- Institute of Digestive Diseases, Longhua Hospital, Shanghai University of Traditional Chinese Medicine, Shanghai, China
Nonalcoholic fatty liver disease (NAFLD) has become one of the most common chronic liver diseases in the world. The risk factor for NAFLD is often considered to be obesity, but it can also occur in people with lean type, which is defined as lean NAFLD. Lean NAFLD is commonly associated with sarcopenia, a progressive loss of muscle quantity and quality. The pathological features of lean NAFLD such as visceral obesity, insulin resistance, and metabolic inflammation are inducers of sarcopenia, whereas loss of muscle mass and function further exacerbates ectopic fat accumulation and lean NAFLD. Therefore, we discussed the association of sarcopenia and lean NAFLD, summarized the underlying pathological mechanisms, and proposed potential strategies to reduce the risks of lean NAFLD and sarcopenia in this review.
1 Introduction
Nonalcoholic fatty liver disease (NAFLD), also known as metabolic-associated fatty liver disease (MAFLD), is the most common chronic liver disease in the world (1), which composed of a series of progressive liver diseases, including NAFL, non-alcoholic steatohepatitis (NASH), and fibrosis (2). Lean NAFLD has been an emerging topic of the current clinical study, given a considerable portion of NAFLD patients without obesity and its distinguishing courses of disease and manifestations (3–5). Lean NAFLD is usually defined by Body Mass Index (BMI), with BMI <23kg/m2 in Asians and <25kg/m2 in European and American (6). An epidemiological survey showed that the global prevalence of NAFLD has risen to 32.4% (7). Although obesity is a major contributing factor to NAFLD, approximately 40% of patients with NAFLD are nonobese and 20% are lean (8). Lean MAFLD is often occurred in old people, with a higher proportion of females (9). The study by Younossi et al. (10) found that the prevalence of lean NAFLD varies among different ethnic groups, ranging from 5%- 45% in Asians to 5%- 20% in Europeans. Among 6905 Chinese people with BMI<25kg/m2, 7. 27% had hepatic steatosis; In another study, 18% of 2000 Chinese residents with BMI<24kg/m2 were diagnosed with NAFLD (11). This may be related to regional differences in dietary habits (including high fructose, low protein and dietary fiber intake (11–14) and lifestyle (such as sedentary, lack of physical exercise and sleep habits) (15–17).
2 Clinical features and risks of lean NAFLD
Obesity is one of the major characteristics of NAFLD, and is known as a risk factor for the development of NAFLD (6). People with normal BMI are often overlooked in screening for NAFLD. Moreover, lean NAFLD is often considered to be benign in contrast to obese NAFLD, even images show identical steatosis (18). Chen et al. (3) included 538 patients with NAFLD confirmed by liver biopsy, and found that compared with non-obese patients, lean patients had better metabolic status and lower incidence and severity of T2DM and metabolic syndrome. But these differences occur in the early stage of liver disease, and the advanced liver disease progression of lean NAFLD isn’t improved (3). This suggests the homeostasis in lean NAFLD is gradually destroyed and subsequent progression can’t be avoided, despite the baseline metabolic and histological status of them being better than non-lean subjects (3).
HOMA-insulin resistance (Homeostasis model assessment-insulin resistance) is significantly higher among the lean NAFLD group in comparison to the obese group in a study by Parth et al. (19). And multiple studies have found that both non-obese and lean NAFLD have long-term hepatic and extrahepatic complications (10, 11). This may explain why some studies have reported higher advanced liver disease severity and mortality in lean NAFLD than in non-lean NAFLD. Lean NAFLD patients have a higher risk of metabolic syndrome and all-cause mortality in comparison to obese NAFLD patients (12, 18–21). This suggests that lean NAFLD is not a metabolically healthy status. Lean NAFLD patients have the same pathological features as obese NAFLD, and the same or even shorter course of progression to NASH (22). Numerous studies have reported that lean NAFLD patients showed metabolic risk factors, such as dyslipidemia, arterial hypertension, insulin resistance and type 2 diabetes mellitus (T2DM), which are closely related to the severity of liver disease (12, 23, 24). A large, prospective, community-based cohort (25) provides evidence that lean NAFLD subjects have more progressive liver disease and poorer clinical outcomes, independent from the usual risk factors and lifestyle. This is confirmed in another meta-analysis on mortality and liver events in patients with lean NAFLD (20), as well as in a cohort study in China (21).
