- 1Dental and Periodontal Research Center, Tabriz University of Medical Sciences, Tabriz, Iran
- 2Infectious and Tropical Diseases Research Center, Tabriz University of Medical Sciences, Tabriz, Iran
- 3Department of Medical Physics, School of Medicine, Tabriz University of Medical Sciences, Tabriz, Iran
- 4Students Research Committee, Tabriz University of Medical Sciences, Tabriz, Iran
- 5Department of Oral and Maxillofacial Medicine, Faculty of Dentistry, Tabriz University of Medical Sciences, Tabriz, Iran
Periodontitis (PD) is the most common oral infectious disease. The primary etiologic cause of the onset and development of PD is dental plaque, which consists of bacterial biofilm domiciled within a complex extracellular mass. In PD patients, there is a progressive breakdown of the periodontal ligament and the alveolar bone. In more advanced stages, tooth loss occurs. The progression of this chronic inflammatory disease involves interactions among numerous microbial pathogens particularly, bacteria, the host’s immune factors, and various environmental factors. Due to persistent infection by periodonto-pathogenic bacteria, there is an impairment of both innate and acquired immunity, leading to tissue destruction. Chronic inflammation in PD may be associated with several systemic diseases, including cardiovascular conditions, respiratory issues, diabetes, neurological diseases, cancer, and adverse pregnancy outcomes. Antibiotic treatment is one of the effective strategies for treating PD cases, although the emergence of some resistant strains may limit the effectiveness some antibiotics. In this review study, we discussed the main bacteria in PD, the interaction with the immune response, the pathogenesis of bacteria in PD and antibiotic treatment. We also outlined the emergence of resistance to antibiotics among these pathogens.
Introduction
Periodontitis (PD) is a significant public health challenge with a high prevalence and noticeable socio-economic impacts. PD significantly affects patients’ quality of life. It is reported that, over the last decade, the prevalence of PD has reached nearly 60%, which is an increased compare to the same time period in the past (Trindade et al., 2023). PD is a chronic inflammatory condition, primarily caused by the formation of the microbial on dental surfaces, which damages the soft tissue surrounding the teeth. The biofilm consists of a complex community of microorganisms surrounded by an extracellular matrix of biological macromolecules. Biofilm is highly resistant to antimicrobial drugs and host immunity mechanisms. An imbalance in the microbial composition of biofilms, triggers host’s immune response, which leading to a chronic inflammatory condition. Gingivitis is a mild and reversible form of inflammation. If not treated properly, it can progress to PD, a chronic condition that can eventually lead to the entry of bacteria or their byproducts into the bloodstream. This, in turn, triggers the host’s inflammatory response through various mechanisms (Schenkein et al., 2020; Zhang et al., 2022). PD is characterized by damage to the periodontal ligament, pockets formation, and resorption of alveolar bone. These symptoms are induced by the host’s inflammatory response to bacterial biofilms, which express bacterial virulence factors (Preshaw and Bissett, 2019). Cytokines and immune mediators play a crucial role in the progression of PD by regulating inflammatory signals that control local inflammation and tissue destruction (Neurath and Kesting, 2024). The long-lasting presence of plaque on the surface of teeth leads to its migration into the neighboring periodontal tissues and stimulates the infiltration of host immune cells. The progression of PD is often affected by life style factors, such as deficient oral hygiene and smoking, compounded with genetic and disease-related compromise of the body’s immune response (Gao et al., 2018; Jensen et al., 2018; Deo and Deshmukh, 2019). The most common pathogens that caused PD are Porphyromonas gingivalis, Treponema denticola and Tannerella forsythia (Saquib et al., 2021). Understanding the microbiology and pathogenesis of PD, as well as researching treatment options and drug resistance of the causative agents, will greatly improve the effectiveness of treatment. Currently there are few studies that comprehensively outline the microbiological aspects, inflammatory pathways involved in pathogenesis, antibiotic resistance, virulence factors of the causative microbial agents, and alternative treatment options for PD. The aim of the present study was to discuss the main bacteria involved in PD and their interaction of these bacteria with the immune response. We also provided an overview of the pathogenesis of bacteria in periodontitis and antibiotic treatment and emergence of antibiotics resistance. In this literature review, data on microbiology, pathogenesis, microbial resistance, and treatment of PD were gathered from databases including Google Scholar, Scopus, and PubMed. Searches were conducted using keywords such as “PD microbiology,” “PD pathogenesis,” “PD treatment,” and “antimicrobial resistance in PD-causing pathogens.” All English language articles retrieved were independently reviewed by two individuals.
Pathogenesis of periodontitis
The prolonged presence of plaque on the surface of teeth causes it to migrate into the surrounding periodontal tissues. This migration stimulates the infiltration of host immune cells, particularly polymorphonuclear neutrophils (PMNs), from the blood to the site of infection. It has been proposed that severe forms of PD, which primarily affect young individuals previously categorized as having “aggressive periodontitis” (AgPD) or grade 3 periodontitis (involving resorption of ≥2 mm over five years), may be associated with more unfavorable impacts compared to the more prevalent form known as “chronic periodontitis” (CPD) (Borilova Linhartova et al., 2020; Fuller et al., 2020). AgPD cases are commonly reported in individuals under the age of 30 years and exhibit a rapid progression that poses challenges in treatment (Clark et al., 2017). In contrast, CPD tends to progress at a slower rate. Despite their differences, both AgPD and CPD can present in more severe forms ultimately leading to tooth loss and edentulism (Brito et al., 2018). Although AgPD and CPD share several similarities, there are notable clinical differences between the two, including: (i) age of disease manifestation (i.e.detection), (ii) rate of disease progression, (iii) destructive patterns, (iv) indications of inflammation, and (v) plaque and calculus levels (Armitage and Cullinan, 2010). CPD is characterized by the gradual progression of the disease over time in the absence of appropriate treatment, while AgPD is characterized by rapid attachment loss and bone destruction (Cardoso et al., 2018).
The primary cause of periodontal inflammation is the microbial biofilms that form on the surface of teeth and expand to the gingival crevice (Larsen and Fiehn, 2017). Bacteria in these biofilms exploit their pathogenicity by causing damage to gingival tissue using direct and/or indirect mechanisms. Direct damage is caused by several bacterial factors that affect the cells and intercellular matrix of the host connective tissue. These bacterial factors generally involve secreted bioactive molecules, such as exoenzymes, toxins, and metabolic end-products (Siqueira and Rôças, 2024). Moreover, some somatic contents of bacteria, such as cell wall contents (peptidoglycan and lipoteichoic acid of Gram-positive bacteria and lipopolysaccharide (LPS) of Gram-negative bacteria), fimbriae, flagella, outer membrane proteins (OMPs), vesicles (OMVs), nucleic acids, and exopolysaccharides, are released into the adjacent tissues and stimulate the host immune mediators (Siqueira and Rôças, 2019). Table 1 is presents the virulence factors of common bacterial pathogens involved in PD. Bacterial components can trigger inflammatory and non-inflammatory host cells to express immunological factors and mediators, such as cytokines and prostaglandins. These mediators have a stimulating effect on bone tissue resorption, which is a characteristic feature observed in PD lesions (Lamont et al., 2020) (Figure 1). The purulent exudate formed in acute apical abscesses is another example of indirect bacterial damage to gingival tissue. The host’s immune response to bacterial antigens originating from the root canal is considered the primary factor in the development of pus associated with abscesses (Karamifar et al., 2020). When periodontal tissues encounter bacterial cell-associated components known as pathogen-associated molecular patterns (PAMPs), certain host cell receptors referred as pattern recognition receptors (PRRs), such as Toll-like receptors (TLRs) recognize the pathogens and trigger the host immune response. TLRs are expressed on the surface of gingival epithelial cells, fibroblasts, dendritic cells (DCs), and macrophages (Becerra−Ruiz et al., 2022). PRRs including TLRs, are capable of recognizing conserved regions of PAMPs such as LPS, peptidoglycans, bacterial DNA, and lipoproteins (Li and Wu, 2021). TLRs play an essential role in triggering innate immunity. They are evolutionarily conserved and provide the first line of defense against microbial pathogens upon entry into host tissues (Kawai et al., 2024). Proinflammatory reactions induced by TLRs rely on the stimulation of the nuclear factor kappa B (NF-κB) and mitogen-activated protein kinase (MAPK) pathways. These pathways coordinate the transcription and synthesis of proinflammatory mediators such as cytokines and chemokines (Collins et al., 2019). Thereafter, non-resident leukocytes, such as PMNs are attracted to the site of infection in response to specific cytokines and chemokines produced (Duan et al., 2022). PMNs are the most infiltrated leukocytes (50%-70%) and serves as an initial defense against the microbial pathogens in dental plaque. PMNs play a critical role in maintaining the healthy condition of periodontal tissue and provide the first-line mechanisms in the innate immune system. PMNs employ various specific mechanisms of action, including degranulation, chemotaxis, phagocytosis, formation of reactive oxygen species (ROSs), and the formation of neutrophil extracellular traps (NETs) (Sczepanik et al., 2020).
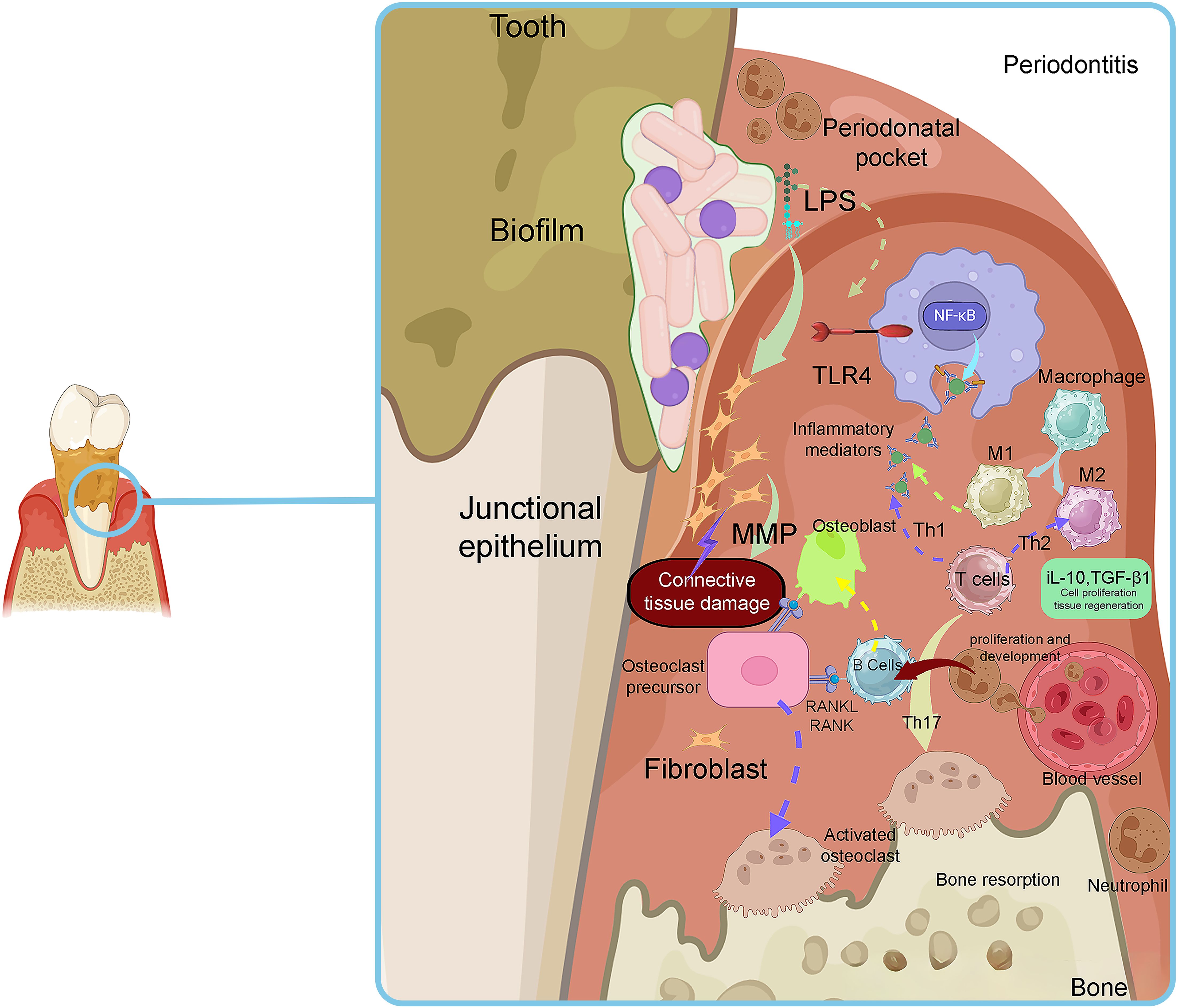
Figure 1. The histopathological aspects of plaque-induced inflammation in PD. Adapted from Sara I Pai et al (Pai et al., 2023).
PMNs are central player in inflammation as they migrate to infected tissues to eliminate invading microorganisms. While PMNs in the gingival crevice can interact with bacterial biofilms on tooth surfaces, they are unable to completely eliminate the entire population of periodontal microorganisms (Alfakry et al., 2016). Whether there is an overabundance or a deficiency of activated PMNs, their role in causing periodontitis remains crucial (Hajishengallis et al., 2015). On the contrary, excessive activation of PMNs can lead to elevated levels of toxic substances and ROS, which characterize localized AgPD (Hasturk and Kantarci, 2015). ROS production can activate granular proteases, leading to the formation of NETs, which are networks composed of extracellular fibers of DNA that restrict invading microorganisms. Moreover, ROS can increase the permeability of bacterial membranes, causing damage to their DNA, proteins, and cell envelope. ROS formation serves as a potent antimicrobial defense mechanism and plays a crucial role in innate immunity against pathogens (Brings et al., 2020; Veenith et al., 2022).
In various types of PD, PMNs can either contribute to antimicrobial defense or tissue damage (Ramadan et al., 2020). PMNs also play a role in periodontal tissue damage by releasing degradative enzymes, such as matrix metalloproteinases (MMPs). MMPs are a class of enzymes responsible for breaking down the ECM contents, including collagen and elastin. In PD, the synthesis of MMPs is increased, primarily by immune cells and resident cells in the periodontal tissues. The overactivity of MMPs leads to the degradation of connective tissue fibers and contributes to alveolar bone loss in PD. PMNs can directly promote osteoclastic bone resorption by increasing the expression of membrane-bound receptor activator of nuclear factor-κB ligand (RANKL), a critical osteoclastogenic cytokine. PMNs also have the ability to release collagenase, which plays an essential role in bone resorption (Hajishengallis et al., 2016). However, PMNs typically do not express soluble RANKL (Chakravarti et al., 2009), and are only capable of mediating periodontal bone resorption when they are adjacent to the bone tissue. During inflammatory conditions, osteoclast progenitors respond to several mediators produced by induced CD4+ T cells including RANKL, TNF-α, and interleukins (IL)-17. Among CD4+ T cells, Th17 cells have been demonstrated to promote osteoclast differentiation. Th17 cells increase expression of IL-17 in inflammatory conditions, which is associated with increased bone destruction and osteoclastogenesis by upregulating RANK in osteoclast progenitors and increasing RANKL expression in osteoblasts (Madel et al., 2019). Osteoprotegerin (OPG) is a member of the TNF receptor superfamily, and acts as a soluble decoy receptor. It inhibits the interaction of RANKL with its functional receptor on osteoclast precursors (Liu and Zhang, 2015). The expression of RANKL and OPG may also be affected by amelogenin, which regulates odontoclast formation (Galler et al., 2021). A recent study showed that bone resorption is a common feature of PD. This process is intensified by increased RANKL expression and simultaneous downregulation of OPG, leading to an elevated RANKL/OPG ratio and subsequent activation of osteoclasts (Abdullameer and Abdulkareem, 2023).
Approximately one third of the variance observed in the frequency of periodontitis can be attributed to genetic factors. This heritability index has been consistent across different populations studied and tends to increase with the severity of PD (Nibali et al., 2019). Genetic factors play a role in regulating inflammatory responses within affected tissues and the damage induced in the alveolar bone (Tettamanti et al., 2017). Numerous scientific studies have investigated the influence of genes and their variants (polymorphisms) on host responses in periodontitis. Genetic polymorphisms can lead to changes in the encoded proteins or their expression, potentially altering innate and adaptive immune responses, and influencing disease outcomes. Interestingly, certain genetic polymorphisms may also provide protection against disease (Laine et al., 2012). Polymorphisms, defined as genetic variants occurring in at least 1% of a population, originate from mutations. Approximately 90% of polymorphisms are Single Nucleotide Polymorphisms (SNPs), in which a single nucleotide base is replaced by another. While most SNPs in genes do not alter the protein produced, they can still affect the gene’s function. Given that all forms of PD are associated with bacterial infections, it remains challenging to delineate the relative contributions of genetic and environmental factors to these complex disorders. Specific genetic polymorphisms, such as those in Interleukin-1 (IL-1), IL-6, IL-10, Fcγ receptors (FccR), Vitamin D receptor (VDR), and TNF-α genes, have been shown to be connected with periodontitis (Laine et al., 2012; Sayad et al., 2020). Similarly, polymorphisms in matrix metalloproteinase (MMP) genes may influence the expression or activity of MMPs, potentially increasing susceptibility to periodontal conditions (Li et al., 2016). Some studies have identified associations between the expression of RANKL/RANK/OPG triad elements and PD (Cochran, 2008). Although no genetic polymorphism in the RANKL/RANK/OPG triad genes has been confirmed as a risk factor for root resorption, further research is needed to explore this possibility. Studies have shown that overexpression of RANK in monocyte/macrophage lineage cells and gingival epithelial cells in mice led to decreased alveolar bone height and an increased number of TRAP-positive cells in the alveolar bone. This suggests excessive osteoclast activity and accelerated bone resorption, possibly due to inflammation in the gingival epithelium, which occurs prior to any detectable bone loss (Sojod et al., 2017).
Dental plaque formation
Dental plaque, also known as microbial plaque, oral biofilm, or dental biofilm, is a complex and highly organized community of microorganisms. Biofilm forms on the surfaces of teeth and is embedded in a matrix of polymers from both host and bacterial origins. Oral biofilms play a crucial role in the development of a variety of diseases in the oral cavity and throat, including dental caries, PD, endodontic infections, tonsillitis, and alveolitis (Colombo et al., 2015). Biofilm formation begins with the initial adhesion of single free floating, planktonic cells to a surface (Sauer et al., 2022) (Figure 2). Loose, long-range physicochemical interactions between the microbial cell surfaces and the pellicle-coated tooth enable reversible adhesion through van der Waals forces and hydrophobic interactions. This is followed by more robust and closer interactions between ligands on the surface of the early colonizers and matching receptors in the pellicle, ultimately leading to permanent attachment through their pili (see Figure 2). Thus, the acquired pellicle, largely derived from the host, actively guides the pattern of initial microbial colonization (Barnier et al., 2021; Darveau and Curtis, 2021). Bacterial biofilm is composed of bacteria that are enclosed in a polymeric matrix that they produce (Bîrluţiu et al., 2017). During this process, a self-generated matrix of extracellular polymeric substance (EPS) forms a tough barrier, shielding the bacteria from external stress (Das, 2022). The accumulation of small bacterial clusters and layers leads to the formation of plaque biofilm. In PD, mature biofilm is characterized by matrix macromolecules such as extracellular DNA (exDNA). This exDNA exists in two forms within the matrix: free DNA and extracellular DNA traps (ETs). Regardless of its origin, exDNA is essential for initiating biofilm formation and maintaining its three-dimensional structure (Wei et al., 2024). Microbial communities within the biofilm engage in a series of physical, metabolic, and molecular interactions that can influence antibiotic resistance and pathogenicity. Studies have shown that microbial cells embedded in the biofilm are 10–1000 times more resistant to antibiotics compared to planktonic cells (Digel et al., 2020). Bacterial attachment to biomaterial surfaces involves a variety of physicochemical interactions and biological processes, with mechanisms that are specific to either the bacteria or the substrate (Kreve and Dos Reis, 2021). When saliva contacts the teeth, proteins from the saliva stick to the tooth surface, creating an acquired salivary pellicle. This pellicle covers all tooth surfaces in the mouth and serves as a link between the dental hard tissue and the oral environment (Chawhuaveang et al., 2021). The pellicle is primarily composed of proteins, amino acids, fatty acids, glycoproteins, carbohydrates, lipids, and other compounds present in saliva. It also contains microorganisms like bacteria and fungi. This layer acts as a lubricant and provides protective properties for the teeth (Enax et al., 2023). The attachment of bacteria to the surfaces of teeth during dental plaque formation occurs through a hydrophobic interaction. This interaction involves a phenylalanine side chain of bacterial surface molecules and a leucine side chain of a salivary glycoprotein in the formed pellicle. Additionally, calcium bridging (takes place, which is the results of an electrostatic attraction) between a negatively charged carboxyl group of a bacterial protein and a positively charged calcium ion.
