- 1State Key Laboratory of Oral Diseases and National Center for Stomatology and National Clinical Research Center for Oral Diseases, West China Hospital of Stomatology, Sichuan University, Chengdu, Sichuan, China
- 2Department of Pediatric Dentistry, West China Hospital of Stomatology, Sichuan University, Chengdu, Sichuan, China
Objective: To elucidate the microbial diversities and compositions of dental plaque in children with severe early childhood caries (S-ECC) before and after comprehensive dental treatment under general anesthesia, contributing evidence for the reestablishment of oral microecological balance.
Design: Twenty children aged 2.2-5.5 years diagnosed with S-ECC without systemic diseases were enrolled in this study. Dental plaque samples were collected before and after treatment, analyzed using high-throughput sequencing on the Illumina Miseq platform. Bioinformatics methods were employed to compare the dental plaque microbial diversity and compositions differences before and after comprehensive dental treatment under general anesthesia.
Results: Significant enhancements in dental plaque microbial diversity were observed post-comprehensive treatment (P < 0.05). At the phylum level, the relative abundance of Actinobacteria significantly decreased after treatment (P < 0.05). At the genus level, the relative abundance of Streptococcus, Neisseria, and Rothia significantly declined (P < 0.05), while Prevotella showed a significant increase after treatment (P < 0.05).
Conclusions: Following comprehensive dental treatment under general anesthesia, children with S-ECC exhibit significant changes in the microbial diversity and composition of dental plaque, indicating a shift towards a more balanced oral microecological state. This study highlights the importance of dental intervention in positively altering oral microbiota.
Introduction
Dental caries is the most common chronic infectious oral disease worldwide, particularly affecting young children. It not only affects essential oral functions such as chewing, speech, and aesthetics, but also impacts facial appearance, self-esteem, and psychological well-being (Selwitz et al., 2007). In severe cases, it can exacerbate systemic health problems, such as children growth, cardiovascular disease, immune system disease, and kidney diseases, thereby affecting both oral and overall health in children (Clark and Braun, 2021; Zou et al., 2022). According to the “2022 Global Oral Health Status Report”, dental caries affects 29% of people with permanent teeth (nearly two billion individuals), and has an even more significant presence in primary teeth, with a prevalence rate of 43%, affecting approximately 514 million children (Lemos et al., 2019). Given this high prevalence, the prevention and management of caries in primary dentition remains a global public health priority.
Dental caries is closely associated with an imbalance in the oral microbial ecosystem. According to the ecological plaque hypothesis, diverse and stable oral microbial communities are essential for maintaining oral health (Gao et al., 2018). These balanced communities act as protective barriers against pathogenic shifts in microbial composition (Lamont et al., 2018). This dynamic balance is maintained through intricate interactions among various oral microorganisms and between these microorganisms and host (Blostein et al., 2022). However, alterations in local environmental conditions, such as changes in dietary sugar intake, pH fluctuations, and other biofilm-disrupting factors, can disturb this balance (Li et al., 2005; Rosier et al., 2018). These disturbances favor the growth of acid-producing and acid-tolerant bacteria (Sedghi et al., 2021; Santonocito et al., 2022; Suez et al., 2022). Such shifts reduce microbial diversity and promote the predominance of cariogenic pathogens. This disrupts biofilm stability and contributes to the development of caries (Jakubovics et al., 2021).
Emerging research highlights a marked difference in the oral microbial diversity between caries-free children and those afflicted with early childhood caries (ECC) (Hurley et al., 2019). Notably, a trend towards decreased microbial diversity has been observed in children with ECC, even before the clinical onset of the disease (Becker et al., 2002; Tao et al., 2013). This trend suggests that microbial diversity may serve as an early indicator of caries risk (Valm, 2019; Chen et al., 2020; Xiao et al., 2020). Furthermore, the presence and abundance of specific cariogenic bacteria such as Streptococcus, Actinomyces, and Lactobacillus increase in the context of ECC, while the numbers of health-associated bacterial species, such as those belonging to the Bacteroidaceae family, are significantly reduced (Xiao et al., 2019; Garcia et al., 2021; Li et al., 2023).
Severe early childhood caries (S-ECC), represents an extreme manifestation of ECC, defined as smooth surface caries in children younger than 36 months old or decayed missed filled surfaces (dmfs) ≥ 4 (3 years old), dmfs ≥ 5 (4 years old) and dmfs ≥ 6 (5 years old) (Zou et al., 2022). Children with S-ECC are very young and often experience anxiety and fear towards dental treatment (Seligman et al., 2017). It has been reported that 3%-43% of these children are unable to cooperate or even refuse treatment due to anxiety which delays their treatment time (Folayan et al., 2004). Due to the physiological and psychological characteristics of children, in order to quickly restore the function of the affected teeth, children with S-ECC who have complex treatment plans are often treated with one-time comprehensive dental treatment under general anesthesia in clinical practice to restore the shape of the teeth and their masticatory function in the shortest time (Schmoeckel et al., 2020; Kries et al., 2023). Despite the advancements in treatment modalities and our growing understanding of the microbial etiology of ECC, there remains a significant gap in our knowledge regarding the impact of comprehensive dental treatments on the oral microbial communities of children with S-ECC. Specifically, the changes in the diversity and composition of dental plaque biofilms following such treatments have not been explored.
This study explores the alterations in dental plaque microbial communities of children with diagnosed with S-ECC, both before and after comprehensive treatment under general anesthesia. By investigating the shifts in microbial diversity and composition associated with treatment, this research aims to provide new insights into the microbial basis for comprehensive dental interventions reestablishing oral microecological balance.
Materials and methods
Study subjects selection
Twenty children aged 2.2-5.5 years with dental caries were selected from the Department of Pediatric Dentistry, West China Hospital of Stomatology, Sichuan University, Chengdu, Sichuan, China, based on the following criteria:
Inclusion criteria:
1) Normal mental and intellectual abilities, with a Body Mass Index (BMI) within the normal range.
2) Clinically diagnosed with S-ECC.
3) Eligible for treatment under general anesthesia with parental consent for the treatment plan.
Exclusion criteria:
1) Presence of serious systemic diseases or infectious diseases, for which pediatricians do not recommend surgery under general anesthesia.
2) Use of antibiotics within three months prior to sampling, or a history of systemic medication use.
3) Prior dental disease treatments.
4) Dental structure abnormalities, such as enamel hypoplasia or dentinogenesis imperfecta.
All subjects and their guardians voluntarily participated in the study, with informed consent forms signed by the guardians.
