- 1German Center for Diabetes Research (DZD e. V.), Neuherberg, Germany
- 2Institute of Diabetes Research and Metabolic Disease (IDM) of the Helmholtz Center Munich, University of Tübingen, Tübingen, Germany
- 3Department of Internal Medicine IV, Endocrinology, Diabetology and Nephrology, University Hospital Tübingen, Tübingen, Germany
- 4Department of Psychiatry and Psychotherapy, Charité Universitätsmedizin, Berlin, Germany
- 5Department of Pediatrics and Adolescent Medicine, University Medical Center Ulm, Ulm, Germany
- 6Division of Pediatric Endocrinology and Diabetes, Department of Pediatrics and Adolescent Medicine, University Center Ulm, Ulm, Germany
- 7Experimental and Clinical Research Center, Charité Universitätsmedizin Berlin and Max Delbruck Center for Molecular Medicine, Berlin, Germany
- 8Berlin Institute of Health, Berlin, Germany
- 9Institute for Diabetes and Obesity, Helmholtz Diabetes Center, Helmholtz Zentrum München, Neuherberg, Germany
- 10Department for Diagnostic Laboratory Medicine, Institute for Clinical Chemistry and Pathobiochemistry, University Hospital Tübingen, Tübingen, Germany
- 11Institute of Aerospace Medicine, German Aerospace Center (DLR), Cologne, Germany
- 12Chair of Aerospace Medicine, Medical Faculty, University of Cologne, Cologne, Germany
- 13Department of Diabetes, Life Sciences & Medicine Cardiovascular Medicine & Sciences, Kings College London, London, United Kingdom
Introduction: Atrial natriuretic peptide (ANP), a hormone secreted from the heart, controls cardiovascular and renal functions including arterial blood pressure and natriuresis. ANP also exerts metabolic effects in adipose tissue, liver and skeletal muscle, and interacts with the secretion of adipokines. We tested the hypothesis that ANP lowers concentrations of the anorexigenic adipokine leptin in healthy humans in vivo.
Methods: Human ANP or matching placebo was infused intravenously (iv) into healthy men in a controlled clinical trial.
Results: Within 135 minutes of iv ANP infusion, we observed an acute decrease in plasma leptin levels compared to controls. Free fatty acids markedly increased with ANP infusion in vivo, indicating activated lipolysis. In human SGBS adipocytes, ANP suppressed leptin release.
Discussion: The study shows that the cardiac hormone ANP reduces the levels of the anorexigenic adipokine leptin in healthy humans, providing further support for ANP as a cardiomyokine in a heart - adipose tissue axis. (registered in the German Clinical Trials Register and the WHO International Clinical Trials Registry Platform was granted under DRKS00024559)
Introduction
Atrial natriuretic peptide (ANP), a hormone of the natriuretic peptide family, has a well-established role in blood pressure and volume regulation (1, 2). Moreover, others and we have shown that ANP also exerts metabolic effects, such as enhancing lipolysis, lipid oxidation and mitochondrial respiration (3). ANP mediates the browning of white adipose tissue and has been implicated in the release of adipokines, including adiponectin (4, 5). In congestive heart failure as well as upon exercise, ANP concentrations increase, while in conditions such as obesity, insulin resistance, and type 2 diabetes, ANP levels decrease (1). Leptin, released by adipocytes is an anorexigenic hormone with glucose-lowering, insulin-sensitizing, and catabolic properties (6). In obesity, leptin levels increase, while they decrease during physical exercise. A combining humoral factor capable of mediating both of these effects is unknown.
In-vitro and ex-vivo studies as well as animal models (7, 8) suggested that ANP is able to reduce leptin levels from adipose tissue. Whether or not these findings translate into humans, remains unknown. Here, we tested the hypothesis that ANP reduces leptin concentrations in a post-hoc analysis of a placebo controlled, clinical trial involving healthy humans. To better understand the underlying mechanisms, we also applied ANP on human immortalized SGBS adipocytes (9).
