- Department of Andrology, The First Affiliated Hospital of Hunan University of Chinese Medicine, Changsha, China
Prostate cancer (PCa) is one of the most prevalent malignancies among men, with its incidence steadily increasing worldwide. Recent advances in microbiome research have opened new avenues for understanding and treating PCa; however, studies focusing specifically on the prostate tissue microbiome remain limited. Evidence suggests that the microbial communities within PCa tissues exhibit significant diversity and regional variability, with certain bacteria potentially contributing to PCa initiation and progression through chronic inflammation. The prostate microbiome comprises not only bacteria but also viruses, fungi, and parasites, and its diversity is influenced by a complex interplay of genetic, environmental, and lifestyle factors. Methodological limitations and sample contamination further complicate the interpretation of microbiome data. The urinary microbiome is similarly diverse and shaped by multiple overlapping influences. Although urine, prostatic fluid, and prostate tissue are anatomically and functionally connected, whether urine and prostatic fluid can accurately reflect the prostate tissue microbiome remains to be conclusively determined. Among the microorganisms detected, Cutibacterium acnes is frequently identified in prostate tissue, urine, and prostatic fluid from PCa patients. This bacterium is known to elicit inflammatory responses through various pathways, potentially impacting tumorigenesis and cancer progression. Nevertheless, findings across studies remain inconsistent. Further research is necessary to elucidate the underlying mechanisms by which the microbiome influences PCa. Such efforts may offer novel insights and strategies for the diagnosis, treatment, and prevention of this disease.
1 Introduction
1.1 Contemporary clinical settings and treatment approaches
Prostate cancer (PCa) is one of the most prevalent malignancies in men, with a steadily increasing global incidence. According to recent statistics, PCa currently ranks as the most commonly diagnosed malignancy among men in the United States (Miller et al., 2022). Mutations in the androgen receptor (AR) gene are a hallmark of disease progression, with nearly all patients eventually developing castration-resistant prostate cancer (CRPC). At advanced stages, bone metastases frequently occur, significantly impairing patients’ quality of life (Coleman et al., 2020; Tilki et al., 2024). The underlying mechanisms of PCa bone metastasis remain incompletely understood. Tumor-derived factors such as extracellular vesicles, inflammatory chemokines, and interactions between cancer cells and the extracellular matrix (ECM) are believed to contribute to metastatic progression. Pain and skeletal-related events, particularly fractures, are major determinants of decreased quality of life in affected patients (Kang et al., 2022). Current European guidelines recommend a personalized, risk-adapted approach to PCa screening and treatment. Risk stratification plays a central role in determining appropriate therapeutic strategies, which may include active surveillance, radical prostatectomy, androgen deprivation therapy, radiotherapy, and immunotherapy (Adamo et al., 2024; Cornford et al., 2024; Kumar Am et al., 2024). Multidisciplinary collaboration is emphasized to enhance treatment efficacy and improve patient prognosis. Despite these advancements, significant challenges remain. High recurrence rates and treatment resistance continue to limit long-term outcomes (Almeeri et al., 2024). These challenges underscore the complexity of PCa and highlight the need for more comprehensive and patient-centered multimodal treatment strategies aimed at improving both survival and quality of life (Ahmadzadehfar et al., 2024). In recent years, the role of the human microbiome in cancer biology has received growing attention. Preliminary evidence suggests that the microbiome may influence cancer initiation, progression, and metastasis (Fares et al., 2020; Herrera-Quintana et al., 2024). A deeper understanding of the interactions between the microbiome and PCa may provide novel insights and therapeutic targets, potentially leading to more effective treatment paradigms and better clinical outcomes.
1.2 The microbiome as a new frontier in precision cancer therapy
The human body hosts a diverse and dynamic array of microorganisms, including bacteria, viruses, fungi, and archaea, collectively referred to as the microbiome (Zuber et al., 2023). This complex ecosystem plays a crucial role in maintaining physiological homeostasis, and its composition is highly individualized and shaped by genetic, environmental, and lifestyle factors over time. In recent years, the microbiome has emerged as a promising area of research in the field of precision oncology (El Tekle and Garrett, 2023). In the context of PCa, microbiome research primarily focuses on two domains: the microbiomes of prostate tissue and urine, and those of the oral cavity and gastrointestinal tract (Pernigoni et al., 2023; Shyanti et al., 2024). While studies on the oral and gut microbiomes are relatively abundant, investigations into the microbial communities in prostate tissue and urine remain limited. In particular, evidence concerning the prostate tissue microbiome is still insufficient and warrants further exploration (Munteanu et al., 2023). The oral and gut microbiomes represent highly complex microbial environments that influence host immune responses, metabolic regulation, and systemic inflammation (Helmink et al., 2019). Strong evidence linking gut microbiota dysbiosis to cancer development is primarily concentrated in colorectal cancer (Tilg et al., 2018). Specific bacterial species have been identified as contributors to colorectal tumorigenesis, with microbial imbalance disrupting the equilibrium between pro-tumorigenic pathogens and anti-tumor commensals (Wong and Yu, 2023). Emerging research suggests that alterations in the gut microbiome may also play a role in the pathogenesis and therapeutic responsiveness of PCa. These effects are thought to be mediated through mechanisms involving modulation of androgen metabolism, immune regulation, and intestinal barrier integrity (Romano et al., 2024; Zha et al., 2023). Therefore, targeting the gut microbiome and its metabolites represents a novel and potentially transformative strategy for the diagnosis and treatment of prostate-related diseases.
Unlike the gut microbiome, the urinary tract microbiome primarily comprises microbial communities in the prostate tissue and urine (Tsai et al., 2022). Although direct evidence linking these microbiomes to clinical outcomes in PCa is currently limited compared to the extensive research on the gut microbiome (Mjaess et al., 2023), emerging studies suggest their potential roles in modulating cancer treatment resistance and immune responses within the tumor microenvironment. For example, tumor-resident Proteobacteria have been shown to alter the metabolism of chemotherapeutic agents such as gemcitabine, thereby contributing to drug resistance in pancreatic cancer (Geller et al., 2017). Moreover, intratumoral microbiota can both enhance and suppress host immune responses, ultimately influencing the efficacy of immunotherapies (Pushalkar et al., 2018; Zheng et al., 2017). Given these findings, in-depth investigation into the prostate tissue and urine microbiomes is essential to unravel the complex interactions between microbial populations and PCa pathophysiology. A conceptual framework of these interactions and potential regulatory mechanisms is illustrated in Figure 1. Such research may pave the way for novel therapeutic strategies. Promising avenues include antibiotic regimens, fecal microbiota transplantation, immunotherapy, and molecular targeted therapies (Dicks and Vermeulen, 2022; Rizzo et al., 2022).
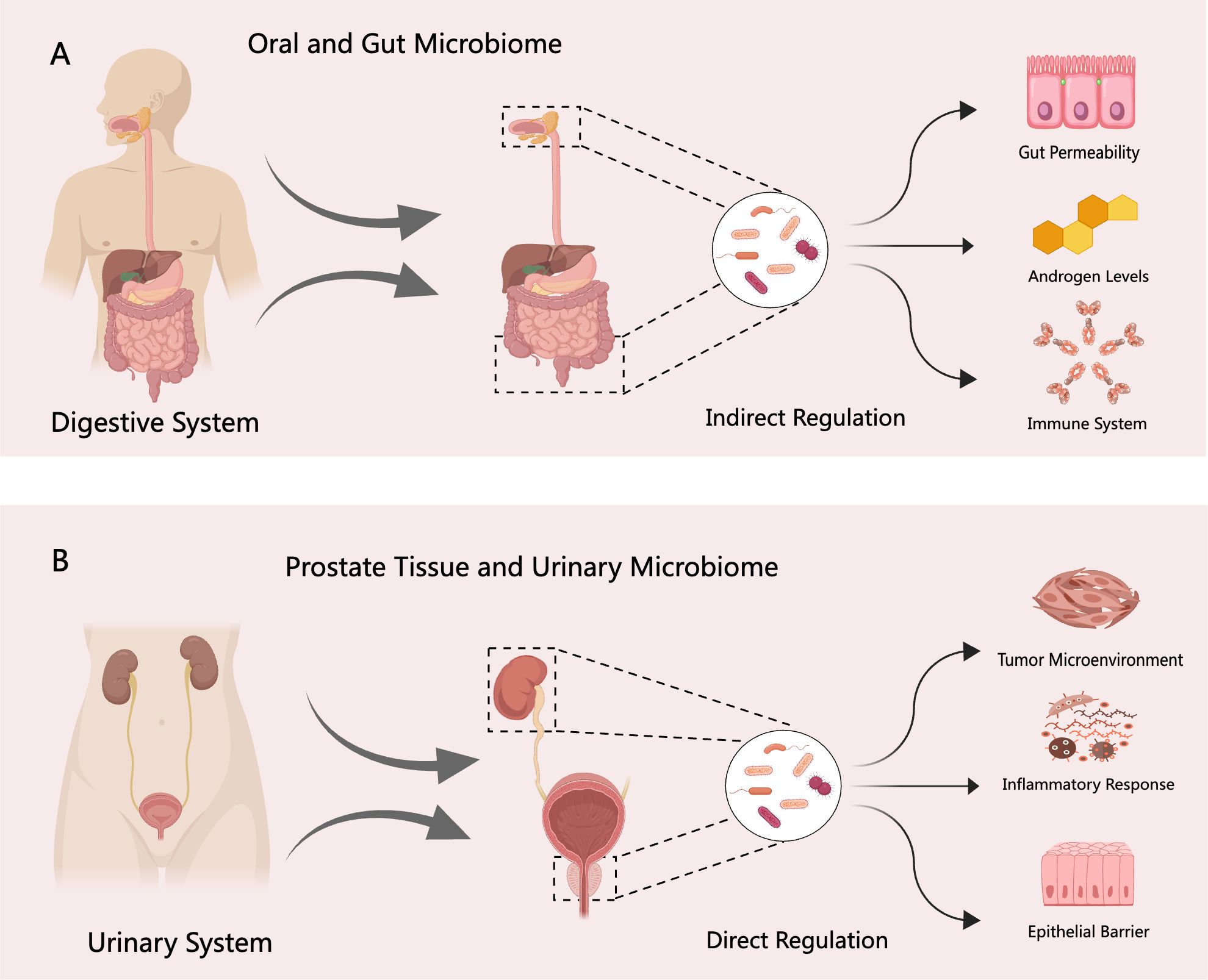
Figure 1. Regulatory mechanisms of the digestive and urinary system microbiomes in PCa. (A) The digestive system microbiome—including oral and gut microbial communities—may indirectly influence the initiation and progression of PCa through several pathways, such as disruption of the intestinal barrier, modulation of androgen levels, and regulation of host immune responses. (B) In contrast, the urinary system microbiome—comprising prostate tissue and urinary microbial communities—may directly contribute to PCa pathogenesis by altering the tumor microenvironment, inducing inflammatory responses, and impairing epithelial barrier integrity.
