- 1Infertility and IVF Unit, Department of Obstetrics and Gynecology, Chaim Sheba Medical Center (Tel Hashomer), Ramat Gan, Israel
- 2Sackler Faculty of Medicine, Tel Aviv University, Tel Aviv, Israel
- 3The Tarnesby-Tarnowski Chair for Family Planning and Fertility Regulation, Sackler Faculty of Medicine, Tel-Aviv University, Tel Aviv, Israel
- 4Centre for Big Data Research in Health & School of Women’s and Children’s Health, UNSW Medicine, University of New South Wales, Sydney, NSW, Australia
- 5IVF Australia, Sydney, NSW, Australia
- 6Assisted Reproductive Technology (ART), Fertility Clinics, Abu Dhabi, United Arab Emirates
- 7Global Medical Affairs, Research and Development, Merck Healthcare KGaA, Darmstadt, Germany
- 8Research Group Reproductive Medicine, Department of Development and Regeneration, Organ Systems, Group Biomedical Sciences, KU Leuven (University of Leuven), Leuven, Belgium
- 9Department of Obstetrics, Gynecology, and Reproductive Sciences, Yale School of Medicine, New Haven, CT, United States
- 10IVF Unit, Fertility Center Hamburg, Hamburg, Germany
- 11Center of Reproduction “Life Line”, Moscow, Russia
- 12Department of Obstetrics and Gynecology, Russian Medical Academy of Continuous Professional Education, Moscow, Russia
- 13Pregna Medicina Reproductiva, Buenos Aires, Argentina
- 14Service de Médecine de la Reproduction et Préservation de la Fertilité, Hôpital Antoine Béclère, Clamart, France
- 15Global Clinical Development, Merck Serono, Italy, an Affiliate of Merck KGaA, Darmstadt, Germany
- 16ANDROFERT, Andrology and Human Reproduction Clinic, Center for Male Reproduction, Campinas, Brazil
- 17Faculty of Health, Aarhus University, Aarhus, Denmark
- 18Faculty of Life Sciences and Medicine, King’s College London, London, United Kingdom
- 19Medical Center for Human Reproduction, Beijing Chao-yang Hospital, Capital Medical University, Beijing, China
- 20Department of Neuroscience, Reproductive Science and Odontostomatology, University of Naples Federico II, Naples, Italy
Background: A Delphi consensus was conducted to evaluate global expert opinions on key aspects of assisted reproductive technology (ART) treatment.
Methods: Ten experts plus the Scientific Coordinator discussed and amended statements plus supporting references proposed by the Scientific Coordinator. The statements were distributed via an online survey to 35 experts, who voted on their level of agreement or disagreement with each statement. Consensus was reached if the proportion of participants agreeing or disagreeing with a statement was >66%.
Results: Eighteen statements were developed. All statements reached consensus and the most relevant are summarised here. (1) Follicular development and stimulation with gonadotropins (n = 9 statements): Recombinant human follicle stimulating hormone (r-hFSH) alone is sufficient for follicular development in normogonadotropic patients aged <35 years. Oocyte number and live birth rate are strongly correlated; there is a positive linear correlation with cumulative live birth rate. Different r-hFSH preparations have identical polypeptide chains but different glycosylation patterns, affecting the biospecific activity of r-hFSH. r-hFSH plus recombinant human LH (r-hFSH:r-hLH) demonstrates improved pregnancy rates and cost efficacy versus human menopausal gonadotropin (hMG) in patients with severe FSH and LH deficiency. (2) Pituitary suppression (n = 2 statements): Gonadotropin releasing hormone (GnRH) antagonists are associated with lower rates of any grade ovarian hyperstimulation syndrome (OHSS) and cycle cancellation versus GnRH agonists. (3) Final oocyte maturation triggering (n=4 statements): Human chorionic gonadotropin (hCG) represents the gold standard in fresh cycles. The efficacy of hCG triggering for frozen transfers in modified natural cycles is controversial compared with LH peak monitoring. Current evidence supports significantly higher pregnancy rates with hCG + GnRH agonist versus hCG alone, but further evidence is needed. GnRH agonist trigger, in GnRH antagonist protocol, is recommended for final oocyte maturation in women at risk of OHSS. (4) Luteal-phase support (n = 3 statements): Vaginal progesterone therapy represents the gold standard for luteal-phase support.
Conclusions: This Delphi consensus provides a real-world clinical perspective on the specific approaches during the key steps of ART treatment from a diverse group of international experts. Additional guidance from clinicians on ART strategies could complement guidelines and policies, and may help to further improve treatment outcomes.
Introduction
Infertility is considered a major health care burden worldwide and is defined as the inability to achieve a viable pregnancy after 1 year of attempting to conceive (1, 2). Infertility is usually investigated after a year, but interventions may be initiated sooner based on age and/or medical, sexual or reproductive history (1, 2). The World Health Organization (WHO) recognises infertility as a disease state and has ranked infertility in women as the fifth highest serious global disability (3). They have also highlighted that infertility treatment comprises an essential part of fertility care (4). Although it is difficult to predict the prevalence of infertility (5), the WHO estimates that as many as 48 million couples and 186 million individuals live with infertility globally (4).
Following continued development, assisted reproductive technology (ART) procedures now have enormous potential as a tool for treating infertility (6). The numerous advances in this field over recent decades have led to the development of increasingly complex diagnostic tools and treatment options (7, 8). It is estimated that over 9 million babies have been born following ART treatments worldwide (9) since the first in vitro fertilisation (IVF) baby was born in 1978 (10). Approximately 2.4 million ART cycles are estimated each year worldwide, with approximately 500,000 babies born annually (9). However, despite considerable improvements in technologies, the ART process remains inefficient, with overall live birth rates per oocyte retrieved reported to be low (5–10%) (11–14). Only ~40–60% of couples visiting infertility centres will achieve the desired goal of a live birth following their treatment (15, 16). Furthermore, ART treatments are associated with the risk of ovarian hyperstimulation syndrome (OHSS) and multiple pregnancy (17), in addition to a potentially higher prevalence of certain birth defects, although at present it is unclear if the latter is related to procedures inherent to ART or to patient factors (18–20)
Success rates in ART are dependent on both medical management (including the related technologies used during infertility treatment) and patient characteristics, highlighting the need for tailored treatment approaches (21, 22). Personalised management strategies have been proposed to optimise efficacy and safety outcomes (21–23). Furthermore, a personalised approach, encompassing shared decision-making between patients and clinicians, could help to alleviate the psychological burden associated with treatment (24). Such strategies may have the added benefit of reducing discontinuation rates (22, 24), as psychological stress is reported to be the most common reason, after funding, for ART treatment discontinuation (25–28).
Clinical guidelines, such as the European Society of Human Reproduction and Embryology (ESHRE) 2019 guideline on ovarian stimulation for IVF/intracytoplasmic sperm injection (ICSI), provide clinicians with valuable evidence-based recommendations to optimise ovarian stimulation in ART (29). However, data from such guidelines are limited by the fact that only a small proportion of patients are included in randomised controlled trials (RCTs), with one study reporting that only ~35% of the general patient population would meet the inclusion criteria used in large-scale clinical trials (30). A Delphi consensus was therefore conducted to gather and evaluate expert opinions from a global perspective on the specific approaches during the key steps of ART treatment, including follicular development and stimulation with gonadotropins, pituitary suppression, final oocyte maturation triggering and luteal-phase support. This was intended to supplement evidence from the ESHRE guidelines and further improve treatment outcomes.
Assessment of Statements According to Delphi Consensus Process
Role of the Sponsor
The Delphi consensus was coordinated by a healthcare consulting and training company (Sanitanova Srl, Milan, Italy). The consensus concept was initiated and funded by Merck KGaA, Darmstadt, Germany. The sponsor was involved early in the process, defining the overarching topic to be discussed, but did not participate in the development of the statements or in any of the meetings or discussions involved in developing the Delphi consensus. The statements were, therefore, developed independently of the industry sponsor. The authors from Merck KGaA, Darmstadt, Germany, were only involved in the development of the manuscript, critically revising it for important intellectual content, especially in the Introduction, Results and Discussion sections, but could not alter the consensus statements in any way.
Consensus Participants
The Delphi consensus initially involved a Scientific Board, comprising the Scientific Coordinator (RO) and 10 additional experts (Table 1). Each member of the Scientific Board suggested an additional 1–4 experts, resulting in a panel of 35 experts (the Extended Panel) (Table 1).
The Consensus Process
The Delphi consensus involved three steps (Figure 1). The Scientific Coordinator generated the initial statements and supporting references, based on an evaluation of the most up-to-date scientific literature. In Step 1, the Scientific Board discussed these statements during two web conferences, and could add, remove or amend the proposed statements and references. The final selection of statements and references to be used in Step 2 were decided by consensus and were approved by the Scientific Coordinator and Scientific Board. The aim of Step 2 was to identify a consensus of opinion from the Extended Panel on the statements developed by the Scientific Board during Step 1. An online survey of the statements was circulated to the Extended Panel. Each participant anonymously rated their level of agreement with each statement using a five-item Likert scale: 1 = absolutely disagree; 2 = disagree; 3 = agree; 4 = more than agree; 5 = absolutely agree. Participants were also asked to provide the main reasons (free text) for their chosen level of agreement or disagreement. Consensus was considered to have been achieved if the proportion of participants either disagreeing with a statement (responding 1 or 2) or agreeing with a statement (responding 3, 4 or 5) exceeded 66% (31, 32). If the proportion of participants either agreeing or disagreeing with a statement did not exceed 66%, that statement would be revised according to the feedback received and another survey initiated that included only the statement(s) not reaching consensus. This process would be repeated, with the statements being revised, until consensus was reached for every statement. For those statements that received ≥25% disagreement during Step 2 (i.e. ≥25% participants voted ‘disagree’ or ‘absolutely disagree’), the reason(s) for this disagreement were explored further. In Step 3, web conferences were arranged to provide feedback to all participants (Scientific Board and Extended Panel) and enable reflection on the statements. Before discussing the results of the online survey, the previous stages of the Delphi consensus were briefly described, and the names of the Scientific Board members and Extended Panel were disclosed. These web conferences were not compulsory to attend and were intended to communicate the outcome of Step 2 to participants, in order to report on the level of consensus for each statement.
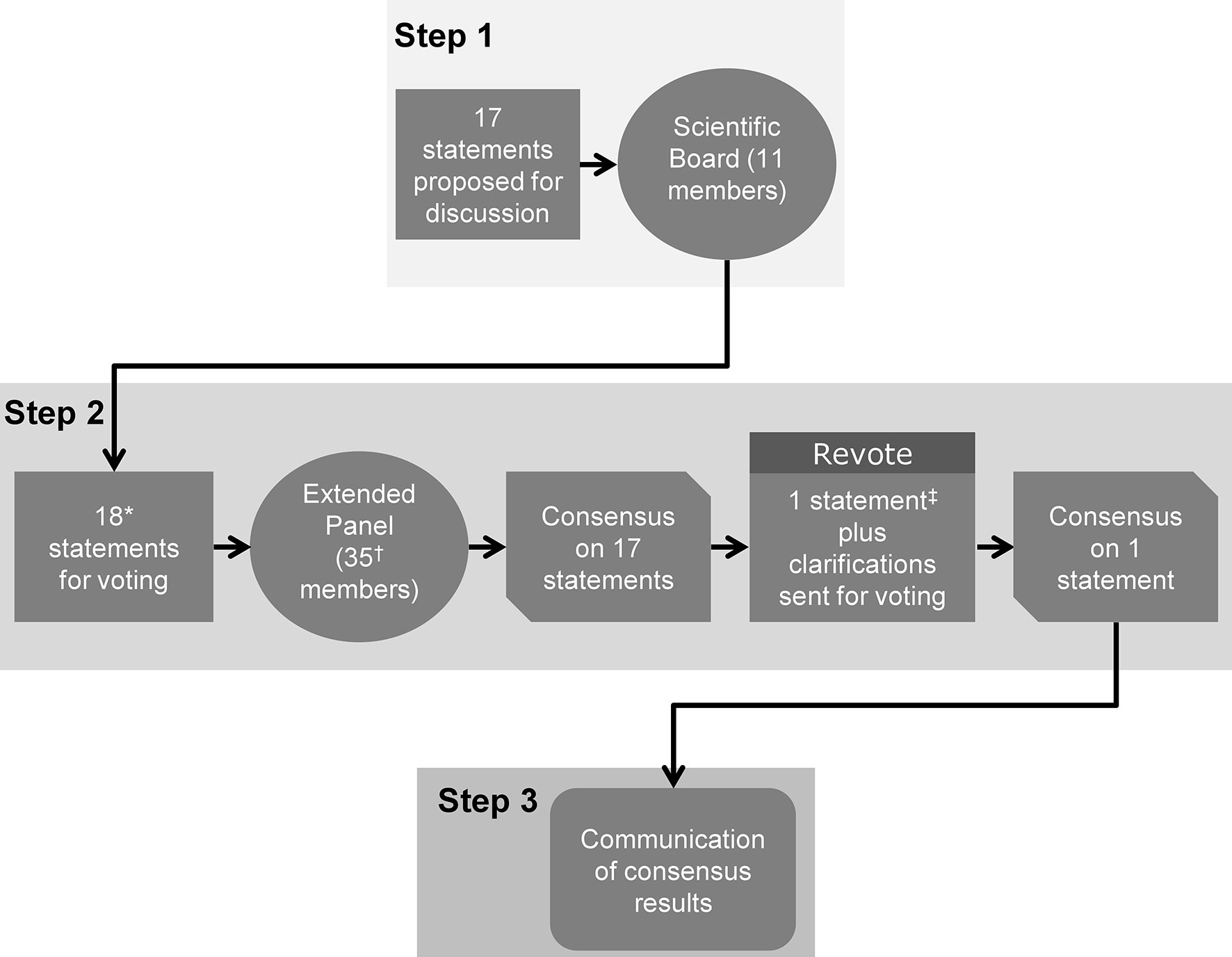
Figure 1 The three steps of the Delphi consensus process. The consensus comprised three steps. Step 1: Statements and supporting references initially developed by the Scientific Coordinator were discussed and amended by the 11 members of the Scientific Board. Step 2: An extended panel of 35 experts voted on their level of agreement or disagreement with each statement. Step 3: The consensus results were communicated to the participating experts. *One new statement (Statement 18) was added during Step 1, following disagreement on the wording of Statement 17; †28/35 (80%) of panel members completed the entire survey; ‡Statement 17 reworded and sent for a second round of voting during Step 2.
Results of the Consensus and Actionable Recommendations (Including Supportive Evidence)
Overall Results
The Scientific Coordinator initially proposed 17 statements for discussion with the Scientific Board. During Step 1, four statements were agreed on without modification, 13 statements were agreed on with modifications, and additional supporting references were added for four statements (five references in total). One additional statement (Statement 18) and three additional references were added following disagreement on the wording of one of the statements (Statement 17). The 18 statements approved by the Scientific Board that were voted on in Step 2 are shown in Table 2; these related to follicular development and stimulation with gonadotropins (n = 9 statements), pituitary suppression (n = 2 statements), final oocyte maturation triggering (n = 4 statements) and luteal-phase support (n = 3 statements). The Extended Panel comprised fertility experts from different world regions, including Europe, US, South America, Asia, Pacific and Middle East.
Overall, 28 of the 35 members of the Extended Panel (80%) completed the entire survey. Consensus was achieved after the first round of voting for all statements except Statement 17, with three statements achieving 100% consensus (Figure 2). A high level of agreement (>80% of votes were ‘agree’, ‘more than agree’ or ‘absolutely agree’) was achieved on >50% of statements. Statement 17, which had a total agreement level of 50% (i.e. only 50% of votes were ‘agree’, ‘more than agree’ or ‘absolutely agree’), was reworded and reached consensus after a second round of voting (67% total agreement). Four additional references were added prior to the revote. Four statements (Statements 8, 11, 17, 18) had a total disagreement level of >25% (i.e. 25% of votes were ‘disagree’ or ‘absolutely disagree’), and for three statements one expert voted ‘absolutely disagree’. The reasons that experts provided when voting to disagree with a given statement are shown in Supplementary Table 1. The 18 statements, grouped according to their main area of focus (follicular development and stimulation with gonadotropins, pituitary suppression, final oocyte maturation triggering and luteal-phase support), together with their associated references are discussed below. Overall, the Extended Panel achieved good consensus on follicular development and stimulation with gonadotropins, pituitary suppression and final oocyte maturation triggering. Luteal-phase support remains a more debated area.
