- 1Department of Pathology, University of Pisa, Pisa, Italy
- 2Department of Clinical and Experimental Medicine, University of Pisa, Pisa, Italy
3,5-diiodo-L-thyronine (3,5-T2) is an endogenous derivative of thyroid hormone with potential metabolic effects. It has been detected in human blood by immunological methods, but a reliable assay based on mass spectrometry (MS), which is now regarded as the gold standard in clinical chemistry, is not available yet. Therefore, we aimed at developing a novel ad-hoc optimized method to quantitate 3,5-T2 and its isomers by MS in human serum. Serum samples were obtained from 28 healthy subjects. Two ml of serum were deproteinized with acetonitrile and then subjected to an optimized solid phase extraction-based procedure. To lower background noise, the samples were furtherly cleaned by hexane washing and acetonitrile precipitation of residual proteins. 3,5-T2 and its isomers 3,3′-T2 and 3′,5′-T2 were then analyzed by HPLC coupled to tandem MS. Accuracy and precision for T2 assay were 88–104% and 95–97%, respectively. Recovery and matrix effect averaged 78% and +8%, respectively. 3,5-T2 was detected in all samples and its concentration averaged (mean ± SEM) 41 ± 5 pg/ml, i.e., 78 ± 9 pmol/l. In the same samples the concentration of 3,3′-T2 averaged 133±15 pg/ml, i.e., 253±29 pmol/l, while 3′,5′-T2 was not detected. 3,5-T2 concentration was significantly related to 3,3′-T2 concentration (r = 0.540, P < 0.01), while no significant correlation was observed with either T3 or T4 in a subset of patients in which these hormones were assayed. In conclusion, our method is able to quantify 3,5-T2 and 3,3′-T2 in human serum. Their concentrations lie in the subnanomolar range, and a significant correlation was detected between these two metabolites in healthy individuals.
Introduction
The term thyroid hormones, classically referred to both 3,5,3′-triiodothyronine (T3) and thyroxine (T4), seems nowadays to be simplistic; indeed, it has been shown that some T3 and T4 metabolites, particularly 3,5-diiodothyronine (3,5-T2) and 3-iodothyronamine, are independent chemical messengers, with specific metabolic effects (1).
Two deiodinase enzymes, namely D1- and D3-deiodinase, can potentially catalyse the synthesis of distinct diiodothyronines: 3,5-diiodothyronine (3,5-T2), 3,3′- diiodothyronine (3,3′-T2) and 3′,5′- diiodothyronine (3′,5′-T2) (2). Until recent years, all T2 isomers were regarded as inactive metabolites of T3 and T4, because of their very low affinity for nuclear thyroid hormone receptors. However, this view has been challenged by the observation that 3,5-T2 can also interact with mitochondrial targets and that administration of exogenous 3,5-T2 to experimental animals produces significant functional effects on lipid metabolism and mitochondrial function (3).
A major pitfall in the investigations about the alleged physiological and pathophysiological relevance of 3,5-T2 is the difficulty in assaying its low serum or tissue concentration, either in animal models or in human.
Polyclonal antibody-based radio-immunoassays were developed in the 1970's. The first radio-immunoassay from Meinhold et al. allowed detection of serum 3,5-T2 in 10 normal subject (100 pmol/L) and in 5 hyperthyroid patients (380 nmol/L) (4). Subsequently, in 1982 Faber et al. reported a serum 3,5-T2 concentration close to 100 pmol/L with a RIA method based on gel separation and antibody extraction (GSAE) (5). Further RIA methods have been subsequently employed, but high variability was reported for serum 3,5-T2 concentration, i.e., between 10 and 190 pmol/L (6). In 2014, Lehmphul et al. developed a new CLIA method for 3,5-T2 evaluation with a lower detection limit of 200 pmol/L. The average concentration was 430 pmol/L in 31 hypothyroid patients and 310 pmol/L in 24 hyperthyroid patients, vs. 290 pmol/L in the control healthy group, although this difference was not statistically significant (7).
