- 1Laboratory of Medical & Industrial Biotechnology (LABMI)-Porto Research, Technology and Innovation Center (PORTIC), Porto, Portugal
- 2Departamento de Biomedicina, Faculdade de Medicina da Universidade do Porto, Porto, Portugal
- 3Instituto de Investigação e Inovação em Saúde (i3S), Universidade do Porto, Porto, Portugal
- 4ESS-IPP – Escola Superior de Saúde, Instituto Politécnico do Porto, Porto, Portugal
Type 2 Diabetes (T2D) is currently one of the fastest growing health challenging, a non-communicable disease result of the XXI century lifestyle. Given its growing incidence and prevalence, it became increasingly imperative to develop new technologies and implement new biomarkers for early diagnosis in order to promote lifestyle changes and thus cause a setback of the disease. Promising biomarkers have been identified as predictive of T2D development; however, none of them have yet been implemented in clinical practice routine. Moreover, many prediabetic biomarkers can also represent potential therapeutical targets in disease management. Previous studies have identified the most popular biomarkers, which are being thoroughly investigated. However, there are some biomarkers with promising preliminary results with limited associated studies; hence there is still much to be understood about its mechanisms and associations in T2D pathophysiology. This work identifies and discusses the promising results of Galectin-3, Ophthalmate and Fetuin-A.
Introduction
Type 2 Diabetes (T2D) is already considered a worldwide pandemic, no longer restricted to developed countries (1), responsible for more than 4.2 million deaths in 2019 (2) and a trigger for other non‐communicable diseases like cancer and cardiovascular diseases. It is a complex disease with different pathophysiology profiles concomitant with inflammation, adiposity/obesity, lipid oxidation, hyperglycemia, glycation/glycosylation, and oxidative stress, further discussed. Such mechanisms can lead to insulin secretion deficiency from pancreatic beta cells, tissue insulin resistance, and impaired compensatory response to insulin (3).
Pro-inflammatory signals lead to immune cell activation and recruitment, an immune response that can result in insulin resistance. Previous studies have detected that this likely happens due to phosphorylation in the insulin receptor substrate (4). A low state of chronic inflammation is found upon adipose tissue accumulation, particularly in the abdominal depot (5). Already well-established, the adipose tissue is an endocrine organ, metabolically active, responsible for numerous functions, hence releasing several factors (6). Such factors are dynamic intervenients in processes like food uptake (asprosin, leptin), inflammation (TNF-α, IL-1β, IL-6, resistin, among others), energy expenditure (visfatin), immunity (MCP-1, MIP-1α, among others), and metabolism homeostasis (UCP-1) (5, 6).
Common knowledge states that obesity is the imbalance between energy consumption and energy expenditure. When the expenditure rate is lower than the consumption rate, the accumulation in the fat deposits will increase, leading to obesity and consequently deregulated energy metabolism. A shift towards lipid oxidation to the detriment of glucose oxidation occurs (7) since fatty acids and glucose are the main substrates for oxidation. The Glucose/Fatty Acid Cycle (8) expresses the metabolic relationship between the two substrates. An increase in fatty acid levels leads to the inhibition of glucose oxidation and cell uptake, which can be stored as glycogen, resulting in hyperglycemia and, ultimately, in insulin resistance (9, 10).
Prolonged exposure to a hyperglycemic state also causes tissue damage by promoting glycation, where sugars are covalently bound to proteins without enzymatic intervention. These Amadori products produce complex irreversible glycation adducts, the so-called Advanced Glycation End products (AGEs), which are capable of damaging cells (like pancreatic beta cells), tissues, and processes (11). AGEs are also responsible for causing oxidative stress.
Oxidative stress state is a condition where the free radical production is higher than the antioxidant defense activity. The human defense to free radicals consists of two main mechanisms: the enzymatic domain, which includes catalase, glutathione peroxidase and reductase, and superoxide dismutase; and the non-enzymatic domain that includes antioxidants, such as vitamins – A, C and E, glutathione and uric acid (12).
Recently, metabolomic studies have been used to identify, quantify, and characterize the metabolites’ physicochemical properties of such pathways cascades, allowing a better comprehension of the overall metabolism (13). Identification of early alterations in the pathophysiological profiles of the prediabetic individual can be critical in order to break the diabetic cascade. Our aim is to identify promising biomarkers able to represent the metabolic alterations that occur during the pathophysiological transformation of prediabetes to the clinical stage of T2D.
A recent study performed a survey regarding review articles of the last decade identifying biomarkers (metabolites and microRNA) associated with prediabetes, impaired fasting glucose and impaired glucose tolerance (14). Interestingly enough, the most popular and characterized biomarkers aren’t yet implemented in the clinical practice. We believe that the present work could present and expose the underestimated prediabetic biomarkers to be academically embraced to a more extended comprehension. We identify Galectin-3, Ophthalmate and Fetuin-a, as encouraging metabolites to address, which have been neglected in the last 10 years.
Galectin-3
Galectin 3 (Gal3) is a member of an evolutionarily conserved family of soluble β-galactosidases binding lectins. It is known to be a relevant modulator of many biological functions (Figure 1) and an emerging player in the pathogenesis of common disease conditions, including cancer (15–17), immune/inflammatory diseases (18–20), and metabolic disorders like T2D (21–24).