Central obesity is a typical characteristic of lean NAFLD (26). Unlike subcutaneous fat storage, excessive visceral fat leads to the accumulation of pro-inflammatory cytokines including tumor necrosis factor-α (TNF-α) and interleukin-6 (IL-6), and reduction of many anti-inflammatory factors such as adiponectin, which promotes metabolic inflammation (27, 28). Visceral fat is reported to be more closely associated with metabolic syndrome than subcutaneous fat (28, 29). The proportion of central obesity of lean NAFLD is observably increased, despite the BMI of lean NAFLD patients is in the normal range or even lower (23). It is reported that the waist circumference and total abdominal fat levels in the non-obese group were lower than those in the obese group, but there was no prominent difference in visceral fat levels between the two groups. In some non-obese patients, the visceral fat area even exceeds 100cm2, which is higher in contrast to that in obese patients (30). Therefore, excessive visceral fat might be a driving factor for the disease progression of lean NAFLD.
3 Association between lean NAFLD and sarcopenia
Skeletal muscle as a locomotor organ as well as a metabolic organ accounts for 40-50% of lean body mass in adults, which plays one of the most crucial roles in the control of energy metabolism (31). Sarcopenia, previously considered an aging-related syndrome involving a decrease in muscle quantity and quality as well as physical performance, is now recognized as a progressive pathological process associated with type 2 diabetes mellitus (T2DM), metabolic syndrome, liver disease, and cardiovascular disease (32). It is primarily associated with aging and secondarily with diseases mediated by systemic inflammation and insulin resistance (32).
There is a close relationship between skeletal muscle and the liver, in particular, in terms of glucose, amino acid, and ammonia metabolism (33). In a Korean epidemiological study of 3305 patients with metabolic syndrome, 739 (22. 4%) had sarcopenia (34). And the coexistence of NAFLD and sarcopenia have been reported in a series of clinical studies (Table 1) (35–44). NAFLD patients with sarcopenia had a 2-fold higher risk of developing NASH and significant fibrosis independent of obesity and insulin resistance (45). Lean NAFLD patients are especially prone to sarcopenia (46). The prevalence of sarcopenia is significantly increased in patients with NASH compared to that in healthy subjects (35.0% versus 8.7%) (45). A recent study demonstrated that NAFLD developed in 14.8% of its participants during a 7-year follow-up, with an increased incidence in participants with the lowest tertile of skeletal muscle mass at baseline (47). Sarcopenia is associated with poor clinical outcomes, including severe hepatic fibrosis and increased mortality, in NAFLD patients (48–51). Therefore, low skeletal muscle mass may be an important risk factor for NAFLD, especially lean NAFLD. In addition, sarcopenia is also associated with cirrhosis and is a prognostic determinant of cirrhosis (33, 52).
4 Potential mechanisms of lean NAFLD and sarcopenia
4.1 Dietary intake and lean NAFLD
The pathophysiology of lean NAFLD can be impressed by diet and nutrients (53, 54). A matched case-control study included 351 Chinese adults, and found the average weekly exercise and daily sleep duration of lean NAFLD people were lower than that of healthy lean NAFLD people, while the average daily intake of total calories, carbohydrate, total cholesterol, fat and protein was higher than that of healthy lean NAFLD people (55). Total starchy foods intake was higher in those with NAFLD compared to the lean healthy group, but no significant difference was found among lean and non-lean NAFLD participants regarding nutrients and food items, indicating that the chance of lean NAFLD would increase by an increase in carbohydrate, potato, and fat intake (56). But in the study of Younossi et al. (57), no difference was found in macronutrients intake (carbohydrate, fat, and protein), vitamins, and minerals between lean NAFLD subjects and lean healthy controls. They suggested that this may indicate that NAFLD in lean patients has occurred due to other metabolic abnormalities. Others have postulated that the cause of lean NAFLD could be due to genetic characteristics, impaired intestinal motility, and some other metabolic disturbances that are not related to weight status (58). Since studies on the association between lean NAFLD and diet are less at present, and is still controversial, it is urgent to assess dietary intakes and pattern, and diet quality with adequate sample size in lean NAFLD people to find if there is any association between them.
