al. (2022). The non-receptor protein tyrosine phosphatase PTPN6 mediates a positive regulatory approach from the interferon regulatory factor to the JAK/STAT pathway in Litopenaeus vannamei. Front. Immunol. 13, 913955. doi:10.3389/fimmu.2022.913955
Mahjoor, M., Mahmoudvand, G., Farokhi, S., Shadab, A., Kashfi, M., and Afkhami, H. (2023). Double-edged sword of JAK/STAT signaling pathway in viral infections: novel insights into virotherapy. Cell Commun. Signal 21 (1), 272. doi:10.1186/s12964-023-01240-y
Majoros, A., Platanitis, E., Kernbauer-Hölzl, E., Rosebrock, F., Müller, M., and Decker, T. (2017). Canonical and non-canonical aspects of JAK-STAT signaling: lessons from interferons for cytokine responses. Front. Immunol. 8, 29. doi:10.3389/fimmu.2017.00029
Mármol, I., Sánchez-De-Diego, C., Pradilla Dieste, A., Cerrada, E., and Rodriguez Yoldi, M. J. (2017). Colorectal carcinoma: a general overview and future perspectives in colorectal cancer. Int. J. Mol. Sci. 18 (1), 197. doi:10.3390/ijms18010197
Mclornan, D. P., Pope, J. E., Gotlib, J., and Harrison, C. N. (2021). Current and future status of JAK inhibitors. Lancet 398 (10302), 803–816. doi:10.1016/s0140-6736(21)00438-4
Midgley, R., and Kerr, D. (1999). Colorectal cancer. Lancet 353 (9150), 391–399. doi:10.1016/s0140-6736(98)07127-x
Modest, D. P., Pant, S., and Sartore-Bianchi, A. (2019). Treatment sequencing in metastatic colorectal cancer. Eur. J. Cancer 109, 70–83. doi:10.1016/j.ejca.2018.12.019
Morse, M. A., Hochster, H., and Benson, A. (2020). Perspectives on treatment of metastatic colorectal cancer with immune checkpoint inhibitor therapy. Oncologist 25 (1), 33–45. doi:10.1634/theoncologist.2019-0176
Müller, P., Boutros, M., and Zeidler, M. P. (2008). Identification of JAK/STAT pathway regulators--insights from RNAi screens. Semin. Cell Dev. Biol. 19 (4), 360–369. doi:10.1016/j.semcdb.2008.06.001
Nalli, M., Puxeddu, M., La Regina, G., Gianni, S., and Silvestri, R. (2021). Emerging therapeutic agents for colorectal cancer. Molecules 26 (24), 7463. doi:10.3390/molecules26247463
Nguyen, M. T., and Weinberg, D. S. (2016). Biomarkers in colorectal cancer screening. J. Natl. Compr. Canc Netw. 14 (8), 1033–1040. doi:10.6004/jnccn.2016.0109
Niu, G. J., Xu, J. D., Yuan, W. J., Sun, J. J., Yang, M. C., He, Z. H., et al. (2018). Protein inhibitor of activated STAT (PIAS) negatively regulates the JAK/STAT pathway by inhibiting STAT phosphorylation and translocation. Front. Immunol. 9, 2392. doi:10.3389/fimmu.2018.02392
O’shea, J. J., Schwartz, D. M., Villarino, A. V., Gadina, M., Mcinnes, I. B., and Laurence, A. (2015). The JAK-STAT pathway: impact on human disease and therapeutic intervention. Annu. Rev. Med. 66, 311–328. doi:10.1146/annurev-med-051113-024537
Pálmer, H. G., Sánchez-Carbayo, M., Ordóñez-Morán, P., Larriba, M. J., Cordón-Cardó, C., and Muñoz, A. (2003). Genetic signatures of differentiation induced by 1alpha,25-dihydroxyvitamin D3 in human colon cancer cells. Cancer Res. 63 (22), 7799–7806.