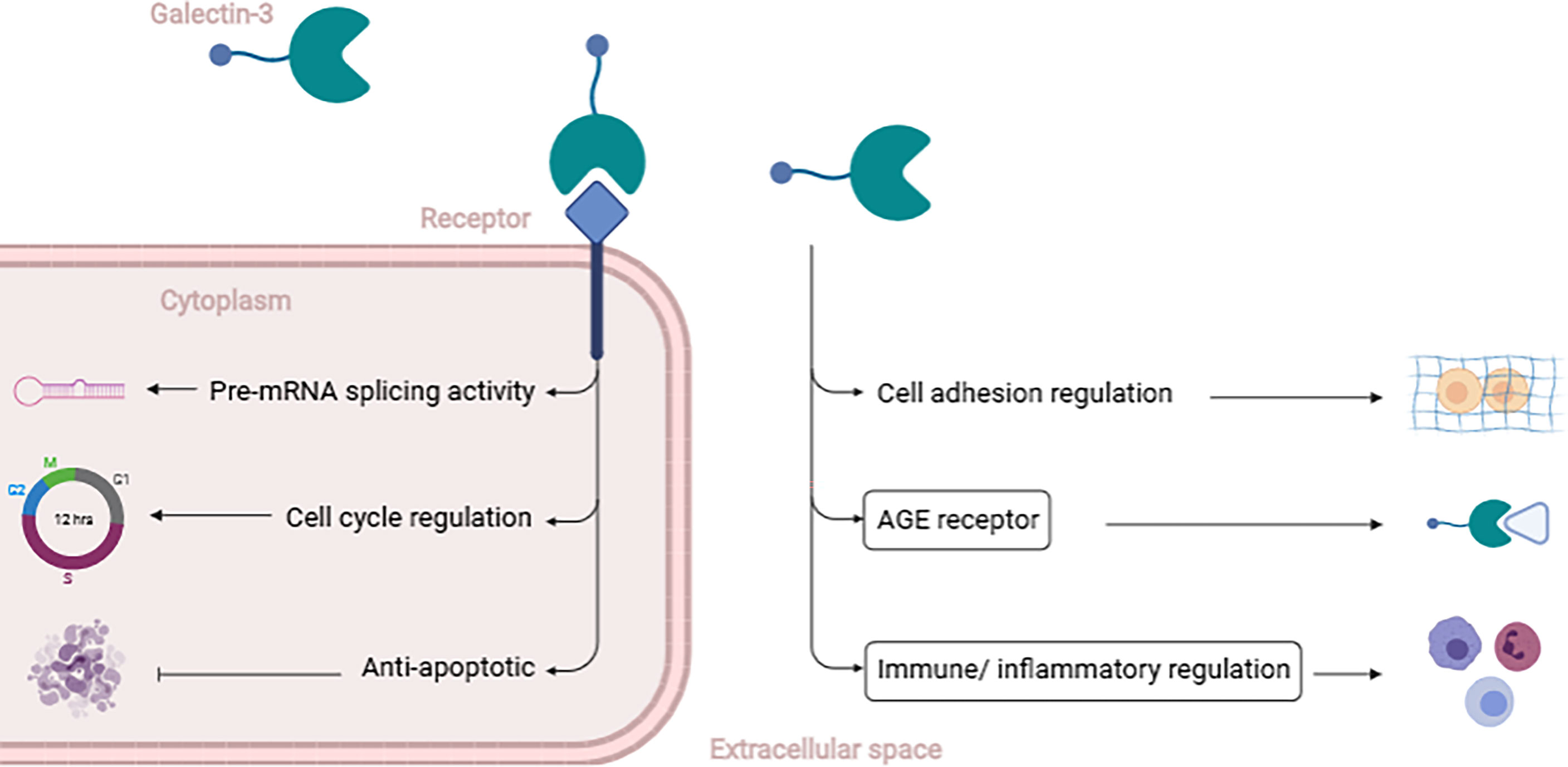
Figure 1 Brief description of Gal3’s functions. Gal3 exerts different functions depending on its location. Intracellularly, Gal3 can act as a Pre-mRNA splicing factor, regulating the cell cycle, promoting proliferation, and protecting from apoptosis. Extracellularly, Gal3 is able to regulate cell adhesion (either cell-to-cell or cell-to-matrix) and mediate features of T2D pathophysiology like binding to AGEs, regulating inflammatory and immune pathways (emphasized by the boxes) [20]. (Created with BioRender.com).
In animal models, Gal3 is associated with increased oxidative status, a common feature in T2D. Our group has observed increased levels of Gal3 in diabetic mice (22), concomitant with higher concentrations of AGEs and 3-nitrotyrosine in the liver and kidney. Pejnovic et al. also described that Gal3 knockout mice revealed a higher Body Mass Index (BMI) and increased levels of inflammation on visceral adipose tissue. Therefore, aggravated inflammatory status on pancreatic islets associated with AGE accumulation which resulted in impaired glucose homeostasis (25).
In human studies, circulating Gal3 was elevated in obese individuals and negatively correlated with HbA1c in T2D patients. The connection between Gal3 and HbA1c is still not fully understood, although it is postulated that Gal3 may reduce oxidative stress by scavenging AGEs, which leads to lower levels of HbA1c (26). This association between Gal3 and the incidence of T2D was suggested in the Dallas Heart Study with logistic regression models. Results demonstrated that Gal3 levels were correlated with inflammation, subcutaneous adiposity, and insulin secretion, concordant with the previous results obtained in animal models (27). Moreover, as a prediabetic biomarker, a study performed in First Degree Relatives of subjects with T2D observed that Gal3 is correlated with increased waist circumference, HbA1c, glucose, and high-sensitivity C-reactive protein levels and can also be determinant for the prediction of diabetes (28).
Ophthalmate
Ophthalmate (OPH) or ophthalmic acid belongs to the class of organic compounds known as oligopeptides isolated from the crystalline lens. It is a tripeptide analog of glutathione (GSH), composed of glutamate and glycine residues as GSH, but where GSH cysteine residue is replaced by α-aminobutyrate (α-AB) (29). GSH is synthesized in two steps, first glutamate and cysteine produce γ-glutamylcysteine catalyzed by γ-glutamylcysteine synthetase. Then, glycine by glutathione synthetase is added, and GSH is produced (30), having a crucial role in the antioxidant metabolism. The limiting step of GSH synthesis is the low cysteine levels produced exclusively by the transsulfuration pathway in conjugation with the methionine metabolism (31).
Studies performed in mice showed that overall oxidative stress related to T2D might lead to the depletion of glutathione, which motivates the depletion of cysteine and, consequently, activates the ophthalmate synthesis (32). In OPH, cysteine is replaced by α-AB, a contributor metabolite of several pathways, including amino acid catabolism (30) (Figure 2). Therefore, OPH levels are inversely correlated with GSH concentration. Given that GSH quantification is unstable, therefore unreliable due to auto-oxidation, by lacking the reducing cysteine moiety, OPH could represent a more accurate biomarker due to its stability.
