- 1Shandong Key Laboratory of Animal Disease Control and Breeding, Institute of Animal Science and Veterinary Medicine, Shandong Academy of Agricultural Sciences, Jinan, China
- 2Key Laboratory of Livestock and Poultry Multi-omics of Ministry of Agriculture and Rural Affairs (MARA), Jinan, China
Porcine reproductive and respiratory syndrome (PRRS) is one of the most economically devastating infectious diseases of pigs globally. The pathogen, porcine reproductive and respiratory syndrome virus (PRRSV), is an enveloped positive-stranded RNA virus, which is considered to be the key triggers for the activation of effective innate immunity through pattern recognition receptor (PRR)-dependent signaling pathways. Toll-like receptors (TLRs), RIG-I-like receptors (RLRs), C-type lectin receptors (CLRs), NOD-like receptors (NLRs) and Cytoplasmic DNA receptors (CDRs) are used as PRRs to identify distinct but overlapping microbial components. The innate immune system has evolved to recognize RNA or DNA molecules from microbes through pattern recognition receptors (PRRs) and to induce defense response against infections, including the production of type I interferon (IFN-I) and inflammatory cytokines. However, PRRSV is capable of continuous evolution through gene mutation and recombination to evade host immune defenses and exploit host cell mechanisms to synthesize and transport its components, thereby facilitating successful infection and replication. This review presents the research progress made in recent years in the study of these PRRs and their associated adapters during PRRSV infection.
Introduction
PRRS is a viral infectious disease that causes reproductive failure in pregnant sows and severe respiratory diseases in pigs of all ages. It is one of the most significant diseases affecting the global pig industry (Butler et al., 2014). The first documented cases of PRRS were reported in the Netherlands in 1991 and in the United States in 1992 (Wensvoort et al., 1991; Collins et al., 1992). PRRSV exhibits a number of unique characteristics, such as mutation and recombination, persistent infection, immunosuppression, and antibody-dependent enhancement of replication (Liu et al., 2017; Chen N. et al., 2018). The rapid evolution and mutation lead to the constant emergence of mutant strains and new strains (Guo et al., 2019). Furthermore, the humoral and cellular immune responses to PRRSV infection are delayed and low. Vaccination represents the primary strategy for the prevention and control of PRRS. However, the vaccines currently in use exhibit suboptimal immune effects and low immune protection rates, which preclude the achievement of complete control of the disease (Han et al., 2017). Therefore, the development of safe and effective vaccines has become a significant challenge in this field. In recent years, the search for host proteins that inhibit PRRSV replication has become the primary focus of anti-PRRSV research.
The innate immune system plays an important role in the process of host antiviral infection, acting as the host’s first line of defense against pathogen invasion. Pathogen associated molecular patterns (PAMPs) are recognized by host germline-encoded pattern recognition receptors, including Toll-like receptors (TLRs), RIG-I-like receptors (RLRs), NOD-like receptors (NLRs), C-type lectin receptors (CLRs), and cytosolic DNA receptors (CDRs) (Beutler, 2004; Mair et al., 2014; Chen et al., 2017). Upon activation, these receptors transmit to the signal adaptor protein, which then activates the expression of the transcription factors interferon regulatory factor 3/7 (IRF3/IRF7) and nuclear factor κB (NF-κB) through a series of signaling pathways. This induces the production of antiviral IFN-I and pro-inflammatory cytokines, collectively serving to resist infection by the pathogen (Liu et al., [[NoYear]]a; Liu et al., [[NoYear]]b).
Toll-like receptors
TLRs are widely expressed in myeloid cells, including monocytes, macrophages, granulocytes, and dendritic cells (DCs). As host PRRs, they play an important role in the recognition of microbial PAMPs and the subsequent activation of specific signaling pathways that induce the transcription of inflammatory and/or anti-inflammatory cytokines (Zhou and Zhang, 2012; Schlaepfer et al., 2014). TLRs bind as homo- or heterodimers to the corresponding ligands on the plasma or organelle membrane and are mainly involved in downstream signaling via two adaptor proteins, Myeloid differentiation primary response protein 88 (MyD88) and Toll-interleukin-1 receptor (TIR)-domain-containing adaptor-inducing IFN-β(TRIF) (Gebremeskel et al., 2021; Liu et al., 2024). The signaling pathway of TLRs can be divided into two categories: the MyD88-dependent signaling pathway and the MyD88 non-dependent signaling pathway, which is based on the identified adaptor proteins. Upon stimulation by an external signal, the TIR domain at the C-terminal of the adaptor protein binds to the TIR domain of TLRs to form a complex. This complex is then recruited by the death domain (DD) at the N-terminal of MyD88, which binds to the signaling protein of the IL-1R-related kinase (IRAKs) family to activate NF-κB transported into the nucleus and induce the expression of pro-inflammatory cytokines (Tapping, [[NoYear]]; Yang and Seki, [[NoYear]]).
There have been several reports on the correlation between TLR and PRRSV infection. Infection with PRRSV in vivo tended to up-regulate the mRNA expression of TLR2, 3, 4, 7, and 8 in at least one of the lymphoid tissues, suggesting that TLR-mediated innate immunity likely plays a critical role in the pathogenesis of PRRSV infection in pigs (Liu et al., 2009). In contrast, the downregulation of TLR7 and 8 by poly(I:C) stimulation and PRRSV infection was observed in both PAMs and immature DCs (Chaung et al., 2010). However, when the TLR3 signaling pathway was activated by poly (I:C), the viral load of PRRSV was significantly reduced, while the expression of TLR3 was inhibited, resulting in enhenced infectivity of PRRSV (Sang et al., 2008; Kuzemtseva et al., 2014). The TLR7 ligand (SZU101) can prevent the replication of PRRSV (Huang et al., 2022). Therefore, TLR3 and TLR7/8 ligands are promising adjuvant candidates for the development of novel vaccines against PRRSV (Zhang et al., 2013a). In a separate study, it was observed that highly pathogenic PRRSV infection induced higher expression levels of TLR3, 7, and 8 mRNA in PAMs and cerebral medullar tissues than low pathogenic PRRSV, indicating that the TLR expression levels were correlated with PRRSV virulence (Zhang et al., 2013b). Another report indicated that the PRRSV 3’ UTR pseudoknot region could act as PAMPs recognized by RIG-I and TLR3 to induce IFN-I production to suppress PRRSV infection (Xie et al., 2018). TLR4 has long been thought to be involved in innate immunity, mediating inflammatory responses by recognizing lipopolysaccharide (LPS) or bacterial endotoxins (Zhang et al., 2022). However, the study found that LPS inhibits PRRSV infection by down-regulating the expression of CD163 through the TLR4-NF-κB pathway (Zhu et al., 2020). PRRSV infection can also induce IL-1β maturation by activating the Nucleotide-binding oligomerization domain (NOD)- leucine-rich repeat (LRR)-and pyrin domain-containing protein 3 (NLRP3) inflammasome through the TLR4-MyD88-NF-κB pathways and then inhibit the proliferation of classical swine fever virus (Chen et al., 2023). In addition, the adaptor protein MyD88 was found to be involved in PRRSV-induced IL-1β production in microglia (Chen et al., 2018) and to play an important role in IL-10 induction during PRRSV infection (Song et al., 2013).