2 Diversity and regional differences of the PCa microbiome
2.1 The relationship between bacteria and inflammation in PCa
Growing evidence suggests that the prostate microbiota plays a potentially significant role in the initiation, progression, and prognosis of PCa within the tumor microenvironment (Ohadian Moghadam and Momeni, 2021). While the use of bacteria from the tumor microenvironment for targeted therapy holds promise, comprehensive experimental validation is still lacking (Che et al., 2021). Historically, the prostate was believed to be a sterile organ; however, subsequent studies have challenged this notion (Krieger et al., 2000; Leskinen et al., 2003). Although bacteria are typically absent in prostate tissues from healthy individuals, both bacterial presence and inflammation have been observed in radical prostatectomy specimens. These findings suggest that microbial colonization may contribute to localized inflammatory microenvironments, potentially playing a role in prostate pathology (Hochreiter et al., 2000).
Prostate inflammation can arise from various sources, including microbial infections (e.g., sexually transmitted pathogens), cellular injury (e.g., urine reflux, prostatic calculi), hormonal fluctuations, and environmental exposures (De Marzo et al., 2007). These stimuli may trigger local tissue damage and immune activation, resulting in a breakdown of immune tolerance to self-antigens within the prostate. Chronic inflammation, in turn, may foster a microenvironment conducive to carcinogenesis. Early investigations using 16S rRNA gene sequencing revealed that bacterial species detected in localized PCa were not specific to chronic prostatitis (Keay et al., 1999). Subsequently, Banarjee et al. employed microarray-based metagenomic analysis and found no statistically significant differences in microbial composition between PCa and benign prostatic hyperplasia (BPH) patients (Banerjee et al., 2019). Similarly, Feng et al., using an integrated approach combining metagenomics and metatranscriptomics, reported no clear association between the prostate microbiome and metastatic progression in PCa (Feng et al., 2019b).
However, recent studies have presented alternative perspectives regarding the role of the prostate microbiome in PCa. Ma et al. were the first to utilize microbial data from TCGA to comprehensively investigate the potential pro-tumorigenic and anti-tumorigenic roles of the prostate microbiota in PCa (Ma et al., 2020). Their analysis revealed a correlation between thermophilic bacteria and Streptococcus pneumoniae with PSA levels, suggesting a potential involvement in the inflammatory microenvironment of PCa. Additionally, several bacterial taxa were negatively associated with Gleason scores, TNM staging, and AR expression. These findings were further supported by the work of Guner et al., who demonstrated that chronic inflammation of the prostate, associated with microbial presence, was linked to a higher risk of Gleason score upgrading and increased tumor aggressiveness (Guner et al., 2021).
While microbial infections may indeed contribute to inflammation within the prostate microenvironment, it is important to recognize that prostate inflammation is a multifactorial process. The direct relationship between the prostate microbiome and inflammation remains insufficiently characterized (Sfanos et al., 2018). Moreover, certain bacterial sequences may exist within prostate tissues that are not detectable through conventional culture methods or standard sequencing technologies, making accurate identification challenging (Riley et al., 1998). Recent evidence has also highlighted associations between pathogenic microbiota—particularly those involved in urinary tract infections (UTIs) and vesicoureteral reflux—and PCa development (Prakash et al., 2024). The prostate microbiome, which originates from factors such as urinary tract infections and local trauma, may contribute to the progression of prostate cancer (PCa) through the induction of localized inflammatory responses (Picardo et al., 2019). The interaction between microbiome-induced inflammation and the pathogenesis of PCa is illustrated in Figure 2.
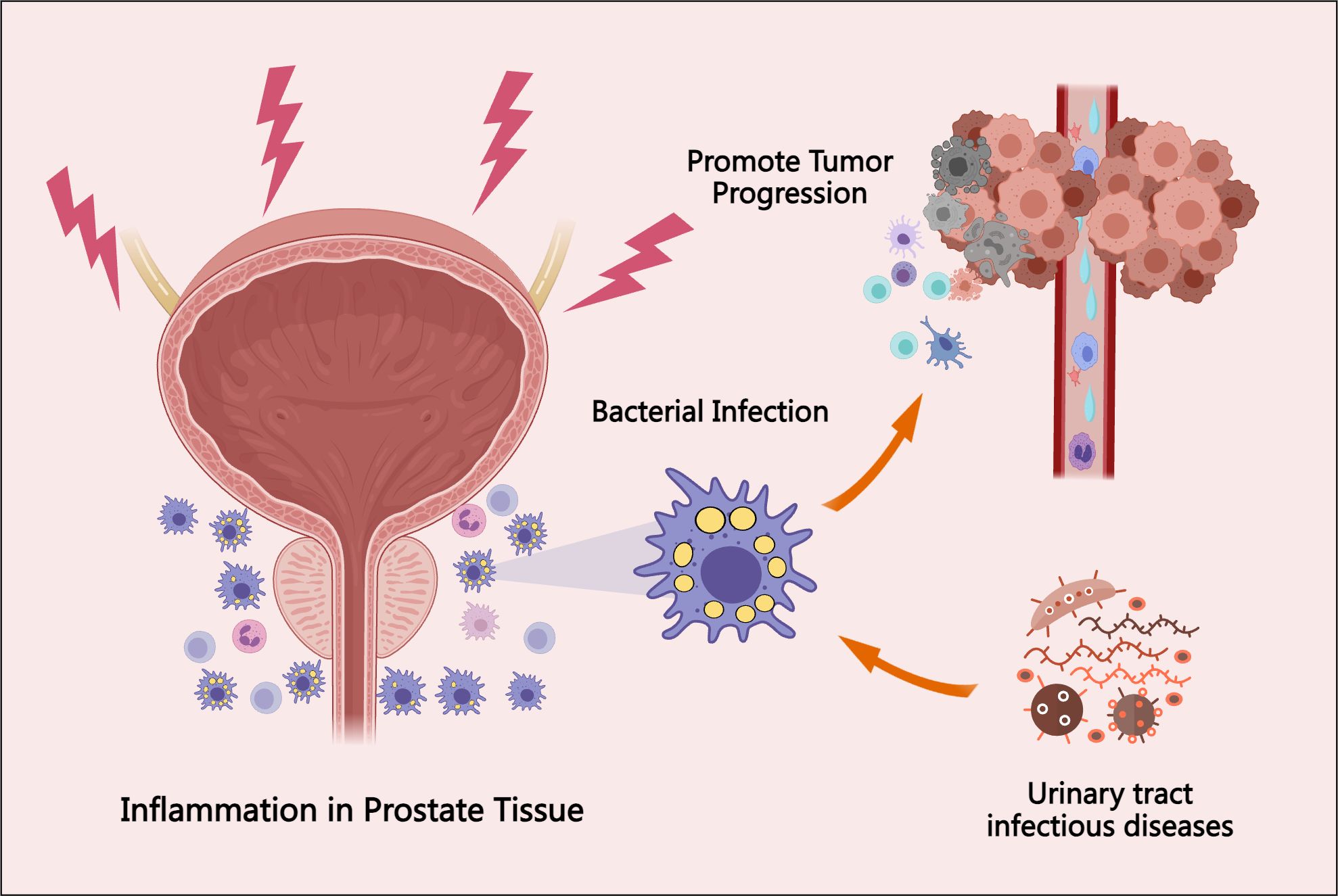
Figure 2. The association between prostate tissue bacterial infection, inflammatory responses, and PCa progression. Bacterial infections in the prostate may originate from urinary tract infections, local tissue injury, hormonal fluctuations, and environmental exposures. These infections elicit localized inflammatory responses, which can promote tumor initiation and progression. This figure highlights the involvement of the prostate microbiome in the underlying mechanisms and pathophysiological processes of PCa.
2.2 Bacterial community composition and differential analysis in PCa tissues
As sequencing technologies have advanced, whole-genome and metagenomic sequencing based on next-generation sequencing (NGS) platforms are increasingly employed to investigate the composition and variations of prostate tissue microbiota between PCa patients and healthy controls (Lloréns-Rico et al., 2022). Although these techniques are now widely utilized in human microbiome studies, they present distinct limitations and technical challenges. For example, while 16S rRNA gene sequencing is cost-effective and widely accessible, it often fails to detect low-abundance microbial taxa, especially those that are difficult to culture under standard laboratory conditions. Additionally, many of these low-abundance species are underrepresented in metagenomic reference databases (Almeida et al., 2021). In contrast, metagenomics allows for comprehensive, unbiased sequencing of all genetic material within a sample, offering higher taxonomic resolution down to the species and even strain level. However, metagenomic analysis is more expensive, computationally demanding, and particularly susceptible to host DNA contamination—an issue that is especially pronounced in low-biomass environments such as the prostate and urinary tract (Wensel et al., 2022). Early studies using 16S rRNA sequencing technology provided initial insights into the prostate microbiome. Cohen et al. reported bacterial culture positivity in 56% of PCa tissue samples, identifying organisms such as C. acnes and coagulase-negative staphylococci (Cohen et al., 2005). Similarly, Sfanos et al. analyzed core prostate tissue samples from 30 cancer patients and found Escherichia spp., Acinetobacter spp., and Pseudomonas spp. to be the most frequently detected bacterial taxa (Sfanos et al., 2008). Notably, these bacteria were present in 95% of analyzed samples, but considerable regional heterogeneity in bacterial composition was observed among different cores from the same individual—potentially reflecting localized variations in the tissue microenvironment. Building on these findings, Yow et al. applied both 16S rRNA sequencing and total RNA sequencing to prostate tissue and identified Escherichia spp. and Cutibacterium acnes as predominant species, primarily from the Enterobacteriaceae family (Yow et al., 2017). Cavarretta et al. performed a comparative analysis of tumor, tumor-adjacent, and non-tumor prostate tissues, revealing a microbiome dominated by Actinobacteria, followed by Firmicutes and Proteobacteria (Cavarretta et al., 2017). At the genus level, Staphylococcus spp. were more abundant in tumor and adjacent tissues, whereas Streptococcus spp. were more prevalent in non-tumorous regions, suggesting intra-organ microbial heterogeneity that may be associated with PCa pathogenesis. Further supporting these observations, Banerjee et al. used microarray-based metagenomic analysis and found high relative abundances of Proteobacteria, Firmicutes, Actinobacteria, and Bacteroidetes in PCa tissues (Banerjee et al., 2019). These results were consistent with earlier findings by Sfanos and Yow. Feng et al., using an integrative approach combining metagenomics and metatranscriptomics, reported significant enrichment of Escherichia spp., Acinetobacter spp., Pseudomonas spp., and C. acnes in PCa tissues (Feng et al., 2019b). In a separate metatranscriptomic study, Salachan et al. confirmed the high relative abundance of Acinetobacter, Enterobacter, Streptococcus, and Escherichia in prostate tissue samples from PCa patients (Salachan et al., 2022).