Material and methods
Infusion studies in humans
We obtained approval by the local ethics committee and written consent from study participants for all procedures prior to the experiment. 11 healthy men without any medications underwent an overnight fast, after which we catheterized the large antecubital veins of both arms to allow for separate infusion and sampling access. After a rest period of at least 60 minutes, human ANP was infused for 135 minutes at a maximum rate of 25 ng/kg/min as previously described (10, 11). Plasma leptin concentrations were analyzed from blood drawings at baseline and at the end of ANP or placebo iv infusion at 135 mins. Plasma leptin levels were measured using ELISA (Merck Chemicals GmbH, Darmstadt, Germany). As control, 6 age- and BMI-matched healthy men underwent the same treatment, but with a placebo infusion of isotonic saline. The infusion study and leptin measurements were conducted between 2005-2007, data were analyzed in 2020 and 2021. Results of the initial trial have been reported previously (10, 11). A registration of the analysis reported here in the German Clinical Trials Register and the WHO International Clinical Trials Registry Platform was granted under DRKS00024559.
Stimulation studies in SGBS cells
We cultured Simpson-Golabi-Behmel syndrome (SGBS) cells as described previously (9). After 14 day of adipogenic differentiation, cultures were treated with 10 nM human ANP (Clinalfa-Bachem #H-2095) or vehicle (PBS) in serum-free basal medium (DMEM:F12 with 3.3 mM biotin, 1.7 mM panthotenate, and antibiotics). After 24, 48, and 72 h, we collected media supernatants and either extracted total RNA or lysed cells for protein extraction. We performed RT-qPCR analysis as described previously (12). Primer sequences are available on request. Protein lysates and media supernatants were subjected to Western blot analysis as described (12) using the following antibodies: anti-β-actin (Merck #A5441), anti-leptin (Biovendor, # RD181001220).
Statistics
Statistics were carried out using IBM SPSS Statistics Version 25. Graphs were made using GraphPad Prism 6. For parametric assessment of baseline confounders between the groups, as well as plasma leptin levels between time points 0 and 135 min in the ANP group, we used 2-tailed student’s t-test and standard deviation. For comparison of plasma leptin levels, we calculated a generalized linear model using an identity link function under a normal distribution for the leptin delta between the two groups. To assess goodness of fit and model superiority, we used Pearson Chi-Square test and Omnibus test. Wald confidence interval and Wald Chi-square metric were used for variance analysis. To assess dose dependency of plasma leptin decrease on ANP level, we calculated the Pearson correlation using a 1-tailed test of significance. Confidence levels were set at 95% and significance was assumed at α = 0.05. mRNA expression in SGBS cells was assessed using the ΔCt method, with HPRT used as reference gene. We compared mRNA levels and protein densitometry values between the groups using two-sided t-test.
Results
ANP infusion lowers leptin levels in healthy men compared to placebo
Participants in the ANP and placebo (PLC) group did not differ in age, BMI (PLC 23.0 ± 1.1 Kg/m2 vs ANP 24.1 ± 0.5, p=0.21) and basal leptin levels. ANP infusion over 135 minutes led to a mean increase in plasma ANP levels by 405.98 pg/ml (p < 0.001 compared to baseline, data not shown), a concentration in the high pathophysiological range observed in heart failure. ANP or PLC infusion over 135 mins did not result in any adverse events. Blood pressure did not change with PLC infusion and decreased with ANP infusion (Systolic 116 ± 3 mm Hg to 110 ± 3 mm Hg; Diastolic 63 ± 2 mmHg to 58 ± 2 mm Hg, both p<0.01) as reported previously (10, 13), in accordance with the vasodilatory and sodium excreting effect of ANP. After infusion of ANP or PLC over 135 minutes, leptin levels were lower in the ANP group compared to the PLC group (B = -0.201, 95% Wald CI [-0.376, -0.026] vs. B = 0.044, 95% Wald CI [-0.097, 0.185] for ANP vs. placebo, respectively; p = 0.024; Figure 1A). Assessing the ANP-treated group for dose-dependency of the relation between ANP and leptin levels, we observed a trend for an inverse correlation between ANP and leptin levels (Kendall’s Tau-b = -0.367, p = 0.059; Figure 1B). Simultaneously, we observed a strong and highly significant increase in plasma free fatty acid (FFA) levels in the ANP-treated group over time (300.51 ± 105.12 µmol/l at 0 min vs. 588.13 ± 198.37 µmol/l at 135 min, p < 0.001), indicative of activation of lipolysis, confirming previous work (1, 8, 10, 13).