RIG-like receptors
RLRs including retinoic acid-induced gene I (RIG-I), melanoma differentiation associated gene 5 (MDA5) and laboratory of genetics and physiology 2 (LGP2), recognize viral or other xenogeneic nucleic acid in the cytoplasm (Loo and Gale, 2011). RIG-I and MDA5 consist of caspase activation and recruitment domains (CARD), the DExD/H-box helicase domain and a C-terminal domain (CTD) (Yoneyama et al., 2004; Yoneyama et al., 2005). Activation of RIG-I or MDA5 interacts via CARD-CARD interactions with mitochondrial antiviral signaling (MAVS) protein (Seth et al., 2005). These interactions facilitate the relocation of RLRs to mitochondria membranes and the formation of MAVS signalosome with the downstream signaling molecules TANK-binding kinase 1 (TBK1) and IκB kinase-ϵ (IKK-ϵ). This complex phosphorylates and activates IRF3 and IRF7 (Moore and Ting, 2008), which in turn initiate the transcription of a variety of cytokines to resist virus infection. MAVS also recruits the Fas-associated death domain protein (FADD), which activates caspase-10 and caspase-8, driving NF-κB activation (Kawai and Akira, 2007). Upon activation, IRF3/7 and NF-κB translocate to the nucleus to trigger the transcription of a variety of cytokines, which are responsible for resisting virus infection. In comparison to RIG-I and MDA5, LGP2 has helicase and regulatory domains, but lacks the N-terminal CARDs, which are essential for antiviral signal transduction (Zhu et al., 2014). Consequently, LGP2 is unable to initiate the downstream signaling pathway independently and requires binding to RIG-I or MDA5 for signal transduction.
Furthermore, there are associations between PRRSV and members of RLR family. It has been reported that the overexpression of LGP2 can inhibit PRRSV replication. PRRSV Nsp1 and Nsp2 interacted with LGP2 and promoted K63-linked ubiquitination of LGP2, which ultimately led to the degradation of LGP2 (Zhu et al., 2023). The endoribonuclease activity of PRRSV Nsp11 is critical for both viral replication and the inhibition of IFN-I production. Of note, Nsp11 suppresses both MAVS and RIG-I expression to antagonize IFN-I production (Sun et al., 2016). In addition, studies have shown that endogenous expression of the porcine 2’, 5’-oligoadenylate synthetase (OAS2) gene can be enhanced by interferon IFN-β or PRRSV infection in porcine alveolar macrophages (PAMs). Additionally, the porcine OAS2 has been shown to suppress the replication of PRRSV in a RIG-I-dependent manner (Zhao et al., 2017). And the N protein of PRRSV interferes with TRIM25-mediated RIG-I ubiquitination to suppress the host innate immune response (Zhao et al., 2019).The study indicated that MAVS is involved in the interaction between PRRSV and the host immune response and that MAVS mediates a powerful anti-PRRSV process (Zhao et al., 2019). However, Nsp4 of HP-PRRSV has been demonstrated to cleave MAVS to impair antiviral responses mediated by RIG-I-like receptors (Huang et al., 2016). And PRRSV infection promotes glycolysis to produce lactate, which targets MAVS to inhibit RLR signaling and thus promote viral replication (Zhang et al., 2023).
NOD-like receptors
The NLR pathway is also instrumental in the detection of cytosolic DNA and the initiation of inflammation-dependent innate immune signals (Petrilli et al., 2007). Some NLRs are sensitive to many PAMPs and release the inflammatory cytokines of the IL-1 family via caspase-1, including IL-1β, IL-18 and IL-33 (Meylan et al., 2006; Yu and Finlay, 2008). The inflammatory bodies of NLR protein can be divided into three main types: the NALP3 inflammasome (also known as the NLRP3 inflammasome), the NALP1 (NLRP1) inflammasome and the IPAF (NLRC4) inflammasome (Kawai and Akira, 2009). These inflammasomes involve an adaptor—apoptosis-associated speck-like protein containing a CARD (ASC), which links these NLRs to caspase-1 (Kawai and Akira, 2009). Furthermore, all NLRs except NOD1 and NOD2 activate inflammasome formation independently of gene transcription through oligomerization of the adaptor ASC, which induces maturation of IL-1β and IL-18 (Hoss et al., 2017). In contrast to other NLRs, the activation of NOD1 and NOD2 recruits a common downstream signaling adapter, RIPK2, which in turn activates downstream NF-κB and the production of pro-inflammatory cytokines (He and Wang, 2018). Studies have shown that RIPK2 is involved in the activation of NF-κB and MAPK (McCarthy et al., 1998), while porcine RIPK2 does not activate IRF3 (ISRE) activity (Ao et al., 2020). It has been demonstrated that ASC functions as a dual regulator of NF-κB, whereas ectopic ASC is only a weak activator of NF-κB (Stehlik et al., 2002).
DDX19A, a member of the DEAD/H-box protein family, was identified as a novel component of NLRP3 inflammasome. The research found that DDX19A interacted with PRRSV RNA and NLRP3. Knockdown of DDX19A expression efficiently inhibited procaspase-1 cleavage and IL-1β secretion in PRRSV-infected or PRRSV RNA-stimulated PAMs (Li et al., 2015). NLRP3 inflammasome is activated by PRRSV in microglia, which is required for IL-1β secretion (Chen et al., 2018), but the virus protein Nsp11 can inhibit this effect (Wang et al., 2015). PRRSV infection increased the expression of NOD2, NLRP3 and RIP2 and enhances phosphorylation of RIP2 (Jing et al., 2014). NLRX1, a member of the NLR family proteins, is initially identified as key mediators of immune defense and inflammation (Tattoli et al., 2016).The LRR domain of NLRX1 could interacted with the RNA-dependent RNA polymerase (RdRp) domain of Nsp9 to inhibit PRRSV replication (Jing et al., 2019).