Although these studies have shed light on the characteristics of the prostate microbiome in PCa patients, notable inconsistencies remain across the findings. For example, Salachan et al. reported a marked decrease in Bacteroides fragilis, Staphylococcus saprophyticus, and Vibrio parahaemolyticus, alongside an abnormal increase in Sphingomonas, further enriching the pool of potential microbial candidates associated with PCa progression (Salachan et al., 2022). In another study, Sarkar et al. utilized 16S rRNA sequencing to compare microbiome differences between patients with BPH and PCa (Sarkar et al., 2022). They found that Prevotella, Cupriavidus, and C. acnes were the most abundant bacterial genera in prostate lesions. LEfSe analysis identified Cupriavidus and Methylobacterium as significantly enriched in PCa samples, whereas Corynebacterium and Fibrobacter were more abundant in BPH samples. Gonçalves et al. also applied 16S rRNA sequencing and observed significant compositional differences between PCa and normal prostate samples, with lower microbial species richness detected in PCa tissues (Gonçalves et al., 2023). In PCa samples, Alistipes, Sutterella, Klebsiella, and Rothia were relatively enriched, while Actinomyces, Bacteroides, Prevotella, and Muribaculum predominated in normal tissues. However, contrasting results were reported by Kim et al., who found no significant differences in microbiome composition or diversity between PCa patients with biochemical recurrence and those without recurrence (Kim et al., 2023). Nevertheless, LEfSe analysis suggested a potential link between biochemical recurrence and the presence of Lactobacillus, which may represent a prognostic marker for PCa recurrence. In a follow-up study, Kim et al. expanded their analysis using 16S rRNA sequencing and reported that Proteobacteria, Bacteroidetes, and Firmicutes were the predominant phyla in PCa samples. At lower taxonomic levels, Bacteroidales, Clostridiales, Burkholderiales, and Rhizobiales were the most common bacterial orders, while Ruminococcaceae, Comamonadaceae, Rhizobiaceae, and Enterococcaceae were the most represented families (Kim et al., 2024). Other studies have yielded similar disparities. For example, Lee et al. found no significant differences in microbial diversity or composition between PCa patients and healthy controls using 16S rRNA sequencing (Lee et al., 2024). However, their analysis indicated that Sutterella, Escherichia, Delftia, and Gordonia were more abundant in normal prostate tissues, whereas Sphingomonas, Peptostreptococcus, Sphingobacterium, and Enterobacter were more prevalent in PCa tissues. Although various studies have identified associations between specific bacterial genera and PCa, consistent diagnostic or prognostic biomarkers have yet to be established (Crocetto et al., 2020). The observed heterogeneity in microbial profiles and their correlations with PCa across different cohorts underscores the need for further in-depth and standardized investigations. Elucidating the complex and dynamic relationship between the prostatic microbiome and PCa remains a critical step toward understanding its pathophysiological relevance and potential clinical utility.
2.3 The impact of race and environmental factors on the bacterial characteristics of PCa
Ethnic and environmental factors appear to play a significant role in shaping the prostate microbiome and may influence PCa progression. Feng et al. investigated the effects of ethnicity (African vs. European ancestry) and geographic environment (African vs. Australian cohorts) on the prostate microbiome. Their analysis revealed that African samples exhibited significantly greater microbial diversity, aligning with previous findings from China. These samples were predominantly composed of C. acnes, Escherichia coli, and Pseudomonas spp., with Proteobacteria identified as the dominant phylum in PCa microbiomes. Compared to European samples, African specimens showed increased species richness, potentially correlating with a higher tumor mutation burden (Feng et al., 2019a). Additionally, genera such as Bacteroides, Firmicutes, Prevotella, and Fusobacterium were detected exclusively in African samples. While such differences may reflect true biological variation, the potential for sample contamination must be carefully considered. Notably, nearly half of the core human gut bacterial taxa were absent in these datasets. As reported by Feng, Salachan, and others, no consistent correlation has been observed between the prostate microbiome and local PCa progression. This may be attributable to the low microbial biomass typically present in prostate tissues, as well as the risk of contamination during sampling and sequencing procedures. Chen et al. further highlighted these issues by demonstrating that bacterial DNA yields from biopsy-derived prostate tissues were markedly lower than those obtained from urine samples or skin swabs (Chen et al., 2024). Their findings also showed that Lactobacillus and Staphylococcus were highly abundant in perineal regions, reinforcing the need to rigorously exclude potential skin and environmental contaminants when analyzing prostate tissue microbiomes.
Contaminant bacteria introduced during sampling or laboratory procedures may be mistakenly identified as constituents of the prostate microbiome, a well-recognized limitation in current research. In Salachan et al.’s sequencing study, seven of the ten most abundant microorganisms were classified as likely laboratory contaminants. Only Salmonella enterica, Campylobacter jejuni, and Clostridium difficile were considered potential true microbiota, although these species are typically associated with the gastrointestinal tract (Salachan et al., 2022). Similarly, previous studies have identified genera such as Acinetobacter, Enterobacter, Streptococcus, Escherichia, Bacillus, Mycobacterium, Pseudomonas, and Staphylococcus as common sources of contamination (Eisenhofer et al., 2019). These microorganisms may originate from external sources, adjacent tissue cross-contamination, or the laboratory environment, potentially confounding microbiome profiling and interpretation. Therefore, strict contamination control protocols are essential for obtaining accurate and reliable results. While some of these microbes may indeed be involved in PCa pathogenesis, their specific roles remain unclear and require further investigation.
To address concerns regarding contamination, Chen et al. proposed an innovative approach utilizing transperineal biopsy for prostate microbiome analysis. This technique circumvents the potential introduction of gut microbiota that often accompanies traditional transrectal biopsy methods (Chen et al., 2024). Beyond procedural improvements, researchers have also emphasized optimizing experimental design to reduce the influence of confounding factors on microbiome profiling. For instance, Lee et al. employed 64 negative control samples to rigorously eliminate potential contaminant sequences, thereby minimizing bias in sequencing-based analyses. Their findings indicated that the microbiome composition in PCa patients was affected by comorbid diabetes, suggesting that metabolic conditions may modulate the prostate tissue microenvironment via microbiome alterations (Lee et al., 2024). These efforts reflect a growing awareness among researchers regarding the impact of contamination and the importance of continuous methodological refinement. Moreover, pharmacological interventions may also influence PCa risk and progression by modulating microbial composition. Certain medications can suppress pro-inflammatory bacteria or promote the enrichment of beneficial microbes (Barone et al., 2023; Crocetto et al., 2023). For instance, long-term antibiotic use has been linked to persistent dysbiosis in both gut and urogenital microbiota. These disruptions may indirectly affect the immune status and microecological balance of the prostate, potentially contributing to tumorigenesis and influencing the characteristics of the tumor microenvironment in PCa.
2.4 Viral, fungal, and parasitic characteristics in the PCa microbiota
The prostate is a biologically complex and dynamic organ that can harbor mixed microbial infections, including not only bacteria but also viruses, fungi, and parasites. Several viruses with established or suspected oncogenic potential have been detected in prostate tissue, such as BK virus (BKV), JC virus (JCV), Simian Virus 40 (SV40), Xenotropic Murine Leukemia Virus-related virus (XMRV), Human Papillomavirus (HPV), and Human Cytomegalovirus (HCMV) (Samanta et al., 2003; Zambrano et al., 2002). In a study by Martinez-Fierro et al., HPV, XMRV, and HCMV were detected in PCa tissues. Notably, a positive association was observed between HPV infection and PCa, while BKV, JCV, and SV40 were not detected (Martinez-Fierro et al., 2010). In addition to bacterial analysis, Banerjee et al. performed a comprehensive pan-pathogen microarray metagenomic study to investigate viral, fungal, and parasitic diversity in PCa tissues. Their results indicated that herpesviruses and papillomaviruses—specifically HCMV, HPV16, and HPV18—were more prevalent in cancer cohorts. Fungal species from the phylum Ascomycota, such as dermatophytes and Candida spp., were commonly identified. Among parasitic taxa, nematodes such as Hookworm and Ascaris were the most frequently detected (Banerjee et al., 2019). Importantly, HPV16 and HPV18—both high-risk oncogenic HPV genotypes—are considered potential contributors to PCa pathogenesis. Miyake et al. also detected HPV16, HPV18, and Mycoplasma genitalium in PCa cohorts (Miyake et al., 2019). Similarly, Sarkar et al. confirmed a significant association between HPV16, HPV18, and PCa, while also implicating Epstein-Barr virus (EBV) and Hepatitis B virus (HBV) as potential microbial signatures relevant to PCa (Sarkar et al., 2022).
In contrast, Feng et al. did not detect viruses, fungi, or archaea in their metagenomic analysis, instead identifying only bacterial taxa (Feng et al., 2019a). Ala-Almohadesin et al. employed quantitative TaqMan real-time PCR to detect sexually transmitted infection (STI) pathogens in prostate tissues from patients with BPH and PCa. Their results indicated that only Gardnerella vaginalis (GV) and Herpes Simplex Virus 2 (HSV-2) were significantly associated with PCa, while no significant differences were observed for HPV, HCMV, or other herpesviruses (Ala-Almohadesin et al., 2019). Similarly, Gonçalves et al. did not detect typical STI-associated bacteria in their PCa cohort (Gonçalves et al., 2023). These findings suggest that not all oncogenic pathogens are directly involved in PCa, or that their effects may be mediated indirectly through chronic inflammation. In other studies, Salachan et al. reported that the abundance of Haemophilus β-herpesvirus was significantly lower in malignant tissues compared to benign counterparts (Salachan et al., 2022). Ethnic and geographic variations have also been observed in viral profiles. For example, Chen et al. found SV40, HCMV, and EBV in Chinese patient samples, whereas these viruses were absent in samples from Western patients (Chen and Wei, 2015). These disparities suggest that the diversity and distribution of PCa-associated microbiota may be influenced by ethnicity, geography, and individual host factors. Further research is essential to elucidate the roles of these non-bacterial microbes in prostate carcinogenesis and their potential as diagnostic or therapeutic targets. A summary of these findings is presented in Table 1.