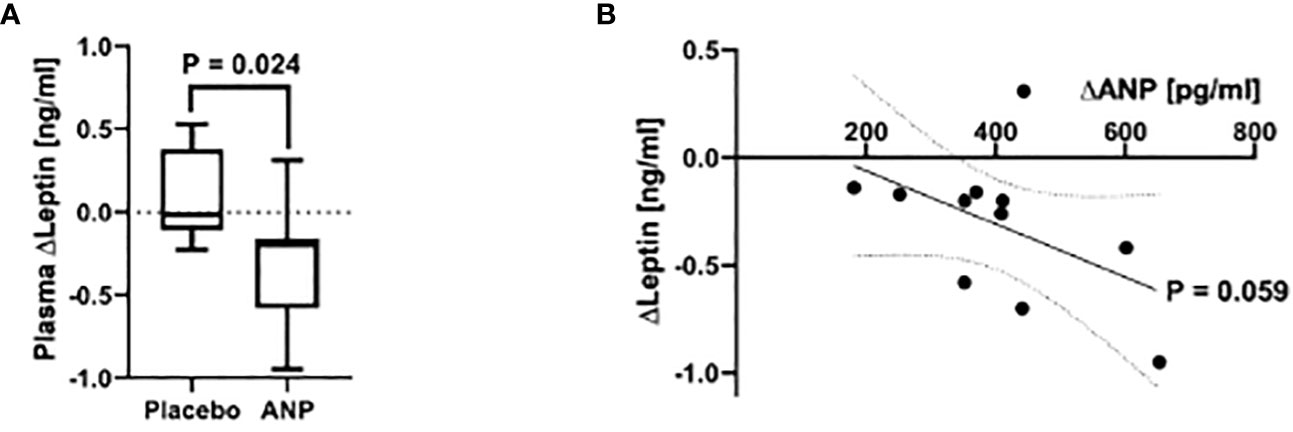
Figure 1 ANP reduces plasma leptin levels in healthy men. (A) Plasma leptin levels after acute i.v. infusion of ANP or Placebo. After infusion of ANP over 135 minutes, participants in the treatment group showed a significantly stronger decrease in plasma leptin concentrations than those in the placebo group (B = -0.201, 95% Wald CI [-0.376, -0.026] vs. B = 0.044, 95% Wald CI [-0.097, 0.185] for ANP vs. placebo, respectively; p = 0.024). (B) Plasma leptin levels in relation to ANP concentrations after infusion. Plasma ANP concentration showed a trend to be associated with a decrease in leptin levels in a dose-dependent manner, as shown by an inverse correlation (slope: -0.001nm/pg, Kendall’s Tau-b = -0.367, p = 0.059).
Treatment of a human adipocyte cell line with ANP reduced leptin secretion
Leptin is an adipocyte-derived hormone. Therefore, we aimed to understand by which mechanism ANP reduces leptin levels. Using human SGBS adipocytes, an in vitro model of human adipocyte biology (9, 12), we observed no effect of ANP on leptin mRNA and protein expression (Figures 2A-C). However, there was a significant reduction of leptin accumulation in the media supernatants of ANP-treated vs control cells (Figures 2B, D). These suggest that the effect in SGBS cells was mediated by reduced leptin secretion.
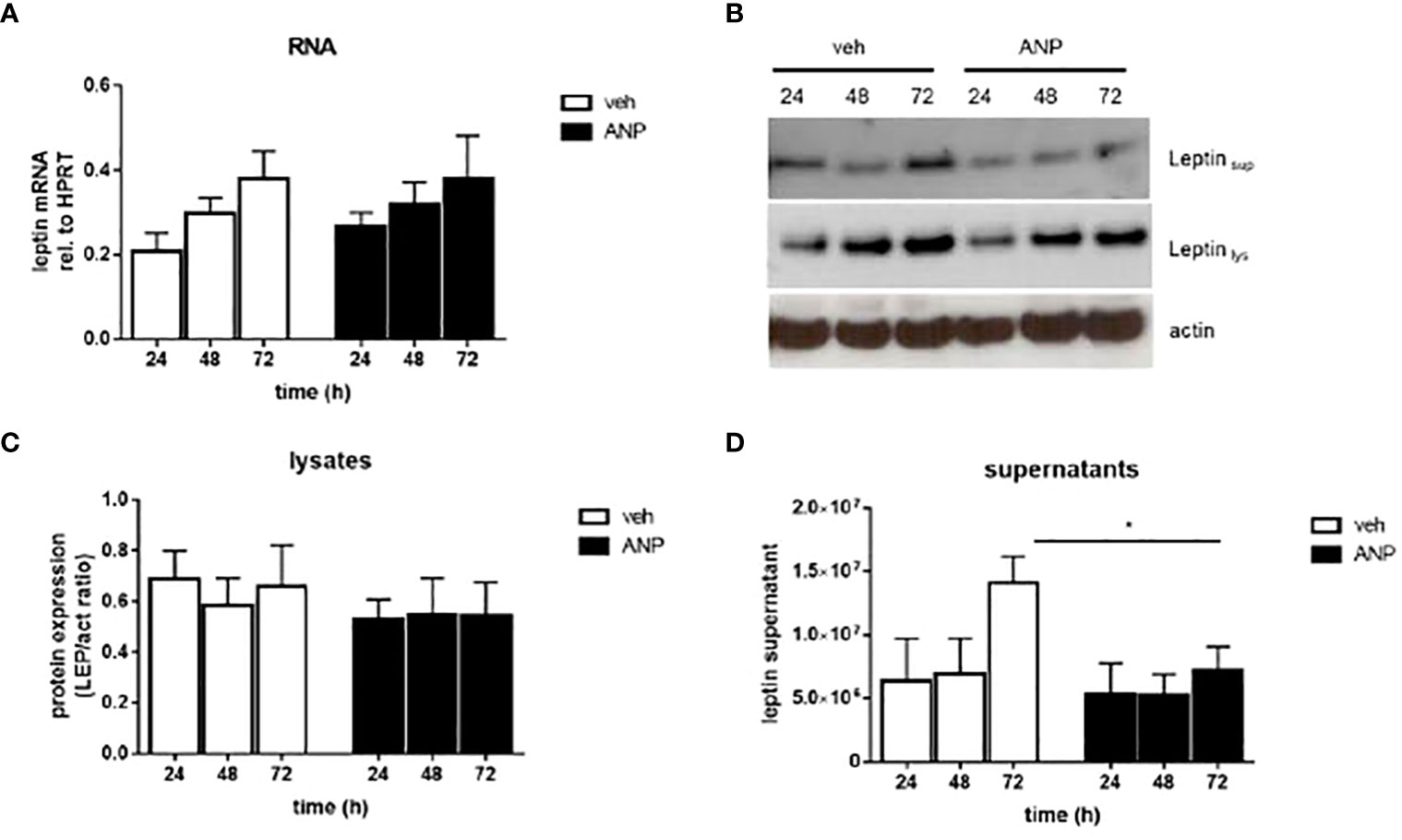