CLR like receptor
CLR is comprised of over 1,000 receptors, including soluble or membrane-bound receptors, which are distinguished by the presence of at least one carbohydrate recognition domain (CRD) or C-type lectin-like domain (CTLD) (Zelensky and Gready, 2005). It is well established that CLRs, including Dectin-1, Dectin-2, Dectin-3 and Mincle, play an important role in the host defense against fungal infections by using their CRD to recognize the cell wall component from the infected microorganisms (Shiokawa et al., 2017; Tang et al., 2018). Upon recognition of their carbohydrate agonists, they induce activation of the immunoreceptor tyrosine activating motif (ITAM)-like motif (Hem ITAM) in Dectin-1 or ITAM in the interaction adapter FCRγ of Dectin-2, Dectin-3 and Mincle via Src family kinases. The splenic tyrosine kinase (Syk) was recruited and phosphorylated by activated Hem-TAM/ITAM, and the phosphorylated Syk then triggered the formation of the caspase recruitment domain complex, which included caspase recruitment domain 9 (CARD9), B-cell lymphoma/leukemia 10 (BCL10) and mucosa-associated lymphoid tissue 1 (MALT1). The CARD9-BCL10-MALT1 (CBM) adaptor complex or signalosome subsequently activates the NF-κB pathway through various mechanisms (Ruland and Hartjes, 2019), and produces the cytokines IL-1β, IL-6, and IL-23 (Tang et al., 2018).
The CBM signaling components are highly conserved in evolution and have been extensively studied in humans and mice, and identified in ray-finned fishes (Staal et al., 2018; Ruland and Hartjes, 2019). CARD9, one of the receptors of CLRs, is expressed exclusively in myeloid cells (Gross et al., 2006; Hara et al., 2007). BCL10 is the central adaptor protein whose amino-terminal CARD mediates homologous interaction with CARD9, CARD10, CARD11 or CARD14 (Bertin et al., 2001; Wang et al., 2001; Ruland and Hartjes, 2019). In our previous study, CARD9 was found to be essential for CBM maximal signaling, although it is not constitutively active. Furthermore, BCL10 and MALT1 exhibited optimal synergism when present in moderate amounts of BCL10 and low amounts of MALT1 (Jiang et al., 2020). Among these, MALT1 is rapidly induced upon PRRSV infection and mediates the degradation of two anti-PRRSV RNases, MCPIP1 and N4BP1, relying on its proteolytic activity to facilitate PRRSV replication. Several PRRSV Nsps, including Nsp11, Nsp7β, and Nsp4, has been shown to contribute to MALT1 induction. Finally, PRRSV Nsp6 was found to mediate significant MALT1 degradation via the ubiquitination-proteasome pathway (Gu et al., 2022).
CDR like receptors
CDRs, such as cGAS and IFI16, utilize the adaptor protein STING to activate the expression of transcription factors IRF3/IRF7 and NF-κB, subsequently inducing the downstream production of antiviral IFN-I and proinflammatory cytokines. The innate DNA receptor cGAS is capable of recognizing both self and non-self double-stranded DNA. It is a cyclic GMP-AMP(cGAMP) synthase, and catalyzes the synthesis of 2’3’-cGAMP from ATP and GTP. Subsequently, 2’3’-cGAMP, as a second message, binds STING on endoplasmic reticulum (ER) and triggers the STING translocation from ER. Next, the STING recruits TBK1 which activates IRF3 and NF-κB transcriptions (Sun et al., 2013; Hopfner and Hornung, 2020). The gene transcriptions result in the generation of downstream IFNs, IFN-stimulated genes (ISGs), and proinflammatory cytokines, which play an important antiviral role in virus infections (Sun et al., 2013; Hopfner and Hornung, 2020). STING was initially identified as an IFN-stimulating factor and is widely expressed in a variety of tissues and cells, suggesting that it may have a function in immune regulation (Bo et al., [[NoYear]]; Ishikawa and Barber, [[NoYear]]). Furthermore, STING plays a crucial role in mediating the innate immune response to viruses, intracellular bacteria and even intracellular parasites. Additionally, it is involved in the IFN-I signal pathway which is initiated by pathogenic RNA viruses, through its interaction with the adaptor protein MAVS. It has been demonstrated that the host cGAS-STING signaling pathway plays an important antiviral role in PRRSV infection (Xu et al., 2021). cGAS can restrict PRRSV replication by sensing the mtDNA in the cytoplasm by increasing cGAMP activity (Liu et al., 2022). Recent research has shown that astragaloside IV has the capacity to mitigate the adverse effects of PRRSV infection on innate immune function, reactive the inhibited cGAS-STING signaling pathway, and enhance the expression of IFN-I, ultimately exerting antiviral effects (Song et al., 2023). In addition, PRRSV Nsp2 retains STING in the ER by increasing Ca2+ sensor stromal interaction molecule 1 (STIM1) protein level and deubiquitinates STIM1 through its papain-like protease 2 (PLP2) deubiquitination activity to limit PRRSV replication (Diao et al., 2023).
IFI16, a member of the PYHIN protein family containing a pyrin domain and two DNA-binding HIN domains, displays diverse activity due to its ability to bind to various target proteins and modulate various functions including direct actions in regulation of transcription, proliferation, differentiation, apoptosis, antiviral restriction, and inflammation (Gariano et al., 2012; Orzalli et al., 2012; Jakobsen and Paludan, 2014). IFI16 has been reported to play a broader role in the regulation of interferon-stimulated gene expression, leading to responses not only to DNA viruses, but also to RNA viruses such as Sendai virus (Unterholzner et al., 2010; Thompson et al., 2014). Overexpression of IFI16 could significantly suppress PRRSV-2 replication, and silencing of endogenous IFI16 expression by small interfering RNAs resulted in the promotion of PRRSV-2 replication in MARC-145 cells. Toosendanin inhibits PRRSV-2 via an IFI16-dependent pathway (Zhang et al., 2022). In addition, IFI16 could promote MAVS-mediated IFN-I production and interact with MAVS. More importantly, IFI16 exerted anti-PRRSV effects in a MAVS-dependent manner (Chang et al., 2019).
Perspectives and conclusion
The discovery of transmembrane TLRs and cytoplasmic sensing systems (such as RLRs, NLRs and CDRs) shows that the innate immune system has many recognition mechanisms in different cellular compartments (such as plasma membrane, endosome, lysosome, cytoplasm) and different cell types (such as TLR7 and TLR9 in pDCs and RLR in cDCs) (Kawai and Akira, 2009). Indeed, the relationship between signal adaptors is complex. For example, STING enhanced the activity of MAVS and NF-κB, while MyD88 enhanced the activity of STING ISRE. TIRAP-MyD88 (TLR2 and TLR4 signaling) and TRAM-TRIF (TLR4 signaling) induce inflammatory responses by recruiting TRAF6 (a member of the TRAF protein family) (Kawai and Akira, 2009). MyD88 recruits TRAF3 through TLR7 or TLR9 signaling and TRIF3 recruits TRAF3 in TLR3 signaling, all of which induce IFN-I (Hacker et al., 2006; Oganesyan et al., 2006). TRAF3 is also involved in RLR-mediated IFN-I induction (Saha et al., 2006). In addition, the RLR RIG-I directly interacts and cross-interferes with NOD2 (Morosky et al., 2011). ASC has been shown to interact with MAVS and inhibit MAVS-induced IFN-β production (Han et al., 2018). NLRC3, a member of the NLR protein family, has been shown to be a negative regulator of innate immune signals induced by the DNA sensor STING (Zhang et al., 2014). CARD9 is also involved in TLR, NOD1 and NOD2 mediated MAPK activation (Kawai and Akira, 2009). It appears that each PRR signaling pathway plays an essential role in pathogen elimination or tolerance maintenance (Figure 1).