Mass spectrometry-based methods should provide a more specific and sensitive quantification technology for the assay of thyroid hormone and their metabolites, including 3,5-T2. In recent years, several groups have published method for 3,5-T2 detection based on Liquid Chromatography-tandem Mass Spectrometry (LC-MS-MS) (8), but so far, the application of these techniques to human serum samples usually produced negative results.
Due to these methodological challenges, the aim of this study was to develop an offline pre-analytical sample preparation (sample extraction) procedure and an LC-MS-MS method for quantification of T2 isomers in human serum.
Materials and Methods
Sample Collection
Serum samples were obtained from 28 patients, including 8 healthy volunteers and 20 women undergoing endocrinological screening and found to be euthyroid. Serum was obtained by using the remaining part of samples obtained for independent clinical indications. The study had the approval of the local Ethical Committee. All subjects gave a written informed consent and since no additional blood drawings were performed, ethical committee approval was not required.
Reagents and Materials
3,3′,5,5-tetraiodo-L-thyronine (T4), 3,3′,5-triiodo-L-thyronine (T3), 3,3′,5′-triiodo-L-thyronine (rT3), 3,5-diiodo-L-thyronine (3,5-T2), 3,3′-diiodo-L-thyronine (3,3′-T2), 3,3′,5,5-tetraiodo-L-thyronine-13C6 (13C6-T4), and 3,3′,5-triiodo-L-thyronine-13C6 (13C6-T3), were provided by Sigma-Aldrich (Saint Louis, MO, USA). Acetonitrile (LC-MS grade), methanol (LC-MS grade), ultra-pure water (LC-MS grade), hexane (HPLC grade), glacial acetic acid (ACS grade), hydrochloric acid (ACS grade), and ammonium hydroxide (ACS grade), were also from Sigma -Aldrich. 3,3′-diiodo-L-tyronine-13C6 (13C6-T2) was purchased from Isosciences (Ambler, PA, USA), while 3,5-diiodo-L-tyronine-13C9-15N (13N-T2) was kindly provided by Prof. Thomas S. Scanlan (Portland, OR, USA).
Sample Preparation
The optimization of the sample preparation procedure, as well as the instrumental method development, took advantage of the expertise gained in the assay of T4, T3, rT3, and some of their metabolites in human and animal biological matrices (9–11). The general strategy of the pre-analytical procedure consisted in using a relatively large amount of starting material (human serum) that was concentrated up to 40-fold. Since this approach tends to generate a high matrix effect, additional steps were introduced to ensure extensive cleaning of the sample, both before and after the extraction step. Briefly, 2 mL of serum from each sample were placed in a 2 mL microcentrifuge tube (Eppendorf, Hamburg, Germany) and spiked with appropriate internal standards amount in order to achieve the final concentration of 1 ng/mL, 5 ng/mL, 50 ng/mL, and 200 ng/mL for 13C6-T2, 13N-T2, 13C6-T3, and 13C6-T4, respectively. After a 2 min shaking at room temperature, the sample was split into 4 aliquots of 500 μL each, and all of them was added with 500 μL of a mixture containing ice-cold acetonitrile (79% as v/v), water (20%), and formic acid (1%) for deproteinization. After vortexing and sonication (15 min), 1 mL of ice-cold acetonitrile was added to each aliquot, which was then shacked for 2 min, sonicated for 15 min, and centrifuged for 15 min at 14,000 × g. The resulting supernatants were warmed up to 40°C and evaporated up to ~0.5 mL under a gentle stream of nitrogen. The liquid residues from the different aliquots of the same sample were pooled and loaded onto an Agilent (Santa Clara, CA, USA) Bond-Elut Certify 130 mg SPE cartridge, previously conditioned by sequential wetting with 2 mL of methanol and 3 mL of water. After washing with 2 mL of water, 2 mL of 0.1 M HCl, and 5 mL of methanol, the column was dried, and the sample was eluted with 2 mL of methanol/ammonium hydroxide (95:5 by volume). The eluate was dried under a gentle stream of nitrogen and reconstituted with 200 μL of water/acetonitrile (70:30) containing 0.1% formic acid, and then shaken for 6 min at room temperature. The reconstituted solution was washed with 600 μL of hexane to extract lipid residues, which were then removed, while the aqueous solution containing the analytes was added with 500 μL acetonitrile, shaken for 2 min, and centrifuged for 15 min. After removing the pellet, the supernatant was dried under nitrogen and stored at −20oC until processing. Immediately before analysis, it was reconstituted with 50 μL of water/acetonitrile (70:30) containing 0.1% formic acid, shaken for 15 min, centrifuged for 15 min and injected (20 μL of the supernatant) into the HPLC-MS-MS system.
