- 1Department of Growth and Reproduction, Copenhagen University Hospital, Rigshospitalet, Copenhagen, Denmark
- 2International Center for Research and Research Training in Endocrine Disruption of Male Reproduction and Child Health (EDMaRC), Rigshospitalet, University of Copenhagen, Copenhagen, Denmark
Aim: Evidence suggests that bisphenol A diglycidyl ether (BADGE), bisphenol A (BPA), and BPA analogs can interfere with human male fertility. However, the effect directly on human sperm function is not known. The CatSper Ca2+ channel in human sperm controls important sperm functions and is necessary for normal male fertility. Environmental chemicals have been shown to activate CatSper and thereby affect Ca2+ signaling in human sperm. BPA has previously been investigated for effects on Ca2+ signaling human sperm, whereas the effects of other BPA analogs are currently unknown. The aim of this study is thus to characterize the effect of BADGE, BPA, and the eight analogs BPG, BPAF, BPC, BPB, BPBP, BPE, BPF, BPS on Ca2+ signaling, and CatSper in human sperm.
Methods: Direct effects of the bisphenols on Ca2+ signaling in human sperm cells were evaluated using a Ca2+ fluorimetric assay measuring changes in intracellular Ca2+. Effects via CatSper were assessed using the specific CatSper inhibitor RU1968. Effects on human sperm function was assessed using an image cytometry-based acrosome reaction assay and the modified Kremer's sperm–mucus penetration assay.
Results: At 10 μM the bisphenols BPG, BPAF, BPC, BADGE, BPB, and BPBP induced Ca2+ signals in human sperm cells, whereas BPE, BPF, BPS, and BPA had no effect. The efficacy of the chemicals at 10 μM is BPG > BPAF > BPC > BADGE > BPB > BPBP. Dose-response relations of BPG, BPAF, BPC, BADGE, BPB, and BPBP yielded EC50-values in the nM-μM range. The induced Ca2+ signals were almost completely abolished using the CatSper inhibitor RU1968, indicating an effect of the bisphenols on CatSper. All bisphenols, except BPBP, were found to dose-dependently inhibit progesterone-induced Ca2+ signals, with BPG and BPAF displaying inhibition even in low μM doses. BPG and BPAF were shown to affect human sperm function in a progesterone-like manner.
Conclusion: Our results show that the bisphenols BPG, BPAF, BPC, BADGE, BPB, and BPBP can affect Ca2+ signaling in human sperm cells through activation of CatSper. This could potentially disrupt human sperm function by interfering with normal CatSper-signaling and thus be a contributing factor in human infertility, either alone or in mixtures with other chemicals.
Introduction
Humans are widely exposed to bisphenol A (BPA), a high-production-volume chemical (1), and bisphenol A diglycidyl ether (BADGE), both widely used in the production of, e.g., epoxy resins and food container linings (2). Due to concerns of the safety of BPA, it is increasingly substituted with analogous chemicals (3, 4). Although evidence suggests that BPA and its analogs can interfere with human male fertility (4–8), the effects directly on human sperm function are less well-studied.
Ca2+ signaling is a key regulator of human sperm function (9). The CatSper Ca2+ channel is the principal Ca2+ channel in human sperm (10, 11) and is activated by the female sex steroid progesterone, released in high amounts from the cumulus cells surrounding the oocyte (10, 12). The activation of CatSper by progesterone controls important sperm functions (13). A suboptimal progesterone-induced Ca2+ influx is associated with reduced male fertility (14–20) and men who lack functional CatSper are sterile (18, 21–29), illustrating the importance of CatSper and Ca2+ signaling for normal male fertility. Studies have shown that human CatSper can be promiscuously activated by various signaling molecules (30), steroids (31, 32), small molecules (33), and environmental chemicals (34–39). As only BPA, and none of its structural analogs, has previously been investigated for effects on Ca2+ signaling in human sperm cells (34, 40), we set out to screen BADGE, BPA, and its eight structural analogs BPG, BPAF, BPC, BPB, BPBP, BPE, BPF, BPS for effects on Ca2+ signaling, and CatSper in human sperm, as well as on human sperm cell function.
Materials and Methods
Chemicals and Reagents
Bisphenols were purchased from Sigma-Aldrich (MO, USA) and dissolved in DMSO at a stock concentration of 10 mM. Progesterone, prostaglandin-E1 (PGE1) and ionomycin were obtained from Sigma-Aldrich (MO, USA) and dissolved in DMSO at stock concentrations of 20, 20, and 1 mM, respectively. RU1968 was obtained from Professor Timo Strünker and dissolved in DMSO at a stock concentration of 10 mM. Fluo-4, AM, and BCECF, AM were purchased from Invitrogen (CA, USA). Fluorescein isothiocyanate conjugated Pisum sativum agglutinin (FITC-PSA), and 4,000 cP methylcellulose were obtained from Sigma-Aldrich (MO, USA). Propidium iodide (PI), Hoechst-33342 (Hoechst), and S100 were obtained from ChemoMetec A/S (Allerød, Denmark). Human serum albumin (HSA) was obtained from Irvine Scientific (CA, USA).
Semen Samples and Ethical Approval
Healthy human volunteers donated the semen samples after their prior consent. The semen samples were produced by masturbation and ejaculated into wide-mouthed plastic containers, on the same day as the experiment and allowed to liquefy for 15–30 min at 37°C before the purification of motile sperm cells via swim-up. The volunteers were recruited from the semen donor corps, which is routinely donating samples for quality control analyses at the Department of Growth and Reproduction, Rigshospitalet. All volunteers fulfilled WHO criteria for normal sperm quality. After delivery, the samples were fully anonymized and no data on the donors fertility status, general health, or exposure to bisphenols were provided. We presumed that the donors were exposed to the same levels of bisphenols as the general population. Each donor received a fee of 500 DKK (about 75 UD dollars) per sample for their inconvenience. All samples were analyzed on the same day of delivery and destroyed immediately after the laboratory analyses. Each experimental replicate was thus based on sperm cells from a single sperm sample. Because of the full anonymization of the samples and the destruction of the samples immediately after the laboratory analyses, no ethical approval was needed for this work, according to the regional scientific ethical committee of the Capital Region of Denmark.
Purification of Motile Sperm Cells via Swim-Up
Motile spermatozoa were isolated from the semen sample by the swim-up method (41). Briefly 1 mL of semen was gently placed in the bottom of a 50 mL tube containing 4 mL of human tubular fluid (HTF+) medium with the composition: 97.8 mM NaCl, 4.69 mM KCl, 0.2 mM MgSO4, 0.37 mM KH2PO4, 2.04 mM CaCl2, 0.33 mM Na-pyruvate, 21.4 mM Na-lactate, 2.78 mM glucose, 21 mM HEPES, and 4 mM NaHCO3, adjusted to pH 7.3–7.4 with NaOH. After 1 h at 37°C, the upper swim-up fraction was carefully removed and after two washes, the sperm concentration was determined by image cytometry (42) and the sample adjusted to 10 × 106 sperm cells/ml in HTF+ with human serum albumin (3 mg/ml). Hereafter the sperm cells were incubated for at least 1 h at 37°C. For the experiments with capacitated sperm cells, the semen samples were resuspended in a capacitating medium with the following composition: 72.8 mM NaCl, 4.69 mM KCl, 0.2 mM MgSO4, 0.37 mM KH2PO4, 2.04 mM CaCl2, 0.33 mM Na-pyruvate, 21.4 mM Na-lactate, 2.78 mM glucose, 21 mM HEPES, and 25 mM NaHCO3, adjusted to pH 7.3–7.4 with NaOH. Human serum albumin (3 mg/ml) was added to the capacitating medium and the sperm cells were incubated for >3 h at 37°C in a 5% CO2 atmosphere.