Collection of plaque samples
Parents were explicitly instructed that the children should refrain from brushing their teeth starting the night before sampling and continue avoiding tooth brushing on the morning of the plaque collection. Plaque samples were collected consistently from standardized locations during both preoperative assessments and the one-month postoperative follow-up. Specifically, plaque samples were collected exclusively from supragingival plaque deposits on the buccal enamel surfaces of both the first and second primary molars on the left and right sides of the mandible. Samples were carefully scraped using sterile oral swabs under standardized conditions, ensuring only supragingival plaque was included and avoiding contamination with gingival crevicular fluid, gingival tissue. Following comprehensive dental treatment under general anesthesia, parents received detailed oral hygiene instructions, including proper tooth brushing techniques, dietary recommendations, and regular oral hygiene practices to maintain oral health and minimize caries recurrence. The collected plaque samples were immediately placed in 1 mL TE buffer (pH value 7.4) and transported to the laboratory within 2 hours on ice, and stored at -20°C for further DNA extraction.
DNA extraction and 16S rRNA gene sequencing
Total bacterial DNA was extracted using the QIAamp® DNA Micro Kit (QIAGEN, USA), following the manufacturer’s instructions. The extracted plaque sample DNA was stored at -80°C and sent to Shanghai Meiji Biomedical Technology Co., Ltd., under dry ice refrigeration conditions for subsequent sequencing and bioinformatic analysis. PCR amplification targeting the V3-V4 region of the bacterial 16S rRNA gene was performed, with the products analyzed using the Illumina Miseq sequencing platform.
Microbial diversity analysis
The Usearch software (version 7.1) with the RDP classifier Bayesian algorithm was used to perform taxonomic analysis on OTU representative sequences at 97% similarity level, and the community composition of each sample was counted at various levels (domain, kingdom, phylum, class, order, family, genus, species). The richness and diversity of the microbial communities in the samples were analyzed using Chao, ACE, Shannon, and Simpson indexes.
Microbial community difference analysis
The indexes of microbial diversity in dental plaque and the relative abundance of various bacterial communities before and after general anesthesia treatment in children were analyzed for significant differences using SPSS 18.0 software and paired t-test. The results were expressed as mean ± standard deviation, with a significant level of α=0.05.
Results
Clustering analysis of operational taxonomic units
Through Illumina MiSeq sequencing, a total of 1,782,512 sequences were obtained from 40 samples, with 802,183 sequences before treatment, averaging 40,109 sequences per sample, and 980,329 sequences after treatment, averaging 49,016 sequences per sample. The average length of each sequence was 442.98 bp, with nearly all sequences falling within the ranges of 421-440 bp (27.11%) and 441-460 bp (72.87%), suitable for further analysis (Table 1).

Table 1. Basic sequencing information of supragingival plaque samples collected from 20 children with severe early childhood caries (S-ECC) before and one month after comprehensive dental treatment under general anesthesia.
Based on the principle of similarity greater than 97%, the obtained high-quality sequences were compared with the SILVA database (Quast et al., 2013), and a total of 259 OTUs were obtained, with 251 before treatment and 252 after treatment, sharing 244 OTUs between the two groups (Fouts et al., 2012). The average OTU count for plaque microbiome was 142 ± 29 before treatment, significantly increasing to157 ± 20 after treatment (P < 0.05).
OTU-based analysis of microbial diversity
After undergoing comprehensive treatment of dental disease under general anesthesia, Chao1 value and Shannon index significantly increased (P < 0.05), while there were no significant differences in the ACE value and Simpson index (P > 0.05), suggesting an increase in the microbial diversity of plaque biofilms. The coverage values before and after treatment exceeded 0.999, indicating high library coverage and the sequencing results accurately represented the microbial situation in the samples (Table 2).

Table 2. Alpha diversity analysis of supragingival plaque microbial communities before and after treatment.
Composition analysis of microbial communities
The 259 OTUs were classified into 12 phyla, 20 classes, 30 orders, 46 families, and 87 genera. At the phylum level, 12 phylum bacteria were detected (Figure 1), with more than 99% primarily belonging to six dominant phyla: Firmicutes (37.5%), Fusobacteria (15.1%), Bacteroidetes (14.8%), Proteobacteria (14.2%), Actinobacteria (10.6%), and Saccharibacteria (TM7) (7.5%). At the genus level, bacteria from 87 genera were detected between the two groups (Figure 2), with genera having a relative abundance >1% including Actinobacillus (3.8%), Corynebacterium (4.3%), Rotella (2.1%), and Porphyromonas (2.1%). Prevotella (7.2%), Capnocytophaga (4.7%), Streptococcus (11.6%), Centipeda (1.7%), Selenomonas (9.6%), Veillonella (9.3%), Fusobacterium (4.6%), Leptotrichia (10.5%), Lautropia (2.3%), Neisseria (5.6%), Campylobacter (1.0%), Haemophilus (1.7%), and Candidate_division_TM7_norank (7.5%).