Pandey, R., Bakay, M., and Hakonarson, H. (2023). SOCS-JAK-STAT inhibitors and SOCS mimetics as treatment options for autoimmune uveitis, psoriasis, lupus, and autoimmune encephalitis. Front. Immunol. 14, 1271102. doi:10.3389/fimmu.2023.1271102
Pang, Q., You, L., Meng, X., Li, Y., Deng, T., Li, D., et al. (2023). Regulation of the JAK/STAT signaling pathway: the promising targets for cardiovascular disease. Biochem. Pharmacol. 213, 115587. doi:10.1016/j.bcp.2023.115587
Parikh, A. R., Szabolcs, A., Allen, J. N., Clark, J. W., Wo, J. Y., Raabe, M., et al. (2021). Radiation therapy enhances immunotherapy response in microsatellite stable colorectal and pancreatic adenocarcinoma in a phase II trial. Nat. Cancer 2 (11), 1124–1135. doi:10.1038/s43018-021-00269-7
Park, H., Lee, S., Lee, J., Moon, H., and Ro, S. W. (2023). Exploring the JAK/STAT signaling pathway in hepatocellular carcinoma: unraveling signaling complexity and therapeutic implications. Int. J. Mol. Sci. 24 (18), 13764. doi:10.3390/ijms241813764
Park, S. Y., Lee, C. J., Choi, J. H., Kim, J. H., Kim, J. W., Kim, J. Y., et al. (2019). The JAK2/STAT3/CCND2 Axis promotes colorectal Cancer stem cell persistence and radioresistance. J. Exp. Clin. Cancer Res. 38 (1), 399. doi:10.1186/s13046-019-1405-7
Patel, M., Pennel, K. a.F., Quinn, J. A., Hood, H., Chang, D. K., Biankin, A. V., et al. (2022). Spatial expression of IKK-alpha is associated with a differential mutational landscape and survival in primary colorectal cancer. Br. J. Cancer 126 (12), 1704–1714. doi:10.1038/s41416-022-01729-2
Payandeh, Z., Khalili, S., Somi, M. H., Mard-Soltani, M., Baghbanzadeh, A., Hajiasgharzadeh, K., et al. (2020). PD-1/PD-L1-dependent immune response in colorectal cancer. J. Cell Physiol. 235 (7-8), 5461–5475. doi:10.1002/jcp.29494
Pecharromán, I., Solé, L., Álvarez-Villanueva, D., Lobo-Jarne, T., Alonso-Marañón, J., Bertran, J., et al. (2023). IκB kinase-α coordinates BRD4 and JAK/STAT signaling to subvert DNA damage-based anticancer therapy. Embo J. 42 (21), e114719. doi:10.15252/embj.2023114719
Pennel, K. a.F., Hatthakarnkul, P., Wood, C. S., Lian, G. Y., Al-Badran, S. S. F., Quinn, J. A., et al. (2024). JAK/STAT3 represents a therapeutic target for colorectal cancer patients with stromal-rich tumors. J. Exp. Clin. Cancer Res. 43 (1), 64. doi:10.1186/s13046-024-02958-4
Phuengkham, H., Ren, L., Shin, I. W., and Lim, Y. T. (2019). Nanoengineered immune niches for reprogramming the immunosuppressive tumor microenvironment and enhancing cancer immunotherapy. Adv. Mater 31 (34), e1803322. doi:10.1002/adma.201803322
Pickhardt, P. J., Hassan, C., Halligan, S., and Marmo, R. (2011). Colorectal cancer: CT colonography and colonoscopy for detection--systematic review and meta-analysis. Radiology 259 (2), 393–405. doi:10.1148/radiol.11101887
Qin, Y., Shembrey, C., Smith, J., Paquet-Fifield, S., Behrenbruch, C., Beyit, L. M., et al. (2020). Laminin 521 enhances self-renewal via STAT3 activation and promotes tumor progression in colorectal cancer. Cancer Lett. 476, 161–169. doi:10.1016/j.canlet.2020.02.026
Rah, B., Rather, R. A., Bhat, G. R., Baba, A. B., Mushtaq, I., Farooq, M., et al. (2022). JAK/STAT signaling: molecular targets, therapeutic opportunities, and limitations of targeted inhibitions in solid malignancies. Front. Pharmacol. 13, 821344. doi:10.3389/fphar.2022.821344