Measurement of Changes in [Ca2+]i
Changes in the free intracellular Ca2+ concentration [Ca2+]i in human sperm cells were measured in 384 multi-well-plates in a fluorescence plate reader (Fluostar Omega, BMG Labtech, Germany) at 30°C as described in Rehfeld et al. (41). Briefly, sperm cells were incubated with the fluorescent Ca2+ indicator Fluo-4, AM (10 μM) for 45 min at 37°C. Excess dye was removed by centrifugation (700 × g, 10 min, RT) and the sperm pellet was resuspended in HTF+ to 5 × 106 sperm cells/mL. Aliquots of 50 μL were loaded to the wells of a 384-well-plate using an automatic repeater pipette. Fluorescence was excited at 480 nm and emission was recorded at 520 nm with bottom optics. Fluorescence was recorded before and after addition of 25 μL bisphenol solutions, negative control (buffer with vehicle), positive control (progesterone, 5 μM final concentration) manually with an electronic multichannel pipette to duplicate wells. Changes in Fluo-4 fluorescence are shown as ΔF/F0 (%), indicating the percentage change in fluorescence (ΔF) with respect to the mean basal fluorescence (F0) before addition of bisphenols, positive control, and negative control. For the inhibition studies mean basal fluorescence (F0) was defined as the last 5 cycles before addition of 100 nM progesterone.
Measurement of Changes in pH(i)
Changes in pH(i) in human sperm cells were measured in 384-well-plates in a fluorescence plate reader (Fluostar Omega, BMG Labtech, Germany) at 30°C as in Schiffer et al. (34). Sperm cells were loaded with the fluorescent pH indicator BCECF (10 μM) for 15 min at 37°C. Excess dye was removed by centrifugation (700 × g, 10 min, RT) and the sperm pellet was resuspended in HTF+ to 5 × 106 sperm/ml. Aliquots of 50 μL were loaded to the wells of the multi-well-plate. Fluorescence was excited at 440 and 480 nm (dual excitation) and emission was recorded at 520 nm with bottom optics. Fluorescence was recorded before and after addition of 25 μL of bisphenol solutions, negative control (buffer with vehicle), positive control (NH4CL, 30 mM final concentration) manually with an electronic multichannel pipette to duplicate wells. Changes in the ratio of BCECF fluorescence between the 440 and 480 nm excitation are shown as ΔR/R0 (%), indicating the percentage change in the ratio of fluorescence between the two modes of excitation (ΔR) with respect to the mean basal ratio of fluorescence between the two modes of excitation (R0) before addition of bisphenols, positive control, and negative control.
Assessment of Sperm Penetration Into a Viscous Medium
Assessment of sperm penetration was done using sperm penetration tests with 4,000 cP methylcellulose (1% w/v) as an artificial viscous medium as described in Alasmari et al. (43). The viscous methylcellulose (1% w/v) medium was prepared in HTF+ by adding 10 mg methylcellulose per mL HTF+ and mixing it by rotation overnight at RT. The viscous methylcellulose (1% w/v) medium was introduced into glass capillary tubes [borosilicate microslides (VitroTubes) 0.20 mm × 2.0 mm × 10 cm (VitroCom, USA)] by capillary forces, by placing the glass tubes vertically in a 1.5 mL microfuge tube with 750 μL methylcellulose (1% w/v) for 15min. Care was taken to prevent air bubbles from entering the glass tubes. The end of the glass tube that was placed in the microfuge tube was sealed with wax (Hounisens laboratorieudstyr A/S, Denmark). Hereafter the other end was cut within the part filled with methylcellulose, just before the methylcellulose-air transition, and additional wax was added to the other end to push out a small droplet of methylcellulose at the cut end. The cut end is then placed in a 1.4 mL tube (Eppendorf, Germany) with 200 μL non-capacitated sperm sample (10 × 106/ml in HTF+). Just prior to the insertion of the glass tubes, either bisphenols (10 μM), 5 μM progesterone (positive control), 5 μM PGE1, or 0.1% DMSO (negative control) were added to the sperm sample. The sperm cells were allowed to migrate into the methylcellulose (1% w/v) for 60 min at 37°C. The glass tube was then removed, wiped to remove residual sperm cells from the surface of the glass, placed under a UV lamp (302 nm) in a BIO-RAD Universal Hood III (BIO-RAD, CA, US) for 3 min to paralyze the sperm cells (44) and hereafter examined using phase contrast optics on an Olympus BX45 microscope at a total magnification of ×200 (Olympus, Denmark). The number of sperm cells were counted at 2 cm distance from the opening of the tube, with two fields in each of four planes counted. Throughout the study, all samples were counted by the same observer. Only experiments with a positive increment in cell density at 2 cm for the positive control compared to the negative control and with more than 40 sperm cells counted at 2 cm for the positive control were used for the analysis.
Assessment of Acrosome Reaction
The amount of live acrosome reacted sperm cells was measured using an image cytometry-based acrosome reaction assay, as described in Rehfeld et al. (41). Briefly, capacitated sperm cells (10 × 106/ml) were divided into equal aliquots and mixed thoroughly with a staining solution containing 5 μg/mL FITC-PSA, 0.5 μg/mL PI, and 10 μg/mL Hoechst in HTF+. Bisphenols (10 μM) were added to the aliquots of stained capacitated sperm cells together with the positive controls, ionomycin (10 μM), and progesterone (10 μM). As a negative control, HTF+ with 0.2% DMSO was used. After addition of bisphenols and controls the samples were mixed well and placed on a gentle mixing heating plate at 37°C. After 30 min of incubation, the aliquots were mixed thoroughly by pipetting and a 50 μL sample was drawn and added to a 100 μL aliquot of an immobilizing solution containing 0.6 M NaHCO3 and 0.37% (v/v) formaldehyde in distilled water. This solution was mixed well by pipetting, immediately loaded in an A2 slide (ChemoMetec A/S, Allerød, Denmark) and assessed in a NC-3000 image cytometer (ChemoMetec A/S, Allerød, Denmark). The following protocol was applied: 2-color flexicyte with Hoechst defining the sperm cells to be analyzed; Ex475-Em560/35: exposure time 3,000 ms, Ex530-Em675/75: exposure time 500 ms, with a minimum of 5,000 analyzed cells (positive for Hoechst). PI intensity as a function of FITC-PSA intensity was plotted on bi-exponential scales and specific quadrant gates were used to distinguish four groups:
1. PI positive and FITC-PSA positive cells: Acrosome reacted non-viable sperm cells.
2. PI negative and FITC-PSA positive cells: Acrosome reacted viable sperm cells.
3. PI positive and FITC-PSA negative cells: Acrosome intact non-viable sperm cells.
4. PI negative and FITC-PSA negative cells: Acrosome intact viable sperm cells.
Only experiments with an increase of live acrosome reacted sperm cells for both positive controls compared to the negative control at ≥100% were included in the analysis.
Statistical Analysis
Data from sperm penetration tests and the acrosome reaction assay were analyzed using a mixed effects model with Geissner-Greenhouse correction. Normality was assumed based on a QQ-plot of residuals. P-values were corrected for multiple comparison type I error inflation by Dunnett's method. Statistical analyses were performed using GraphPad Prism 8.3.1 (GraphPad Software Inc., USA).