3 The potential value of urine and prostate fluid microbiota in PCa prediction
Recent research supports the utility of urine as a non-invasive source for identifying biomarkers predictive of PCa (Nardelli et al., 2024; Zemskova et al., 2020). Tsai et al., using 16S rRNA sequencing, demonstrated distinct differences in the urinary microbiota compositions of patients with BPH and PCa (Tsai et al., 2022). Specifically, Lactobacillus and Staphylococcus exhibited statistically significant variation between these patient groups and healthy controls. In a large-scale cohort study, Pan et al. reported a significant association between PCa and UTIs, including prostatitis, cystitis, and pyelonephritis. This association implies increased susceptibility of PCa patients to alterations in urinary microbiota, particularly those linked to infection and inflammation (Pan et al., 2023). Supporting this, Prakash et al. proposed that pathogen-associated microbiota involved in UTIs may contribute to PCa development. For instance, bladder-ureteral reflux could facilitate pathogen migration and induce prostatic inflammation, underscoring the need for early diagnosis and close microbial monitoring of recurrent UTIs (Prakash et al., 2024). The urogenital microbiome is relatively well characterized and lends itself to repeated sampling over time. Studies have shown that urinary microbiota predominantly belong to five major phyla: Firmicutes, Bacteroidetes, Actinobacteria, Clostridia, and Proteobacteria (Siddiqui et al., 2011). Shrestha et al. found that the urine microbiota in men with both positive and negative PCa biopsies was typically dominated by a single bacterial genus, most commonly Bacillus, Staphylococcus, or Streptococcus (Shrestha et al., 2018). Other commonly detected genera include Lactobacillus and Prevotella (Kustrimovic et al., 2023). However, notable differences in bacterial abundance and composition have been observed between voided urine and prostate tissue. Since urine samples also contain microbiota from the bladder and urethra, even midstream urine collection may not accurately reflect the true microbial landscape of the prostate. This raises important concerns regarding the reliability of urine as a surrogate for studying the prostate microbiome (Kim et al., 2022).
Due to variations in sample selection, anatomical source, and procedural techniques, discrepancies persist among studies investigating the urinary and prostate fluid microbiota in PCa. For example, Hurst et al. analyzed urine and prostate tissue samples collected after digital rectal examination and identified four novel bacterial species commonly present in patient urine—Porphyromonas, Bacteroides, Vagococcus, and Fusobacterium. Furthermore, specific anaerobic bacterial communities detected in urine were associated with higher PCa risk, as classified by the D’Amico criteria, suggesting potential prognostic relevance (Hurst et al., 2022). Alanee et al. performed high-throughput 16S rRNA gene sequencing on urine samples from 30 patients with suspected PCa and found that microbial alterations were primarily driven by changes in bacterial abundance rather than diversity. Notably, Lactobacillus abundance decreased, while Streptococcus abundance increased in PCa patients (Alanee et al., 2019). In a follow-up study, Lee et al. reported the presence of bacterial toxin genes—including colicin, cytotoxic necrotizing factor, and cytolytic pore-forming toxins—in the urine of high-grade PCa patients. However, these toxins were not considered diagnostically significant and were instead hypothesized to be associated with prior infections or environmental exposures (Lee et al., 2023). In African cohorts, Akinpelu et al. observed higher pathogen isolation rates in PCa samples compared to BPH, with Escherichia coli identified as the most prevalent pathogen, followed by Pseudomonas aeruginosa (Akinpelu et al., 2024). Microbiota identified in urinary microbiome studies may originate from various anatomical sites, including the prostate, bladder, and glans penis. Yu et al. conducted 16S rRNA sequencing on urine, expressed prostatic secretions (EPS), and semen from PCa and BPH patients. In PCa patients, EPS samples showed an increased abundance of Bacteroidetes, Proteobacteria, and Firmicutes, along with significant decreases in anaerobic genera such as Selenomonas and Deinococcus. Quantitative real-time PCR revealed decreased counts of E. coli and Enterococcus in urine, but increased abundance in prostatic fluid and semen (Yu et al., 2015). In another study, Ma et al. analyzed EPS microbiota using 16S rRNA sequencing and reported reduced microbial diversity in PCa patients. Streptococcus, Bacillus, Enterobacteriaceae, and Lactococcus were more abundant in the EPS of PCa patients, whereas Marinobacter and Thermobacterium were more prevalent in non-PCa controls (Ma et al., 2019). Gonçalves et al. compared microbiota from urine and the glans penis and found their compositions to be highly similar. In the urine of PCa patients, elevated levels of Streptococcus, Prevotella, and Peptostreptococcus were observed, while Methylobacterium, Bacteroides, and Clostridium were more abundant in controls (Gonçalves et al., 2023). Collectively, these findings demonstrate significant variability in microbial profiles across different urogenital sampling sites in PCa, as detailed in Table 2. Further research is needed to determine whether the observed microbial differences in urine and prostate fluid represent true biological signatures and whether they correlate with clinical outcomes.
4 The role of C. acnes in PCa progression
Among the various pathogens investigated for their potential involvement in PCa, HPV remains the only microorganism with a well-established carcinogenic role. However, other bacteria—including C. acnes, Escherichia coli, and Neisseria gonorrhoeae—have been implicated in contributing to chronic inflammation associated with PCa pathogenesis (Lawson and Glenn, 2022). C. acnes, a common skin commensal, has recently garnered substantial attention for its role in promoting PCa progression through the induction of chronic inflammation. Multiple studies have reported that prostate host cells respond to C. acnes infection by secreting pro-inflammatory cytokines and chemokines, such as IL-6, IL-8, IL-10, and TNF-α (Davidsson et al., 2016; Kim et al., 2024). These inflammatory mediators are known to activate key oncogenic signaling pathways, including NF-κB, STAT3, and cGAS-STING axis (Fassi Fehri et al., 2011). Moreover, different subspecies of C. acnes possess distinct surface structures, which influence their tissue colonization capacity and pathogenic potential at both cutaneous and non-cutaneous sites (Mak et al., 2013a). Sahdo et al. demonstrated that C. acnes activates caspase-1 in human peripheral neutrophils, potentially initiating inflammation-driven prostate pathology (Sahdo et al., 2013). In support of this, Bae et al. performed immunohistochemical analyses and found C. acnes to be frequently present in non-cancerous glandular epithelial cells and stromal macrophages in PCa tissues. The infection was associated with nuclear NF-κB activation in prostate cells, suggesting a mechanistic link between C. acnes-induced inflammation and tumor progression (Bae et al., 2014). Animal model studies have further validated these findings. In rat models of prostate infection, C. acnes induced chronic histological inflammation and persistent infection, reinforcing its pathogenic potential in prostate tissue (Olsson et al., 2012; Shinohara et al., 2013). Recent investigations have also revealed that C. acnes may activate immune responses through non-classical pathways. For example, Fischer et al. demonstrated that C. acnes can stimulate the cGAS-STING pathway in human macrophages, leading to IFN-I signaling and innate immune activation (Fischer et al., 2020). Additionally, Davidsson et al. reported a strong positive correlation between C. acnes presence and Treg infiltration in PCa tissues, suggesting that C. acnes may contribute to an immunosuppressive tumor microenvironment that facilitates cancer progression (Davidsson et al., 2021). Furthermore, Ashida et al. found that C. acnes infection altered the expression of genes involved in homologous recombination and the Fanconi anemia pathway in prostate epithelial cells. These changes impaired DNA repair mechanisms and induced a “BRCAness” phenotype (Ashida et al., 2024). This research highlights the potential role of C. acnes in driving PCa via both classical inflammatory and non-classical immune and genetic mechanisms.
Extensive research has suggested that the C. acnes Type II subspecies—considered a more defensive phenotype—may be closely associated with the development and progression of PCa (Dekio et al., 2021). Notably, the prevalence of C. acnes Type II is higher in adult men than in adolescents, implying a potential age-related factor in its colonization. While Type I strains predominantly inhabit facial skin, Type II strains are more frequently detected in the urinary tract (Ba et al., 2006). In early studies, Cohen et al. cultured prostate tissue from PCa patients and identified C. acnes in 35% of the samples. The presence of C. acnes was significantly correlated with increased prostatic inflammation, and the strains isolated were genetically distinct from classical skin-associated strains (Cohen et al., 2005). Further evidence was provided by Mak et al., who used 16S rRNA gene sequencing and multi-locus sequence typing to confirm that prostate-isolated C. acnes strains were affiliated with sequence types typical of the urethral microbiota, differing from acne-associated skin strains (Mak et al., 2013b). Ba et al. detected C. acnes antibodies in patients undergoing prostate biopsy and found that elevated antibody titers were significantly associated with increased PSA levels. Antibody titers were also independently linked to patient age, prostate volume, and the degree of inflammation in BPH patients (Ba et al., 2008). Interestingly, Severi et al. reported an inverse relationship between circulating C. acnes antibody titers and PCa risk, particularly in advanced-stage cases (Severi et al., 2010). However, this study could not distinguish whether the elevated titers originated from acne-related colonization or later prostate infection, thus limiting the interpretation of causality. Chen et al. used RNA sequencing and detected C. acnes gene expression in both tumor and adjacent non-tumorous prostate tissue, but not in samples from healthy individuals (Chen and Wei, 2015), highlighting a potential role in early PCa pathogenesis. Davidsson et al., using bacterial culture and PCR, demonstrated that both Type IA and IB C. acnes were capable of colonizing the prostate, with significantly higher prevalence in PCa patients compared to controls (Davidsson et al., 2016). Subsequent studies have proposed that the presence of C. acnes in glandular tissue may serve as an independent early biomarker for PCa, potentially offering superior diagnostic value over PSA alone (Kakegawa et al., 2017). Additional evidence from Yow, Kim, Ahn, and Sarkar further supported the enrichment of C. acnes in PCa patients (Ahn et al., 2022; Kim et al., 2024; Sarkar et al., 2022; Yow et al., 2017). Notably, Kim et al. reported higher C. acnes abundance in high-grade PCa samples. LEfSe analysis identified C. acnes as a key microbial biomarker for distinguishing cancer, implicating it in PCa cell proliferation and inflammatory regulation (Kim et al., 2024). In addition to tissue-based studies, urinary microbiome analyses also demonstrated a significant increase in C. acnes among PCa patients (Ahn et al., 2022). Manente et al. detected C. acnes genomic DNA in urine and semen using real-time PCR, with no detection following antibiotic treatment (Manente et al., 2022). This suggests that C. acnes may promote the initiation and progression of PCa by triggering chronic inflammation and activating multiple signaling pathways, and can be detected through genomic analysis, as shown in Figure 3.