4.2 The Role of skeletal muscle and glucose metabolism
The concerted regulation of glucose uptake, utilization, and storage by tissues is critical to maintaining blood glucose homeostasis (59). Insulin increases glucose uptake into peripheral tissues, primarily skeletal muscle and adipose tissues, which express the GLUT4 isoform of the glucose transporter. In unstimulated fat or muscle cells (basal state), 3~10% of GLUT4 is located at the cell surface and >90% is in intracellular compartments (60). GLUT4 participates in a cycle that consists of exocytic movement of the transporter within post-biosynthetic vesicles of endosomal origin towards the plasma membrane, and of endocytic movement from the membrane back to the sorting endosomal system (61). GLUT4 exocytosis is regulated by Insulin-derived signals, including mobilization to the cellular periphery, vesicle tethering, docking and fusion, thereby increasing the cell-surface amount of GLUT4 and the rate of glucose uptake (62).
Skeletal muscle expresses high levels of GLUT4, responsible for approximately 80% of glucose clearance under physiological conditions (32, 46). In mice, muscle-specific knockout of GLUT4 leads to severe glucose intolerance (63). According to the morphology and metabolic features, muscle fibers are mainly divided into type I and type II subtypes. Muscles composed of different types of muscle fibers show great differences in blood glucose metabolism, and the density of glucose transporter 4 (GLUT4) in type I muscle fibers is higher than that in type II muscle fibers (64). Gaster et al. (65) found that GLUT4 expression was reduced in type I fibers in T2DM patients by immunohistochemistry and morphometry. Therefore, it can be assumed that the decrease in insulin-stimulated glucose uptake in the skeletal muscle of T2DM patients might be due to the decrease of GLUT4 in type I fibers. In addition, muscle fibers (especially type IIb muscle fibers) can ameliorate metabolic abnormalities by secreting proteins and muscle factors, while loss of skeletal muscle due to aging or underlying diseases can exacerbate glucose intolerance (66).
4.3 Sarcopenia and insulin resistance
Insulin resistance is an important pathogenesis of sarcopenia and NAFLD, regardless of lean type or non-lean type (67–69). Insulin resistance refers to the reduction of glucose uptake and utilization. To sustain stable levels of blood glucose, islet β cells secret excessive insulin to compensate, leading to hyperinsulinemia (70). Increased insulin content results in decreased affinity to insulin receptors thus, further exaggerating the insensitivity of insulin. Simultaneously, increased levels of adipokines and inflammatory cytokines (such as IL-1, IL-6 and TNF-α) activate NF-κB signaling pathway and promote insulin resistance (71). Meanwhile, the weakened insulin signaling transduction also affects the downstream mediator-glycogen synthase kinase-3β (GSK3β) and glycogen synthesis (72). Collectively, excessive insulin secretion, adipokines, and inflammatory factors directly or indirectly interfere with the insulin signaling pathway, and contribute to the development of NAFLD (73).
Insulin resistance leads to increased lipolysis and consequent release of free fatty acids (FFAs) from adipose tissue to the liver, which is the main contributor to the increase of De novo lipogenesis (DNL) (63, 66). The mechanism of insulin-mediated decrease of muscle anabolic metabolism may be related to the activation of p38 mitogen-activated protein kinase (MAPK) and mammalian target of rapamycin (mTOR)/p70S6 kinase (74). Insulin resistance-induced hyperinsulinemia also increases myostatin levels, which further reduces skeletal muscle mass (48). Insulin resistance leads to inhibition of β-oxidation, increased gluconeogenesis, increased expression of sterol regulatory element binding protein-1c (SREBP-1c), as well as increased production of FFAs, leading to accumulation of triglycerides in skeletal muscle and liver, and the long-term level of inflammation might be responsible for the disruption of environmental homeostasis in muscle cells, ultimately leading to systemic metabolic disorders (75, 76). Conversely, a 2-year follow-up cohort included 194 community-dwelling nondiabetic older adults found that loss of lower limb muscle mass is a significant risk factor for development of insulin resistance independent of obesity (77). Sarcopenic obesity patients had higher HOMA-IR index in contrast to subjects without sarcopenia, indicating that muscle mass is also a determinant for insulin sensitivity (67).