Results
Bisphenols Induce Ca2+ Signals in Human Sperm Cells
We investigated the 10 bisphenols BADGE, BPA, BPG, BPAF, BPC, BPB, BPBP, BPE, BPF, and BPS for their ability to induce Ca2+ signals in human sperm cells (Table 1), using a Ca2+ fluorimetric assay (34). The bisphenols were screened at a concentration of 10 μM, along a positive control (progesterone, 5 μM), and negative control (HTF+ with vehicle). Changes in [Ca2+]i were recorded for 4 min after addition of the chemicals and controls. We calculated the relative peak Ca2+ signal in % induced by the bisphenols, by dividing the peak Ca2+ signal with that of the paired positive control, in order to compare data from the different experiments. Six of the ten bisphenols tested induced a mean relative peak Ca2+ signal larger than that of negative controls (HTF+ with vehicle) ± 3 × SD (0.0 ± 3 × 2.3%, giving a maximal value of 6.9%, Table 1). These six bisphenols were categorized as “positive hits” and investigated in further detail.
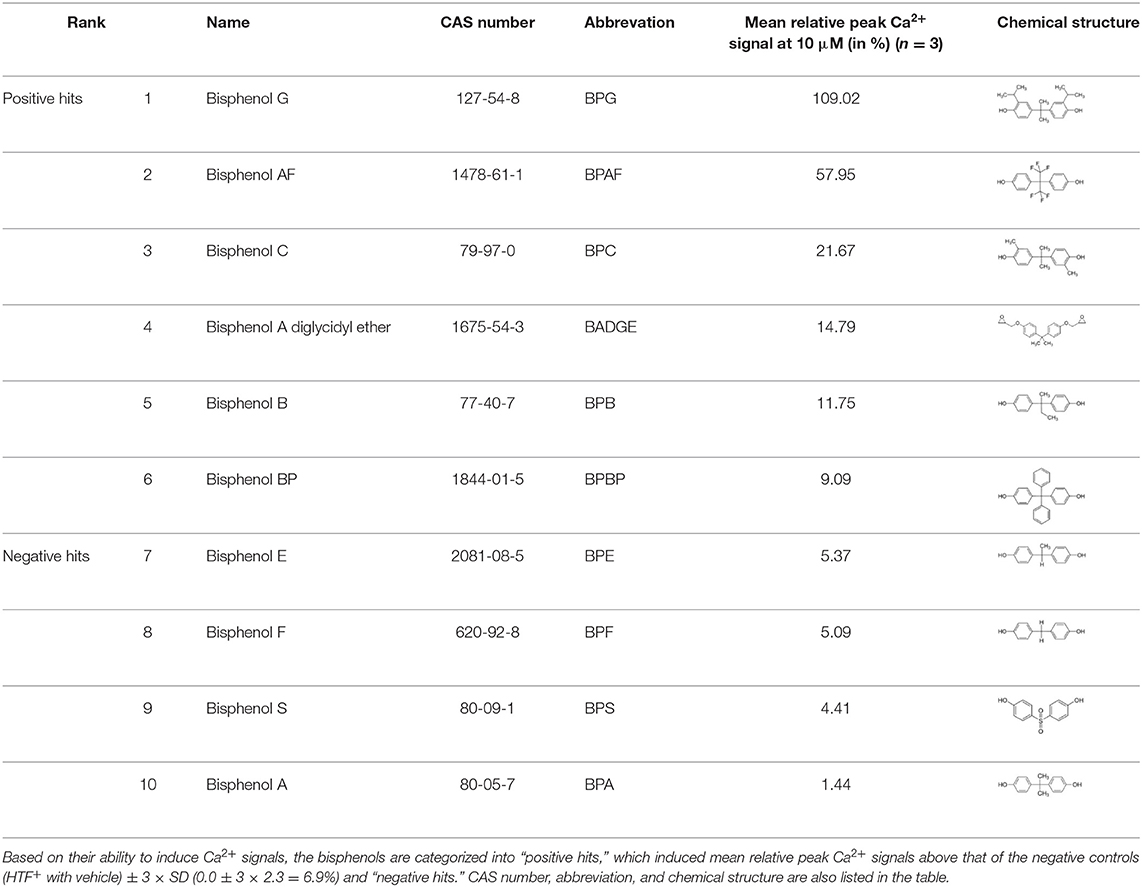
Table 1. Bisphenols ranked according to the mean relative peak Ca2+ signal induced at 10 μM, i.e., the peak Ca2+ signal induced by the bisphenol at 10 μM divided by the peak Ca2+ signal induced by progesterone at 5 μM in the same experiment.
Dose Response Relationship for the “Positive Hit” Bisphenols
Dose response relations were assessed for the “positive hit” bisphenols to examine whether they induced Ca2+ signals in human sperm cells at physiologically relevant levels. Saturating dose response relations could be made for all six bisphenols, with mean EC50-values within the concentration range 0.79–15.87 μM and mean EC05-values within the concentration range 0.18–2.37 μM (Table 2, Figure 1).
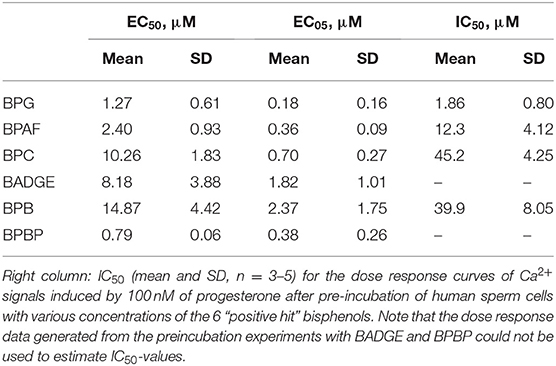
Table 2. Left and middle columns: EC50 and EC05 for the dose response curves (mean and SD, n = 3–7) of all “positive hit” bisphenols.
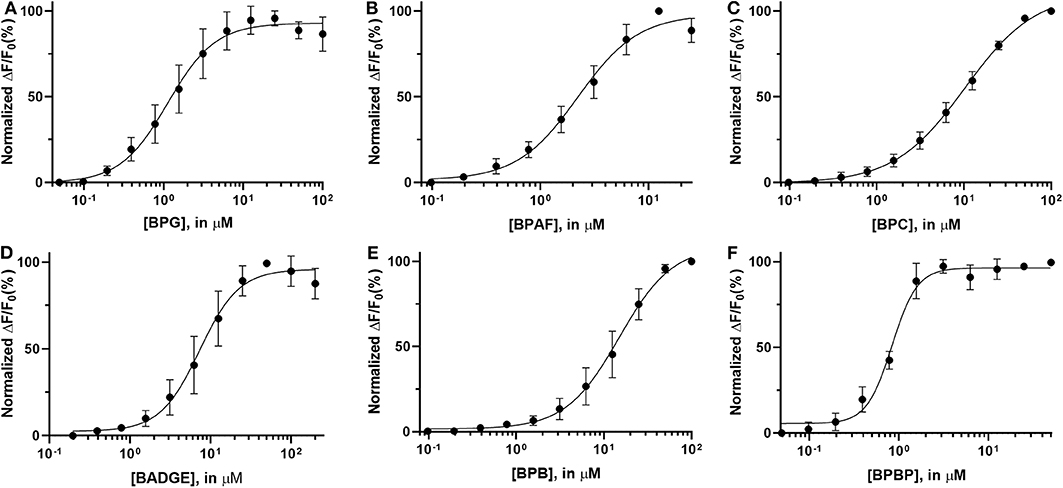
Figure 1. Normalized dose response curves (mean ± SD) for “positive hit” bisphenols. (A) BPG, (B) BPAF, (C) BPC, (D) BADGE, (E) BPB, and (F) BPBP, n = 3–7.