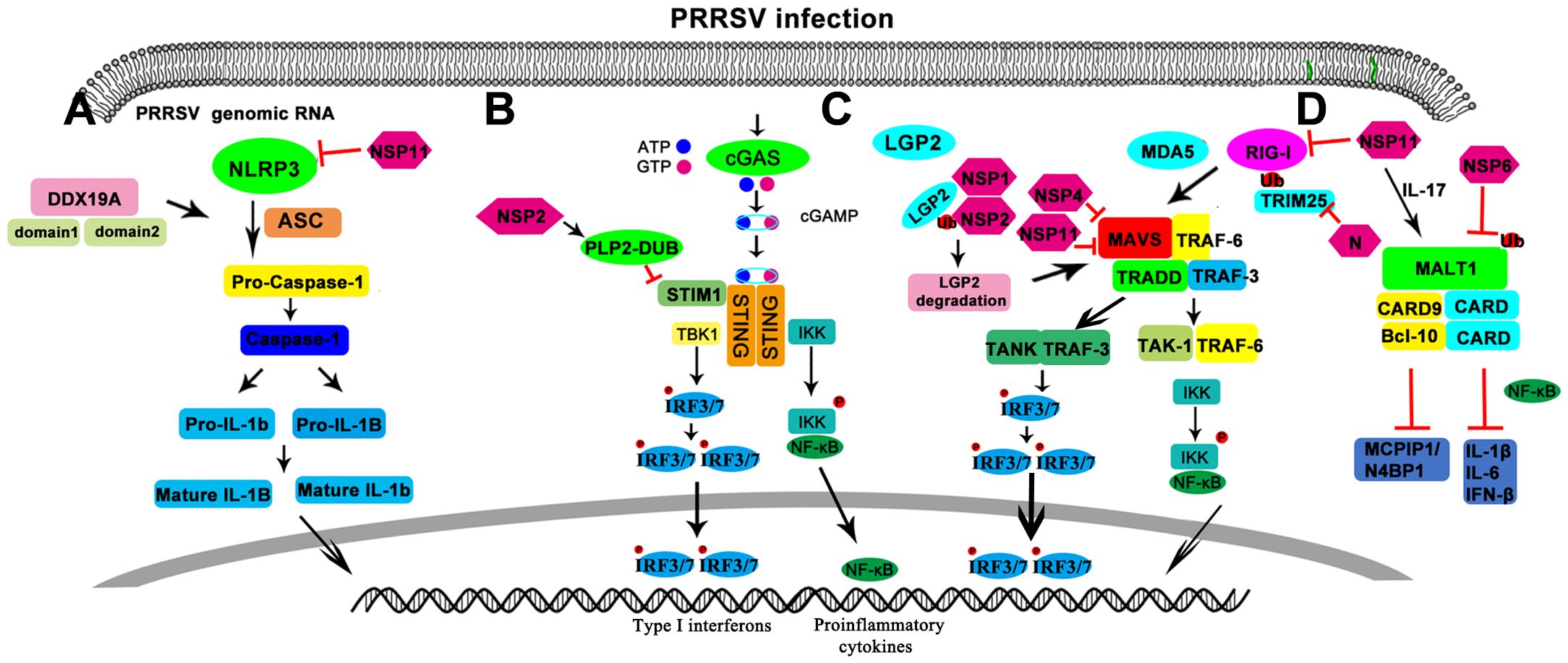
Figure 1. Schematic diagram of regulation of the pattern recognition receptors in PRRSV infection. (A) NOD-like receptors. (B) CDR like receptors. (C) RIG-like receptors. (D) CLR like receptor.
Over the past decade, although scientists from around the world have conducted more research on PRRS and its related signaling pathways, people’s understanding of the pig innate immune signaling pathway is not clear enough. In particular, there are many innate immune signal adaptors and their signaling pathways, making it difficult to have a comprehensive understanding. Therefore, we still need to better understand the dynamics and breadth of innate immunity in tissues-, species- and host-specific manners. Although more adaptor proteins have been found in PRRS viruses, their role in the pathogenesis of PRRS has not been clearly elucidated. Therefore, there is a lack of research and understanding of the effect of swine innate immunity on PRRSV replication and its mechanism. Filling these gaps will provide new ideas for the in-depth study of the molecular mechanisms by which the host recognizes and clears PRRSV infection and by which PRRSV evades the host immune system. In summary, we have reviewed and described the roles of PPRs in host defense against PRRSV infection and proposed areas of research that require further investigation. With the development and application of new immunological approaches available in swine, new insights into the PPRs against PRRSV after infection will be discovered. This will facilitate the development of vaccines against PRRSV and improve our understanding of antiviral immunity in swine.
Author contributions
YX: Writing – original draft. LD: Writing – review & editing. YZ: Writing – review & editing. SR: Writing – review & editing. JL: Writing – review & editing. FL: Writing – review & editing. WS: Writing – review & editing. ZC: Writing – review & editing. JY: Writing – review & editing. JW: Writing – review & editing, Funding acquisition, Supervision.
Funding
The author(s) declare financial support was received for the research, authorship, and/or publication of this article. This study was supported by National Natural Science Funds (32070178), Key R&D Program of Shandong Province, China (2022TZXD0041, 2022CXPT010), Shandong Province Pig Industrial Technology System (SDAIT-08), New high schools 20 in Jinan of Shandong Province (202333065), and Taishan Scholars Program.
Conflict of interest
The authors declare that the research was conducted in the absence of any commercial or financial relationships that could be construed as a potential conflict of interest.
Publisher’s note
All claims expressed in this article are solely those of the authors and do not necessarily represent those of their affiliated organizations, or those of the publisher, the editors and the reviewers. Any product that may be evaluated in this article, or claim that may be made by its manufacturer, is not guaranteed or endorsed by the publisher.
