- Department of Anatomy and Physiology, University of Melbourne, Melbourne, VIC, Australia
Osteoarthritis pain is often thought of as a pain driven by nerves that innervate the soft tissues of the joint, but there is emerging evidence for a role for nerves that innervate the underlying bone. In this mini review we cite evidence that subchondral bone lesions are associated with pain in osteoarthritis. We explore recent studies that provide evidence that sensory neurons that innervate bone are nociceptors that signal pain and can be sensitized in osteoarthritis. Finally, we describe neuronal remodeling of sensory and sympathetic nerves in bone and discuss how these processes can contribute to osteoarthritis pain.
Introduction
Osteoarthritis (OA) is a progressive degenerative disease of the articular cartilage that impacts on surrounding synovial tissues and bone, and is characterized by swelling, stiffness, and pain. Pain is the most debilitating aspect of OA, and both the onset and severity of pain are key factors that lead those who suffer OA to seek medical advice (1). While there is a justified focus on finding disease-modifying treatments that stop or slow the progression of OA, we can also make significant improvements to quality of life and economic burden by treating the underlying pain.
Cartilage is aneural, but the soft tissues and the subchondral bone around joints are richly innervated by sensory nerve endings that respond to noxious stimuli, can be sensitized by inflammation, and are relevant to the pathophysiology of OA pain (2–26). However, because OA has in the past mostly been considered a joint disease, and because the innervation of soft tissues of the joint are easily accessible in animal models, there has been significant focus on the role of the nerves that specifically innervate the articular tissues of the joint. There have already been a number of excellent reviews that summarize this literature (25, 27–31). In this review, we will instead provide a contemporary overview of the pathogenesis of pain in OA, with a particular focus on the role of the sensory innervation of the subchondral bone, which is likely to be important particularly in late-stage disease when there is significant damage to the bone surrounding osteoarthritic joints.
Role of the innervation of synovial tissues and joint capsule in and around the joint
There have already been a number of excellent reviews that summarize the role of the articular tissue in the literature (25, 27–31). Articular nociceptors innervate the joint capsule and synovium, respond to noxious mechanical stimulation and known algesic substances applied to the joint, and can be sensitized by inflammatory mediators released in OA (3–8, 11–13). In studies of humans with OA, inflammatory mediators released in the inflamed synovium provoke pain, and there are a number of studies that report an association between knee pain and synovitis (32–34). However, while it is clear from radiographic evidence that joint damage predisposes to pain, there is little relation between the severity of joint damage and the pain experienced (35–38). In a study of 58 patients with OA and 33 pain-free controls, there was no association between the extent of synovitis and the degree of pain (39). These findings suggest that the activation of articular nociceptors as a result of joint damage and/or inflammation may not be the main contributor to the severe pain experienced by patients with OA.
Clinical evidence for the involvement of the subchondral bone in the pathogenesis of OA pain
There is a growing body of evidence for the involvement of the subchondral bone in the pathogenesis of OA and OA pain (29, 40, 41). When the articular cartilage breaks down with the progression of OA, subchondral and synovial compartments become increasingly continuous, and histopathological changes in the subchondral bone (including microdamage, bone marrow lesions, and bone cysts) emerge (42, 43). These histopathological changes occur during the most debilitating stage of OA when pain is poorly controlled. In a cross-sectional observational study of 401 patients with knee OA, 351 patients reported painful knees and 50 patients reported no pain. Subchondral bone lesions were noted in 77.5% of those that reported knee pain, but in only 30% of those without knee pain (44). Importantly, the size of the bone lesion was independently associated with pain (44). This association was also observed in another cross-sectional study of over 1400 patients, where it was reported that the size of bone marrow lesions correlated specifically with weight-bearing pain (45). In advanced OA, severe pain may also be a result of raised intraosseous pressure in the bones around joints (46–51). In some of these cases, the increase in pressure has been associated with pain which can be relieved by fenestration, suggesting that increased pressure in the marrow cavity produces pain.
Interestingly, several clinical studies have tested whether bisphosphonates, a group of drugs that slow bone loss by reducing osteoclast activity, can reduce bone marrow lesion size and also OA pain. A randomized controlled trial of 60 patients with OA found that patients given four intravenous doses of neridronate had reduced bone marrow lesion size and reported less pain (52). A similar result was seen in a clinical trial of 59 patients given a different bisphosphonate, zoledronic acid (53). Patients with knee OA displayed reduced bone marrow lesion size and reduced pain at 6 months after a single infusion with zoledronic acid compared to placebo (53).
Together, these findings highlight a clear link between damage to the subchondral bone and pain. This role appears to be particularly important in late-stage OA, when there is cartilage breakdown, and provides evidence that changes to the function of nerves that innervate the subchondral bone may drive pain during this late stage of disease.
Sensory innervation of subchondral bone
There is a long history of work documenting the innervation of the bone (9, 54–61). Nerves can be found entering the bone through the nutrient foramina, Haversian canals, the osteochondral (OC) junction, and at the attachments of the synovial membrane (23, 62–65). They branch extensively in the periosteum, bone marrow, and subchondral bone associated with synovial joints, where they end as unencapsulated, free fiber nerve endings (23, 60, 66). The bone is a highly vascularized structure, and most nerves found within the bone run with the vasculature (63, 65, 67), so many authors suggested they had a vasculature function but did not comment further. The advent of retrograde tracing in combination with immunohistochemistry has provided clear evidence that nerves within the bone are of both sensory and autonomic origin, and those that are sensory predominantly function as nociceptors (68, 69).