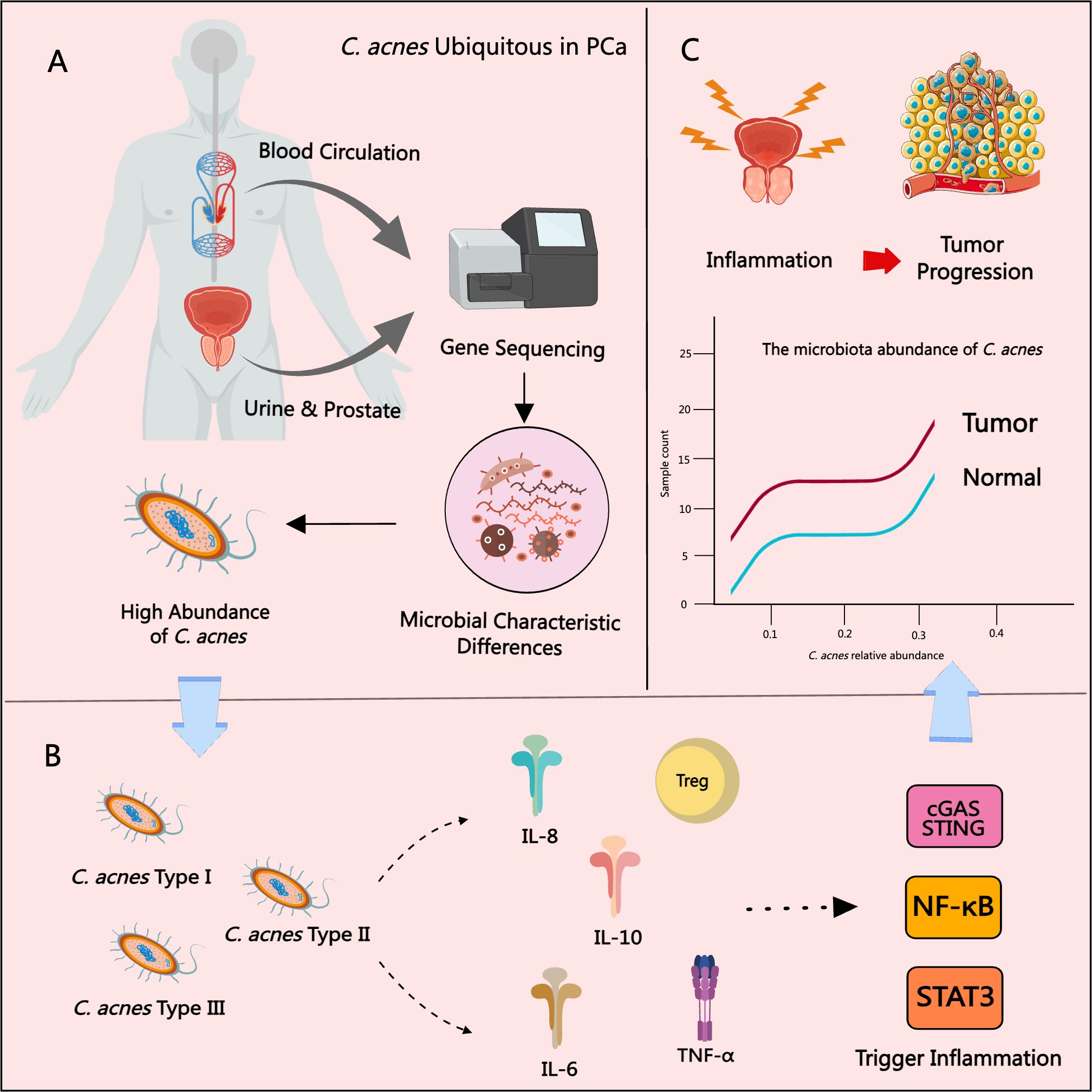
Figure 3. The role of C. acnes in PCa progression. (A) C. acnes is frequently detected in prostate tissue, urine, and blood from PCa patients. (B) The type II subtype of C. acnes is most strongly associated with PCa, promoting chronic inflammation by inducing inflammatory cytokines and chemokines and activating pathways such as NF-κB, STAT3, and cGAS–STING. (C) The relative abundance of C. acnes in PCa tissues is significantly correlated with increased tissue inflammation and higher tumor grade.
However, not all studies support the hypothesis that C. acnes promotes PCa progression through inflammatory responses. Alexeyev et al. conducted two independent studies using 16S rRNA sequencing and fluorescence in situ hybridization, identifying C. acnes as the most frequently detected bacterium in both BPH and PCa tissues. Their findings demonstrated that C. acnes is capable of establishing persistent infections within the prostate (Alexeyev et al., 2006, 2007). Similarly, Sfanos et al. screened a large number of prostate tissue samples for the presence of C. acnes, Trichomonas vaginalis, and other microbial DNA. While microbial DNA was detected in 87% of samples, culture-based methods isolated far fewer viable organisms, and no significant association was found between C. acnes presence and histological signs of inflammation (Sfanos et al., 2008). Additionally, a population-based survey study found no significant correlation between self-reported acne and PCa risk, suggesting that acne history cannot serve as a reliable biomarker for PCa susceptibility (Cremers et al., 2014). In a clinical cohort of 99 PCa patients, C. acnes was identified in 60 individuals; however, there were no significant differences in serum levels of inflammatory mediators between infected and non-infected patients. These findings imply that C. acnes may induce only localized, low-grade inflammation that does not manifest systemically, though it may still influence PCa onset and progression via microenvironmental modulation (Ugge et al., 2018a). Supporting this perspective, Radej et al. observed the presence of C. acnes in both PCa and BPH patients and proposed that the bacterium may modulate immune responses through Tregs (Radej et al., 2020). Cavarretta et al., using 16S rRNA sequencing, reported high C. acnes abundance not only in tumor tissues but also in peritumoral and non-tumorous prostate regions (Cavarretta et al., 2017), further suggesting that this bacterium may not be specific to malignant transformation. In line with this, Kim et al. found that C. acnes abundance was lower in high-grade tumors compared to intermediate-grade tumors, implying that the role of specific C. acnes subtypes in PCa may vary and remains to be clearly defined (Kim et al., 2024). Despite the lack of strong statistical associations, many researchers continue to explore the potential role of C. acnes in prostate inflammation and tumor development. For instance, a prospective cohort study by Ugge et al. revealed that severe acne during late adolescence may be linked to an elevated risk of PCa later in life (Ugge et al., 2018b). It is important to note that microbial contamination during sampling significantly affects detection reliability. As C. acnes is a common anaerobic skin commensal, strict adherence to sterile collection protocols is essential to avoid false-positive findings and ensure data integrity (Sfanos and Isaacs, 2008). Overall, although correlations between C. acnes and PCa have been observed, future investigations should utilize more precise and contamination-controlled methodologies to accurately determine the bacterium’s pathogenic role and clinical relevance.
5 Discussion and prospect
PCa is one of the most common and increasingly prevalent malignancies affecting men worldwide. In recent years, microbiome research has emerged as a promising frontier in oncology, offering novel insights and therapeutic possibilities for the diagnosis and treatment of PCa. However, compared to the relatively well-established research on the gut microbiome, investigations into the prostate tissue microbiome remain limited and underdeveloped. Existing studies have demonstrated that the microbiome within PCa tissues exhibits notable diversity and significant regional heterogeneity. These microbial communities may contribute to tumor initiation and progression by promoting chronic inflammation, particularly prostatitis. Furthermore, pronounced differences in microbial composition have been observed across populations of different ethnic and geographic backgrounds, suggesting that environmental exposures, dietary patterns, and host genetic factors may play critical roles in shaping the prostate microbiome. Importantly, the variability in current findings may also stem from methodological differences and issues related to sample contamination. Beyond bacterial taxa, the prostate-associated microbiome encompasses a broader spectrum of microorganisms, including viruses, fungi, and parasites. The interplay between these microbial constituents and host factors contributes to a complex and dynamic microecological system within the prostate tumor microenvironment. In parallel, urine and prostate fluid have been proposed as non-invasive alternatives for characterizing the prostate microbiome, particularly in PCa patients. Several studies suggest that the urinary microbiome may reflect disease-related microbial shifts and play a potential role in PCa pathogenesis. However, given anatomical and compositional differences—particularly in microbial abundance—between urine and prostate tissue, further validation is required to determine the reliability of urine as a surrogate marker for the prostate microbiome.
Among the various prostate-associated microorganisms, C. acnes has garnered considerable attention due to its stable enrichment within prostate tissues. Accumulating evidence indicates that C. acnes may contribute to the initiation and progression of PCa by inducing chronic inflammatory responses, suppressing local immune surveillance, and remodeling the tumor microenvironment. Although microbial population heterogeneity exists, multiple independent cohort studies have consistently observed the enrichment of C. acnes in PCa tissues, highlighting its potential as a broadly applicable biomarker. Moving forward, C. acnes holds promise as a microbial marker for early screening and monitoring of disease progression in PCa. Furthermore, precision antimicrobial strategies targeting C. acnes may offer novel avenues for adjunctive therapeutic interventions in PCa management. In conclusion, the relationship between PCa and the microbiome represents a complex and evolving field. It involves intricate interactions between diverse microbial populations and their regulation of immune, inflammatory, and oncogenic pathways within the tumor microenvironment. Future research should prioritize mechanistic investigations to elucidate how specific microbes influence PCa initiation and progression. Such efforts may lead to the identification of novel microbial biomarkers and therapeutic targets, paving the way for early diagnosis, personalized treatment, and microbiome-based interventions in PCa management.
Author contributions
FF: Writing – original draft, Writing – review & editing. YY: Writing – review & editing. BW: Visualization, Writing – review & editing. XZ: Visualization, Writing – review & editing. NW: Visualization, Writing – review & editing. JY: Visualization, Writing – review & editing. KW: Writing – review & editing. QZ: Conceptualization, Funding acquisition, Project administration, Supervision, Writing – review & editing.
Funding
The author(s) declare that financial support was received for the research and/or publication of this article. This research was funded by the National Natural Science Foundation of China (Grant No. 8237152750).
Conflict of interest
The authors declare that the research was conducted in the absence of any commercial or financial relationships that could be construed as a potential conflict of interest.
Generative AI statement
The author(s) declare that no Generative AI was used in the creation of this manuscript.
Publisher’s note
All claims expressed in this article are solely those of the authors and do not necessarily represent those of their affiliated organizations, or those of the publisher, the editors and the reviewers. Any product that may be evaluated in this article, or claim that may be made by its manufacturer, is not guaranteed or endorsed by the publisher.