In addition, anabolic hormones such as insulin-like growth Factor1 (IGF-1) also contribute to the progression of sarcopenia and lean NAFLD (78), as well as non-lean NAFLD (68). Skeletal muscle mass loss inhibits the growth hormone/insulin-like growth factor-1 (GH/IGF-1) axis, and blocks skeletal muscle protein synthesis (35). The GH/IGF-1 axis is involved in protein metabolism and bone growth and remodeling in skeletal muscle (79). GH/IGF-1 affects carbohydrate and lipid metabolism and have opposite effects: IGF-1 stimulates glucose uptake and facilitates insulin signaling, while GH induces lipolysis, which induces insulin resistance and elevated levels of free fatty acids (FFAs) (80). IGF-1 level decreases when insulin resistance occurs, leading to lipid deposition in intramuscular and intermuscular adipose cells, increased infiltration of adipose between muscle bundles, and aggravated damage to muscle cells (81, 82). Patients with GH deficiency are often comorbidity with NAFLD, which contributes to NASH development and progression (83). Some studies also reported low levels of IGF-1 in NAFLD patients (84, 85). These indicate that GH/IGH-1 axis is another important association between sarcopenia and lean NAFLD.
4.4 Sarcopenia and cytokine disorders
Sarcopenia and lean NAFLD often present with systemic chronic inflammation, elevated levels of CRP and pro-inflammatory cytokines, and reduced level of anti-inflammatory cytokines (86). This is due to the high proportion of visceral adipose tissue in patients with lean NAFLD, and then more adipose cells secret inflammatory cytokines, and the muscle tissue is in a state of continuous chronic inflammation, leading to an increased risk of muscle atrophy (87). In patients with metabolic disorders, white adipocytes become hypertrophy and proliferated, and these white adipocytes are infiltrated by activated inflammatory macrophages and other immune cells (88). Contractile myoglobin expression is reduced in muscle tubes cocultured with white adipocytes from obese individuals (89). This may indicate that too much adipose tissue disrupts the living environment of muscle cells and promotes their apoptosis. Adipose tissue produces a large number of pro-inflammatory cytokines, such as TNF-α, monocyte chemotactic protein-1 (MCP-1), IL-6, and C-reactive protein, which promote chronic inflammation, decreased muscle protein synthesis, and insulin resistance (90). The increased production of systemic inflammatory molecules and inhibited production of certain adipokines (such as leptin and appetite suppressor hormone) in lean NAFLD patients, further adversely affect the liver, pancreas and skeletal muscle (75). Altered adipokine secretion leads to increased food intake, decreased energy expenditure, and decreased insulin sensitivity in muscles (90). Immune cells and adipocytes release inflammatory cytokines, which aggravate myocyte insulin resistance and further worsen sarcopenia (91). These processes can also occur in the pathogenesis of non-lean NAFLD/T2DM and sarcopenia (68, 92).
Inflammation and oxidative stress appear to be major factors in the etiology of muscle loss (93). Muscle cells are particularly vulnerable to oxidative damage because they are post-mitotic cells and are particularly prone to the accumulation of oxidative damage molecules (94). In addition, skeletal muscle accounts for a prominent portion of total oxygen consumption, increasing the inherent risk of elevated mitochondrial-derived ROS such as H2O2 (95). Increased reactive oxygen species (ROS) production and inefficient clearance in muscle cells trigger cellular senescence and eventually muscle loss (96). The imbalance of mitochondrial unfolded protein response and mitochondrial phagocytosis modified integrated stress response systems involved in the development of sarcopenia (97). Oxidative stress also increases the production and circulation of inflammatory cytokines, which promote protein degradation in muscle (98). TNF-α also induces lipid accumulation in the liver by activating DNL (71). It is reported in many rodent studies that inhibition of TNF-α attenuates muscle proteolysis, while an infusion of TNF-α increases Myo-fibrillation lysis (99, 100). This indicates that pro-inflammatory cytokines (CRP, IL-6, and TNF-α) may increase proteolysis and muscle atrophy.
The development of sarcopenia and lean NAFLD is accompanied by a decrease in some protective factors, such as adiponectin (27, 28). Adiponectin is a hormone secreted by adipose tissue that mediates glucose and lipid metabolism in insulin-sensitive tissues such as the liver and muscle (101). In the liver, adiponectin improves insulin resistance by activating AMP-activated protein kinase (AMPK), thus promoting glucose usage and fatty acid oxidation. In addition, adiponectin plays the role of anti-inflammatory by inhibiting TNF-α, improving liver steatosis and inflammation (102, 103). Fat accumulation and aging damage the signaling pathway of the GH/IGF-1 axis, leading to decreased levels of muscle synthesis (104, 105). In an animal model, NAFLD is reported to be associated with decreased muscle mass and strength as well as decreased IGF-1 levels, suggesting that decreased IGF-1 may contribute to the development of NAFLD-related sarcopenia (106).