Pain is transmitted by two main classes of peripheral nociceptors (70). Small-diameter myelinated sensory neurons, known as Aδ nociceptors, transmit fast, intense pain, while small-diameter unmyelinated sensory neurons, known as C nociceptors, encode slow, aching pain. In the dorsal root ganglia (DRG), the soma of peripheral sensory neurons that innervate the medullary cavity, trabecular bone, and the periosteum are almost exclusively small-diameter myelinated (neurofilament rich) or unmyelinated (neurofilament poor) neurons (10, 15–17, 19, 21). They express markers characteristic of nociceptive neurons, with rodent studies finding that approximately half express calcitonin gene-related peptide (CGRP), two thirds express tyrosine receptor kinase A (TrkA), a quarter express Substance P, almost half express transient receptor potential vanilloid 1 (TRPV1), and some bind isolectin B4 (10, 16, 17, 20, 68, 71, 72). Both peptidergic and non-peptidergic bone-projecting nociceptors have been identified in DRG on the basis of various combinations of these markers (10, 72), and these molecular phenotypes are likely maintained in their peripheral nerve terminals in the bone (23, 66, 73, 74). Thus, it is clear that sensory neurons that innervate the bone have a morphology and molecular phenotype consistent with a role in nociception.
Until recently, there were few published studies recording the response of peripheral sensory neurons to noxious stimulation of the bone marrow (75, 76). These studies used anesthetized dogs to make whole-nerve recordings from branches of a nerve that innervates the tibial marrow cavity. Application of noxious mechanical and chemical stimulation to the marrow cavity evoked an increase in whole-nerve activity, but no attempts were made to characterize the response of individual bone marrow nociceptors to different types of noxious stimuli. More recently, an in vivo bone–nerve preparation was developed to record the activity of nerves that innervate the tibial marrow cavity of rats (18, 20). Electrophysiological recordings were made from a small branch of the tibial nerve, proximal to its entry into the tibia, in response to increasing intra-osseous pressure (to provide noxious mechanical stimulation), or through the application of noxious chemical stimuli, directly to the marrow cavity. Single bone nociceptors were isolated from the whole-nerve recordings with spike discrimination software providing unprecedented insight into the function of single sensory neurons that innervate the bone. This approach has been used to show that single sensory neurons that innervate the bone have conduction velocities consistent with Aδ and C nociceptor classifications, can be activated and/or sensitized by inflammatory mediators and known algesic substances directly applied to bone, and can respond to noxious increases in intraosseous pressure (16–22). Importantly, many of the inflammatory agents used in these electrophysiological recording studies also produced altered pain behavior when applied within the bone, providing a clear link between the altered function of sensory neurons that innervate the bone and pain (20–22). These physiological data provide strong evidence that sensory neurons found in bones have a role in nociception.
Activation and sensitization of bone nociceptors in OA
Whilst there have been many high-quality studies that have explored mechanisms related to how OA affects the function of articular afferent neurons (13, 28, 77–80), there has been only a single report of how OA specifically affects those that innervate the surrounding bone (81). The latter study applied the in vivo bone–nerve preparation described above to make electrophysiological recordings of the nociceptors that innervate the subchondral bone (by recording the nerve to the rat tibia), or the articular tissues around the knee (by recording from the medial articular nerve), in a rat model of monoiodoacetate (MIA)-induced OA. MIA-induced OA is a robust and reliable animal model of OA that results in rapid changes in weight-bearing within a day post-injection, and which continue beyond 28 days (81–83). It is characterized by an early inflammatory response in the joint that is obvious in the first week (early-stage OA), and significant cartilage breakdown that predisposes to the inflammation and damage of the subchondral bone later in disease progression (late-stage OA) (81, 82). The authors showed that there were significant changes in the function of knee joint nociceptors, but not bone nociceptors, early in the progression of OA (day 3 post-MIA injection), when there was histological evidence of inflammation in the joint capsule, but no damage to either the articular cartilage or surrounding subchondral bone. The changes in articular nerve function noted in early MIA-induced OA included increased spontaneous activity, decreased thresholds for mechanical activation, and increased discharge frequencies in response to mechanical stimuli compared to animals without OA (81). Changes in the function of bone nociceptors occurred later in the progression of OA, when there was histological evidence of damage to the articular cartilage and surrounding subchondral bone. The changes in bone–nerve function noted at this later stage of MIA-induced OA included decreased thresholds for mechanical activation of Aδ bone nociceptors, and increased discharge frequencies for both Aδ and C bone nociceptors during mechanical stimulation. These data are the first to show that the progression of OA pathology from the joint into the subchondral bone is accompanied by functional changes to the nociceptors innervating the subchondral bone, and could account for the increased pain in OA patients later in the disease when there is pathology in the bone surrounding the joint (Figure 1).