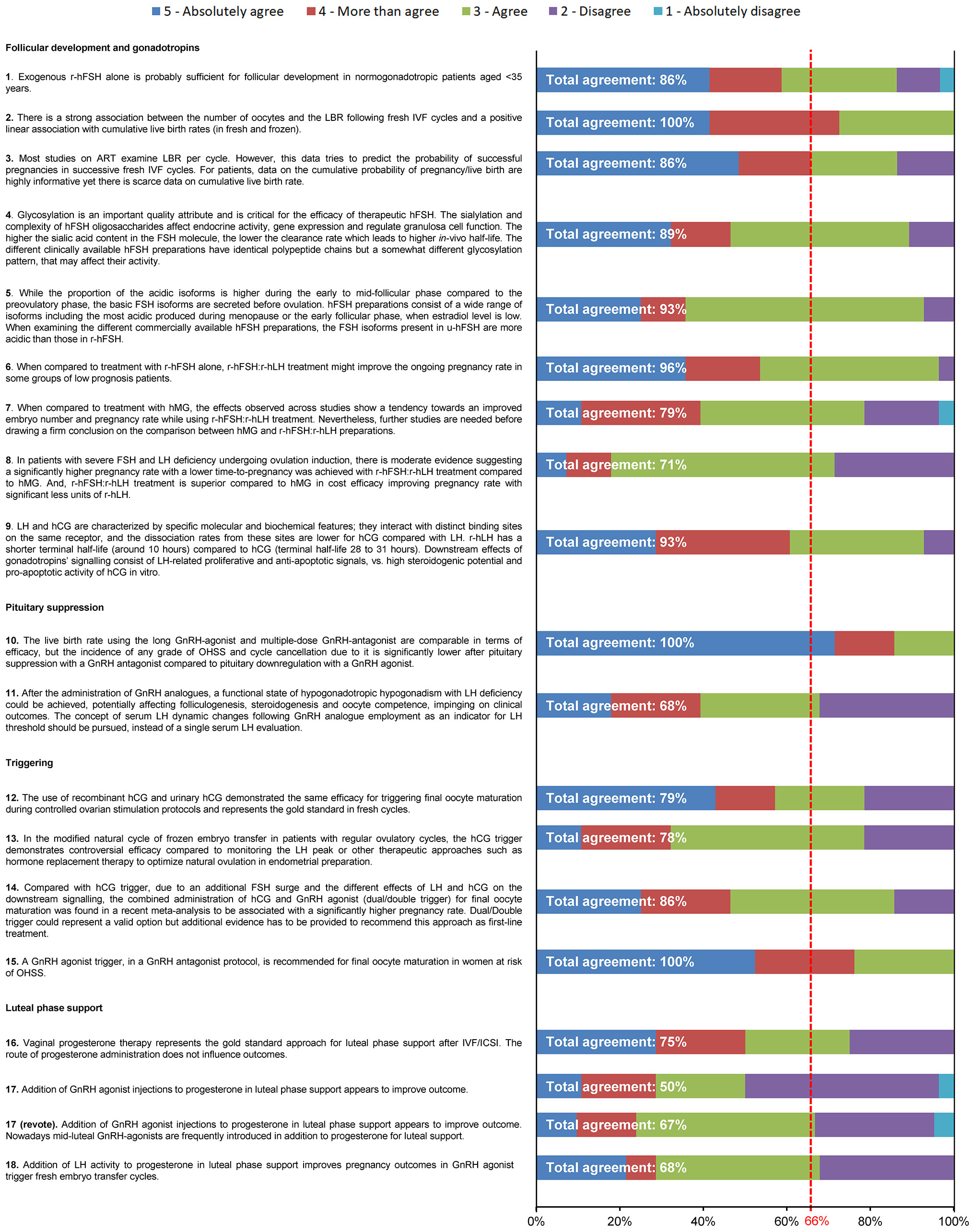
Figure 2 Level of agreement/disagreement with each statement (Step 2). The 35-member Extended Panel voted on their levels of agreement/disagreement with each of the 18 statements using a 5-item Likert scale (1 = absolutely disagree, 2 = disagree, 3 = agree, 4 = more than agree, 5 = absolutely agree). Consensus was considered to have been achieved if the proportion of participants either agreeing with the statement (responding 3, 4 or 5) or disagreeing with the statement (responding 1 or 2) exceeded 66%.
Follicular Development and Stimulation With Gonadotropins
Statement 1: Exogenous Recombinant Human Follicle Stimulating Hormone (r-hFSH) Alone is Probably Sufficient for Follicular Development in Normogonadotropic Patients Aged <35 years
This statement received 86% total agreement from the Extended Panel (Figure 2). A number of studies suggest that, in normogonadotropic women (normal ovarian reserve) aged <35 years undergoing controlled ovarian stimulation (COS) during ART treatment, r-hFSH alone is probably sufficient for optimal ovarian stimulation (33, 34). In a retrospective study of 465 women receiving r-hFSH alone versus r-hFSH in combination with human menopausal gonadotropin (hMG) after administration of a gonadotropin releasing hormone (GnRH) antagonist, the number of oocytes retrieved in the r-hFSH alone group was significantly higher compared with the r-hFSH + hMG group in women aged <35 years (mean [SD] 13.7 [10.2] vs 9.2 [4.2]; p = 0.04) (34). Furthermore, a meta-analysis comprising 70 studies demonstrated that women treated with FSH (r-hFSH or urinary human FSH [u-hFSH]) alone had a higher number of oocytes retrieved than women treated with FSH plus recombinant human luteinizing hormone (r-hLH; FSH:r-hLH) (p = 0.01, 29 studies, 5840 patients) or hMG alone (p <0.001, 20 studies, 5512 patients) (33). In contrast, a systematic review and meta-analysis that included five trials comparing r-hFSH:r-hLH with r-hFSH alone in women with unexpected hypo-response to ovarian stimulation suggested that this patient group might benefit from r-hFSH:r-hLH treatment (35). Clinical pregnancy rates were significantly higher in the r-hFSH:r-hLH group than in the r-hFSH alone group (OR 2.03 [95% CI 1.27 3.25], p = 0.003). Implantation rates (OR 2.62, p = 0.004) and the number of oocytes retrieved (weight mean differences: 1.98 [95% CI 0.17, 3.80], p = 0.03) were also higher with r-hFSH:r-hLH compared with r-hFSH alone (35).
The Conforti study highlights the importance of identifying patients with unexpectedly poor or suboptimal response to gonadotropin stimulation during COS (35), suggesting that assessment of ovarian reserve and ovarian sensitivity may help to determine whether a patient would benefit from treatment with r-hFSH alone versus r-hFSH:r-hLH. Ovarian responsiveness is generally assessed by ovarian markers such as antral follicle count (AFC) and anti-Müllerian (AMH), in conjunction with age, in order to predict poor, normal or hyper-response (29, 36). However, patients with unexpected poor response [POSEIDON Groups 1 and 2 (37, 38)] have normal AFC/AMH levels and it is therefore difficult to predict their response based on standard testing. In 2011, Genro et al. proposed a model to assess unexpected hypo-responsiveness based on the follicle output rate (FORT), calculated as the ratio of the number of pre-ovulatory follicles obtained in response to ovarian stimulation, to the pre-existing pool of small antral follicles (39). Based on this model, Alviggi et al. proposed the concept of follicle-to-oocyte index (FOI), calculated as the ratio of the total number of oocytes collected at the end of ovarian stimulation to the number of antral follicles available at the start of stimulation (40). The FOI is a metric to help identify hypo-responders (for example, POSEIDON groups 1 and 2 patients) and is aligned with the POSEIDON concept, which relies on the number of oocytes retrieved (actual or expected) (38, 40). By contrast, the FORT does not take into consideration the oocyte yield (39). Until genotype profiling of relevant polymorphisms are widely available to identify patients with unexpected poor response to gonadotropin stimulation, models such as FORT and FOI may be helpful to identify this patient group.
Statement 2: There is a Strong Association Between the Number of Oocytes and the LBR (Live Birth Rate) Following Fresh IVF Cycles and a Positive Linear Association with Cumulative Live Birth Rates (in Fresh and Frozen)
This statement received 100% total agreement from the Extended Panel (Figure 2), based on a number of retrospective studies that support a strong association between the number of oocytes and live birth rate (41–44). There are some differences in the conclusions of these studies regarding the optimal number of oocytes retrieved following COS during ART treatment. A large study that analysed data obtained from the Human Fertilization and Embryology Authority (HFEA) from 400,135 fresh IVF cycles reported that live birth rate rose with an increasing number of oocytes up to approximately 15 oocytes, then plateaued at 15–20 oocytes, after which live birth rate steadily decreased (44). Similarly, a study in 2455 women undergoing IVF treatment at a medical centre in China demonstrated that, in fresh cycles, LBR per started cycle increased with the number of retrieved oocytes up to an optimal number of 6 to 15 oocytes (42). A study that included data from 1099 women attending a tertiary medical centre in Belgium (41) reported non-significant differences in live birth rates in fresh cycles, when comparing either high (>15 oocytes) versus normal (10–15 oocytes) responders, or normal (10–15 oocytes) versus suboptimal (4–9 oocytes) responders. However, live birth rates were significantly higher between high, normal and suboptimal responders when compared with the low ovarian responder group (1–3 oocytes). Moreover, patients with high ovarian response demonstrated a significantly higher cumulative live birth rate compared with patients who had poor (p = 0.001), suboptimal (p = 0.001) or normal (p = 0.014) ovarian response.
Furthermore, a large, multicentre study analysing data from 14,469 patients, demonstrated a steady increase in cumulative live birth rates as the number of oocytes increased, reaching 70% when ≥25 oocytes were retrieved (43). This study did not report a plateau in cumulative live birth rate and a further increase of 5% was detected beyond 27 oocytes. A recent systematic review of 16 studies (comprising five studies evaluating live birth rate from fresh cycles, five studies evaluating cumulative live birth rate from stimulated cycles and six studies evaluating both) suggested that 12–18 oocytes was the optimal number of oocytes associated with maximal fresh live birth rate, whereas cumulative live birth rate continued to increase with the number of oocytes retrieved (45).
Statement 3: Most Studies on ART Examine LBR per Cycle. However, This Data Tries to Predict the Probability of Successful Pregnancies in Successive Fresh IVF Cycles. For Patients, Data on the Cumulative Probability of Pregnancy/Live Birth Are Highly Informative yet There is Scarce Data on Cumulative Live Birth Rate
This statement received 86% total agreement from the Extended Panel (Figure 2). Despite considerable advances in ART treatment, less than a third of couples achieve a live birth after one cycle of treatment (15), with the probability of a live birth improving after multiple cycles of treatment, and/or with increased numbers of oocytes retrieved. When an ART cycle fails, couples understandably want to know what their chance of having a live birth is if they continue treatment. Conventionally, studies have focussed on the outcome of fresh ART cycles; however, cumulative live birth rate, following the transfer of all fresh and frozen–thawed/warmed embryos, is increasingly recognised as a suitable way of reporting the success of an ART programme (46–50). Cumulative live birth rate is considered the most meaningful outcome from the patients’ perspective, as it gives the couple a more accurate prognosis of achieving a live birth after ART (46–50)
The International Committee for Monitoring Assisted Reproductive Technologies (ICMART) defines cumulative live birth rate as the cumulative live birth delivery rate per one initiated/aspirated cycle, including all fresh and/or frozen transfers (1); however, definitions often vary between studies (50), making it difficult to compare data. For example, the numerator for cumulative live birth may be calculated as the number of women with at least one live birth or all live birth episodes from an index cycle; and the denominator could be all women who attempt ovarian stimulation as part of ART or all women who have undergone oocyte collection (50). In addition, the number of treatment cycles varies between studies and there is no consensus on how many treatment cycles cumulative data should be based on (50).
Despite an increasing number of studies now reporting cumulative live birth rate (41, 46, 47, 49, 51), available data on this outcome are still limited (52). In 1999, Engmann et al. assessed cumulative live birth rate in 232 women who had committed to a three-cycle IVF treatment package within 1 year (46). The cumulative live birth rate was 33.2% after two cycles of treatment compared with 48.2% after three cycles of treatment. In another study, comprising 974 women undergoing 1985 stimulated cycles, the overall cumulative live birth rate after three completed cycles (including freezing/thawing cycles) was 63.1%, 55.5%, and 65.5% in ‘realistic’, ‘conservative’ and ‘optimistic’ drop-out terms, respectively (47). Furthermore, 65% of couples who did not achieve a live birth had interrupted the full three-cycle treatment programme (47).
As expected, cumulative live birth rate has been shown to decline with advancing maternal age (46, 47, 49, 53). A Danish study in 1338 women reported 5-year cumulative live birth rates of 74.9% in women aged <35 years compared with 52.2% in women aged ≥35 years (p = 0.001), with female age <35 years found to be a positive prognostic factor for delivery (OR 0.49 [95% CI 0.32, 0.75], p = 0.001]) (54). A retrospective cohort study in 27,906 ART cycles reported conservative cumulative live birth rates after three cycles of 59%, 51%, 40% and 19% for women aged <35 years, 35–<38 years, 38–<40 years and ≥40 years (p <0.001), respectively, and optimistic cumulative live birth rates of 67%, 58%, 47% and 24%, respectively (48, 55). In another retrospective cohort study in 146 patients aged 41–44, cumulative live birth rate increased up to the thirteenth cycle, reaching a cumulative live birth rate of 33.6%; with older patient age significantly associated with a lower probability of a live birth (RR for 43 vs 41 years: 0.47; [95% CI 0.25, 0.87]; p = 0.01) (56). These studies highlight that, while ART can help overcome subfertility in younger patients, the effect of age on fertility poses a significant challenge to treatment, especially in women aged >40 years (49).
ESHRE selected cumulative live birth rate as one of two critical efficacy outcomes (the other being live birth rate) for their 2019 guideline on ovarian stimulation for IVF/ICSI (29).
Statement 4: Glycosylation is an Important Quality Attribute and is Critical for the Efficacy of Therapeutic Human Follicle-Stimulating Hormone (hFSH). The Sialylation and Complexity of hFSH Oligosaccharides Affect Endocrine Activity, Gene Expression and Regulate Granulosa Cell Function. The Higher the Sialic Acid Content in the FSH Molecule, the Lower the Clearance Rate Which Leads to Higher In Vivo Half-Life. The Different Clinically Available hFSH Preparations Have Identical Polypeptide Chains but a Somewhat Different Glycosylation Pattern, That May Affect Their Activity
This statement received 89% total agreement from the Extended Panel (Figure 2). FSH is a heterodimeric glycoprotein, comprised of an α-subunit non-covalently linked to a β-subunit (57). Each subunit contains two N-glycosylation sites carrying sialylated complex type N-glycans (58). The oligosaccharide composition and glycosylation patterns vary considerably, resulting in multiple isoforms of FSH [Supplementary Figure 1 (59)]. Each isoform is characterised by an Isoelectric point (pI) that represents the pH at which net charge of the isoform becomes zero. Glycosylation is driven by three different levels of molecular complexity: i) glycan site occupancy; ii) glycan antennarity, which reflects the branching number of oligosaccharides; iii) degree of terminal sialylation of each carbohydrate moiety. The combination of all these parameters determine the molecular weight and the net charge of each FSH isoform (60).
Glycosylation has been shown to play an important role in protein folding, secretion and metabolism of FSH, and in the regulation of its half-life, activity at the target cell level and signal transduction (61). Glycosylation of FSH affects the plasma half-life in animal models and the activity of the hormone on target organs (62). When FSH isoforms are tested individually according to their pI in an in vitro cell model, less sialylated (less acidic) isoforms exhibit higher receptor binding and higher in vitro biological receptor activation than their more sialylated (more acidic) counterparts (63). In an in vivo rat model, less sialylated isoforms have a shorter plasma half-life and exhibit a lower biological activity than their more sialylated counterparts (63–65). Biological activity of an FSH source is defined as the ability of an exogenous FSH to increase ovarian weight in immature female rats resulting in ovarian hypertrophy, also known as the Steelman and Pohley assay (66). Potency of FSH is expressed in international units (IU) of biological activity assessed by the Steelman and Pohley assay, calculated versus an international standard. The FSH potency is used to determine the clinical treatment dose; therefore, the biological activity of an FSH protein is related to the Steelman and Pohly bioassay. However, it is important to note that the FSH product potency, based on the IU of biological activity needed to obtain ovarian weight gain in an in vivo rat model (Steelman-Pohley assay), does not predict the clinical effect of FSH in humans. Indeed, the same dose (in IU) of two different FSH products does not necessarily translate into the same clinical efficacy and safety outcomes (67). Clinical studies are necessary to assess clinical efficacy and safety (68).
Differences in glycosylation patterns have also been observed between r-hFSH originator and biosimilar preparations. The follitropin alfa (herein referred to as r-hFSH-alfa throughout) biosimilar, Bemfola®, has been shown to be more glycosylated (bulkier glycan structures and greater sialylation) than the originator r-hFSH-alfa reference preparation, GONAL-f® (69). The r-hFSH-alfa biosimilar, Ovaleap®, is also more glycosylated (higher amount of the sialic acid N-glycolyl neuraminic acid) than originator r-hFSH-alfa (70). RCTs comparing originator r-hFSH-alfa with Bemfola® (71) and Ovaleap® (72) have shown non-inferiority of biosimilars versus originator r-hFSH-alfa in terms of the number of oocytes retrieved and, although the studies were not powered for other secondary outcomes, data have shown significant differences in favour of originator r-hFSH-alfa with respect to live birth, ongoing pregnancy (73) and clinical pregnancy rates (73, 74), as well as reductions in the incidence of OHSS (64). The reported differences in clinical outcomes between these biosimilar products could potentially be related to differences in glycosylation patterns, although further studies would be needed to confirm this.
Statement 5: While the Proportion of Acidic FSH Isoforms is Higher During the Early to Mid-Follicular Phase Compared to the Preovulatory Phase, the Basic FSH Isoforms Are Secreted Before ovulation. hFSH Preparations Consist of a Wide Range of Isoforms Including the Most Acidic Produced During Menopause or the Early Follicular Phase, When Estradiol Level is Low. When Examining the Different Commercially Available hFSH Preparations, the FSH Isoforms present in u-hFSH Are More Acidic Than Those in r-hFSH
This statement received 93% total agreement from the Extended Panel (Figure 2). FSH exists as a family of isohormones that differ in their oligosaccharide structures, including the degree of terminal sialylation (75). FSH heterogeneity is well established, and FSH isoforms have distinct properties (76, 77). The extent and pattern of glycosylation appears to be under endocrine control, and is predominantly influenced by GnRH (75–77). Glycosylation composition confers a distinct electric charge on each FSH isoform. Human pituitary FSH presents an isoelectric pattern ranging from pI of 3.0 to 7.0 (77). Accordingly, dynamic changes in FSH isoform abundance occur during different menstrual phases (78). The acidic FSH isoforms are less abundant during the pre-ovulatory and ovulatory phase (79) and more abundant during the early to mid-follicular phase (80), whereas basic FSH isoforms are more abundant before ovulation (77). In addition, acidic FSH isoforms are most abundant in the menopausal period when serum estradiol levels are low (78).