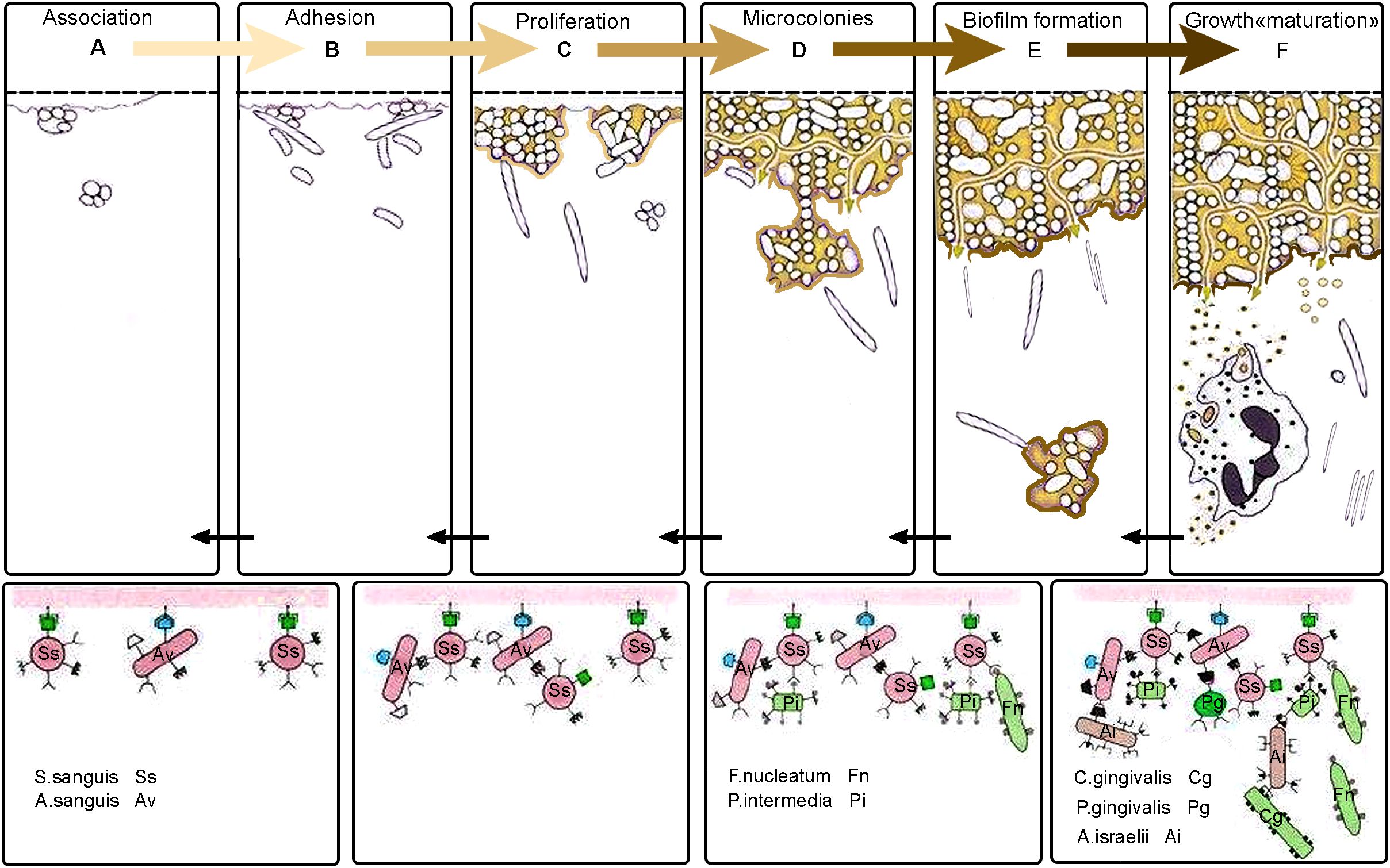
Figure 2. Biofilm formation stages on teeth surface. (A) Association: Free-floating bacteria come into contact with the dental surface, which is coated with an acquired pellicle. This pellicle is a layer made up of proteins and glycoproteins that come from saliva. (B) Adhesion: Primary colonizing microorganisms firmly attach to the pellicle using specific adhesion molecules. (C) Proliferation: These bacteria multiply and begin to form microcolonies, producing extracellular polymeric substances (EPS) that strengthen and stabilize the developing biofilm structure. (D) Microcolonies: Facultative anaerobic and anaerobic bacteria, such as Fusobacterium nucleatum and Prevotella intermedia, integrate into the microbial community. This integration allows for interactions with more pathogenic bacteria, like Porphyromonas gingivalis. (E) Biofilm Formation: As the biofilm continues to develop, it becomes increasingly complex and denser. The structural organization of the biofilm allows for the formation of channels that regulate nutrient diffusion and waste removal within the microbial community. (F) Growth "maturation": Some bacteria, particularly planktonic forms, detach from the biofilm. These detached cells become free-floating within the fluid environment of the oral cavity. Once separated, these planktonic forms can reattach to the acquired pellicle that forms on freshly cleaned tooth surfaces. This initiates a new cycle, continuing through the phases of association, adhesion, proliferation, microcolony, and biofilm maturation. This cycle repeats, leading to continuous biofilm development.
Furthermore, dietary sucrose is transformed by bacterial glucosyltransferase into glucan. Glucan contains multiple functional residues that can interact with amino acid side chains like serine, tyrosine, and threonine. Bacterial fimbriae play a role in providing the terminal adhesin portion necessary to bind to a sugar component of a salivary glycoprotein in the acquired pellicle (Digel et al., 2020).
Streptococci compete for adhesion sites on the saliva-covered surface of teeth and can produce antimicrobial substances. S. mutans can dominate oral biofilms, leading to the development of dental caries (Moschioni et al., 2010). S. mutans is the primary early colonizing bacteria responsible for dental caries because of its ability to recognize salivary pellicle receptors. S. mutans antigen I/II (Ag I/II) is a key factor in this process, playing a vital role in adhering to the tooth surface and in microbial co-aggregation during the initial stages of biofilm formation (Rivera-Quiroga et al., 2020). Oral bacteria that are unable to adhere to surfaces are carried into the digestive system through salivary flow. However, many oral bacteria have developed mechanisms to attach to solid surfaces including teeth covered with salivary films or other bacteria already attached to these surfaces (co-aggregation), as well as epithelial layers (Kolenbrander et al., 2010). In addition to Ag I/II (also known as SpaP, Pac, P1), S. mutans also produces glycosyltransferases (Gtfs), various glucan-binding proteins (Gbps), and collagen-binding proteins, which play a role in coordinating the plaque formation (Matsumoto-Nakano, 2018). S. mutans biofilm formation is a complex process involving interactions between proteins and bacteria. It begins with the adhesion of a single cell, followed by accumulation, microcolony formation, and eventually developing into a mature biofilm (Krzyściak et al., 2014). Adherence to host tissues is a crucial step in the pathogenic process, typically facilitated by bacterial surface-exposed proteins. In S. mutans, adhesion mechanisms include both sucrose-dependent (requiring Gtfs) and Ag I/II -dependent pathways (Scharnow et al., 2019).
Without sucrose, S. mutans produces key adhesins like Ag I/II, which specifically attach to a glycoprotein known as salivary agglutinin (SAG) (Lamont et al., 1991; Mitchell, 2003). It has been suggested that this also plays a role in bacterial adhesion to teeth (Jakubovics et al., 2005), and biofilm formation. Various oral bacteria have the LuxS/AI-2 quorum sensing (QS) system. LuxS contributes to biofilm formation, regulates acid and oxidative stress tolerance, and controls the production of the lantibiotic mutacin I. Additionally, AI-2-like signaling molecules can influence carbohydrate metabolism and biofilm matrix composition (Wang et al., 2017).
Microbiology of periodontitis
It is crucial to highlight that PD seems to be initiated by a relatively small group of periodontal pathogens within the intricate dental biofilm (Popova et al., 2013). The currently identified periodontal pathogens constitute only a small fraction of the approximately 600 bacterial species that can colonize dental surfaces both above and below the gingival margin, as well as the oral mucous membranes. Clinical and experimental evidence confirm that certain bacterial strains in the periodontal environment can cause inflammation of the gingival tissue and lead to bone destruction. These strains are known as periodontal pathogens (Paster et al., 2006). Over the past three decades, there has become widely believed that PD is polymicrobial infections (Armitage, 2004; Taba et al., 2005). Only a small percentage of the bacteria in dental biofilm are considered pathogenic for periodontal tissues. It is well established that the majority of periodontal pathogens are anaerobes. However, the biofilm can also contain facultative aerobes, capnophiles, and microaerophiles. Their numbers vary based on the conditions within the established biofilm and periodontal pocket. Most periodontal pathogens are the primary agents responsible for actual PD. Certain bacterial species in the periodontal environment, such as Actinomyces, Streptococcus, and Staphylococcus spp., which are part of the commensal flora, can cause opportunistic infections if the ecosystem is disturbed. Detection of Enterobacteria, viruses, and Saccharomyces spp. in periodontal pockets may indicate a superinfection linked to a destructive periodontal process. Some studies have focused on identifying the subgingival flora most characteristic of specific PD. While findings have linked certain periodonto-pathogens to specific periodontal conditions, there is still no definitive evidence that particular bacterial species are unique to different types of PD. Even in small amounts, these bacteria can cause damage to periodontal structures (Socransky et al., 1998; Mombelli et al., 2002).
Microorganisms can cause disease directly by invading host tissues or indirectly through bacterial factors and toxins. In order to be considered a pathogen associated with PD, a microorganism must possess several key characteristics including:
● The microorganism must be present in higher numbers at infection sites compared to healthy tissues.
● Eliminating the microorganism should be associated with inhibiting the progression of infection.
● The microorganism should express virulence factors that are relevant to the infection process and be capable of eliciting a host immune response.
● In vivo pathogenicity assays should indicate the potential of microorganisms to progress PD (Kesic et al., 2008; Posch et al., 2012).
Studies on endodontic-periodontic bacteria commonly refer to the six complexes initially described by Socranski et al. These complexes are color-coded as blue, green, yellow, purple, orange, and red (Socransky et al., 1998) (Figure 3). The red complex bacteria are commonly linked to PD and are often found together in dental plaque, especially in deeper areas near to the epithelial layers of the periodontal pocket. This is largely due to interactions, co-aggregation, and metabolic dependencies among these three bacterial species (Nayak et al., 2018).
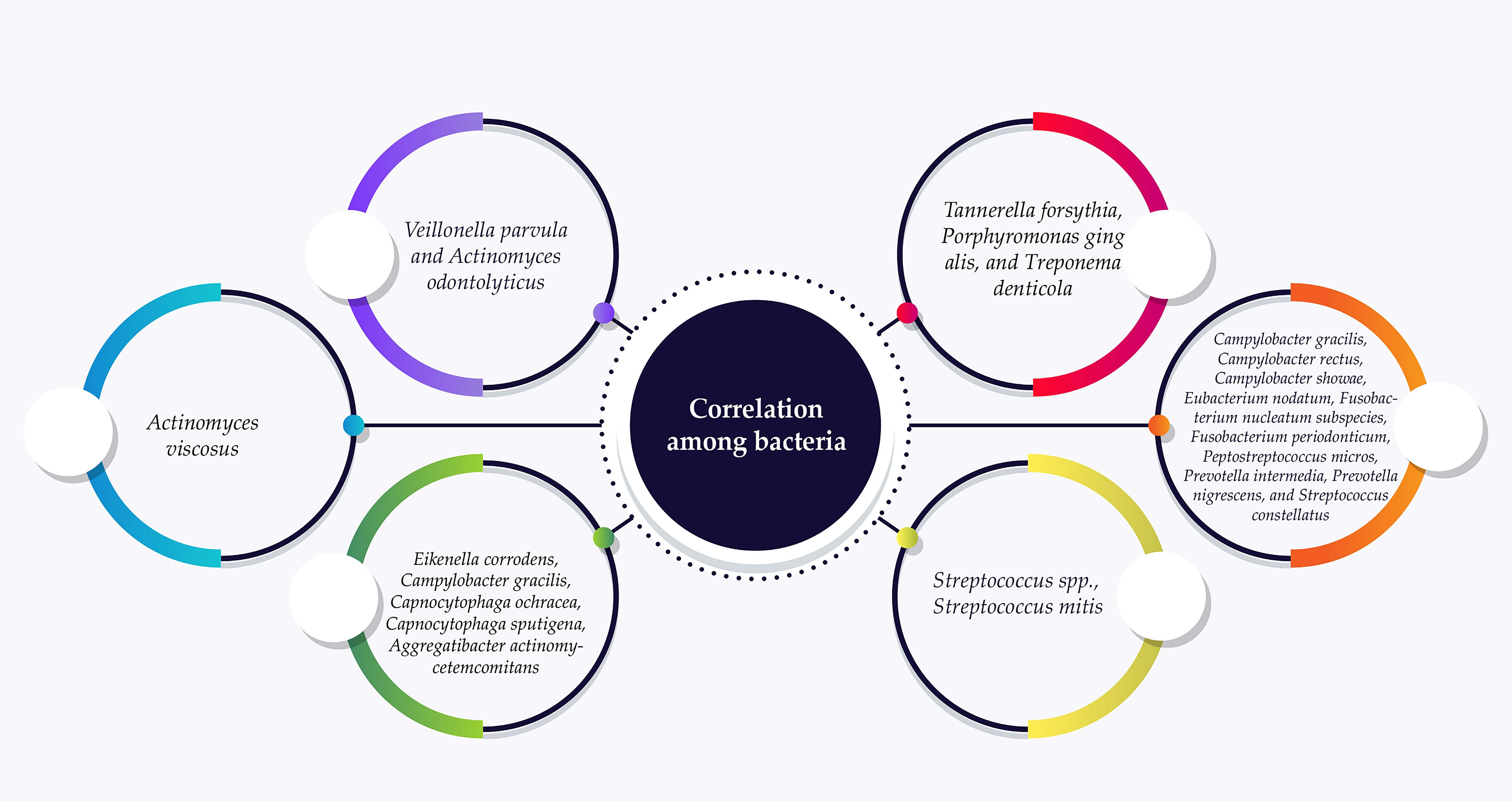
Figure 3. Endodontic-periodontic bacteria commonly assigned in the six complexes according to their association with PD progression. The red complex bacteria are frequently associated with PD and are often detected in deeper spaces near to the epithelial layers of the periodontal pocket.
The presence of the red complex is closely linked to advanced periodontal lesions, which are characterized by deeper pockets and bleeding (Silva and Cascales, 2021). Additionally, the prevalence and quantity of the red complex increase as the depth of these pockets increases (Mohanty et al., 2019). Next-generation sequencing (NGS) technologies have revealed a wider range of diversity within the oral microbiome and have shown an intricate relationship between microbiome composition and periodontal disease states. This includes a correlation between increased microbial diversity and pocket depth. Recent studies have discovered new microbial connections with PD, emphasizing genera such as Schwartzia and Aerococcus as linked to the condition. These findings indicate that the microbial environment of PD is more intricate than previously believed (Sisk-Hackworth et al., 2021). A recently identified bacterium from the Bacteriodetes phylum, known as Candidatus Bacteroides periocalifornicus (CBP), has been discovered in dental plaque and shows a strong association with the pathogenic “red complex” found in deep periodontal pockets. CBP is commonly found in the oral cavities of both healthy and diseased individuals but is not detected in gut or skin samples. The abundance of CBP increases with pocket depth and it coexists with F. nucleatum, T. denticola, and P. gingivalis. Its presence is closely linked to members of the red complex rather than healthy commensals, suggesting that CBP could be a new candidate addition to the symbiotic and pathogenic red complex (Torres et al., 2019).
The blue, yellow, green, and purple complexes consist of bacterial species typically associated with a healthy periodontal environment (Carrouel et al., 2016).
Despite the significant focus on the composition of the human microbiome in recent years, the exact mechanisms by which these microbial communities influence disease and maintain health remain largely unknown. Nonetheless, recent studies have revealed that several chronic conditions affecting the mouth and gastrointestinal tract are linked to changes in the microbiome, known as “dysbiosis.” Dysbiosis refers to a harmful shift in the relative abundances and individual components of the microbiome, which vary during healthy states. This shift leads to major dysbiosis-related diseases in humans, including periodontitis, irritable bowel syndrome, chronic vaginosis, and others. Among these, periodontal disease is a prominent dysbiotic condition due to the diverse genera present in both healthy and periodontal microbiomes (Sudhakara et al., 2018).
Oral microbial dysbiosis is a significant factor in the development of oral diseases, including dental caries and periodontal conditions (Hajishengallis, 2015).
This parasitic or pathogenic state, where microbes cause disease in the host, is known as “dysbiosis “or an “unbalanced microbiome” Inflammation plays a key role in altering the microbial community, leading to a continuous cycle of dysbiosis, immune response, and tissue breakdown. The environmental conditions within inflamed periodontal pockets—such as low oxygen levels, enriched nutrients from host protein breakdown, and increased gingival fluid volume—along with synergistic microbial interactions, create a favorable environment for inflammophilic, anaerobic, proteolytic, and fastidious organisms (Kawamoto et al., 2021).
According to Peterson et al., dysbiosis can be identified through three distinct, yet not mutually exclusive, scenarios that may occur simultaneously: i) a general loss of microbial diversity; ii) the loss of beneficial microbes; and iii) the expansion of pathogenic microbes (Petersen and Round, 2014; Van Dyke et al., 2020) These changes provoke an exaggerated inflammatory response in the host via virulence factors, resulting in tissue destruction (Hajishengallis and Lamont, 2012).
In addition to bacteria, the oral mucosal tissues also harbor to various other microorganisms, including archaea, fungi, and viruses. However, research on the relationship between PD and systemic diseases tends to focus primarily on the bacterial component of the microbiome (Unniachan et al., 2020). Candida albicans is the predominant fungal species observed in both healthy and infected oral cavities, accounting for over 80% of oral fungal isolates (Talapko et al., 2021). It continues to be the leading fungal pathogen identified in individuals with PD (Jabri et al., 2021). Studies have shown that C. albicans can promote the invasion of P. gingivalis into human gingival epithelial and fibroblast cells in vitro (Tamai et al., 2011). This indicates that C.albicans may contribute to the development or worsening of periodontal disease. The hyphal-specific adhesin Als3, found on the surface of the fungal, is believed be essential for its interaction with P. gingivalis. Additionally, pretreating gingival epithelial cells and fibroblasts with C. albicans has been demonstrated to increase the invasion of P. gingivalis (Montelongo-Jauregui and Lopez-Ribot, 2018). Previous research has investigated the role of Candida species in dental caries, revealing that C. albicans significantly contributes to biofilm formation and accumulation (Falsetta et al., 2014; Sztajer et al., 2014). A synergistic relationship between C. albicans and oral bacteria enhances the virulence of polymicrobial biofilms, thereby increasing the resistance of fungal cells to antimicrobial agents and environmental stresses (Bartnicka et al., 2019). Specifically in the context of periodontitis, oxygen consumption by C. albicans appears to create an oxygen-deficient environment that facilitates the growth of P. gingivalis and promotes its ability to invade host cells (Jabri et al., 2022). The pathogenicity of C. albicans is linked to its ability to adhere to various surfaces, form hyphae, produce hydrolytic enzymes, invade host tissues, and trigger inflammatory responses (Cavalcanti et al., 2016).