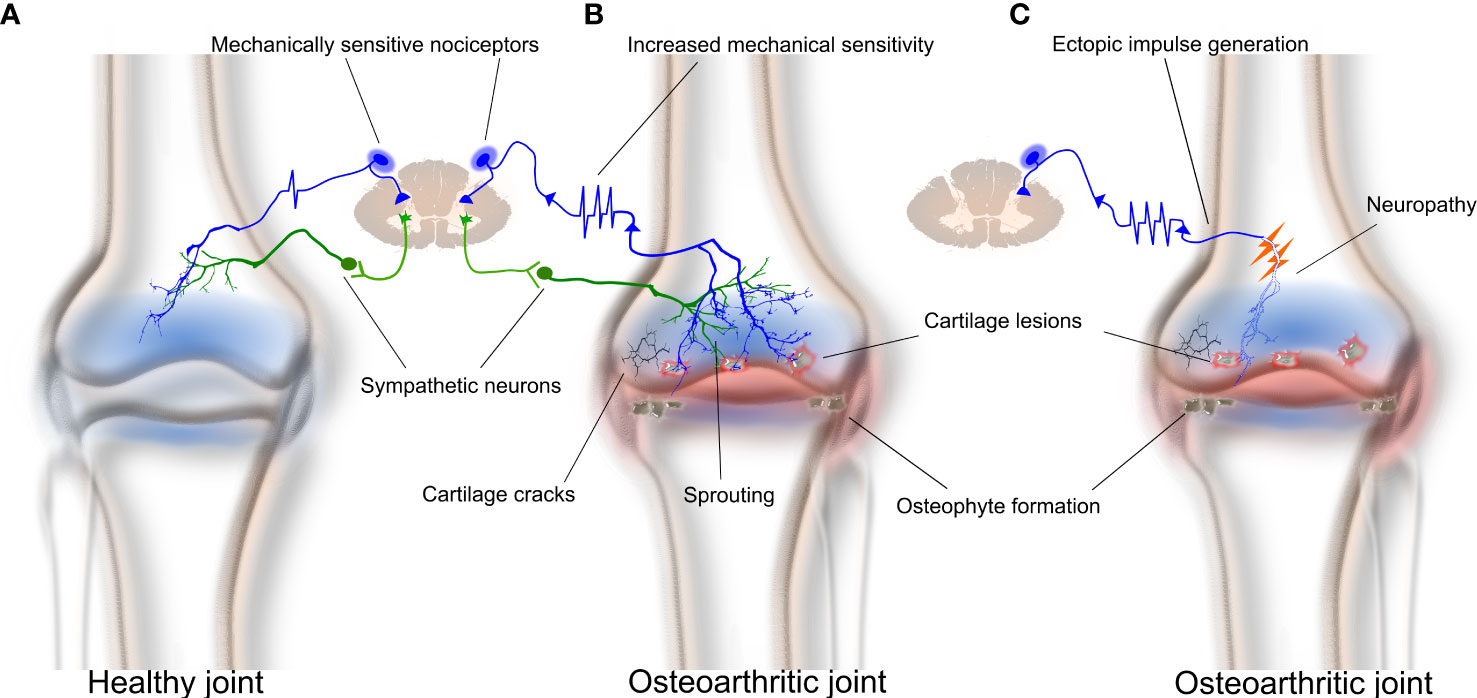
Figure 1 The subchondral bone contributes to osteoarthritis pain through mechanical sensitization, sprouting, and/or neuropathy of bone nociceptors. Osteoarthritis results in joint inflammation, reduced synovial space, breakdown of cartilage and cartilage lesions, osteophyte formation, and subchondral bone lesions and sclerosis. (A) A healthy bone with typical sympathetic and nociceptor innervation. (B) Subchondral bone remodeling in advanced osteoarthritis can result in increased neuronal sprouting of bone nociceptors and sympathetic neurons within bone. Sprouting sensitizes peripheral sensory neurons and makes them hyperexcitable. (C) Neuropathy of bone nociceptors may also contribute to osteoarthritis pain through generating ectopic spontaneous discharge.
Most of this work has been in MIA-induced OA. There have been a number of studies that have explored the temporal characteristics of pain associated with histopathological changes in the bone in alternative models, including partial meniscectomy and destabilization of the medial meniscus (30, 84–89). However, none of these have documented mechanisms by which the nerves in the bone contribute to this pain.
The findings of sensitization of nerves that innervate the bone cited here are important because they highlight the need to target the peripheral nociceptors that innervate the bone, in addition to those that innervate the joint, for therapeutic benefit in late-stage OA to be realized.
Neuronal sprouting in bone and OA pain
Neuronal remodeling is a phenomenon that can occur as a result of tissue damage and can result in hyperalgesia, allodynia, and ectopic firing (90–94). One type of neuronal remodeling is neuronal or axonal sprouting, where axons from undamaged neurons in neighboring areas sprout into the damaged tissue region (95). There is significant evidence that sprouting of both nociceptors and sympathetic neurons promote pain in and around joints. In a painful animal model of experimental inflammation, injection of Complete Freund’s adjuvant into the joint resulted in the sprouting of sympathetic and sensory neurons into the synovium, and the blockade of nerve growth factor (NGF) signaling reduced both sympathetic and sensory neuron sprouting and pain behavior (96).
There is also evidence of increased innervation in the subchondral bone marrow of femoral heads taken from patients with OA compared to those without (97). In a more recent study, increased sensory and sympathetic innervation was observed at the OC junction, and in the bone marrow and osteophytes, of patients with tibiofemoral OA (62). These findings are supported by animal studies showing that both nerve fibers and vessels sprout through channels at the OC junction to get access to the inflamed joint cavity (98, 99) and provide a potential structural explanation for increased pain sensation in OA patients (Figure 1). Aso et al. (100) further examined the effect and pain outcomes of anti-NGF treatment on the innervation of subchondral bone in rats with meniscal transection-induced OA. Rats that received anti-NGF treatment had less sprouting of CGRP-immunoreactive nerves through OC junctions and displayed reduced pain behavior (100), suggesting a clear association between sprouting and OA pain.
Neuropathy in bone and OA pain
There is emerging evidence for a role for neuropathy of nociceptors that innervate the bone in the pathophysiology of OA (28, 29, 101). One meta-analysis found that 23% of patients sampled reported neuropathic pain associated with their OA (102). Patients suffering from OA often use neuropathic descriptors to describe their pain, such as burning, numbness and pins and needles (103, 104), and application of therapeutics used to treat neuropathic pain in other conditions, such as gabapentin, often resolves the pain (105). In rats with MIA-induced OA, there is an increase in the number of DRG neurons that express ATF-3, a marker of sensory and motor neuron damage (106), and MIA administration results in the upregulation of other markers of neuronal injury and neuropathic pain in the DRG, including neuropeptide Y and interleukin-6 (107, 108).
One hypothesis for the cause of neuropathy in OA is damage to the subchondral bone in late-stage OA (109). It is possible that nociceptive nerve fibers become damaged when there is destruction of subchondral bone, such as that which is associated with bone marrow lesions and sclerosis (Figure 1). Damaged nerve axons are known to spontaneously and ectopically fire, and therefore can signal pain to the central nervous system (CNS) (110). Another potential mechanism is known as ‘cross talk’ or ‘ephaptic cross talk’ (111). This term refers to the phenomenon whereby regenerating nerve axons form synapse-like links with adjacent nerve axons following injury. The result of this process is abnormal nerve-to-nerve communication that can contribute to changes in the pain signals presented to the CNS.
Whilst it is possible that some of the physiological changes to the function of nociceptors reported are a response to the damage of peripheral nerve terminal endings in the subchondral bone in late-stage OA, a clear link between the two remains to be determined. It will be important in the future to determine how neuropathy affects nociceptors that innervate the bone, and its contribution to the pathogenesis of OA.
Conclusions
The role of nerves that innervate bone in OA pain has been of increasing interest due to the relationship between pain and subchondral bone pathology. There is evidence that bone nociceptors contribute to OA pain in several ways including sensitization, sprouting, and neuropathy. Targeting nociceptors that innervate the bone may provide an effective strategy to treat OA pain.