References
Adamo, D. A., Greenwood, B. M., Ghanouni, P., and Arora, S. (2024). MR imaging-guided prostate cancer therapies. Radiologic Clinics North America 62, 121–133. doi: 10.1016/j.rcl.2023.06.012
Ahmadzadehfar, H., Seifert, R., Afshar-Oromieh, A., Kratochwil, C., and Rahbar, K. (2024). Prostate cancer theranostics with 177Lu-PSMA. Semin. Nuclear Med. 54, 581–590. doi: 10.1053/j.semnuclmed.2024.02.007
Ahn, H. K., Kim, K., Park, J., and Kim, K. H. (2022). Urinary microbiome profile in men with genitourinary Malignancies. Invest. Clin. Urol. 63, 569–576. doi: 10.4111/icu.20220124
Akinpelu, S. O., Olasehinde, G. I., Ikuerowo, S. O., and Akinnola, O. O. (2024). Prevalence and antibiotic susceptibility patterns of uropathogens in men with prostate cancer and benign prostate hyperplasia from Southwestern Nigeria. BMC Microbiol. 24, 361. doi: 10.1186/s12866-024-03524-w
Ala-Almohadesin, A., Mohammadbeygi, M., Bahavar, A., Mohammadi, M. A., Mohamadzadeh, N., Abolhasani, M., et al. (2019). Molecular detection of pathogens causing sexually transmissible infections in patients with prostate cancer and hyperplasia by quantitative TaqMan real-time PCR assay. Clin. Lab. 65. doi: 10.7754/Clin.Lab.2019.181243
Alanee, S., El-Zawahry, A., Dynda, D., Dabaja, A., McVary, K., Karr, M., et al. (2019). A prospective study to examine the association of the urinary and fecal microbiota with prostate cancer diagnosis after transrectal biopsy of the prostate using 16sRNA gene analysis. Prostate 79, 81–87. doi: 10.1002/pros.23713
Alexeyev, O., Bergh, J., Marklund, I., Thellenberg-Karlsson, C., Wiklund, F., Grönberg, H., et al. (2006). Association between the presence of bacterial 16S RNA in prostate specimens taken during transurethral resection of prostate and subsequent risk of prostate cancer (Sweden). Cancer Causes Control: CCC 17, 1127–1133. doi: 10.1007/s10552-006-0054-2
Alexeyev, O. A., Marklund, I., Shannon, B., Golovleva, I., Olsson, J., Andersson, C., et al. (2007). Direct visualization of Propionibacterium acnes in prostate tissue by multicolor fluorescent in situ hybridization assay. J. Clin. Microbiol. 45, 3721–3728. doi: 10.1128/JCM.01543-07
Almeeri, M. N. E., Awies, M., and Constantinou, C. (2024). Prostate cancer, pathophysiology and recent developments in management: A narrative review. Curr. Oncol. Rep. 26, 1511–1519. doi: 10.1007/s11912-024-01614-6
Almeida, A., Nayfach, S., Boland, M., Strozzi, F., Beracochea, M., Shi, Z. J., et al. (2021). A unified catalog of 204,938 reference genomes from the human gut microbiome. Nat. Biotechnol. 39, 105–114. doi: 10.1038/s41587-020-0603-3
Ashida, S., Kawada, C., Tanaka, H., Kurabayashi, A., Yagyu, K., Sakamoto, S., et al. (2024). Cutibacterium acnes invades prostate epithelial cells to induce BRCAness as a possible pathogen of prostate cancer. Prostate 84, 1056–1066. doi: 10.1002/pros.24723
Ba, S., Rj, C., and Kl, G. (2006). Polymerase chain reaction-based identification of Propionibacterium acnes types isolated from the male urinary tract: Evaluation of adolescents, normal adults and men with prostatic pathology. BJU Int. 98, 388–392. doi: 10.1111/j.1464-410X.2006.06273.x
Ba, S., Rj, C., and Kl, G. (2008). The antibody response to Propionibacterium acnes is an independent predictor of serum prostate-specific antigen levels in biopsy-negative men. BJU Int. 101, 429–435. doi: 10.1111/j.1464-410X.2007.07214.x
Bae, Y., Ito, T., Iida, T., Uchida, K., Sekine, M., Nakajima, Y., et al. (2014). Intracellular Propionibacterium acnes infection in glandular epithelium and stromal macrophages of the prostate with or without cancer. PloS One 9, e90324. doi: 10.1371/journal.pone.0090324
Banerjee, S., Alwine, J. C., Wei, Z., Tian, T., Shih, N., Sperling, C., et al. (2019). Microbiome signatures in prostate cancer. Carcinogenesis 40, 749–764. doi: 10.1093/carcin/bgz008
Barone, B., Mirto, B. F., Falcone, A., Del Giudice, F., Aveta, A., Napolitano, L., et al. (2023). The efficacy of flogofilm® in the treatment of chronic bacterial prostatitis as an adjuvant to antibiotic therapy: A randomized prospective trial. J. Clin. Med. 12, 2784. doi: 10.3390/jcm12082784
Cavarretta, I., Ferrarese, R., Cazzaniga, W., Saita, D., Lucianò, R., Ceresola, E. R., et al. (2017). The microbiome of the prostate tumor microenvironment. Eur. Urol. 72, 625–631. doi: 10.1016/j.eururo.2017.03.029
Che, B., Zhang, W., Xu, S., Yin, J., He, J., Huang, T., et al. (2021). Prostate microbiota and prostate cancer: A new trend in treatment. Front. Oncol. 11. doi: 10.3389/fonc.2021.805459
Chen, V. S., James, C., Khemmani, M., Desai, S., Doshi, C., Rac, G., et al. (2024). A prospective evaluation of the prostate microbiome in Malignant and benign tissue using transperineal biopsy. Prostate 84, 1251–1261. doi: 10.1002/pros.24763
Chen, Y. and Wei, J. (2015). Identification of pathogen signatures in prostate cancer using RNA-seq. PloS One 10, e0128955. doi: 10.1371/journal.pone.0128955
Cohen, R. J., Shannon, B. A., McNeal, J. E., Shannon, T., and Garrett, K. L. (2005). Propionibacterium acnes associated with inflammation in radical prostatectomy specimens: A possible link to cancer evolution? J. Urol. 173, 1969–1974. doi: 10.1097/01.ju.0000158161.15277.78
Coleman, R. E., Croucher, P. I., Padhani, A. R., Clézardin, P., Chow, E., Fallon, M., et al. (2020). Bone metastases. Nat. Rev. Dis. Primers 6, 83. doi: 10.1038/s41572-020-00216-3
Cornford, P., van den Bergh, R. C. N., Briers, E., Van den Broeck, T., Brunckhorst, O., Darraugh, J., et al. (2024). EAU-EANM-ESTRO-ESUR-ISUP-SIOG guidelines on prostate cancer-2024 update. Part I: screening, diagnosis, and local treatment with curative intent. Eur. Urol. 86, 148–163. doi: 10.1016/j.eururo.2024.03.027
Cremers, R. G., Aben, K. K., Vermeulen, S. H., den Heijer, M., van Oort, I. M., van de Kerkhof, P. C., et al. (2014). Self-reported acne is not associated with prostate cancer. Urologic Oncol. 32, 941–945. doi: 10.1016/j.urolonc.2014.02.019
Crocetto, F., Balsamo, R., Amicuzi, U., De Luca, L., Falcone, A., Mirto, B. F., et al. (2023). Novel key ingredients in urinary tract health-the role of D-mannose, chondroitin sulphate, hyaluronic acid, and N-acetylcysteine in urinary tract infections (Uroial PLUS®). Nutrients 15, 3573. doi: 10.3390/nu15163573
Crocetto, F., Barone, B., De Luca, L., and Creta, M. (2020). Granulomatous prostatitis: A challenging differential diagnosis to take into consideration. Future Oncol. (London England) 16, 805–806. doi: 10.2217/fon-2020-0185
Davidsson, S., Mölling, P., Rider, J. R., Unemo, M., Karlsson, M. G., Carlsson, J., et al. (2016). Frequency and typing of Propionibacterium acnes in prostate tissue obtained from men with and without prostate cancer. Infect. Agents Cancer 11, 26. doi: 10.1186/s13027-016-0074-9
Davidsson, S., MöCarlsson, J., Greenberg, L., Wijkander, J., Söderquist, B., Erlandsson, A., et al. (2021). Cutibacterium acnes Induces the Expression of Immunosuppressive Genes in Macrophages and is Associated with an Increase of Regulatory T-Cells in Prostate Cancer. Microbiology spectrum 9, e0149721. doi: 10.1128/spectrum.01497-21
Dekio, I., Asahina, A., and Shah, H. N. (2021). Unravelling the eco-specificity and pathophysiological properties of Cutibacterium species in the light of recent taxonomic changes. Anaerobe 71, 102411. doi: 10.1016/j.anaerobe.2021.102411
De Marzo, A. M., Platz, E. A., Sutcliffe, S., Xu, J., Grönberg, H., Drake, C. G., et al. (2007). Inflammation in prostate carcinogenesis. Nat. Rev. Cancer 7, 256–269. doi: 10.1038/nrc2090
Dicks, L. M. T. and Vermeulen, W. (2022). Do bacteria provide an alternative to cancer treatment and what role does lactic acid bacteria play? Microorganisms 10, 1733. doi: 10.3390/microorganisms10091733
Eisenhofer, R., Minich, J. J., Marotz, C., Cooper, A., Knight, R., and Weyrich, L. S. (2019). Contamination in low microbial biomass microbiome studies: issues and recommendations. Trends Microbiol. 27, 105–117. doi: 10.1016/j.tim.2018.11.003
El Tekle, G. and Garrett, W. S. (2023). Bacteria in cancer initiation, promotion and progression. Nat. Rev. Cancer 23, 600–618. doi: 10.1038/s41568-023-00594-2
Fares, J., Fares, M. Y., Khachfe, H. H., Salhab, H. A., and Fares, Y. (2020). Molecular principles of metastasis: A hallmark of cancer revisited. Signal Transduction Targeted Ther. 5, 28. doi: 10.1038/s41392-020-0134-x
Fassi Fehri, L., Mak, T. N., Laube, B., Brinkmann, V., Ogilvie, L. A., Mollenkopf, H., et al. (2011). Prevalence of Propionibacterium acnes in diseased prostates and its inflammatory and transforming activity on prostate epithelial cells. Int. J. Med. Microbiology: IJMM 301, 69–78. doi: 10.1016/j.ijmm.2010.08.014
Feng, Y., Jaratlerdsiri, W., Patrick, S. M., Lyons, R. J., Haynes, A.-M., Collins, C. C., et al. (2019a). Metagenomic analysis reveals a rich bacterial content in high-risk prostate tumors from African men. Prostate 79, 1731–1738. doi: 10.1002/pros.23897