Viruses are recognized as important contributors to the onset and progression of periodontitis. Among them, herpesviruses—including herpes simplex virus-1 (HSV-1), Epstein–Barr virus (EBV), and cytomegalovirus (CMV)—either individually or in combination with other subgingival pathogens, play a pivotal role in both the initiation and progression of the disease (Abooj and Varma, 2021). Herpesvirus infections have the potential to promote the proliferation of bacterial pathogens within periodontal tissues. Macrophages infected with cytomegalovirus or Epstein–Barr virus exhibit a diminished immune response when confronted with periodontal bacteria. Furthermore, proteins produced by herpesviruses on the surface of infected cells can create new binding sites that facilitate the attachment and growth of periodontopathic bacteria (Slots, 2015).
Within the oral microbiome, archaea represent a minor fraction, predominantly consisting of methanogenic phylotypes—strict anaerobes that produce methane. Notable species include Methanobrevibacter massiliense (M. massiliense), Methanobrevibacter smithii (M. smithii), and Methanobrevibacter oralis (M. oralis), which have been linked to periodontitis (Mosaddad et al., 2019; Pilliol et al., 2024). Methanogens have been identified in subgingival samples collected from patients suffering from periodontitis, peri-implantitis, and infected root canals. They have also been detected in saliva specimens from these individuals (Sogodogo et al., 2019). M. oralis is the most common archaeal phylotype found in the subgingival biofilm of both healthy individuals and those with aggressive periodontitis, indicating that it may be a regular component of the oral microbiota. While Archaea are generally less abundant and diverse in subgingival regions, the higher levels of methanogens seen in individuals with generalized aggressive periodontitis (GAgP) compared to periodontally healthy (PH) individuals suggest their role in the ecological changes of the microbiota associated with aggressive periodontitis (Matarazzo et al., 2011).
Tannerella forsythia
T. forsythia is an anaerobic Gram-negative bacterium belonging to the Porphyromonadaceae family (Posch et al., 2012). It was first discovered in the subgingival periodontal pocket (Miao et al., 2024). T. forsythia is non-motile and has a filamentous cell morphology (Assandri et al., 2023). T. forsythia employs a highly effective strategy similar to “biological warfare” to enhance its pathogenicity. Specifically, its virulence factors are transported as virulent cargo using outer membrane vesicles (OMVs). T. forsythia possess two glycoproteins that play a crucial role in its pathogenicity (Friedrich et al., 2015). T. forsythia expresses various important virulence factors, including the leucine-rich repeat BspA protein, sialidases, surface (S)-layer glycoproteins, and dipeptidyl aminopeptidase IV (Table-1) (Sharma, 2020). S-layer glycosylation in T. forsythia may be associated with reducing Th17 and innate PMN responses, thereby enhancing the pathogen’s survival within the host. T. forsythia also capitalizes on TLR-2-mediated Th2 responses to thrive in its primary ecological niche within periodontal pockets. This adaptation ultimately leads to alveolar bone resorption and expansion of its habitat (Settem et al., 2013). Sakakibara et al. described that the glycosylated S-layer of T. forsythia contributes to the binding and invasion of human epithelial cell-like gingival carcinoma cells (Ca9–22) and KB cells. Therefore, the S-layer of T. forsythia plays a crucial role in the initial stages of PD (Sakakibara et al., 2007). T. forsythia lacks the ability to metabolize sugars. It relies on peptides that are degraded by trypsin-like proteases which are involved in the breakdown of smaller peptides but do not play a central role in bacterial virulence, and PrtH cysteine-like proteases (Sharma, 2010). T. forsythia expresses a family of six multidomain proteases known as KLIKK proteases, which include three serine proteases (mirolase, miropsin-1, and miropsin-2) and three metalloproteases (karilysin, mirolysin, and forsilysin) (Zak et al., 2021). Karilysin has been found to act as a sheddase, facilitating the release of TNF-α from cell surfaces, and also to cleave and inactivate the antimicrobial peptide LL-37 (Skottrup et al., 2023). Increased levels of T. forsythia have been observed in dental plaque samples from post-menopausal women who are obese (Amano et al., 2014), and the bacteria have also been isolated from patients with type 2 diabetes (Miranda et al., 2017). T. forsythia is also associated with systemic diseases such as cardiovascular diseases (Carra et al., 2023), and arthritis (Martínez-Rivera et al., 2017). A recent case control prospective study suggested that T. forsythia is associated with the development of esophageal cancers (Peters et al., 2017), and lung abscesses (Lv et al., 2023).
Porphyromonas gingivalis
P. gingivalis is a black-pigmented, Gram-negative, rod-shaped, immotile, obligate anaerobe and member of the phylum Bacteroidetes. P. gingivalis uses of protein degradation products for providing metabolic energy, heme and vitamin K for its growth (Reyes, 2021; Tanaka et al., 2022). The primary habitat of P. gingivalis is the subgingival crevice, but it can develop into a periodontal pocket during PD. Although P. gingivalis is native to the human oral cavity, it is detectable in only a small percentage of periodontally healthy individuals (Griffen et al., 1998). The most known P. gingivalis virulence factors are fimbriae, hemolysin, hemagglutinins, capsule, OMVs, LPS, and gingipains (Aleksijević et al., 2022). P. gingivalis can infiltrate connective tissues by breaking down the epithelial layers and moving between cells, facilitating the spread of the bacteria and may leading to its entry into the bloodstream (de Jongh et al., 2023). The invasiveness of P. gingivalis relies on fimbriae. A FimA mutant exhibited a reduced ability to invade gingival epithelial and fibroblast cells. After attachment to gingival sulcular epithelial cells, P. gingivalis enters cells and induces remodeling of the actin and tubulin cytoskeleton. Fallowing intracellular proliferation, P. gingivalis invades the epithelial cells through actin-based cytoskeletal rearrangements, mediated by FimA interactions with surface epithelial β1 integrins (Khammissa et al., 2018; Chen et al., 2023). P. gingivalis produces two types of fimbriae, including FimA and Mfa1, which are essential for the bacteria ability to attach to oral Streptococcus spp., other bacteria in dental biofilms, salivary peptides, and host cells. These fimbriae play a vital role in infections and survival within in the host (Kloppsteck et al., 2016).
Remarkably, both fimbriae of P. gingivalis are involved in the invasion of dendritic cells and the induction of adaptive host immune responses (Shahoumi et al., 2023). Gingipains, a group of cysteine proteinases, play a determining role in the pathogenicity of P. gingivalis in PD (Xu et al., 2020). They are primarily secreted by a type IX secretion system (T9SS) expressed on the outer membranes and OMVs of most P. gingivalis strains or occasionally released into the extracellular milieu as soluble proteins by some P. gingivalis strains (HG66) (Ciaston et al., 2022). So far, three types of gingipains have been described in P. gingivalis including lysine-specific gingipain (Kgp), arginine-specific gingipain A (RgpA), and arginine-specific gingipain B (RgpB). In addition to their function as proteolytic enzymes for breaking down proteinaceous nutrients to support bacterial growth, gingipains also play an essential role in processing fimbria proteins and in bacterial adhesion and colonization of the host (Singh and Singh, 2022). Gingipains produced by P. gingivalis break down C3 into C3a and cleave C5 into the bioactive C5a of the complement system. This process increases proinflammatory cytokines, suppresses the caspase 11-dependent non-canonical inflammasome pathway, and inhibits cell apoptosis, allowing P. gingivalis to utilize host cells for its survival (Chopra et al., 2020). The trypsin-like activity of gingipains enables P. gingivalis to cleave a variety of host proteins including immunity mediators, blood proteins, ECM proteins, and host cell surface proteins (Li and Collyer, 2011).
The capsule of P. gingivalis enhances its pathogenicity by allowing it to evade immune cell recognition and increasing its tolerance to phagocytosis (Sharaf and Hijazi, 2022). P. gingivalis primarily acquires heme through mechanisms involving hemagglutinin, hemolysin, and gingipains (Śmiga et al., 2023).
The association of P. gingivalis has been described with the development of various gastrointestinal cancers (Tan et al., 2022), pancreatic cancer (Gnanasekaran et al., 2020), atherosclerosis (Zhang et al., 2021), Alzheimer’s disease (Ryder, 2020), rheumatoid arthritis (Li et al., 2022), bacterial vaginosis (Fiorillo et al., 2019), preterm birth (Udagawa et al., 2018), respiratory tract infections (Bregaint et al., 2022), and type 2 diabetes (Tian et al., 2020).
Treponema denticola
Treponema denticola is a Gram-negative, spirochete and anaerobic bacterium commonly detected in CP lesions, often along with P. gingivalis and T. forsythia (Dashper et al., 2019). The principal virulence determinants of T. denticola are found within the toxin–antitoxin (TA) system, specifically transposases (Kuboniwa et al., 2012). These include outer sheath proteins, particularly the major outer sheath protein (MOSP) (Puthenveetil et al., 2017), trypsin-like protease activity (Usui et al., 2022), lipoproteins (Goetting-Minesky et al., 2021), and dentilisin (Ganther et al., 2021). The motility of T. denticola plays a crucial role in its ability to penetrate oral epithelial cell layers and is closely tied to its virulence. Like to other spirochetes, the flagella in T. denticola are internal and located between the protoplasmic cylinder and the outer sheath. This internal positioning enables the flagella to rotate the cell, facilitating motility. The internal flagella motility helps spirochetes penetrate more viscous layers more effectively than microorganisms with external flagella (Nakamura et al., 2006). This ability plays a crucial role in the colonization of T. denticola through biofilm formation (Ng et al., 2019). T. denticola activates TLR2/MyD88, leading to the nuclear translocation of the transcription factor Sp1, which is a key regulator of T. denticola-dependent MMP transcription. T. denticola produces dentilisin, which promotes tissue-destructive cellular processes through a TLR2/MyD88/Sp1-dependent mechanism (Ganther et al., 2021). Dentilisin is a surface protease, that degrades host proteins such as transferrin, fibrinogen, gelatin, fibronectin, and laminin. It exhibits a cytopathic effect on human epithelial cells and is involved in fibrinogen binding (Kokubu et al., 2021). Additionally, it mediates adherence to fibronectin and plays a role in immunomodulation (Arai et al., 2020). It has been described that T. denticola can infiltrate the brain and directly influence nerve cells, potentially contributing to the deposition of Aβ and the subsequent pathological progression of Alzheimer’s disease (Su et al., 2021).
Aggregatibacter actinomycetemcomitans
A. actinomycetemcomitans is a non-motile, facultative anaerobic or capnophilic, Gram-negative coccobacilli bacterium. It grows well in microaerophilic conditions of 5–10% CO2 and plays a primary role in the development of PD. It is also linked to AgPD, which can lead to premature tooth loss in adolescents. Its involvement in gingival dysbiosis during the early stages of PD is crucial. A. actinomycetemcomitans harbors a 14-gene operon, known as the widespread colonization island, housing the tight adherence (tad) genes. This operon is associated with strong biofilm formation capabilities and robust attachment to enamel-like substrates in vitro (Velusamy et al., 2019; Fine et al., 2020; Isola et al., 2020). A. actinomycetemcomitans colonizes the oral cavity by adhering to gingival epithelial cells through both fimbria andnon-fimbria- dependent adhesins, including Aae. Additionally, it contributes to the formation of dental biofilms (Ishikawa et al., 2021). Both fimbriae and nonfimbrial adhesins can either specifically target a particular substrate or show affinity to several different cellular targets. In addition to their role in biofilm formation, they play an important role in the invasion of host cells (Danforth et al., 2021). A. actinomycetemcomitans can damage human white blood cells by producing exotoxins, including leukotoxin (LtxA) and the cytolethal distending toxin (CDT) (Khzam et al., 2024). CDT is a genotoxin that disrupts the host cell cycle, kills host cells and contributes to tissue destruction. CDT consists of three subunits including CdtA, CdtB, and CdtC. The active subunit, CdtB, possesses DNase activity and infiltrates host cells, causing DNA damage. This mechanism aligns with its role in the pathogenesis of PD (DiRienzo, 2014). LtxA, an important virulence determinant, specifically targets leukocytes and triggers rapid caspase-1 activation, leading to substantial IL-1β secretion in human monocytes and macrophages. The NLRP and AIM2 inflammasomes, along with ROS and cathepsin B, likely participate in this process (Cheng et al., 2020). LtxA also triggers PMN degranulation and the extracellular release of proteolytic enzymes such as elastase and MMPs, which cause host cell death by inhibiting cell proliferation and enhancing the expression of receptor activator of RANKL, a key factor in osteoclastogenesis (Åberg et al., 2015). A. actinomycetemcomitans is the only pathogen associated with CPD capable of synthesizing LtxA. This toxin mimics the citrullinated pattern observed in joints affected by rheumatoid arthritis (RA) by activating P. gingivalis peptidylarginine deiminase and inducing hypercitrullination in neutrophils through pore formation (Huang and Ni, 2024).
A. actinomycetemcomitans employs various strategies to survive within multispecies biofilms. One such strategy is its ability to regulate catalase production, which helps break down hydrogen peroxide (H2O2) produced by other organisms such as Streptococci. Another strategy involves the production of dispersin B, which aids in releasing of A. actinomycetemcomitans cells from the biofilm matrix (Stacy et al., 2014). Six distinct serotypes of A. actinomycetemcomitans have been characterized based on LPS antigenicity. Among these, serotype C is the most commonly isolated from patients with PD (Shahoumi et al., 2023). A. actinomycetemcomitans employs several strategies to evade the host defense, including hindering leukocyte chemotaxis, producing immunosuppressive factors, secreting IgG-cleaving proteases, and synthesizing Fc-binding proteins (Bapat et al., 2022). In addition to PD, A. actinomycetemcomitans may contribute to the development and progression of systemic conditions, including infective endocarditis, bacteremia, meningitis, and skin infections (Nørskov-Lauritsen et al., 2019).
Fusobacterium nucleatum
Fusobacterium nucleatum is an obligate anaerobic, Gram-negative, rod-shape bacterium belongs to the genus Fusobacterium. It is named for its slender appearance and spindle-like tips at both ends. F. nucleatum often found as a resident in various parts of the human body microbiota, especially in the oral cavity (Ghosh et al., 2024). It is present in small quantities in healthy subgingival dental biofilm but becomes more abundant in periodontal pockets (Jung et al., 2017). F. nucleatum plays a crucial structural role in connecting early and late colonizers of dental plaque. Recent studies have shown that the cross-feeding and trophic interactions between F. nucleatum and early-colonizing microorganisms can impact biofilm formation and the prolifertion of later colonizing pathogens, such as P. gingivalis (Baker et al., 2024). F. nucleatum pathogenesis is primarily associated with several virulence determinants including adhesins on its surface, such as RadD, Aid1, and FomA, which can co-aggregate bacteria to facilitate biofilm formation (Chen et al., 2022a). Fusobacterium adhesin A (FadA) is the only adhesin expressed by F. nucleatum that binds to host cells. FadA exists in two forms: non-secreted, intact pre-FadA, and secreted, mature FadA (mFadA). Through its interactions with host cells, FadA induces tumorigenic responses and facilitates bacterial invasion (Groeger et al., 2022). F. nucleatum can invade various cell lines, including oral, colonic, placental epithelial cells, immune cells, and keratinocytes. Once internalized, F. nucleatum induces the expression of specific cytokines, regulates cell proliferation and apoptosis, and other biological behaviors, ultimately leading to epithelial cell dysfunction and destruction of periodontal tissue (Zhang et al., 2022). LPS is another virulence factor of F. nucleatum that plays a crucial role in the production of epithelial cytokines by activating TLR-4 (Engevik et al., 2021). F. nucleatum secretes a serine protease that not only meets its nutritional needs but also damages host tissues. This enzyme degrades ESM proteins, leading to the breakdown of periodontal connective tissues, immunoglobulins, and complement proteins in the host immune system. Specifically, it cleaves the α-chain of IgA, supporting F. nucleatum in evading the host’s immune system (De Andrade et al., 2019). Butyric acid, a short-chain fatty acid (SCFA) produced by F. nucleatum, can influence the damage and healing of periodontal tissues. Elevated levels of butyric acid can increase ROS formation in osteoblasts, which in turn stimulates the secretion of 8-isoprostaglandin and MMP-2. This process leads to bone destruction and impairs bone repair (Chen et al., 2022a). P. gingivalis is sensitive to acidic conditions. However, F. nucleatum can ferment glutamate and aspartate to produce ammonia, creating a more neutral environment that supports the colonization of P. gingivalis (McIlvanna et al., 2021). In addition to PD, the potential role of F. nucleatum has been described in a variety of diseases, such as, endodontic infections, gingivitis, tonsillitis, head and neck tumor, appendicitis, inflammatory bowel diseases, gastrointestinal tumor, endocarditis, atherosclerosis, respiratory tract infections, abscesses, bone infections, and adverse pregnancy outcomes (including preterm labor, stillbirth, and chorioamnionitis), as well as urinary tract infections (Brennan and Garrett, 2019).
Prevotella spp.
Prevotella are Gram-negative, obligate anaerobes typically found in the human vaginal microbiota, gastrointestinal tract, respiratory tract, and oral cavity (Larsen, 2017). These species are characterized as non-motile, non-spore-forming rods, with colony colors ranging from shiny white to black (George et al., 2024). Periodontal pathogens from the Prevotella genus including P. intermedia, P. melaninogenica, P. nigrescens, P. denticola, P. corporis, and P. disiens have been identified (Arora et al., 2014). P. intermedia, a black-pigmented anaerobic rod that possesses various virulence factors such as adhesion, hemolysin, hemagglutinin, proteolytic and hydrolytic enzymes. These factors allow P. intermedia to establish itself in the oral cavity, evade and modulate the hosts immune defenses, and cause tissue damage (Eley and Cox, 2003). Additionally, P. intermedia has been shown to induce the expression of pro-MMP-2 and pro-MMP-9 in fetal mouse osteoblasts (Pelt et al., 2002).
Additionally, it has been shown that P. intermedia can stimulate the production of MMP-9 in human periodontal ligament (hPDL) cells (Guan et al., 2008). It upregulates the mRNA expression and protein secretion of MMP-1 and MMP-8 through MAPK signaling pathways and PGE2 synthesis in these cells. This suggests that P. intermedia may play a role in the degradation of periodontal connective tissue and bone matrix during CP by increasing the expression of multiple MMPs (Guan et al., 2009).
Campylobacter gracilis
Campylobacter gracilis, formerly known as Bacteroides gracilis, was first identified in patients with gingivitis and PD (Tanner et al., 1981). C. gracilis is an anaerobic Gram-negative rod shaped bacterium that is non-motile and non-spore-forming. The type strain of C. gracilis type strain possesses multiple potential virulence factors, such as hemagglutinins, toxins, immunity proteins, and other predicted factors. Furthermore, the genomic island containing zonula occludens toxin (zot) has been found in this strain (Miller and Yee, 2015). It primarily inhabits the gingival sulcus and is known for its contribution to the progression of PD. While its involvement in PD is well established, systemic infections caused by C. gracilis are extremely rare, with only a few cases reported in the medical literature (Arakawa et al., 2024). C. gracilis has been linked to various diseases, such as Crohn’s disease in children and ulcerative colitis in adults (Man, 2011).