Figure 2 ANP inhibits leptin secretion in human adipocytes. SGBS adipocytes were treated with 10 nM ANP or vehicle in serum-free basal medium. Media supernatants, RNA and protein were harvested after 24, 48, and 72h. (A) The mRNA expression of leptin was assessed by qRT-PCR using the ΔCt method, HPRT was used as reference gene. Mean + SEM of 5 independently performed experiments is shown. (B) Leptin protein expression in cell lysates and media supernatants was determined by Western blot analysis. One representative experiment out of three performed is shown. β-actin was used as loading control. (C, D). Densitometric analysis of Western blot. Mean + SEM of 3 independently performed experiments is shown. *p<0.05.
Discussion
Our results, in a post-hoc analysis of an interventional, placebo-controlled trial, demonstrate acute suppression of leptin levels by ANP in healthy humans. Moreover, our data suggest that the effect of ANP on leptin is dose-dependent. In the human adipocyte cell line SGBS, leptin secretion into the supernatant was lower with ANP treatment compared to vehicle treated cells, but no significant effect on leptin mRNA or intracellular lysate protein expression could be detected. These findings may indicate that ANP reduces leptin secretion, which is in line with previous in vitro and ex vivo studies (4, 5). This effect may potentially be mediated either via cyclic guanosine monophosphate (cGMP), the second messenger of the ANP- receptor NPR-A, or indirectly via free fatty acids, which have also been shown to reduce leptin secretion (14).