Ranasinghe, R., Mathai, M., and Zulli, A. (2022). A synopsis of modern - day colorectal cancer: where we stand. Biochim. Biophys. Acta Rev. Cancer 1877 (2), 188699. doi:10.1016/j.bbcan.2022.188699
Rendón, J. P., Cañas, A. I., Correa, E., Bedoya-Betancur, V., Osorio, M., Castro, C., et al. (2022). Evaluation of the effects of genistein in vitro as a chemopreventive agent for colorectal cancer-strategy to improve its efficiency when administered orally. Molecules 27 (20), 7042. doi:10.3390/molecules27207042
Riley, J. M., Cross, A. W., Paulos, C. M., Rubinstein, M. P., Wrangle, J., and Camp, E. R. (2018). The clinical implications of immunogenomics in colorectal cancer: a path for precision medicine. Cancer 124 (8), 1650–1659. doi:10.1002/cncr.31214
Rim, S. H., Seeff, L., Ahmed, F., King, J. B., and Coughlin, S. S. (2009). Colorectal cancer incidence in the United States, 1999-2004: an updated analysis of data from the national program of cancer registries and the surveillance, epidemiology, and end results program. Cancer 115 (9), 1967–1976. doi:10.1002/cncr.24216
Rumpold, H., Niedersüß-Beke, D., Heiler, C., Falch, D., Wundsam, H. V., Metz-Gercek, S., et al. (2020). Prediction of mortality in metastatic colorectal cancer in a real-life population: a multicenter explorative analysis. BMC Cancer 20 (1), 1149. doi:10.1186/s12885-020-07656-w
Saltz, L. (2013). Systemic therapy for metastatic colorectal cancer. J. Natl. Compr. Canc Netw. 11 (5 Suppl. l), 649–652. doi:10.6004/jnccn.2013.0193
Seyfinejad, B., and Jouyban, A. (2022). Importance of method validation in the analysis of biomarker. Curr. Pharm. Anal. 18 (6), 567–569. doi:10.2174/1573412918666211213142638
Shah, M. A., Yoshino, T., Tebbutt, N. C., Grothey, A., Tabernero, J., Xu, R. H., et al. (2023). Napabucasin plus FOLFIRI in patients with previously treated metastatic colorectal cancer: results from the open-label, randomized phase III CanStem303C study. Clin. Colorectal Cancer 22 (1), 100–110. doi:10.1016/j.clcc.2022.11.002
Shao, Z., Wang, H., Ren, H., Sun, Y., and Chen, X. (2023). The anticancer effect of napabucasin (BBI608), a natural naphthoquinone. Molecules 28 (15), 5678. doi:10.3390/molecules28155678
Shi, J., Sun, Z., Gao, Z., Huang, D., Hong, H., and Gu, J. (2023). Radioimmunotherapy in colorectal cancer treatment: present and future. Front. Immunol. 14, 1105180. doi:10.3389/fimmu.2023.1105180
Shin, A. E., Giancotti, F. G., and Rustgi, A. K. (2023). Metastatic colorectal cancer: mechanisms and emerging therapeutics. Trends Pharmacol. Sci. 44 (4), 222–236. doi:10.1016/j.tips.2023.01.003
Siegel, R. L., Miller, K. D., Goding, S. A., Fedewa, S. A., Butterly, L. F., Anderson, J. C., et al. (2020). Colorectal cancer statistics, 2020. CA Cancer J. Clin. 70 (3), 145–164. doi:10.3322/caac.21601
Silva, V. R., Santos, L. S., Dias, R. B., Quadros, C. A., and Bezerra, D. P. (2021). Emerging agents that target signaling pathways to eradicate colorectal cancer stem cells. Cancer Commun. (Lond). 41 (12), 1275–1313. doi:10.1002/cac2.12235
Singh, S., and Singh, S. (2020). JAK-STAT inhibitors: immersing therapeutic approach for management of rheumatoid arthritis. Int. Immunopharmacol. 86, 106731. doi:10.1016/j.intimp.2020.106731