Feng, Y., Ramnarine, V. R., Bell, R., Volik, S., Davicioni, E., Hayes, V. M., et al. (2019b). Metagenomic and metatranscriptomic analysis of human prostate microbiota from patients with prostate cancer. BMC Genomics 20, 146. doi: 10.1186/s12864-019-5457-z
Fischer, K., Tschismarov, R., Pilz, A., Straubinger, S., Carotta, S., McDowell, A., et al. (2020). Cutibacterium acnes Infection Induces Type I Interferon Synthesis Through the cGAS-STING Pathway. Front. Immunol. 11. doi: 10.3389/fimmu.2020.571334
Geller, L. T., Barzily-Rokni, M., Danino, T., Jonas, O. H., Shental, N., Nejman, D., et al. (2017). Potential role of intratumor bacteria in mediating tumor resistance to the chemotherapeutic drug gemcitabine. Science 357, 1156–1160. doi: 10.1126/science.aah5043
Gonçalves, M. F. M., Pina-Vaz, T., Fernandes, Â.R., Miranda, I. M., Silva, C. M., Rodrigues, A. G., et al. (2023). Microbiota of urine, glans and prostate biopsies in patients with prostate cancer reveals a dysbiosis in the genitourinary system. Cancers 15, 1423. doi: 10.3390/cancers15051423
Guner, E., Danacioglu, Y. O., Arikan, Y., Seker, K. G., Polat, S., Baytekin, H. F., et al. (2021). The presence of chronic inflammation in positive prostate biopsy is associated with upgrading in radical prostatectomy. Archivio Italiano Di Urologia Andrologia: Organo Ufficiale [Di] Societa Italiana Di Ecografia Urologica E Nefrologica 93, 280–284. doi: 10.4081/aiua.2021.3.280
Helmink, B. A., Khan, M. A. W., Hermann, A., Gopalakrishnan, V., and Wargo, J. A. (2019). The microbiome, cancer, and cancer therapy. Nat. Med. 25, 377–388. doi: 10.1038/s41591-019-0377-7
Herrera-Quintana, L., Vázquez-Lorente, H., Lopez-Garzon, M., Cortés-Martín, A., and Plaza-Diaz, J. (2024). Cancer and the microbiome of the human body. Nutrients 16, 2790. doi: 10.3390/nu16162790
Hochreiter, W. W., Duncan, J. L., and Schaeffer, A. J. (2000). Evaluation of the bacterial flora of the prostate using a 16S rRNA gene based polymerase chain reaction. J. Urol. 163, 127–130. doi: 10.1016/S0022-5347(05)67987-6
Hurst, R., Meader, E., Gihawi, A., Rallapalli, G., Clark, J., Kay, G. L., et al. (2022). Microbiomes of urine and the prostate are linked to human prostate cancer risk groups. Eur. Urol. Oncol. 5, 412–419. doi: 10.1016/j.euo.2022.03.006
Kakegawa, T., Bae, Y., Ito, T., Uchida, K., Sekine, M., Nakajima, Y., et al. (2017). Frequency of propionibacterium acnes infection in prostate glands with negative biopsy results is an independent risk factor for prostate cancer in patients with increased serum PSA titers. PloS One 12, e0169984. doi: 10.1371/journal.pone.0169984
Kang, J., La Manna, F., Bonollo, F., Sampson, N., Alberts, I. L., Mingels, C., et al. (2022). Tumor microenvironment mechanisms and bone metastatic disease progression of prostate cancer. Cancer Lett. 530, 156–169. doi: 10.1016/j.canlet.2022.01.015
Keay, S., Zhang, C. O., Baldwin, B. R., and Alexander, R. B. (1999). Polymerase chain reaction amplification of bacterial 16s rRNA genes in prostate biopsies from men without chronic prostatitis. Urology 53, 487–491. doi: 10.1016/s0090-4295(98)00553-6
Kim, J. H., Seo, H., Kim, S., Rahim, M. A., Jo, S., Barman, I., et al. (2024). Different prostatic tissue microbiomes between high- and low-grade prostate cancer pathogenesis. Int. J. Mol. Sci. 25, 8943. doi: 10.3390/ijms25168943
Kim, J. H., Seo, H., Kim, S., Ul-Haq, A., Rahim, M. A., Jo, S., et al. (2023). Biochemical recurrence in prostate cancer is associated with the composition of lactobacillus: microbiome analysis of prostatic tissue. Int. J. Mol. Sci. 24, 10423. doi: 10.3390/ijms241310423
Kim, J. K., Song, S. H., Jung, G., Song, B., and Hong, S. K. (2022). Possibilities and limitations of using low biomass samples for urologic disease and microbiome research. Prostate Int. 10, 169–180. doi: 10.1016/j.prnil.2022.10.001
Krieger, J. N., Riley, D. E., Vesella, R. L., Miner, D. C., Ross, S. O., and Lange, P. H. (2000). Bacterial dna sequences in prostate tissue from patients with prostate cancer and chronic prostatitis. J. Urol. 164, 1221–1228. doi: 10.1016/S0022-5347(05)67145-5
Kumar Am, S., Rajan, P., Alkhamees, M., Holley, M., and Lakshmanan, V.-K. (2024). Prostate cancer theragnostics biomarkers: An update. Invest. Clin. Urol. 65, 527–539. doi: 10.4111/icu.20240229
Kustrimovic, N., Bombelli, R., Baci, D., and Mortara, L. (2023). Microbiome and prostate cancer: A novel target for prevention and treatment. Int. J. Mol. Sci. 24, 1511. doi: 10.3390/ijms24021511
Lawson, J. S. and Glenn, W. K. (2022). Multiple pathogens and prostate cancer. Infect. Agents Cancer 17, 23. doi: 10.1186/s13027-022-00427-1
Lee, J.-J., Kim, J. K., Oh, B., Hong, S. K., and Kim, B.-S. (2024). Influence of diabetes on microbiome in prostate tissues of patients with prostate cancer. Front. Oncol. 14. doi: 10.3389/fonc.2024.1445375
Lee, J., Wickes, B. L., Fu, J., Brockman, N. E., Garg, H., Jobin, C., et al. (2023). Prevalence of genotoxic bacteria in men undergoing biopsy for prostate cancer. Prostate 83, 663–669. doi: 10.1002/pros.24500
Leskinen, M. J., Rantakokko-Jalava, K., Manninen, R., Leppilahti, M., Marttila, T., Kylmälä, T., et al. (2003). Negative bacterial polymerase chain reaction (PCR) findings in prostate tissue from patients with symptoms of chronic pelvic pain syndrome (CPPS) and localized prostate cancer. Prostate 55, 105–110. doi: 10.1002/pros.10218
Lloréns-Rico, V., Simcock, J. A., Huys, G. R. B., and Raes, J. (2022). Single-cell approaches in human microbiome research. Cell 185, 2725–2738. doi: 10.1016/j.cell.2022.06.040
Ma, X., Chi, C., Fan, L., Dong, B., Shao, X., Xie, S., et al. (2019). The microbiome of prostate fluid is associated with prostate cancer. Front. Microbiol. 10. doi: 10.3389/fmicb.2019.01664
Ma, J., Gnanasekar, A., Lee, A., Li, W. T., Haas, M., Wang-Rodriguez, J., et al. (2020). Influence of intratumor microbiome on clinical outcome and immune processes in prostate cancer. Cancers 12, 2524. doi: 10.3390/cancers12092524
Mak, T. N., Schmid, M., Brzuszkiewicz, E., Zeng, G., Meyer, R., Sfanos, K. S., et al. (2013a). Comparative genomics reveals distinct host-interacting traits of three major human-associated propionibacteria. BMC Genomics 14, 640. doi: 10.1186/1471-2164-14-640
Mak, T. N., Yu, S. H., De Marzo, A. M., Brüggemann, H., and Sfanos, K. S. (2013b). Multilocus sequence typing (MLST) analysis of Propionibacterium acnes isolates from radical prostatectomy specimens. Prostate 73, 770–777. doi: 10.1002/pros.22621
Manente, L., Gargiulo, U., Gargiulo, P., and Dovinola, G. (2022). Propionibacterium acnes in urine and semen samples from men with urinary infection. Archivio Italiano Di Urologia Andrologia: Organo Ufficiale [Di] Societa Italiana Di Ecografia Urologica E Nefrologica 94, 62–64. doi: 10.4081/aiua.2022.1.62
Martinez-Fierro, M. L., Leach, R. J., Gomez-Guerra, L. S., Garza-Guajardo, R., Johnson-Pais, T., Beuten, J., et al. (2010). Identification of viral infections in the prostate and evaluation of their association with cancer. BMC Cancer 10, 326. doi: 10.1186/1471-2407-10-326
Miller, K. D., Nogueira, L., Devasia, T., Mariotto, A. B., Yabroff, K. R., Jemal, A., et al. (2022). Cancer treatment and survivorship statistics 2022. CA: A Cancer J. Clinicians 72, 409–436. doi: 10.3322/caac.21731
Miyake, M., Ohnishi, K., Hori, S., Nakano, A., Nakano, R., Yano, H., et al. (2019). Mycoplasma genitalium infection and chronic inflammation in human prostate cancer: detection using prostatectomy and needle biopsy specimens. Cells 8, 212. doi: 10.3390/cells8030212
Mjaess, G., Karam, A., Roumeguère, T., Diamand, R., Aoun, F., McVary, K., et al. (2023). Urinary microbiota and prostatic diseases: The key for the lock? A systematic review. Prostate Cancer Prostatic Dis. 26, 451–460. doi: 10.1038/s41391-022-00602-w
Munteanu, R., Feder, R.-I., Onaciu, A., Munteanu, V. C., Iuga, C.-A., and Gulei, D. (2023). Insights into the human microbiome and its connections with prostate cancer. Cancers 15, 2539. doi: 10.3390/cancers15092539
Nardelli, C., Aveta, A., Pandolfo, S. D., Tripodi, L., Russo, F., Imbimbo, C., et al. (2024). Microbiome profiling in bladder cancer patients using the first-morning urine sample. Eur. Urol. Open Sci. 59, 18–26. doi: 10.1016/j.euros.2023.11.003
Ohadian Moghadam, S. and Momeni, S. A. (2021). Human microbiome and prostate cancer development: Current insights into the prevention and treatment. Front. Med. 15, 11–32. doi: 10.1007/s11684-019-0731-7
Olsson, J., Drott, J. B., Laurantzon, L., Laurantzon, O., Bergh, A., and Elgh, F. (2012). Chronic prostatic infection and inflammation by Propionibacterium acnes in a rat prostate infection model. PloS One 7, e51434. doi: 10.1371/journal.pone.0051434
Pan, S.-Y., Chen, W.-C., Huang, C.-P., Hsu, C. Y., and Chang, Y.-H. (2023). The association of prostate cancer and urinary tract infections: A new perspective of prostate cancer pathogenesis. Medicina (Kaunas Lithuania) 59, 483. doi: 10.3390/medicina59030483