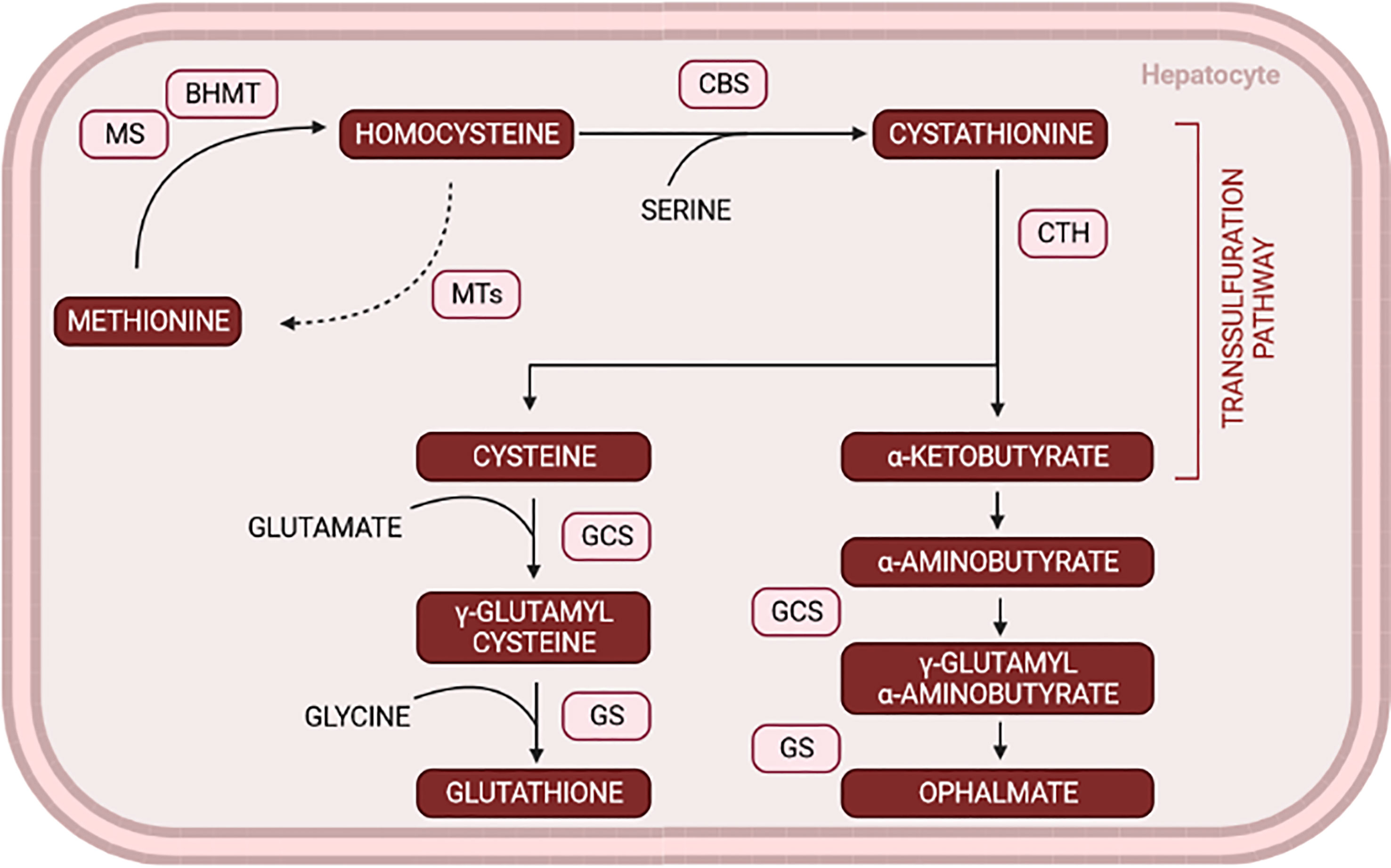
Figure 2 Glutathione and ophthalmate shared pathways via transsulfuration in conjugation with methionine metabolism. The increase in OPH levels works as a rather compensatory mechanism when glutathione depletion occurs. MS, methionine synthase; BHMT, Betaine homocysteine methyltransferase; MTs, methyltransferases; CBS, cystathionine β-synthase; CTH, cystathionase; GCS, glutamyl-cysteine synthase; GS, Glutathione synthetase. (Created with BioRender.com).
Soga and colleagues established a method to determine OPH levels in response to oxidative stress in animal models. They used different compounds to deplete GSH levels and observed that most of the metabolites detected in the liver were also detected in the serum and that ophthalmate increased 5-fold 1h after treatment concomitantly with a GSH decrease. These results, among others (30, 33), can help validate OPH as a biomarker and evaluate therapeutical assessments, potentially helping in the early detection of several diseases associated with oxidative stress like T2D (32).
Metabolomic studies including OPH are being performed in humans with positive insights, but not in prediabetes (34). Hence, much more data is needed, like cutoffs, quantification methods, and full comprehension of the limitations and benefits to implement OPH as a prediabetic biomarker.
Fetuin-a
Fetuin-A is a 64-kDa glycoprotein secreted by the adipose tissue and the liver, associated with several metabolic functions and pathological progressions in insulin resistance, vascular calcification, cell dysfunction, among others (35, 36). Fetuin-A is increased in pathologies like T2D, metabolic syndrome, obesity, and obesity-related complications such as non-alcoholic fatty liver disease and chronic kidney disease. Fetuin-A inhibits the insulin receptor through tyrosine phosphorylation, the capture of macrophages, and TLR4 activation by acting as an endogenous ligand and activating NF-kB and triacylglycerol accumulation (Figure 3) (35, 37, 38).