Free fatty acids generated during lipolysis are important intracellular signaling molecules inhibiting leptin secretion. Yet, the effect has been shown to involve leptin transcription (15), which we did not observe in SGBS cells. A more likely mechanistic explanation is a cGMP-cAMP crosstalk. While ANP signals via its receptor NPR-A, which is highly expressed on adipocytes, through cGMP, leptin secretion in adipocytes is reduced by increasing levels of cyclic adenosine monophosphate (cAMP). Intracellular levels of cAMP are primarily modulated by phosphodiesterase type 3B (PDE3B), which is highly expressed in adipocytes and hydrolyzes cAMP to 5’-AMP. ANP, via cGMP, inhibits PDE3B, ultimately increasing cAMP concentrations and thus, may reduce leptin secretion via this route (16).
While the reduction in leptin levels was detected within 135 minutes in vivo, it took longer to detect a difference in leptin secretion in vitro. Leptin is a 16 kD molecule that is filtered by the renal glomeruli and extensively metabolized and degraded in the renal tubules (17). We suspect that this mechanism may contribute to the different time frame in the in vivo and in vitro studies. Other mechanisms may further contribute.
ANP levels are reduced in patients with obesity (1). ANP levels increase in heart failure and with exercise (3, 18). Interestingly, leptin is increased in persons with obesity and reduced with strong physical exercise (15). While the increase in leptin concentrations in obesity is largely explained by the expansion of adipose tissue mass and leptin resistance, the reduction during exercise is less well understood. Some data suggest that the effect is mediated by improvements in leptin resistance (15), but direct mechanistic insight is lacking. ANP may be a contributing factor.
Interestingly, cross sectional studies in patients with chronic heart failure, a condition with elevated ANP and BNP concentrations, have shown increased leptin levels (19). This observation is likely explained by the fact that obesity, a condition which triggers congestive heart failure, goes along with leptin resistance and increased leptin levels. Leptin, in turn, has been hypothesized to further contribute to the progress of heart failure. From this perspective, our findings may suggest that the heart, by secreting ANP, tries to mitigate the deleterious effects of leptin on its own function. This notion is supported by observations from an inverse relationship between the natriuretic peptide BNP, which signals via the same receptor as ANP, and leptin in the Jackson Heart Study (20).
Hyperleptinemia and leptin resistance are a common finding in patients with obesity and insulin resistance, and a reduction in circulating leptin levels has been suggested recently to be an effective way to increase leptin sensitivity and restore its functional homeostasis in hyperleptinemic individuals, resulting in anti-obesogenic and antidiabetic effects (21). Our observation may therefore suggest that agonism of NPR-A could be an attractive therapeutic strategy to overcome leptin resistance in obesity and T2D. Interestingly, ANP overexpression and infusion in mice has shown beneficial metabolic effects, including protection from diet-induced obesity and insulin resistance. In this regard, enhancing natriuretic peptide signaling in adipose tissue, but not in muscle, protected from diet-induced obesity and insulin resistance (22). Advantages of an NPR-A agonistic compound include beneficial effects on body weight and insulin sensitivity as well as desirable cardiovascular effects, including lowering of arterial blood pressure, and preventing cardiac hypertrophy and fibrosis (1, 23, 24). Clearly, this notion warrants further research in humans.
Limitations of our analysis include the small sample size consisting of male participants, the post-hoc analysis and the lack of further molecular signaling mechanisms. However, the observations of our study are supported by data from cell and animal models (1, 8).
While ANP has already been implicated in a number of metabolic functions, a physiological link to leptin regulation further highlights the important role of this cardiac hormone in metabolic regulation and validates this facet of the heart - adipose axis. Given the well-established blood pressure-lowering, heart failure-protective and beneficial metabolic effects of ANP, agonists of the ANP system appear to be ideal targets for combating cardio - metabolic disease.
Data availability statement
The original contributions presented in the study are included in the article/supplementary material. Further inquiries can be directed to the corresponding author.
Ethics statement
The studies involving human participants were reviewed and approved by Ethics Committee of the Charite-Berlin. The patients/participants provided their written informed consent to participate in this study.
Author contributions
AB, JJ, MB and PF-P designed the experiments. AB and PF-P performed experiments. MD, PF-P and MW analyzed data. MD, PF-P, SF-P, SH, AB wrote the manuscript. AB, SE, MB, TM, AP, MW, JJ, MD, SH, PF-P, and RJvS edited the manuscript. All authors contributed to the article and approved the submitted version.
Funding
TM received research support from the German Research Foundation (DFG-TRR152 and TRR296). PF-P was supported by the Deutsche Forschungsgemeinschaft – Projektnummer 398707781 – Heisenberg professorship. AB received research support from the German Research Foundation (BI1292/10-1, BI1292/9-1, BI1229/12-1, GRK 2816). This work was funded in part by the German Center for Diabetes Research (DZD, 01GI0925). RJvS receives support from the Helmholtz Association by a Helmholtz Young Investigator Group.