Bisphenols Induce Ca2+ Signals Through an Activation of CatSper
To test if the six “positive hit” bisphenols induced Ca2+ signals through CatSper, we used the novel and specific CatSper inhibitor RU1968 (13). We compared the Ca2+ signals induced by the bisphenols at doses inducing peak Ca2+ signals (5–50 μM) and progesterone at 5 μM, in the presence or absence of 30 μM of RU1968 (Figure 2). We found that the Ca2+ signals induced by all six bisphenols, like that of progesterone, were highly inhibited by RU1968. This strongly indicates that the bisphenols induce Ca2+ signals via a specific activation of CatSper in human sperm cells. Furthermore, the shape of the Ca2+ signals induced by the bisphenols at these doses, except BPBP, which only induce a small Ca2+ signal, resembles that induced by progesterone (Figure 2). This further indicates an action of the bisphenols on CatSper. As CatSper can be activated both by the endogenous ligands progesterone and prostaglandins, as well as by intracellular alkalization, we examined if the bisphenols induced changes in pH(i). At bisphenol doses inducing peak Ca2+ signals (5–50 μM) no increase pH(i) was observed (n = 3, Figure 3). This suggests that the bisphenols most likely act on the ligand-dependent pathway of either progesterone or prostaglandins leading to activation of CatSper.
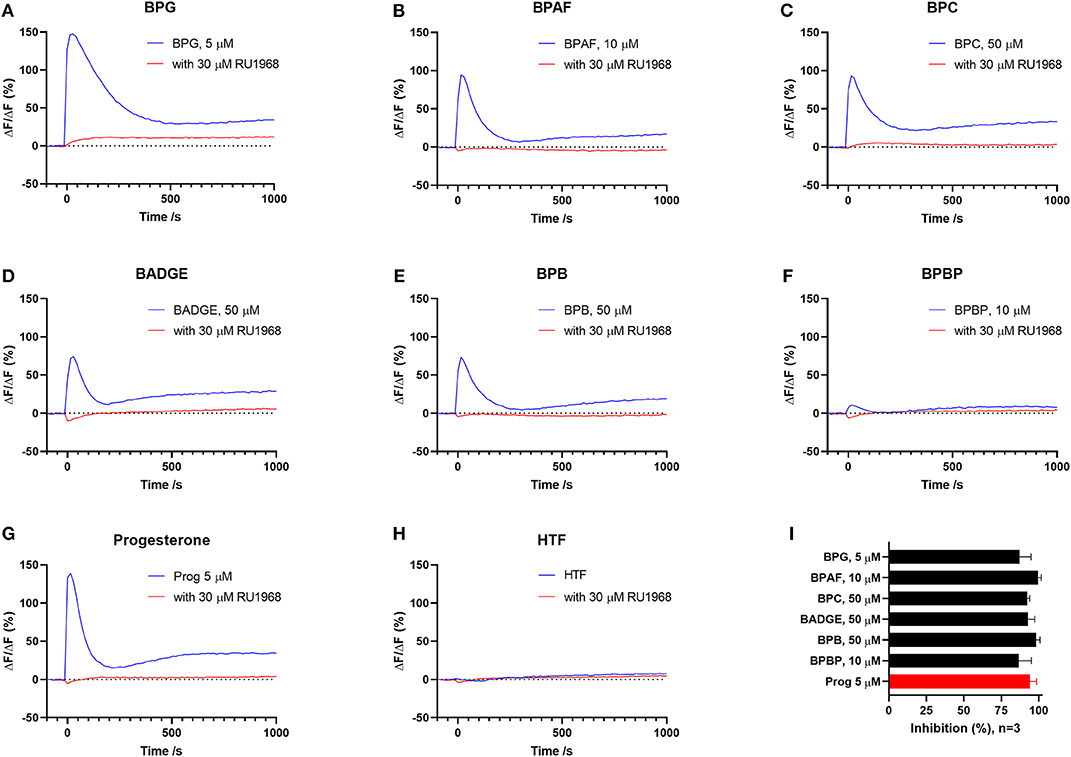
Figure 2. Ca2+ signals induced by (A) BPG, (B) BPAF, (C) BPC, (D) BADGE, (E) BPB, (F) BPBP, (G) the endogenous CatSper ligand progesterone, and (H) HTF+ buffer in the absence and presence of CatSper inhibitor RU1968, 30 μM. (I) Mean inhibition (in %) of the induced Ca2+signal ±SD in the presence of RU1968, 30 μM (n = 3).
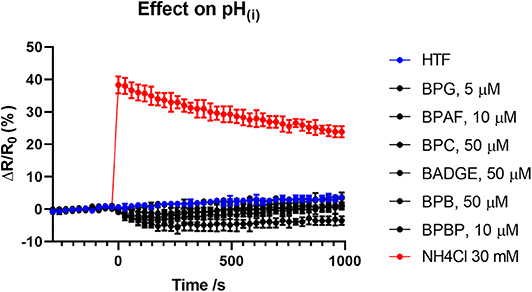
Figure 3. Changes (mean ± SD) in pH(i) induced by the bisphenols (5–50 μM), HTF+ buffer and positive control NH4Cl at 30 mM (n = 3).
Bisphenols Dose-Dependently Inhibit Progesterone-Induced Ca2+ Signals
As the bisphenols were found to induce Ca2+ signals through CatSper we examined whether pre-incubating the human sperm cells with the bisphenols could inhibit progesterone-induced Ca2+ signals. We compared the amplitude of the Ca2+ signals induced by 100 nM of progesterone in human sperm cells after 5 min of pre-incubation with serially diluted doses of the bisphenols or a negative buffer control. Our results showed that all bisphenols, except BPBP, were able to dose dependently inhibit the progesterone-induced Ca2+ signals (Figure 4). The mean IC50-values estimated from the fitted dose response curves were within the concentration range 1.86–45.2 μM (Table 2).