Slattery, M. L., Lundgreen, A., Kadlubar, S. A., Bondurant, K. L., and Wolff, R. K. (2013). JAK/STAT/SOCS-signaling pathway and colon and rectal cancer. Mol. Carcinog. 52 (2), 155–166. doi:10.1002/mc.21841
Stintzing, S., Wirapati, P., Lenz, H. J., Neureiter, D., Fischer Von Weikersthal, L., Decker, T., et al. (2019). Consensus molecular subgroups (CMS) of colorectal cancer (CRC) and first-line efficacy of FOLFIRI plus cetuximab or bevacizumab in the FIRE3 (AIO KRK-0306) trial. Ann. Oncol. 30 (11), 1796–1803. doi:10.1093/annonc/mdz387
Sun, B., Kawahara, M., Ehata, S., and Nagamune, T. (2014). AAG8 promotes carcinogenesis by activating STAT3. Cell Signal 26 (9), 1863–1869. doi:10.1016/j.cellsig.2014.04.001
Sun, H., Ma, D., Cheng, Y., Li, J., Zhang, W., Jiang, T., et al. (2023). The JAK-STAT signaling pathway in epilepsy. Curr. Neuropharmacol. 21 (10), 2049–2069. doi:10.2174/1570159x21666221214170234
Tabernero, J., Van Cutsem, E., Lakomý, R., Prausová, J., Ruff, P., Van Hazel, G. A., et al. (2014). Aflibercept versus placebo in combination with fluorouracil, leucovorin and irinotecan in the treatment of previously treated metastatic colorectal cancer: prespecified subgroup analyses from the VELOUR trial. Eur. J. Cancer 50 (2), 320–331. doi:10.1016/j.ejca.2013.09.013
Taheri, M., Azimi, G., Sayad, A., Mazdeh, M., Arsang-Jang, S., Omrani, M. D., et al. (2018). Expression analysis of protein inhibitor of activated STAT (PIAS) genes in IFNβ-treated multiple sclerosis patients. J. Inflamm. Res. 11, 457–463. doi:10.2147/jir.S187414
Taniguchi, H., Masuishi, T., Kawazoe, A., Muro, K., Kadowaki, S., Bando, H., et al. (2021). Phase I study of napabucasin in combination with FOLFIRI + bevacizumab in Japanese patients with metastatic colorectal cancer. Int. J. Clin. Oncol. 26 (11), 2017–2024. doi:10.1007/s10147-021-01987-9
Tanuma, N., Shima, H., Nakamura, K., and Kikuchi, K. (2001). Protein tyrosine phosphatase epsilonC selectively inhibits interleukin-6- and interleukin- 10-induced JAK-STAT signaling. Blood 98 (10), 3030–3034. doi:10.1182/blood.v98.10.3030
Tian, J., Chen, J. H., Chao, S. X., Pelka, K., Giannakis, M., Hess, J., et al. (2023). Combined PD-1, BRAF and MEK inhibition in BRAF(V600E) colorectal cancer: a phase 2 trial. Nat. Med. 29 (2), 458–466. doi:10.1038/s41591-022-02181-8
Tobin, J. M., and Cooper, M. A. (2024). PTPN2 deficiency: amping up JAK/STAT. J. Exp. Med. 221 (9), e20240980. doi:10.1084/jem.20240980
Trivedi, S., and Starz-Gaiano, M. (2018). Drosophila jak/STAT signaling: regulation and relevance in human cancer and metastasis. Int. J. Mol. Sci. 19 (12), 4056. doi:10.3390/ijms19124056
Vainchenker, W., and Constantinescu, S. N. (2013). JAK/STAT signaling in hematological malignancies. Oncogene 32 (21), 2601–2613. doi:10.1038/onc.2012.347
Van Cutsem, E., Borràs, J. M., Castells, A., Ciardiello, F., Ducreux, M., Haq, A., et al. (2013). Improving outcomes in colorectal cancer: where do we go from here? Eur. J. Cancer 49 (11), 2476–2485. doi:10.1016/j.ejca.2013.03.026
Venook, A. P., Niedzwiecki, D., Lenz, H. J., Innocenti, F., Fruth, B., Meyerhardt, J. A., et al. (2017). Effect of first-line chemotherapy combined with cetuximab or bevacizumab on overall survival in patients with kras wild-type advanced or metastatic colorectal cancer: a randomized clinical trial. Jama 317 (23), 2392–2401. doi:10.1001/jama.2017.7105