Author contributions
MM, VN, KH, and JI all contributed to literature searches and writing the manuscript. All authors have read and approved the final version of the manuscript.
Funding
This work was supported by funding from the Australian National Health and Medical Research Council.
Acknowledgments
The authors acknowledge Associate Professor James Brock and Ms. Jenny Thai for their comments on the manuscript.
Conflict of interest
The authors declare that the research was conducted in the absence of any commercial or financial relationships that could be construed as a potential conflict of interest.
Publisher’s note
All claims expressed in this article are solely those of the authors and do not necessarily represent those of their affiliated organizations, or those of the publisher, the editors and the reviewers. Any product that may be evaluated in this article, or claim that may be made by its manufacturer, is not guaranteed or endorsed by the publisher.
Abbreviations
- OA, Osteoarthritis; OC, osteochondral; DRG, dorsal root ganglia; CGRP, calcitonin gene-related peptide; TrkA, tyrosine receptor kinase A; MIA, monoiodoacetate; NGF, nerve growth factor
References
1. Bedson J, Mottram S, Thomas E, Peat G. Knee pain and osteoarthritis in the general population: what influences patients to consult? Fam Pract (2007) 24:443–53. doi: 10.1093/fampra/cmm036
2. Hatch RJ, Jennings EA, Ivanusic JJ. Peripheral hyperpolarization-activated cyclic nucleotide-gated channels contribute to inflammation-induced hypersensitivity of the rat temporomandibular joint. Eur J Pain (2013) 17:972–82. doi: 10.1002/j.1532-2149.2012.00261.x
3. Heppelmann B, Messlinger K, Neiss WF, Schmidt RF. Fine sensory innervation of the knee joint capsule by group III and group IV nerve fibers in the cat. J Comp Neurol (1995) 351:415–28. doi: 10.1002/cne.903510308
4. Heppelmann B, Messlinger K, Neiss WF, Schmidt RF. Ultrastructural three-dimensional reconstruction of group III and group IV sensory nerve endings ("free nerve endings") in the knee joint capsule of the cat: evidence for multiple receptive sites. J Comp Neurol (1990) 292:103–16. doi: 10.1002/cne.902920107
5. Brucini M, Duranti R, Galletti R, Pantaleo T, Zucchi PL. Pain thresholds and electromyographic features of periarticular muscles in patients with osteoarthritis of the knee. Pain (1981) 10:57–66. doi: 10.1016/0304-3959(81)90045-2
6. Miller RE, Malfait AM. Osteoarthritis pain: What are we learning from animal models? Best Pract Res Clin Rheumatol (2017) 31:676–87. doi: 10.1016/j.berh.2018.03.003
7. Philpott HT, O'Brien M, McDougall JJ. Attenuation of early phase inflammation by cannabidiol prevents pain and nerve damage in rat osteoarthritis. Pain (2017) 158:2442–51. doi: 10.1097/j.pain.0000000000001052
8. Grubb BD. Activation of sensory neurons in the arthritic joint. Novartis Found Symp (2004) 260:28–36. discussion 36-48, 100-4, 277-9.
9. Brazill JM, Beeve AT, Craft CS, Ivanusic JJ, Scheller EL. Nerves in bone: Evolving concepts in pain and anabolism. J Bone Miner Res (2019) 34:1393–406. doi: 10.1002/jbmr.3822
10. Ivanusic JJ. Size, neurochemistry, and segmental distribution of sensory neurons innervating the rat tibia. J Comp Neurol (2009) 517:276–83. doi: 10.1002/cne.22160
11. Sagar DR, Nwosu L, Walsh DA, Chapman V. Dissecting the contribution of knee joint NGF to spinal nociceptive sensitization in a model of OA pain in the rat. Osteoarthr Cartil (2015) 23:906–13. doi: 10.1016/j.joca.2015.01.010
12. Kidd BL, Photiou A, Inglis JJ. The role of inflammatory mediators on nociception and pain in arthritis. Novartis Found Symp (2004) 260:122–33. doi: 10.1002/0470867639.ch9
13. Schuelert N, McDougall JJ. Electrophysiological evidence that the vasoactive intestinal peptide receptor antagonist VIP6-28 reduces nociception in an animal model of osteoarthritis. Osteoarthr Cartil (2006) 14:1155–62. doi: 10.1016/j.joca.2006.04.016
14. Ivanusic JJ. Molecular mechanisms that contribute to bone marrow pain. Front Neurol (2017) 8:458. doi: 10.3389/fneur.2017.00458
15. Ivanusic JJ, Mahns DA, Sahai V, Rowe MJ. Absence of large-diameter sensory fibres in a nerve to the cat humerus. J Anat (2006) 208:251–5. doi: 10.1111/j.1469-7580.2006.00519.x
16. Morgan M, Nencini S, Thai J, Ivanusic JJ. TRPV1 activation alters the function of adelta and c fiber sensory neurons that innervate bone. Bone (2019) 123:168–75. doi: 10.1016/j.bone.2019.03.040
17. Morgan M, Thai J, Trinh P, Habib M, Effendi KN, Ivanusic JJ. ASIC3 inhibition modulates inflammation-induced changes in the activity and sensitivity of adelta and c fiber sensory neurons that innervate bone. Mol Pain (2020) 16:1744806920975950. doi: 10.1177/1744806920975950
18. Nencini S, Ivanusic J. Mechanically sensitive adelta nociceptors that innervate bone marrow respond to changes in intra-osseous pressure. J Physiol (2017) 595:4399–415. doi: 10.1113/JP273877