Analyses of the different commercially available FSH preparations have revealed differences between u-hFSH and r-hFSH (76, 81). For example, u-hFSH highly purified (HP) has an isoform distribution showing a slightly higher abundance of more acidic FSH isoforms than r-hFSH-alfa originator (76, 81), although both distributions are within the physiological spectrum observed for pituitary hFSH [pI 3.0–5.2 for u-hFSH HP and pI 3.5–5.8 for r-hFSH-alfa originator (81)]. The clinical impact of the differences between r-hFSH and u-hFSH has been highlighted in an RCT conducted by Frydman et al., 2000 (82) and in a meta-analysis conducted by Van Wely et al., which analysed data from 42 RCTs comparing COS outcomes obtained with different gonadotropins (83). Patients treated with u-hFSH had a significantly lower number of oocytes retrieved compared with those treated with r-hFSH-alfa (u-hFSH 8.8 ± 4.8 vs r-hFSH-alfa 11.0 ± 5.9; p <0.05) (82). Compared with u-hFSH, r-hFSH was associated with similar pregnancy rates and live birth rates (1.03 [95% CI 0.94, 1.13] and 1.03 [95% CI 0.90, 1.18], respectively), but data on cumulative live birth rate are lacking (83). In one study, as an attempt to mimic the physiological shift from an acidic to a more basic FSH isoform during oocyte maturation, Gurgan et al. showed improved efficacy with sequential simulation of u-hFSH followed by r-hFSH, compared with r-hFSH alone or u-hFSH alone (84).
Statement 6: When Compared to Treatment With r-hFSH Alone, r-hFSH:r-hLH Treatment Might Improve the Ongoing Pregnancy Rate in Some Groups of Low Prognosis Patients
This statement received 96% total agreement from the Extended Panel (Figure 2). During ART, one of the various regimens that COS can be performed with is ovarian stimulation with r-hFSH in combination with a GnRH analogue to prevent premature LH surges (85). Since GnRH analogues deprive the growing follicles of LH (86), a number of studies have investigated whether live birth rates could be improved through supplementation with r-hLH. Two studies in unselected populations of women undergoing COS have demonstrated improved efficacy with r-hFSH:r-hLH treatment compared with r-hFSH alone (33, 87). A Cochrane systematic review by Mochtar et al. reported higher ongoing pregnancy rates with r-hFSH:r-hLH compared with r-hFSH alone (OR 1.20 [95% CI 1.01, 1.42]; n = 3129 women; 19 studies; I2 = 2%, moderate-quality evidence) (87). Furthermore, in a meta-analysis of 29 studies involving 5565 women by Santi et al, FSH:r-hLH demonstrated significantly higher pregnancy rates compared with FSH alone (OR 1.20 [95% CI 1.06, 1.37]; p = 0.004) (33).
A number of studies in women with poor ovarian response suggest that r-hFSH:r-hLH treatment may be particularly beneficial in this patient group. A systematic review and meta-analysis of five studies comparing r-hFSH:r-hLH with r-hFSH alone in women with unexpected poor response to ovarian stimulation (hypo-responders) demonstrated significantly higher clinical pregnancy rates (OR 2.03; p = 0.003), implantation rates (OR 2.62; p = 0.004) and number of oocytes retrieved (weight mean differences: 1.98, p = 0.03) with r-hFSH:r-hLH compared with r-hFSH alone (35). In a systematic review of 30 RCTs in normogonadotropic women undergoing COS in ART, Alviggi et al. examined different patient subgroups and reported that the two subgroups benefiting from r-hFSH:r-hLH treatment were: (1) women with unexpected poor ovarian response and (2) women aged 36–39 years (88). There was no evidence of a benefit in patients with normal ovarian response aged <35 years. Lehert et al. also examined the efficacy of r-hFSH:r-hLH treatment in different patient populations, in a systematic review and meta-analysis comprising 40 RCTs (6443 women) (89). r-hFSH:r-hLH was associated with significantly higher clinical pregnancy rates compared with r-hFSH alone in the overall population (RR 1.09 [95% CI 1.01, 1.18]), and this difference was more pronounced in women with poor ovarian response (n = 1179; RR 1.30 [95% CI 1.01, 1.67]). Significantly more oocytes were retrieved with r-hFSH:r-hLH compared with r-hFSH alone in women with poor ovarian response (n = 1077; weighted mean difference 0.75 [95% CI 0.14, 1.36]), whereas no difference was observed between treatment groups in the overall population. Furthermore, a retrospective study from a registry of 12 French ART centres that analysed data from 9787 women undergoing COS (5218 r-hFSH:r-hLH, 4569 r-hFSH-alfa alone), reported significantly higher cumulative live birth rates with r-hFSH:r-hLH compared with r-hFSH-alfa alone in moderately and severely poor ovarian responders (moderate: OR 1.37, [1.07, 1.35], RR 1.30, p=0.013; severe: OR 2.40 [1.48,3.89], RR 1.88, p<0.001), with no difference between either treatment in mildly poor ovarian responders (OR 0.95 [95% CI 0.78, 1.15], RR 0.95, p=0.60) (90).
Statement 7: When Compared to Treatment With hMG, The Effects Observed Across Studies Show a Tendency Towards an Improved Embryo Number and Pregnancy Rate While Using r-hFSH:r-hLH Treatment. Nevertheless, Further Studies Are Needed Before Drawing a Firm Conclusion on the Comparison Between hMG and r-hFSH:r-hLH Preparations
This statement received 79% total agreement from the Extended Panel (Figure 2). A number of studies have demonstrated improved pregnancy rates (33, 91, 92) and implantation rates (91) in women treated with r-hFSH:r-hLH compared with hMG. Furthermore, in a cost-utility analysis, r-hFSH +r-hLH showed higher cost-effectiveness than HP-hMG in women undergoing COS in IVF (93). However, an RCT in 122 patients found that pregnancy rates, implantation rates and embryo quality were comparable between r-hFSH:r-hLH and HP-hMG (94). Recently, a critical appraisal of studies comparing r-hFSH-alfa/-beta + r-hLH and hMG was performed (95). Of the 11 studies included, most were observational and only two were RCTs. When all prospective and retrospective studies were analysed together, no statistically significant between-group differences were observed in patients’ age, total and daily dose of gonadotrophin used, stimulation variables, and clinical pregnancy and live birth rates, although values seemed to differ for the mean [SD] number of oocytes retrieved in the r-hFSH-alfa/beta:r-hLH group versus the hMG group (10.12 [4.44] versus 8.74 [4.1], respectively; p = 0.06).
The higher total disagreement level for this statement (21%) probably reflects the differences in outcomes reported between these studies.
The ESHRE 2019 guideline on ovarian stimulation for IVF/ICSI states that the use of r-hLH + r-hFSH is probably not recommended over hMG in GnRH agonist protocols due to similar pregnancy outcomes and an apparent higher risk of OHSS with r-hLH + r-hFSH, although this recommendation does not apply to GnRH antagonist cycles (29). This recommendation was labelled by ESHRE as conditional (‘probably recommend’), defined as a recommendation for which clinicians should recognise that different choices will be appropriate for individual patients, as opposed to recommendations labeled as ‘strong’ (‘recommend’), for which ESHRE suggest most individuals should receive the intervention. Furthermore, the RCT supporting this recommendation (94) was graded as ‘very low quality’ owing to its small sample size and lack of transparency, suggesting that further studies may be required.
Statement 8: In Patients With Severe FSH and LH Deficiency Undergoing Ovulation Induction, There is Moderate Evidence Suggesting a Significantly Higher Pregnancy Rate with a Lower time-to-Pregnancy was Achieved with r-hFSH:r-hLH Treatment Compared to hMG. And, r-hFSH:r-hLH Treatment is Superior Compared to hMG in Cost Efficacy Improving Pregnancy Rate with Significant Less Units of r-hLH
This statement received 71% total agreement from the Extended Panel (Figure 2). WHO type I hypogonadotropic anovulation (congenital hypogonadotropic hypogonadism) is a rare condition characterised by severe FSH and LH deficiency and negligible oestrogen activity (96). Clinically, the condition manifests as absent or incomplete puberty, leading to anovulation in women and infertility. Carone et al. compared the efficacy of r-hFSH:r-hLH versus HP-hMG, in a randomised open-label study in 35 women with congenital hypogonadotropic hypogonadism aged 25–36 years (97). The proportion of women achieving ovulation induction was 70% with r-hFSH:r-hLH versus 88% with HP-hMG (p=0.11). However, pregnancy rates were significantly higher in patients receiving r-hFSH:r-hLH compared with HP-hMG (55.6% vs 23.3; p=0.01). r-hFSH:r-hLH treatment appears to be superior in terms of cost effectiveness compared to hMG. In a cost-utility analysis in 848 women undergoing IVF, r-hFSH:r-hLH showed higher cost-effectiveness compared with HP-hMG, with a slightly lower overall cost per pregnancy despite a higher cost per medication (93). In addition to congenital hypogonadotropic hypogonadism, severe FSH and LH deficiency may occur in normogonadotropic women undergoing ART treatment, particularly those receiving pituitary down-regulation with a GnRH analog (see Statement 7) or in women aged >35 years (98).
This statement received 29% disagreement from the extended panel. The motivations supporting these disagreements are outlined in Supplementary Table 1. In summary, some members of the extended panel believed that the current evidence was of a low quality and that further studies were needed to support this statement. One participant explained that they believed that the hLH dose was more important than the type of hLH (recombinant or urinary), and that use of r-hLH enabled the hLH dose to be controlled independently of FSH.
Statement 9: LH and Human Chorionic Gonadotropin (hCG) are Characterised by Specific Molecular and Biochemical Features; They Interact with Distinct Binding Sites on the same Receptor, and the Dissociation Rates From These Sites are Lower for hCG Compared with LH. r-hLH has a Shorter Terminal Half-Life (around 10 hours) Compared to hCG (terminal half-life 28 to 31 hours). Downstream Effects of Gonadotropins’ Signalling Consist of LH-Related Proliferative and Anti-Apoptotic Signals, vs. High Steroidogenic Potential and Pro-Apoptotic Activity of hCG In Vitro
This statement received 93% total agreement from the Extended Panel (Figure 2). LH and hCG are heterodimeric glycoproteins comprised of a common α-subunit and a distinct β-subunit (99). Both hormones bind to the LH chorionic gonadotropin receptor (LHCGR) (100), activating the cAMP/protein kinase A (PKA) steroidogenic pathway. Although previously considered “equivalent”, each hormone is characterised by specific molecular and biochemical features (99). r-hLH has a shorter terminal elimination half-life compared with hCG (10–12 hours vs with en rule: 28–31 hours, respectively) (101–103). Furthermore, LH and hCG hormones each activate different signalling pathways resulting in distinct physiological responses (99). LH activates mainly the extracellular signal-regulated kinase 1/2 (ERK1/2)-dependent and protein kinase B (Akt)-dependent pathways, whereas hCG activates mainly the steroidogenic and pro-apoptotic pathways via protein kinase A and cAMP response element-binding protein (CREB) (104, 105).
In vitro binding studies examining LH and hCG suggested that a hinge region encoded by exon 10 of the LHCGR was able to distinguish between LH and hCG (106). Although both ligands could bind normally to a mutated version of the receptor lacking exon 10, cAMP production was significantly impaired when stimulated by LH whereas hCG signalling was unaffected (106). In contrast, experiments altering the structure of exon 10 of the LHCGR by introduction of a double-proline mutation were shown to negatively affect hCG signalling, but had no impact on LH signalling (107). These experiments support the existence of distinct LH- and hCG-specific signalling pathways, which are predominantly determined in the L2-beta loop of the hormones and in the hinge region of the receptor (107). Accordingly, gene expression profiles obtained from women undergoing COS with r-hLH + r-hFSH or with HP-hMG (containing u-hCG as LH-like activity) indicate that these treatments activate different intracellular pathways involved in oocyte development and maturation compared with r-hFSH alone, with gene clusters showing up- or downregulation depending on the stimulation protocols compared with expression levels after treatment with r-hFSH alone (108).
It has been reported that co-treatment with FSH potentiates different LH- and hCG-dependent responses in human granulosa-luteinised cells, corresponding to their different physiological functions (109). hCG biopotency, evaluated by cAMP amplification, was shown to be five-fold higher in the presence of FSH compared with hCG alone, resulting in increased CREB phosphorylation and steroid production, whereas the effects of LH on steroidogenic cAMP/PKA pathway activation were unchanged in the presence of FSH compared with LH alone (109). In contrast, prolonged ovine LH treatment was shown to promote growth and proliferation in goat ovarian granulosa cells, whereas hCG treatment resulted in higher cAMP levels and decreased proliferation (110).
Pituitary Suppression
Statement 10: The Live Birth Rate Using the Long GnRH-Agonist and Multiple-Dose GnRH-Antagonist are Comparable in terms of Efficacy, but the Incidence of any Grade of OHSS and Cycle Cancellation Due to it is Significantly Lower After Pituitary Suppression with a GnRH Antagonist Compared to Pituitary Downregulation with a GnRH Agonist
This statement received 100% agreement from the extended panel (Figure 2). GnRH analogs are administered along with a gonadotropin during COS to prevent premature LH surges (111). GnRH agonists have been used for this purpose since the early 1980s and act to suppress gonadotropin release by pituitary desensitization following an initial short period of gonadotropin hypersecretion (112). Over the last decade or so there has been a steady rise in the use of GnRH antagonists, with a number of studies supporting their use over agonists (113–116). GnRH antagonists competitively bind to the GnRH receptor and rapidly inhibit gonadotrophin release. Their suppression effects are faster than that of GnRH agonists (112, 117) and are fully reversible (118). As a result, GnRH antagonists are associated with a shorter treatment duration and lower OHSS rates than GnRH agonists (113–115).
There have previously been conflicting results regarding pregnancy outcomes with GnRH analogue use (119), with several studies reporting improved pregnancy rates with GnRH agonists versus antagonists (114, 120, 121). However, these studies were conducted at a time when GnRH agonist use was considerably higher than GnRH antagonist use (119), resulting in a lack of clinical experience with antagonist protocols (122, 123). In contrast, a number of more recent studies have reported no significant differences in live birth rates and ongoing pregnancy rates between GnRH agonists and antagonists (113, 115). In a Cochrane review of 73 RCTs in 12,212 women comparing GnRH antagonist to long-course GnRH agonist protocols, Al-Inany et al. reported that live birth rates were similar between GnRH antagonists and GnRH agonists (OR 1.02 [95% CI 0.85, 1.23], 12 RCTs, n = 2303, I2 = 27%, moderate quality evidence) (115). However, GnRH antagonist treatment was associated with a lower incidence of any grade OHSS (OR 0.61 [95% CI 0.51, 0.72], 36 RCTs, n = 7944, I2 = 31%, moderate quality evidence) and a lower cycle cancellation rate due to a high OHSS risk (OR 0.47 [95% CI 0.32, 0.69], 19 RCTs, n=4256, I2 = 0%) compared with agonists (115). In a Cochrane review by Lambalk et al., GnRH antagonist and agonist protocols were compared in different patient populations, including general IVF patients (34 studies), patients with PCOS (10 studies) and patients with poor ovarian response (6 studies) (114). Ongoing pregnancy rates were significantly lower in women receiving GnRH antagonists compared with GnRH agonists in the general IVF population (RR 0.89 [95% CI 0.82, 0.96]), whereas ongoing pregnancy rates were similar between treatment groups in women with PCOS (RR 0.97 [95% CI 0.84, 1.11]) or poor ovarian response (RR 0.87 [95% CI 0.65, 1.17]). Lambalk et al. also reported significantly lower OHSS rates with antagonist treatment in general IVF patients (RR 0.63 [95% CI 0.50, 0.81]) and in women with PCOS (RR 0.53 [95% CI 0.30, 0.95]), with no data on OHSS available in poor responders (114). However, there were several issues with the internal validity of this meta-analysis that should be considered when interpreting these findings, such as the inclusion of studies with asymmetric co-interventions and the misclassification of eligible studies (124). Furthermore, this meta-analysis compared the long-agonist protocol with the combination of every potential antagonist protocol.
The ESHRE 2019 guideline on ovarian stimulation for IVF/ICSI recommends a GnRH antagonist protocol for women with predicted normal or high ovarian response and for women with PCOS, and equally recommends GnRH antagonists and GnRH agonists for women with predicted poor response (29).
Statement 11: After the Administration of GnRH Analogues, a Functional State of Hypogonadotropic Hypogonadism with LH Deficiency Could be Achieved, Potentially Affecting Folliculogenesis, Steroidogenesis and Oocyte Competence, Impinging On Clinical Outcomes. The Concept of Serum LH Dynamic Changes Following GnRH Analogue Employment as an Indicator for LH Threshold Should be Pursued, Instead of a Single Serum LH Evaluation
This statement received 68% total agreement from the Extended Panel (Figure 2). LH is essential for normal follicular development and oocyte maturation (125), and several studies have highlighted the importance of maintaining adequate LH levels during COS to optimise clinical outcomes (126–129). The use of GnRH analogues during COS may restrict the amount of LH available to the developing follicles, which could be detrimental to the development of normal healthy follicles (98). LH suppression patterns differ between GnRH agonists and GnRH antagonists. GnRH agonists induce pituitary desensitization, resulting in a profound reduction in LH levels (130, 131), whereas GnRH antagonists are generally initiated later, resulting in higher LH levels early on during COS, after which LH levels rapidly decline, often followed by a gradual rise later in the cycle (132–134).