Instrumental Layout and Operative Conditions
Samples were processed by using an instrument layout consisting of an AB Sciex API 4000 triple quadrupole mass spectrometer (Concord, ON, Canada), equipped with an electrospray (ESI) Turbo V ion source, coupled to an Agilent 1290 Infinity UHPLC system (Santa Clara, CA, USA), including autosampler outfitted with peltier tray, binary pump, and column oven, used for the chromatographic separation. A ten port divert valve (Valco Instruments Co. Inc., Huston, TX, USA) allowed the discarding of both head and tail of the HPLC runs, while a quaternary HPLC pump (Series 200, PerkinElmer, Boston, MA, USA) supplied the mass spectrometer with solvent when the flow from the analytical system was diverted to waste, to prevent the occurrence of high voltage discharge in the ion source. Chromatographic separation was performed by a Phenomenex (Torrance, CA, USA) Gemini C18 110 Å, 3 μm, 50 x 2 mm HPLC column protected by a C18 4 x 2.0 mm ID security guard cartridge. Data acquisition and system control were carried out by an AB Sciex Analyst® version 1.6.3 software.
Twenty micro liters of each sample was injected into the Agilent UPLC system, where it was submitted to chromatographic separation by a mixture methanol/acetonitrile (20/80) added with 0.1% formic acid, as solvent A, and water also containing 0.1% of formic acid, as solvent B, under the gradient conditions shown in Table 1. The chromatographic column was kept at 20°C.
Mass spectrometry method was based on selected reaction monitoring (SRM) in positive ion mode and made use of three transitions for each compound, one of which was used as a quantifier (Q) and the others as qualifier (q). All of them were monitored using optimized declustering potential (DPs), collision energies (CEs), and collision exit potentials (CXPs), which are reported in Table 2. Further operative parameters were: nebulizer current (NC), 5.0 KV; gas source 1 (GS1) zero air, 70; gas source 2 (GS2) zero air, 55; source temperature (TEM), 650°C; entrance potential (EP), 10 V; IQ1 lens potential, −9.8 V; stubby rod Q1 (ST),−15.5 V; collision gas (CAD) nitrogen, operative pressure with CAD gas on, 5.7 mPa.
Method Performances
Quality control was performed according to Matuszewski et al. (12). Accuracy is the ratio of measured concentration to spiked concentration after adding known amounts of analytes at two different concentration levels: precision is the coefficient of variation (standard deviation/mean) of repeated measurements within the same assay under the same conditions as described above; recovery is defined as the ratio of internal standard spiked before extraction to internal standard spiked after extraction; matrix effect is defined as the ratio of internal standard spiked after extraction to internal standard dissolved in the reconstitution solvent.
Statistical analysis was performed with GraphPad Prism version 7.0 for Windows (GraphPad Software, San Diego, CA). Data are reported as mean ± SEM. Correlation analysis was performed through linear regression, taking P = 0.05 as the conventional limit of statistical significance.
Results
Quality control data for T2 assay averaged as follows, without apparent differences between isomers: accuracy 88–104%, precision 95–97%, recovery 78%, matrix effect +8%. For T3 and T4, recovery and precision were 104–128 and 85–94%, respectively. Recovery, matrix effect and precision averaged 67, −15, and 115% for T3; 44, −7, and 90% for T4. The lower limit of detection, determined with a signal to noise ratio of 3, was 11.5 pg/mL (22 pmol/L) for T2, 9.1 pg/mL (14 pmol/L) for T3, and 8.5 pg/mL (11 pmol/L) for T4.