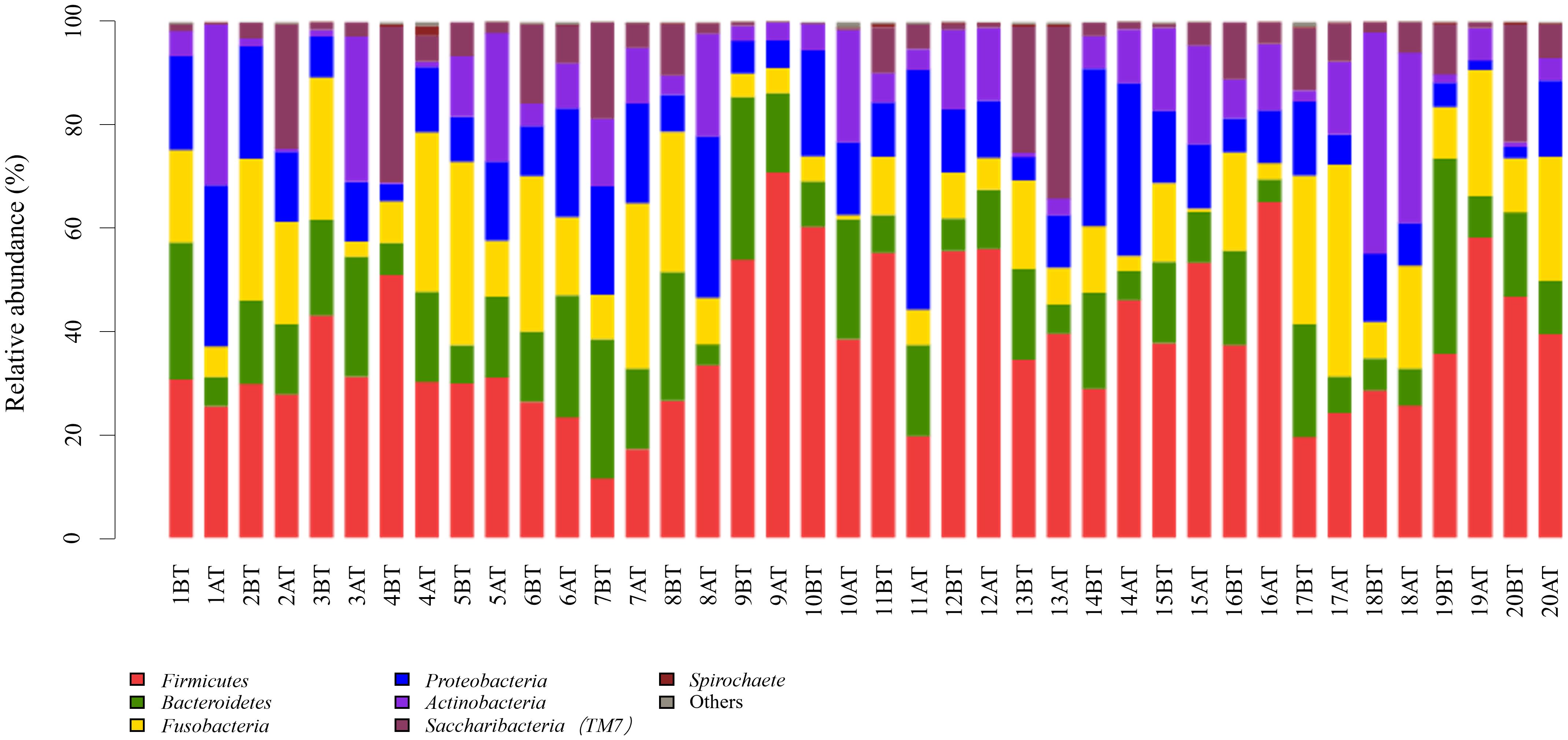
Figure 1. Relative abundance of bacterial phyla in supragingival plaque samples collected from 20 children diagnosed with S-ECC before and one month after comprehensive dental treatment under general anesthesia. Each bar represents the phylum-level taxonomic composition of a sample, calculated based on the percentage of total sequences. BT, before treatment; AT, after treatment.
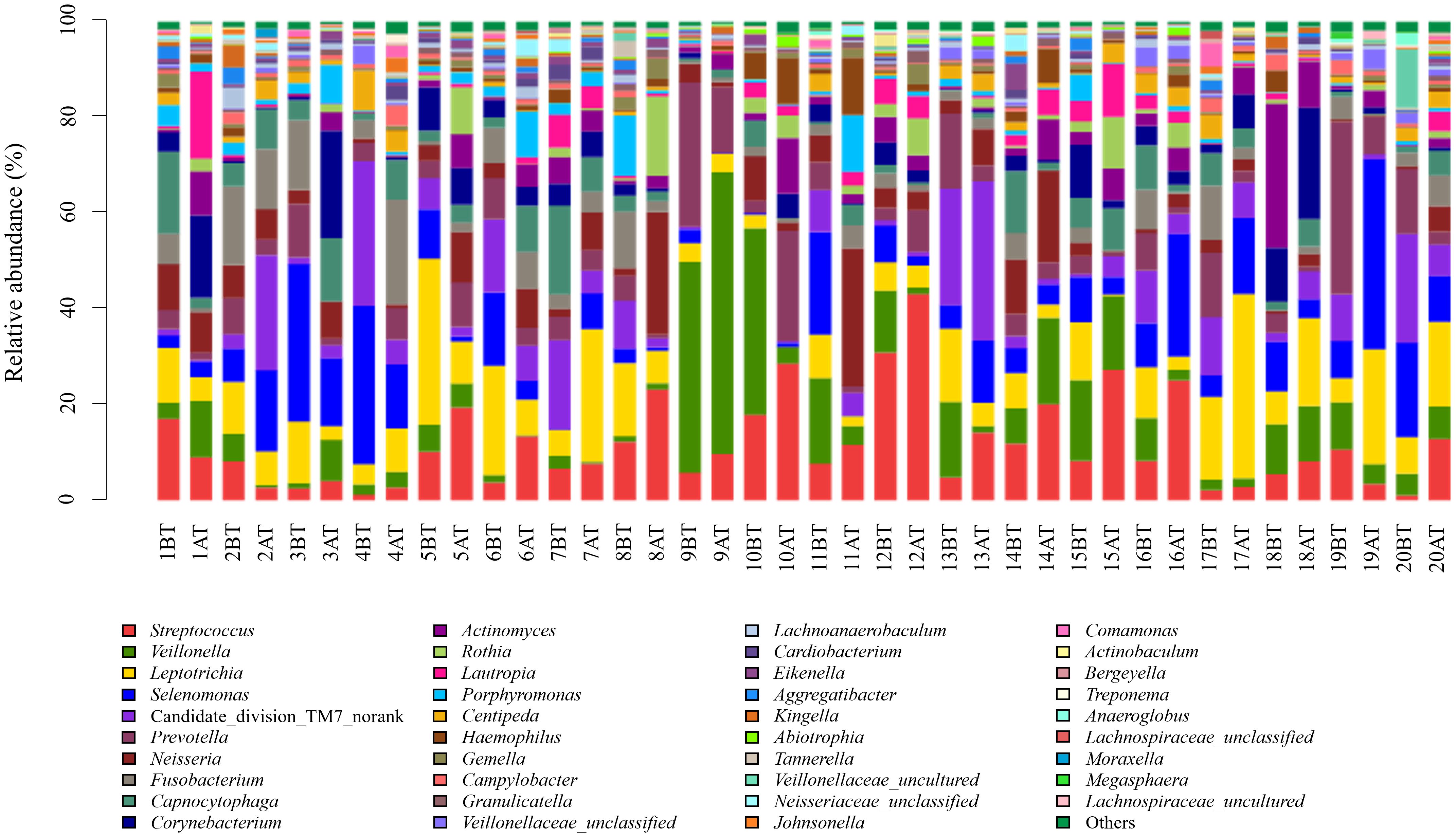
Figure 2. Relative abundance of bacterial genera in supragingival plaque samples collected from 20 children diagnosed with S-ECC before and one month after comprehensive dental treatment under general anesthesia. Each bar represents the genus-level taxonomic composition of a sample, calculated based on the percentage of total sequences. BT, before treatment; AT, after treatment.