A non-randomised proof of concept study in 50 women receiving a standard (0.25 mg) dose of GnRH antagonist demonstrated that 26% of patients hyper-responded to GnRH antagonist treatment, whereby their LH level was <50% of the pre-treatment level (135, 136). The concept of an “LH clinical treatment window,” has been proposed, below which oestrogen production is inadequate and above which LH levels may be detrimental to follicular development (137). Accordingly, a dose finding study designed to investigate the effect on pregnancy outcomes of different serum LH levels induced by a range of GnRH antagonist doses, reported that excessive or insufficient suppression of LH levels, irrespective of the GnRH dose, resulted in a reduction in clinical pregnancy rates (127). This study indicates that follicular development is dependent on the direction and rate of change in LH levels rather than the actual LH level at a particular time point.
Owing to the importance of LH on follicular development and clinical outcomes, there has been continued debate in the literature regarding the use of r-hFSH:r-hLH treatment during COS (128, 129, 138, 139). Although r-hFSH:r-hLH treatment in unselected patients does not appear to be beneficial, several studies support its use in certain patient populations, including older patients, poor responders and hypo-responders (35, 40, 129, 138). The benefits of r-hFSH:r-hLH treatment also appear to depend on the type of GnRH analogue used (129, 138, 139), consistent with the distinct LH suppression patterns observed between GnRH agonist and antagonist cycles. Collectively, these studies support an individualised approach to identify subgroups of women who may benefit from r-hFSH:r-hLH treatment.
This statement received 32% disagreement from the extended panel. The motivations supporting these disagreements are outlined in Supplementary Table 1. Some participants felt that there was insufficient evidence to support this statement, while others questioned whether GnRH analogue use would result in a functional state of hypogonadotropic hypogonadism. Furthermore, some participants did not believe that measuring serum LH dynamic changes would be beneficial compared with a single serum LH measurement and were uncertain whether there was adequate technology to measure dynamic changes.
Final Oocyte Maturation Triggering
Statement 12: The Use of Recombinant hCG and Urinary hCG Demonstrated the same Efficacy for Triggering Final Oocyte Maturation During Controlled Ovarian Stimulation Protocols and Represents the Gold Standard in Fresh Cycles
This statement received 79% total agreement from the Extended Panel (Figure 2). hCG has been used since the mid-1970s to trigger luteinisation of the granulosa cells and final oocyte maturation, and remains the most common treatment (140). However, more recently several studies suggest that, in GnRH antagonist cycles, triggering final oocyte maturation with a bolus of GnRH agonist may also be beneficial (141). Following pituitary suppression with a GnRH antagonist, the GnRH agonist displaces the GnRH antagonist from the receptor, inducing a release of FSH and LH (142), similar to that seen in the natural cycle, although for a shorter duration (24–36 hours with a GnRH agonist compared with 48 hours in the natural cycle) (140). The shorter duration of LH surge with a GnRH agonist trigger may help to reduce the risk of OHSS (143). A Cochrane review by Youssef et al. compared GnRH agonists with hCG for triggering final oocyte maturation in women undergoing COS during ART treatment using a GnRH antagonist protocol, utilising data from 17 RCTs (n = 1847) (144). Use of GnRH agonists for ovulation triggering was associated with a lower live birth rate compared with use of hCG for ovulation triggering (OR 0.47 [95% CI 0.31, 0.70], 5 RCTs, 532 women, I2 = 56%, moderate-quality evidence). GnRH agonist ovulation triggering was also associated with a lower incidence of mild, moderate or severe OHSS compared with hCG ovulation triggering (OR 0.15 [95% CI 0.05, 0.47], 8 RCTs, 989 women, IO=42%, moderate-quality evidence). A second Cochrane review by the same author assessed the effects of subcutaneous rhCG and high dose rLH versus uhCG for inducing final oocyte maturation, using data from 18 studies involving 2952 women undergoing ART treatment (145). There was no evidence of a difference between rhCG and uhCG in terms of live birth/ongoing pregnancy rates (OR 1.15 [95% CI 0.89, 1.49] 7 RCTs, N = 1136, I2 = 0%, moderate quality evidence) or mild, moderate or severe OHSS (moderate to severe OHSS [OR 1.76 (95% CI 0.37, 8.45); 3 RCTs, N = 417, I2 = 0%, low quality evidence], moderate OHSS [OR 0.78 (95% CI 0.27, 2.27); 1 RCT, N = 243, I2 = 0%, low quality evidence], mild to moderate OHSS [OR 1.00 (95% CI 0.42, 2.38); 2 RCTs, N = 320, I2 = 0%, low quality evidence]) (145).
The ESHRE 2019 guideline on ovarian stimulation for IVF/ICSI equally recommends the use of recombinant hCG and urinary hCG for triggering final oocyte maturation (29).
Statement 13: In the Modified Natural Cycle of Frozen Embryo Transfer in Patients with Regular Ovulatory Cycles, the hCG Trigger Demonstrates Controversial Efficacy Compared to Monitoring the LH Peak or Other Therapeutic Approaches such as Hormone Replacement Therapy to Optimise Natural Ovulation in Endometrial Preparation
This statement received 78% total agreement from the Extended Panel (Figure 2). During frozen embryo transfer, some or all embryos may be frozen, to be thawed and transferred at a later stage. The most common regimens for frozen embryo transfer are natural cycle with or without an hCG trigger, or endometrial preparation with hormone therapy with or without a GnRH agonist. However, there is conflicting evidence regarding the benefit of hCG triggering in frozen embryo transfer during natural cycle compared with other protocols (146). A Cochrane Database systematic review of 18 RCTs in 3815 women did not find sufficient evidence of a difference between an HCG trigger in a modified natural cycle versus hormone therapy with or without GnRH agonist suppression, with similar live birth rates between the groups (hCG vs hormone therapy: OR 1.34 [95% CI 0.88, 2.05], 1 RCT, n = 959, low-quality evidence; hCG vs hormone therapy + GnRH agonist: OR 1.11 [95% CI 0.66, 1.87], 1 RCT, n = 236, low-quality evidence) (147). A systematic review and meta-analysis of 33 studies also reported no statistically significant difference in clinical pregnancy or live birth between natural cycles without hCG triggering (based on spontaneous serum LH peak monitoring) versus modified natural cycles with hCG triggering (148). In addition, when an hCG trigger in a natural cycle (144 embryos transferred in 108 cycles) was compared with hormone therapy (357 embryos transferred in 224 cycles) at a single IVF centre, in women undergoing frozen embryo transfer, no significant differences were reported between the groups in implantation rate, clinical pregnancy rate or live birth rate (149).
Some studies have demonstrated improved pregnancy outcomes with hCG compared with other protocols (150). In a single centre study that collected data from all women undergoing frozen embryo transfer over a 3-year period, implantation, clinical pregnancy and ongoing pregnancy rates were significantly higher in patients undergoing natural cycle with modified luteal phase support consisting of hCG (31%, 51%, and 46%, respectively), compared with natural cycle without hCG (17%, 26%, and 20%, respectively), or hormone therapy (15%, 22% and 17%, respectively) (150). Furthermore, in patients undergoing natural cycle frozen embryo transfer, hCG triggering has been shown to significantly reduce the number of visits required for cycle monitoring without affecting clinical outcomes, potentially providing a more convenient option for patients as well as improving cycle cost effectiveness (151).
hCG triggering has also been associated with potential negative effects on pregnancy outcomes in women undergoing frozen–thawed embryo transfers (152, 153). In a single centre study, data were analysed from all consecutive cycles over a 5-year period in women undergoing frozen embryo transfer with natural cycle without hCG triggering or luteal phase support (n = 501) (Group 1), natural cycle without hCG triggering but with luteal phase support (n = 828) (Group 2) or modified natural cycle with hCG triggering and luteal phase support (n = 1024) (Group 3). The clinical pregnancy rate was significantly higher in Group 1 (natural cycle without hCG triggering or luteal phase support) (46.9%) compared with Group 3 (modified natural cycle) (29.7%, p <0.001) but not compared with Group 2 (natural cycle with luteal phase support) (39.9%, p = 0.069) (153). Furthermore, in an RCT in 168 patients undergoing frozen embryo transfer, ongoing pregnancy rates were significantly higher in natural cycles without an hCG trigger compared with modified natural cycles with an hCG trigger (31.1% vs. 14.3%; difference 16.9% [95% CI 4.4, 28.8]) (152).
Statement 14: Compared with hCG Trigger, due to an Additional FSH Surge and the Different Effects of LH and hCG on the Downstream Signalling, the Combined Administration of hCG and GnRH Agonist (dual/double trigger) for Final Oocyte Maturation was Found in a Recent Meta-Analysis to be Associated with a Significantly Higher Pregnancy Rate. Dual/Double Trigger Could Represent a Valid Option but Additional Evidence has to be Provided to Recommend this Approach as First-Line Treatment
This statement received 86% total agreement from the Extended Panel (Figure 2). During COS, use of a GnRH antagonist protocol for pituitary suppression with a GnRH agonist trigger for final follicular maturation has been reported to eliminate the risk of OHSS (154, 155), but some studies have reported reduced clinical pregnancy rates and increased first trimester pregnancy loss with this protocol (156, 157).
In an attempt to improve clinical outcomes, several studies have examined the potential benefits of a dual trigger with hCG and a GnRH agonist (158–163). A systematic review and meta-analysis of four studies in 527 women undergoing ART treatment demonstrated significantly improved clinical pregnancy rates with a dual trigger (GnRH agonist + hCG) compared with hCG alone (OR 0.48 [95% CI 0.31, 0.77], p = 0.002), although there was no significant difference in ongoing pregnancy rates between the groups (158). However, in the four studies included, there was heterogeneity in the criteria used for triggering, different doses were used in the trials that used only hCG triggering and different agonists were used concomitantly with hCG for dual triggering in the studies (158). In another systematic review and meta−analysis of four studies in 527 women undergoing IVF treatment, women receiving a dual trigger had a significantly higher pregnancy rate compared with those receiving hCG alone (RR 1.55 [95% CI 1.17, 2.06]) (164). However, there was no significant difference in the number of oocytes retrieved or implantation rates between the groups. Furthermore, two studies that compared a dual trigger (GnRH agonist + hCG) with a previous IVF cycle using hCG alone, demonstrated that a dual trigger may be beneficial in patients with a low oocyte yield (160) and in patients with a high proportion of immature oocytes (162). A recently published RCT, randomising 155 normal responder patients to receive either hCG or a dual trigger for final oocyte maturation, demonstrated that using a dual trigger for final follicular maturation resulted in improved outcomes (163). The use of a dual trigger significantly increased the number of oocytes retrieved (11.1 vs 13.4, p = 0.002), MII oocytes (8.6 vs 10.3, p = 0.009), total number of blastocysts (2.9 vs 3.9, p = 0.01) and top-quality blastocysts transferred (44.7% vs 64.9%; p = 0.003) compared with the hCG group. Moreover, the clinical pregnancy rate (24.3% vs 46.1%, OR 2.65 [95% CI 1.43, 1.93], p = 0.009) and the live birth rate per transfer (22% vs 36.2%, OR 1.98 [95% CI 1.05, 3.75], p = 0.03) were significantly higher in the dual trigger group compared with the hCG group (163).
The ESHRE 2019 guideline on ovarian stimulation for IVF/ICSI states that a dual trigger with hCG plus a GnRH agonist for final oocyte maturation is probably not recommended for predicted normal responders (29).
Statement 15: A GnRH Agonist Trigger, in a GnRH Antagonist Protocol, is Recommended for Final Oocyte Maturation in Women at risk of OHSS
This statement received 100% agreement from the extended panel (Figure 2). During COS, a GnRH antagonist protocol for pituitary suppression in combination with a GnRH agonist trigger for final follicular maturation is associated with a lower risk of OHSS and may be particularly beneficial in high-risk patients (23, 53). In an RCT including 66 patients aged ≤40 years who were at increased risk of OHSS (PCOS, polycystic ovarian morphology or a previous high ovarian response during ART treatment), the use of a GnRH agonist trigger after GnRH antagonist treatment reduced the risk of any form of OHSS compared with an hCG trigger (0% vs 31%, respectively), with similar rates of implantation (36.0% vs 31.0% with GnRH agonists vs hCG, respectively), clinical pregnancy (56.7% vs 51.7%, respectively) and ongoing pregnancy (53.3% vs 48.3%, respectively) between the groups (165). Furthermore, a Cochrane review of 17 RCTs (n = 1847) in a general patient population (i.e. including studies of women with both low and high risk of OHSS) reported a lower incidence of mild, moderate or severe OHSS with a GnRH agonist trigger compared with an hCG trigger in autologous cycles (OR 0.15 [95% CI 0.05, 0.47], 8 RCTs, 989 women, IO = 42%, moderate-quality evidence) and donor-recipient cycles (OR 0.05 [95% CI 0.01, 0.28]; 3 RCTs, 374 women, IO = 0%) (144). However, GnRH agonists were associated with a lower live birth rate in autologous cycles (OR 0.47 [95% CI 0.31, 0.70], 5 RCTs, 532 women, I2 = 56%, moderate-quality evidence), with no significant difference in live birth rates reported in donor-recipient cycles (OR 0.92 [95% CI 0.53, 1.61]; 1 RCT, 212 women) (144). This study suggests that a GnRH agonist trigger may be beneficial in certain patient populations, including women who choose to avoid fresh transfers, women who donate oocytes, or women who choose to freeze their eggs as part of fertility preservation (144). A retrospective study in women with high ovarian response (n = 272) did not find any significant differences in cumulative live birth rate between a GnRH agonist trigger and an hCG trigger, although GnRH agonists were associated with a lower implantation rate compared with hCG (39% vs 48%, respectively) and required a higher number of embryos transferred to achieve a live birth (57% vs 33%, respectively) (166).
The ESHRE 2019 guideline on ovarian stimulation for IVF/ICSI recommends the use of a GnRH agonist trigger in women at risk of OHSS (29).
Luteal-Phase Support
Statement 16: Vaginal Progesterone Therapy Represents the Gold Standard Approach for Luteal Phase Support After IVF/ICSI. The Route of Progesterone Administration does not Influence Outcomes
This statement received 75% total agreement from the Extended Panel (Figure 2). Progesterone secretion during the luteal phase of the menstrual cycle prepares the endometrium for embryo implantation by inducing endometrial secretory transformation and promoting receptivity, in response to hCG produced by the corpus luteum (167). During ART treatment, the luteal phase is often defective due to reduced progesterone production by the corpus luteum (168), necessitating luteal phase support with exogenous progesterone, GnRH agonists or hCG in some patients. A Cochrane systematic review of 94 RCTs (26,198 women) evaluated the efficacy and safety of different types of luteal phase support, including progesterone, hCG and GnRH agonist supplementation (169). Progesterone supplementation resulted in improved live birth or ongoing pregnancy rates compared with no treatment when data were analysed with a fixed effect model (OR 1.77 [95% CI 1.09, 2.86], 5 RCTs, 642 women, I2 = 35%, very low-quality evidence), although there was no clear difference between the groups using a random-effects model (OR 1.77 [95% CI 0.96, 3.26]). Progesterone treatment was also associated with similar live birth or ongoing pregnancy rates (OR 0.95 [95% CI 0.65, 1.38], 5 RCTs, 833 women, I2 = 0%, low-quality evidence) and lower OHSS rates (OR 0.46 [95% CI 0.30, 0.71] 5 RCTs, 1293 women, I2 = 48%) compared with hCG treatment (169). Pregnancy outcomes were compared in women receiving progesterone luteal phase support versus no luteal phase support in a systematic review and meta-analysis of 11 RCTs comprising 2842 patients undergoing ovulation induction and intrauterine insemination. Women who received progesterone support had improved clinical pregnancy rates (RR 1.56 [95% CI 1.21, 2.02]) and live birth rates (RR 1.77 [95% CI 1.30, 2.42]) compared with women who received no luteal phase support (170).
Although progesterone is considered the standard treatment for luteal phase support during ART (169–171), there remains substantial debate regarding the timing and routes of progesterone supplementation (171–173). In an RCT assessing the route of progesterone administration for luteal phase support in 168 women undergoing IVF treatment, rectal administration was demonstrated to be as effective as vaginal administration (173). Furthermore, an RCT including 233 women aged ≤35 years undergoing IVF using a GnRH agonist long protocol reported similar clinical pregnancy rates, implantation rates, live birth rates and miscarriage rates when progesterone support was given 1 day after oocyte retrieval or on the day of oocyte retrieval (172).
The ESHRE 2019 guideline on ovarian stimulation for IVF/ICSI recommends the use of progesterone for luteal phase support, by any non-oral administration route (29).