19. Nencini S, Morgan M, Thai J, Jobling AI, Mazzone SB, Ivanusic JJ. Piezo2 knockdown inhibits noxious mechanical stimulation and NGF-induced sensitization in a-delta bone afferent neurons. Front Physiol (2021) 12:644929. doi: 10.3389/fphys.2021.644929
20. Nencini S, Ringuet M, Kim DH, Chen YJ, Greenhill C, Ivanusic JJ. Mechanisms of nerve growth factor signaling in bone nociceptors and in an animal model of inflammatory bone pain. Mol Pain (2017) 13:1744806917697011. doi: 10.1177/1744806917697011
21. Nencini S, Ringuet M, Kim DH, Greenhill C, Ivanusic JJ. GDNF, neurturin, and artemin activate and sensitize bone afferent neurons and contribute to inflammatory bone pain. J Neurosci (2018) 38:4899–911. doi: 10.1523/JNEUROSCI.0421-18.2018
22. Nencini S, Thai J, Ivanusic JJ. Sequestration of artemin reduces inflammation-induced activation and sensitization of bone marrow nociceptors in a rodent model of carrageenan-induced inflammatory bone pain. Eur J Pain (2019) 23:397–409. doi: 10.1002/ejp.1315
23. Thai J, Kyloh M, Travis L, Spencer NJ, Ivanusic JJ. Identifying spinal afferent (sensory) nerve endings that innervate the marrow cavity and periosteum using anterograde tracing. J Comp Neurol (2020) 528:1903–16. doi: 10.1002/cne.24862
24. Ivanusic JJ, Beaini D, Hatch RJ, Staikopoulos V, Sessle BJ, Jennings EA. Peripheral n-methyl-d-aspartate receptors contribute to mechanical hypersensitivity in a rat model of inflammatory temporomandibular joint pain. Eur J Pain (2011) 15:179–85. doi: 10.1016/j.ejpain.2010.07.001
25. Krustev E, Rioux D, McDougall JJ. Mechanisms and mediators that drive arthritis pain. Curr Osteoporos Rep (2015) 13:216–24. doi: 10.1007/s11914-015-0275-y
26. McDougall JJ. Arthritis and pain. neurogenic origin of joint pain. Arthritis Res Ther (2006) 8:220. doi: 10.1186/ar2069
27. Eitner A, Hofmann GO, Schaible HG. Mechanisms of osteoarthritic pain. studies in humans and experimental models. Front Mol Neurosci (2017) 10:349. doi: 10.3389/fnmol.2017.00349
28. McDougall JJ, Linton P. Neurophysiology of arthritis pain. Curr Pain Headache Rep (2012) 16:485–91. doi: 10.1007/s11916-012-0300-0
29. Fu K, Robbins SR, McDougall JJ. Osteoarthritis: the genesis of pain. Rheumatol (Oxford) (2018) 57:iv43–50. doi: 10.1093/rheumatology/kex419
30. Vincent TL. Peripheral pain mechanisms in osteoarthritis. Pain (2020) 161 Suppl 1:S138–46. doi: 10.1097/j.pain.0000000000001923
31. Syx D, Tran PB, Miller RE, Malfait AM. Peripheral mechanisms contributing to osteoarthritis pain. Curr Rheumatol Rep (2018) 20:9. doi: 10.1007/s11926-018-0716-6
32. Baker K, Grainger A, Niu J, Clancy M, Guermazi A, Crema M, et al. Relation of synovitis to knee pain using contrast-enhanced MRIs. Ann Rheum Dis (2010) 69:1779–83. doi: 10.1136/ard.2009.121426
33. de Lange-Brokaar BJ, Ioan-Facsinay A, Yusuf E, Visser AW, Kroon HM, van Osch GJ, et al. Association of pain in knee osteoarthritis with distinct patterns of synovitis. Arthritis Rheumatol (2015) 67:733–40. doi: 10.1002/art.38965
34. Hill CL, Hunter DJ, Niu J, Clancy M, Guermazi A, Genant H, et al. Synovitis detected on magnetic resonance imaging and its relation to pain and cartilage loss in knee osteoarthritis. Ann Rheum Dis (2007) 66:1599–603. doi: 10.1136/ard.2006.067470
35. Lawrence JS, Bremner JM, Bier F. Osteo-arthrosis. prevalence in the population and relationship between symptoms and x-ray changes. Ann Rheumatol Dis (1966) 25:1–24. doi: 10.1136/ard.25.1.1
36. Davis MA, Ettinger WH, Neuhaus JM, Barclay JD, Segal MR. Correlates of knee pain among US adults with and without radiographic knee osteoarthritis. J Rheumatol (1992) 19:1943–9.
37. Felson DT, Lawrence RC, Dieppe PA, Hirsch R, Helmick CG, Jordan JM, et al. Osteoarthritis: new insights. part 1: the disease and its risk factors. Ann Intern Med (2000) 133:635–46. doi: 10.7326/0003-4819-133-8-200010170-00016
38. McAlindon TE, Cooper C, Kirwan JR, Dieppe PA. Knee pain and disability in the community. Br J Rheumatol (1992) 31:189–92. doi: 10.1093/rheumatology/31.3.189
39. Petersen KK, Siebuhr AS, Graven-Nielsen T, Simonsen O, Boesen M, Gudbergsen H, et al. Sensitization and serological biomarkers in knee osteoarthritis patients with different degrees of synovitis. Clin J Pain (2016) 32:841–8. doi: 10.1097/AJP.0000000000000334
40. Klement MR, Sharkey PF. The significance of osteoarthritis-associated bone marrow lesions in the knee. J Am Acad Orthop Surg (2019) 27:752–9. doi: 10.5435/JAAOS-D-18-00267
41. Suri S, Walsh DA. Osteochondral alterations in osteoarthritis. Bone (2012) 51:204–11. doi: 10.1016/j.bone.2011.10.010
42. Felson DT, McLaughlin S, Goggins J, LaValley MP, Gale ME, Totterman S, et al. Bone marrow edema and its relation to progression of knee osteoarthritis. Ann Intern Med (2003) 139:330–6. doi: 10.7326/0003-4819-139-5_Part_1-200309020-00008
43. Burr DB, Radin EL. Microfractures and microcracks in subchondral bone: are they relevant to osteoarthrosis? Rheumatol Dis Clin North Am (2003) 29:675–85. doi: 10.1016/S0889-857X(03)00061-9
44. Felson DT, Niu J, Guermazi A, Roemer F, Aliabadi P, Clancy M, et al. Correlation of the development of knee pain with enlarging bone marrow lesions on magnetic resonance imaging. Arthritis & Rheumatism (2007) 56:2986–92. doi: 10.1002/art.22851