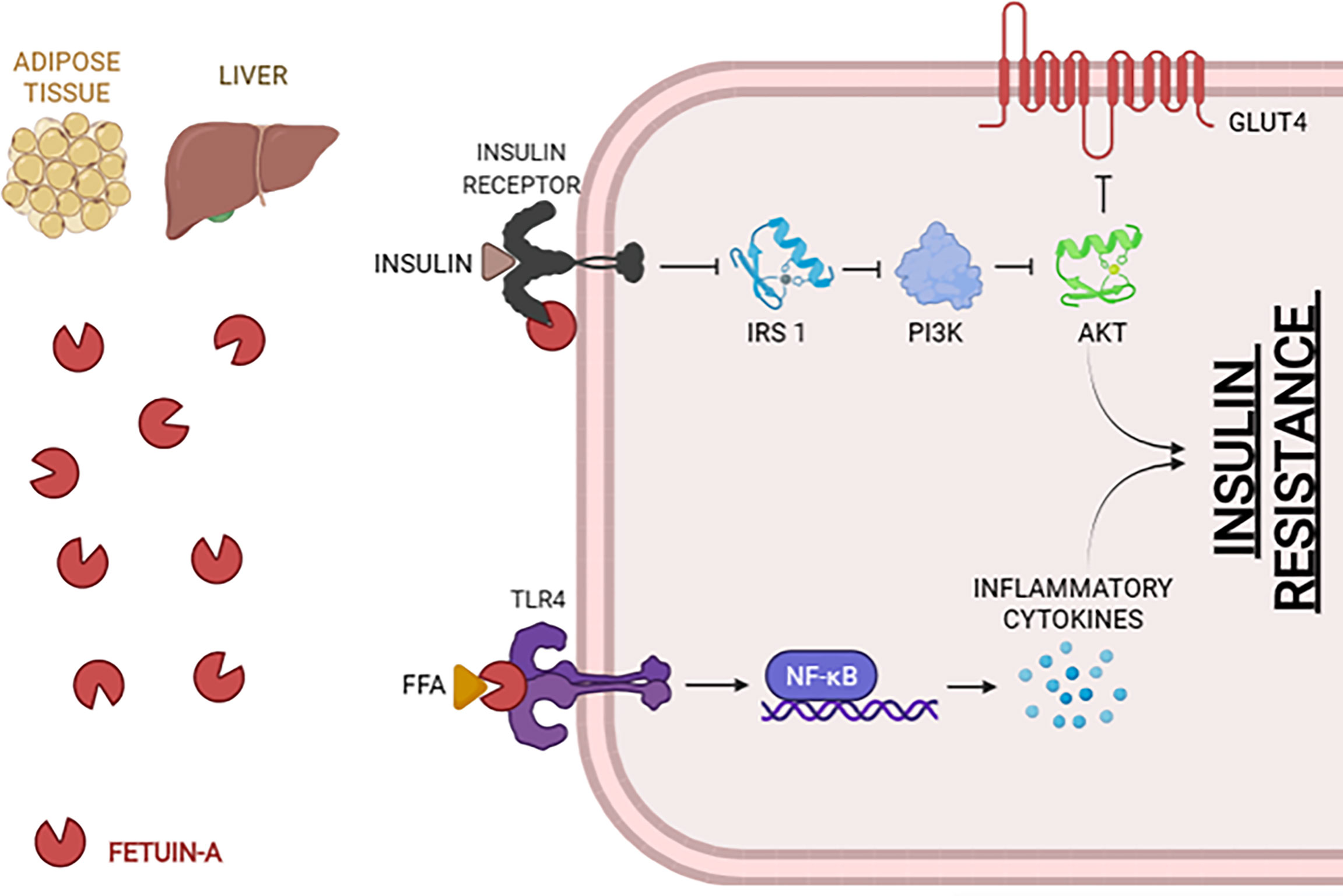
Figure 3 Mechanisms of Fetuin-A in order to promote insulin resistance. The association of Fetuin-A with the insulin receptor inhibits IRS1 and, therefore, the cascade that will block GLUT4. Via TLR4, Fetuin-A will activate the NF-kB genes, which will promote the secretion of inflammatory cytokines and lead to insulin resistance. FFA, Free Fatty Acids; NF-kB, Nuclear Factor Kappa-light-chain-enhancer of activated B cells; IRS1, Insulin Receptor Substrate 1; PI3K, Phosphoinositide 3-Kinases; AKT, Protein kinase B; GLUT4, Glucose transporter type 4. (Created with BioRender.com).
In the case-cohort study performed by Stefan et al. in 2008 (39), a considerable number of individuals (n= 2500) were randomly selected within the European Prospective Investigation into Cancer and Nutrition (EPIC)-Potsdam study. Of the 2500 individuals, 2,164 were nondiabetic at baseline. After 7 years follow-up, a total of 703 were subject to analysis. The results indicated that Fetuin-A was an independent risk factor for Type 2 Diabetes. Circulating Fetuin-A levels remained positively correlated to diabetic risk even after adjustment for sex, BMI, waist circumference, and lifestyle risk factors (39).
A study performed in eastern India by Dutta and colleagues included the quantification of Fetuin-A in 2119 individuals. The main observation was that progressors had higher levels of Fetuin‐A (40). The same observation was described in another case-cohort, the Nurses’ Health Study (NHS; 470 cases), where individuals with higher levels of Fetuin-A had the highest risk of developing diabetes (41).
Though in a more advanced investigation stage than the other biomarkers, Fetuin-A has revealed some controversial results regarding the association with diabetic complications like cardiac pathologies (42, 43).
Discussion and Conclusion
Type 2 Diabetes is a complex pathology with high prevalence and morbidity rates. We believe that the identification of new metabolites able to translate diabetic progression will significantly decrease the prevalence of T2D. Our present work focused on potential metabolites with limited associated studies but with promising results.
The background knowledge was a previous work which identified the most popular biomarkers, the most likely to endorse the diagnostic clinical routine in T2D prevention (14). We recognized that such could be, by itself, a limitation to our study. Moreover, due to the nature of the publication, we did not assess publication bias or strength of evidence of the included articles. To present a more embracing review, we summarize our study in Table 1.