References
Ao, D., Li, S., Jiang, S., Luo, J., Chen, N., Meurens, F., et al. (2020). Inter-relation analysis of signaling adaptors of porcine innate immune pathways. Mol. Immunol. 121, 20–27. doi: 10.1016/j.molimm.2020.02.013
Bertin, J., Wang, L., Guo, Y., Jacobson, M. D., Poyet, J. L., Srinivasula, S. M., et al. (2001). CARD11 and CARD14 are novel caspase recruitment domain (CARD)/membrane-associated guanylate kinase (MAGUK) family members that interact with BCL10 and activate NF-kappa B. J. Biol. Chem. 276, 11877–11882. doi: 10.1074/jbc.M010512200
Beutler, B. (2004). Innate immunity: an overview. Mol. Immunol. 40, 845–859. doi: 10.1016/j.molimm.2003.10.005
Bo, Z., Yan, Y., Shu, L., Wang, Y. Y., Ying, L., Diao, F., et al. (2008). The adaptor protein MITA links virus-sensing receptors to IRF3 transcription factor activation. Immunity 29 (4), 538–550. doi: 10.1016/j.immuni.2008.09.003
Butler, J. E., Lager, K. M., Golde, W., Faaberg, K. S., Sinkora, M., Loving, C., et al. (2014). Porcine reproductive and respiratory syndrome (PRRS): an immune dysregulatory pandemic. Immunol. Res. 59, 81–108. doi: 10.1007/s12026-014-8549-5
Chang, X., Shi, X., Zhang, X., Wang, L., Li, X., Wang, A., et al. (2019). IFI16 inhibits porcine reproductive and respiratory syndrome virus 2 replication in a MAVS-dependent manner in MARC-145 cells. Viruses 11 (12), 1160. doi: 10.3390/v11121160
Chaung, H. C., Chen, C. W., Hsieh, B. L., Chung, W. B. (2010). Toll-Like Receptor expressions in porcine alveolar macrophages and Dendritic Cells in responding to poly IC stimulation and porcine reproductive and respiratory syndrome virus (PRRSV) infection. Comp. Immunol. Microbiol. Infect. Dis. 33 (3), 197–213. doi: 10.1016/j.cimid.2008.10.001
Chen, N., Xia, P., Li, S., Zhang, T., Wang, T.T., Zhu, J. (2017). RNA sensors of the innate immune system and their detection of pathogens. IUBMB Life 69, 297. doi: 10.1002/iub.1625
Chen, N., Ye, M., Shuai, L., Huang, Y., Zhou, R., Yu, X., et al. (2018). Emergence of a novel highly pathogenic recombinant virus from three lineages of porcine reproductive and respiratory syndrome virus 2 in China 2017. Transboundary. Emerging. Dis. 65 (6), 1775–1785. doi: 10.1111/tbed.2018.65.issue-6
Chen, X. X., Guo, Z., Jin, Q., Qiao, S., Li, R., Li, X., et al. (2018). Porcine reproductive and respiratory syndrome virus induces interleukin-1beta through MyD88/ERK/AP-1 and NLRP3 inflammasome in microglia. Vet. Microbiol. 227, 82–89. doi: 10.1016/j.vetmic.2018.10.030
Chen, D., Tuo, T., Zhang, Y., Zhou, L., Ge, X., Han, J., et al. (2023). PRRSV inhibited the proliferation of CSFV by inducing IL-1beta maturation via NLRP3 inflammasome activation. Vet. Microbiol. 284, 109825. doi: 10.1016/j.vetmic.2023.109825
Collins, J. E., Benfield, D. A., Christianson, W. T., Harris, L., Hennings, J. C., Shaw, D. P., et al. (1992). Isolation of swine infertility and respiratory syndrome virus (isolate ATCC VR-2332) in North America and experimental reproduction of the disease in gnotobiotic pigs. J. Vet. Diagn. Invest. 4, 117–126. doi: 10.1177/104063879200400201
Diao, F., Bai, J., Jiang, C., Sun, Y., Gao, Y., Nauwynck, H., et al. (2023). The papain-like protease of porcine reproductive and respiratory syndrome virus impedes STING translocation from the endoplasmic reticulum to the golgi apparatus by deubiquitinating STIM1. J. Virol. 97, e0018823. doi: 10.1128/jvi.00188-23
Gariano, G. R., Dell'Oste, V., Bronzini, M., Gatti, D., Luganini, A., De Andrea, M., et al. (2012). The intracellular DNA sensor IFI16 gene acts as restriction factor for human cytomegalovirus replication. PloS Pathog. 8, e1002498. doi: 10.1371/journal.ppat.1002498
Gebremeskel, S., Schanin, J., Coyle, K. M., Butuci, M., Luu, T., Brock, E. C., et al. (2021). Mast cell and eosinophil activation are associated with COVID-19 and TLR-mediated viral inflammation: implications for an anti-siglec-8 antibody. Front. Immunol. 12, 650331. doi: 10.3389/fimmu.2021.650331
Gross, O., Gewies, A., Finger, K., Schafer, M., Sparwasser, T., Peschel, C., et al. (2006). Card9 controls a non-TLR signalling pathway for innate anti-fungal immunity. Nature 442, 651–656. doi: 10.1038/nature04926
Gu, H., Zheng, S., Han, G., Yang, H., Deng, Z., Liu, Z., et al. (2022). Porcine reproductive and respiratory syndrome virus adapts antiviral innate immunity via manipulating MALT1. mBio 13, e0066422. doi: 10.1128/mbio.00664-22
Guo, Z., Chen, X. X., Li, X., Qiao, S., Deng, R., Zhang, G. (2019). Prevalence and genetic characteristics of porcine reproductive and respiratory syndrome virus in central China during 2016-2017: NADC30-like PRRSVs are predominant. Microb. Pathog. 135, 103657. doi: 10.1016/j.micpath.2019.103657
Hacker, H., Redecke, V., Blagoev, B., Kratchmarova, I., Hsu, L. C., Wang, G. G., et al. (2006). Specificity in Toll-like receptor signalling through distinct effector functions of TRAF3 and TRAF6. Nature 439, 204–207. doi: 10.1038/nature04369
Han, J., Zhou, L., Ge, X., Guo, X., Yang, H. (2017). Pathogenesis and control of the Chinese highly pathogenic porcine reproductive and respiratory syndrome virus. Vet. Microbiol. 209, 30–47. doi: 10.1016/j.vetmic.2017.02.020
Han, Y., He, X., Wei, C., Song, T., Zou, L., Li, Z., et al. (2018). Negative regulation of MAVS-mediated innate immune response by ASC. Mol. Cell Biochem. 445, 35–43. doi: 10.1007/s11010-017-3249-9
Hara, H., Ishihara, C., Takeuchi, A., Imanishi, T., Xue, L., Morris, S. W., et al. (2007). The adaptor protein CARD9 is essential for the activation of myeloid cells through ITAM-associated and Toll-like receptors. Nat. Immunol. 8, 619–629. doi: 10.1038/ni1466