Representative chromatograms obtained in two patients are shown in Figure 1. 3,5-T2 and 3,3′-T2 were present in all samples, while 3′,5′-T2 was never detected. Peak identity was determined by comparison with appropriate standard based on retention times and ratio between the three transitions.
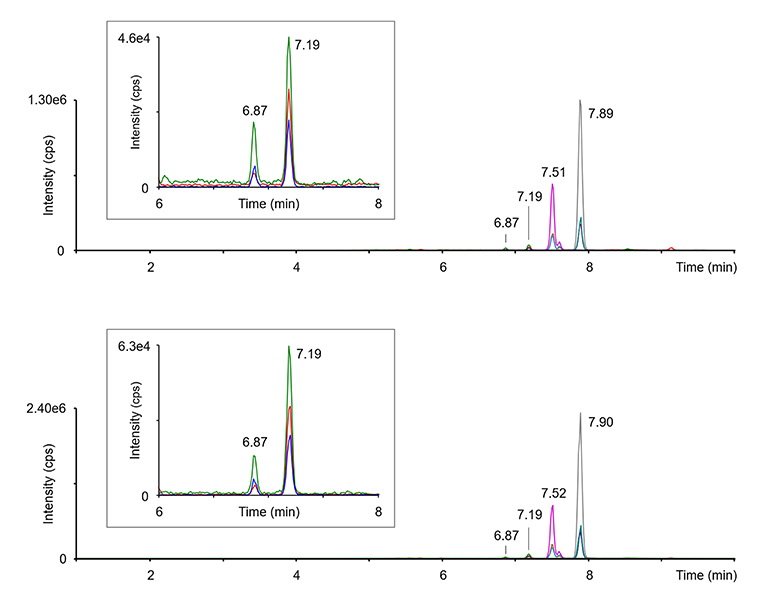
Figure 1. Representative chromatograms obtained in two different subjects. The green, red, and blue tracings, reported also as an expanded view in the framed panels, refer to the three transitions monitored for 3, 5-T2 (6.87min.) and 3,3′-T2 (7.19min.), namely m/z 529.9 → 352.9, 529.9 → 381.8, and 525.9 → 479.9. Three more peaks are attributable to T3 (7.51–7.52min.), rT3 (small peak next to T3, at 7.60–7.61min), and T4 (7.89–7.90min.). Peak identity was confirmed by the comparison to appropriate standards, as detailed in the methods section.
Analyte concentration was determined by appropriate calibration curves and was based on the first transition, namely Q transition in Table 2. Scatter plot of the results obtained for 3,5-T2 and 3,3′-T2 are shown in Figure 2. 3,5-T2 concentration averaged 41±5 pg/mL, i.e., 78 ± 9 pmol/L. In the same samples the concentration of 3,3′-T2 was about 3-fold higher and averaged 133±15 pg/mL, i.e., 253±29 pmol/L.
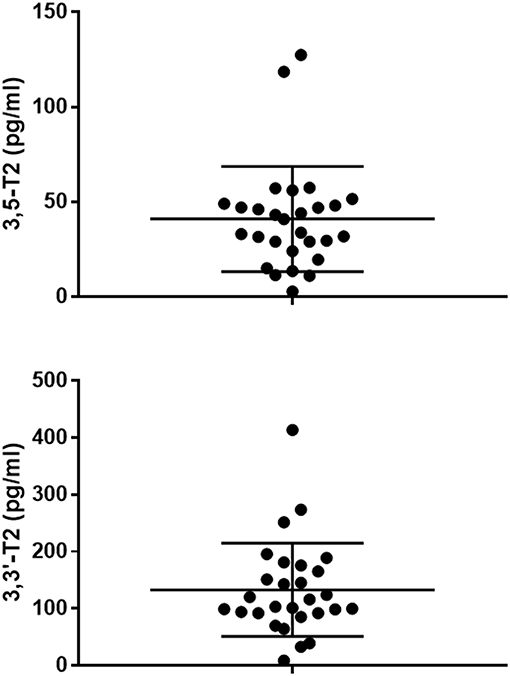
Figure 2. Scatter plots showing the serum concentrations of 3,5-T2 (upper panel) and 3,3′-T2 (lower panel) in the 28 patients included in our series. The horizontal lines represent mean ± SD. Please note a different scale was used in the two panels.