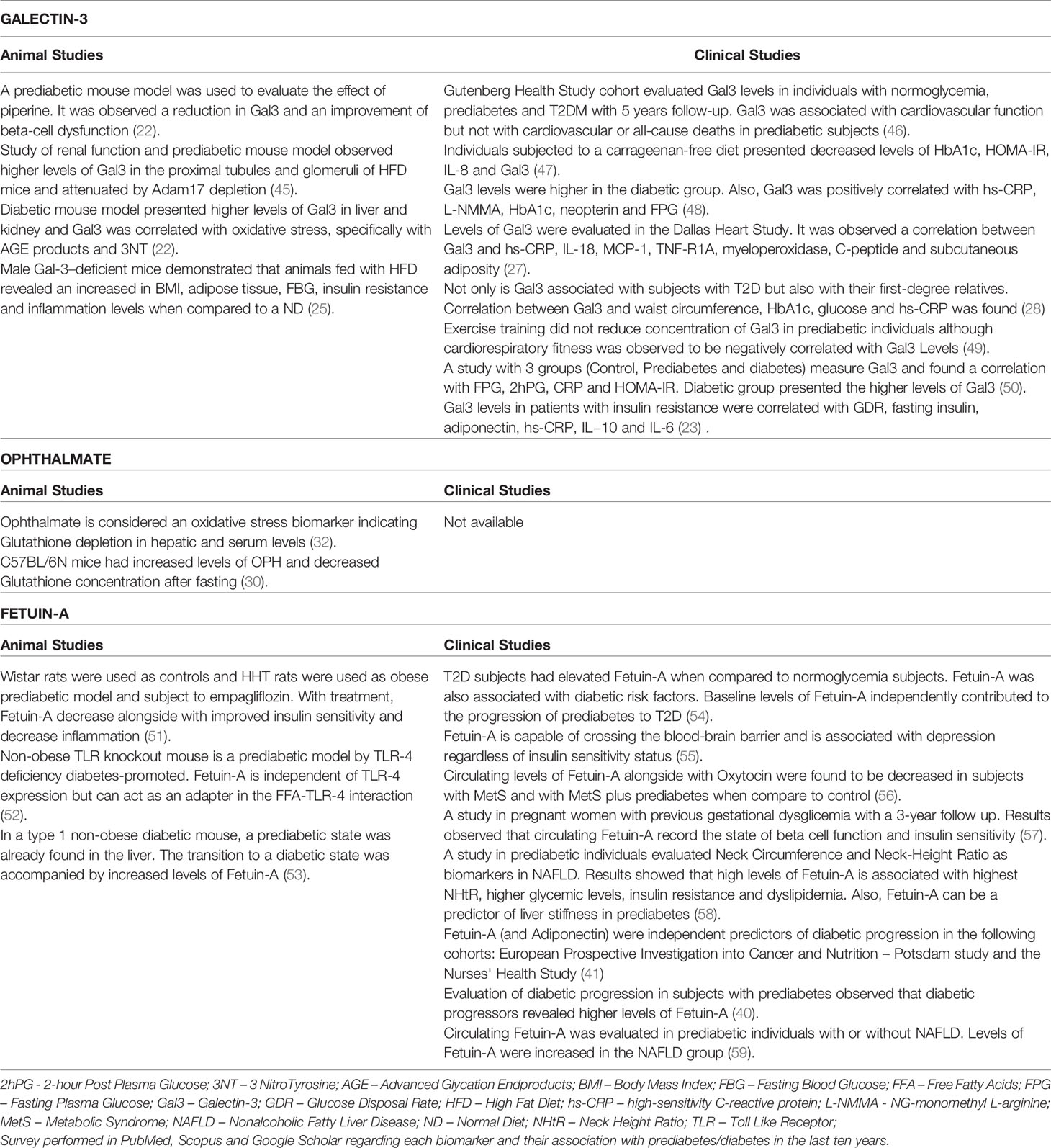
Table 1 Description of the main results of original articles of Galectin-3; Ophthalmate; Fetuin-A in animal and clinical studies.
All displayed biomarkers have a correlation with one or more pathways diabetes-associated such as oxidative stress, insulin resistance, and impaired glucose metabolism. Galectin-3 is associated with pathologies connected to fibrosis, inflammation and oxidative stress. Ophthalmate associated with glutathione metabolism, and therefore oxidative stress and Fetuin-A is associated with calcification, inflammation, and insulin resistance. A broad analysis of the three biomarkers (Table 1) uncover that ophthalmate is still in an early research stage. However, Galectin-3 and Fetuin-A are already under investigation in human cohorts, evidencing the potential to become new biomarkers of prediabetes.
The main objective of our study was to assess the importance of underestimated biomarkers and expose the results obtained until now. The importance of implementing new screening methods in routine clinical practice will definitely improve prediabetes diagnosis. An early diagnosis will allow for well-timed lifestyle alterations, minimizing pharmacological drugs and alleviating national health systems. Moreover, tracking metabolome alterations will unravel new therapeutical targets. A controlled hyperglycemic status will help avoid other diabetes-associated comorbidities, like cardiovascular, neuropathy, nephropathy, retinopathy diseases, among others.
Author Contributions
Conceptualization: RF and PB. Writing—original draft preparation: CL. Writing—review and editing: CL, PB, RS, and RF. Supervision: RF and RS. All authors contributed to the article and approved the submitted version.
Funding
This work was supported by FCT – Fundação para a Ciência e Tecnologia (REF UID/BIM/04293/2013) and by the following scholarships: (Ref. SAICT2016/FEDER/BIO4DIA/BTI) and (SFRH/BD/146489/2019).
Conflict of Interest
The authors declare that the research was conducted in the absence of any commercial or financial relationships that could be construed as a potential conflict of interest.
Publisher’s Note
All claims expressed in this article are solely those of the authors and do not necessarily represent those of their affiliated organizations, or those of the publisher, the editors and the reviewers. Any product that may be evaluated in this article, or claim that may be made by its manufacturer, is not guaranteed or endorsed by the publisher.
Acknowledgments
The authors would also like to acknowledge the support from Nuno Meireles, Secretariat office of LABMI-PORTIC, Laboratory of Medical & Industrial Biotechnology, Porto Research, Technology and Innovation Center, Porto Polytechnic.
References
1. Chatterjee S, Khunti K, Davies MJ. Type 2 Diabetes. Lancet (2017) 389(10085):2239–51. doi: 10.1016/S0140-6736(17)30058-2
2. Federation, International Diabetes. IDF Diabetes Atlas 9th Edition. In: International Diabetes Federation Diabetes Atlas, Ninth Edition, vol. 1. International Diabetes Federation (2019). p. 10–5.