Veillonella parvula
Veillonella parvula is an anaerobic, small and nonmotile Gram-negative coccus that is part of the human normal flora. Although Veillonella spp. are generally considered commensal microorganisms, they have occasionally been implicated in infections, particularly in immunocompromised individuals (Gouze et al., 2019). Veillonella spp. are typically present in biofilms and often co-aggregate with lactic acid bacteria (Rojas-Tapias et al., 2022). V. parvula is an important early colonizer of dental plaque, aiding in the biofilm’s formation. It promotes the growth of multiple species and plays a crucial role in the community’s metabolism by consuming lactic acid (Béchon et al., 2020). Despite its role in biofilms, V. parvula is also known as an opportunistic pathogen associated with various infections such as osteomyelitis, endocarditis, spondylodiscitis, abscesses, and systemic infections (Hirai et al., 2016; Li et al., 2017; Stefani et al., 2017; Hyo et al., 2019; Wellens et al., 2019). V. parvula does not ferment carbohydrates instead, it relies on lactate produced by Streptococci as its carbon source for growth. In dual-species biofilms, V. parvula has been shown to enhance the growth and extracellular polysaccharide (EPS) synthesis of S. mutans (Liu et al., 2020). Delwiche et al (Delwiche et al., 1985). described that Veillonella spp. generate significant amounts of LPS. Additionally, V. parvula LPS induces cytokine production and activates p38 MAPK dependent on TLR-4 (Matera et al., 2009). These characteristics of Veillonella SPP. are believed to complicate the treatment of V. parvula-associated PD (Mashima et al., 2016). Veillonella are regarded as a bridging species because of its capacity to modify the microbiome environment. The catalase produced by V. parvula can provide a more desirable low redox potential for oxygen-sensitive anaerobes, thereby enhancing the potential of pathogenic microorganisms to thrive in the oral microbiome (Garcia, 2022).
Treatment of periodontitis
The successful treatment of an infection relies on accurately diagnosing of the pathogens contributing to its etiopathogenesis. Diagnosing an infection can be challenging, especially in patients with a polymicrobial infection in organs are naturally colonized by bacteria, like the skin, gastrointestinal tract and oral cavity. Methodological problems in detecting the complex subgingival microbial population, which is heavily colonized by numerous species of strict and facultative anaerobes and fastidious bacteria, have significantly hindered the accurate diagnosis and antibiotic therapy of PD (Socransky and Haffajee, 1994). The treatment of PD should be started as soon as possible. Mild to moderate cases of PD are typically treated using nonsurgical procedures, such as supplementary antimicrobial agents, dental scaling, and root planning (Slots, 2012; Albandar, 2014). Nonsurgical treatments are typically not enough for sever PD. Surgical procedures are necessary to reduce pocket depth and establish anatomical contours at the periodontal junction (Yang et al., 2021). Systemic antimicrobial drugs are administered as monotherapy or in combination therapy. However, it is highly recommended to combine antibiotics with non-surgical periodontal therapy to achieve optimal clinical outcomes (Souza et al., 2020). The most common antibiotic regimens for PD include β-lactams, tetracyclines, quinolones and metronidazole (Keestra et al., 2015). The combination of metronidazole and amoxicillin is the most frequently used antimicrobial therapy for PD (Elashiry et al., 2021). This combination has been shown to have synergic effects, reducing the necessary levels of both antibiotics for biological effects (Mugri, 2022). A 7-day regimen of 500/500 mg or 500/400 mg of amoxicillin and metronidazole is recommended for PD (McGowan et al., 2018).
Azithromycin is a macrolide agent generally administer as an alternative in case of penicillin-allergy. The suggested regimen for azithromycin is 500 mg/day for 3 days and it an assists in non-surgical procedures and mechanical plaque elimination in the treatment of PD (Jones and Hoyle, 2022). Macrolides should not be combine with clindamycin, due to their similar cellular target and antagonistic effects (Flynn, 2019).
Clindamycin is a lincosamide agent that has a bacteriostatic effect on anaerobic bacteria and is highly effective against mixed infections caused by both anaerobic and aerobic bacteria. It has favorable pharmacokinetics and has become a commonly prescribed alternative for oral infections in patients with penicillin allergy. The common oral dosage of clindamycin is 300 mg every 6 h (Jeske, 2024). Clindamycin is the only antimicrobial drug that reduces the attachment of bacteria to epithelial cells on the mucosal surface by decreasing the expression of microbial factors. It also decreases the production of proinflammatory cytokines, such as TNF-α and IL-1β, which can lead to additional destruction of periodontal tissues, when overexpressed by microorganisms and neighboring cells. Therefore, the decreased release of TNF-α and chemokine CXCL-1 are additional effects of clindamycin, which help to suppress the inflammatory condition such as PD (Luchian et al., 2021).
Tetracycline derivatives are generally administer as supportive agents in PD antimicrobial therapy (Mohammad et al., 2022). Tetracycline has a long shelf-life, which preserve its antibacterial effects for an extended period, and is slowly released from the tooth surface (Kafle et al., 2018).
Tetracycline also possesses a surprising capacity to prevent host-derived MMP effects and connective tissue damage decreases the release of inflammatory mediators, and lead to the concept of host-modulation therapy in the treatment of PD. Upregulated collagen synthesis, osteoblast activity, and bone formation are non-antimicrobial properties of tetracycline, making it an appropriate option for PD treatment (Golub and Lee, 2020).
Ciprofloxacin is a broad-spectrum antibiotic that is effective against various pathogens -associated PD, including A. actinomycetemcomitans. It efficiently penetrated the infected periodontal tissues and can reach higher levels in the crevicular fluid compared to the blood.
Because periodontal infections are commonly polymicrobial and caused by a variety of periodontal pathogens, a combination of antibiotics is often used to treat AgPD (Bidault et al., 2007).
Moxifloxacin, another quinolone, has shown significant antimicrobial effects on PD-associated pathogens in vitro and in a clinical study involving AgPD cases. It has been shown that daily 400 mg of moxifloxacin, used in conjunction with one-stage full-mouth cleaning and root planning improved clinical outcomes compared to mechanical treatment alone in AgPD cases (Ardila et al., 2015). However, moxifloxacin is not typically administered as the first-line drug due to its high cost, and is usually reserved for case where first-line antimicrobials and surgical procedures have been unsuccessful (Holmes and Pellecchia, 2016).
Alternative treatments of periodontitis: over antibiotics and surgical procedures
Understanding the role of oral microbiota dysbiosis in the initiation and progression of PD is crucial for devising effective therapeutic strategies aimed at reducing bacterial load and restoring microbiological balance (Sachelarie et al., 2025). Conventional treatments for periodontitis often don’t completely eliminate harmful pathogens (Matsubara et al., 2016). However, when executed meticulously, these treatments can promote a healthier oral environment by modifying the composition and population of the microbial community and aiding in the maturation of the host immune response (Fragkioudakis et al., 2021). The primary methods for treating periodontitis include controlling biofilms, mechanically removing plaque, and using antibiotic therapy (Slots, 2017). In recent years, the main goal of these treatments has evolved to focus on restoring homeostasis within the oral microbiota. However, bacterial strains involved in PDs are increasingly becoming resistant to antibiotics, posing a significant challenge to conventional treatments (Haque et al., 2022). Consequently, there has been a growing focus on complementary approaches to enhance traditional mechanical treatments. Among these, probiotics have gained attention from researchers and clinicians for their potential to help restore microbial balance. Probiotics produce compounds such as lactic acid, hydrogen peroxide, and bacteriocins, which can reduce pathogenic bacterial biofilms and decrease levels of pro-inflammatory factors like cytokines, collagenases, elastases, and prostaglandin E2. These probiotic bacteria then adhere more firmly to the oral cavity’s surface, preventing new pathogens from colonizing. Through this process, bacterial aggregation and co-aggregation occur, leading to a new microbial balance that forms a healthy biofilm (Mishra et al., 2020). The incorporation of probiotics in periodontal treatment represents a promising innovation with the potential to significantly enhance long-term clinical results. While phage therapy has demonstrated efficacy against numerous infections, its application in the treatment of oral diseases remains underexplored.
Bacteriophage therapy offers a novel and promising alternative method of treatment (Shlezinger et al., 2017). Bacteriophages, also known as phages, are viruses that infect bacteria by entering the host cell and initiating a cycle of phage production. They play a crucial role in the infectious cycle of lytic phages, ultimately leading to the lysis or death of the bacterial host (Zhu et al., 2025). Phages, which are highly selective, non-toxic, self-replicating, and capable of infiltrating biofilms, offer a novel alternative to traditional biofilm prevention techniques (Szafrański et al., 2017). Their ability to combat dental plaque is highlighted by their small size, allowing them to penetrate biofilm layers with high efficiency. Phage therapy could become a crucial treatment option for root canal infections that are resistant to conventional endodontic methods and has shown effectiveness against root canal infections caused by Enterococcus faecalis, a foodborne pathogen associated with various diseases and potentially implicated in periodontal health (Łasica et al., 2024; Hosseini Hooshiar et al., 2024).
Over the past century, lasers have been used to treat variety of diseases, including periodontitis. A laser device generates electromagnetic radiation at a specific wavelength and a low-intensity beam, which has significant effects on tissues. The use of lasers is considered beneficial for treating a range of infectious and inflammatory conditions (Isola et al., 2021). Several studies have shown that lasers can promote periodontal wound healing and regeneration by effectively removing and decontaminating diseased tissues, as well as by modulating or activating cell metabolism in the surrounding tissues. In the past decade, low-intensity diode lasers have been used in combination with photosensitizers to activate topical photosensitizing agents such as antimicrobial photodynamic therapy (aPDT), to help reduce or eliminate periodontopathogen bacteria as a complement to mechanical debridement in patients with periodontitis (Chambrone et al., 2018). Photodynamic therapy (PDT) is a non-thermal photochemical reaction that requires the simultaneous presence of visible light, oxygen, and a dye or photosensitizer (PS). Various PS have been studied for their ability to bind to bacteria and effectively produce reactive oxygen species (ROS) when exposed to light. These ROS are generated through type I or II mechanisms and can deactivate various types of bacterial cells (Sperandio F et al., 2013). The most common laser applications for periodontal therapy include diode, carbon dioxide (CO2), and neodymium-doped lasers. These wavelengths can be used in conjunction with mechanical non-surgical instrumentation to debride connective tissue and epithelium within periodontal pockets, inactivate bacteria, and ablate subgingival calculus (Salvi et al., 2020).
Various local and systemic approaches have been employed to effectively treat periodontitis. Currently, controlled local drug delivery is more favored compared to systemic methods. This is because it focuses on enhancing therapeutic outcomes by achieving factors such as site-specific delivery, low dose requirements, bypassing first-pass metabolism, reducing gastrointestinal side effects, and decreasing dosing frequency (Joshi et al., 2016). Selecting the right antimicrobial agent with the appropriate route of administration is crucial for successful periodontal therapy. Local drug delivery systems (LDDS) such as irrigating systems, fibers, gels, strips, films, microparticles, nanoparticles, and low-dose antimicrobial agents are available to deliver antimicrobial agents to sub-gingival diseased sites with minimal or no side effects on other body sites (Rajeshwari et al., 2019).
Antimicrobial resistance
Several studies have revealed that levels of resistance to certain antibiotics increased have in microbial agents involved PD. The most significant factor contributing to the rise in antimicrobial resistance is the misuse or overuse of these agents. Resistance to metronidazole in PD- associated pathogens has been reported in several studies (Mínguez et al., 2019). Higher levels of metronidazole resistance have been reported in A. actinomycetemcomitans compared to P. gingivalis. The resistance frequency of P. gingivalis strains from Colombia to amoxicillin, azithromycin, and metronidazole has been reported as 24.6%, 21.3% and 24.6%, respectively (Ardila and Bedoya-García, 2020). However, some studies have described the sensitivity of all P. gingivalis strains to all or most tested antimicrobial agents (Mínguez et al., 2019; Rams et al., 2023).
Some β-lactamase-producing pathogens may contribute to refractory PD. Since PDs commonly are polymicrobial, the presence of β-lactamase-producing pathogens may prevent nonproducing organisms in subgingival plaques from being affected by β-lactam agents, leading to treatment failure or disease recurrence (Handal et al., 2004). A significant increase in resistance to clindamycin and amoxicillin has been reported over a 20-year period among P. gingivalis isolated from severe PD patients in the United States (Rams et al., 2023).
Choosing the appropriate antibiotics for PD is a complex and challenging due to the presence of over 700 bacterial species in the oral cavity (Radaic and Kapila, 2021). On the other hand, selecting an antimicrobial agent for PD treatment based on microbiological analysis and antibiotic susceptibility pattern is practically impossible. There are few guidelines on how to determine and interpret antimicrobial resistance patterns of anaerobic oral bacteria because there is inadequate information on the relationship between minimal inhibitory concentrations (MIC) of antibiotics, actual local (oral) levels in vivo (PK/PD data) and clinical outcomes (Jepsen et al., 2021). On the other hand, most of the bacteria that cause PD are fastidious, requiring complex culture media and nutritional supplements for in vitro growth, as well as a long incubation period.
It is well known that antibiotic resistance varies between countries based on their usage patterns and levels of antibiotic administration in general clinical settings (Van Winkelhoff et al., 2005). Antimicrobial drugs are prescribed empirically based on the periodic antibiotic sensitivity patterns reported by national researches (Luan et al., 2023). Undoubtedly, prospective clinical and surveillance studies, as well as monitoring the resistance levels to antibiotics will be effective in the optimal usage of antibiotics and control of drug-resistant strains.
Conclusion
The microbial-biofilm formation and dental plaque is the initial stage of PD. The extended presence of plaque on the teeth’s surface causes it to migrate into the surrounding periodontal tissues. This spread triggers the infiltration of host immune cells, leading to inflammation of the gingival tissue and ultimately bone destruction. The damage and injuries caused by PD result from immune system mediators and virulence factors produced by pathogenic bacteria. These effects are primarily due to interactions, co-aggregation, and metabolic dependencies among the pathogens. Therefore, prevention of dental plaques and antimicrobial therapy can have a significant impact on prevention and treatment of PD. The prospective clinical and surveillance studies, as well as monitoring the resistance levels to antibiotics will be effective in the optimal usage of antibiotics and control of drug-resistant pathogens caused PD. Novel approaches like NGS and OMICS-based methods can be used to more accurately study the microbiology and pathology of PD. Understanding the role and effects of bacterial secondary metabolites, such as short-chain fatty acids, produced by the microbiome in the prognosis and pathogenesis of PD provides valuable data the prevention and the development of new therapeutic and diagnostic methods. Because PD is polymicrobial and there is increasing antibiotic resistance among its pathogens, there is an urgent need to update laboratory and clinical guidelines and assess precise and reliable methods for detecting resistant strains.
Author contributions
MY: Conceptualization, Methodology, Software, Writing – original draft, Writing – review & editing. MD: Investigation, Software, Writing – original draft, Writing – review & editing. SF: Methodology, Writing – original draft, Writing – review & editing. SS: Investigation, Writing – original draft, Writing – review & editing. SM: Investigation, Methodology, Validation, Writing – original draft, Writing – review & editing. MYM: Conceptualization, Investigation, Methodology, Supervision, Validation, Writing – original draft, Writing – review & editing.
Funding
The author(s) declare that financial support was received for the research and/or publication of this article. This project was supported by the Infectious and Tropical Diseases Research Center and Students Research Committee, Tabriz University of Medical Sciences.
Conflict of interest
The authors declare that the research was conducted in the absence of any commercial or financial relationships that could be construed as a potential conflict of interest.
Generative AI statement
The author(s) declare that no Generative AI was used in the creation of this manuscript.
Publisher’s note
All claims expressed in this article are solely those of the authors and do not necessarily represent those of their affiliated organizations, or those of the publisher, the editors and the reviewers. Any product that may be evaluated in this article, or claim that may be made by its manufacturer, is not guaranteed or endorsed by the publisher.
References
Abdullameer, M. A., Abdulkareem, A. A. (2023). Diagnostic potential of salivary interleukin-17, RANKL, and OPG to differentiate between periodontal health and disease and discriminate stabl e and unstabl e periodontitis: A case-control study. Health Sci. Reports. 6, :e1103. doi: 10.1002/hsr2.1103
Åberg, C., Kelk, P., Johansson, A. (2015). Aggregatibacter actinomycetemcomitans: virulence of its leukotoxin and association with aggressive periodontitis. Virulence. 6, 188–195. doi: 10.4161/21505594.2014.982428
Abooj, J., Varma, S. A. (2021). Prevalence of herpes virus in chronic periodontitis patients with and without type 2 diabetes mellitus: A clinico-microbiological study. J. Maxillofacial Pathology. 25, 141–146. doi: 10.4103/jomfp.JOMFP_154_20
Albandar, J. M. (2014). Aggressive and acute periodontal diseases. Periodontology 2000. 65, 7–12. doi: 10.1111/prd.2014.65.issue-1
Aleksijević, L. H., Aleksijević, M., Škrlec, I., Šram, M., Šram, M., Talapko, J. (2022). Porphyromonas gingivalis virulence factors and clinical significance in periodontal disease and coronary artery diseases. Pathogens. 11, 1173. doi: 10.3390/pathogens11101173
Alfakry, H., Malle, E., Koyani, C. N., Pussinen, P. J., Sorsa, T. (2016). Neutrophil proteolytic activation cascades: a possible mechanistic link between chronic periodontitis and coronary heart disease. Innate Immunity. 22, 85–99. doi: 10.1177/1753425915617521
Amano, A., Chen, C., Honma, K., Li, C., Settem, R., Sharma, A. (2014). Genetic characteristics and pathogenic mechanisms of periodontal pathogens. Adv. Dental Res. 26, 15–22. doi: 10.1177/0022034514526237
Arai, Y., Kikuchi, Y., Okamoto-Shibayama, K., Kokubu, E., Shintani, S., Ishihara, K. (2020). Investigation of the potential regulator proteins associated with the expression of major surface protein and dentilisin in Treponema denticola. J. microbiology. 12, 1829404. doi: 10.1080/20002297.2020.1829404
Arakawa, Y., Yagi, Y., Mimoto, A., Nishida, Y., Kuwana, S., Nakai, E., et al. (2024). Management of a large cerebral abscess in children caused by campylobacter gracilis: A case report and review of the literature. Cureus 16:e62744. doi: 10.7759/cureus.62744
Ardila, C.-M., Bedoya-García, J.-A. (2020). Antimicrobial resistance of Aggregatibacter actinomycetemcomitans, Porphyromonas gingivalis and Tannerella forsythia in periodontitis patients. J. Global Antimicrobial Resistance. 22, 215–218. doi: 10.1016/j.jgar.2020.02.024
Ardila, C. M., Martelo-Cadavid, J. F., Boderth-Acosta, G., Ariza-Garcés, A. A., Guzmán, I. C. (2015). Adjunctive moxifloxacin in the treatment of generalized aggressive periodontitis patients: clinical and microbiological results of a randomized, triple-blind and placebo-controlled clinical trial. J. Clin. Periodontology. 42, 160–168. doi: 10.1111/jcpe.2015.42.issue-2
Armitage, G. C. (2004). The complete periodontal examination. Periodontology 2000. 34, 22–33. doi: 10.1046/j.0906-6713.2002.003422.x
Armitage, G. C., Cullinan, M. P. (2010). Comparison of the clinical features of chronic and aggressive periodontitis. Periodontology 2000. 53:12–27. doi: 10.1111/j.1600-0757.2010.00353.x
Arora, N., Mishra, A., Chugh, S. (2014). Microbial role in periodontitis: Have we reached the top? Some unsung bacteria other than red complex. J. Indian Soc. Periodontology. 18, 9–13. doi: 10.4103/0972-124X.128192
Asakawa, R., Komatsuzawa, H., Kawai, T., Yamada, S., Goncalves, R. B., Izumi, S., et al. (2003). Outer membrane protein 100, a versatile virulence factor of Actinobacillus actinomycetemcomitans. Mol. microbiology. 50, 1125–1139. doi: 10.1046/j.1365-2958.2003.03748.x
Assandri, M. H., Malamud, M., Trejo, F. M., de los A Serradell, M. (2023). S-layer proteins as immune players: Tales from pathogenic and non-pathogenic bacteria. Curr. Res. Microbial Sci. 4, 100187. doi: 10.1016/j.crmicr.2023.100187
Baker, J. L., Mark Welch, J. L., Kauffman, K. M., McLean, J. S., He, X. (2024). The oral microbiome: diversity, biogeography and human health. Nat. Rev. Microbiology. 22, 89–104. doi: 10.1038/s41579-023-00963-6
Bapat, A., Lucey, O., Eckersley, M., Ciesielczuk, H., Ranasinghe, S., Lambourne, J. (2022). Invasive Aggregatibacter infection: shedding light on a rare pathogen in a retrospective cohort analysis. J. Med. Microbiology. 71, 001612. doi: 10.1099/jmm.0.001612
Barnier, J.-P., Meyer, J., Kolappan, S., Bouzinba-Ségard, H., Gesbert, G., Jamet, A., et al. (2021). The minor pilin PilV provides a conserved adhesion site throughout the antigenically variable meningococcal type IV pilus. Proc. Natl. Acad. Sci. 118, e2109364118. doi: 10.1073/pnas.2109364118
Bartnicka, D., Karkowska-Kuleta, J., Zawrotniak, M., Satała, D., Michalik, K., Zielinska, G., et al. (2019). Adhesive protein-mediated cross-talk between Candida albicans and Porphyromonas gingivalis in dual species biofilm protects the anaerobic bacterium in unfavorable oxic environment. Sci. Reports. 9, 4376. doi: 10.1038/s41598-019-40771-8
Becerra−Ruiz, J. S., Guerrero−Velázquez, C., Martínez-Esquivias, F., Martínez-Pérez, LA, Guzmán−Flores, J. M. (2022). Innate and adaptive immunity of periodontal disease. From etiology to alveolar bone loss diseases. Oral. Dis. 28, 1441–1447. doi: 10.1111/odi.13884
Béchon, N., Jiménez-Fernández, A., Witwinowski, J., Bierque, E., Taib, N., Cokelaer, T., et al. (2020). Autotransporters drive biofilm formation and autoaggregation in the diderm firmicute Veillonella parvula. J. Bacteriology. 202, e00461-20. doi: 10.1128/jb.00461-20
Bidault, P., Chandad, F., Grenier, D. (2007). Systemic antibiotic therapy in the treatment of periodontitis. J. Can. Dental Association. 73, 515–20.