Conflict of interest
The authors declare that the research was conducted in the absence of any commercial or financial relationships that could be construed as a potential conflict of interest.
Publisher’s note
All claims expressed in this article are solely those of the authors and do not necessarily represent those of their affiliated organizations, or those of the publisher, the editors and the reviewers. Any product that may be evaluated in this article, or claim that may be made by its manufacturer, is not guaranteed or endorsed by the publisher.
References
1. Ramos HR, Birkenfeld AL, de Bold AJ. INTERACTING DISCIPLINES: cardiac natriuretic peptides and obesity: perspectives from an endocrinologist and a cardiologist. Endocrine Connections (2015) 4:R25–36. doi: 10.1530/EC-15-0018
2. Jordan J, Birkenfeld AL, Melander O, Moro C. Natriuretic peptides in cardiovascular and metabolic crosstalk: implications for hypertension management. Hypertension (2018) 72:270–6. doi: 10.1161/HYPERTENSIONAHA.118.11081
3. Schlueter N, de Sterke A, Willmes DM, Spranger J, Jordan J, Birkenfeld AL. Metabolic actions of natriuretic peptides and therapeutic potential in the metabolic syndrome. Pharmacol Ther (2014) 144:12–27. doi: 10.1016/j.pharmthera.2014.04.007
4. Bordicchia M, Liu D, Amri EZ, Ailhaud G, Dessi-Fulgheri P, Zhang C, et al. Cardiac natriuretic peptides act via p38 MAPK to induce the brown fat thermogenic program in mouse and human adipocytes. J Clin Invest (2012) 122:1022–36. doi: 10.1172/JCI59701
5. Birkenfeld AL, Boschmann M, Engeli S, Moro C, Arafat AM, Luft FC, et al. Atrial natriuretic peptide and adiponectin interactions in man. PloS One (2012) 7:e43238. doi: 10.1371/journal.pone.0043238
6. Quarta C, Sanchez-Garrido MA, Tschop MH, Clemmensen C. Renaissance of leptin for obesity therapy. Diabetologia (2016) 59:920–7. doi: 10.1007/s00125-016-3906-7
7. Fain JN, Kanu A, Bahouth SW, Cowan GSM, Hiler ML. Inhibition of leptin release by atrial natriuretic peptide (ANP) in human adipocytes. Biochem Pharmacol (2003) 65:1883–8. doi: 10.1016/S0006-2952(03)00154-0
8. Moro C, Klimcakova E, Lolmède K, Berlan M, Lafontan M, Stich V, et al. Atrial natriuretic peptide inhibits the production of adipokines and cytokines linked to inflammation and insulin resistance in human subcutaneous adipose tissue. Diabetologia (2007) 50:1038–47. doi: 10.1007/s00125-007-0614-3
9. Wabitsch M, Brenner RE, Melzner I, Braun M, Möller P, Heinze E, et al. Characterization of a human preadipocyte cell strain with high capacity for adipose differentiation. Int J Obes (2001) 25:8–15. doi: 10.1038/sj.ijo.0801520
10. Birkenfeld AL, Boschmann M, Moro C, Adams F, Heusser K, Franke G, et al. Lipid mobilization with physiological atrial natriuretic peptide concentrations in humans. J Clin Endocrinol Metab (2005) 90:3622–8. doi: 10.1210/jc.2004-1953
11. Birkenfeld AL, Boschmann M, Moro C, Adams F, Heusser K, Tank J, et al. Beta-adrenergic and atrial natriuretic peptide interactions on human cardiovascular and metabolic regulation. J Clin Endocrinol Metab (2006) 91:5069–75. doi: 10.1210/jc.2006-1084
12. Tews D, Pula T, Funcke JB, Jastroch M, Keuper M, Debatin KM, et al. Elevated UCP1 levels are sufficient to improve glucose uptake in human white adipocytes. Redox Biol (2019) 26:101286–6. doi: 10.1016/j.redox.2019.101286
13. Birkenfeld AL, Budziarek P, Boschmann M, Moro C, Adams F, Franke G, et al. Atrial natriuretic peptide induces postprandial lipid oxidation in humans. Diabetes (2008) 57:3199–204. doi: 10.2337/db08-0649