45. Aso K, Shahtaheri SM, McWilliams DF, Walsh D. Association of subchondral bone marrow lesion localization with weight-bearing pain in people with knee osteoarthritis: data from the osteoarthritis initiative. Arthritis Res Ther (2021) 23:1–9. doi: 10.1186/s13075-021-02422-0
46. Arnoldi CC, Djurhuus JC, Heerfordt J, Karle A. Intraosseous phlebography, intraosseous pressure measurements and 99mTC-polyphosphate scintigraphy in patients with various painful conditions in the hip and knee. Acta Orthop Scand (1980) 51:19–28. doi: 10.3109/17453678008990764
47. Arnoldi CC, Lemperg K, Linderholm H. Intraosseous hypertension and pain in the knee. J Bone Joint Surg Br (1975) 57:360–3. doi: 10.1302/0301-620X.57B3.360
48. Arnoldi CC, Lemperg R, Linderholm H. Immediate effect of osteotomy on the intramedullary pressure in the femoral head and neck in patients with degenerative osteoarthritis. Acta Orthop Scand (1971) 42:454–5. doi: 10.3109/17453677108989056
49. Arnoldi CC, Linderholm H, Mussbichler H. Venous engorgement and intraosseous hypertension in osteoarthritis of the hip. J Bone Joint Surg Br (1972) 54:409–21. doi: 10.1302/0301-620X.54B3.409
50. Dieppe P. Subchondral bone should be the main target for the treatment of pain and disease progression in osteoarthritis. Osteoarthr Cartil (1999) 7:325–6. doi: 10.1053/joca.1998.0182
51. Simkin PA. Bone pain and pressure in osteoarthritic joints. Novartis Found Symp (2004) 260:179–86. doi: 10.1002/0470867639.ch12
52. Varenna M, Zucchi F, Failoni S, Becciolini A, Berruto M. Intravenous neridronate in the treatment of acute painful knee osteoarthritis: a randomized controlled study. Rheumatol (Oxford) (2015) 54:1826–32. doi: 10.1093/rheumatology/kev123
53. Laslett LL, Dore DA, Quinn SJ, Boon P, Ryan E, Winzenberg TM, et al. Zoledronic acid reduces knee pain and bone marrow lesions over 1 year: a randomised controlled trial. Ann Rheum Dis (2012) 71:1322–8. doi: 10.1136/annrheumdis-2011-200970
54. Takase B, Nomura S. Studies on the innervation of the bone marrow. J Comp Neurol (1957) 108:421–43. doi: 10.1002/cne.901080305
55. Nencini S, Ivanusic JJ. The physiology of bone pain. how much do we really know? Front Physiol (2016) 7:157. doi: 10.3389/fphys.2016.00157
56. Calvo W. The innervation of the bone marrow in laboratory animals. Am J Anat (1968) 123:315–28. doi: 10.1002/aja.1001230206
57. Miller MR, Kasahara M. Observations on the innervation of human long bones. Anat Rec (1963) 145:13–23. doi: 10.1002/ar.1091450104
58. Cooper RR, Milgram JW, Robinson RA. Morphology of the osteon. an electron microscopic study. J Bone Joint Surg Am (1966) 48:1239–71. doi: 10.2106/00004623-196648070-00001
59. Thurston TJ. Distribution of nerves in long bones as shown by silver impregnation. J Anat (1982) 134(Pt 4):719–28.
60. Sakada S, Maeda K. Characteristics of innervation and nerve ending in cat's mandibular periosteum. Bull Tokyo Dent Coll (1967) 8:77–94.
62. Suri S, Gill SE, Massena de Camin S, Wilson D, McWilliams DF, Walsh DA. Neurovascular invasion at the osteochondral junction and in osteophytes in osteoarthritis. Ann Rheum Dis (2007) 66:1423–8. doi: 10.1136/ard.2006.063354
63. Chartier SR, Mitchell SAT, Majuta LA, Mantyh PW. The changing sensory and sympathetic innervation of the young, adult and aging mouse femur. Neuroscience (2018) 387:178–90. doi: 10.1016/j.neuroscience.2018.01.047
64. Gronblad M, Liesi P, Korkala O, Karaharju E, Polak J. Innervation of human bone periosteum by peptidergic nerves. Anat Rec (1984) 209:297–9. doi: 10.1002/ar.1092090306
65. Steverink JG, Oostinga D, van Tol FR, van Rijen MHP, Mackaaij C, Verlinde-Schellekens S, et al. Sensory innervation of human bone: An immunohistochemical study to further understand bone pain. J Pain (2021) 22:1385–95. doi: 10.1016/j.jpain.2021.04.006
66. Castaneda-Corral G, Jimenez-Andrade JM, Bloom AP, Taylor RN, Mantyh WG, Kaczmarska MJ, et al. The majority of myelinated and unmyelinated sensory nerve fibers that innervate bone express the tropomyosin receptor kinase a. Neuroscience (2011) 178:196–207. doi: 10.1016/j.neuroscience.2011.01.039
68. Hill EL, Elde R. Calcitonin gene-related peptide-immunoreactive nerve fibers in mandibular periosteum of rat: evidence for primary afferent origin. Neurosci Lett (1988) 85:172–8. doi: 10.1016/0304-3940(88)90347-3
69. Hohmann EL, Elde RP, Rysavy JA, Einzig S, Gebhard RL. Innervation of periosteum and bone by sympathetic vasoactive intestinal peptide-containing nerve fibers. Science (1986) 232:868–71. doi: 10.1126/science.3518059
70. Dubin AE, Patapoutian A. Nociceptors: the sensors of the pain pathway. J Clin Invest (2010) 120:3760–72. doi: 10.1172/JCI42843
71. Gajda M, Litwin JA, Adriaensen D, Timmermans JP, Cichocki T. Segmental distribution and morphometric features of primary sensory neurons projecting to the tibial periosteum in the rat. Folia Histochem Cytobiol (2004) 42:95–9.