Bîrluţiu, R. M., Bîrluţiu, V., Cismasiu, R. S., Mihalache, P., Mihalache, M. (2017). Bacterial biofilm: a mini-review of an emerging life form of bacteria. Acta Med. Transilvanica. 22, 68–71.
Borilova Linhartova, P., Danek, Z., Deissova, T., Hromcik, F., Lipovy, B., Szaraz, D., et al. (2020). Interleukin gene variability and periodontal bacteria in patients with generalized aggressive form of periodontitis. Int. J. Mol. Sci. 21, 4728. doi: 10.3390/ijms21134728
Bregaint, S., Boyer, E., Fong, S. B., Meuric, V., Bonnaure-Mallet, M., Jolivet-Gougeon, A. (2022). Porphyromonas gingivalis outside the oral cavity. Odontology. 110, 1–19. doi: 10.1007/s10266-021-00647-8
Brennan, C. A., Clay, S. L., Lavoie, S. L., Bae, S., Lang, J. K., Fonseca-Pereira, D., et al. (2021). Fusobacterium nucleatum drives a pro-inflammatory intestinal microenvironment through metabolite receptor-dependent modulation of IL-17 expression. Gut Microbes. 13, 1987780. doi: 10.1080/19490976.2021.1987780
Brennan, C. A., Garrett, W. S. (2019). Fusobacterium nucleatum—symbiont, opportunist and oncobacterium. Nat. Rev. Microbiology. 17, 156–166. doi: 10.1038/s41579-018-0129-6
Brings, C., Cadeddu, R.-P., Fröbel, J., Schelzig, H., Haas, R., Gattermann, N. (2020). Impaired formation of neutrophil extracellular traps (NETs) in patients with myelodysplastic syndrome (MDS). Blood. 136, 5–6. doi: 10.1182/blood-2020-138409
Brito, L. F., Taboza, Z. A., Silveira, V. R., Furlaneto, F. A., Rosing, C. K., Rego, R. O. (2018). Aggressive periodontitis presents a higher degree of bilateral symmetry in comparison with chronic periodontitis. J. science. 60, 97–104. doi: 10.2334/josnusd.16-0669
Cardoso, E. M., Reis, C., Manzanares-Céspedes, M. C. (2018). Chronic periodontitis, inflammatory cytokines, and interrelationship with other chronic diseases. Postgraduate medicine. 130, 98–104. doi: 10.1080/00325481.2018.1396876
Carra, M. C., Rangé, H., Caligiuri, G., Bouchard, P. (2023). Periodontitis and atherosclerotic cardiovascular disease: A critical appraisal. Periodontology 2000, 1–34. doi: 10.1111/prd.12528
Carrouel, F., Viennot, S., Santamaria, J., Veber, P., Bourgeois, D. (2016). Quantitative molecular detection of 19 major pathogens in the interdental biofilm of periodontally healthy young adults. Front. microbiology. 7, 840. doi: 10.3389/fmicb.2016.00840
Cavalcanti, Y. W., Wilson, M., Lewis, M., Del-Bel-Cury, A. A., da Silva, W. J., Williams, D. W. (2016). Modulation of Candida albicans virulence by bacterial biofilms on titanium surfaces. Biofouling. 32, 123–134. doi: 10.1080/08927014.2015.1125472
Cecil, J. D., O’Brien-Simpson, N. M., Lenzo, J. C., Holden, J. A., Chen, Y.-Y., Singleton, W., et al. (2016). Differential responses of pattern recognition receptors to outer membrane vesicles of three periodontal pathogens. PloS One 11, e0151967. doi: 10.1371/journal.pone.0151967
Chakravarti, A., Raquil, M.-A., Tessier, P., Poubelle, P. E. (2009). Surface RANKL of Toll-like receptor 4–stimulated human neutrophils activates osteoclastic bone resorption. Blood J. Am. Soc. Hematology. 114, 1633–1644. doi: 10.1182/blood-2008-09-178301
Chambrone, L., Wang, H. L., Romanos, G. E. (2018). Antimicrobial photodynamic therapy for the treatment of periodontitis and peri-implantitis: An American Academy of Periodontology best evidence review. J. periodontology. 89, 783–803. doi: 10.1902/jop.2017.170172
Chawhuaveang, D. D., Yu, O. Y., Yin, I. X., Lam, W. Y.-H., Mei, M. L., Chu, C.-H. (2021). Acquired salivary pellicle and oral diseases: A literature review. J. Dental Sci. 16, 523–529. doi: 10.1016/j.jds.2020.10.007
Chen, W. A., Dou, Y., Fletcher, H. M., Boskovic, D. S. (2023). Local and systemic effects of Porphyromonas gingivalis infection. Microorganisms. 11, 470. doi: 10.3390/microorganisms11020470
Chen, Y., Huang, Z., Tang, Z., Huang, Y., Huang, M., Liu, H., et al. (2022). More than just a periodontal pathogen–the research progress on Fusobacterium nucleatum. Front. Cell. Infection Microbiology. 12, 815318. doi: 10.3389/fcimb.2022.815318
Chen, G., Sun, Q., Cai, Q., Zhou, H. (2022). Outer membrane vesicles from fusobacterium nucleatum switch M0-like macrophages toward the M1 phenotype to destroy periodontal tissues in mice. Front. Microbiology. 13, 815638. doi: 10.3389/fmicb.2022.815638
Cheng, R., Wu, Z., Li, M., Shao, M., Hu, T. (2020). Interleukin-1β is a potential therapeutic target for periodontitis: a narrative review. Int. J. science. 12, 2. doi: 10.1038/s41368-019-0068-8
Chi, B., Qi, M., Kuramitsu, H. K. (2003). Role of dentilisin in Treponema denticola epithelial cell layer penetration. Res. microbiology. 154, 637–643. doi: 10.1016/j.resmic.2003.08.001
Chopra, A., Bhat, S. G., Sivaraman, K. (2020). Porphyromonas gingivalis adopts intricate and unique molecular mechanisms to survive and persist within the host: a critical update. J. Microbiology. 12, 1801090. doi: 10.1080/20002297.2020.1801090
Ciaston, I., Budziaszek, J., Satala, D., Potempa, B., Fuchs, A., Rapala-Kozik, M., et al. (2022). Proteolytic activity-independent activation of the immune response by gingipains from Porphyromonas gingivalis. Mbio. 13, e03787–e03721. doi: 10.1128/mbio.03787-21
Clark, D., Febbraio, M., Levin, L. (2017). Aggressive periodontitis: The unsolved mystery. Quintessence Int. 48, 103–111. doi: 10.3290/j.qi.a37387
Cochran, D. L. (2008). Inflammation and bone loss in periodontal disease. J. periodontology. 79, 1569–1576. doi: 10.1902/jop.2008.080233
Collins, P. E., Somma, D., Kerrigan, D., Herrington, F., Keeshan, K., Nibbs, R. J., et al. (2019). The IκB-protein BCL-3 controls Toll-like receptor-induced MAPK activity by promoting TPL-2 degradation in the nucleus. Proc. Natl. Acad. Sci. 116, 25828–25838. doi: 10.1073/pnas.1900408116
Colombo, A. P. V., do Souto, R. M., da Silva-Boghossian, C. M., Miranda, R., Lourenço, T. G. B. (2015). Microbiology of oral biofilm-dependent diseases: have we made significant progress to understand and treat these diseases? Curr. Health Rep. 2, 37–47. doi: 10.1007/s40496-014-0041-8
Danforth, D. R., Melloni, M., Tristano, J., Mintz, K. P. (2021). Contribution of adhesion proteins to Aggregatibacter actinomycetemcomitans biofilm formation. Mol. microbiology. 36, 243–253. doi: 10.1111/omi.12346
Danforth, D. R., Tang-Siegel, G., Ruiz, T., Mintz, K. P. (2019). A nonfimbrial adhesin of Aggregatibacter actinomycetemcomitans mediates biofilm biogenesis. Infection Immunity. 87, e00704-18. doi: 10.1128/iai.00704-18
Darveau, R. P., Curtis, M. A. (2021). Oral biofilms revisited: a novel host tissue of bacteriological origin. Periodontology 2000. 86, 8–13. doi: 10.1111/prd.12374
Das, S. (2022). Genetic regulation, biosynthesis and applications of extracellular polysaccharides of the biofilm matrix of bacteria. Carbohydr. polymers. 291, 119536. doi: 10.1016/j.carbpol.2022.119536
Dashper, S. G., Nastri, A., Abbott, P. V. (2019) Odontogenic bacterial infectionsin Contemporary oral medicineEdsFarah, C., Balasubramaniam, R., McCullough, M.. (Cham, Switzerland: Springer International Publishing), 1–53.
De Andrade, K. Q., Almeida-da-Silva, C. L. C., Coutinho-Silva, R. (2019). Immunological pathways triggered by Porphyromonas gingivalis and Fusobacterium nucleatum: therapeutic possibilities? Mediators Inflammation 2019, 7241312. doi: 10.1155/2019/7241312
de Jongh, C. A., de Vries, T. J., Bikker, F. J., Gibbs, S., Krom, B. P. (2023). Mechanisms of Porphyromonas gingivalis to translocate over the oral mucosa and other tissue barriers. J. Microbiology. 15, 2205291. doi: 10.1080/20002297.2023.2205291
Delwiche, E., Pestka, J., Tortorello, M. (1985). The veillonellae: gram-negative cocci with a unique physiology. Annu. Rev. Microbiology. 39, 175–193. doi: 10.1146/annurev.mi.39.100185.001135
Deo, P. N., Deshmukh, R. (2019). Oral microbiome: Unveiling the fundamentals. J. maxillofacial pathology. 23, 122–128. doi: 10.4103/jomfp.JOMFP_304_18
Digel, I., Kern, I., Geenen, E. M., Akimbekov, N. (2020). Dental plaque removal by ultrasonic toothbrushes. Dentistry J. 8, 28. doi: 10.3390/dj8010028
DiRienzo, J. M. (2014). Breaking the gingival epithelial barrier: role of the aggregatibacter actinomycetemcomitans cytolethal distending toxin in oral infectious disease. Cells. 3, 476–499. doi: 10.3390/cells3020476
Duan, T., Du, Y., Xing, C., Wang, H. Y., Wang, R.-F. (2022). Toll-like receptor signaling and its role in cell-mediated immunity. Front. Immunol. 13, 812774. doi: 10.3389/fimmu.2022.812774
Eckert, M., Mizgalska, D., Sculean, A., Potempa, J., Stavropoulos, A., Eick, S. (2018). In vivo expression of proteases and protease inhibitor, a serpin, by periodontal pathogens at teeth and implants. Mol. microbiology. 33, 240–248. doi: 10.1111/omi.2018.33.issue-3
Elashiry, M., Morandini, A. C., Cornelius Timothius, C. J., Ghaly, M., Cutler, C. W. (2021). Selective antimicrobial therapies for periodontitis: Win the “battle and the war. Int. J. Mol. Sci. 22, 6459. doi: 10.3390/ijms22126459
Eley, B. M., Cox, S. W. (2003). Proteolytic and hydrolytic enzymes from putative periodontal pathogens: characterization, molecular genetics, effects on host defenses and tisues and detection in gingival crevice fluid. Periodontology 2000. 31, 105–24. doi: 10.1034/j.1600-0757.2003.03107.x
Enax, J., Ganss, B., Amaechi, B. T., Schulze zur Wiesche, E., Meyer, F. (2023). The composition of the dental pellicle: an updated literature review. Front. Health 4, 1260442. doi: 10.3389/froh.2023.1260442
Engevik, M. A., Danhof, H. A., Ruan, W., Engevik, A. C., Chang-Graham, A. L., Engevik, K. A., et al. (2021). Fusobacterium nucleatum secretes outer membrane vesicles and promotes intestinal inflammation. MBio. 12, e02706-20. doi: 10.1128/mbio.02706-20
Falsetta, M. L., Klein, M. I., Colonne, P. M., Scott-Anne, K., Gregoire, S., Pai, C.-H., et al. (2014). Symbiotic relationship between Streptococcus mutans and Candida albicans synergizes virulence of plaque biofilms in vivo. Infection Immun. 82, 1968–1981. doi: 10.1128/IAI.00087-14
Fine, D. H., Schreiner, H., Velusamy, S. K. (2020). Aggregatibacter, a low abundance pathobiont that influences biogeography, microbial dysbiosis, and host defense capabilities in periodontitis: the history of a bug, and localization of disease. Pathogens. 9, 179. doi: 10.3390/pathogens9030179
Fiorillo, L., Cervino, G., Laino, L., D’Amico, C., Mauceri, R., Tozum, T. F., et al. (2019). Porphyromonas gingivalis, periodontal and systemic implications: a systematic review. Dentistry J. 7, 114. doi: 10.3390/dj7040114
Flynn, T. R. (2019). “Evidence-based principles of antibiotic therapy,” in Evidence-based oral surgery: a clinical guide for the general dental practitioner (Springer), 283–316. doi: 10.1007/978-3-319-91361-2_15
Fragkioudakis, I., Riggio, M. P., Apatzidou, D. A. (2021). Understanding the microbial components of periodontal diseases and periodontal treatment-induced microbiological shifts. J. Med. Microbiology. 70, 001247. doi: 10.1099/jmm.0.001247
Friedrich, V., Gruber, C., Nimeth, I., Pabinger, S., Sekot, G., Posch, G., et al. (2015). Outer membrane vesicles of Tannerella forsythia: biogenesis, composition, and virulence. Mol. microbiology. 30, 451–473. doi: 10.1111/omi.2015.30.issue-6
Fuller, J., Donos, N., Suvan, J., Tsakos, G., Nibali, L. (2020). Association of oral health-related quality of life measures with aggressive and chronic periodontitis. J. periodontal Res. 55, 574–580. doi: 10.1111/jre.12745
Galler, K. M., Grätz, E.-M., Widbiller, M., Buchalla, W., Knüttel, H. (2021). Pathophysiological mechanisms of root resorption after dental trauma: a systematic scoping review. BMC Health 21, 1–14. doi: 10.1186/s12903-021-01510-6
Ganther, S., Radaic, A., Malone, E., Kamarajan, P., Chang, N.-Y. N., Tafolla, C., et al. (2021). Treponema denticola dentilisin triggered TLR2/MyD88 activation upregulates a tissue destructive program involving MMPs via Sp1 in human oral cells. PloS pathogens. 17, e1009311. doi: 10.1371/journal.ppat.1009311
Gao, L., Xu, T., Huang, G., Jiang, S., Gu, Y., Chen, F. (2018). Oral microbiomes: more and more importance in oral cavity and whole body. Protein Cell. 9, 488–500. doi: 10.1007/s13238-018-0548-1
Garcia, B. L. (2022). Veillonella strain-specific virulence in oral disease (San Antonio: The University of Texas Health Science Center).