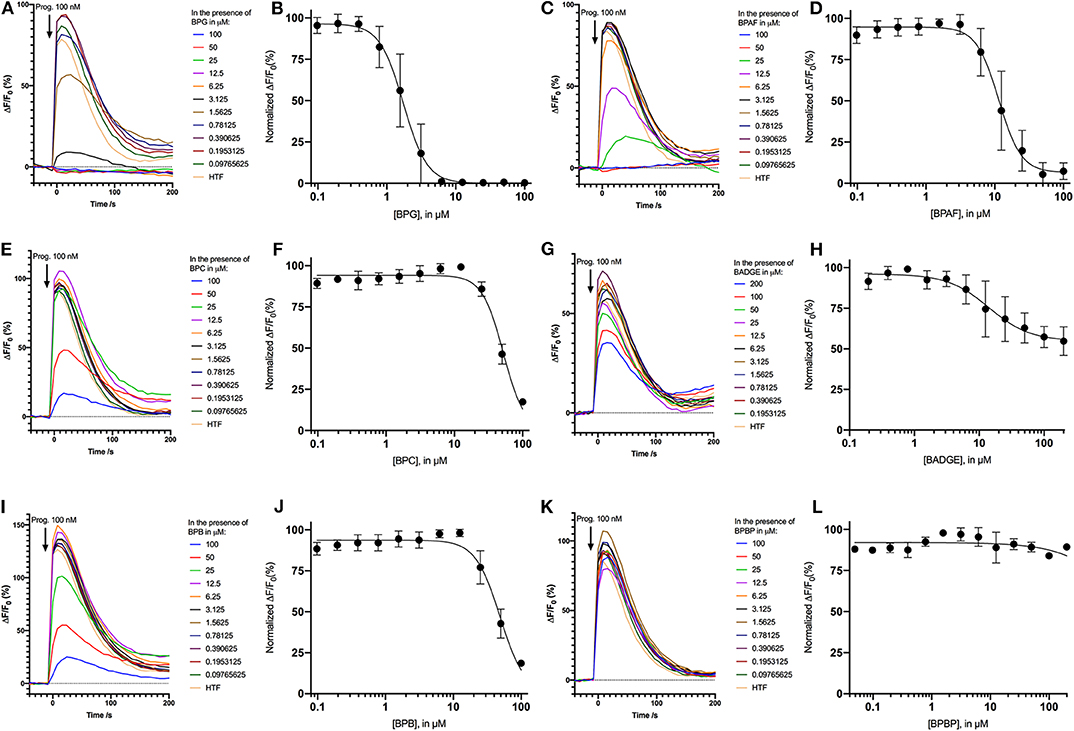
Figure 4. Inhibition of Ca2+ signals induced by 100 nM progesterone after 5 min of pre-incubation with the negative control HTF and different concentrations the bisphenols: (A) BPG, (C) BPAF, (E) BPC, (G) BADGE, (I) BPB, and (K) BPBP. Normalized dose response relations (n = 3−5) of Ca2+ signals induced by 100 nM progesterone after 5 min of pre-incubation with the different concentrations the bisphenols: (B) BPG, (D) BPAF, (F) BPC, (H) BADGE, (J) BPB, and (L) BPBP.
Effects of Bisphenols on CatSper-Mediated Human Sperm Responses
To examine whether the bisphenols could affect CatSper-mediated human sperm responses, we examined the effect of the two most efficacious bisphenols, BPG and BPAF at 10 μM, on sperm penetration into a viscous medium, as well as on acrosome reaction. BPG and BPAF were found to induce a significant increase in the numbers of human sperm cells penetrating into a viscous medium (Figure 5), similar to the effect of the endogenous CatSper ligands, progesterone, and prostaglandin E1 at 5 μM. Furthermore, BPG and BPAF were found to induce a significant increase in live acrosome reacted sperm cells (Figure 6), similar to the effect of the endogenous CatSper ligand progesterone at 10 μM, in capacitated human sperm cells.
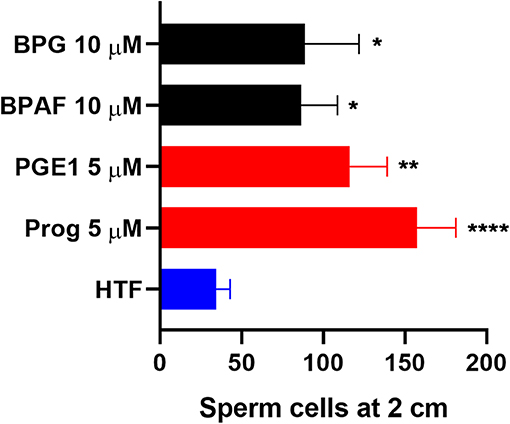
Figure 5. Human sperm cells at 2 cm into a viscous medium (mean ± SEM) after treatment with negative control (HTF+ with 0.1% DMSO “HTF”), positive controls (5 μM progesterone “Prog” and prostaglandin E1 “PGE1”), 10 μM BPG, and 10 μM BPAF (n ≥ 5). Statistics from multiple comparison between negative control and treatments: ****adjusted P ≤ 0.0001; **adjusted P = 0.0029; *adjusted P ≤ 0.0295.
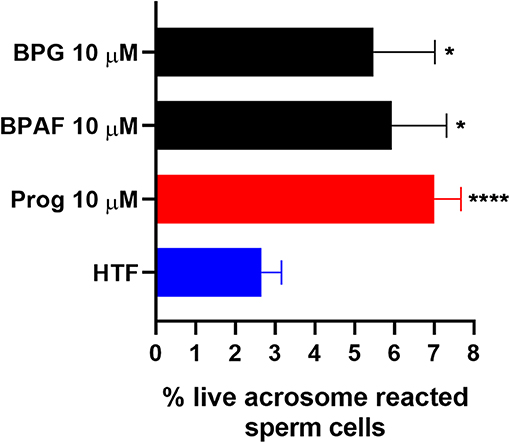
Figure 6. Percentage live acrosome reacted sperm cells (mean ± SEM) after 30 min treatment of capacitated human sperm cells with negative control (HTF+ with 0.2% DMSO “HTF”), positive control (10 μM progesterone “Prog”), 10 μM BPG, and 10 μM BPAF (n ≥ 8). Statistics from multiple comparison between negative control and treatments: ****adjusted P ≤ 0.0001; *adjusted P ≤ 0.0249.
Discussion
Our study showed that BADGE and the five bisphenol analogs BPG, BPAF, BPC, BPB, and BPBP can induce Ca2+ signals in human sperm cells at 10 μM, whereas BPA and three other bisphenols BPE, BPF, and BPS induced no Ca2+ signals in human sperm cells at this concentration (Table 1). The efficacy of the chemicals at 10 μM was BPG > BPAF > BPC > BADGE > BPB. It seems that the bisphenols with larger/bulkier side chains are more efficacious and that relatively small molecular differences between the bisphenols can alter their effects significantly (Table 1). This is in line with a previous study showing that the read-across approach was non-applicable for otherwise structurally comparable bisphenols (45). Interestingly, low doses of BPAF, BPB, BPF, BPS, and BPA have all been shown to induce Ca2+ signals in SKBR3 cells via the G protein-coupled estrogen receptor (GPER) (46), with BPAF and BPB being more efficacious than BPF, BPS, and BPA. This is somewhat similar to our findings, although we in our assay see no effect for BPF, BPS, and BPA. Furthermore, even though BPA showed no effect in our assay, it has been shown both to activate (47) and inhibit other voltage-activated Ca2+ channels (48).
The induced Ca2+ signals could be used to form saturating dose response curves for all six “positive hit” bisphenols (Figure 1). The EC50-values estimated from these curves ranged from 0.79 to 14.87 μM and the lowest effective dose values (EC05) ranged from 0.18 to 2.37 μM (Table 2). In the literature, we could only identify human plasma or serum levels for BPAF, BADGE, and BPB out of the six “positive hit” bisphenols (49–53). A reported maximal human serum concentration of BADGE (3.45 μM) (50) is above the EC05 estimated in our study (1.82 μM), whereas the reported maximal human serum levels of BPAF (0.05 μM) (50) and BPB (0.59 μM) (50) are below the estimated EC05-values of 0.36 μM for BPAF and 2.37 μM for BPB.
We found that the induced Ca2+ signals were almost completely inhibited by the specific CatSper inhibitor RU1968 (Figure 2), like the Ca2+ signal induced by the endogenous CatSper ligand progesterone. This indicates that the six bisphenols induce Ca2+ signals in human sperm cells via CatSper. Furthermore, the shape of the Ca2+ signals induced by all bisphenols, except BPBP, which only induced a small peak Ca2+ signal, resembled that of the Ca2+ signal induced by progesterone (Figure 2), similarly suggesting an effect of these bisphenols on CatSper. Human CatSper can be activated by a ligand-dependent pathway, by the endogenous CatSper ligands progesterone and prostaglandins (10, 12), as well as by a ligand-independent pathway through intracellular alkalization (10, 12). Our data showed that the induction of Ca2+ signals by the bisphenols is not due to an increase in pH(i) (Figure 3), suggesting that the bisphenols act on the ligand-dependent pathways of either progesterone or prostaglandins leading to activation of CatSper in human sperm cells (10, 12). Interestingly, progesterone has been suggested to activate CatSper through an activation of the enzyme ABHD2, whereas the molecular target of prostaglandins leading to CatSper activation remains unknown (54). The direct action of the bisphenols on the ligand-dependent pathway leading to activation of CatSper in human sperm cells, is similar to what has been shown for multiple other environmental chemicals previously (34, 35, 55).