He, S., Wang, X. (2018). RIP kinases as modulators of inflammation and immunity. Nat. Immunol. 19, 912–922. doi: 10.1038/s41590-018-0188-x
Hopfner, K. P., Hornung, V. (2020). Molecular mechanisms and cellular functions of cGAS-STING signalling. Nat. Rev. Mol. Cell Biol. 21, 501–521. doi: 10.1038/s41580-020-0244-x
Hoss, F., Rodriguez-Alcazar, J. F., Latz, E. (2017). Assembly and regulation of ASC specks. Cell Mol. Life Sci. 74, 1211–1229. doi: 10.1007/s00018-016-2396-6
Huang, C., Du, Y., Yu, Z., Zhang, Q., Liu, Y., Tang, J., et al. (2016). Highly pathogenic porcine reproductive and respiratory syndrome virus nsp4 cleaves VISA to impair antiviral responses mediated by RIG-I-like receptors. Sci. Rep. 6, 28497. doi: 10.1038/srep28497
Huang, Q., Yang, H., Yang, D., Hao, Y., Yu, S., Guo, Z., et al. (2022). A synthetic toll-like receptor 7 agonist inhibits porcine reproductive and respiratory syndrome virus replication in piglets. Vet. Microbiol. 271, 109475. doi: 10.1016/j.vetmic.2022.109475
Ishikawa, H., Barber, G. N. (2008). STING is an endoplasmic reticulum adaptor that facilitates innate immune signaling 48 (7213), 674–678. doi: 10.1038/nature07317
Jakobsen, M. R., Paludan, S. R. (2014). IFI16: At the interphase between innate DNA sensing and genome regulation. Cytokine Growth Factor. Rev. 25, 649–655. doi: 10.1016/j.cytogfr.2014.06.004
Jiang, S., Ao, D., Ni, J., Chen, N., Meurens, F., Zhu, J. (2020). The signaling relations between three adaptors of porcine C-type lectin receptor pathway. Dev. Comp. Immunol. 104, 103555. doi: 10.1016/j.dci.2019.103555
Jing, H., Fang, L., Wang, D., Ding, Z., Luo, R., Chen, H., et al. (2014). Porcine reproductive and respiratory syndrome virus infection activates NOD2-RIP2 signal pathway in MARC-145 cells. Virology 458-459, 162–171. doi: 10.1016/j.virol.2014.04.031
Jing, H., Song, T., Cao, S., Sun, Y., Wang, J., Dong, W., et al. (2019). Nucleotide-binding oligomerization domain-like receptor X1 restricts porcine reproductive and respiratory syndrome virus-2 replication by interacting with viral Nsp9. Virus Res. 268, 18–26. doi: 10.1016/j.virusres.2019.05.011
Kawai, T., Akira, S. (2007). SnapShot: pattern-recognition receptors. Cell 129, 1024. doi: 10.1016/j.cell.2007.05.017
Kawai, T., Akira, S. (2009). The roles of TLRs, RLRs and NLRs in pathogen recognition. Int. Immunol. 21, 317–337. doi: 10.1093/intimm/dxp017
Kuzemtseva, L., de la Torre, E., Martin, G., Soldevila, F., Ait-Ali, T., Mateu, E., et al. (2014). Regulation of toll-like receptors 3, 7 and 9 in porcine alveolar macrophages by different genotype 1 strains of porcine reproductive and respiratory syndrome virus. Vet. Immunol. Immunopathol. 158, 189–198. doi: 10.1016/j.vetimm.2014.01.009
Li, J., Hu, L., Liu, Y., Huang, L., Mu, Y., Cai, X., et al. (2015). DDX19A senses viral RNA and mediates NLRP3-dependent inflammasome activation. J. Immunol. 195, 5732–5749. doi: 10.4049/jimmunol.1501606
Liu, S., Cai, X., Wu, J., Cong, Q., Chen, X., Li, T., et al. (2015). Phosphorylation of innate immune adaptor proteins MAVS, STING, and TRIF induces IRF3 activation. Science 347(6227):aaa2630. doi: 10.1126/science.aaa2630
Liu, S., Jueqi, C., Xin, C., Jiaxi, W., Xiang, C., You-Tong, W., et al. (2013). MAVS recruits multiple ubiquitin E3 ligases to activate antiviral signaling cascades. Elife 2:e00785. doi: 10.7554/eLife.00785
Liu, C. H., Chaung, H. C., Chang, H. L., and Peng, Y. T., Chung, W. B. (2009). Expression of Toll-like receptor mRNA and cytokines in pigs infected with porcine reproductive and respiratory syndrome virus. Vet. Microbiol. 136, 266–276. doi: 10.1016/j.vetmic.2008.11.016
Liu, J. K., Zhou, X., Zhai, J. Q., Li, B., Wei, C. H., Dai, A. L., et al. (2017). Emergence of a novel highly pathogenic porcine reproductive and respiratory syndrome virus in China. Transbound Emerg. Dis. 64, 2059–2074. doi: 10.1111/tbed.12617
Liu, X. N., Li, L. W., Gao, F., Jiang, Y. F., Yuan, W. Z., , G. X., et al. (2022). cGAS Restricts PRRSV Replication by Sensing the mtDNA to Increase the cGAMP Activity. Front. Immunol. 13, 887054. doi: 10.3389/fimmu.2022.887054
Liu, B. M., Li, N. L., Wang, R., Li, X., Li, Z. A., Marion, T. N., et al. (2024). Key roles for phosphorylation and the Coiled-coil domain in TRIM56-mediated positive regulation of TLR3-TRIF-dependent innate immunity. J. Biol. Chem. 300, 107249. doi: 10.1016/j.jbc.2024.107249
Loo, Y. M., Gale, M. (2011). Immune signaling by RIG-I-like receptors. Immunity 34, 680–692. doi: 10.1016/j.immuni.2011.05.003
Mair, K. H., Sedlak, C., K?Ser, T., Pasternak, A., Levast, B., Gerner, W., et al. (2014). The porcine innate immune system: An update. Dev. Comp. Immunol. 45, 321–343. doi: 10.1016/j.dci.2014.03.022
McCarthy, J. V., Ni, J., Dixit, V. M. (1998). RIP2 is a novel NF-kappaB-activating and cell death-inducing kinase. J. Biol. Chem. 273, 16968–16975. doi: 10.1074/jbc.273.27.16968
Meylan, E., Tschopp, J., Karin, M. (2006). Intracellular pattern recognition receptors in the host response. Nature 442, 39–44. doi: 10.1038/nature04946
Moore, C. B., Ting, J. P. (2008). Regulation of mitochondrial antiviral signaling pathways. Immunity 28, 735–739. doi: 10.1016/j.immuni.2008.05.005
Morosky, S. A., Zhu, J., Mukherjee, A., Sarkar, S. N., Coyne, C. B. (2011). Retinoic acid-induced gene-I (RIG-I) associates with nucleotide-binding oligomerization domain-2 (NOD2) to negatively regulate inflammatory signaling. J. Biol. Chem. 286, 28574–28583. doi: 10.1074/jbc.M111.227942
Oganesyan, G., Saha, S. K., Guo, B., He, J. Q., Shahangian, A., Zarnegar, B., et al. (2006). Critical role of TRAF3 in the Toll-like receptor-dependent and -independent antiviral response. Nature 439, 208–211. doi: 10.1038/nature04374