Garcia-Vello, P., Di Lorenzo, F., Lamprinaki, D., Notaro, A., Speciale, I., Molinaro, A., et al. (2021). Structure of the O-antigen and the lipid A from the lipopolysaccharide of fusobacterium nucleatum ATCC 51191. ChemBioChem. 22, 1252–1260. doi: 10.1002/cbic.202000751
George, S. D., Van Gerwen, O. T., Dong, C., Sousa, L. G., Cerca, N., Elnaggar, J. H., et al. (2024). The role of prevotella species in female genital tract infections. Pathogens. 13, 364. doi: 10.3390/pathogens13050364
Ghosh, A., Jaaback, K., Boulton, A., Wong-Brown, M., Raymond, S., Dutta, P., et al. (2024). Fusobacterium nucleatum: An Overview of Evidence, Demi-Decadal Trends, and Its Role in Adverse Pregnancy Outcomes and Various Gynecological Diseases, including Cancers. Cells. 13, 717. doi: 10.3390/cells13080717
Gnanasekaran, J., Binder Gallimidi, A., Saba, E., Pandi, K., Eli Berchoer, L., Hermano, E., et al. (2020). Intracellular Porphyromonas gingivalis promotes the tumorigenic behavior of pancreatic carcinoma cells. Cancers. 12, 2331. doi: 10.3390/cancers12082331
Goetting-Minesky, M. P., Godovikova, V., Fenno, J. C. (2021). Approaches to understanding mechanisms of dentilisin protease complex expression in Treponema denticola. Front. Cell. Infection Microbiology. 11, 668287. doi: 10.3389/fcimb.2021.668287
Golub, L. M., Lee, H. M. (2020). Periodontal therapeutics: Current host-modulation agents and future directions. Periodontology 2000. 82, 186–204. doi: 10.1111/prd.12315
Gouze, H., Noussair, L., Padovano, I., Salomon, E., De Laroche, M., Duran, C., et al. (2019). Veillonella parvula spondylodiscitis. Médecine Maladies Infectieuses. 49, 54–58. doi: 10.1016/j.medmal.2018.10.001
Griffen, A. L., Becker, M. R., Lyons, S. R., Moeschberger, M. L., Leys, E. J. (1998). Prevalence of Porphyromonas gingivalis and periodontal health status. J. Clin. microbiology. 36, 3239–3242. doi: 10.1128/JCM.36.11.3239-3242.1998
Groeger, S., Zhou, Y., Ruf, S., Meyle, J. (2022). Pathogenic mechanisms of Fusobacterium nucleatum on oral epithelial cells. Front. Health 3, 831607. doi: 10.3389/froh.2022.831607
Gu, J.-Y., Fu, Z-b, Chen, J.-L., Liu, Y.-J., Xz, C., Sun, Y. (2022). Endotoxin tolerance induced by Porphyromonas gingivalis lipopolysaccharide alters macrophage polarization. Microbial pathogenesis. 164, 105448. doi: 10.1016/j.micpath.2022.105448
Guan, S.-M., Nagata, H., Shizukuishi, S., Wu, J.-Z. (2006). Degradation of human hemoglobin by Prevotella intermedia. Anaerobe. 12, 279–282. doi: 10.1016/j.anaerobe.2006.09.001
Guan, S.-M., Shu, L., Fu, S.-M., Liu, B., Xu, X.-L., Wu, J.-Z. (2008). Prevotella intermedia induces matrix metalloproteinase-9 expression in human periodontal ligament cells. FEMS Microbiol. letters. 283, 47–53. doi: 10.1111/j.1574-6968.2008.01140.x
Guan, S.-M., Shu, L., Fu, S.-M., Liu, B., Xu, X.-L., Wu, J.-Z. (2009). Prevotella intermedia upregulates MMP-1 and MMP-8 expression in human periodontal ligament cells. FEMS Microbiol. letters. 299, 214–222. doi: 10.1111/j.1574-6968.2009.01748.x
Hajishengallis, G. (2015). Periodontitis: from microbial immune subversion to systemic inflammation. Nat. Rev. Immunol. 15, 30–44. doi: 10.1038/nri3785
Hajishengallis, G., Chavakis, T., Hajishengallis, E., Lambris, J. D. (2015). Neutrophil homeostasis and inflammation: novel paradigms from studying periodontitis. J. Leucocyte Biol. 98, 539–548. doi: 10.1189/jlb.3VMR1014-468R
Hajishengallis, G., Lamont, R. J. (2012). Beyond the red complex and into more complexity: the polymicrobial synergy and dysbiosis (PSD) model of periodontal disease etiology. Mol. microbiology. 27, 409–419. doi: 10.1111/j.2041-1014.2012.00663.x
Hajishengallis, G., Moutsopoulos, N. M., Hajishengallis, E., Chavakis, T. (2016). “Immune and regulatory functions of neutrophils in inflammatory bone loss,” Semin. Immunol. 28:146–58. doi: 10.1016/j.smim.2016.02.002
Handal, T., Olsen, I., Walker, C., Caugant, D. (2004). β-Lactamase production and antimicrobial susceptibility of subgingival bacteria from refractory periodontitis. Microbiol. Immunol. 19, 303–308. doi: 10.1111/j.1399-302x.2004.00159.x
Hansen, G., Hilgenfeld, R. (2013). Architecture and regulation of HtrA-family proteins involved in protein quality control and stress response. Cell. Mol. Life Sci. 70, 761–775. doi: 10.1007/s00018-012-1076-4
Haque, M. M., Yerex, K., Kelekis-Cholakis, A., Duan, K. (2022). Advances in novel therapeutic approaches for periodontal diseases. BMC Health 22, 492. doi: 10.1186/s12903-022-02530-6
Hasegawa, Y., Nagano, K. (2021). Porphyromonas gingivalis FimA and Mfa1 fimbriae: Current insights on localization, function, biogenesis, and genotype. Japanese Dental Sci. Review. 57, 190–200. doi: 10.1016/j.jdsr.2021.09.003
Hasturk, H., Kantarci, A. (2015). Activation and resolution of periodontal inflammation and its systemic impact. Periodontology 2000. 69, 255–273. doi: 10.1111/prd.2015.69.issue-1
He, Z., Zhang, X., Song, Z., Li, L., Chang, H., Li, S., et al. (2020). Quercetin inhibits virulence properties of Porphyromas gingivalis in periodontal disease. Sci. reports. 10, 18313. doi: 10.1038/s41598-020-74977-y
Hernández-Jaimes, T., Monroy-Pérez, E., Garzón, J., Morales-Espinosa, R., Navarro-Ocaña, A., García-Cortés, L. R., et al. (2022). High virulence and multidrug resistance of escherichia coli isolated in periodontal disease. Microorganisms. 11, 45. doi: 10.3390/microorganisms11010045
Hinson, A. N., Hawkes, C. G., Blake, C. S., Fitzsimonds, Z. R., Zhu, B., Buck, G., et al. (2022). Treponema denticola induces interleukin-36γ expression in human oral gingival keratinocytes via the parallel activation of nf-κb and mitogen-activated protein kinase pathways. Infection Immunity. 90, e00247–e00222. doi: 10.1128/iai.00247-22
Hirai, J., Yamagishi, Y., Kinjo, T., Hagihara, M., Sakanashi, D., Suematsu, H., et al. (2016). Osteomyelitis caused by Veillonella species: Case report and review of the literature. J. Infection Chemotherapy. 22, 417–420. doi: 10.1016/j.jiac.2015.12.015
Holmes, C. J., Pellecchia, R. (2016). Antimicrobial therapy in management of odontogenic infections in general dentistry. Dental Clinics. 60, 497–507. doi: 10.1016/j.cden.2015.11.013
Hosseini Hooshiar, M., Salari, S., Nasiri, K., Salim, U. S., Saeed, L. M., Yasamineh, S., et al. (2024). The potential use of bacteriophages as antibacterial agents in dental infection. Virol. J. 21, 258. doi: 10.1186/s12985-024-02510-y
Huang, Y., Ni, S. (2024). Aggregatibacter actinomycetemcomitans with periodontitis and rheumatoid arthritis. Int. Dental J. 74, 58–65. doi: 10.1016/j.identj.2023.06.011
Hyo, Y., Fukushima, H., Harada, T., Hara, H. (2019). Nasal septal abscess caused by anaerobic bacteria of oral flora. Auris Nasus Larynx. 46, 147–150. doi: 10.1016/j.anl.2018.05.014
Inouye, T., Ohta, H., Kokeguchi, S., Fukui, K., Kato, K. (1990). Colonial variation and fimbriation of Actinobacillus actinomycetemcomitans. FEMS Microbiol. Lett. 69, 13–17. doi: 10.1111/j.1574-6968.1990.tb04167.x
Ishikawa, K. H., Bueno, M. R., Kawamoto, D., Simionato, M. R., Mayer, M. P. (2021). Lactobacilli postbiotics reduce biofilm formation and alter transcription of virulence genes of Aggregatibacter actinomycetemcomitans. Mol. Microbiology. 36, 92–102. doi: 10.1111/omi.12330
Isola, G., Polizzi, A., Patini, R., Ferlito, S., Alibrandi, A., Palazzo, G. (2020). Association among serum and salivary A. actinomycetemcomitans specific immunoglobulin antibodies and periodontitis. BMC Health 20, 1–13. doi: 10.1186/s12903-020-01258-5
Isola, G., Polizzi, A., Santonocito, S., Dalessandri, D., Migliorati, M., Indelicato, F. (2021). New frontiers on adjuvants drug strategies and treatments in periodontitis. Scientia pharmaceutica. 89, 46. doi: 10.3390/scipharm89040046
Jabri, B., Iken, M., Achmit, M., Rida, S., Ennibi, O. K. (2021). Occurrence of Candida albicans in periodontitis. Int. J. Dentistry. 2021, 5589664. doi: 10.1155/2021/5589664
Jabri, B., Iken, M., Ait-Ou-Amar, S., Rida, S., Bouziane, A., Ok, E. (2022). andida albicans and Candida dubliniensis in periodontitis in adolescents and young adults. Int. J. Microbiology. 2022, 4625368. doi: 10.1155/2022/4625368
Jakubovics, N. S., Strömberg, N., Van Dolleweerd, C. J., Kelly, C. G., Jenkinson, H. F. (2005). Differential binding specificities of oral streptococcal antigen I/II family adhesins for human or bacterial ligands. Mol. Microbiology. 55, 1591–1605. doi: 10.1111/j.1365-2958.2005.04495.x
Jensen, A., Ladegaard Grønkjær, L., Holmstrup, P., Vilstrup, H., Kilian, M. (2018). Unique subgingival microbiota associated with periodontitis in cirrhosis patients. Sci. reports. 8, 10718. doi: 10.1038/s41598-018-28905-w
Jepsen, K., Falk, W., Brune, F., Fimmers, R., Jepsen, S., Bekeredjian-Ding, I. (2021). Prevalence and antibiotic susceptibility trends of periodontal pathogens in the subgingival microbiota of German periodontitis patients: A retrospective surveillance study. J. Clin. Periodontology. 48, 1216–1227. doi: 10.1111/jcpe.13468
Jeske, A. H. (2024). “Antibiotics and antibiotic prophylaxis,” in Contemporary dental pharmacology: evidence-based considerations, vol. p. (Cham: Springer), 43–51. doi: 10.1007/978-3-031-53954-1_5
Jones, O. P., Hoyle, P. J. (2022). Azithromycin as an adjunct to subgingival professional mechanical plaque removal in the treatment of grade C periodontitis: a systematic review and meta-analysis. J. Periodontal Implant Science. 52, 352. doi: 10.5051/jpis.2200340017
Joshi, D., Garg, T., Goyal, A. K., Rath, G. (2016). Advanced drug delivery approaches against periodontitis. Drug delivery. 23, 363–377. doi: 10.3109/10717544.2014.935531
Jung, Y.-J., Jun, H.-K., Choi, B.-K. (2017). Porphyromonas gingivalis suppresses invasion of Fusobacterium nucleatum into gingival epithelial cells. J. microbiology. 9, 1320193. doi: 10.1080/20002297.2017.1320193
Kachlany, S. C., Planet, P. J., DeSalle, R., Fine, D. H., Figurski, D. H., Kaplan, J. B. (2001). flp-1, the first representative of a new pilin gene subfamily, is required for non-specific adherence of Actinobacillus actinomycetemcomitans. Mol. microbiology. 40, 542–554. doi: 10.1046/j.1365-2958.2001.02422.x
Kachlany, S. C., Vega, B. A. (2024). Therapeutic applications of aggregatibacter actinomycetemcomitans leukotoxin. Pathogens. 13, 354. doi: 10.3390/pathogens13050354
Kafle, S., Pradhan, S., Gupta, S. (2018). Locally delivered tetracycline fibres in the treatment of chronic periodontitis. J. Nepalese Soc. Periodontology Implantology. 2, 45–48. doi: 10.3126/jnspoi.v2i2.23612
Kaplan, C. W., Ma, X., Paranjpe, A., Jewett, A., Lux, R., Kinder-Haake, S., et al. (2010). Fusobacterium nucleatum outer membrane proteins Fap2 and RadD induce cell death in human lymphocytes. Infection immunity. 78, 4773–4778. doi: 10.1128/IAI.00567-10
Karamifar, K., Tondari, A., Saghiri, M. A. (2020). Endodontic periapical lesion: an overview on the etiology, diagnosis and current treatment modalities. Eur. endodontic J. 5, 54. doi: 10.14744/eej.2020.42714
Kawai, T., Ikegawa, M., Ori, D., Akira, S. (2024). Decoding Toll-like receptors: Recent insights and perspectives in innate immunity. Immunity. 57, 649–673. doi: 10.1016/j.immuni.2024.03.004
Kawamoto, D., Borges, R., Ribeiro, R. A., de Souza, R. F., Amado, P. P. P., Saraiva, L., et al. (2021). Oral dysbiosis in severe forms of periodontitis is associated with gut dysbiosis and correlated with salivary inflammatory mediators: a preliminary study. Front. Health 2, 722495. doi: 10.3389/froh.2021.722495
Keestra, J., Grosjean, I., Coucke, W., Quirynen, M., Teughels, W. (2015). Non-surgical periodontal therapy with systemic antibiotics in patients with untreated chronic periodontitis: a systematic review and meta-analysis. J. periodontal Res. 50, 294–314. doi: 10.1111/jre.2015.50.issue-3
Kesic, L., Milasin, J., Igic, M., Obradovic, R. (2008). Microbial etiology of periodontal disease-mini review. Med. Biol. 15, 1–6.
Khammissa, R., Ballyram, R., Jadwat, Y., Fourie, J., Lemmer, J., Feller, L. (2018). Vitamin D deficiency as it relates to oral immunity and chronic periodontitis. Int. J. dentistry. 2018, 7315797. doi: 10.1155/2018/7315797
Khzam, N., Kujan, O., Haubek, D., Arslan, A., Johansson, A., Oscarsson, J., et al. (2024). Prevalence of subgingival aggregatibacter actinomycetemcomitans: descriptive cross-sectional study. Pathogens. 13, 531. doi: 10.3390/pathogens13070531
Kim, T. J., MacElroy, A. S., Defreitas, A., Shenker, B. J., Boesze-Battaglia, K. (2024). A synthetic small molecule, LGM2605: A promising modulator of increased pro-inflammatory cytokine and osteoclast differentiation by aggregatibacter actinomycetemcomitans cytolethal distending toxin. Dentistry J. 12, 195. doi: 10.3390/dj12070195
Kloppsteck, P., Hall, M., Hasegawa, Y., Persson, K. (2016). Structure of the fimbrial protein Mfa4 from Porphyromonas gingivalis in its precursor form: implications for a donor-strand complementation mechanism. Sci. reports. 6, 22945. doi: 10.1038/srep22945
Kokubu, E., Kikuchi, Y., Okamoto-Shibayama, K., Nakamura, S., Ishihara, K. (2021). Crawling motility of Treponema denticola modulated by outer sheath protein. Microbiol. Immunol. 65, 551–558. doi: 10.1111/1348-0421.12940
Kolenbrander, P. E., Palmer, R. J., Jr., Periasamy, S., Jakubovics, N. S. (2010). Oral multispecies biofilm development and the key role of cell–cell distance. Nat. Rev. Microbiology. 8, 471–480. doi: 10.1038/nrmicro2381
Kreve, S., Dos Reis, A. C. (2021). Bacterial adhesion to biomaterials: What regulates this attachment? A review. Japanese Dental Sci. review. 57, 85–96. doi: 10.1016/j.jdsr.2021.05.003
Krzyściak, W., Jurczak, A., Kościelniak, D., Bystrowska, B., Skalniak, A. (2014). The virulence of Streptococcus mutans and the ability to form biofilms. Eur. J. Clin. Microbiol. Infect. Diseases. 33, 499–515. doi: 10.1007/s10096-013-1993-7
Ksiazek, M., Mizgalska, D., Eick, S., Thøgersen, I. B., Enghild, J. J., Potempa, J. (2015). KLIKK proteases of Tannerella forsythia: putative virulence factors with a unique domain structure. Front. microbiology. 6, 312. doi: 10.3389/fmicb.2015.00312
Kuboniwa, M., Tribble, G. D., Hendrickson, E. L., Amano, A., Lamont, R. J., Hackett, M. (2012). Insights into the virulence of oral biofilms: discoveries from proteomics. Expert Rev. proteomics. 9, 311–323. doi: 10.1586/epr.12.16
Laine, M. L., Crielaard, W., Loos, B. G. (2012). Genetic susceptibility to periodontitis. Periodontology 2000. 58, 37–68. doi: 10.1111/j.1600-0757.2011.00415.x
Lamont, R., Demuth, D., Davis, C., Malamud, D., Rosan, B. (1991). Salivary-agglutinin-mediated adherence of Streptococcus mutans to early plaque bacteria. Infection immunity. 59, 3446–3450. doi: 10.1128/iai.59.10.3446-3450.1991
Lamont, R. J., Hajishengallis, G. N., Koo, H. M., Jenkinson, H. F. (2020). Oral microbiology and immunology (John Wiley & Sons).