Pre-incubation of the human sperm cells with the bisphenols BPG, BPAF, BPC, BADGE, and BPB was found to dose-dependently inhibit progesterone-induced Ca2+ signals (Figure 4). BPG and BPAF were found to be much more potent inhibitors of progesterone-induced Ca2+ signals than the other bisphenols, which only inhibited progesterone-induced Ca2+ signals at high μM doses (Table 2). Exposure of human sperm cells to these bisphenols may thereby inhibit the action of progesterone on CatSper, as has been shown for other environmental chemicals acting on the ligand-dependent pathway (34–36, 38).
In addition, our results showed that the two most efficacious bisphenols at 10 μM, BPG and BPAF, could both increase sperm penetration into a viscous medium, like the response induced by the endogenous CatSper ligands progesterone and PGE1 (Figure 5), and induce acrosome reaction in capacitated human sperm cells, similar to the response induced by progesterone (Figure 6). Again, this is in line with previous studies where other environmental chemicals activating CatSper were found to exert progesterone-like effects on human sperm function (34, 36–38, 56).
Only few studies have examined the effect of bisphenols on human sperm cell function. One study showed that very high doses of BPA (≥300 μM) induced mitochondrial dysfunction in human sperm (57), another study showed that BPA at 0.1 nM−1 μM could affect human sperm motility parameters and that BPA at 1 μM could induce a rapid, transient increase in [Ca2+]i in a whole population of observed single human sperm cells (40), whereas BPA at 0.1, 1, and 10 μM did not affect [Ca2+]i in human sperm cells in a large screening of environmental chemicals by Schiffer et al. (34). Our results here support the findings by Schiffer et al. (34) that BPA at concentrations up to 10 μM do not induce Ca2+ signals in human sperm cells.
Our findings add BADGE and the five bisphenol analogs BPG, BPAF, BPC, BPB, and BPBP to the growing list of environmental chemicals that can induce Ca2+ signals in human sperm cells through CatSper (34–39). Studies have shown that chemicals acting on CatSper can cooperate in low dose mixtures to activate CatSper both additively (34, 35) and synergistically (55). As humans in the industrialized part of the world are suggested to be exposed to thousands of environmental chemicals (58), such a low dose mixture exposure scenario is quite realistic. This indicates that the bisphenols could affect Ca2+ signaling in human sperm cells even at doses well below the EC05, when present in mixtures with other chemicals acting on CatSper. This is important as only BADGE has been found with a maximal serum concentration (3.45 μM) (50) above the EC05 estimated in our study (1.82 μM).
Whether exposure of the human sperm cells, either within the male or female reproductive tract, to environmental chemicals acting on CatSper can interfere with the fertilization process remains to be shown. However, the fact that impaired progesterone-signaling is associated with reduced male fertility (14–20) and that men who lack functional CatSper are completely infertile (18, 21–29) hints that environmental chemicals interfering with this signaling pathway could make it more difficult for the human sperm cells to successfully locate and fertilize the oocyte. As our experiments have been performed on sperm cells in vitro future studies would be needed to validate our results and to examine the effects of exposure to bisphenols on fertility in vivo.
Data Availability Statement
The raw data supporting the conclusions of this article will be made available by the authors, without undue reservation, to any qualified researcher upon request.
Ethics Statement
Because of the full anonymization of the samples and the destruction of the samples immediately after the laboratory analyses, no ethical approval was needed for this work, according to the regional scientific ethical committee of the Capital Region of Denmark. The patients/participants provided their written informed consent to participate in this study.
Author Contributions
AR, AA, and NS conceived the study and drafted the manuscript. AR designed, planned, and performed the experiments.
Funding
This study was supported by a EDMaRC research grant from the Kirsten and Freddy Johansen's Foundation and the Danish Center on Endocrine Disrupters.
Conflict of Interest
The authors declare that the research was conducted in the absence of any commercial or financial relationships that could be construed as a potential conflict of interest.
Acknowledgments
The authors would like to acknowledge Sissel Marie Bredesen for her assistance in performing the laboratory experiments and Brian Vendelbo Hansen for his assistance with obtaining reagents and chemicals from the various providers.
References
1. Vandenberg LN, Chahoud I, Heindel JJ, Padmanabhan V, Paumgartten FJR, Schoenfelder G. Urinary, circulating, and tissue biomonitoring studies indicate widespread exposure to bisphenol A. Environ Health Perspect. (2010) 118:1055–70. doi: 10.1289/ehp.0901716
2. Chamorro-García R, Kirchner S, Li X, Janesick A, Casey SC, Chow C, et al. Bisphenol A diglycidyl ether induces adipogenic differentiation of multipotent stromal stem cells through a peroxisome proliferator-activated receptor gamma-independent mechanism. Environ Health Perspect. (2012) 120:984–9. doi: 10.1289/ehp.1205063
3. Frederiksen H, Nielsen O, Koch HM, Skakkebaek NE, Juul A, Jørgensen N, et al. Changes in urinary excretion of phthalates, phthalate substitutes, bisphenols and other polychlorinated and phenolic substances in young Danish men; 2009-2017. Int J Hyg Environ Health. (2020) 223:93–105. doi: 10.1016/j.ijheh.2019.10.002
4. Sartain CV, Hunt PA. An old culprit but a new story: bisphenol A and “NextGen” bisphenols. Fertil Steril. (2016) 106:820–6. doi: 10.1016/j.fertnstert.2016.07.1114
5. Siracusa JS, Yin L, Measel E, Liang S, Yu X. Effects of bisphenol A and its analogs on reproductive health: a mini review. Reprod Toxicol. (2018) 79:96–123. doi: 10.1016/j.reprotox.2018.06.005
6. Matuszczak E, Komarowska MD, Debek W, Hermanowicz A. The impact of bisphenol A on fertility, reproductive system, and development: a review of the literature. Int J Endocrinol. (2019) 2019:4068717. doi: 10.1155/2019/4068717
7. Cariati F, D'Uonno N, Borrillo F, Iervolino S, Galdiero G, Tomaiuolo R. “Bisphenol a: an emerging threat to male fertility.” Reprod Biol Endocrinol. (2019) 17:6. doi: 10.1186/s12958-018-0447-6
8. Desdoits-Lethimonier C, Lesné L, Gaudriault P, Zalko D, Antignac JP, Deceuninck Y, et al. Parallel assessment of the effects of bisphenol A and several of its analogs on the adult human testis. Hum Reprod. (2017) 32:1465–73. doi: 10.1093/humrep/dex093
9. Publicover S, Harper CV, Barratt C. [Ca2+]i signalling in sperm–making the most of what you've got. Nat Cell Biol. (2007) 9:235–42. doi: 10.1038/ncb0307-235
10. Strünker T, Goodwin N, Brenker C, Kashikar ND, Weyand I, Seifert R, et al. The CatSper channel mediates progesterone-induced Ca2+ influx in human sperm. Nature. (2011) 471:382–6. doi: 10.1038/nature09769
11. Lishko PV, Kirichok Y, Ren D, Navarro B, Chung J-J, Clapham DE. The control of male fertility by spermatozoan ion channels. Annu Rev Physiol. (2012) 74:453–75. doi: 10.1146/annurev-physiol-020911-153258
12. Lishko PV, Botchkina IL, Kirichok Y. Progesterone activates the principal Ca2+ channel of human sperm. Nature. (2011) 471:387–91. doi: 10.1038/nature09767
13. Rennhack A, Schiffer C, Brenker C, Fridman D, Nitao ET, Cheng Y-M, et al. A novel cross-species inhibitor to study the function of CatSper Ca2+ channels in sperm. Br J Pharmacol. (2018) 175:3144–61. doi: 10.1111/bph.14355
14. Krausz C, Bonaccorsi L, Luconi M, Fuzzi B, Criscuoli L, Pellegrini S, et al. Intracellular calcium increase and acrosome reaction in response to progesterone in human spermatozoa are correlated with in-vitro fertilization. Hum Reprod. (1995) 10:120–4. doi: 10.1093/humrep/10.1.120
15. Oehninger S, Blackmore P, Morshedi M, Sueldo C, Acosta AA, Alexander NJ. Defective calcium influx and acrosome reaction (spontaneous and progesterone-induced) in spermatozoa of infertile men with severe teratozoospermia. Fertil Steril. (1994) 61:349–54. doi: 10.1016/S0015-0282(16)56530-3
16. Shimizu Y, Nord EP, Bronson RA. Progesterone-evoked increases in sperm [Ca2+]i correlate with the egg penetrating ability of sperm from fertile but not infertile men. Fertil Steril. (1993) 60:526–32. doi: 10.1016/S0015-0282(16)56172-X
17. Falsetti C, Baldi E, Krausz C, Casano R, Failli P, Forti G. Decreased responsiveness to progesterone of spermatozoa in oligozoospermic patients. J Androl. (1993) 14:17–22.