3. Galicia-Garcia U, Benito-Vicente A, Jebari S, Larrea-Sebal A, Siddiqi H, Uribe KB, et al. And César Martín. 2020. “Pathophysiology of Type 2 Diabetes Mellitus. Int J Mol Sci (2020) 21:1–34. doi: 10.3390/ijms21176275
4. Kolb H, Mandrup-Poulsen T. The Global Diabetes Epidemic as a Consequence of Lifestyle-Induced Low-Grade Inflammation. Diabetologia (2010) 53(1):10–20. doi: 10.1007/s00125-009-1573-7
5. Esser N, Legrand-Poels S, Piette J, Scheen AJ, Paquot N. Inflammation as a Link Between Obesity, Metabolic Syndrome and Type 2 Diabetes. Diabetes Res Clin Pract (2014) 105(2):141–50. doi: 10.1016/j.diabres.2014.04.006
6. Coelho M, Oliveira T, Fernandes R. Biochemistry of Adipose Tissue: An Endocrine Organ. Arch Med Sci (2013) 9(2):191–200. doi: 10.5114/aoms.2013.33181
7. Felber JP, Ferrannini E, Golay A, Meyer HU, Theibaud D, Curchod B, et al. Role of Lipid Oxidation in Pathogenesis of Insulin Resistance of Obesity and Type II Diabetes. Diabetes (1987) 36(11):1341–50. doi: 10.2337/diab.36.11.1341
8. Hue L, Maisin L, Rider MH. Palmitate Inhibits Liver Glycolysis. Involvement of Fructose 2,6-Bisphosphate in the Glucose/Fatty Acid Cycle. Biochem J (1988) 251(2):541–5. doi: 10.1042/bj2510541
9. Boden G. Role of Fatty Acids in the Pathogenesis of Insulin Resistance and NIDDM. Diabetes (1997) 46(1):3–10. doi: 10.2337/diab.46.1.3
10. Randle PJ. Regulatory Interactions Between Lipids and Carbohydrates: The Glucose Fatty Acid Cycle After 35 Years. Diabetes/Metabolism Rev (1998) 14(4):263–83. doi: 10.1002/(SICI)1099-0895(199812)14:4<263::AID-DMR233>3.0.CO;2-C
11. Gillery P. Oxidative Stress and Protein Glycation in Diabetes Mellitus. Annales Biol Clin (2006) 64:(4).
12. Finaud J, Lac G, Filaire E. Oxidative Stress: Relationship With Exercise and Training. Sports Med (2006) 36(4):327–58. doi: 10.2165/00007256-200636040-00004
13. Segers K, Declerck S, Mangelings D, Vander Heyden Y, Van Eeckhaut A. Analytical Techniques for Metabolomic Studies: A Review. Bioanalysis (2019) 11(24):2297–318. doi: 10.4155/bio-2019-0014
14. Luís C, Baylina P, Soares R, Fernandes R. Metabolic Dysfunction Biomarkers as Predictors of Early Diabetes. Biomolecules (2021) 11(1589):1–15. doi: 10.3390/biom11111589
15. Hsu DK, Dowling CA, George Jeng K, Chen J-tC, Yang R-y, Liu F-T. Galectin-3 Expression Is Induced in Cirrhotic Liver and Hepatocellular Carcinoma. Int J Cancer (1999) 81:519–26. doi: 10.1002/(SICI)1097-0215(19990517)81:4<519::AID-IJC3>3.0.CO;2-0
16. Funasaka T, Raz A, Nangia-Makker P. Galectin-3 in Angiogenesis and Metastasis. Glycobiology (2014) 24(10):886–91. doi: 10.1093/glycob/cwu086
17. Park S-H, Sook Min H, Kim B, Myung J, Ha Paek S. Galectin-3: A Useful Biomarker for Differential Diagnosis of Brain Tumors. Neuropathol : Off J Jpn Soc Neuropathol (2008) 28(5):497–506. doi: 10.1111/j.1440-1789.2008.00909.x
18. Henderson NC, Sethi T. The Regulation of Inflammation by Galectin-3. Immunol Rev (2009) 230(1):160–71. doi: 10.1111/j.1600-065X.2009.00794.x
19. Liu F-T. Galectins: A New Family of Regulators of Inflammation. Clin Immunol (2000) 97(2):79–88. doi: 10.1006/clim.2000.4912
20. Rabinovich GA, Rubinstein N, Toscano MA. Role of Galectins in Inflammatory and Immunomodulatory Processes. Biochim Biophys Acta (2002) 1572(2-3):274–84. doi: 10.1016/S0304-4165(02)00314-8
21. Pugliese G, Iacobini C, Ricci C, Blasetti Fantauzzi C, Menini S. Galectin-3 in Diabetic Patients. Clin Chem Lab Med : CCLM/FESCC (2014) 52(10):1413–23. doi: 10.1515/cclm-2014-0187
22. Luís C, Costa R, Rodrigues I, Castela Â, Coelho P, Guerreiro S, et al. Xanthohumol and 8-Prenylnaringenin Reduce Type 2 Diabetes–Associated Oxidative Stress by Downregulating Galectin-3. Porto Biomed J (2019) 4(1):1–7. doi: 10.1016/j.pbj.0000000000000023
23. Ohkura T, Fujioka Y, Nakanishi R, Shiochi H, Sumi K, Yamamoto N, et al. Low Serum Galectin-3 Concentrations Are Associated With Insulin Resistance in Patients With Type 2 Diabetes Mellitus. Diabetol Metab Syndrome (2014) 6(1):1. doi: 10.1186/1758-5996-6-106
24. Menini S, Iacobini C, Blasetti Fantauzzi C, Pesce CM, Pugliese G. Role of Galectin-3 in Obesity and Impaired Glucose Homeostasis. Oxid Med Cell Longevity Hindawi Publishing Corporation (2016) 2016:1–7. doi: 10.1155/2016/9618092
25. Pejnovic NN, Pantic JM, Jovanovic IP, Radosavljevic GD, Milovanovic MZ, Nikolic IG, et al. Galectin-3 Deficiency Accelerates High-Fat Diet-Induced Obesity and Amplifies Inflammation in Adipose Tissue and Pancreatic Islets. Diabetes (2013) 62(6):1932–44. doi: 10.2337/db12-0222