Larsen, J. M. (2017). The immune response to Prevotella bacteria in chronic inflammatory disease. Immunology. 151, 363–374. doi: 10.1111/imm.2017.151.issue-4
Larsen, T., Fiehn, N. E. (2017). Dental biofilm infections–an update. Apmis. 125, 376–384. doi: 10.1111/apm.2017.125.issue-4
Łasica, A., Golec, P., Laskus, A., Zalewska, M., Gędaj, M., Popowska, M. (2024). Periodontitis: etiology, conventional treatments, and emerging bacteriophage and predatory bacteria therapies. Front. Microbiology. 15, 1469414. doi: 10.3389/fmicb.2024.1469414
Li, J., Chen, P., Li, J., Gao, X., Chen, X., Chen, J. (2017). A new treatment of sepsis caused by veillonella parvula: A case report and literature review. J. Clin. Pharm. Ther. 42, 649–652. doi: 10.1111/jcpt.2017.42.issue-5
Li, N., Collyer, C. A. (2011). Gingipains from Porphyromonas gingivalis—complex domain structures confer diverse functions. Eur. J. Microbiol. Immunol. 1, 41–58. doi: 10.1556/EuJMI.1.2011.1.7
Li, Y., Guo, R., Oduro, P. K., Sun, T., Chen, H., Yi, Y., et al. (2022). The relationship between porphyromonas gingivalis and rheumatoid arthritis: a meta-analysis. Front. Cell. infection microbiology. 12, 956417. doi: 10.3389/fcimb.2022.956417
Li, D., Wu, M. (2021). Pattern recognition receptors in health and diseases. Signal transduction targeted Ther. 6, 291. doi: 10.1038/s41392-021-00687-0
Li, Y., Xing, S., Chen, F., Li, Q., Dou, S., Huang, Y., et al. (2023). Intracellular Fusobacterium nucleatum infection attenuates antitumor immunity in esophageal squamous cell carcinoma. Nat. Communications. 14, 5788. doi: 10.1038/s41467-023-40987-3
Li, W., Zhu, Y., Singh, P., Ajmera, D. H., Song, J., Ji, P. (2016). Association of common variants in MMPs with periodontitis risk. Dis. markers. 2016, 1545974. doi: 10.1155/2016/1545974
Liu, S., Chen, M., Wang, Y., Zhou, X., Peng, X., Ren, B., et al. (2020). Effect of Veillonella parvula on the physiological activity of Streptococcus mutans. Arch. Biol. 109, 104578. doi: 10.1016/j.archoralbio.2019.104578
Liu, W., Zhang, X. (2015). Receptor activator of nuclear factor-κB ligand (RANKL)/RANK/osteoprotegerin system in bone and other tissues. Mol. Med. Reports. 11, 3212–3218. doi: 10.3892/mmr.2015.3152
Luan, J., Li, R., Xu, W., Sun, H., Li, Q., Wang, D., et al. (2023). Functional biomaterials for comprehensive periodontitis therapy. Acta Pharm. Sin. B. 13, 2310–2333. doi: 10.1016/j.apsb.2022.10.026
Luchian, I., Goriuc, A., Martu, M. A., Covasa, M. (2021). Clindamycin as an alternative option in optimizing periodontal therapy. Antibiotics. 10, 814. doi: 10.3390/antibiotics10070814
Lv, H., Zhuang, Y., Wu, W. (2023). “Lung abscess caused by tannerella forsythia infection: A case report,” in Infect Drug Res, 6975–6981. doi: 10.2147/IDR.S434494
Madel, M.-B., Ibáñez, L., Wakkach, A., De Vries, T. J., Teti, A., Apparailly, F., et al. (2019). Immune function and diversity of osteoclasts in normal and pathological conditions. Front. Immunol. 10, 1408. doi: 10.3389/fimmu.2019.01408
Man, S. M. (2011). The clinical importance of emerging Campylobacter species. Nat. Rev. Gastroenterology hepatology. 8, 669–685. doi: 10.1038/nrgastro.2011.191
Marre, A., RM, D., Lobo, L. A. (2020). Adhesion of anaerobic periodontal pathogens to extracellular matrix proteins. Braz. J. microbiology. 51, 1483–1491. doi: 10.1007/s42770-020-00312-2
Martínez-Rivera, J. I., Xibillé-Friedmann, D. X., González-Christen, J., de la Garza-Ramos, M. A., Carrillo-Vázquez, S. M., Montiel-Hernández, J. L. (2017). Salivary ammonia levels and Tannerella forsythia are associated with rheumatoid arthritis: A cross sectional study. Clin. Exp. Dental Res. 3, 107–114. doi: 10.1002/cre2.v3.3
Mashima, I., Theodorea, C. F., Thaweboon, B., Thaweboon, S., Nakazawa, F. (2016). Identification of Veillonella species in the tongue biofilm by using a novel one-step polymerase chain reaction method. PloS One 11, e0157516. doi: 10.1371/journal.pone.0157516
Matarazzo, F., Ribeiro, A. C., Feres, M., Faveri, M., Mayer, M. P. A. (2011). Diversity and quantitative analysis of Archaea in aggressive periodontitis and periodontally healthy subjects. J. Clin. periodontology. 38, 621–627. doi: 10.1111/j.1600-051X.2011.01734.x
Matera, G., Muto, V., Vinci, M., Zicca, E., Abdollahi-Roodsaz, S., van de Veerdonk, F. L., et al. (2009). Receptor recognition of and immune intracellular pathways for Veillonella parvula lipopolysaccharide. Clin. vaccine Immunol. 16, 1804–1809. doi: 10.1128/CVI.00310-09
Matsubara, V. H., Bandara, H., Ishikawa, K. H., Mayer, M. P. A., Samaranayake, L. P. (2016). The role of probiotic bacteria in managing periodontal disease: a systematic review. Expert Rev. anti-infective Ther. 14, 643–655. doi: 10.1080/14787210.2016.1194198
Matsumoto-Nakano, M. (2018). Role of Streptococcus mutans surface proteins for biofilm formation. Japanese Dental Sci. Review. 54, 22–29. doi: 10.1016/j.jdsr.2017.08.002
McGowan, K., McGowan, T., Ivanovski, S. (2018). Optimal dose and duration of amoxicillin-plus-metronidazole as an adjunct to non-surgical periodontal therapy: A systematic review and meta-analysis of randomized, placebo-controlled trials. J. Clin. periodontology. 45, 56–67. doi: 10.1111/jcpe.2018.45.issue-1
McIlvanna, E., Linden, G. J., Craig, S. G., Lundy, F. T., James, J. A. (2021). Fusobacterium nucleatum and oral cancer: a critical review. BMC cancer. 21, 1–11. doi: 10.1186/s12885-021-08903-4
Megson, Z., Koerdt, A., Schuster, H., Ludwig, R., Janesch, B., Frey, A., et al. (2015). Characterization of an α-l-fucosidase from the periodontal pathogen Tannerella forsythia. Virulence. 6, 282–292. doi: 10.1080/21505594.2015.1010982
Miao, X., Yang, W., Wang, S., Tang, J., Luo, X., Chen, T. (2024). Lung abscess caused by the anaerobic pathogen Tannerella forsythia. Respirology Case Reports. 12, e01391. doi: 10.1002/rcr2.v12.6
Miller, W. G., Yee, E. (2015). Complete genome sequence of Campylobacter gracilis ATCC 33236T. Genome Announcements. 3, e01087-15. doi: 10.1128/genomea.01087-15
Mínguez, M., Ennibi, O., Perdiguero, P., Lakhdar, L., Abdellaoui, L., Sánchez, M., et al. (2019). Antimicrobial susceptibilities of Aggregatibacter actinomycetemcomitans and Porphyromonas gingivalis strains from periodontitis patients in Morocco. Clin. Investigations. 23, 1161–1170. doi: 10.1007/s00784-018-2539-2
Mintz, K. P. (2004). Identification of an extracellular matrix protein adhesin, EmaA, which mediates the adhesion of Actinobacillus actinomycetemcomitans to collagen. Microbiology. 150, 2677–2688. doi: 10.1099/mic.0.27110-0
Miranda, T. S., Feres, M., Retamal-Valdes, B., Perez-Chaparro, P. J., Maciel, S. S., Duarte, P. M. (2017). Influence of glycemic control on the levels of subgingival periodontal pathogens in patients with generalized chronic periodontitis and type 2 diabetes. J. Appl. Science. 25, 82–89. doi: 10.1590/1678-77572016-0302
Mishra, S., Rath, S., Mohanty, N. (2020). Probiotics—A complete oral healthcare package. J. Integr. Medicine. 18, 462–469. doi: 10.1016/j.joim.2020.08.005
Mitchell, T. J. (2003). The pathogenesis of streptococcal infections: from tooth decay to meningitis. Nat. Rev. Microbiology. 1, 219–230. doi: 10.1038/nrmicro771
Miyai-Murai, Y., Okamoto-Shibayama, K., Sato, T., Kikuchi, Y., Kokubu, E., Potempa, J., et al. (2023). Localization and pathogenic role of the cysteine protease dentipain in Treponema denticola. Mol. microbiology. 38, 212–223. doi: 10.1111/omi.12406
Mohammad, C. A., Ali, K. M., Al-Rawi, R. A., Gul, S. S. (2022). Effects of curcumin and tetracycline gel on experimental induced periodontitis as an anti-inflammatory, osteogenesis promoter and enhanced bone density through altered Iron levels: histopathological study. Antibiotics. 11, 521. doi: 10.3390/antibiotics11040521
Mohanty, R., Asopa, S. J., Joseph, M. D., Singh, B., Rajguru, J. P., Saidath, K., et al. (2019). Red complex: Polymicrobial conglomerate in oral flora: A review. J. Family Med. primary Care 8, 3480–3486. doi: 10.4103/jfmpc.jfmpc_759_19
Mombelli, A., Casagni, F., Madianos, P. N. (2002). Can presence or absence of periodontal pathogens distinguish between subjects with chronic and aggressive periodontitis? A systematic review. J. Clin. periodontology. 29, 10–21. doi: 10.1034/j.1600-051X.29.s3.1.x
Montelongo-Jauregui, D., Lopez-Ribot, J. L. (2018). Candida interactions with the oral bacterial microbiota. J. fungi. 4, 122. doi: 10.3390/jof4040122
Mosaddad, S. A., Tahmasebi, E., Yazdanian, A., Rezvani, M. B., Seifalian, A., Yazdanian, M., et al. (2019). Oral microbial biofilms: an update. Eur. J. Clin. Microbiol. Infect. Diseases. 38, 2005–2019. doi: 10.1007/s10096-019-03641-9
Moschioni, M., Pansegrau, W., Barocchi, M. A. (2010). Adhesion determinants of the Streptococcus species. Microbial Biotechnol. 3, 370–388. doi: 10.1111/j.1751-7915.2009.00138.x
Mugri, M. H. (2022). Efficacy of systemic amoxicillin–metronidazole in periodontitis patients with diabetes mellitus: A systematic review of randomized clinical trials. Medicina. 58, 1605. doi: 10.3390/medicina58111605
Naito, M., Shoji, M., Sato, K., Nakayama, K. (2022). Insertional inactivation and gene complementation of Prevotella intermedia type IX secretion system reveals its indispensable roles in black pigmentation, hemagglutination, protease activity of interpain A, and biofilm formation. J. Bacteriology. 204, e00203–e00222. doi: 10.1128/jb.00203-22
Nakamura, S., Adachi, Y., Goto, T., Magariyama, Y. (2006). Improvement in motion efficiency of the spirochete Brachyspira pilosicoli in viscous environments. Biophys. J. 90, 3019–3026. doi: 10.1529/biophysj.105.074336
Nayak, A., Bhat, K., Shivanaikar, S., Pushpa, P., Kugaji, M., Kumbar, V. (2018). Detection of red complex organisms in chronic periodontitis by multiplex polymerase chain reaction. J. Advanced Clin. Res. Insights. 5, 139–144. doi: 10.15713/ins.jcri.231
Neurath, N., Kesting, M. (2024). Cytokines in gingivitis and periodontitis: from pathogenesis to therapeutic targets. Front. Immunol. 15,1435054. doi: 10.3389/fimmu.2024.1435054
Ng, H. M., Slakeski, N., Butler, C. A., Veith, P. D., Chen, Y.-Y., Liu, S. W., et al. (2019). The role of Treponema denticola motility in synergistic biofilm formation with Porphyromonas gingivalis. Front. Cell. infection microbiology. 9, 432. doi: 10.3389/fcimb.2019.00432
Nibali, L., Bayliss-Chapman, J., Almofareh, S., Zhou, Y., Divaris, K., Vieira, A. (2019). What is the heritability of periodontitis? A systematic review. J. Dental Res. 98, 632–641. doi: 10.1177/0022034519842510
Nørskov-Lauritsen, N., Claesson, R., Jensen, A. B., Åberg, C. H., Haubek, D. (2019). Aggregatibacter actinomycetemcomitans: Clinical significance of a pathobiont subjected to ample changes in classification and nomenclature. Pathogens. 8, 243. doi: 10.3390/pathogens8040243
Nygren, H., Dahlen, G., Nilsson, L. (1979). Human complement activation by lipopolysaccharides from bacteroides oralis, fusobacterium nucleatum, and veillonella parvula. Infection immunity. 26, 391–396. doi: 10.1128/iai.26.2.391-396.1979
Pai, S. I., Matheus, H. R., Guastaldi, F. P. (2023). Effects of periodontitis on cancer outcomes in the era of immunotherapy. Lancet Healthy Longevity. 4, e166–ee75. doi: 10.1016/S2666-7568(23)00021-1
Paillat, M., Lunar Silva, I., Cascales, E., Doan, T. (2023). A journey with type IX secretion system effectors: selection, transport, processing and activities. Microbiology. 169, 001320. doi: 10.1099/mic.0.001320
Paster, B. J., Olsen, I., Aas, J. A., Dewhirst, F. E. (2006). The breadth of bacterial diversity in the human periodontal pocket and other oral sites. Periodontology 2000. 42, 80–7. doi: 10.1111/j.1600-0757.2006.00174.x
Pelt, P., Zimmermann, B., Ulbrich, N., Bernimoulin, J.-P. (2002). Effects of lipopolysaccharide extracted from Prevotella intermedia on bone formation and on the release of osteolytic mediators by fetal mouse osteoblasts in vitro. Arch. Biol. 47, 859–866. doi: 10.1016/S0003-9969(02)00150-4
Peters, B. A., Wu, J., Pei, Z., Yang, L., Purdue, M. P., Freedman, N. D., et al. (2017). Oral microbiome composition reflects prospective risk for esophageal cancers. Cancer Res. 77, 6777–6787. doi: 10.1158/0008-5472.CAN-17-1296
Petersen, C., Round, J. L. (2014). Defining dysbiosis and its influence on host immunity and disease. Cell. microbiology. 16, 1024–1033. doi: 10.1111/cmi.2014.16.issue-7
Philips, A., Stolarek, I., Handschuh, L., Nowis, K., Juras, A., Trzciński, D., et al. (2020). Analysis of oral microbiome from fossil human remains revealed the significant differences in virulence factors of modern and ancient Tannerella forsythia. BMC Genomics 21, 1–14. doi: 10.1186/s12864-020-06810-9
Pignatelli, P., Nuccio, F., Piattelli, A., Curia, M. C. (2023). The role of Fusobacterium nucleatum in oral and colorectal carcinogenesis. Microorganisms. 11, 2358. doi: 10.3390/microorganisms11092358
Pilliol, V., Mahmoud Abdelwadoud, B., Aïcha, H., Lucille, T., Gérard, A., Hervé, T., et al. (2024). Methanobrevibacter oralis: a comprehensive review. J. Microbiology. 16, 2415734. doi: 10.1080/20002297.2024.2415734
Pisani, F., Pisani, V., Arcangeli, F., Harding, A., Singhrao, S. K. (2023). Treponema denticola Has the Potential to Cause Neurodegeneration in the Midbrain via the Periodontal Route of Infection—Narrative Review. Int. J. Environ. Res. Public Health 20, 6049. doi: 10.3390/ijerph20116049
Popova, C., Dosseva-Panova, V., Panov, V. (2013). Microbiology of periodontal diseases. A review. Biotechnol. Biotechnol. Equipment. 27, 3754–3759. doi: 10.5504/BBEQ.2013.0027
Posch, G., Sekot, G., Friedrich, V., Megson, Z. A., Koerdt, A., Messner, P., et al. (2012). Glycobiology aspects of the periodontal pathogen Tannerella forsythia. Biomolecules. 2, 467–482. doi: 10.3390/biom2040467
Preshaw, P. M., Bissett, S. M. (2019). Periodontitis and diabetes. Br. Dental J. 227, 577–584. doi: 10.1038/s41415-019-0794-5
Prucsi, Z., Płonczyńska, A., Potempa, J., Sochalska, M. (2021). Uncovering the oral dysbiotic microbiota as masters of neutrophil responses in the pathobiology of periodontitis. Front. Microbiology. 12,729717. doi: 10.3389/fmicb.2021.729717
Puthenveetil, R., Kumar, S., Caimano, M. J., Dey, A., Anand, A., Vinogradova, O., et al. (2017). The major outer sheath protein forms distinct conformers and multimeric complexes in the outer membrane and periplasm of Treponema denticola. Sci. Reports. 7, 13260. doi: 10.1038/s41598-017-13550-6
Radaic, A., Kapila, Y. L. (2021). The oralome and its dysbiosis: New insights into oral microbiome-host interactions. Comput. Struct. Biotechnol. J. 19, 1335–1360. doi: 10.1016/j.csbj.2021.02.010
Rajeshwari, H., Dhamecha, D., Jagwani, S., Rao, M., Jadhav, K., Shaikh, S., et al. (2019). Local drug delivery systems in the management of periodontitis: A scientific review. J. Controlled Release. 307, 393–409. doi: 10.1016/j.jconrel.2019.06.038
Ramadan, D. E., Hariyani, N., Indrawati, R., Ridwan, R. D., Diyatri, I. (2020). Cytokines and chemokines in periodontitis. Eur. J. dentistry. 14, 483–495. doi: 10.1055/s-0040-1712718
Rams, T. E., Sautter, J. D., van Winkelhoff, A. J. (2023). Emergence of antibiotic-resistant Porphyromonas gingivalis in United States periodontitis patients. Antibiotics. 12, 1584. doi: 10.3390/antibiotics12111584
Reyes, L. (2021). Porphyromonas gingivalis. Trends Microbiology. 29, 376–377. doi: 10.1016/j.tim.2021.01.010
Rivera-Quiroga, R. E., Cardona, N., Padilla, L., Rivera, W., Rocha-Roa, C., Diaz De Rienzo, M. A., et al. (2020). In silico selection and in vitro evaluation of new molecules that inhibit the adhesion of Streptococcus mutans through Antigen I/II. Int. J. Mol. Sci. 22, 377. doi: 10.3390/ijms22010377
Rôças, I., Siqueira, J. (2006). Culture-independent detection of Eikenella corrodens and Veillonella parvula in primary endodontic infections. J. endodontics. 32, 509–512. doi: 10.1016/j.joen.2005.07.004
Rojas-Tapias, D. F., Brown, E. M., Temple, E. R., Onyekaba, M. A., Mohamed, A. M., Duncan, K., et al. (2022). Inflammation-associated nitrate facilitates ectopic colonization of oral bacterium Veillonella parvula in the intestine. Nat. microbiology. 7, 1673–1685. doi: 10.1038/s41564-022-01224-7
Rose, J. E., Meyer, D. H., Fives-Taylor, P. M. (2003). Aae, an autotransporter involved in adhesion of Actinobacillus actinomycetemcomitans to epithelial cells. Infection immunity. 71, 2384–2393. doi: 10.1128/IAI.71.5.2384-2393.2003
Ryder, M. I. (2020). Porphyromonas gingivalis and Alzheimer disease: Recent findings and potential therapies. J. periodontology. 91, S45–SS9. doi: 10.1002/JPER.20-0104
Sachelarie, L., Scrobota, I., Romanul, I., Iurcov, R., Potra Cicalau, G. I., Todor, L. (2025). Probiotic therapy as an adjuvant in the treatment of periodontal disease: an innovative approach. Medicina. 61, 126. doi: 10.3390/medicina61010126
Sakakibara, J., Nagano, K., Murakami, Y., Higuchi, N., Nakamura, H., Shimozato, K., et al. (2007). Loss of adherence ability to human gingival epithelial cells in S-layer protein-deficient mutants of Tannerella forsythensis. Microbiology. 153, 866–876. doi: 10.1099/mic.0.29275-0
Salvi, G. E., Stähli, A., Schmidt, J. C., Ramseier, C. A., Sculean, A., Walter, C. (2020). Adjunctive laser or antimicrobial photodynamic therapy to non-surgical mechanical instrumentation in patients with untreated periodontitis: A systematic review and meta-analysis. J. Clin. periodontology. 47, 176–198. doi: 10.1111/jcpe.v47.s22
Săndulescu, M., Sîrbu, V. D., Popovici, I. A. (2023). Bacterial species associated with peri-implant disease–a literature review. Germs. 13, 352. doi: 10.18683/germs.2023.1405
Saquib, S. A., AlQahtani, N. A., Ahmad, I., Arora, S., Asif, S. M., Javali, M. A., et al. (2021). Synergistic antibacterial activity of herbal extracts with antibiotics on bacteria responsible for periodontitis. J. Infection Developing Countries. 15, 1685–1693. doi: 10.3855/jidc.14904
Sauer, K., Stoodley, P., Goeres, D. M., Hall-Stoodley, L., Burmølle, M., Stewart, P. S., et al. (2022). The biofilm life cycle: expanding the conceptual model of biofilm formation. Nat. Rev. Microbiology. 20, 608–620. doi: 10.1038/s41579-022-00767-0