Orzalli, M. H., DeLuca, N. A., Knipe, D. M. (2012). Nuclear IFI16 induction of IRF-3 signaling during herpesviral infection and degradation of IFI16 by the viral ICP0 protein. Proc. Natl. Acad. Sci. U.S.A. 109, E3008–E3017. doi: 10.1073/pnas.1211302109
Petrilli, V., Dostert, C., Muruve, D.A., Tschopp, J. (2007). The inflammasome: a danger sensing complex triggering innate immunity. Curr. Opin. Immunol. 19, 615–622. doi: 10.1016/j.coi.2007.09.002
Ruland, J., Hartjes, L. (2019). CARD-BCL-10-MALT1 signalling in protective and pathological immunity. Nat. Rev. Immunol. 19, 118–134. doi: 10.1038/s41577-018-0087-2
Saha, S. K., Pietras, E. M., He, J. Q., Kang, J. R., Liu, S .Y., Oganesyan, G., et al. (2006). Regulation of antiviral responses by a direct and specific interaction between TRAF3 and Cardif. EMBO J. 25, 3257–3263. doi: 10.1038/sj.emboj.7601220
Sang, Y., Ross, C. R., Rowland, R. R., Blecha, F. (2008). Toll-like receptor 3 activation decreases porcine arterivirus infection. Viral Immunol. 21, 303–313. doi: 10.1089/vim.2008.0042
Schlaepfer, E., Rochat, M. A., Duo, L., Speck, R. F. (2014). Triggering TLR2, -3, -4, -5, and -8 reinforces the restrictive nature of M1- and M2-polarized macrophages to HIV. J. Virol. 88, 9769–9781. doi: 10.1128/JVI.01053-14
Seth, R. B., Sun, L., Ea, C. K., Chen, Z. J. (2005). Identification and characterization of MAVS, a mitochondrial antiviral signaling protein that activates NF-kappaB and IRF 3. Cell 122, 669–682. doi: 10.1016/j.cell.2005.08.012
Shiokawa, M., Yamasaki, S., Saijo, S. (2017). C-type lectin receptors in anti-fungal immunity. Curr. Opin. Microbiol. 40, 123–130. doi: 10.1016/j.mib.2017.11.004
Song, S., Bi, J., Wang, D., Fang, L., Zhang, L., Li, F., et al. (2013). Porcine reproductive and respiratory syndrome virus infection activates IL-10 production through NF-kappaB and p38 MAPK pathways in porcine alveolar macrophages. Dev. Comp. Immunol. 39, 265–272. doi: 10.1016/j.dci.2012.10.001
Song, K., Yu, J. Y., Li, J., Li, M., Peng, L. Y., Yi, P. F. (2023). Astragaloside IV regulates cGAS-STING signaling pathway to alleviate immunosuppression caused by PRRSV infection. Viruses 15 (7), 1586. doi: 10.3390/v15071586
Staal, J., Driege, Y., Haegman, M., Borghi, A., Hulpiau, P., Lievens, L., et al. (2018). Ancient origin of the CARD-coiled coil/bcl10/MALT1-like paracaspase signaling complex indicates unknown critical functions. Front. Immunol. 9, 1136. doi: 10.3389/fimmu.2018.01136
Stehlik, C., Fiorentino, L., Dorfleutner, A., Bruey, J. M., Ariza, E. M., Sagara, J., et al. (2002). The PAAD/PYRIN-family protein ASC is a dual regulator of a conserved step in nuclear factor kappaB activation pathways. J. Exp. Med. 196, 1605–1615. doi: 10.1084/jem.20021552
Sun, L., Wu, J., Du, F., Chen, X., Chen, Z. J. (2013). Cyclic GMP-AMP synthase is a cytosolic DNA sensor that activates the type I interferon pathway. Science 339, 786–791. doi: 10.1126/science.1232458
Sun, Y., Ke, H., Han, M., Chen, N., Fang, W., Yoo, D. (2016). Nonstructural protein 11 of porcine reproductive and respiratory syndrome virus suppresses both MAVS and RIG-I expression as one of the mechanisms to antagonize type I interferon production. PloS One 11, e0168314. doi: 10.1371/journal.pone.0168314
Tang, J., Lin, G., Langdon, W. Y., Tao, L., Zhang, J. (2018). Regulation of C-type lectin receptor-mediated antifungal immunity. Front. Immunol. 9, 123. doi: 10.3389/fimmu.2018.00123
Tapping, R. I.(2009). Innate immune sensing and activation of cell surface Toll-like receptors. Semin. Immunol. 21 (4), 175–184. doi: 10.1016/j.smim.2009.05.003
Tattoli, I., Killackey, S. A., Foerster, E. G., Molinaro, R., Maisonneuve, C., Rahman, M. A., et al. (2016). NLRX1 acts as an epithelial-intrinsic tumor suppressor through the modulation of TNF-mediated proliferation. Cell Rep. 14, 2576–2586. doi: 10.1016/j.celrep.2016.02.065
Thompson, M. R., Sharma, S., Atianand, M., Jensen, S. B., Carpenter, S., Knipe, D. M., et al. (2014). Interferon gamma-inducible protein (IFI) 16 transcriptionally regulates type i interferons and other interferon-stimulated genes and controls the interferon response to both DNA and RNA viruses. J. Biol. Chem. 289, 23568–23581. doi: 10.1074/jbc.M114.554147
Unterholzner, L., Keating, S. E., Baran, M., Horan, K. A., Jensen, S. B., Sharma, S., et al. (2010). IFI16 is an innate immune sensor for intracellular DNA. Nat. Immunol. 11, 997–1004. doi: 10.1038/ni.1932
Wang, L., Guo, Y., Huang, W. J., Ke, X., Poyet, J. L., Manji, G. A., et al. (2001). Card10 is a novel caspase recruitment domain/membrane-associated guanylate kinase family member that interacts with BCL10 and activates NF-kappa B. J. Biol. Chem. 276, 21405–21409. doi: 10.1074/jbc.M102488200
Wang, C., Shi, X., Zhang, X., Wang, A., Wang, L., Chen, J., et al. (2015). The endoribonuclease activity essential for the nonstructural protein 11 of porcine reproductive and respiratory syndrome virus to inhibit NLRP3 inflammasome-mediated IL-1beta induction. DNA Cell Biol. 34, 728–735. doi: 10.1089/dna.2015.2929
Wensvoort, G., Terpstra, C., Pol, J. M., ter Laak, E. A., Bloemraad, M., de Kluyver, E. P., et al. (1991). Mystery swine disease in The Netherlands: the isolation of Lelystad virus. Vet. Q. 13, 121–130. doi: 10.1080/01652176.1991.9694296
Xie, S., Chen, X. X., Qiao, S., Li, R., Sun, Y., Xia, S., et al. (2018). Identification of the RNA Pseudoknot within the 3’ End of the Porcine Reproductive and Respiratory Syndrome Virus Genome as a Pathogen-Associated Molecular Pattern To Activate Antiviral Signaling via RIG-I and Toll-Like Receptor 3. J. Virol. 92, e00097–18. doi: 10.1128/JVI.00097-18