Analysis of phylum-level differences in microbial composition
Before treatment, the relative abundance of dominant phyla in plaque biofilms was Firmicutes (37.9%), Proteobacteria (16.4%), Actinobacteria (13.7%), Fusobacteria (13.6%), Bacteroidetes (12.2%), and Saccharibacteria (TM7) (5.9%). After treatment, the relative abundance was Firmicutes (37.1%), Bacteroidetes (17.4%), Clostridium (16.8%), Proteobacteria (11.9%), Saccharibacteria (TM7) (9.0%), and Actinobacteria (7.5%), with Actinobacteria being significantly decreased compared to before treatment (Figure 3, Table 3).
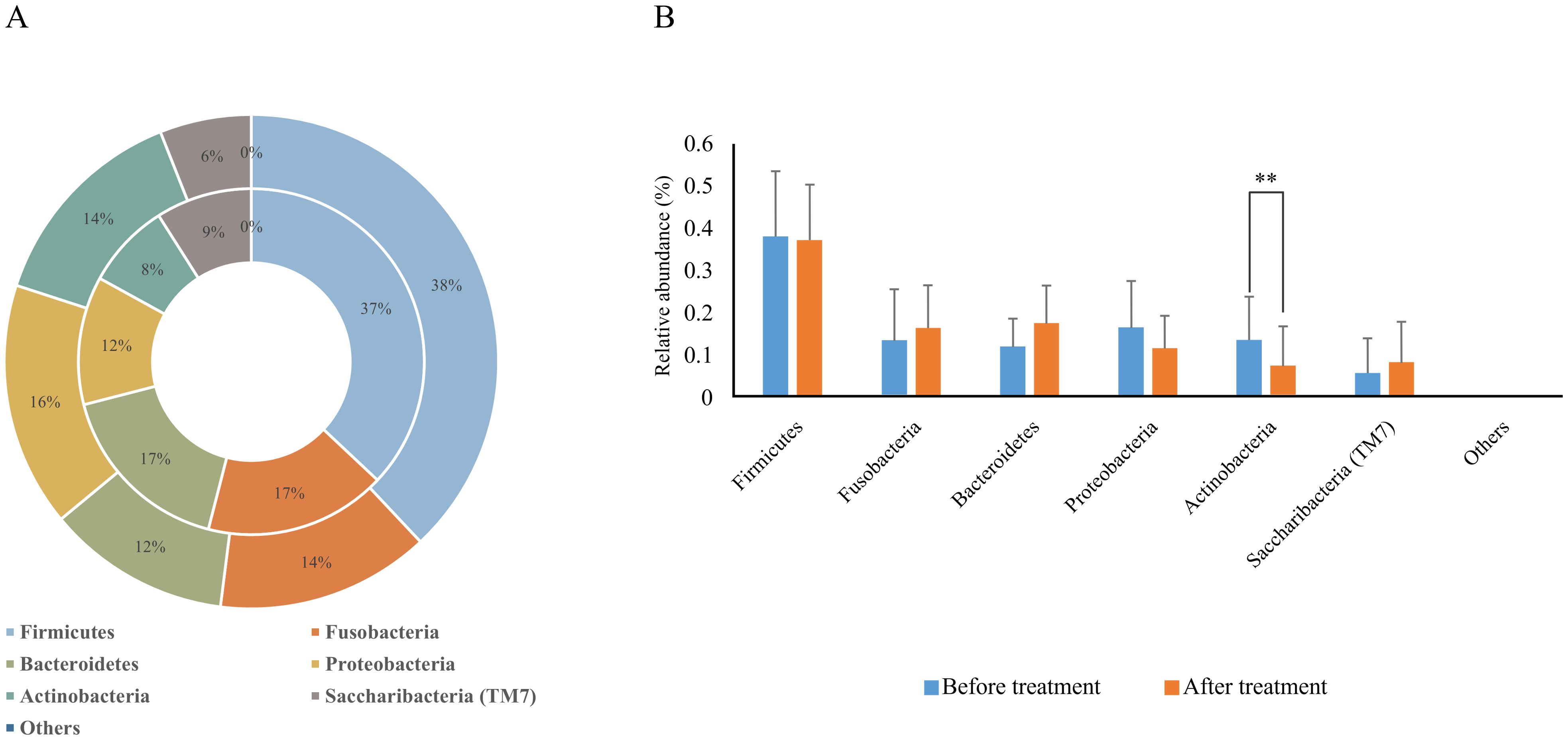
Figure 3. Relative abundance of dominant bacterial phyla in supragingival plaque samples collected from 20 children diagnosed with S-ECC before and one month after comprehensive dental treatment under general anesthesia. (A) Taxonomic composition at the phylum level is shown. The outer circle represents before-treatment samples and the inner circle represents after-treatment samples. (B) Bar plots of mean relative abundance (%) of each dominant phylum. **P < 0.01.

Table 3. Comparison of relative abundance of bacterial phyla in supragingival plaque before and after treatment.
Analysis of genus-level differences in microbial composition
Before treatment, 85 genera were detected, with those having a relative abundance > 1% being, in descending order, Streptococcus (14.5%), Leptothrix (9.8%), Lunomonas (8.9%), Veillonella (8.0%) and Neisseria (7.5%), Candidate_division_TM7_norank (5.9%), Corynebacterium (5.2%), Prevotella (5.0%), Actinobacillus (4.7%), Fibrophagocytophages (4.4%), Fusobacterium (3.7%), Roxella (3.4%), Lautropia (3.1%), Porphyromonas (2.2%), Haemophilus (2.2%), and Centipeda (1.4%); After treatment, 86 genera were detected, with the relative abundance > 1% being Leptothrix (11.1%), Veillonella (10.7%), Lunomonas (10.3%), Prevotella (9.5%), Candidate_division_TM7_norank (9.0%), Streptococcus (8.8%), Clostridium (5.6%), Capnophage (5.1%), Neisseria (3.8%), Corynebacterium (3.3%), Actinobacillus (3.0%), Porphyromonas (2.0%), Centipeda (2.0%), Lautropia (1.5%), Campylobacter (1.2%), and Hemophilus (1.2%). Post-comprehensive treatment, four genera with a relative abundance > 1% showed significant changes (P < 0.05). The relative abundance of Streptococcus decreased from 14.5% before treatment to 8.8% after, Neisseria from 7.5% to 3.8%, Rothia from 3.4% to 0.8%, while Prevotella increased from 5.0% to 9.5%. No significant differences were observed in the relative abundance of other bacteria genera before and after treatment (P > 0.05) (Figure 4, Table 4).