Verhoeven, Y., Tilborghs, S., Jacobs, J., De Waele, J., Quatannens, D., Deben, C., et al. (2020). The potential and controversy of targeting STAT family members in cancer. Semin. Cancer Biol. 60, 41–56. doi:10.1016/j.semcancer.2019.10.002
Wang, J., Zhang, Y., Song, H., Yin, H., Jiang, T., Xu, Y., et al. (2021). The circular RNA circSPARC enhances the migration and proliferation of colorectal cancer by regulating the JAK/STAT pathway. Mol. Cancer 20 (1), 81. doi:10.1186/s12943-021-01375-x
Wang, Y., Wang, Z., Li, S., Ma, J., Dai, X., and Lu, J. (2024). Deciphering JAK/STAT signaling pathway: a multifaceted approach to tumorigenesis, progression and therapeutic interventions. Int. Immunopharmacol. 131, 111846. doi:10.1016/j.intimp.2024.111846
Wang, Z., Jin, H., Xu, R., Mei, Q., and Fan, D. (2009). Triptolide downregulates Rac1 and the JAK/STAT3 pathway and inhibits colitis-related colon cancer progression. Exp. Mol. Med. 41 (10), 717–727. doi:10.3858/emm.2009.41.10.078
Wong, S. H., and Yu, J. (2019). Gut microbiota in colorectal cancer: mechanisms of action and clinical applications. Nat. Rev. Gastroenterol. Hepatol. 16 (11), 690–704. doi:10.1038/s41575-019-0209-8
Wu, F., Sun, G., Nai, Y., Shi, X., Ma, Y., and Cao, H. (2024). NUP43 promotes PD-L1/nPD-L1/PD-L1 feedback loop via TM4SF1/JAK/STAT3 pathway in colorectal cancer progression and metastatsis. Cell Death Discov. 10 (1), 241. doi:10.1038/s41420-024-02025-z
Xin, P., Xu, X., Deng, C., Liu, S., Wang, Y., Zhou, X., et al. (2020). The role of JAK/STAT signaling pathway and its inhibitors in diseases. Int. Immunopharmacol. 80, 106210. doi:10.1016/j.intimp.2020.106210
Xing, F., Zheng, R., Liu, B., Huang, K., Wang, D., Su, R., et al. (2023). A new strategy for searching determinants in colorectal cancer progression through whole-part relationship combined with multi-omics. Talanta 259, 124543. doi:10.1016/j.talanta.2023.124543
Xiong, J., He, J., Zhu, J., Pan, J., Liao, W., Ye, H., et al. (2022). Lactylation-driven METTL3-mediated RNA m(6)A modification promotes immunosuppression of tumor-infiltrating myeloid cells. Mol. Cell 82 (9), 1660–1677.e10. doi:10.1016/j.molcel.2022.02.033
Xu, D., and Qu, C. K. (2008). Protein tyrosine phosphatases in the JAK/STAT pathway. Front. Biosci. 13, 4925–4932. doi:10.2741/3051
Xue, C., Li, G., Lu, J., and Li, L. (2021). Crosstalk between circRNAs and the PI3K/AKT signaling pathway in cancer progression. Signal Transduct. Target Ther. 6 (1), 400. doi:10.1038/s41392-021-00788-w
Xue, C., Yao, Q., Gu, X., Shi, Q., Yuan, X., Chu, Q., et al. (2023). Evolving cognition of the JAK-STAT signaling pathway: autoimmune disorders and cancer. Signal Transduct. Target Ther. 8 (1), 204. doi:10.1038/s41392-023-01468-7
Yang, H., Li, S., Qu, Y., Li, L., Li, Y., and Wang, D. (2022). Anti-colorectal cancer effects of inonotus hispidus (Bull.: Fr.) P. Karst. Spore powder through regulation of gut microbiota-mediated JAK/STAT signaling. Nutrients 14 (16), 3299. doi:10.3390/nu14163299
Yoshimura, A., Naka, T., and Kubo, M. (2007). SOCS proteins, cytokine signalling and immune regulation. Nat. Rev. Immunol. 7 (6), 454–465. doi:10.1038/nri2093
You, A. B., Yang, H., Lai, C. P., Lei, W., Yang, L., Lin, J. L., et al. (2023). CMTR1 promotes colorectal cancer cell growth and immune evasion by transcriptionally regulating STAT3. Cell Death Dis. 14 (4), 245. doi:10.1038/s41419-023-05767-3