Pernigoni, N., Guo, C., Gallagher, L., Yuan, W., Colucci, M., Troiani, M., et al. (2023). The potential role of the microbiota in prostate cancer pathogenesis and treatment. Nat. Rev. Urol. 20, 706–718. doi: 10.1038/s41585-023-00795-2
Picardo, S. L., Coburn, B., and Hansen, A. R. (2019). The microbiome and cancer for clinicians. Crit. Rev. Oncology/Hematology 141, 1–12. doi: 10.1016/j.critrevonc.2019.06.004
Prakash, P., Verma, S., and Gupta, S. (2024). Influence of microbiome in intraprostatic inflammation and prostate cancer. Prostate 84, 1179–1188. doi: 10.1002/pros.24756
Pushalkar, S., Hundeyin, M., Daley, D., Zambirinis, C. P., Kurz, E., Mishra, A., et al. (2018). The pancreatic cancer microbiome promotes oncogenesis by induction of innate and adaptive immune suppression. Cancer Discov. 8, 403–416. doi: 10.1158/2159-8290.CD-17-1134
Radej, S., Płaza, P., Olender, A., Szewc, M., Bar, K., and Maciejewski, R. (2020). Infiltrating Treg and Th17 cells of the prostate hypertrophy gland associated with propionibacterium acnes infection. Res. Rep. Urol. 12, 593–597. doi: 10.2147/RRU.S284066
Riley, D. E., Berger, R. E., Miner, D. C., and Krieger, J. N. (1998). Diverse and related 16S rRNA-encoding DNA sequences in prostate tissues of men with chronic prostatitis. J. Clin. Microbiol. 36, 1646–1652. doi: 10.1128/JCM.36.6.1646-1652.1998
Rizzo, A., Santoni, M., Mollica, V., Fiorentino, M., Brandi, G., and Massari, F. (2022). Microbiota and prostate cancer. Semin. Cancer Biol. 86, 1058–1065. doi: 10.1016/j.semcancer.2021.09.007
Romano, L., Napolitano, L., Crocetto, F., Sciorio, C., Sio, M. D., Miranda, A., et al. (2024). Prostate and gut: Any relationship? A narrative review on the available evidence and putative mechanisms. Prostate 84, 513–524. doi: 10.1002/pros.24675
Sahdo, B., Särndahl, E., Elgh, F., and Söderquist, B. (2013). Propionibacterium acnes activates caspase-1 in human neutrophils. APMIS: Acta Pathologica Microbiologica Immunologica Scandinavica 121, 652–663. doi: 10.1111/apm.12035
Salachan, P. V., Rasmussen, M., Fredsøe, J., Ulhøi, B., Borre, M., and Sørensen, K. D. (2022). Microbiota of the prostate tumor environment investigated by whole-transcriptome profiling. Genome Med. 14, 9. doi: 10.1186/s13073-022-01011-3
Samanta, M., Harkins, L., Klemm, K., Britt, W. J., and Cobbs, C. S. (2003). High prevalence of human cytomegalovirus in prostatic intraepithelial neoplasia and prostatic carcinoma. J. Urol. 170, 998–1002. doi: 10.1097/01.ju.0000080263.46164.97
Sarkar, P., Malik, S., Banerjee, A., Datta, C., Pal, D. K., Ghosh, A., et al. (2022). Differential microbial signature associated with benign prostatic hyperplasia and prostate cancer. Front. Cell. Infection Microbiol. 12. doi: 10.3389/fcimb.2022.894777
Severi, G., Shannon, B. A., Hoang, H. N., Baglietto, L., English, D. R., Hopper, J. L., et al. (2010). Plasma concentration of Propionibacterium acnes antibodies and prostate cancer risk: Results from an Australian population-based case–control study. Br. J. Cancer 103, 411–415. doi: 10.1038/sj.bjc.6605757
Sfanos, K. S. and Isaacs, W. B. (2008). An evaluation of PCR primer sets used for detection of Propionibacterium acnes in prostate tissue samples. Prostate 68, 1492–1495. doi: 10.1002/pros.20820
Sfanos, K. S., Sauvageot, J., Fedor, H. L., Dick, J. D., De Marzo, A. M., and Isaacs, W. B. (2008). A molecular analysis of prokaryotic and viral DNA sequences in prostate tissue from patients with prostate cancer indicates the presence of multiple and diverse microorganisms. Prostate 68, 306–320. doi: 10.1002/pros.20680
Sfanos, K. S., Yegnasubramanian, S., Nelson, W. G., and De Marzo, A. M. (2018). The inflammatory microenvironment and microbiome in prostate cancer development. Nat. Rev. Urol. 15, 11–24. doi: 10.1038/nrurol.2017.167
Shinohara, D. B., Vaghasia, A. M., Yu, S.-H., Mak, T. N., Brüggemann, H., Nelson, W. G., et al. (2013). A mouse model of chronic prostatic inflammation using a human prostate cancer-derived isolate of Propionibacterium acnes. Prostate 73, 1007–1015. doi: 10.1002/pros.22648
Shrestha, E., White, J. R., Yu, S.-H., Kulac, I., Ertunc, O., De Marzo, A. M., et al. (2018). Profiling the urinary microbiome in men with positive versus negative biopsies for prostate cancer. J. Urol. 199, 161–171. doi: 10.1016/j.juro.2017.08.001
Shyanti, R. K., Greggs, J., Malik, S., and Mishra, M. (2024). Gut dysbiosis impacts the immune system and promotes prostate cancer. Immunol. Lett. 268, 106883. doi: 10.1016/j.imlet.2024.106883
Siddiqui, H., Nederbragt, A. J., Lagesen, K., Jeansson, S. L., and Jakobsen, K. S. (2011). Assessing diversity of the female urine microbiota by high throughput sequencing of 16S rDNA amplicons. BMC Microbiol. 11, 244. doi: 10.1186/1471-2180-11-244
Tilg, H., Adolph, T. E., Gerner, R. R., and Moschen, A. R. (2018). The intestinal microbiota in colorectal cancer. Cancer Cell 33, 954–964. doi: 10.1016/j.ccell.2018.03.004
Tilki, D., van den Bergh, R. C. N., Briers, E., Van den Broeck, T., Brunckhorst, O., Darraugh, J., et al. (2024). EAU-EANM-ESTRO-ESUR-ISUP-SIOG guidelines on prostate cancer. Part II-2024 update: treatment of relapsing and metastatic prostate cancer. Eur. Urol. 86, 164–182. doi: 10.1016/j.eururo.2024.04.010
Tsai, K.-Y., Wu, D.-C., Wu, W.-J., Wang, J.-W., Juan, Y.-S., Li, C.-C., et al. (2022). Exploring the Association between Gut and Urine Microbiota and Prostatic Disease including Benign Prostatic Hyperplasia and Prostate Cancer Using 16S rRNA Sequencing. Biomedicines 10, 2676. doi: 10.3390/biomedicines10112676
Ugge, H., Carlsson, J., Söderquist, B., Fall, K., Andén, O., and Davidsson, S. (2018a). The influence of prostatic Cutibacterium acnes infection on serum levels of IL6 and CXCL8 in prostate cancer patients. Infect. Agents Cancer 13, 34. doi: 10.1186/s13027-018-0204-7
Ugge, H., Udumyan, R., Carlsson, J., Andrén, O., Montgomery, S., Davidsson, S., et al. (2018b). Acne in late adolescence and risk of prostate cancer. Int. J. Cancer 142, 1580–1585. doi: 10.1002/ijc.31192
Wensel, C. R., Pluznick, J. L., Salzberg, S. L., and Sears, C. L. (2022). Next-generation sequencing: Insights to advance clinical investigations of the microbiome. J. Clin. Invest. 132, e154944. doi: 10.1172/JCI154944
Wong, C. C. and Yu, J. (2023). Gut microbiota in colorectal cancer development and therapy. Nat. Rev. Clin. Oncol. 20, 429–452. doi: 10.1038/s41571-023-00766-x
Yow, M. A., Tabrizi, S. N., Severi, G., Bolton, D. M., Pedersen, J., Australian Prostate Cancer BioResource, et al. (2017). Characterisation of microbial communities within aggressive prostate cancer tissues. Infect. Agents Cancer 12, 4. doi: 10.1186/s13027-016-0112-7
Yu, H., Meng, H., Zhou, F., Ni, X., Shen, S., and Das, U. N. (2015). Urinary microbiota in patients with prostate cancer and benign prostatic hyperplasia. Arch. Med. Science : AMS 11, 385–394. doi: 10.5114/aoms.2015.50970
Zambrano, A., Kalantari, M., Simoneau, A., Jensen, J. L., and Villarreal, L. P. (2002). Detection of human polyomaviruses and papillomaviruses in prostatic tissue reveals the prostate as a habitat for multiple viral infections. Prostate 53, 263–276. doi: 10.1002/pros.10157
Zemskova, M. Y., Marinets, M. V., Sivkov, A. V., Pavlova, J. V., Shibaev, A. N., and Sorokin, K. S. (2020). Integrin alpha V in urine: A novel noninvasive marker for prostate cancer detection. Front. Oncol. 10. doi: 10.3389/fonc.2020.610647
Zha, C., Peng, Z., Huang, K., Tang, K., Wang, Q., Zhu, L., et al. (2023). Potential role of gut microbiota in prostate cancer: Immunity, metabolites, pathways of action? Front. Oncol. 13. doi: 10.3389/fonc.2023.1196217
Zheng, J. H., Nguyen, V. H., Jiang, S.-N., Park, S.-H., Tan, W., Hong, S. H., et al. (2017). Two-step enhanced cancer immunotherapy with engineered Salmonella typhimurium secreting heterologous flagellin. Sci. Trans. Med. 9, eaak9537. doi: 10.1126/scitranslmed.aak9537
Keywords: prostate cancer, microbiome, bacteria, Cutibacterium acnes, urine
Citation: Fu F, Yu Y, Wang B, Zhao X, Wang N, Yin J, Wu K and Zhou Q (2025) Prostate and urinary microbiomes in prostate cancer development: focus on Cutibacterium acnes. Front. Cell. Infect. Microbiol. 15:1562729. doi: 10.3389/fcimb.2025.1562729
Received: 18 January 2025; Accepted: 05 May 2025;
Published: 21 May 2025.
Edited by:
Xin Xu, Sichuan University, ChinaReviewed by:
Biagio Barone, ASL Napoli 1 Centro, ItalyVinagolu K. Rajasekhar, Memorial Sloan Kettering Cancer Center, United States
Copyright © 2025 Fu, Yu, Wang, Zhao, Wang, Yin, Wu and Zhou. This is an open-access article distributed under the terms of the Creative Commons Attribution License (CC BY). The use, distribution or reproduction in other forums is permitted, provided the original author(s) and the copyright owner(s) are credited and that the original publication in this journal is cited, in accordance with accepted academic practice. No use, distribution or reproduction is permitted which does not comply with these terms.
*Correspondence: Qing Zhou, MzEwMDk0QGhudWNtLmVkdS5jbg==