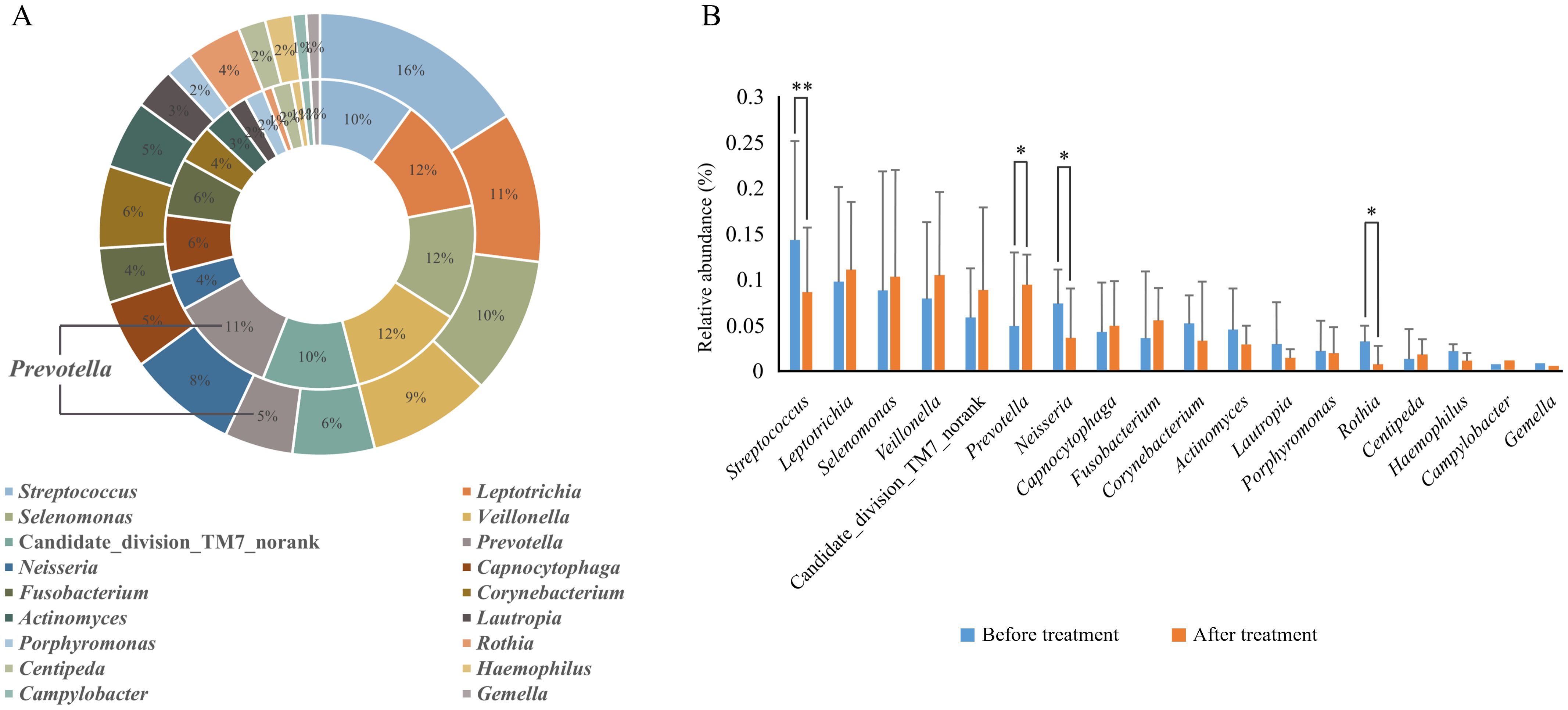
Figure 4. Relative abundance of dominant bacterial genera (> 1% in average relative abundance) in supragingival plaque samples collected from 20 children diagnosed with S-ECC before and one month after comprehensive dental treatment under general anesthesia. (A) Taxonomic composition at the genus level is shown. The outer circle represents before-treatment samples and the inner circle represents after-treatment samples. (B) Bar plots of mean relative abundance (%) of each dominant genus. *P < 0.05 or **P < 0.01.
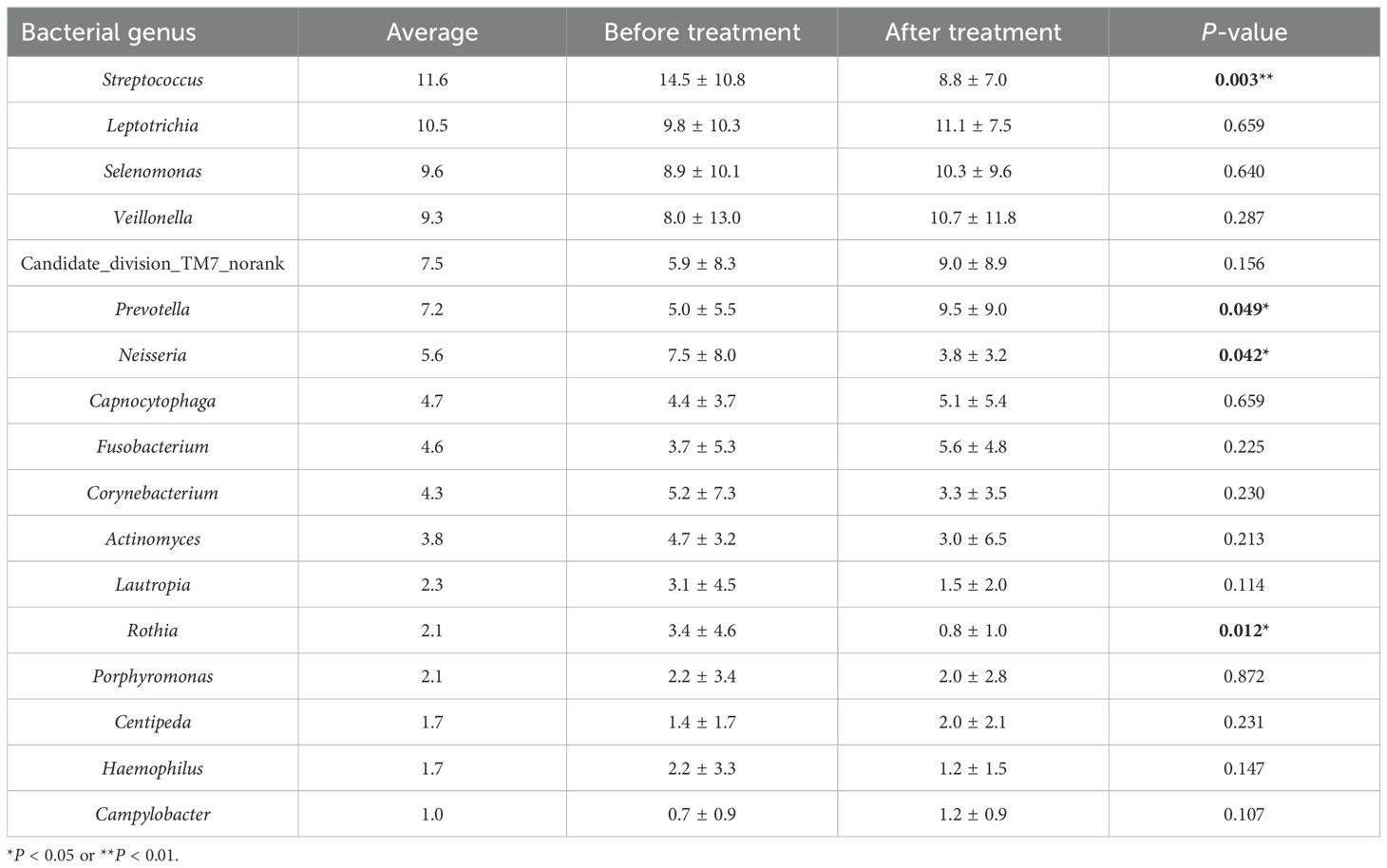
Table 4. Comparison of relative abundance of bacterial genera (> 1% in average relative abundance) in supragingival plaque before and after treatment.