Sayad, A., Gholami, L., Mirzajani, S., Omrani, M. D., Ghafouri-Fard, S., Taheri, M. (2020). Genetic susceptibility for periodontitis with special focus on immune-related genes: A concise review. Gene Reports. 21, 100814. doi: 10.1016/j.genrep.2020.100814
Schäffer, C., Andrukhov, O. (2024). The intriguing strategies of Tannerella forsythia’s host interaction. Front. Health 5, 1434217. doi: 10.3389/froh.2024.1434217
Scharnow, A. M., Solinski, A. E., Wuest, W. M., Targeting, S. (2019). mutans biofilms: a perspective on preventing dental caries. Medchemcomm. 10, 1057–1067. doi: 10.1039/C9MD00015A
Schenkein, H. A., Papapanou, P. N., Genco, R., Sanz, M. (2020). Mechanisms underlying the association between periodontitis and atherosclerotic disease. Periodontology 2000. 83, 90–106. doi: 10.1111/prd.12304
Sczepanik, F. S. C., Grossi, M. L., Casati, M., Goldberg, M., Glogauer, M., Fine, N., et al. (2020). Periodontitis is an inflammatory disease of oxidative stress: We should treat it that way. Periodontology 2000. 84, 45–68. doi: 10.1111/prd.12342
Seers, C. A., Mahmud, A. S. M., Huq, N. L., Cross, K. J., Reynolds, E. C. (2021). Porphyromonas gingivalis laboratory strains and clinical isolates exhibit different distribution of cell surface and secreted gingipains. J. microbiology. 13, 1858001. doi: 10.1080/20002297.2020.1858001
Settem, R. P., Honma, K., Nakajima, T., Phansopa, C., Roy, S., Stafford, G. P., et al. (2013). A bacterial glycan core linked to surface (S)-layer proteins modulates host immunity through Th17 suppression. Mucosal Immunol. 6, 415–426. doi: 10.1038/mi.2012.85
Shahoumi, L., Saleh, M., Meghil, M. (2023). Virulence factors of the periodontal pathogens: tools to evade the host immune response and promote carcinogenesis. Microorganisms 11, 115. doi: 10.3390/microorganisms11010115
Shahoumi, L. A., Saleh, M. H., Meghil, M. M. (2023). Virulence factors of the periodontal pathogens: tools to evade the host immune response and promote carcinogenesis. Microorganisms. 11, 115. doi: 10.3390/microorganisms11010115
Sharaf, S., Hijazi, K. (2022). Modulatory mechanisms of pathogenicity in porphyromonas gingivalis and other periodontal pathobionts. Microorganisms. 11, 15. doi: 10.3390/microorganisms11010015
Sharma, A. (2010). Virulence mechanisms of Tannerella forsythia. Periodontology 2000. 54, 106. doi: 10.1111/j.1600-0757.2009.00332.x
Sharma, A. (2020). Persistence of Tannerella forsythia and Fusobacterium nucleatum in dental plaque: a strategic alliance. Curr. Health reports. 7, 22–28. doi: 10.1007/s40496-020-00254-6
Sharpe, S. W., Kuehn, M. J., Mason, K. M. (2011). Elicitation of epithelial cell-derived immune effectors by outer membrane vesicles of nontypeable Haemophilus influenzae. Infection immunity. 79, 4361–4369. doi: 10.1128/IAI.05332-11
Shenker, B. J., Walker, L. P., Zekavat, A., Korostoff, J., Boesze-Battaglia, K. (2022). Aggregatibacter actinomycetemcomitans cytolethal distending toxin-induces cell cycle arrest in a glycogen synthase kinase (GSK)-3-dependent manner in oral keratinocytes. Int. J. Mol. Sci. 23, 11831. doi: 10.3390/ijms231911831
Shlezinger, M., Khalifa, L., Houri-Haddad, Y., Coppenhagen-Glazer, S., Resch, G., Que, Y.-A., et al. (2017). Phage therapy: a new horizon in the antibacterial treatment of oral pathogens. Curr. topics medicinal Chem. 17, 1199–1211. doi: 10.2174/1568026616666160930145649
Silva, I. L., Cascales, E. (2021). Molecular strategies underlying Porphyromonas gingivalis virulence. J. Mol. Biol. 433, 166836. doi: 10.1016/j.jmb.2021.166836
Singh, S., Singh, A. K. (2022). Porphyromonas gingivalis in oral squamous cell carcinoma: a review. In: Ørstavik, D.. Microbes infection. 24, 104925. doi: 10.1016/j.micinf.2021.104925
Siqueira, J. F., Jr., Rôças, I. N. (2019). “Microbiology of apical periodontitis,” in Perry, E. S., Patel, S., Kanagasingam, S., Hamer, S.. Essential endodontology: prevention and treatment of apical periodontitis (Wiley), 91–142. doi: 10.1002/9781119272014.ch4
Siqueira, J. F., Jr., Rôças, I. N. (2024). “Microbiology of primary apical periodontitis,” in Pitt ford’s problem-based learning in endodontology, 1–14. doi: 10.1002/9781119565987.ch1.1
Sisk-Hackworth, L., Ortiz-Velez, A., Reed, M. B., Kelley, S. T. (2021). Compositional data analysis of periodontal disease microbial communities. Front. microbiology. 12, 617949. doi: 10.3389/fmicb.2021.617949
Skottrup, P. D., Książek, M., Potempa, J. (2023). Phage display selection of human single domain antibodies towards karilysin, a metalloproteinase and secreted virulence factor from Tannerella forsythia. J. Immunological Methods 516, 113458. doi: 10.1016/j.jim.2023.113458
Slots, J. (2012). Low-cost periodontal therapy. Periodontology 2000. 60, 110–137. doi: 10.1111/j.1600-0757.2011.00429.x
Slots, J. (2015). Periodontal herpesviruses: prevalence, pathogenicity, systemic risk. Periodontology 2000. 69, 28–45. doi: 10.1111/prd.2015.69.issue-1
Slots, J. (2017). Periodontitis: facts, fallacies and the future. Periodontology 2000. 75, 7–23. doi: 10.1111/prd.2017.75.issue-1
Śmiga, M., Ślęzak, P., Wagner, M., Olczak, T. (2023). Interplay between Porphyromonas gingivalis hemophore-like protein HmuY and Kgp/RgpA gingipains plays a superior role in heme supply. Microbiol. Spectrum. 11, e04593–e04522. doi: 10.1128/spectrum.04593-22
Socransky, S. S., Haffajee, A. D. (1994). Evidence of bacterial etiology: a historical perspective. Periodontology 2000. 5, 7–25. doi: 10.1111/j.1600-0757.1994.tb00016.x
Socransky, S., Haffajee, A., Cugini, M., Smith, C., Kent, R. (1998). Microbial complexes in subgingival plaque. J. Clin. periodontology. 25, 134–144. doi: 10.1111/j.1600-051X.1998.tb02419.x
Sogodogo, E., Drancourt, M., Grine, G. (2019). Methanogens as emerging pathogens in anaerobic abscesses. Eur. J. Clin. Microbiol. Infect. Diseases. 38, 811–818. doi: 10.1007/s10096-019-03510-5
Sojod, B., Chateau, D., Mueller, C. G., Babajko, S., Berdal, A., Lézot, F., et al. (2017). RANK/RANKL/OPG signalization implication in periodontitis: new evidence from a RANK transgenic mouse model. Front. Physiol. 8, 338. doi: 10.3389/fphys.2017.00338
Souza, E. Q. M., da Rocha, T. E., Toro, L. F., Guiati, I. Z., Ervolino, E., Garcia, V. G., et al. (2020). Antimicrobial photodynamic therapy compared to systemic antibiotic therapy in non-surgical treatment of periodontitis: Systematic review and meta-analysis. Photodiagnosis photodynamic Ther. 31, 101808. doi: 10.1016/j.pdpdt.2020.101808
Sperandio F, F., Huang Y-Y, R., Hamblin, M. (2013). Antimicrobial photodynamic therapy to kill Gram-negative bacteria. Recent patents anti-infective Drug discovery. 8, 108–120. doi: 10.2174/1574891X113089990012
Srisatjaluk, R., Doyle, R., Justus, D. (1999). Outer membrane vesicles of Porphyromonas gingivalis inhibit IFN-γ-mediated MHC class II expression by human vascular endothelial cells. Microbial pathogenesis. 27, 81–91. doi: 10.1006/mpat.1999.0287
Stacy, A., Everett, J., Jorth, P., Trivedi, U., Rumbaugh, K. P., Whiteley, M. (2014). Bacterial fight-and-flight responses enhance virulence in a polymicrobial infection. Proc. Natl. Acad. Sci. 111, 7819–7824. doi: 10.1073/pnas.1400586111
Stefani, S., Campana, S., Cariani, L., Carnovale, V., Colombo, C., Lleo, M., et al. (2017). Relevance of multidrug-resistant Pseudomonas aeruginosa infections in cystic fibrosis. Int. J. Med. Microbiology. 307, 353–362. doi: 10.1016/j.ijmm.2017.07.004
Su, X., Tang, Z., Lu, Z., Liu, Y., He, W., Jiang, J., et al. (2021). Oral treponema denticola infection induces Aβ 1–40 and Aβ 1–42 accumulation in the hippocampus of C57BL/6 mice. J. Mol. Neurosci. 71, 1506–1514. doi: 10.1007/s12031-021-01827-5
Sudhakara, P., Gupta, A., Bhardwaj, A., Wilson, A. (2018). Oral dysbiotic communities and their implications in systemic diseases. Dentistry J. 6, 10. doi: 10.3390/dj6020010
Szafrański, S. P., Winkel, A., Stiesch, M. (2017). The use of bacteriophages to biocontrol oral biofilms. J. Biotechnol. 250, 29–44. doi: 10.1016/j.jbiotec.2017.01.002
Sztajer, H., Szafranski, S. P., Tomasch, J., Reck, M., Nimtz, M., Rohde, M., et al. (2014). Cross-feeding and interkingdom communication in dual-species biofilms of Streptococcus mutans and Candida albicans. ISME J. 8, 2256–2271. doi: 10.1038/ismej.2014.73
Taba, M., Kinney, J., Kim, A. S., Giannobile, W. V. (2005). Diagnostic biomarkers for oral and periodontal diseases. Dental Clinics. 49, 551–571. doi: 10.1016/j.cden.2005.03.009
Talapko, J., Juzbašić, M., Matijević, T., Pustijanac, E., Bekić, S., Kotris, I., et al. (2021). Candida albicans—the virulence factors and clinical manifestations of infection. J. Fungi. 7, 79. doi: 10.3390/jof7020079
Tamai, R., Sugamata, M., Kiyoura, Y. (2011). Candida albicans enhances invasion of human gingival epithelial cells and gingival fibroblasts by Porphyromonas gingivalis. Microbial pathogenesis. 51, 250–254. doi: 10.1016/j.micpath.2011.06.009
Tan, Q., Ma, X., Yang, B., Liu, Y., Xie, Y., Wang, X., et al. (2022). Periodontitis pathogen Porphyromonas gingivalis promotes pancreatic tumorigenesis via neutrophil elastase from tumor-associated neutrophils. Gut Microbes. 14, 2073785. doi: 10.1080/19490976.2022.2073785
Tanaka, A., Kogami, M., Nagatomo, Y., Takeda, Y., Kanzawa, H., Kawaguchi, Y., et al. (2022). Subcutaneous abscess due to empyema necessitans caused by Porphyromonas gingivalis in a patient with periodontitis. IDCases. 27, e01458. doi: 10.1016/j.idcr.2022.e01458
Tang, G., Kitten, T., Munro, C. L., Wellman, G. C., Mintz, K. P. (2008). EmaA, a potential virulence determinant of Aggregatibacter actinomycetemcomitans in infective endocarditis. Infection immunity. 76, 2316–2324. doi: 10.1128/IAI.00021-08
Tanner, A. C., Badger, S., Lai, C.-H., Listgarten, M. A., Visconti, R. A., Socransky, S. S. (1981). Wolinella gen. nov., Wolinella succinogenes (Vibrio succinogenes Wolin et al.) comb. nov., and description of Bacteroides gracilis sp. nov., Wolinella recta sp. nov., Campylobacter concisus sp. nov., and Eikenella corrodens from humans with periodontal disease. Int. J. Systematic Evolutionary Microbiol. 31, 432–445. doi: 10.1099/00207713-31-4-432
Tettamanti, L., Gaudio, R., Iapichino, A., Mucchi, D., Tagliabue, A. (2017). Genetic susceptibility and periodontal disease: A retrospective study on a large italian sample. implantology. 10, 20. doi: 10.11138/orl/2017.10.1.020
Tian, J., Liu, C., Zheng, X., Jia, X., Peng, X., Yang, R., et al. (2020). Porphyromonas gingivalis induces insulin resistance by increasing BCAA levels in mice. J. Dental Res. 99, 839–846. doi: 10.1177/0022034520911037
Torres, P. J., Thompson, J., McLean, J. S., Kelley, S. T., Edlund, A. (2019). Discovery of a novel periodontal disease-associated bacterium. Microbial ecology. 77, 267–276. doi: 10.1007/s00248-018-1200-6
Trindade, D., Carvalho, R., MaChado, V., Chambrone, L., Mendes, J. J., Botelho, J. (2023). Prevalence of periodontitis in dentate people between 2011 and 2020: a systematic review and meta-analysis of epidemiological studies. J. Clin. periodontology. 50, 604–626. doi: 10.1111/jcpe.13769
Udagawa, S., Katagiri, S., Maekawa, S., Takeuchi, Y., Komazaki, R., Ohtsu, A., et al. (2018). Effect of Porphyromonas gingivalis infection in the placenta and umbilical cord in pregnant mice with low birth weight. Acta Odontologica Scandinavica. 76, 433–441. doi: 10.1080/00016357.2018.1426876
Unniachan, A. S., Jayakumari, N. K., Sethuraman, S. (2020). Association between Candida species and periodontal disease: A systematic review. Curr. Med. mycology. 6, 63. doi: 10.18502/CMM.6.2.3420
Usui, M., Iwasaki, M., Ariyoshi, W., Kobayashi, K., Kasai, S., Yamanaka, R., et al. (2022). The ability of a novel trypsin-like peptidase activity assay kit to detect red-complex species. Diagnostics. 12, 2172. doi: 10.3390/diagnostics12092172
Van Dyke, T. E., Bartold, P. M., Reynolds, E. C. (2020). The nexus between periodontal inflammation and dysbiosis. Front. Immunol. 11,511. doi: 10.3389/fimmu.2020.00511
Van Winkelhoff, A., Herrera, D., Oteo, A., Sanz, M. (2005). Antimicrobial profiles of periodontal pathogens isolated from periodontitis patients in The Netherlands and Spain. J. Clin. Periodontology. 32, 893–898. doi: 10.1111/j.1600-051X.2005.00782.x
Veenith, T., Martin, H., Le Breuilly, M., Whitehouse, T., Gao-Smith, F., Duggal, N., et al. (2022). High generation of reactive oxygen species from neutrophils in patients with severe COVID-19. Sci. reports. 12, 10484. doi: 10.1038/s41598-022-13825-7
Velusamy, S. K., Sampathkumar, V., Ramasubbu, N., Paster, B. J., Fine, D. H. (2019). Aggregatibacter actinomycetemcomitans colonization and persistence in a primate model. Proc. Natl. Acad. Sci. 116, 22307–22313. doi: 10.1073/pnas.1905238116
Visentin, D., Gobin, I., Maglica, Ž. (2023). Periodontal pathogens and their links to neuroinflammation and neurodegeneration. Microorganisms. 11, 1832. doi: 10.3390/microorganisms11071832
Wang, X., Li, X., Ling, J. (2017). Streptococcus gordonii LuxS/autoinducer-2 quorum-sensing system modulates the dual-species biofilm formation with Streptococcus mutans. J. basic microbiology. 57, 605–616. doi: 10.1002/jobm.201700010
Wei, Y., G-p, D., Ren, Z.-Y., Wan, M.-C., Wang, C.-Y., Li, H.-B., et al. (2024). Recent advances in the pathogenesis and prevention strategies of dental calculus. NPJ Biofilms Microbiomes. 10, 56. doi: 10.1038/s41522-024-00529-1
Wellens, L., Casteels, I., Huygens, M. (2019). Veillonella parvula periorbital cellulitis: an unusual pathogen causing a common clinical sign. GMS Ophthalmol. Cases. 9. doi: 10.3205/oc000106
Wu, Y., Guo, S., Chen, F., Li, Y., Huang, Y., Liu, W., et al. (2023). Fn-Dps, a novel virulence factor of Fusobacterium nucleatum, disrupts erythrocytes and promotes metastasis in colorectal cancer. PloS Pathogens. 19, e1011096. doi: 10.1371/journal.ppat.1011096
Xu, W., Zhou, W., Wang, H., Liang, S. (2020). Roles of Porphyromonas gingivalis and its virulence factors in periodontitis. Adv. Protein Chem. Struct. Biol. 120, 45–84. doi: 10.1016/bs.apcsb.2019.12.001
Yamamoto, T., Eguchi, T. (2020). “Heat shock proteins and periodontitis–cross-reaction between bacterial and human HSP in periodontal infection linking with cardiovascular diseases,” in Heat shock proteins in inflammatory diseases, vol. p. (Cham: Springer), 19–32. doi: 10.1007/7515_2020_24
Yanagisawa, M., Kuriyama, T., Williams, D. W., Nakagawa, K., Karasawa, T. (2006). Proteinase activity of prevotella species associated with oral purulent infection. Curr. microbiology. 52, 375–378. doi: 10.1007/s00284-005-0261-1
Yang, B., Pang, X., Li, Z., Chen, Z., Wang, Y. (2021). Immunomodulation in the treatment of periodontitis: Progress and perspectives. Front. Immunol. 12, 781378. doi: 10.3389/fimmu.2021.781378
Ye, C., Liu, X., Liu, Z., Pan, C., Zhang, X., Zhao, Z., et al. (2024). Fusobacterium nucleatum in tumors: from tumorigenesis to tumor metastasis and tumor resistance. Cancer Biol. Ther. 25, 2306676. doi: 10.1080/15384047.2024.2306676
Yokogawa, T., Nagano, K., Fujita, M., Miyakawa, H., Iijima, M. (2022). Characterization of a Treponema denticola ATCC 35405 mutant strain with mutation accumulation, including a lack of phage-derived genes. PloS One 17, e0270198. doi: 10.1371/journal.pone.0270198
Yost, S., Duran-Pinedo, A. E. (2018). The contribution of Tannerella forsythia dipeptidyl aminopeptidase IV in the breakdown of collagen. Mol. microbiology. 33, 407–419. doi: 10.1111/omi.2018.33.issue-6
Yu, F., Iyer, D., Anaya, C., Lewis, J. P. (2006). Identification and characterization of a cell surface protein of Prevotella intermedia 17 with broad-spectrum binding activity for extracellular matrix proteins. Proteomics. 6, 6023–6032. doi: 10.1002/(ISSN)1615-9861
Yue, G., Kaplan, J. B., Furgang, D., Mansfield, K. G., Fine, D. H. (2007). A second Aggregatibacter actinomycetemcomitans autotransporter adhesin exhibits specificity for buccal epithelial cells in humans and Old World primates. Infection immunity. 75, 4440–4448. doi: 10.1128/IAI.02020-06
Zak, K. M., Bostock, M. J., Waligorska, I., Thøgersen, I. B., Enghild, J. J., Popowicz, G. M., et al. (2021). Latency, thermal stability, and identification of an inhibitory compound of mirolysin, a secretory protease of the human periodontopathogen Tannerella forsythia. J. Enzyme Inhibition Medicinal Chem. 36, 1267–1281. doi: 10.1080/14756366.2021.1937619
Zhang, Y., Ding, Y., Guo, Q. (2022). Probiotic species in the management of periodontal diseases: an overview. Front. Cell. infection microbiology. 12, 806463. doi: 10.3389/fcimb.2022.806463
Zhang, Z., Liu, S., Zhang, S., Li, Y., Shi, X., Liu, D., et al. (2022). Porphyromonas gingivalis outer membrane vesicles inhibit the invasion of Fusobacterium nucleatum into oral epithelial cells by downregulating FadA and FomA. J. Periodontology. 93, 515–525. doi: 10.1002/JPER.21-0144
Zhang, J., Xie, M., Huang, X., Chen, G., Yin, Y., Lu, X., et al. (2021). The effects of porphyromonas gingivalis on atherosclerosis-related cells. Front. Immunol. 12, 766560. doi: 10.3389/fimmu.2021.766560
Keywords: periodontitis, biofilm, inflammation, microbiology, treatment
Citation: Yekani M, Dastgir M, Fattahi S, Shahi S, Maleki Dizaj S and Memar MY (2025) Microbiological and molecular aspects of periodontitis pathogenesis: an infection-induced inflammatory condition. Front. Cell. Infect. Microbiol. 15:1533658. doi: 10.3389/fcimb.2025.1533658
Received: 24 November 2024; Accepted: 07 April 2025;
Published: 08 May 2025.
Edited by:
Matthias Tröltzsch, Center for Oral, Maxillofacial and Facial Reconstructive Surgery, GermanyReviewed by:
Rajendra Prasad Settem, University at Buffalo, United StatesArmelia Sari Widyarman, Trisakti University, Indonesia
Copyright © 2025 Yekani, Dastgir, Fattahi, Shahi, Maleki Dizaj and Memar. This is an open-access article distributed under the terms of the Creative Commons Attribution License (CC BY). The use, distribution or reproduction in other forums is permitted, provided the original author(s) and the copyright owner(s) are credited and that the original publication in this journal is cited, in accordance with accepted academic practice. No use, distribution or reproduction is permitted which does not comply with these terms.
*Correspondence: Mohammad Yousef Memar, eS5tZW1hckB5YWhvby5jb20=