Statement 17 (revote): Addition of GnRH Agonist Injections to Progesterone in Luteal Phase Support Appears to Improve Outcome. Nowadays Mid-Luteal GnRH-Agonists are Frequently Introduced in Addition to Progesterone for Luteal Support
This statement received 67% total agreement from the Extended Panel (Figure 2). GnRH agonist use in luteal phase support is understood to improve embryo developmental potential by directly acting on the embryo, as well as potentially by affecting uterine receptivity and corpus luteum function (150, 174–176). The addition of a GnRH agonist to progesterone luteal phase support has been supported by a number of studies (169, 177–179). In a Cochrane systematic review by van der Linden et al, luteal phase support using progesterone alone resulted in lower live birth or ongoing pregnancy rates compared with progesterone plus GnRH agonist treatment (OR 0.62 [95% CI 0.48, 0.81], 7 RCTs, 2861 women, I2 = 55%, low-quality evidence), with a similar risk of OHSS between the groups (OR 1.00 [95% CI 0.33, 3.01], 1 RCT, 300 women, very low quality evidence) (169). In a study of 50 women who had abnormally low luteal-phase serum progesterone levels after a first failed IVF attempt, women were assigned to receive luteal phase support with progesterone plus GnRH agonist supplementation versus progesterone alone during a second IVF attempt (177). The progesterone plus GnRH agonist group had significantly higher progesterone levels in the second IVF attempt compared with the first attempt (p < 0.001), and 48% of them achieved clinical pregnancy and birth; whereas in the progesterone only group, progesterone levels and pregnancy outcomes were similar between the first and second attempt. In an RCT in 98 women undergoing natural cycle frozen embryo transfer, there were numerically more clinical pregnancies and live births in women receiving luteal phase support with progesterone plus GnRH agonist compared with progesterone alone, but the difference did not reach statistical significance (178). In an RCT in 279 patients, Yildiz et al. reported numerical improvements in ongoing pregnancy rates with the addition of a GnRH agonist to progesterone luteal phase support compared with progesterone alone, but the difference did not reach statistical significance (p = 0.09) (179).
The ESHRE 2019 guideline on ovarian stimulation for IVF/ICSI recommends that the addition of a GnRH agonist, in progesterone luteal phase support in hCG triggered cycles, can only be used in the context of a clinical trial (29).
This statement received 33% disagreement from the extended panel, although it is important to note that only 21 of the 35 experts (60%) voted on this statement. The motivations supporting these disagreements are outlined in Supplementary Table 1. The majority of experts disagreeing with this statement did not agree with the word ‘frequently’, suggesting that the addition of a GnRH agonist to progesterone luteal phase support may differ between countries.
Statement 18: Addition of LH Activity to Progesterone in Luteal Phase Support Improves Pregnancy Outcomes in GnRH Agonist Trigger Fresh Embryo Transfer Cycles
This statement received 68% total agreement from the Extended Panel (Figure 2). In GnRH antagonist cycles in which ovulation was triggered with a GnRH agonist, low dose hCG (1500 IU) has been demonstrated to sustain implantation and luteal ovarian steroidogenesis, providing luteal phase support in normal responders and in patients at high risk of developing OHSS (180, 181). However, there is still risk of developing OHSS with low-dose hCG luteal support following agonist triggering (182, 183). A systematic review and meta-analysis of five studies in 859 women undergoing IVF/ICSI with a GnRH antagonist protocol reported similar live birth rates in women receiving a GnRH agonist trigger plus modified luteal phase support with supplementation with exogenous r-hLH or hCG, compared with an hCG trigger plus standard luteal phase support (OR 0.84 [95% CI 0.62, 1.14], I2 = 22%) (184). hCG luteal phase support was investigated in a small proof of concept study (11 IVF cycles) in women at high risk of OHSS (160). All women received “intense” luteal phase support with oestradiol and progesterone, and those women showing no signs of early moderate OHSS on Day 3 after oocyte retrieval also received hCG. The women receiving luteal phase support plus hCG had significantly higher mid-luteal phase progesterone levels and a non-significant increase in pregnancy rates compared with those women receiving luteal phase support alone (40% vs 17%, respectively). No cases of severe OHSS were reported in either group. This study suggests a possible role for the use of delayed (Day 3) hCG treatment, with the possibility of identifying those women who may benefit from hCG without developing severe OHSS, but more studies are needed to confirm these data (160).
The ESHRE 2019 guideline on ovarian stimulation for IVF/ICSI only recommends the addition of r-hLH to progesterone luteal phase support in the context of a clinical trial (29).
This statement received 32% total disagreement from the extended panel. The motivations supporting these disagreements are outlined in Supplementary Table 1. Most experts cited a lack of evidence as the reason for their disagreement.
Discussion
This Delphi consensus provides a real-world clinical perspective on the specific approaches during key steps of ART treatment from a diverse group of international experts.
Strengths
The consensus has a number of strengths, including the fact that it used the Delphi methodology and benefited from the knowledge of an international panel of highly respected experts. The consensus enabled the inclusion of more topics than would typically be addressed in a systematic review or in a guideline approach, which are usually based on strict methodology, limiting the scope of investigation. For example, the ESHRE 2019 guideline on ovarian stimulation for IVF/ICSI (29) was developed based on the Manual for ESHRE guideline development (185), which provides a stepwise approach to guideline development. The types of studies included in the guideline focussed on prospective (randomised) controlled studies following a hierarchical approach, with priority given to systematic reviews and meta-analyses, followed by RCTs, then cohort studies and case reports. In contrast, this consensus supported the inclusion of not only RCTs but also other evidence and expert experience. The results of the consensus are supplementary to the ESHRE 2019 guideline on ovarian stimulation for IVF/ICSI (29) and, as outlined in the Results and Discussion section, its outcomes are mostly in-line with recommendations from ESHRE, but often provide additional specific considerations.
Limitations
The consensus also has some limitations; for example, the consensus does not represent an exhaustive list of statements and the statements only represent the collective opinion of the experts included. Not all statements reached 100% agreement, with some statements reaching consensus even though some participants disagreed with them. Furthermore, although these statements represent the point of view of the experts, individual patient characteristics should always be taken into consideration with regards to treatment options.
Conclusions
This Delphi consensus provides expert opinion on specific approaches during ART treatment, including follicular development and stimulation with gonadotropins, pituitary suppression, final oocyte maturation triggering and luteal-phase support. The fertility experts participating in the consensus were from a diverse range of global regions, including Europe, Asia, Pacific, Middle East and South America, reflecting the quality of healthcare and different approaches to infertility treatment in different parts of the world. All statements reached consensus and a high level of agreement was achieved on >50% of statements. The extended panel achieved a good level of agreement for follicular development and ovarian stimulation, pituitary suppression, and oocyte triggering. Furthermore, this consensus confirmed that luteal-phase support remains a more debated area. The clinical perspectives included in this consensus supplement clinical guidelines and policies that help to further improve treatment outcomes.
Data Availability Statement
Any requests for data by qualified scientific and medical researchers for legitimate research purposes will be subject to Merck KGaA’s Data Sharing Policy. All requests should be submitted in writing to Merck KGaA’s data sharing portal https://www.merckgroup.com/en/research/our-approach-to-research-and-development/healthcare/clinical-trials/commitment-responsible-data-sharing.html. When Merck KGaA has a co-research, co-development, or co-marketing or co-promotion agreement, or when the product has been out-licensed, the responsibility for disclosure might be dependent on the agreement between parties. Under these circumstances, Merck KGaA will endeavour to gain agreement to share data in response to requests.
Author Contributions
RO contributed to project direction and coordination, assisted with drafting the article and revising it critically for important intellectual content, and provided final approval of the version to be published. CV, HF, RF, YK, MH, MG, SE, SS, YL and CA were expert panel members and survey participants and assisted with editing and reviewing the manuscript and provided final approval of the version to be published. TD and SL contributed to overall concept and design, assisted with manuscript drafting, revision and provided final approval of the version to be published. All authors contributed to the article and approved the submitted version.
Funding
The work was funded by Merck KGaA, Darmstadt, Germany.
Conflict of Interest
TD and SL are employees of Merck KGaA, Darmstadt, Germany. RO received speaker fees/honoraria from Ferring and Merck KGaA, Darmstadt, Germany. CV is supported by a NHMRC Early Career Fellowship (GNT1147154). He also has equity interests in Virtus Health and reports grants, personal fees and non-financial support from Merck KGaA, Darmstadt, Germany, personal fees and non-financial support from MSD, grants and non-financial support from Ferring, personal fees from Besins Healthcare, and grants and non-financial support from Abbott. HF received speaker fees/honoraria/grants from MSD, Ferring, Besin, Merck KGaA, Darmstadt, Germany, and Sun Pharma. RF received consulting fees and honoraria from Merck KGaA, Darmstadt, Germany. YK received speaker fees/honoraria/grants from MSD, Merck KGaA, Darmstadt, Germany, Besins Healthcare, Gedeon Richter, Abbott, Bayer, and Sun Pharma. MH declares receipt of research grants from Merck KGaA, Darmstadt, Germany, and lecture fees from Merck KGaA, Darmstadt, Germany and Ferring. MG received fees from Merck KGaA, Darmstadt, Germany, Ferring, Gedeon Richter, MSD, IBSA. SE declares receipt of unrestricted research grants from Merck KGaA, Darmstadt, Germany, and lecture fees from Merck KGaA, Darmstadt, Germany, Gedeon Richter and Medical Education Academy. SS was speaker at non-promotional educational symposia by Merck KGaA, Darmstadt, Germany and Ferring in the last 12 months. YL received speaker fees/grants from Merck KGaA, Darmstadt, Germany, MSD, and Bayer. CA received consulting fees and payment/honoraria from Merck KGaA, Darmstadt, Germany.
Acknowledgments
Medical writing support was provided by Helen Brereton, inScience Communication, and funded by Merck KGaA, Darmstadt, Germany. The Delphi consensus process was coordinated by Sanitanova Srl.
Supplementary Material
The Supplementary Material for this article can be found online at: https://www.frontiersin.org/articles/10.3389/fendo.2021.675670/full#supplementary-material
References
1. Zegers-Hochschild F, Adamson GD, Dyer S, Racowsky C, de Mouzon J, Sokol R, et al. The International Glossary on Infertility and Fertility Care, 2017. Hum Reprod (Oxford England) (2017) 32(9):1786–801. doi: 10.1093/humrep/dex234
2. Practice Committee of the American Society for Reproductive Medicine. Definitions of Infertility and Recurrent Pregnancy Loss: A Committee Opinion. Fertil Steril (2020) 113(3):533–5. doi: 10.1016/j.fertnstert.2019.11.025
3. World Health Organization. World Report on Disability (2011). Available at: https://apps.who.int/iris/bitstream/handle/10665/70670/WHO_NMH_VIP_11.01_eng.pdf;jsessionid=BEA4F8A359F5142D6DA005DD6C32981A?sequence=1 (Accessed 27 May 2020).
4. World Health Organization. Fact Sheet: Fertility (2020). Available at: https://www.who.int/news-room/fact-sheets/detail/infertility (Accessed 27/01/2021).
5. Greenhall E, Vessey M. The Prevalence of Subfertility: A Review of the Current Confusion and a Report of Two New Studies. Fertil Steril (1990) 54(6):978–83. doi: 10.1016/s0015-0282(16)53990-9
6. Wang J, Sauer MV. In Vitro Fertilization (IVF): A Review of 3 Decades of Clinical Innovation and Technological Advancement. Ther Clin Risk Manag (2006) 2(4):355–64. doi: 10.2147/tcrm.2006.2.4.355
7. Brezina PR, Ning N, Mitchell E, Zacur HA, Baramki TA, Zhao Y. Recent Advances in Assisted Reproductive Technology. Curr Obstetrics Gynecol Rep (2012) 1:166–73. doi: 10.1007/s13669-012-0019-2
8. Casper R, Haas J, Hsieh TB, Bassil R, Mehta C. Recent Advances in In Vitro Fertilization. F1000Res (2017) 6:1616. doi: 10.12688/f1000research.11701.1
9. European Society of Human Reproduction and Embryology. ART Fact Sheet (2020). Available at: https://www.eshre.eu/Press-Room/Resources (Accessed 27/01/2021).
10. Steptoe PC, Edwards RG. Birth After the Reimplantation of a Human Embryo. Lancet (1978) 2(8085):366. doi: 10.1016/s0140-6736(78)92957-4
11. Kovalevsky G, Patrizio P. High Rates of Embryo Wastage With Use of Assisted Reproductive Technology: A Look At the Trends Between 1995 and 2001 in the United States. Fertil Steril (2005) 84(2):325–30. doi: 10.1016/j.fertnstert.2005.04.020
12. Martin JR, Bromer JG, Sakkas D, Patrizio P. Live Babies Born Per Oocyte Retrieved in a Subpopulation of Oocyte Donors With Repetitive Reproductive Success. Fertil Steril (2010) 94(6):2064–8. doi: 10.1016/j.fertnstert.2010.02.004
13. Patrizio P, Bianchi V, Lalioti MD, Gerasimova T, Sakkas D. High Rate of Biological Loss in Assisted Reproduction: it is in the Seed, Not in the Soil. Reprod BioMed Online (2007) 14(1):92–5. doi: 10.1016/s1472-6483(10)60769-9
14. Patrizio P, Sakkas D. From Oocyte to Baby: A Clinical Evaluation of the Biological Efficiency of In Vitro Fertilization. Fertil Steril (2009) 91(4):1061–6. doi: 10.1016/j.fertnstert.2008.01.003
15. McLernon DJ, Steyerberg EW, Te Velde ER, Lee AJ, Bhattacharya S. Predicting the Chances of a Live Birth After One or More Complete Cycles of In Vitro Fertilisation: Population Based Study of Linked Cycle Data From 113 873 Women. BMJ (2016) 355:i5735. doi: 10.1136/bmj.i5735