Discussion
ECC, particularly S-ECC, is a biofilm-mediated condition that poses significant challenges in pediatric dentistry. S-ECC can lead to severe complications in primary dentition, such as pulp and periapical diseases, potentially affecting the development of permanent teeth. Comprehensive dental treatment under general anesthesia offers an effective solution for S-ECC by removing carious lesions in a single session, thus addressing both the immediate dental needs and anxiety-related challenges faced by young patients. However, reports on the microbial community shifts within dental plaque biofilms pre- and post-comprehensive treatment under general anesthesia in children with S-ECC are scarce.
Our study employed metagenomics to analyze the microbial dynamics of dental plaque in children with S-ECC, pre- and post-comprehensive treatment under general anesthesia. Post-comprehensive treatment, we observed a significant increase in microbial diversity, as evidenced by increases in the Chao1, and Shannon index, aligning with previous findings by Li et al. (2007) and Tao et al. (2013). This enrichment in microbial diversity post-comprehensive treatment mirrors the microecological equilibrium found in caries-free children, suggesting that comprehensive treatment positively influences oral microbial ecology.
Our results suggest that the relative abundance of the Actinobacteria phylum significantly decreases post-comprehensive treatment, implying a potential inverse correlation with oral health, consistent with Tang et al.’s findings (Tang et al., 2003). Actinomyces and Streptococcus have been shown to exhibit synergistic interactions in the early stages of carious development (Sarkonen et al., 2000), where the cariogenic role of Actinobacteria is to promote caries by altering the microecological balance. At the genus level, post-comprehensive treatment analysis indicated a significant reduction in the relative abundance of genera Streptococcus, Rothia, and Neisseria, which may be positively correlated with the progression of caries. Streptococcus mutans and Streptococcus sobrinus within the Streptococcus genus are recognized as primary etiological agents of S-ECC (Veena and Nagarathna, 2020), with a higher caries rate in children harboring both species compared to those with only S. mutans (Zhou et al., 2011). Rothia species’ prevalence and activity within carious lesions (Belda-Ferre et al., 2015), and their association with dentine caries and subgingival biofilm formation in anaerobic conditions (Jagathrakshakan et al., 2015), support their relevance in caries pathology. The decline in Neisseria post-comprehensive treatment suggests a potential role in S-ECC’s pathogenesis, warranting further investigation.
Additionally, the relative abundance of Prevotella significantly increased post-comprehensive treatment in this study. While Prevotella is a common and abundant genus in the oral cavity, its role in oral health and disease remains complex and species-dependent. Previous studies have indicated that elevated levels of Prevotella in individuals with active caries, suggesting that certain species within this genus may contribute to dysbiosis and disease progression (Kanasi et al., 2010; Tanner et al., 2011). However, other Prevotella species, such as Prevotella melaninogenica and Prevotella histicola, are frequently found in the healthy oral microbiome and may contribute to maintaining ecological balance (Jiang et al., 2016; He et al., 2018). Therefore, the increased abundance of Prevotella in our study may not directly imply a heightened risk of caries recurrence, but could reflect a shift toward greater microbial diversity and ecological restructuring post-comprehensive treatment. It is also important to consider that this change may be influenced by inter-individual variability in factors such as diet, oral hygiene, and lifestyle, as well as the relatively small sample size of the present study. Further species-level and functional analyses are needed to determine whether the increase in Prevotella represents a beneficial reestablishment of microbial homeostasis or serves as an early microbial indicator of caries risk.
The research conducted by Klinke et al. (2014) indicates that while the abundance of cariogenic bacteria significantly decreases following caries treatment, this reduction may be transient, with the potential for these taxa to rebound over time. In our study, we observed significant shifts in the microbial composition one month after comprehensive dental treatment under general anesthesia, suggesting an initial positive response in terms of microbial diversity. However, due to the dynamic nature of oral microbial communities, influenced continuously by environmental factors, dietary habits, and oral hygiene practices, the long-term stability and durability of these treatment-related microbial shifts remain unclear and warrant further investigation. Additionally, the interpretation of our findings is constrained by several limitations, including a small sample size, potentially compromising statistical power and the generalizability of the results. Furthermore, our sequencing approach was limited to genus-level identification, thus precluding a deeper understanding of species-specific shifts that might provide clearer insights into cariogenic or health-related microbial dynamics. Future research incorporating larger cohorts, extended follow-up periods (e.g., 3 to 6 months or longer), and species-level metagenomic analyses will be necessary to better elucidate the sustained effects of comprehensive dental treatment on oral microbial homeostasis and the clinical relevance of these microbial changes.
In conclusion, significant changes in microbial diversity and composition are observed in the dental plaque biofilms of children with S-ECC following comprehensive dental treatment under general anesthesia. The increase in microbial diversity and the concomitant reduction in cariogenic microorganisms of post-comprehensive treatment indicate a shift toward a healthier, more balanced, and stable microbial state. These findings highlight the potential of comprehensive dental treatment in promoting a favorable shift in oral microbiota, emphasizing its theoretical and practical implications in the management of S-ECC from a microbiological perspective.
Data availability statement
The original contributions presented in the study are included in the article/supplementary material. Further inquiries can be directed to the corresponding authors.
Ethics statement
The studies involving humans were approved by Ethics Committee of West China Hospital of Stomatology, Sichuan University (WCHSIRB-D-2014-071-R1). The studies were conducted in accordance with the local legislation and institutional requirements. Written informed consent for participation in this study was provided by the participants’ legal guardians/next of kin.