Yu, H., Lee, H., Herrmann, A., Buettner, R., and Jove, R. (2014). Revisiting STAT3 signalling in cancer: new and unexpected biological functions. Nat. Rev. Cancer 14 (11), 736–746. doi:10.1038/nrc3818
Yu, Z., Gao, J., Zhang, X., Peng, Y., Wei, W., Xu, J., et al. (2022). Characterization of a small-molecule inhibitor targeting NEMO/IKKβ to suppress colorectal cancer growth. Signal Transduct. Target Ther. 7 (1), 71. doi:10.1038/s41392-022-00888-1
Yuan, W., Deng, D., Jiang, H., Tu, C., Shang, X., He, H., et al. (2019). Hyperresponsiveness to interferon gamma exposure as a response mechanism to anti-PD-1 therapy in microsatellite instability colorectal cancer. Cancer Immunol. Immunother. 68 (2), 257–268. doi:10.1007/s00262-018-2270-5
Zhang, X., Wu, T., Cai, X., Dong, J., Xia, C., Zhou, Y., et al. (2022). Neoadjuvant immunotherapy for MSI-H/dMMR locally advanced colorectal cancer: new strategies and unveiled opportunities. Front. Immunol. 13, 795972. doi:10.3389/fimmu.2022.795972
Zhang, Y., Wang, Y., Zhang, B., Li, P., and Zhao, Y. (2023). Methods and biomarkers for early detection, prediction, and diagnosis of colorectal cancer. Biomed. Pharmacother. 163, 114786. doi:10.1016/j.biopha.2023.114786
Zhang, Y. G., Lu, R., Wu, S., Chatterjee, I., Zhou, D., Xia, Y., et al. (2020). Vitamin D receptor protects against dysbiosis and tumorigenesis via the JAK/STAT pathway in intestine. Cell Mol. Gastroenterol. Hepatol. 10 (4), 729–746. doi:10.1016/j.jcmgh.2020.05.010
Zhao, H., Zhao, B., Wu, L., Xiao, H., Ding, K., Zheng, C., et al. (2019). Amplified cancer immunotherapy of a surface-engineered antigenic microparticle vaccine by synergistically modulating tumor microenvironment. ACS Nano 13 (11), 12553–12566. doi:10.1021/acsnano.9b03288
Zhao, Q., Zong, H., Zhu, P., Su, C., Tang, W., Chen, Z., et al. (2024). Crosstalk between colorectal CSCs and immune cells in tumorigenesis, and strategies for targeting colorectal CSCs. Exp. Hematol. Oncol. 13 (1), 6. doi:10.1186/s40164-024-00474-x
Zheng, H., Liu, H., Li, H., Dou, W., Wang, J., Zhang, J., et al. (2022). Characterization of stem cell landscape and identification of stemness-relevant prognostic gene signature to aid immunotherapy in colorectal cancer. Stem Cell Res. Ther. 13 (1), 244. doi:10.1186/s13287-022-02913-0
Keywords: JAK-STAT, colorectal cancer, mechanism, prognosis, therapeutic potential
Citation: Li P and Huang D (2024) Targeting the JAK-STAT pathway in colorectal cancer: mechanisms, clinical implications, and therapeutic potential. Front. Cell Dev. Biol. 12:1507621. doi: 10.3389/fcell.2024.1507621
Received: 08 October 2024; Accepted: 11 November 2024;
Published: 26 November 2024.
Edited by:
Bilal Çiğ, Ahi Evran University, TürkiyeReviewed by:
Jens Staal, Ghent University, BelgiumMansoor-Ali Vaali-Mohammed, King Saud University, Saudi Arabia
Copyright © 2024 Li and Huang. This is an open-access article distributed under the terms of the Creative Commons Attribution License (CC BY). The use, distribution or reproduction in other forums is permitted, provided the original author(s) and the copyright owner(s) are credited and that the original publication in this journal is cited, in accordance with accepted academic practice. No use, distribution or reproduction is permitted which does not comply with these terms.
*Correspondence: Penghui Li, bHBoMDgxOUAxNjMuY29t