72. Aso K, Ikeuchi M, Izumi M, Sugimura N, Kato T, Ushida T, et al. Nociceptive phenotype of dorsal root ganglia neurons innervating the subchondral bone in rat knee joints. Eur J Pain (2014) 18:174–81. doi: 10.1002/j.1532-2149.2013.00360.x
73. Jimenez-Andrade JM, Mantyh WG, Bloom AP, Xu H, Ferng AS, Dussor G, et al. A phenotypically restricted set of primary afferent nerve fibers innervate the bone versus skin: therapeutic opportunity for treating skeletal pain. Bone (2010) 46:306–13. doi: 10.1016/j.bone.2009.09.013
74. Mach DB, Rogers SD, Sabino MC, Luger NM, Schwei MJ, Pomonis JD, et al. Origins of skeletal pain: sensory and sympathetic innervation of the mouse femur. Neuroscience (2002) 113:155–66. doi: 10.1016/S0306-4522(02)00165-3
75. Furusawa S. A neurophysiological study on the sensibility of the bone marrow. Nihon Seikeigeka Gakkai Zasshi (1970) 44:365–70.
76. Seike W. Electrophysiological and histological studies on the sensibility of the bone marrow nerve terminal. Yonago Acta Med (1976) 20:192–211.
77. Schuelert N, McDougall JJ. Cannabinoid-mediated antinociception is enhanced in rat osteoarthritic knees. Arthritis Rheum (2008) 58:145–53. doi: 10.1002/art.23156
78. Schuelert N, McDougall JJ. Grading of monosodium iodoacetate-induced osteoarthritis reveals a concentration-dependent sensitization of nociceptors in the knee joint of the rat. Neurosci Lett (2009) 465:184–8. doi: 10.1016/j.neulet.2009.08.063
79. Schuelert N, McDougall JJ. Involvement of nav 1.8 sodium ion channels in the transduction of mechanical pain in a rodent model of osteoarthritis. Arthritis Res Ther (2012) 14:R5. doi: 10.1186/ar3553
80. Schuelert N, Zhang C, Mogg AJ, Broad LM, Hepburn DL, Nisenbaum ES, et al. Paradoxical effects of the cannabinoid CB2 receptor agonist GW405833 on rat osteoarthritic knee joint pain. Osteoarthr Cartil (2010) 18:1536–43. doi: 10.1016/j.joca.2010.09.005
81. Morgan M, Thai J, Nazemian V, Song R, Ivanusic JJ. Changes to the activity and sensitivity of nerves innervating subchondral bone contribute to pain in late-stage osteoarthritis. Pain (2022) 163:390–402. doi: 10.1097/j.pain.0000000000002355
82. Fernihough J, Gentry C, Malcangio M, Fox A, Rediske J, Pellas T, et al. Pain related behaviour in two models of osteoarthritis in the rat knee. Pain (2004) 112:83–93. doi: 10.1016/j.pain.2004.08.004
83. Mousseau M, Burma NE, Lee KY, Leduc-Pessah H, Kwok CHT, Reid AR, et al. Microglial pannexin-1 channel activation is a spinal determinant of joint pain. Sci Adv (2018) 4:eaas9846. doi: 10.1126/sciadv.aas9846
84. Burleigh A, Chanalaris A, Gardiner MD, Driscoll C, Boruc O, Saklatvala J, et al. Joint immobilization prevents murine osteoarthritis and reveals the highly mechanosensitive nature of protease expression in vivo. Arthritis Rheum (2012) 64:2278–88. doi: 10.1002/art.34420
85. Miller RE, Tran PB, Das R, Ghoreishi-Haack N, Ren D, Miller RJ, et al. CCR2 chemokine receptor signaling mediates pain in experimental osteoarthritis. Proc Natl Acad Sci U.S.A. (2012) 109:20602–7. doi: 10.1073/pnas.1209294110
86. Driscoll C, Chanalaris A, Knights C, Ismail H, Sacitharan PK, Gentry C, et al. Nociceptive sensitizers are regulated in damaged joint tissues, including articular cartilage, when osteoarthritic mice display pain behavior. Arthritis Rheumatol (2016) 68:857–67. doi: 10.1002/art.39523
87. McNamee KE, Burleigh A, Gompels LL, Feldmann M, Allen SJ, Williams RO, et al. Treatment of murine osteoarthritis with TrkAd5 reveals a pivotal role for nerve growth factor in non-inflammatory joint pain. Pain (2010) 149:386–92. doi: 10.1016/j.pain.2010.03.002
88. Miotla Zarebska J, Chanalaris A, Driscoll C, Burleigh A, Miller RE, Malfait AM, et al. CCL2 and CCR2 regulate pain-related behaviour and early gene expression in post-traumatic murine osteoarthritis but contribute little to chondropathy. Osteoarthr Cartil (2017) 25:406–12. doi: 10.1016/j.joca.2016.10.008
89. von Loga IS, El-Turabi A, Jostins L, Miotla-Zarebska J, Mackay-Alderson J, Zeltins A, et al. Active immunisation targeting nerve growth factor attenuates chronic pain behaviour in murine osteoarthritis. Ann Rheum Dis (2019) 78:672–5. doi: 10.1136/annrheumdis-2018-214489
90. Black JA, Nikolajsen L, Kroner K, Jensen TS, Waxman SG. Multiple sodium channel isoforms and mitogen-activated protein kinases are present in painful human neuromas. Ann Neurol (2008) 64:644–53. doi: 10.1002/ana.21527
91. Ceyhan GO, Bergmann F, Kadihasanoglu M, Altintas B, Demir IE, Hinz U, et al. Pancreatic neuropathy and neuropathic pain–a comprehensive pathomorphological study of 546 cases. Gastroenterology (2009) 136:177–186 e1. doi: 10.1053/j.gastro.2008.09.029
92. Janig W, Baron R. Complex regional pain syndrome: mystery explained? Lancet Neurol (2003) 2:687–97. doi: 10.1016/s1474-4422(03)00557-x
93. Jimenez-Andrade JM, Bloom AP, Stake JI, Mantyh WG, Taylor RN, Freeman KT, et al. Pathological sprouting of adult nociceptors in chronic prostate cancer-induced bone pain. J Neurosci (2010) 30:14649–56. doi: 10.1523/JNEUROSCI.3300-10.2010