16. SART. National Summary Report. (2017). Available at: https://www.sartcorsonline.com.
17. Van Voorhis BJ. Outcomes From Assisted Reproductive Technology. Obstet Gynecol (2006) 107(1):183–200. doi: 10.1097/01.AOG.0000194207.06554.5b
18. Hansen M, Kurinczuk JJ, Milne E, de Klerk N, Bower C. Assisted Reproductive Technology and Birth Defects: A Systematic Review and Meta-Analysis. Hum Reprod Update (2013) 19(4):330–53. doi: 10.1093/humupd/dmt006
19. Lazaraviciute G, Kauser M, Bhattacharya S, Haggarty P, Bhattacharya S. A Systematic Review and Meta-Analysis of DNA Methylation Levels and Imprinting Disorders in Children Conceived by IVF/ICSI Compared With Children Conceived Spontaneously. Hum Reprod Update (2015) 21(4):555–7. doi: 10.1093/humupd/dmv017
20. Vermeiden JP, Bernardus RE. Are Imprinting Disorders More Prevalent After Human In Vitro Fertilization or Intracytoplasmic Sperm Injection? Fertil Steril (2013) 99(3):642–51. doi: 10.1016/j.fertnstert.2013.01.125
21. Fauser B. Patient-Tailored Ovarian Stimulation for In Vitro Fertilization. Fertil Steril (2017) 108(4):585–91. doi: 10.1016/j.fertnstert.2017.08.016
22. Mol BW, Bossuyt PM, Sunkara SK, Garcia Velasco JA, Venetis C, Sakkas D, et al. Personalized Ovarian Stimulation for Assisted Reproductive Technology: Study Design Considerations to Move From Hype to Added Value for Patients. Fertil Steril (2018) 109(6):968–79. doi: 10.1016/j.fertnstert.2018.04.037
23. Orvieto R. A Simplified Universal Approach to COH Protocol for IVF: Ultrashort Flare GnRH-agonist/GnRH-antagonist Protocol With Tailored Mode and Timing of Final Follicular Maturation. J Ovarian Res (2015) 8:69. doi: 10.1186/s13048-015-0198-3
24. Holter H, Sandin-Bojo AK, Gejervall AL, Wikland M, Wilde-Larsson B, Bergh C. Patient-Centred Quality of Care in an IVF Programme Evaluated by Men and Women. Hum Reprod (Oxford England) (2014) 29(12):2695–703. doi: 10.1093/humrep/deu254
25. Gameiro S, Boivin J, Peronace L, Verhaak CM. Why do Patients Discontinue Fertility Treatment? A Systematic Review of Reasons and Predictors of Discontinuation in Fertility Treatment. Hum Reprod Update (2012) 18(6):652–69. doi: 10.1093/humupd/dms031
26. Gameiro S, Verhaak CM, Kremer JA, Boivin J. Why We Should Talk About Compliance With Assisted Reproductive Technologies (ART): A Systematic Review and Meta-Analysis of ART Compliance Rates. Hum Reprod Update (2013) 19(2):124–35. doi: 10.1093/humupd/dms045
27. Rajkhowa M, McConnell A, Thomas GE. Reasons for Discontinuation of IVF Treatment: A Questionnaire Study. Hum Reprod (Oxford England) (2006) 21(2):358–63. doi: 10.1093/humrep/dei355
28. Verberg MF, Eijkemans MJ, Heijnen EM, Broekmans FJ, de Klerk C, Fauser BC, et al. Why do Couples Drop-Out From IVF Treatment? A Prospective Cohort Study. Hum Reprod (Oxford England) (2008) 23(9):2050–5. doi: 10.1093/humrep/den219
29. Bosch E, Broer S, Griesinger G, Grynberg M, Humaidan P, Kolibianakis E, et al. ESHRE Guideline: Ovarian Stimulation for IVF/ICSI. Hum Reprod Open (2020) 2020(2):hoaa009. doi: 10.1093/hropen/hoaa009
30. Hershkop E, Segal L, Fainaru O, Kol S. ‘Model’ Versus ‘Everyday’ Patients: can Randomized Controlled Trial Data Really be Applied to the Clinic? Reprod BioMed Online (2017) 34(3):274–9. doi: 10.1016/j.rbmo.2016.11.010
31. Concolino D, Degennaro E, Parini R. Fabry Delphi Working G, Fabry Delphi Working G. Delphi Consensus on the Current Clinical and Therapeutic Knowledge on Anderson-Fabry Disease. Eur J Intern Med (2014) 25(8):751–6. doi: 10.1016/j.ejim.2014.07.009
32. Girolomoni G, Altomare G, Ayala F, Berardesca E, Calzavara Pinton P, Chimenti S, et al. Differential Management of Mild-to-Severe Psoriasis With Biologic Drugs: An Italian Delphi Consensus Expert Panel. J Dermatol Treat (2015) 26(2):128–33. doi: 10.3109/09546634.2014.907466
33. Santi D, Casarini L, Alviggi C, Simoni M. Efficacy of Follicle-Stimulating Hormone (Fsh) Alone, Fsh + Luteinizing Hormone, Human Menopausal Gonadotropin or FSH + Human Chorionic Gonadotropin on Assisted Reproductive Technology Outcomes in the “Personalized” Medicine Era: A Meta-Analysis. Front Endocrinol (Lausanne) (2017) 8:114. doi: 10.3389/fendo.2017.00114
34. Tabata C, Fujiwara T, Sugawa M, Noma M, Onoue H, Kusumi M, et al. Comparison of FSH and hMG on Ovarian Stimulation Outcome With a GnRH Antagonist Protocol in Younger and Advanced Reproductive Age Women. Reprod Med Biol (2015) 14:5–9. doi: 10.1007/s12522-014-0186-0
35. Conforti A, Esteves SC, Di Rella F, Strina I, De Rosa P, Fiorenza A, et al. The Role of Recombinant LH in Women With Hypo-Response to Controlled Ovarian Stimulation: A Systematic Review and Meta-Analysis. Reprod Biol Endocrinol (2019) 17(1):18. doi: 10.1186/s12958-019-0460-4
36. La Marca A, Papaleo E, Grisendi V, Argento C, Giulini S, Volpe A. Development of a Nomogram Based on Markers of Ovarian Reserve for the Individualisation of the Follicle-Stimulating Hormone Starting Dose in In Vitro Fertilisation Cycles. Bjog (2012) 119(10):1171–9. doi: 10.1111/j.1471-0528.2012.03412.x
37. Humaidan P, Alviggi C, Fischer R, Esteves SC. The Novel POSEIDON Stratification of ‘Low Prognosis Patients in Assisted Reproductive Technology’ and its Proposed Marker of Successful Outcome. F1000Res (2016) 5:2911. doi: 10.12688/f1000research.10382.1
38. Poseidon Group, Alviggi C, Andersen CY, Buehler K, Conforti A, De Placido G, et al. A New More Detailed Stratification of Low Responders to Ovarian Stimulation: From a Poor Ovarian Response to a Low Prognosis Concept. Fertil Steril (2016) 105(6):1452–3. doi: 10.1016/j.fertnstert.2016.02.005
39. Genro VK, Grynberg M, Scheffer JB, Roux I, Frydman R, Fanchin R. Serum anti-Mullerian Hormone Levels are Negatively Related to Follicular Output RaTe (FORT) in Normo-Cycling Women Undergoing Controlled Ovarian Hyperstimulation. Hum Reprod (Oxford England) (2011) 26(3):671–7. doi: 10.1093/humrep/deq361
40. Alviggi C, Conforti A, Esteves SC, Vallone R, Venturella R, Staiano S, et al. Understanding Ovarian Hypo-Response to Exogenous Gonadotropin in Ovarian Stimulation and Its New Proposed Marker-the Follicle-To-Oocyte (Foi) Index. Front Endocrinol (Lausanne) (2018) 9:589. doi: 10.3389/fendo.2018.00589
41. Drakopoulos P, Blockeel C, Stoop D, Camus M, de Vos M, Tournaye H, et al. Conventional Ovarian Stimulation and Single Embryo Transfer for IVF/ICSI. How Many Oocytes do We Need to Maximize Cumulative Live Birth Rates After Utilization of All Fresh and Frozen Embryos? Hum Reprod (Oxford England) (2016) 31(2):370–6. doi: 10.1093/humrep/dev316
42. Ji J, Liu Y, Tong XH, Luo L, Ma J, Chen Z. The Optimum Number of Oocytes in IVF Treatment: An Analysis of 2455 Cycles in China. Hum Reprod (Oxford England) (2013) 28(10):2728–34. doi: 10.1093/humrep/det303
43. Polyzos NP, Drakopoulos P, Parra J, Pellicer A, Santos-Ribeiro S, Tournaye H, et al. Cumulative Live Birth Rates According to the Number of Oocytes Retrieved After the First Ovarian Stimulation for In Vitro Fertilization/Intracytoplasmic Sperm Injection: A Multicenter Multinational Analysis Including Approximately 15,000 Women. Fertil Steril (2018) 110(4):661–70 e1. doi: 10.1016/j.fertnstert.2018.04.039
44. Sunkara SK, Rittenberg V, Raine-Fenning N, Bhattacharya S, Zamora J, Coomarasamy A. Association Between the Number of Eggs and Live Birth in IVF Treatment: An Analysis of 400 135 Treatment Cycles. Hum Reprod (Oxford England) (2011) 26(7):1768–74. doi: 10.1093/humrep/der106
45. Law YJ, Zhang N, Kolibianakis EM, Costello MF, Keller E, Chambers GM, et al. Is There an Optimal Number of Oocytes Retrieved At Which Live Birth Rates or Cumulative Live Birth Rates Per Aspiration are Maximized After ART? A Systematic Review. Reprod BioMed Online (2021) 42(1):83–104. doi: 10.1016/j.rbmo.2020.10.008
46. Engmann L, Maconochie N, Bekir JS, Jacobs HS, Tan SL. Cumulative Probability of Clinical Pregnancy and Live Birth After a Multiple Cycle IVF Package: A More Realistic Assessment of Overall and Age-Specific Success Rates? Br J Obstet Gynaecol (1999) 106(2):165–70. doi: 10.1111/j.1471-0528.1999.tb08217.x
47. Olivius K, Friden B, Lundin K, Bergh C. Cumulative Probability of Live Birth After Three In Vitro Fertilization/Intracytoplasmic Sperm Injection Cycles. Fertil Steril (2002) 77(3):505–10. doi: 10.1016/s0015-0282(01)03217-4
48. Malizia BA, Hacker MR, Penzias AS. Cumulative Live-Birth Rates After In Vitro Fertilization. N Engl J Med (2009) 360(3):236–43. doi: 10.1056/NEJMoa0803072
49. Moragianni VA, Penzias AS. Cumulative Live-Birth Rates After Assisted Reproductive Technology. Curr Opin Obstet Gynecol (2010) 22(3):189–92. doi: 10.1097/GCO.0b013e328338493f
50. Maheshwari A, McLernon D, Bhattacharya S. Cumulative Live Birth Rate: Time for a Consensus? Hum Reprod (Oxford England) (2015) 30(12):2703–7. doi: 10.1093/humrep/dev263
51. Abuzeid MI, Bolonduro O, La Chance J, Abozaid T, Urich M, Ullah K, et al. Cumulative Live Birth Rate and Assisted Reproduction: Impact of Female Age and Transfer Day. Facts Views Vis Obgyn (2014) 6(3):145–9.
52. McLernon DJ, Maheshwari A, Lee AJ, Bhattacharya S. Cumulative Live Birth Rates After One or More Complete Cycles of IVF: A Population-Based Study of Linked Cycle Data From 178,898 Women. Hum Reprod (Oxford England) (2016) 31(3):572–81. doi: 10.1093/humrep/dev336
53. Ubaldi FM, Cimadomo D, Vaiarelli A, Fabozzi G, Venturella R, Maggiulli R, et al. Advanced Maternal Age in IVF: Still a Challenge? The Present and the Future of Its Treatment. Front Endocrinol (Lausanne) (2019) 10:94. doi: 10.3389/fendo.2019.00094
54. Pinborg A, Hougaard CO, Nyboe Andersen A, Molbo D, Schmidt L. Prospective Longitudinal Cohort Study on Cumulative 5-Year Delivery and Adoption Rates Among 1338 Couples Initiating Infertility Treatment. Hum Reprod (Oxford England) (2009) 24(4):991–9. doi: 10.1093/humrep/den463
55. Stern JE, Brown MB, Luke B, Wantman E, Lederman A, Missmer SA, et al. Calculating Cumulative Live-Birth Rates From Linked Cycles of Assisted Reproductive Technology (ART): Data From the Massachusetts Sart Cors. Fertil Steril (2010) 94(4):1334–40. doi: 10.1016/j.fertnstert.2009.05.052
56. Lebovitz O, Haas J, James KE, Seidman DS, Orvieto R, Hourvitz A. The Expected Cumulative Incidence of Live Birth for Patients Starting IVF Treatment At Age 41 Years or Older. Reprod BioMed Online (2018) 37(5):533–41. doi: 10.1016/j.rbmo.2018.08.014
57. Gharib SD, Wierman ME, Shupnik MA, Chin WW. Molecular Biology of the Pituitary Gonadotropins. Endocr Rev (1990) 11(1):177–99. doi: 10.1210/edrv-11-1-177
58. Green ED, Baenziger JU. Asparagine-Linked Oligosaccharides on Lutropin, Follitropin, and Thyrotropin. II. Distributions of Sulfated and Sialylated Oligosaccharides on Bovine, Ovine, and Human Pituitary Glycoprotein Hormones. J Biol Chem (1988) 263(1):36–44. doi: 10.1016/S0021-9258(19)57352-5
59. Bousfield GR, May JV, Davis JS, Dias JA, Kumar TR. In Vivo and In Vitro Impact of Carbohydrate Variation on Human Follicle-Stimulating Hormone Function. Front Endocrinol (2018) 9:216. doi: 10.3389/fendo.2018.00216
60. Butnev VY, Butnev VY, May JV, Shuai B, Tran P, White WK, et al. Production, Purification, and Characterization of Recombinant hFSH Glycoforms for Functional Studies. Mol Cell Endocrinol (2015) 405:42–51. doi: 10.1016/j.mce.2015.01.026
61. Ulloa-Aguirre A, Timossi C, Damian-Matsumura P, Dias JA. Role of Glycosylation in Function of Follicle-Stimulating Hormone. Endocrine (1999) 11(3):205–15. doi: 10.1385/ENDO:11:3:205
62. Mulders JW, Derksen M, Swolfs A, Maris F. Prediction of the In Vivo Biological Activity of Human Recombinant Follicle Stimulating Hormone Using Quantitative Isoelectric Focusing. Biologicals (1997) 25(3):269–81. doi: 10.1006/biol.1997.0097
63. D’Antonio M, Borrelli F, Datola A, Bucci R, Mascia M, Polletta P, et al. Biological Characterization of Recombinant Human Follicle Stimulating Hormone Isoforms. Hum Reprod (Oxford England) (1999) 14(5):1160–7. doi: 10.1093/humrep/14.5.1160
64. Orvieto R, Seifer DB. Biosimilar FSH Preparations- are They Identical Twins or Just Siblings? Reprod Biol Endocrinol (2016) 14(1):32. doi: 10.1186/s12958-016-0167-8
65. Vitt UA, Kloosterboer HJ, Rose UM, Mulders JW, Kiesel PS, Bete S, et al. Isoforms of Human Recombinant Follicle-Stimulating Hormone: Comparison of Effects on Murine Follicle Development In Vitro. Biol Reprod (1998) 59(4):854–61. doi: 10.1095/biolreprod59.4.854
66. Steelman SL, Pohley FM. Assay of the Follicle Stimulating Hormone Based on the Augmentation With Human Chorionic Gonadotropin. Endocrinology (1953) 53(6):604–16. doi: 10.1210/endo-53-6-604
67. Orvieto R, Nahum R, Rabinson J, Ashkenazi J, Anteby EY, Meltcer S. Follitropin-Alpha (Gonal-F) Versus Follitropin-Beta (Puregon) in Controlled Ovarian Hyperstimulation for In Vitro Fertilization: Is There Any Difference? Fertil Steril (2009) 91(4 Suppl):1522–5. doi: 10.1016/j.fertnstert.2008.08.112
68. European Medicines Agency. Guideline on Similar Biological Medicinal Products Containing Biotechnology-Derived Proteins as Active Substance: non-Clinical and Clinical Issues (2014). Available at: https://ema.europa.eu/en/documents/scientific-guideline/guideline-similar-biological-medicinal-products-containing-biotechnology-derived-proteins-active_en-2.pdf (Accessed 08 Nov 2020).
69. Mastrangeli R, Satwekar A, Cutillo F, Ciampolillo C, Palinsky W, Longobardi S. In-Vivo Biological Activity and Glycosylation Analysis of a Biosimilar Recombinant Human Follicle-Stimulating Hormone Product (Bemfola) Compared With its Reference Medicinal Product (GONAL-F). PloS One (2017) 12(9):e0184139. doi: 10.1371/journal.pone.0184139
70. de Mora F, Fauser B. Biosimilars to Recombinant Human FSH Medicines: Comparable Efficacy and Safety to the Original Biologic. Reprod BioMed Online (2017) 35(1):81–6. doi: 10.1016/j.rbmo.2017.03.020
71. Rettenbacher M, Andersen AN, Garcia-Velasco JA, Sator M, Barri P, Lindenberg S, et al. A Multi-Centre Phase 3 Study Comparing Efficacy and Safety of Bemfola((R)) Versus Gonal-f((R)) in Women Undergoing Ovarian Stimulation for IVF. Reprod BioMed Online (2015) 30(5):504–13. doi: 10.1016/j.rbmo.2015.01.005
72. Strowitzki T, Kuczynski W, Mueller A, Bias P. Randomized, Active-Controlled, Comparative Phase 3 Efficacy and Safety Equivalence Trial of Ovaleap(R) (Recombinant Human Follicle-Stimulating Hormone) in Infertile Women Using Assisted Reproduction Technology (ART). Reprod Biol Endocrinol (2016) 14:1. doi: 10.1186/s12958-015-0135-8
73. Chua SJ, Longobardi S, Mol BW, Wang R, Venetis CA, Storr A, et al. Live Birth Rates and Ovarian Hyperstimulation Syndrome (OHSS) Risk With Recombinant Follitropin Alfa Biosimilar Preparations Versus Originator Preparations: A Systematic Review and Meta-Analysis. Presented At the American Society for Reproductive Medicine (ASRM) 76th Scientific Congress & Expo. Fertil Steril (2020) 114(3):e164–5. doi: 10.1016/j.fertnstert.2020.08.470
74. Budani MC, Fensore S, Di Marzio M, Tiboni GM. Efficacy and Safety of Follitropin Alpha Biosimilars Compared to Their Reference Product: A Meta-Analysis. Gynecol Endocrinol (2020) 1–9. doi: 10.1080/09513590.2020.1792437
75. Ulloa-Aguirre A, Espinoza R, Damian-Matsumura P, Chappel SC. Immunological and Biological Potencies of the Different Molecular Species of Gonadotrophins. Hum Reprod (Oxford England) (1988) 3(4):491–501. doi: 10.1093/oxfordjournals.humrep.a136734