Author contributions
XW: Conceptualization, Formal Analysis, Investigation, Methodology, Visualization, Writing – original draft. HH: Data curation, Software, Validation, Writing – review & editing. QM: Methodology, Project administration, Supervision, Writing – review & editing. JZ: Funding acquisition, Project administration, Resources, Writing – review & editing.
Funding
The author(s) declare that financial support was received for the research and/or publication of this article. This work was supported by grants from the National Natural Science Foundation of China (82170947 to JZ; 32400094 to QM), the Natural Science Foundation of Sichuan Province (2025ZNSFSC0771 to QM), the Fundamental Research Funds for the Central Universities, Research and Develop Program, West China Hospital of Stomatology Sichuan University (RD-02-202402 to QM), and the Research Funding from West China School/Hospital of Stomatology Sichuan University (RCDWJS2023-10 to QM).
Conflict of interest
The authors declare that the research was conducted in the absence of any commercial or financial relationships that could be construed as a potential conflict of interest.
Generative AI statement
The author(s) declare that no Generative AI was used in the creation of this manuscript.
Publisher’s note
All claims expressed in this article are solely those of the authors and do not necessarily represent those of their affiliated organizations, or those of the publisher, the editors and the reviewers. Any product that may be evaluated in this article, or claim that may be made by its manufacturer, is not guaranteed or endorsed by the publisher.
References
Becker, M. R., Paster, B. J., Leys, E. J., Moeschberger, M. L., Kenyon, S. G., Galvin, J. L., et al. (2002). Molecular analysis of bacterial species associated with childhood caries. J. Clin. Microbiol 40, 1001–1009. doi: 10.1128/JCM.40.3.1001-1009.2002
Belda-Ferre, P., Williamson, J., Simón-Soro, Á., Artacho, A., Jensen, O. N., Mira, A. (2015). The human oral metaproteome reveals potential biomarkers for caries disease. Proteomics 15, 3497–3507. doi: 10.1002/pmic.201400600
Blostein, F., Bhaumik, D., Davis, E., Salzman, E., Shedden, K., Duhaime, M., et al. (2022). Evaluating the ecological hypothesis: early life salivary microbiome assembly predicts dental caries in a longitudinal case-control study. Microbiome 10, 240. doi: 10.1186/s40168-022-01442-5
Chen, W., Jiang, Q., Yan, G., Yang, D. (2020). The oral microbiome and salivary proteins influence caries in children aged 6 to 8 years. BMC Health 20, 295. doi: 10.1186/s12903-020-01262-9
Clark, M. B., Braun, P. A. (2021). Promotion of oral health and prevention of dental caries among children in primary care. JAMA 326, 2139–2140. doi: 10.1001/jama.2021.20396
Folayan, M. O., Idehen, E. E., Ojo, O. O. (2004). The modulating effect of culture on the expression of dental anxiety in children: a literature review. Int. J. Paediatr. Dent. 14, 241–245. doi: 10.1111/j.1365-263X.2004.00563.x
Fouts, D. E., Szpakowski, S., Purushe, J., Torralba, M., Waterman, R. C., MacNeil, M. D., et al. (2012). Next generation sequencing to define prokaryotic and fungal diversity in the bovine rumen. PloS One 7, e48289. doi: 10.1371/journal.pone.0048289
Gao, L., Xu, T., Huang, G., Jiang, S., Gu, Y., Chen, F. (2018). Oral microbiomes: more and more importance in oral cavity and whole body. Protein Cell 9, 488–500. doi: 10.1007/s13238-018-0548-1
Garcia, B. A., Acosta, N. C., Tomar, S. L., Roesch, L. F. W., Lemos, J. A., Mugayar, L. R. F., et al. (2021). Association of Candida albicans and Cbp+ Streptococcus mutans with early childhood caries recurrence. Sci. Rep. 11, 10802. doi: 10.1038/s41598-021-90198-3
He, J., Tu, Q., Ge, Y., Qin, Y., Cui, B., Hu, X., et al. (2018). Taxonomic and functional analyses of the supragingival microbiome from caries-affected and caries-free hosts. Microb Ecol. 75, 543–554. doi: 10.1007/s00248-017-1056-1
Hurley, E., Barrett, M. P. J., Kinirons, M., Whelton, H., Ryan, C. A., Stanton, C., et al. (2019). Comparison of the salivary and dentinal microbiome of children with severe-early childhood caries to the salivary microbiome of caries-free children. BMC Health 19, 13. doi: 10.1186/s12903-018-0693-1
Jagathrakshakan, S. N., Sethumadhava, R. J., Mehta, D. T., Ramanathan, A. (2015). 16S rRNA gene-based metagenomic analysis identifies a novel bacterial co-prevalence pattern in dental caries. Eur. J. Dent. 9, 127–132. doi: 10.4103/1305-7456.149661
Jakubovics, N. S., Goodman, S. D., Mashburn-Warren, L., Stafford, G. P., Cieplik, F. (2021). The dental plaque biofilm matrix. Periodontol 2000 86, 32–56. doi: 10.1111/prd.12361
Jiang, S., Gao, X., Jin, L., Lo, E. C. M. (2016). Salivary microbiome diversity in caries-free and caries-affected children. Int. J. Mol. Sci. 17, 1978. doi: 10.3390/ijms17121978
Kanasi, E., Johansson, I., Lu, S. C., Kressin, N. R., Nunn, M. E., Kent, R., et al. (2010). Microbial risk markers for childhood caries in pediatricians’ offices. J. Dent. Res. 89, 378–383. doi: 10.1177/0022034509360010
Klinke, T., Urban, M., Lück, C., Hannig, C., Kuhn, M., Krämer, N. (2014). Changes in Candida spp., mutans streptococci and lactobacilli following treatment of early childhood caries: a 1-year follow-up. Caries Res. 48, 24–31. doi: 10.1159/000351673
Kries, T., Rupf, S., Hannig, M., Naim, J., Gund, M. P. (2023). The impact of general medical health status, demographical, and patient-specific variables on need for dental treatment of children and adolescents under general anesthesia. Clin. Invest. 27, 4245–4257. doi: 10.1007/s00784-023-05041-y
Lamont, R. J., Koo, H., Hajishengallis, G. (2018). The oral microbiota: dynamic communities and host interactions. Nat. Rev. Microbiol 16, 745–759. doi: 10.1038/s41579-018-0089-x