18. Williams HL, Mansell S, Alasmari W, Brown SG, Wilson SM, Sutton KA, et al. Specific loss of CatSper function is sufficient to compromise fertilizing capacity of human spermatozoa. Hum Reprod. (2015) 30:2737–46. doi: 10.1093/humrep/dev243
19. Forti G, Baldi E, Krausz C, Luconi M, Bonaccorsi L, Maggi M, et al. Effects of progesterone on human spermatozoa: clinical implications. Ann Endocrinol (Paris). (1999) 60:107–10.
20. Krausz C, Bonaccorsi L, Maggio P, Luconi M, Criscuoli L, Fuzzi B, et al. Two functional assays of sperm responsiveness to progesterone and their predictive values in in-vitro fertilization. Hum Reprod. (1996) 11:1661–7. doi: 10.1093/oxfordjournals.humrep.a019466
21. Avenarius MR, Hildebrand MS, Zhang Y, Meyer NC, Smith LLH, Kahrizi K, et al. Human male infertility caused by mutations in the CATSPER1 channel protein. Am J Hum Genet. (2009) 84:505–10. doi: 10.1016/j.ajhg.2009.03.004
22. Smith JF, Syritsyna O, Fellous M, Serres C, Mannowetz N, Kirichok Y, et al. Disruption of the principal, progesterone-activated sperm Ca2+ channel in a CatSper2-deficient infertile patient. Proc Natl Acad Sci U S A. (2013) 110:6823–8. doi: 10.1073/pnas.1216588110
23. Hildebrand MS, Avenarius MR, Fellous M, Zhang Y, Meyer NC, Auer J, et al. Genetic male infertility and mutation of CATSPER ion channels. Eur J Hum Genet. (2010) 18:1178–84. doi: 10.1038/ejhg.2010.108
24. Avidan N, Tamary H, Dgany O, Cattan D, Pariente A, Thulliez M, et al. CATSPER2, a human autosomal nonsyndromic male infertility gene. Eur J Hum Genet. (2003) 11:497–502. doi: 10.1038/sj.ejhg.5200991
25. Zhang Y, Malekpour M, Al-Madani N, Kahrizi K, Zanganeh M, Mohseni M, et al. Sensorineural deafness and male infertility: a contiguous gene deletion syndrome. BMJ Case Rep. (2009) 2009:bcr08.2008.0645. doi: 10.1136/bcr.08.2008.0645
26. Jaiswal D, Singh V, Dwivedi US, Trivedi S, Singh K. Chromosome microarray analysis: a case report of infertile brothers with CATSPER gene deletion. Gene. (2014) 542:263–5. doi: 10.1016/j.gene.2014.03.055
27. Brown SG, Miller MR, Lishko PV, Lester DH, Publicover SJ, Barratt CLR, et al. Homozygous in-frame deletion in CATSPERE in a man producing spermatozoa with loss of CatSper function and compromised fertilizing capacity. Hum Reprod. (2018) 33:1812–816. doi: 10.1093/humrep/dey278
28. Luo T, Chen H-Y, Zou Q-X, Wang T, Cheng Y-M, Wang H-F, et al. A novel copy number variation in CATSPER2 causes idiopathic male infertility with normal semen parameters. Hum Reprod. (2019) 34:414–23. doi: 10.1093/humrep/dey377
29. Schiffer C, Rieger S, Brenker C, Young S, Hamzeh H, Wachten D, et al. Rotational motion and rheotaxis of human sperm do not require functional CatSper channels and transmembrane Ca2+ signaling. EMBO J. (2020) 39:e102363. doi: 10.15252/embj.2019102363
30. Brenker C, Goodwin N, Weyand I, Kashikar ND, Naruse M, Krähling M, et al. The CatSper channel: a polymodal chemosensor in human sperm. EMBO J. (2012) 31:1654–65. doi: 10.1038/emboj.2012.30
31. Brenker C, Schiffer C, Wagner IV, Tüttelmann F, Röpke A, Rennhack A, et al. Action of steroids and plant triterpenoids on CatSper Ca2+ channels in human sperm. Proc Natl Acad Sci U S A. (2018) 115:E344–E6. doi: 10.1073/pnas.1717929115
32. Mannowetz N, Miller MR, Lishko PV. Regulation of the sperm calcium channel CatSper by endogenous steroids and plant triterpenoids. Proc Natl Acad Sci U S A. (2017) 114:5743–8. doi: 10.1073/pnas.1700367114
33. Martins da Silva SJ, Brown SG, Sutton K, King LV, Ruso H, Gray DW, et al. Drug discovery for male subfertility using high-throughput screening: a new approach to an unsolved problem. Hum Reprod. (2017) 32:974–84. doi: 10.1093/humrep/dex055
34. Schiffer C, Müller A, Egeberg DL, Alvarez L, Brenker C, Rehfeld A, et al. Direct action of endocrine disrupting chemicals on human sperm. EMBO Rep. (2014) 15:758–65. doi: 10.15252/embr.201438869
35. Rehfeld A, Dissing S, Skakkebæk NE. Chemical UV filters mimic the effect of progesterone on Ca2+ signaling in human sperm cells. Endocrinology. (2016) 157:4297–308. doi: 10.1210/en.2016-1473
36. Zou Q-X, Peng Z, Zhao Q, Chen H-Y, Cheng Y-M, Liu Q, et al. Diethylstilbestrol activates CatSper and disturbs progesterone actions in human spermatozoa. Hum Reprod. (2017) 32:290–8. doi: 10.1093/humrep/dew332
37. Tavares RS, Mansell S, Barratt CLR, Wilson SM, Publicover SJ, Ramalho-Santos J. p,p′-DDE activates CatSper and compromises human sperm function at environmentally relevant concentrations. Hum Reprod. (2013) 28:3167–77. doi: 10.1093/humrep/det372
38. Yuan Y, Ding X, Cheng Y, Kang H, Luo T, Zhang X, et al. PFOA evokes extracellular Ca2+ influx and compromises progesterone-induced response in human sperm. Chemosphere. (2020) 241:125074. doi: 10.1016/j.chemosphere.2019.125074
39. Shannon M, Rehfeld A, Frizzell C, Livingstone C, McGonagle C, Skakkebaek NE, et al. In vitro bioassay investigations of the endocrine disrupting potential of steviol glycosides and their metabolite steviol, components of the natural sweetener Stevia. Mol Cell Endocrinol. (2016) 427:65–72. doi: 10.1016/j.mce.2016.03.005
40. Kotwicka M, Skibinska I, Piworun N, Jendraszak M, Chmielewska M, Jedrzejczak P. Bisphenol A modifies human spermatozoa motility in vitro. J Med Sci. (2016) 85:39–45. doi: 10.20883/jms.2016.5