As shown in Figure 3, 3,5-T2 concentration was significantly related to 3,3′-T2 concentration (r = 0.540, P < 0.01), while no significant correlation was observed with either T3 or T4 in the subset of patients in which these hormones were assayed.
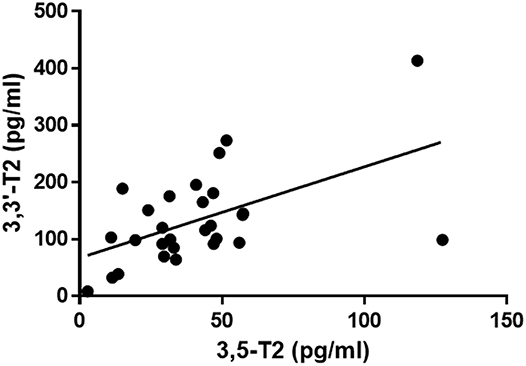
Figure 3. Scatter plot of the relationship between 3,5-T2 and 3,3′-T2 serum concentration in the 28 patients included in our series. Linear regression analysis yielded r = 0.540 with P = 0.003. The regression line is plotted (y = ax + b, with a = 1.600 ± 0.489 and b = 67.29 ± 24.06 pg/mL).
Discussion
Due to the emerging role of 3,5-T2 in lipid and glucose metabolism, the adequate measurement of 3,5-T2 and related compound is a challenging aim of thyroid hormone research. This is necessary to identify the clinical variables correlated with 3,5-T2 concentrations and the effect of physiological and pathophysiological interventions. It would also be crucial to determine the effective concentrations achieved in experimental animals after the administration of exogenous 3,5-T2, and to compare them with the endogenous levels.
In the past, different immunoassays have provided variable results. Recently, a novel immunoassay has been developed and used in a large clinical series (7, 13, 14), but endogenous levels appear to be below the lower detection limit (200 nmol/L) in about one third of the subjects. In addition, the experience acquired with thyroid hormones assays suggests that immunological methods cannot be easily employed to determine tissue concentrations.
Mass spectrometry is a very promising analytical technique, since it displays significant advantages in terms of accuracy, sensitivity and specificity (15–17) and it has been successfully applied to tissue homogenates (18). So far, only a few papers reported 3,5-T2 measurements in humans or in animal models by mass spectrometry-based techniques.
Wang et al. (8) developed a LC-MS-MS method for the simultaneous measurement of 3,5-T2, 3,3′-T2, T3, rT3, and T4 in bovine serum samples and human serum from Standard Reference Materials. Both in bovine and in human serum the concentration of 3,5-T2 and 3,3′-T2 was below the lower detection limit of the method, namely 740 pg/mL (1.41 nmol/L) for 3,5-T2 and 920 pg/mL (1.75 nmol/L) for 3,3′-T2.
In 2014 Jonklaas et al. (19) published the results of a pilot study in which 3,3′T2 concentrations were evaluated by LC-MS-MS in 100 patients, with the primary aim to correlate T2 and clinical variables. Mean detected 3,3′-T2 levels were around 0.013–0.040 pmol/L and they could demonstrate an association between poor clinical conditions and low levels of T2 (19).
In a subsequent investigation, Hansen et al. (20) succeeded in detecting T2 in adult frog (Xenopus laevis) plasma and tadpole (Rana catesbeiana) serum. They developed a new analytical method by optimizing solid phase extraction, eventually choosing hydrophilic-lipophilic balanced mixed-mode polymeric materials (Plexa) and C18-materials to measure 11 thyroid hormone metabolites, including 3,5-T2 and 3,3′-T2, in 50 μl of serum or plasma, with a lower detection limit of 250 pg/mL (476 pmol/L). 3,5-T2 and 3,3′-T2 were detected in 80 and 30% of the samples, respectively, and their concentrations averaged 660 and 640 pmol/L, respectively.