Xu, Y., Zhang, Y., Sun, S., Luo, J., Jiang, S., Zhang, J., et al. (2021). The innate immune DNA sensing cGAS-STING signaling pathway mediates anti-PRRSV function. Viruses 13 (9), 1829. doi: 10.3390/v13091829
Yang, L., Seki, E. (2012). Toll-like receptors in liver fibrosis: cellular crosstalk and mechanisms. Front. Physiol. 3, 138. doi: 10.3389/fphys.2012.00138
Yoneyama, M., Kikuchi, M., Natsukawa, T., Shinobu, N., Imaizumi, T., Miyagishi, M., et al. (2004). The RNA helicase RIG-I has an essential function in double-stranded RNA-induced innate antiviral responses. Nat. Immunol. 5, 730–737. doi: 10.1038/ni1087
Yoneyama, M., Kikuchi, M., Matsumoto, K., Imaizumi, T., Miyagishi, M., Taira, K., et al. (2005). Shared and unique functions of the DExD/H-box helicases RIG-I, MDA5, and LGP2 in antiviral innate immunity. J. Immunol. 175, 2851–2858. doi: 10.4049/jimmunol.175.5.2851
Yu, H. B., Finlay, B. B. (2008). The caspase-1 inflammasome: a pilot of innate immune responses. Cell Host Microbe 4, 198–208. doi: 10.1016/j.chom.2008.08.007
Zelensky, A. N., Gready, J. E. (2005). The C-type lectin-like domain superfamily. FEBS J. 272, 6179–6217. doi: 10.1111/j.1742-4658.2005.05031.x
Zhang, L., Bai, J., Liu, J., Wang, X., Li, Y., Jiang, P. (2013a). Toll-like receptor ligands enhance the protective effects of vaccination against porcine reproductive and respiratory syndrome virus in swine. Vet. Microbiol. 164, 253–260. doi: 10.1016/j.vetmic.2013.02.016
Zhang, L., Liu, J., Bai, J., Wang, X., Li, Y., Jiang, P. (2013b). Comparative expression of Toll-like receptors and inflammatory cytokines in pigs infected with different virulent porcine reproductive and respiratory syndrome virus isolates. Virol. J. 10, 135. doi: 10.1186/1743-422X-10-135
Zhang, L., Mo, J., Swanson, K. V., Wen, H., Petrucelli, A., Gregory, S. M., et al. (2014). NLRC3, a member of the NLR family of proteins, is a negative regulator of innate immune signaling induced by the DNA sensor STING. Immunity 40, 329–341. doi: 10.1016/j.immuni.2014.01.010
Zhang, Y., Liang, X., Bao, X., Xiao, W., Chen, G. (2022). Toll-like receptor 4 (TLR4) inhibitors: Current research and prospective. Eur. J. Med. Chem. 235, 114291. doi: 10.1016/j.ejmech.2022.114291
Zhang, M., Lu, C., Su, L., Long, F., Yang, X., Guo, X., et al. (2022). Toosendanin activates caspase-1 and induces maturation of IL-1beta to inhibit type 2 porcine reproductive and respiratory syndrome virus replication via an IFI16-dependent pathway. Vet. Res. 53, 61. doi: 10.1186/s13567-022-01077-2
Zhang, L., Liu, X., Mao, J., Sun, Y., Gao, Y., Bai, J., et al. (2023). Porcine reproductive and respiratory syndrome virus-mediated lactate facilitates virus replication by targeting MAVS. Vet. Microbiol. 284, 109846. doi: 10.1016/j.vetmic.2023.109846
Zhao, M., Wan, B., Li, H., He, J., Chen, X., Wang, L., et al. (2017). Porcine 2’, 5’-oligoadenylate synthetase 2 inhibits porcine reproductive and respiratory syndrome virus replication in vitro. Microb. Pathog. 111, 14–21. doi: 10.1016/j.micpath.2017.08.011
Zhao, K., Li, L. W., Jiang, Y. F., Gao, F., Zhang, Y. J., Zhao, W. Y., et al. (2019). Nucleocapsid protein of porcine reproductive and respiratory syndrome virus antagonizes the antiviral activity of TRIM25 by interfering with TRIM25-mediated RIG-I ubiquitination. Vet. Microbiol. 233, 140–146. doi: 10.1016/j.vetmic.2019.05.003
Zhao, Y., Song, Z., Bai, J., Liu, X., Nauwynck, H., Jiang, P. (2019). ZAP, a CCCH-type zinc finger protein, inhibits porcine reproductive and respiratory syndrome virus replication and interacts with viral nsp9. J. Virol. 93 (10), e00001–19. doi: 10.1128/JVI.00001-19
Zhou, A., Zhang, S. (2012). Regulation of cell signaling and porcine reproductive and respiratory syndrome virus. Cell Signal 24, 973–980. doi: 10.1016/j.cellsig.2012.01.004
Zhu, Z., Zhang, X., Wang, G., Zheng, H. (2014). The laboratory of genetics and physiology 2: emerging insights into the controversial functions of this RIG-I-like receptor. BioMed. Res. Int. 2014, 960190. doi: 10.1155/2014/960190
Zhu, Z., Zhang, H., Zhang, X., He, S., Dong, W., Wang, X., et al. (2020). Lipopolysaccharide downregulates CD163 expression to inhibit PRRSV infection via TLR4-NF-kappaB pathway. Front. Microbiol. 11, 501. doi: 10.3389/fmicb.2020.00501
Keywords: PRRSV, PRRs, innate immune, PAMPS, signaling pathways
Citation: Xu Y, Ding L, Zhang Y, Ren S, Li J, Liu F, Sun W, Chen Z, Yu J and Wu J (2024) Research progress on the pattern recognition receptors involved in porcine reproductive and respiratory syndrome virus infection. Front. Cell. Infect. Microbiol. 14:1428447. doi: 10.3389/fcimb.2024.1428447
Received: 06 May 2024; Accepted: 29 July 2024;
Published: 15 August 2024.
Edited by:
Masmudur Mohammed Rahman, Arizona State University, United StatesReviewed by:
Benjamin M. Liu, George Washington University, United StatesSreya Ghosh, Boston Children’s Hospital and Harvard Medical School, United States
Copyright © 2024 Xu, Ding, Zhang, Ren, Li, Liu, Sun, Chen, Yu and Wu. This is an open-access article distributed under the terms of the Creative Commons Attribution License (CC BY). The use, distribution or reproduction in other forums is permitted, provided the original author(s) and the copyright owner(s) are credited and that the original publication in this journal is cited, in accordance with accepted academic practice. No use, distribution or reproduction is permitted which does not comply with these terms.
*Correspondence: Jiaqiang Wu, d3VqaWFxaWFuZzIwMDBAc2luYS5jb20=; Jiang Yu, eXVqaWFuZ18yMjEzQDE2My5jb20=