26. Weigert J, Neumeier M, Wanninger J, Bauer S, Farkas S, Scherer MN, et al. Serum Galectin-3 Is Elevated in Obesity and Negatively Correlates With Glycosylated Hemoglobin in Type 2 Diabetes. J Clin Endocrinol Metab (2010) 95(3):1404–11. doi: 10.1210/jc.2009-1619
27. Vora A, de Lemos JA, Ayers C, Grodin JL, Lingvay I. Association of Galectin-3 With Diabetes Mellitus in the Dallas Heart Study. J Clin Endocrinol Metab (2019) 104(10):4494–58. doi: 10.1210/jc.2019-00398
28. Abdella NA, Mojiminiyi OA. 547-P: Utility of Galectin-3 as a Biomarker of Prediabetes and Complications of Type 2 Diabetes Mellitus. Redox Biol (2019) 46:1–13. doi: 10.2337/db19-547-P
29. Cliffe EE, Waley SG. Acidic Peptides of the Lens. 4. The Biosynthesis of Ophthalmic Acid. Biochem J (1958) 69(4):649–55. doi: 10.1042/bj0690649
30. Kobayashi S, Lee J, Takao T, Fujii J. Increased Ophthalmic Acid Production Is Supported by Amino Acid Catabolism Under Fasting Conditions in Mice. Biochem Biophys Res Commun (2017) 491(3):649–55. doi: 10.1016/j.bbrc.2017.07.149
31. Ables GP. Amino Acids | Sulfur Amino Acid-Restricted Diets: Mechanisms and Health Benefits. In: Encyclopedia of Biological Chemistry III. Elsevier (2021). Available at: 10.1016/b978-0-12-819460-7.00061-x.
32. Soga T, Baran R, Suematsu M, Ueno Y, Ikeda S, Sakurakawa T, et al. Differential Metabolomics Reveals Ophthalmic Acid as an Oxidative Stress Biomarker Indicating Hepatic Glutathione Consumption. J Biol Chem (2006) 281(24):16768–76. doi: 10.1074/jbc.M601876200
33. Lee J, Eun Sil Kang S, Homma T, Sato H, Geuk Seo H, Fujii J. The Viability of Primary Hepatocytes Is Maintained Under a Low Cysteine-Glutathione Redox State With a Marked Elevation in Ophthalmic Acid Production. Exp Cell Res (2017) 361(1):178–91. doi: 10.1016/j.yexcr.2017.10.017
34. Kaur G, Leslie EM, Tillman H, Lee WM, Swanlund DP, Karvellas CJ, et al. Detection of Ophthalmic Acid in Serum From Acetaminophen-Induced Acute Liver Failure Patients Is More Frequent in Non-Survivors. PloS One (2015) 10(9):1–10. doi: 10.1371/journal.pone.0139299
35. Trepanowski JF, Mey J, Varady KA. Fetuin-A: A Novel Link Between Obesity and Related Complications. Int J Obes (2015) 39(5):734–41. doi: 10.1038/ijo.2014.203
36. Mori K, Emoto M, Inaba M. Fetuin-A: A Multifunctional Protein. Recent Patents Endocr Metab Immune Drug Discovery (2011) 5(2):124–46. doi: 10.2174/187221411799015372
37. Pal D, Dasgupta S, Kundu R, Maitra S, Das G, Mukhopadhyay S, et al. Fetuin-A Acts as an Endogenous Ligand of TLR4 to Promote Lipid-Induced Insulin Resistance. Nat Med (2012) 18(8):1279–85. doi: 10.1038/nm.2851
38. Dasgupta S, Bhattacharya S, Biswas A, Majumdar SS, Mukhopadhyay S, Ray S, et al. NF-Kb Mediates Lipid-Induced Fetuin-A Expression in Hepatocytes That Impairs Adipocyte Function Effecting Insulin Resistance. Biochem J (2010) 429(3):451–62. doi: 10.1042/BJ20100330
39. Stefa N, Fritsche A, Weikert C, Boeing H, Georg Joost H, Ulrich Häring H, et al. Plasma Fetuin-A Levels and the Risk of Type 2 Diabetes. Diabetes (2008) 57(10):2762–7. doi: 10.2337/db08-0538
40. Dutta D, Mondal SA, Kumar M, Hasanoor Reza AH, Biswas D, Singh P, et al. Serum Fetuin-A Concentration Predicts Glycaemic Outcomes in People With Prediabetes: A Prospective Study From Eastern India. Diabetic Med (2014) 31(12):1594–9. doi: 10.1111/dme.12539
41. Stefan N, Sun Q, Fritsche A, Machann J, Schick F, Gerst F, et al. Impact of the Adipokine Adiponectin and the Hepatokine Fetuin-A on the Development of Type 2 Diabetes: Prospective Cohort- and Cross-Sectional Phenotyping Studies. PloS One (2014) 9(3):1–8. doi: 10.1371/journal.pone.0092238
42. Weikert C, Stefan N, Schulze MB, Pischon T, Berger K, Georg Joost H, et al. And Andreas Fritsche. 2008. Plasma Fetuin-A Levels and the Risk of Myocardial Infarction and Ischemic Stroke. Circulation (2008) 118:2555–62. doi: 10.1161/CIRCULATIONAHA.108.814418
43. Sun Q, Jiménez MC, Townsend MK, Rimm EB, Manson JAE, Albert CM, et al. Plasma Levels of Fetuin-A and Risk of Coronary Heart Disease in US Women: The Nurses’ Health Study. J Am Heart Assoc (2014) 3(3):1–10. doi: 10.1161/JAHA.114.000939
44. Yuan Y, Zhou J, Hu R, Zou L, Ji L, Jiang G. Piperine Protects Against Pancreatic β-Cell Dysfunction by Alleviating Macrophage Inflammation in Obese Mice. Life Sci (2021) 274(June):119312. doi: 10.1016/j.lfs.2021.119312
45. Palau V, SVillanueva J, Benito D, Márquez E, Rodríguez E, José Soler M, et al. Redefining the Role of ADAM17 in Renal Proximal Tubular Cells and Its Implications in an Obese Mouse Model of Pre-Diabetes. Int J Mol Sci (2021) 22(23):1–5. doi: 10.3390/ijms222313093