Quite recently, Min Li et al. (21) optimized an LC-Q-TOF-MS method to determine thyroid hormone metabolites in placenta tissue. With this method, it was possible for the first time to track the presence of T2 (either 3,3′-T2 and 3,5-T2) in human and mice placenta at the concentration of 160–260 and 70–130 pg/g, respectively.
The method we developed has the advantage of a lower detection limit, which allowed 3,5-T2 and 3,3′-T2 detection in all our samples. This improvement is probably due to the higher sample volume and to the lower matrix effect allowed by the extensive washing steps included in the pre-analytical procedure. While this is promising, several drawbacks should be acknowledged: the needed samples volume is still too high for routine clinical use; the method is time consuming; the pre-analytical steps are too complex to be implemented on-line with the MS equipment. In addition, the human factor may be crucial, since each operator needed a significant training period before becoming able to get reproducible results. For these reasons, we believe that further developments are necessary before starting large-scale clinical investigations. In particular, sample preparation should be improved to make it easier and increase its reproducibility, throughput and ruggedness. If this can be achieved, it will become possible to establish a proper age- and gender-related reference range, and to investigate the effects of the different thyroid states and/or thyroid hormone supplementation.
While the present investigation should be regarded as a feasibility study, it may help to get further insight into T2 biochemistry and biology. Our results confirm that previous reports based on mass spectrometry-based techniques may have missed endogenous T2 because of their lower sensitivity. On the other hand, immunological techniques, particularly the recent method developed by Lemphul et al. provided control values for 3,5-T2 that are about 3-fold higher than we observed. The simplest explanation of this difference is the hypothesis that the different techniques allow identification of different T2 fractions. In general, immunological techniques rely on long incubation times and limited sample processing. In the presence of a high affinity antibody the analyte is expected to dissociate from its endogenous binding sites, and a good estimate of its total amount is obtained. On the other hand, mass spectrometry requires extensive sample processing and protein precipitation, making it likely that protein-bound analyte is lost.
T3 and T4 are known to be largely bound to plasma proteins, with a free/total ratio lower than 1/100, and a good agreement is usually observed between the results of mass spectrometry-based assays and the total amounts detected by immunological methods (9). Extensive binding to plasma protein has also be reported for 3-iodothyronamine, whose assay is complicated by additional pitfalls, such as oxidation by serum enzymes and adduct formation, further accounting for a large discrepancy between mass spectrometry and immunological detection (22). At present, very little is known about T2 binding to plasma proteins and/or serum metabolism. Adding T2 to reconstituted systems including specific plasma proteins and comparing the results of immunological and mass spectrometry-based assays might help to clarify this issue.
Our investigation was not aimed at evaluating the relationships between T2 level and other clinical variables. We could simply test the association between different iodothyronines. Interestingly, while 3,5-T2 and 3,3′-T2 levels were significantly related, neither T2 isomer showed any significant correlation with T3 or T4. This is consistent with the observation reported by Lemphul et al. (7) and it supports the hypothesis that T2 production may be specifically regulated. Because of this association, it would be interesting to investigate whether 3,3′-T2 assay may be used in the clinical field as an indirect index of 3,5-T2 availability.
In conclusion, we developed a method to measure 3,5-T2 and 3,3′-T2 in human serum samples, that significantly reduced matrix effect and showed high sensitivity and specificity. The method is promising, and further developments aimed at reducing its technical complexity might eventually provide a novel diagnostic tool amenable to large-scale clinical use.
Ethics Statement
Serum samples were obtained from 28 patients, including 8 healthy volunteers and 20 women undergoing endocrinological screening and found to be euthyroid. Serum was obtained by using the remaining part of samples obtained for independent clinical indications. All subjects gave informed consent and since no additional blood drawings were performed, ethical committee approval was not required.
Author Contributions
LL, NN, GS, ES, and MB performed the experiments. EC and TS collected the human samples. FS and SG analyzed the data. RZ, LL, and AS wrote the manuscript draft.
Funding
This work was partly supported by a grant from Pisa University to RZ (PRA 2018/19).