76. Lambert A, Talbot JA, Anobile CJ, Robertson WR. Gonadotrophin Heterogeneity and Biopotency: Implications for Assisted Reproduction. Mol Hum Reprod (1998) 4(7):619–29. doi: 10.1093/molehr/4.7.619
77. Ulloa-Aguirre A, Midgley AR Jr., Beitins IZ, Padmanabhan V. Follicle-Stimulating Isohormones: Characterization and Physiological Relevance. Endocr Rev (1995) 16(6):765–87. doi: 10.1210/edrv-16-6-765
78. Anobile CJ, Talbot JA, McCann SJ, Padmanabhan V, Robertson WR. Glycoform Composition of Serum Gonadotrophins Through the Normal Menstrual Cycle and in the Post-Menopausal State. Mol Hum Reprod (1998) 4(7):631–9. doi: 10.1093/molehr/4.7.631
79. Padmanabhan V, Lang LL, Sonstein J, Kelch RP, Beitins IZ. Modulation of Serum Follicle-Stimulating Hormone Bioactivity and Isoform Distribution by Estrogenic Steroids in Normal Women and in Gonadal Dysgenesis. J Clin Endocrinol Metab (1988) 67(3):465–73. doi: 10.1210/jcem-67-3-465
80. Zambrano E, Olivares A, Mendez JP, Guerrero L, Diaz-Cueto L, Veldhuis JD, et al. Dynamics of Basal and Gonadotropin-Releasing Hormone-Releasable Serum Follicle-Stimulating Hormone Charge Isoform Distribution Throughout the Human Menstrual Cycle. J Clin Endocrinol Metab (1995) 80(5):1647–56. doi: 10.1210/jcem.80.5.7745013
81. Lispi M, Bassett R, Crisci C, Mancinelli M, Martelli F, Ceccarelli D, et al. Comparative Assessment of the Consistency and Quality of a Highly Purified FSH Extracted From Human Urine (Urofollitropin) and a Recombinant Human FSH (Follitropin Alpha). Reprod BioMed Online (2006) 13(2):179–93. doi: 10.1016/s1472-6483(10)60613-x
82. Frydman R, Howles CM, Truong F. A Double-Blind, Randomized Study to Compare Recombinant Human Follicle Stimulating Hormone (FSH; Gonal-F) With Highly Purified Urinary FSH (Metrodin) HP) in Women Undergoing Assisted Reproductive Techniques Including Intracytoplasmic Sperm Injection. The French Multicentre Trialists. Hum Reprod (Oxford England) (2000) 15(3):520–5. doi: 10.1093/humrep/15.3.520
83. van Wely M, Kwan I, Burt AL, Thomas J, Vail A, Van der Veen F, et al. Recombinant Versus Urinary Gonadotrophin for Ovarian Stimulation in Assisted Reproductive Technology Cycles. Cochrane Database Syst Rev (2011) 2:CD005354. doi: 10.1002/14651858.CD005354.pub2
84. Gurgan T, Montjean D, Demirol A, Menezo YJ. Sequential (hFSH + recFSH) vs Homogenous (hFSH or recFSH Alone) Stimulation: Clinical and Biochemical (Cumulus Cell Gene Expression) Aspects. J Assist Reprod Genet (2014) 31(6):657–65. doi: 10.1007/s10815-014-0208-1
85. Porter RN, Smith W, Craft IL, Abdulwahid NA, Jacobs HS. Induction of Ovulation for in-Vitro Fertilisation Using Buserelin and Gonadotropins. Lancet (1984) 2(8414):1284–5. doi: 10.1016/s0140-6736(84)92840-x
86. Smitz J, Devroey P, Camus M, Deschacht J, Khan I, Staessen C, et al. The Luteal Phase and Early Pregnancy After Combined GnRH-agonist/HMG Treatment for Superovulation in IVF or GIFT. Hum Reprod (Oxford England) (1988) 3(5):585–90. doi: 10.1093/oxfordjournals.humrep.a136750
87. Mochtar MH, Danhof NA, Ayeleke RO, Van der Veen F, van Wely M. Recombinant Luteinizing Hormone (rLH) and Recombinant Follicle Stimulating Hormone (rFSH) for Ovarian Stimulation in IVF/ICSI Cycles. Cochrane Database Syst Rev (2017) 5:CD005070. doi: 10.1002/14651858.CD005070.pub3
88. Alviggi C, Conforti A, Esteves SC, Andersen CY, Bosch E, Buhler K, et al. Recombinant Luteinizing Hormone Supplementation in Assisted Reproductive Technology: A Systematic Review. Fertil Steril (2018) 109(4):644–64. doi: 10.1016/j.fertnstert.2018.01.003
89. Lehert P, Kolibianakis EM, Venetis CA, Schertz J, Saunders H, Arriagada P, et al. Recombinant Human Follicle-Stimulating Hormone (r-hFSH) Plus Recombinant Luteinizing Hormone Versus r-hFSH Alone for Ovarian Stimulation During Assisted Reproductive Technology: Systematic Review and Meta-Analysis. Reprod Biol Endocrinol (2014) 12:17. doi: 10.1186/1477-7827-12-17
90. Arvis P, Massin N, Lehert P. Effect of the Supplementation of Recombinant Luteinizing Hormone on Cumulative Live Birth Rate Compared to Follitropin Alfa Alone on Poor Ovarian Responders. A Real-World Large Study. Reprod BioMed (2020) 42(3):546–54. doi: 10.1016/j.rbmo.2020.08.035
91. Buhler KF, Fischer R. Recombinant Human LH Supplementation Versus Supplementation With Urinary Hcg-Based LH Activity During Controlled Ovarian Stimulation in the Long GnRH-agonist Protocol: A Matched Case-Control Study. Gynecol Endocrinol (2012) 28(5):345–50. doi: 10.3109/09513590.2011.633128
92. Revelli A, Pettinau G, Basso G, Carosso A, Ferrero A, Dallan C, et al. Controlled Ovarian Stimulation With recombinant-FSH Plus recombinant-LH vs. Human Menopausal Gonadotropin Based on the Number of Retrieved Oocytes: Results From a Routine Clinical Practice in a Real-Life Population. Reprod Biol Endocrinol (2015) 13:77. doi: 10.1186/s12958-015-0080-6
93. Mennini FS, Marcellusi A, Viti R, Bini C, Carosso A, Revelli A, et al. Probabilistic Cost-Effectiveness Analysis of Controlled Ovarian Stimulation With Recombinant FSH Plus Recombinant LH vs. Human Menopausal Gonadotropin for Women Undergoing IVF. Reprod Biol Endocrinol (2018) 16(1):68. doi: 10.1186/s12958-018-0386-2
94. Pacchiarotti A, Sbracia M, Frega A, Selman H, Rinaldi L, Pacchiarotti A. Urinary hMG (Meropur) Versus Recombinant FSH Plus Recombinant LH (Pergoveris) in IVF: A Multicenter, Prospective, Randomized Controlled Trial. Fertil Steril (2010) 94(6):2467–9. doi: 10.1016/j.fertnstert.2010.04.035
95. Orvieto R. HMG Versus Recombinant FSH Plus Recombinant LH in Ovarian Stimulation for IVF: Does the Source of LH Preparation Matter? Reprod BioMed Online (2019) 39(6):1001–6. doi: 10.1016/j.rbmo.2019.08.010
96. Crowley WF Jr., Filicori M, Spratt DI, Santoro NF. The Physiology of Gonadotropin-Releasing Hormone (GnRH) Secretion in Men and Women. Recent Prog Horm Res (1985) 41:473–531. doi: 10.1016/b978-0-12-571141-8.50015-9
97. Carone D, Caropreso C, Vitti A, Chiappetta R. Efficacy of Different Gonadotropin Combinations to Support Ovulation Induction in WHO Type I Anovulation Infertility: Clinical Evidences of Human Recombinant FSH/human Recombinant LH in a 2:1 Ratio and Highly Purified Human Menopausal Gonadotropin Stimulation Protocols. J Endocrinol Invest (2012) 35(11):996–1002. doi: 10.3275/8657
98. Raju GA, Chavan R, Deenadayal M, Gunasheela D, Gutgutia R, Haripriya G, et al. Luteinizing Hormone and Follicle Stimulating Hormone Synergy: A Review of Role in Controlled Ovarian Hyper-Stimulation. J Hum Reprod Sci (2013) 6(4):227–34. doi: 10.4103/0974-1208.126285
99. Troppmann B, Kleinau G, Krause G, Gromoll J. Structural and Functional Plasticity of the Luteinizing Hormone/Choriogonadotrophin Receptor. Hum Reprod Update (2013) 19(5):583–602. doi: 10.1093/humupd/dmt023
100. Ascoli M, Fanelli F, Segaloff DL. The Lutropin/Choriogonadotropin Receptor, a 2002 Perspective. Endocr Rev (2002) 23(2):141–74. doi: 10.1210/edrv.23.2.0462
101. le Cotonnec JY, Porchet HC, Beltrami V, Munafo A. Clinical Pharmacology of Recombinant Human Luteinizing Hormone: Part I. Pharmacokinetics After Intravenous Administration to Healthy Female Volunteers and Comparison With Urinary Human Luteinizing Hormone. Fertil Steril (1998) 69(2):189–94. doi: 10.1016/s0015-0282(97)00501-3
102. Trinchard-Lugan I, Khan A, Porchet HC, Munafo A. Pharmacokinetics and Pharmacodynamics of Recombinant Human Chorionic Gonadotrophin in Healthy Male and Female Volunteers. Reprod BioMed Online (2002) 4(2):106–15. doi: 10.1016/s1472-6483(10)61927-x
103. Lunenfeld B, Bilger W, Longobardi S, Alam V, D’Hooghe T, Sunkara SK. The Development of Gonadotropins for Clinical Use in the Treatment of Infertility. Front Endocrinol (Lausanne) (2019) 10:429. doi: 10.3389/fendo.2019.00429
104. Casarini L, Lispi M, Longobardi S, Milosa F, La Marca A, Tagliasacchi D, et al. And hCG Action on the Same Receptor Results in Quantitatively and Qualitatively Different Intracellular Signalling. PloS One (2012) 7(10):e46682. doi: 10.1371/journal.pone.0046682
105. Casarini L, Riccetti L, De Pascali F, Gilioli L, Marino M, Vecchi E, et al. Estrogen Modulates Specific Life and Death Signals Induced by LH and hCG in Human Primary Granulosa Cells In Vitro. Int J Mol Sci (2017) 18(5):926. doi: 10.3390/ijms18050926
106. Muller T, Gromoll J, Simoni M. Absence of Exon 10 of the Human Luteinizing Hormone (LH) Receptor Impairs LH, But Not Human Chorionic Gonadotropin Action. J Clin Endocrinol Metab (2003) 88(5):2242–9. doi: 10.1210/jc.2002-021946
107. Grzesik P, Kreuchwig A, Rutz C, Furkert J, Wiesner B, Schuelein R, et al. Differences in Signal Activation by LH and hCG are Mediated by the LH/CG Receptor’s Extracellular Hinge Region. Front Endocrinol (Lausanne) (2015) 6:140. doi: 10.3389/fendo.2015.00140
108. Gatta V, Tatone C, Ciriminna R, Vento M, Franchi S, d’Aurora M, et al. Gene Expression Profiles of Cumulus Cells Obtained From Women Treated With Recombinant Human Luteinizing Hormone + Recombinant Human Follicle-Stimulating Hormone or Highly Purified Human Menopausal Gonadotropin Versus Recombinant Human Follicle-Stimulating Hormone Alone. Fertil Steril (2013) 99(7):2000–8 e1. doi: 10.1016/j.fertnstert.2013.01.150
109. Casarini L, Riccetti L, De Pascali F, Nicoli A, Tagliavini S, Trenti T, et al. Follicle-Stimulating Hormone Potentiates the Steroidogenic Activity of Chorionic Gonadotropin and the Anti-Apoptotic Activity of Luteinizing Hormone in Human Granulosa-Lutein Cells In Vitro. Mol Cell Endocrinol (2016) 422:103–14. doi: 10.1016/j.mce.2015.12.008
110. Gupta C, Chapekar T, Chhabra Y, Singh P, Sinha S, Luthra K. Differential Response to Sustained Stimulation by hCG & LH on Goat Ovarian Granulosa Cells. Indian J Med Res (2012) 135:331–40.
111. Edwards RG, Lobo R, Bouchard P. Time to Revolutionize Ovarian Stimulation. Hum Reprod (Oxford England) (1996) 11(5):917–9. doi: 10.1093/oxfordjournals.humrep.a019317
112. Huirne JA, Homburg R, Lambalk CB. Are GnRH Antagonists Comparable to Agonists for Use in IVF? Hum Reprod (Oxford England) (2007) 22(11):2805–13. doi: 10.1093/humrep/dem270
113. Al-Inany HG, Youssef MA, Aboulghar M, Broekmans F, Sterrenburg M, Smit J, et al. Gonadotrophin-Releasing Hormone Antagonists for Assisted Reproductive Technology. Cochrane Database Syst Rev (2011) 5:CD001750. doi: 10.1002/14651858.CD001750.pub3
114. Lambalk CB, Banga FR, Huirne JA, Toftager M, Pinborg A, Homburg R, et al. GnRH Antagonist Versus Long Agonist Protocols in IVF: A Systematic Review and Meta-Analysis Accounting for Patient Type. Hum Reprod Update (2017) 23(5):560–79. doi: 10.1093/humupd/dmx017
115. Al-Inany HG, Youssef MA, Ayeleke RO, Brown J, Lam WS, Broekmans FJ. Gonadotrophin-Releasing Hormone Antagonists for Assisted Reproductive Technology. Cochrane Database Syst Rev (2016) 4:CD001750. doi: 10.1002/14651858.CD001750.pub4
116. Kolibianakis EM, Collins J, Tarlatzis BC, Devroey P, Diedrich K, Griesinger G. Among Patients Treated for IVF With Gonadotrophins and GnRH Analogues, is the Probability of Live Birth Dependent on the Type of Analogue Used? A Systematic Review and Meta-Analysis. Hum Reprod Update (2006) 12(6):651–71. doi: 10.1093/humupd/dml038
117. Cheung LP, Lam PM, Lok IH, Chiu TT, Yeung SY, Tjer CC, et al. GnRH Antagonist Versus Long GnRH Agonist Protocol in Poor Responders Undergoing IVF: A Randomized Controlled Trial. Hum Reprod (Oxford England) (2005) 20(3):616–21. doi: 10.1093/humrep/deh668
118. Gordon K, Hodgen GD. GnRH Agonists and Antagonists in Assisted Reproduction. Baillieres Clin Obstet Gynaecol (1992) 6(2):247–65. doi: 10.1016/s0950-3552(05)80085-9
119. Orvieto R, Patrizio P. GnRH Agonist Versus GnRH Antagonist in Ovarian Stimulation: An Ongoing Debate. Reprod BioMed Online (2013) 26(1):4–8. doi: 10.1016/j.rbmo.2012.11.001
120. Al-Inany H, Aboulghar M. Gonadotrophin-Releasing Hormone Antagonists for Assisted Conception. Cochrane Database Syst Rev (2001) 4):CD001750. doi: 10.1002/14651858.CD001750
121. Ludwig M, Katalinic A, Diedrich K. Use of GnRH Antagonists in Ovarian Stimulation for Assisted Reproductive Technologies Compared to the Long Protocol. Meta-Analysis. Arch Gynecol Obstet (2001) 265(4):175–82. doi: 10.1007/s00404-001-0267-2
122. Griesinger G, Felberbaum R, Diedrich K. GnRH Antagonists in Ovarian Stimulation: A Treatment Regimen of Clinicians’ Second Choice? Data From the German National IVF Registry. Hum Reprod (2005) 20(9):2373–5. doi: 10.1093/humrep/dei086
123. Fauser BC, Devroey P. Why is the Clinical Acceptance of Gonadotropin-Releasing Hormone Antagonist Cotreatment During Ovarian Hyperstimulation for In Vitro Fertilization So Slow? Fertil Steril (2005) 83(6):1607–11. doi: 10.1016/j.fertnstert.2005.02.011
124. Kolibianakis EM, Griesinger G, Venetis CA. GnRH Antagonists vs. Long GnRH Agonists in IVF: Significant Flaws in a Meta-Analysis Lead to Invalid Conclusions. Hum Reprod Update (2018) 24(2):242–3. doi: 10.1093/humupd/dmx037
125. Balasch J, Miro F, Burzaco I, Casamitjana R, Civico S, Ballesca JL, et al. The Role of Luteinizing Hormone in Human Follicle Development and Oocyte Fertility: Evidence From in-Vitro Fertilization in a Woman With Long-Standing Hypogonadotrophic Hypogonadism and Using Recombinant Human Follicle Stimulating Hormone. Hum Reprod (Oxford England) (1995) 10(7):1678–83. doi: 10.1093/oxfordjournals.humrep.a136154
126. Gizzo S, Andrisani A, Noventa M, Manfe S, Oliva A, Gangemi M, et al. Recombinant LH Supplementation During IVF Cycles With a GnRH-antagonist in Estimated Poor Responders: A Cross-Matched Pilot Investigation of the Optimal Daily Dose and Timing. Mol Med Rep (2015) 12(3):4219–29. doi: 10.3892/mmr.2015.3904
127. Huirne JA, van Loenen AC, Schats R, McDonnell J, Hompes PG, Schoemaker J, et al. Dose-Finding Study of Daily GnRH Antagonist for the Prevention of Premature LH Surges in IVF/ICSI Patients: Optimal Changes in LH and Progesterone for Clinical Pregnancy. Hum Reprod (Oxford England) (2005) 20(2):359–67. doi: 10.1093/humrep/deh601
128. Vuong TN, Phung HT, Ho MT. Recombinant Follicle-Stimulating Hormone and Recombinant Luteinizing Hormone Versus Recombinant Follicle-Stimulating Hormone Alone During GnRH Antagonist Ovarian Stimulation in Patients Aged >/=35 Years: A Randomized Controlled Trial. Hum Reprod (Oxford England) (2015) 30(5):1188–95. doi: 10.1093/humrep/dev038
129. Younis JS, Laufer N. Recombinant Luteinizing Hormone Supplementation to Recombinant Follicle Stimulating Hormone Therapy in Gonadotropin Releasing Hormone Analogue Cycles: What is the Evidence? Curr Med Res Opin (2018) 34(5):881–6. doi: 10.1080/03007995.2017.1417827
130. Janssens RM, Brus L, Cahill DJ, Huirne JA, Schoemaker J, Lambalk CB. Direct Ovarian Effects and Safety Aspects of GnRH Agonists and Antagonists. Hum Reprod Update (2000) 6(5):505–18. doi: 10.1093/humupd/6.5.505
131. Westergaard LG, Erb K, Laursen SB, Rex S, Rasmussen PE. Human Menopausal Gonadotropin Versus Recombinant Follicle-Stimulating Hormone in Normogonadotropic Women Down-Regulated With a Gonadotropin-Releasing Hormone Agonist Who Were Undergoing In Vitro Fertilization and Intracytoplasmic Sperm Injection: A Prospective Randomized Study. Fertil Steril (2001) 76(3):543–9. doi: 10.1016/s0015-0282(01)01973-2