46. Schmitt VH, Prochaska JH, Föll AS, Schulz A, Keller K, Hahad O, et al. Galectin-3 for Prediction of Cardiac Function Compared to NT-ProBNP in Individuals With Prediabetes and Type 2 Diabetes Mellitus. Sci Rep (2021) 11(1):19012. doi: 10.1038/s41598-021-98227-x
47. Feferman L, Bhattacharyya S, Oates E, Haggerty N, Wang T, Varady K, et al. Carrageenan-Free Diet Shows Improved Glucose Tolerance and Insulin Signaling in Prediabetes: A Randomized, Pilot Clinical Trial. J Diabetes Res (2020) 2020:8267980. doi: 10.1155/2020/8267980
48. Atalar MN, Abuşoğlu S, Unlu A, Tok O, Hilmi İpekçi S, Baldane S, et al. Assessment of Serum Galectin-3, Methylated Arginine and Hs-CRP Levels in Type 2 Diabetes and Prediabetes. Life Sci (2019) 231(August):116577. doi: 10.1016/j.lfs.2019.116577
49. Andonian BJ, Bartlett DB, Huebner JL, Willis L, Hoselton A, Kraus VB, et al. Effect of High-Intensity Interval Training on Muscle Remodeling in Rheumatoid Arthritis Compared to Prediabetes. Arthritis Res Ther (2018) 20(1):283. doi: 10.1186/s13075-018-1786-6
50. Yilmaz H, Cakmak M, Inan O, Darcin T, Akcay A. Increased Levels of Galectin-3 Were Associated With Prediabetes and Diabetes: New Risk Factor? J Endocrinol Invest (2015) 38(5):527–33. doi: 10.1007/s40618-014-0222-2
51. Hüttl M, Markova I, Miklankova D, Zapletalova I, Poruba M, Haluzik M, et al. In a Prediabetic Model, Empagliflozin Improves Hepatic Lipid Metabolism Independently of Obesity and Before Onset of Hyperglycemia. Int J Mol Sci (2021) 22(21):11513. doi: 10.3390/ijms222111513
52. Simon MC, Lena Reinbeck A, Wessel C, Heindirk J, Jelenik T, Kaul K, et al. Distinct Alterations of Gut Morphology and Microbiota Characterize Accelerated Diabetes Onset in Nonobese Diabetic Mice. J Biol Chem (2020) 295(4):969–80. doi: 10.1074/jbc.RA119.010816
53. Jelenik T, Séquaris G, Kaul K, Ouwens DM, Phielix E, Kotzka J, et al. Tissue-Specific Differences in the Development of Insulin Resistance in a Mouse Model for Type 1 Diabetes. Diabetes (2014) 63(11):3856–67. doi: 10.2337/db13-1794
54. Susairaj P, Snehalatha C, Nanditha A, Satheesh K, Raghavan A, Vinitha R, et al. Analysis of an Indian Diabetes Prevention Programme on Association of Adipokines and a Hepatokine With Incident Diabetes. Sci Rep (2021) 11(1):1–9. doi: 10.1038/s41598-021-99784-x
55. Mancuso E, Spiga R, Rubino M, Averta C, Rotundo, Segura-Garcìa C, et al. Effects of Alpha-2-HS-Glycoprotein on Cognitive and Emotional Assessment in Prediabetic and Diabetic Subjects. J Affect Disord (2021) 282:700–6. doi: 10.1016/j.jad.2020.12.135
56. Kasabri V, Shawakri E, Akour A, Naffa R, Khawaja N, Al-Sarraf I, et al. Cross-Sectional Correlates of Increased IL-18 But Reduced Fetuin-A and Oxytocin With Adiposity and Blood Indices in Metabolic Syndrome Patients With and Without Prediabetes. Ther Adv Endocrinol Metab (2018) 9(12):329–38. doi: 10.1177/2042018818788802
57. Pinnaduwage L, Ye C, Hanley AJ, Connelly PW, Sermer M, Zinman B, et al. Changes Over Time in Hepatic Markers Predict Changes in Insulin Sensitivity, β-Cell Function, and Glycemia. J Clin Endocrinol Metab (2018) 103(7):2651–59. doi: 10.1210/jc.2018-00306
58. Mondal SA, Dutta D, Kumar M, Singh P, Basu M, Selvan C, et al. Neck Circumference to Height Ratio Is a Reliable Predictor of Liver Stiffness and Nonalcoholic Fatty Liver Disease in Prediabetes. Indian J Endocrinol Metab (2018) 22(3):347–54. doi: 10.4103/ijem.IJEM_31_18
Keywords: diabetes, biomarkers, early diagnosis, galectin-3, ophthalmate, fetuin-A, prediabetes
Citation: Luís C, Soares R, Baylina P and Fernandes R (2022) Underestimated Prediabetic Biomarkers: Are We Blind to Their Strategy? Front. Endocrinol. 13:805837. doi: 10.3389/fendo.2022.805837
Received: 31 October 2021; Accepted: 31 January 2022;
Published: 07 March 2022.
Edited by:
Jared Rutter, The University of Utah, United StatesReviewed by:
Hongshuo Shi, Shandong University of Traditional Chinese Medicine, ChinaAlexander E. Berezin, Zaporizhia State Medical University, Ukraine
Copyright © 2022 Luís, Soares, Baylina and Fernandes. This is an open-access article distributed under the terms of the Creative Commons Attribution License (CC BY). The use, distribution or reproduction in other forums is permitted, provided the original author(s) and the copyright owner(s) are credited and that the original publication in this journal is cited, in accordance with accepted academic practice. No use, distribution or reproduction is permitted which does not comply with these terms.
*Correspondence: Carla Luís, Y2FybGFsdWlzQG1lZC51cC5wdA==; Rúben Fernandes, cmZlcm5hbmRlc0Blc3MuaXBwLnB0