Lemos, J. A., Palmer, S. R., Zeng, L., Wen, Z. T., Kajfasz, J. K., Freires, I. A., et al. (2019). The biology of Streptococcus mutans. Microbiol Spectr. 7. doi: 10.1128/microbiolspec.GPP3-0051-2018
Li, Y., Ge, Y., Saxena, D., Caufield, P. W. (2007). Genetic profiling of the oral microbiota associated with severe early-childhood caries. J. Clin. Microbiol 45, 81–87. doi: 10.1128/JCM.01622-06
Li, Y., Huang, S., Du, J., Wu, M., Huang, X. (2023). Current and prospective therapeutic strategies: tackling Candida albicans and Streptococcus mutans cross-kingdom biofilm. Front. Cell Infect. Microbiol 13. doi: 10.3389/fcimb.2023.1106231
Li, Y., Ku, C. Y. S., Xu, J., Saxena, D., Caufield, P. W. (2005). Survey of oral microbial diversity using PCR-based denaturing gradient gel electrophoresis. J. Dent. Res. 84, 559–564. doi: 10.1177/154405910508400614
Quast, C., Pruesse, E., Yilmaz, P., Gerken, J., Schweer, T., Yarza, P., et al. (2013). The SILVA ribosomal RNA gene database project: improved data processing and web-based tools. Nucleic Acids Res. 41, D590–D596. doi: 10.1093/nar/gks1219
Rosier, B. T., Marsh, P. D., Mira, A. (2018). Resilience of the oral microbiota in health: mechanisms that prevent dysbiosis. J. Dent. Res. 97, 371–380. doi: 10.1177/0022034517742139
Santonocito, S., Giudice, A., Polizzi, A., Troiano, G., Merlo, E. M., Sclafani, R., et al. (2022). A cross-talk between diet and the oral microbiome: balance of nutrition on inflammation and immune system’s response during periodontitis. Nutrients 14, 2426. doi: 10.3390/nu14122426
Sarkonen, N., Könönen, E., Summanen, P., Kanervo, A., Takala, A., Jousimies-Somer, H. (2000). Oral colonization with Actinomyces species in infants by two years of age. J. Dent. Res. 79, 864–867. doi: 10.1177/00220345000790031301
Schmoeckel, J., Gorseta, K., Splieth, C. H., Juric, H. (2020). How to intervene in the caries process: early childhood caries - a systematic review. Caries Res. 54, 102–112. doi: 10.1159/000504335
Sedghi, L., DiMassa, V., Harrington, A., Lynch, S. V., Kapila, Y. L. (2021). The oral microbiome: Role of key organisms and complex networks in oral health and disease. Periodontol 2000 87, 107–131. doi: 10.1111/prd.12393
Seligman, L. D., Hovey, J. D., Chacon, K., Ollendick, T. H. (2017). Dental anxiety: An understudied problem in youth. Clin. Psychol. Rev. 55, 25–40. doi: 10.1016/j.cpr.2017.04.004
Selwitz, R. H., Ismail, A. I., Pitts, N. B. (2007). Dental caries. Lancet 369, 51–59. doi: 10.1016/S0140-6736(07)60031-2
Suez, J., Cohen, Y., Valdés-Mas, R., Mor, U., Dori-Bachash, M., Federici, S., et al. (2022). Personalized microbiome-driven effects of non-nutritive sweeteners on human glucose tolerance. Cell 185, 3307–3328.e19. doi: 10.1016/j.cell.2022.07.016
Tang, G., Yip, H. K., Samaranayake, L. P., Luo, G., Lo, E. C. M., Teo, C. S. (2003). Actinomyces spp. in supragingival plaque of ethnic Chinese preschool children with and without active dental caries. Caries Res. 37, 381–390. doi: 10.1159/000072172
Tanner, A. C. R., Mathney, J. M. J., Kent, R. L., Chalmers, N. I., Hughes, C. V., Loo, C. Y., et al. (2011). Cultivable anaerobic microbiota of severe early childhood caries. J. Clin. Microbiol 49, 1464–1474. doi: 10.1128/JCM.02427-10
Tao, Y., Zhou, Y., Ouyang, Y., Lin, H. (2013). Dynamics of oral microbial community profiling during severe early childhood caries development monitored by PCR-DGGE. Arch. Biol. 58, 1129–1138. doi: 10.1016/j.archoralbio.2013.04.005
Valm, A. M. (2019). The structure of dental plaque microbial communities in the transition from health to dental caries and periodontal disease. J. Mol. Biol. 431, 2957–2969. doi: 10.1016/j.jmb.2019.05.016
Veena, R. L., Nagarathna, C. (2020). Correlation of Streptococcus mutans and Streptococcus sobrinus colonization with and without caries experience in preschool children. Indian J. Dent. Res. 31, 73–79. doi: 10.4103/ijdr.IJDR_432_18
Xiao, J., Alkhers, N., Kopycka-Kedzierawski, D. T., Billings, R. J., Wu, T. T., Castillo, D. A., et al. (2019). Prenatal oral health care and early childhood caries prevention: a systematic review and meta-analysis. Caries Res. 53, 411–421. doi: 10.1159/000495187
Xiao, J., Fiscella, K. A., Gill, S. R. (2020). Oral microbiome: possible harbinger for children’s health. Int. J. Sci. 12, 12. doi: 10.1038/s41368-020-0082-x
Zhou, Q., Qin, X., Qin, M., Ge, L. (2011). Genotypic diversity of Streptococcus mutans and Streptococcus sobrinus in 3-4-year-old children with severe caries or without caries. Int. J. Paediatr. Dent. 21, 422–431. doi: 10.1111/j.1365-263X.2011.01145.x
Keywords: severe early childhood caries, comprehensive dental treatment, high-throughput sequencing, microecological balance, dental plaque
Citation: Wang X, Huang H, Ma Q and Zou J (2025) Microbial shifts in dental plaque of children with severe early childhood caries following comprehensive dental treatment under general anesthesia. Front. Cell. Infect. Microbiol. 15:1541785. doi: 10.3389/fcimb.2025.1541785
Received: 14 January 2025; Accepted: 04 April 2025;
Published: 02 May 2025.
Edited by:
Chun-Teh Lee, University of Texas Health Science Center at Houston, United StatesReviewed by:
Shensheng Gu, Shanghai Jiao Tong University, ChinaHanan Oubenyahya, Military Hospital, Morocco
Fang Yang, Qingdao Municipal Hospital, China
Copyright © 2025 Wang, Huang, Ma and Zou. This is an open-access article distributed under the terms of the Creative Commons Attribution License (CC BY). The use, distribution or reproduction in other forums is permitted, provided the original author(s) and the copyright owner(s) are credited and that the original publication in this journal is cited, in accordance with accepted academic practice. No use, distribution or reproduction is permitted which does not comply with these terms.
*Correspondence: Qizhao Ma, bXF6MjA4MEBzY3UuZWR1LmNu; Jing Zou, em91amluZ0BzY3UuZWR1LmNu