14. Cammisotto PG, Gélinas Y, Deshaies Y, Bukowiecki LJ. Regulation of leptin secretion from white adipocytes by free fatty acids. Am J Physiol-Endocrinol Metab (2003) 285:E521–6. doi: 10.1152/ajpendo.00052.2003
15. Szkudelski T. Intracellular mediators in regulation of leptin secretion from adipocytes. Physiol Res (2007) 56:503–12. doi: 10.33549/physiolres.931038
16. Armani A, Marzolla V, Rosano GM, Fabbri A, Caprio M. Phosphodiesterase type 5 (PDE5) in the adipocyte: a novel player in fat metabolism? Trends Endocrinol Metab (2011) 22:404–11. doi: 10.1016/j.tem.2011.05.004
17. Cumin F, Baum HP, Levens N. Mechanism of leptin removal from the circulation by the kidney. J Endocrinol (1997) 155:577–85. doi: 10.1677/joe.0.1550577
18. Engeli S, Birkenfeld AL, Badin PM, Bourlier V, Louche K, Viguerie N, et al. Natriuretic peptides enhance the oxidative capacity of human skeletal muscle. J Clin Invest (2012) 122:4675–9. doi: 10.1172/JCI64526
19. Packer M. Leptin-Aldosterone-Neprilysin axis: identification of its distinctive role in the pathogenesis of the three phenotypes of heart failure in people with obesity. Circulation (2018) 137:1614–31. doi: 10.1161/CIRCULATIONAHA.117.032474
20. Horbal SR, Hall ME, Dinh PC, Smiley A, Musani SK, Liu J, et al. Associations of adiponectin and leptin with brain natriuretic peptide in African americans: the Jackson heart study. Cardiovasc Endocrinol Metab (2020) 9:49–55. doi: 10.1097/XCE.0000000000000198
21. Zhao S, Kusminski CM, Elmquist JK, Scherer PE. Leptin: less is more. Diabetes (2020) 69:823–9. doi: 10.2337/dbi19-0018
22. Wu W, Shi F, Liu D, Ceddia RP, Gaffin R, Wei W, et al. Enhancing natriuretic peptide signaling in adipose tissue, but not in muscle, protects against diet-induced obesity and insulin resistance. Sci Signaling (2017) 10:6870–0. doi: 10.1126/scisignal.aam6870
23. Cannone V, Cabassi A, Volpi R, Burnett JC. Atrial natriuretic peptide: a molecular target of novel therapeutic approaches to cardio-metabolic disease. Int J Mol Sci (2019) 20:3265. doi: 10.3390/ijms20133265
Keywords: ANP, atrial natriuretic peptide, obesity, leptin, heart failure, insulin resistance, lipolysis
Citation: Daniels MA, Fischer-Posovszky P, Boschmann M, Jumpertz-von Schwartzenberg R, Müller TD, Sandforth L, Frank-Podlech S, Hülskämper S, Peter A, Wabitsch M, Jordan J and Birkenfeld AL (2023) Atrial natriuretic peptide and leptin interactions in healthy men. Front. Endocrinol. 14:1195677. doi: 10.3389/fendo.2023.1195677
Received: 28 March 2023; Accepted: 09 June 2023;
Published: 30 June 2023.
Edited by:
Bilgen Ekim Üstünel, Heidelberg University Hospital, GermanyReviewed by:
Shawn R. Campagna, The University of Tennessee, United StatesAbsalon Gutierrez, University of Texas Health Science Center at Houston, United States
Copyright © 2023 Daniels, Fischer-Posovszky, Boschmann, Jumpertz-von Schwartzenberg, Müller, Sandforth, Frank-Podlech, Hülskämper, Peter, Wabitsch, Jordan and Birkenfeld. This is an open-access article distributed under the terms of the Creative Commons Attribution License (CC BY). The use, distribution or reproduction in other forums is permitted, provided the original author(s) and the copyright owner(s) are credited and that the original publication in this journal is cited, in accordance with accepted academic practice. No use, distribution or reproduction is permitted which does not comply with these terms.
*Correspondence: Andreas L. Birkenfeld, QW5kcmVhcy5iaXJrZW5mZWxkQG1lZC51bmktdHVlYmluZ2VuLmRl
†These authors have contributed equally to this work and share first authorship