94. Lindqvist A, Rivero-Melian C, Turan I, Fried K. Neuropeptide- and tyrosine hydroxylase-immunoreactive nerve fibers in painful morton's neuromas. Muscle Nerve (2000) 23:1214–8. doi: 10.1002/1097-4598(200008)23:8<1214::aid-mus9>3.0.co;2-a
95. Kuner R, Flor H. Structural plasticity and reorganisation in chronic pain. Nat Rev Neurosci (2017) 18:113. doi: 10.1038/nrn.2017.5
96. Ghilardi JR, Freeman KT, Jimenez-Andrade JM, Coughlin KA, Kaczmarska MJ, Castaneda-Corral G, et al. Neuroplasticity of sensory and sympathetic nerve fibers in a mouse model of a painful arthritic joint. Arthritis Rheum (2012) 64:2223–32. doi: 10.1002/art.34385
97. Reimann I, Christensen SB. A histological demonstration of nerves in subchondral bone. Acta Orthop Scand (1977) 48:345–52. doi: 10.3109/17453677708992006
98. Obeidat AM, Miller RE, Miller RJ, Malfait AM. The nociceptive innervation of the normal and osteoarthritic mouse knee. Osteoarthr Cartil (2019) 27:1669–79. doi: 10.1016/j.joca.2019.07.012
99. Mapp PI, Sagar DR, Ashraf S, Burston JJ, Suri S, Chapman V, et al. Differences in structural and pain phenotypes in the sodium monoiodoacetate and meniscal transection models of osteoarthritis. Osteoarthr Cartil (2013) 21:1336–45. doi: 10.1016/j.joca.2013.06.031
100. Aso K, Shahtaheri SM, Hill R, Wilson D, McWilliams DF, Nwosu LN, et al. Contribution of nerves within osteochondral channels to osteoarthritis knee pain in humans and rats. Osteoarthr Cartil (2020) 28:1245–54. doi: 10.1016/j.joca.2020.05.010
101. McDougall JJ, Albacete S, Schuelert N, Mitchell PG, Lin C, Oskins JL, et al. Lysophosphatidic acid provides a missing link between osteoarthritis and joint neuropathic pain. Osteoarthr Cartil (2017) 25:926–34. doi: 10.1016/j.joca.2016.08.016
102. French HP, Smart KM, Doyle F. Prevalence of neuropathic pain in knee or hip osteoarthritis: A systematic review and meta-analysis. Semin Arthritis Rheum (2017) 47:1–8. doi: 10.1016/j.semarthrit.2017.02.008
103. Hawker GA, Stewart L, French MR, Cibere J, Jordan JM, March L, et al. Understanding the pain experience in hip and knee osteoarthritis–an OARSI/OMERACT initiative. Osteoarthr Cartil (2008) 16:415–22. doi: 10.1016/j.joca.2007.12.017
104. Hochman JR, French MR, Bermingham SL, Hawker GA. The nerve of osteoarthritis pain. Arthritis Care Res (Hoboken) (2010) 62:1019–23. doi: 10.1002/acr.20142
105. Wiffen PJ, Derry S, Bell RF, Rice AS, Tolle TR, Phillips T, et al. Gabapentin for chronic neuropathic pain in adults. Cochrane Database Syst Rev (2017) 6:CD007938. doi: 10.1002/14651858.CD007938.pub4
106. Ivanavicius SP, Ball AD, Heapy CG, Westwood RF, Murray F, Read SJ. Structural pathology in a rodent model of osteoarthritis is associated with neuropathic pain: increased expression of ATF-3 and pharmacological characterisation. Pain (2007) 128:272–82. doi: 10.1016/j.pain.2006.12.022
107. Ferreira-Gomes J, Adaes S, Sousa RM, Mendonca M, Castro-Lopes JM. Dose-dependent expression of neuronal injury markers during experimental osteoarthritis induced by monoiodoacetate in the rat. Mol Pain (2012) 8:50. doi: 10.1186/1744-8069-8-50
108. Malek N, Mrugala M, Makuch W, Kolosowska N, Przewlocka B, Binkowski M, et al. A multi-target approach for pain treatment: dual inhibition of fatty acid amide hydrolase and TRPV1 in a rat model of osteoarthritis. Pain (2015) 156:890–903. doi: 10.1097/j.pain.0000000000000132
109. Ohtori S, Orita S, Yamashita M, Ishikawa T, Ito T, Shigemura T, et al. Existence of a neuropathic pain component in patients with osteoarthritis of the knee. Yonsei Med J (2012) 53:801–5. doi: 10.3349/ymj.2012.53.4.801
110. Sun Q, Tu H, Xing GG, Han JS, Wan Y. Ectopic discharges from injured nerve fibers are highly correlated with tactile allodynia only in early, but not late, stage in rats with spinal nerve ligation. Exp Neurol (2005) 191:128–36. doi: 10.1016/j.expneurol.2004.09.008
Keywords: subchondral bone, sensory innervation, sensitization, sprouting, osteoarthritis pain, neuropathy
Citation: Morgan M, Nazemian V, Harrington K and Ivanusic JJ (2022) Mini review: The role of sensory innervation to subchondral bone in osteoarthritis pain. Front. Endocrinol. 13:1047943. doi: 10.3389/fendo.2022.1047943
Received: 20 September 2022; Accepted: 30 November 2022;
Published: 20 December 2022.
Edited by:
Yong-Can Huang, Shenzhen Hospital, Peking University, ChinaReviewed by:
Dan Yi, Shenzhen Institutes of Advanced Technology (CAS), ChinaCopyright © 2022 Morgan, Nazemian, Harrington and Ivanusic. This is an open-access article distributed under the terms of the Creative Commons Attribution License (CC BY). The use, distribution or reproduction in other forums is permitted, provided the original author(s) and the copyright owner(s) are credited and that the original publication in this journal is cited, in accordance with accepted academic practice. No use, distribution or reproduction is permitted which does not comply with these terms.
*Correspondence: Michael Morgan, michael.morgan@unimelb.edu.au