Conflict of Interest Statement
The authors declare that the research was conducted in the absence of any commercial or financial relationships that could be construed as a potential conflict of interest.
Acknowledgments
Part of these data was presented at ETA Conference Belgrade 2017.
References
1. Accorroni A, Saponaro F, Zucchi R. Tissue thyroid hormones and thyronamines. Heart Fail Rev. (2016) 21:373–90. doi: 10.1007/s10741-016-9553-8
2. Senese R, Cioffi F, De Lange P, Goglia F, Lanni A. Thyroid: biological actions of “nonclassical” thyroid hormones. J Endocrinol. (2014) 221:R1–12. doi: 10.1530/JOE-13-0573
3. Moreno M, Lanni A, Lombardi A, Goglia F. How the thyroid controls metabolism in the rat: different roles for triiodothyronine and diiodothyronines. J Physiol. (1997) 505 (Pt. 2):529–38.
4. Meinhold H, Shurnbarnd P. A radioimmunoassay for 3,5-diiodothyronine*. Clin Endocrinol. (1978) 8:493–7. doi: 10.1111/j.1365-2265.1978.tb02187.x
5. Faber J, Lumholtz IB, Kirkegaard C, Siersbaek-Nielsen KFT. Isolation of radioactive iodothyronines for kinetic studies: a comparison of two methods. Acta Endocrinol. (1982) 99:64–71. doi: 10.1530/acta.0.0990064
6. Pinna G, Meinhold H, Hiedra L, Thoma R, Hoell T, Gräf KJ, et al. Elevated 3,5-diiodothyronine concentrations in the sera of patients with nonthyroidal illnesses and brain tumors. J Clin Endocrinol Metab. (1997) 82:1535–42.
7. Lehmphul I, Brabant G, Wallaschofski H, Ruchala M, Strasburger CJ, Koehrle J, et al. Detection of 3,5-diiodothyronine in sera of patients with altered thyroid status using a new monoclonal antibody based chemiluminescence immunoassay. Thyroid (2014) 24:1350–60. doi: 10.1089/thy.2013.0688
8. Wang D, Stapleton HM. Analysis of thyroid hormones in serum by liquid chromatography-tandem mass spectrometry. Anal Bioanal Chem. (2010) 397:1831–9. doi: 10.1007/s00216-010-3705-9
9. Saba A, Chiellini G, Frascarelli S, Marchini M, Ghelardoni S, Raffaelli A, et al. Tissue distribution and cardiac metabolism of 3-iodothyronamine. Endocrinology (2010) 151:5063–73. doi: 10.1210/en.2010-0491
10. Galli E, Marchini M, Saba A, Berti S., Tonacchera M, Vitti P, et al. Detection of 3-iodothyronamine in human patients: a preliminary study. J Clin Endocrinol Metab. (2012) 97:E69–74. doi: 10.1210/jc.2011-1115
11. Saba A, Donzelli R, Colligiani D, Raffaelli A, Nannipieri M, Kusmic C, et al. Quantification of thyroxine and 3,5,3′-triiodo-thyronine in human and animal hearts by a novel liquid chromatography-tandem mass spectrometry method. Horm Metab Res. (2014) 46:628–34. doi: 10.1055/s-0034-1368717
12. Matuszewski BK, Constanzer ML, Chavez-Eng CM. Strategies for the assessment of matrix effect in quantitative bioanalytical methods based on HPLC-MS/MS. Anal Chem. (2003) 75:3019–30. doi: 10.1021/ac020361s
13. Pietzner M, Lehmphul I, Friedrich N, Schurmann C, Ittermann T, Dörr M, et al. Translating pharmacological findings from hypothyroid rodents to euthyroid humans: is there a functional role of endogenous 3,5-T2? Thyroid (2015) 25:188–97. doi: 10.1089/thy.2014.0262
14. Pietzner M, Homuth G, Budde K, Lehmphul I, Völker U, Völzke H, et al. Urine Metabolomics by <sup>1</sup>H-NMR spectroscopy indicates associations between serum 3,5-T<sub>2</sub> concentrations and intermediary metabolism in euthyroid humans. Eur Thyroid J. (2015) 4 (Suppl. 1):92–100. doi: 10.1159/000381308
15. Soldin OP, Soldin SJ. Thyroid hormone testing by tandem mass spectrometry. Clin Biochem. (2011) 44:89–94. doi: 10.1016/j.clinbiochem.2010.07.020.