132. Huirne JA, van Loenen AC, Schats R, McDonnell J, Hompes PG, Schoemaker J, et al. Dose-Finding Study of Daily Gonadotropin-Releasing Hormone (GnRH) Antagonist for the Prevention of Premature Luteinizing Hormone Surges in IVF/ICSI Patients: Antide and Hormone Levels. Hum Reprod (Oxford England) (2004) 19(10):2206–15. doi: 10.1093/humrep/deh357
133. Oberye JJ, Mannaerts BM, Huisman JA, Timmer CJ. Pharmacokinetic and Pharmacodynamic Characteristics of Ganirelix (Antagon/Orgalutran). Part Ii. Dose-proportionality and Gonadotropin Suppression After Multiple Doses of Ganirelix in Healthy Female Volunteers. Fertil Steril (1999) 72(6):1006–12. doi: 10.1016/s0015-0282(99)00414-8
134. The Ganirelix Dose-Finding Study Group. A Double-Blind, Randomized, Dose-Finding Study to Assess the Efficacy of the Gonadotrophin-Releasing Hormone Antagonist Ganirelix (Org 37462) to Prevent Premature Luteinizing Hormone Surges in Women Undergoing Ovarian Stimulation With Recombinant Follicle Stimulating Hormone (Puregon). The Ganirelix Dose-Finding Study Group. Hum Reprod (Oxford England) (1998) 13(11):3023–31. doi: 10.1093/humrep/13.11.3023
135. Kol S. Individualized Treatment From Theory to Practice: The Private Case of Adding LH During GnRH Antagonist-Based Stimulation Protocol. Clin Med Insights Reprod Health (2014) 8:59–64. doi: 10.4137/CMRH.S17788
136. Kol S. LH Supplementation in Ovarian Stimulation for IVF: The Individual, LH Deficient, Patient Perspective. Gynecol Obstet Invest (2020) 85:307–11. doi: 10.1159/000509162
137. Shoham Z. The Clinical Therapeutic Window for Luteinizing Hormone in Controlled Ovarian Stimulation. Fertil Steril (2002) 77(6):1170–7. doi: 10.1016/s0015-0282(02)03157-6
138. Kol S. To Add or Not to Add LH: Consideration of LH Concentration Changes in Individual Patients. Reprod BioMed Online (2005) 11(6):664–6. doi: 10.1016/s1472-6483(10)61680-x
139. Kol S, Homburg R. Change, Change, Change: Hormonal Actions Depend on Changes in Blood Levels. Hum Reprod (Oxford England) (2008) 23(5):1004–6. doi: 10.1093/humrep/den061
140. Castillo JC, Humaidan P, Bernabeu R. Pharmaceutical Options for Triggering of Final Oocyte Maturation in ART. BioMed Res Int (2014) 2014:580171. doi: 10.1155/2014/580171
141. Humaidan P, Alsbjerg B. GnRHa Trigger for Final Oocyte Maturation: Is HCG Trigger History? Reprod BioMed Online (2014) 29(3):274–80. doi: 10.1016/j.rbmo.2014.05.008
142. Nakano R, Mizuno T, Kotsuji F, Katayama K, Wshio M, Tojo S. “Triggering” of Ovulation After Infusion of Synthetic Luteinizing Hormone Releasing Factor (LRF). Acta Obstet Gynecol Scand (1973) 52(3):269–72. doi: 10.3109/00016347309158325
143. Kol S. Luteolysis Induced by a Gonadotropin-Releasing Hormone Agonist is the Key to Prevention of Ovarian Hyperstimulation Syndrome. Fertil Steril (2004) 81(1):1–5. doi: 10.1016/j.fertnstert.2003.05.032
144. Youssef MA, Van der Veen F, Al-Inany HG, Mochtar MH, Griesinger G, Nagi Mohesen M, et al. Gonadotropin-Releasing Hormone Agonist Versus HCG for Oocyte Triggering in Antagonist-Assisted Reproductive Technology. Cochrane Database Syst Rev (2014) 10):CD008046. doi: 10.1002/14651858.CD008046.pub4
145. Youssef MA, Abou-Setta AM, Lam WS. Recombinant Versus Urinary Human Chorionic Gonadotrophin for Final Oocyte Maturation Triggering in IVF and ICSI Cycles. Cochrane Database Syst Rev (2016) 4:CD003719. doi: 10.1002/14651858.CD003719.pub4
146. Mackens S, Santos-Ribeiro S, van de Vijver A, Racca A, Van Landuyt L, Tournaye H, et al. Frozen Embryo Transfer: A Review on the Optimal Endometrial Preparation and Timing. Hum Reprod (Oxford England) (2017) 32(11):2234–42. doi: 10.1093/humrep/dex285
147. Ghobara T, Gelbaya TA, Ayeleke RO. Cycle Regimens for Frozen-Thawed Embryo Transfer. Cochrane Database Syst Rev (2017) 7:CD003414. doi: 10.1002/14651858.CD003414.pub3
148. Yarali H, Polat M, Mumusoglu S, Yarali I, Bozdag G. Preparation of Endometrium for Frozen Embryo Replacement Cycles: A Systematic Review and Meta-Analysis. J Assist Reprod Genet (2016) 33(10):1287–304. doi: 10.1007/s10815-016-0787-0
149. Kalem Z, Namli Kalem M, Bakirarar B, Kent E, Gurgan T. Natural Cycle Versus Hormone Replacement Therapy Cycle in Frozen-Thawed Embryo Transfer. Saudi Med J (2018) 39(11):1102–8. doi: 10.15537/smj.2018.11.23299
150. Orvieto R, Feldman N, Lantsberg D, Manela D, Zilberberg E, Haas J. Natural Cycle Frozen-Thawed Embryo Transfer-can We Improve Cycle Outcome? J Assist Reprod Genet (2016) 33(5):611–5. doi: 10.1007/s10815-016-0685-5
151. Weissman A, Horowitz E, Ravhon A, Steinfeld Z, Mutzafi R, Golan A, et al. Spontaneous Ovulation Versus HCG Triggering for Timing Natural-Cycle Frozen-Thawed Embryo Transfer: A Randomized Study. Reprod BioMed Online (2011) 23(4):484–9. doi: 10.1016/j.rbmo.2011.06.004
152. Fatemi HM, Kyrou D, Bourgain C, Van den Abbeel E, Griesinger G, Devroey P. Cryopreserved-Thawed Human Embryo Transfer: Spontaneous Natural Cycle is Superior to Human Chorionic Gonadotropin-Induced Natural Cycle. Fertil Steril (2010) 94(6):2054–8. doi: 10.1016/j.fertnstert.2009.11.036
153. Montagut M, Santos-Ribeiro S, De Vos M, Polyzos NP, Drakopoulos P, Mackens S, et al. Frozen-Thawed Embryo Transfers in Natural Cycles With Spontaneous or Induced Ovulation: The Search for the Best Protocol Continues. Hum Reprod (Oxford England) (2016) 31(12):2803–10. doi: 10.1093/humrep/dew263
154. Devroey P, Polyzos NP, Blockeel C. An OHSS-Free Clinic by Segmentation of IVF Treatment. Hum Reprod (Oxford England) (2011) 26(10):2593–7. doi: 10.1093/humrep/der251
155. Orvieto R. Can We Eliminate Severe Ovarian Hyperstimulation Syndrome? Hum Reprod (Oxford England) (2005) 20(2):320–2. doi: 10.1093/humrep/deh613
156. Griesinger G, Diedrich K, Devroey P, Kolibianakis EM. GnRH Agonist for Triggering Final Oocyte Maturation in the GnRH Antagonist Ovarian Hyperstimulation Protocol: A Systematic Review and Meta-Analysis. Hum Reprod Update (2006) 12(2):159–68. doi: 10.1093/humupd/dmi045
157. Orvieto R, Rabinson J, Meltzer S, Zohav E, Anteby E, Homburg R. Substituting HCG With GnRH Agonist to Trigger Final Follicular Maturation–a Retrospective Comparison of Three Different Ovarian Stimulation Protocols. Reprod BioMed Online (2006) 13(2):198–201. doi: 10.1016/s1472-6483(10)60615-3
158. Chen CH, Tzeng CR, Wang PH, Liu WM, Chang HY, Chen HH, et al. Dual Triggering With GnRH Agonist Plus hCG Versus Triggering With hCG Alone for IVF/ICSI Outcome in GnRH Antagonist Cycles: A Systematic Review and Meta-Analysis. Arch Gynecol Obstet (2018) 298(1):17–26. doi: 10.1007/s00404-018-4751-3
159. Eftekhar M, Mojtahedi MF, Miraj S, Omid M. Final Follicular Maturation by Administration of GnRH Agonist Plus HCG Versus HCG in Normal Responders in ART Cycles: An Rct. Int J Reprod BioMed (Yazd) (2017) 15(7):429–34. doi: 10.29252/ijrm.15.7.429
160. Haas J, Kedem A, Machtinger R, Dar S, Hourvitz A, Yerushalmi G, et al. HCG (1500IU) Administration on Day 3 After Oocytes Retrieval, Following GnRH-agonist Trigger for Final Follicular Maturation, Results in High Sufficient Mid Luteal Progesterone Levels - a Proof of Concept. J Ovarian Res (2014) 7:35. doi: 10.1186/1757-2215-7-35
161. Orvieto R. Triggering Final Follicular Maturation–Hcg, GnRH-agonist or Both, When and to Whom? J Ovarian Res (2015) 8:60. doi: 10.1186/s13048-015-0187-6
162. Zilberberg E, Haas J, Dar S, Kedem A, Machtinger R, Orvieto R. Co-Administration of GnRH-agonist and hCG, for Final Oocyte Maturation (Double Trigger), in Patients With Low Proportion of Mature Oocytes. Gynecol Endocrinol (2015) 31(2):145–7. doi: 10.3109/09513590.2014.978850
163. Haas J, Bassil R, Samara N, Zilberberg E, Mehta C, Orvieto R, et al. GnRH Agonist and hCG (Dual Trigger) Versus hCG Trigger for Final Follicular Maturation: A Double-Blinded, Randomized Controlled Study. Hum Reprod (Oxford England) (2020) 35(7):1648–54. doi: 10.1093/humrep/deaa107
164. Ding N, Liu X, Jian Q, Liang Z, Wang F. Dual Trigger of Final Oocyte Maturation With a Combination of GnRH Agonist and hCG Versus a hCG Alone Trigger in GnRH Antagonist Cycle for In Vitro Fertilization: A Systematic Review and Meta-Analysis. Eur J Obstet Gynecol Reprod Biol (2017) 218:92–8. doi: 10.1016/j.ejogrb.2017.09.004
165. Engmann L, DiLuigi A, Schmidt D, Nulsen J, Maier D, Benadiva C. The Use of Gonadotropin-Releasing Hormone (GnRH) Agonist to Induce Oocyte Maturation After Cotreatment With GnRH Antagonist in High-Risk Patients Undergoing In Vitro Fertilization Prevents the Risk of Ovarian Hyperstimulation Syndrome: A Prospective Randomized Controlled Study. Fertil Steril (2008) 89(1):84–91. doi: 10.1016/j.fertnstert.2007.02.002
166. Tannus S, Turki R, Cohen Y, Son WY, Shavit T, Dahan MH. Reproductive Outcomes After a Single Dose of Gonadotropin-Releasing Hormone Agonist Compared With Human Chorionic Gonadotropin for the Induction of Final Oocyte Maturation in Hyper-Responder Women Aged 35-40 Years. Fertil Steril (2017) 107(6):1323–8 e2. doi: 10.1016/j.fertnstert.2017.04.014
167. Young SL. Oestrogen and Progesterone Action on Endometrium: A Translational Approach to Understanding Endometrial Receptivity. Reprod BioMed Online (2013) 27(5):497–505. doi: 10.1016/j.rbmo.2013.06.010
168. Rosenberg SM, Luciano AA, Riddick DH. The Luteal Phase Defect: The Relative Frequency of, and Encouraging Response to, Treatment With Vaginal Progesterone. Fertil Steril (1980) 34(1):17–20. doi: 10.1016/s0015-0282(16)44831-4
169. van der Linden M, Buckingham K, Farquhar C, Kremer JA, Metwally M. Luteal Phase Support for Assisted Reproduction Cycles. Cochrane Database Syst Rev (2015) 7):CD009154. doi: 10.1002/14651858.CD009154.pub3
170. Green KA, Zolton JR, Schermerhorn SM, Lewis TD, Healy MW, Terry N, et al. Progesterone Luteal Support After Ovulation Induction and Intrauterine Insemination: An Updated Systematic Review and Meta-Analysis. Fertil Steril (2017) 107(4):924–33.e5. doi: 10.1016/j.fertnstert.2017.01.011
171. Mesen TB, Young SL. Progesterone and the Luteal Phase: A Requisite to Reproduction. Obstet Gynecol Clin North Am (2015) 42(1):135–51. doi: 10.1016/j.ogc.2014.10.003
172. Gao J, Gu F, Miao BY, Chen MH, Zhou CQ, Xu YW. Effect of the Initiation of Progesterone Supplementation in In Vitro Fertilization-Embryo Transfer Outcomes: A Prospective Randomized Controlled Trial. Fertil Steril (2018) 109(1):97–103. doi: 10.1016/j.fertnstert.2017.09.033
173. Khrouf M, Slimani S, Khrouf MR, Braham M, Bouyahia M, Berjeb KK, et al. Progesterone for Luteal Phase Support in In Vitro Fertilization: Comparison of Vaginal and Rectal Pessaries to Vaginal Capsules: A Randomized Controlled Study. Clin Med Insights Womens Health (2016) 9:43–7. doi: 10.4137/CMWH.S32156
174. Tesarik J, Mendoza-Tesarik R, Mendoza N. Gonadotropin-Releasing Hormone Agonist for Luteal Phase Support: The Origin of the Concept, Current Experience, Mechanism of Action and Future Perspectives. Fertil Steril (2016) 106(2):268–9. doi: 10.1016/j.fertnstert.2016.04.034
175. Tesarik J, Hazout A, Mendoza C. Enhancement of Embryo Developmental Potential by a Single Administration of GnRH Agonist At the Time of Implantation. Hum Reprod (Oxford England) (2004) 19(5):1176–80. doi: 10.1093/humrep/deh235
176. Tesarik J, Hazout A, Mendoza-Tesarik R, Mendoza N, Mendoza C. Beneficial Effect of Luteal-Phase GnRH Agonist Administration on Embryo Implantation After ICSI in Both GnRH Agonist- and Antagonist-Treated Ovarian Stimulation Cycles. Hum Reprod (Oxford England) (2006) 21(10):2572–9. doi: 10.1093/humrep/del173
177. Mendoza-Tesarik R, Mendoza N, Lopez CC, Tesarik J. GnRH Agonist Treatment of Luteal Phase Deficiency in HCG-triggered IVF Cycles: A Matched Case-Control Study. Reprod BioMed Online (2019) 39(2):225–30. doi: 10.1016/j.rbmo.2019.03.215
178. Seikkula J, Anttila L, Polo-Kantola P, Bloigu R, Engblom J, Tinkanen H, et al. Effect of Mid-Luteal Phase GnRH Agonist on Frozen-Thawed Embryo Transfers During Natural Menstrual Cycles: A Randomised Clinical Pilot Study. Gynecol Endocrinol (2016) 32(12):961–4. doi: 10.1080/09513590.2016.1196176
179. Yildiz GA, Sukur YE, Ates C, Aytac R. The Addition of Gonadotrophin Releasing Hormone Agonist to Routine Luteal Phase Support in Intracytoplasmic Sperm Injection and Embryo Transfer Cycles: A Randomized Clinical Trial. Eur J Obstet Gynecol Reprod Biol (2014) 182:66–70. doi: 10.1016/j.ejogrb.2014.08.026
180. Humaidan P, Ejdrup Bredkjaer H, Westergaard LG, Yding Andersen C. 1,500 IU Human Chorionic Gonadotropin Administered At Oocyte Retrieval Rescues the Luteal Phase When Gonadotropin-Releasing Hormone Agonist is Used for Ovulation Induction: A Prospective, Randomized, Controlled Study. Fertil Steril (2010) 93(3):847–54. doi: 10.1016/j.fertnstert.2008.12.042
181. Kol S, Humaidan P. GnRH Agonist Triggering: Recent Developments. Reprod BioMed Online (2013) 26(3):226–30. doi: 10.1016/j.rbmo.2012.11.002
182. Ioannidou PG, Bosdou JK, Lainas GT, Lainas TG, Grimbizis GF, Kolibianakis EM. How Frequent is Severe Ovarian Hyperstimulation Syndrome After GnRH Agonist Triggering in High-Risk Women?A Systematic Review and Meta-Analysis. Reprod BioMed Online (2021) 42(3):635–50. doi: 10.1016/j.rbmo.2020.11.008
183. Seyhan A, Ata B, Polat M, Son WY, Yarali H, Dahan MH. Severe Early Ovarian Hyperstimulation Syndrome Following GnRH Agonist Trigger With the Addition of 1500 IU Hcg. Hum Reprod (Oxford England) (2013) 28(9):2522–8. doi: 10.1093/humrep/det124
184. Haahr T, Roque M, Esteves SC, Humaidan P. Gnrh Agonist Trigger and LH Activity Luteal Phase Support Versus Hcg Trigger and Conventional Luteal Phase Support in Fresh Embryo Transfer Ivf/Icsi Cycles-A Systematic PRISMA Review and Meta-Analysis. Front Endocrinol (Lausanne) (2017) 8:116. doi: 10.3389/fendo.2017.00116
185. Vermeulen N, Le Clef N, D'Angelo A, Tilleman K, Veleva Z, Nelen WL. Manual for ESHRE guideline development. Available at: www.eshre.eu.
Keywords: assisted reproductive technology (ART), optimisation, ovarian stimulation, gonadotropins, luteal phase support, oocyte maturation, trigger, expert opinion
Citation: Orvieto R, Venetis CA, Fatemi HM, D’Hooghe T, Fischer R, Koloda Y, Horton M, Grynberg M, Longobardi S, Esteves SC, Sunkara SK, Li Y and Alviggi C (2021) Optimising Follicular Development, Pituitary Suppression, Triggering and Luteal Phase Support During Assisted Reproductive Technology: A Delphi Consensus. Front. Endocrinol. 12:675670. doi: 10.3389/fendo.2021.675670
Received: 03 March 2021; Accepted: 08 April 2021;
Published: 10 May 2021.
Edited by:
Hakan Yarali, Anatolia IVF Center, TurkeyReviewed by:
Annalisa Racca, University Hospital Brussels, BelgiumMurat Sönmezer, Ankara University, Turkey
Copyright © 2021 Orvieto, Venetis, Fatemi, D’Hooghe, Fischer, Koloda, Horton, Grynberg, Longobardi, Esteves, Sunkara, Li and Alviggi. This is an open-access article distributed under the terms of the Creative Commons Attribution License (CC BY). The use, distribution or reproduction in other forums is permitted, provided the original author(s) and the copyright owner(s) are credited and that the original publication in this journal is cited, in accordance with accepted academic practice. No use, distribution or reproduction is permitted which does not comply with these terms.
*Correspondence: Raoul Orvieto, cmFvdWwub3J2aWV0b0BzaGViYS5oZWFsdGguZ292Lmls