41. Rehfeld A, Egeberg Palme DL, Almstrup K, Juul A, Skakkebaek NE. Medium-throughput screening assays for assessment of effects on Ca2+-Signaling and acrosome reaction in human sperm. J Vis Exp. (2019). doi: 10.3791/59212
42. Egeberg DL, Kjaerulff S, Hansen C, Petersen JH, Glensbjerg M, Skakkebaek NE, et al. Image cytometer method for automated assessment of human spermatozoa concentration. Andrology. (2013) 1:615–23. doi: 10.1111/j.2047-2927.2013.00082.x
43. Alasmari W, Costello S, Correia J, Oxenham SK, Morris J, Fernandes L, et al. Ca2+ signals generated by CatSper and Ca2+ stores regulate different behaviors in human sperm. J Biol Chem. (2013) 288:6248–58. doi: 10.1074/jbc.M112.439356
44. König K, Tadir Y, Patrizio P, Berns MW, Tromberg BJ. Effects of ultraviolet exposure and near infrared laser tweezers on human spermatozoa. Hum Reprod. (1996) 11:2162–4. doi: 10.1093/oxfordjournals.humrep.a019069
45. Padberg F, Tarnow P, Luch A, Zellmer S. Minor structural modifications of bisphenol A strongly affect physiological responses of HepG2 cells. Arch Toxicol. (2019) 93:1529–541. doi: 10.1007/s00204-019-02457-y
46. Cao L-Y, Ren X-M, Li C-H, Zhang J, Qin W-P, Yang Y, et al. Bisphenol AF and bisphenol B exert higher estrogenic effects than bisphenol A via G protein-coupled estrogen receptor pathway. Environ Sci Technol. (2017) 51:11423–30. doi: 10.1021/acs.est.7b03336
47. Asano S, Tune JD, Dick GM. Bisphenol A activates Maxi-K (KCa1.1) channels in coronary smooth muscle. Br J Pharmacol. (2010) 160:160–70. doi: 10.1111/j.1476-5381.2010.00687.x
48. Deutschmann A, Hans M, Meyer R, Häberlein H, Swandulla D. Bisphenol A inhibits voltage-activated Ca2+ channels in vitro: mechanisms and structural requirements. Mol Pharmacol. (2013) 83:501–11. doi: 10.1124/mol.112.081372
49. Zhang B, He Y, Zhu H, Huang X, Bai X, Kannan K, et al. Concentrations of bisphenol A and its alternatives in paired maternal-fetal urine, serum and amniotic fluid from an e-waste dismantling area in China. Environ Int. (2020) 136:105407. doi: 10.1016/j.envint.2019.105407
50. Russo G, Barbato F, Mita DG, Grumetto L. Simultaneous determination of fifteen multiclass organic pollutants in human saliva and serum by liquid chromatography-tandem ultraviolet/fluorescence detection: a validated method. Biomed Chromatogr. (2019) 33:e4427. doi: 10.1002/bmc.4427
51. Song S, Duan Y, Zhang T, Zhang B, Zhao Z, Bai X, et al. Serum concentrations of bisphenol A and its alternatives in elderly population living around e-waste recycling facilities in China: associations with fasting blood glucose. Ecotoxicol Environ Saf. (2019) 169:822–8. doi: 10.1016/j.ecoenv.2018.11.101
52. Li A, Zhuang T, Shi W, Liang Y, Liao C, Song M, et al. Serum concentration of bisphenol analogues in pregnant women in China. Sci Total Environ. (2020) 707:136100. doi: 10.1016/j.scitotenv.2019.136100
53. Wang L, Xue J, Kannan K. Widespread occurrence and accumulation of bisphenol A diglycidyl ether (BADGE), bisphenol F diglycidyl ether (BFDGE) and their derivatives in human blood and adipose fat. Environ Sci Technol. (2015) 49:3150–7. doi: 10.1021/acs.est.5b00096
54. Miller MR, Mannowetz N, Iavarone AT, Safavi R, Gracheva EO, Smith JF, et al. Unconventional endocannabinoid signaling governs sperm activation via the sex hormone progesterone. Science. (2016) 352:555–9. doi: 10.1126/science.aad6887
55. Brenker C, Rehfeld A, Schiffer C, Kierzek M, Kaupp UB, Skakkebæk NE, et al. Synergistic activation of CatSper Ca2+ channels in human sperm by oviductal ligands and endocrine disrupting chemicals. Hum Reprod. (2018) 33:1915–23. doi: 10.1093/humrep/dey275
56. Rehfeld A, Egeberg D, Almstrup K, Holm Petersen J, Dissing S, Skakkebæk NE. Chemical UV filters can affect human sperm function in a progesterone-like manner. Endocr Connect. (2017) 7:16–25.". doi: 10.1530/EC-17-0156
57. Barbonetti A, Castellini C, Di Giammarco N, Santilli G, Francavilla S, Francavilla F. In vitro exposure of human spermatozoa to bisphenol A induces pro-oxidative/apoptotic mitochondrial dysfunction. Reprod Toxicol. (2016) 66:61–7. doi: 10.1016/j.reprotox.2016.09.014
Keywords: endocrine disruption, fertility, CatSper, male reproduction, bisphenol
Citation: Rehfeld A, Andersson AM and Skakkebæk NE (2020) Bisphenol A Diglycidyl Ether (BADGE) and Bisphenol Analogs, but Not Bisphenol A (BPA), Activate the CatSper Ca2+ Channel in Human Sperm. Front. Endocrinol. 11:324. doi: 10.3389/fendo.2020.00324
Received: 05 March 2020; Accepted: 27 April 2020;
Published: 19 May 2020.
Edited by:
Arcangelo Barbonetti, University of L'Aquila, ItalyReviewed by:
Francesco Pallotti, Sapienza University of Rome, ItalyElisabetta Baldi, University of Florence, Italy
Copyright © 2020 Rehfeld, Andersson and Skakkebæk. This is an open-access article distributed under the terms of the Creative Commons Attribution License (CC BY). The use, distribution or reproduction in other forums is permitted, provided the original author(s) and the copyright owner(s) are credited and that the original publication in this journal is cited, in accordance with accepted academic practice. No use, distribution or reproduction is permitted which does not comply with these terms.
*Correspondence: Anders Rehfeld, cmVoZmVsZEBzdW5kLmt1LmRr