16. Carvalho VM. The coming of age of liquid chromatography coupled to tandem mass spectrometry in the endocrinology laboratory. J Chromatogr B Anal Technol Biomed Life Sci. (2012) 883–884:50–8. doi: 10.1016/j.jchromb.2011.08.027
17. Richards K, Rijntjes E, Rathmann D, Köhrle J. Avoiding the pitfalls when quantifying thyroid hormones and their metabolites using mass spectrometric methods: the role of quality assurance. Mol Cell Endocrinol. (2017) 58:44–56. doi: 10.1016/j.mce.2017.01.032
18. Donzelli R, Colligiani D, Kusmic C, Sabatini M, Lorenzini L, Accorroni A, et al. Effect of hypothyroidism and hyperthyroidism on tissue thyroid hormone concentrations in rat. Eur Thyroid J. (2016) 5:27–34. doi: 10.1159/000443523
19. Jonklaas J, Sathasivam A, Wang H, Finigan D, Soldin O, Burman K, et al. 3,3′-Diiodothyronine concentrations in hospitalized or thyroidectomized patients: results from a pilot study. Endocr Pract. (2014) 20:797–807. doi: 10.4158/EP13453.OR
20. Hansen M, Luong X, Sedlak DL, Helbing CC, Hayes T. Quantification of 11 thyroid hormones and associated metabolites in blood using isotope-dilution liquid chromatography tandem mass spectrometry. Anal Bioanal Chem. (2016) 408:5429–42. doi: 10.1007/s00216-016-9614-9
21. Li ZM, Giesert F, Vogt-Weisenhorn D, Main KM, Skakkebæk NE, Kiviranta H, et al. Determination of thyroid hormones in placenta using isotope-dilution liquid chromatography quadrupole time-of-flight mass spectrometry. J Chromatogr A (2018) 1534:85–92. doi: 10.1016/j.chroma.2017.12.048
Keywords: thyroid hormones, thyroid hormones metabolites, 3, 5-diiodo-L-thyronine, mass spectrometry, T2
Citation: Lorenzini L, Nguyen NM, Sacripanti G, Serni E, Borsò M, Saponaro F, Cecchi E, Simoncini T, Ghelardoni S, Zucchi R and Saba A (2019) Assay of Endogenous 3,5-diiodo-L-thyronine (3,5-T2) and 3,3′-diiodo-L-thyronine (3,3′-T2) in Human Serum: A Feasibility Study. Front. Endocrinol. 10:88. doi: 10.3389/fendo.2019.00088
Received: 09 November 2018; Accepted: 31 January 2019;
Published: 19 February 2019.
Edited by:
Pieter de Lange, Università Degli Studi Della Campania Luigi Vanvitelli Caserta, ItalyReviewed by:
Paul Webb, California Institute for Regenerative Medicine, United StatesPaul Michael Yen, Duke-NUS Medical School, Singapore
Copyright © 2019 Lorenzini, Nguyen, Sacripanti, Serni, Borsò, Saponaro, Cecchi, Simoncini, Ghelardoni, Zucchi and Saba. This is an open-access article distributed under the terms of the Creative Commons Attribution License (CC BY). The use, distribution or reproduction in other forums is permitted, provided the original author(s) and the copyright owner(s) are credited and that the original publication in this journal is cited, in accordance with accepted academic practice. No use, distribution or reproduction is permitted which does not comply with these terms.
*Correspondence: Federica Saponaro, ZmVkZXJpY2Euc2Fwb25hcm9AZ21haWwuY29t
Riccardo Zucchi, cmljY2FyZG8uenVjY2hpQG1lZC51bmlwaS5pdA==
Alessandro Saba, YWxlc3NhbmRyby5zYWJhQG1lZC51bmlwaS5pdA==