In addition, Irisin is also involved in the progression of sarcopenia and NAFLD (107, 108). Irisin, an exercise-induced myokine, increases energy expenditure through peroxisome proliferator-activated receptor α-dependent downstream signaling and improves insulin sensitivity and hepatic steatosis by upregulating fibroblast growth factor-21 (108). It increases glucose uptake by enhancing GLUT4 translocation and FFA β-oxidation through activating AMPK in muscle cells (108). Irisin is reported to be inversely associated with the degree of hepatic steatosis and is a potential cause of sarcopenia and NAFLD (107, 109).
4.5 Genetic susceptibility
NAFLD is highly influenced by genetic and environmental factors, and its susceptibility, progression and risks of related complications vary greatly between individuals, and the genetic variations of NAFLD are confirmed in genome-wide association studies (GWAS) (110). It is reported that the proportion of the G allele in PNPLA3 and the T allele in TM6SF2 in lean NAFLD patients is significantly higher than that in non-lean NAFLD patients, and the mutations of these two alleles are highly related to the disorder of glucose and lipid metabolism (111). The PNPLA3 rs738409 G allele is associated with higher liver-related mortality than the C allele in a nationwide population study in the United States (112). The C allele frequency of rs2279026 and G allele frequency of rs2279028 in the TBC1D1 gene as well as the C allele frequency of rs780093 and rs1260326 in GCKR gene in lean NAFLD patients are lower than those in obese patients (113). A cohort study of 5387 Chinese residents (≥60 years old) revealed that the AA genotype frequency of SOD2 gene rs4880 in patients with lean NAFLD is lower than that in lean healthy people, and rs4880 site in SOD2 determined the susceptibility to lean NAFLD (114). The PEMT gene controls liver triglyceride secretion in the form of VLDL and is associated with NAFLD. Bale et al. (115) sequenced the intact exon region of lean NAFLD patients and found that the PEMT rs7946 variant is associated with a three-times increase in the risk of lean NAFLD.
Variation in skeletal muscle traits among individuals can be attributed to both genetic and environmental factors (116). Though the influence of environmental factors, such as physical activity and diet, have been broadly investigated, only a few studies have identified the specific genetic influences on skeletal muscle traits (117). Sarlo et al. enrolled 120 Italian healthy women and found a positive correlation between sarcopenic normal-weight subjects and G/A-308 TNF-α polymorphism (118). The gene TP53, encoding p53, is reported to be involved in the pathogenesis of NAFLD (119). It is also affects myoblast differentiation in severe and rapid skeletal muscle atrophy, which represents a hallmark of cachexia and sarcopenia during aging (120). The G/G genotype of TP53 codon 72 in exon 4 polymorphism is found to increase the risk of sarcopenia up to 20% (121). The skeletal muscle mass is reported to be associated with FTO (Fat mass and obesity-associated) genotype variation. In a study enrolled 559 non-athlete subjects, TT genotype and T allele carriers of the FTO rs9939609 variant had greater total body (4.8% and 4.1%) and total appendicular lean mass (3.0% and 2.1%) compared to AA genotype (122). The FTO genotype variants also play an important role in lipid-related parameters (123). In a cohort study, AA genotype of the FTO rs9939609 is found to be associated with LDL levels lean NAFLD subjects but not in overweight and obese NAFLD subjects (113). Besides, the ACTN3, ACE, VDR, IGF1/IGFBP3, APOE, CNTF/R and UCP2/3 gene polymorphism are also found to be associated with muscle phenotypes (124–130), but their association with lean NAFLD is still to be determined. Therefore, certain genetic factors might be associated with both sarcopenia and lean NAFLD, but further studies are needed to verify the association and underlying mechanisms.
4.6 Differential metabolites
Circulating cholesterol is the major metabolic risk of NAFLD patients, with the potential to develop cardiovascular diseases (131, 132). Both low-density lipoprotein (LDL) and high-density lipoprotein (HDL) are carriers of cholesterol, with LDL moving cholesterol into the arteries and HDL clearing cholesterol from the arteries. Dyslipidemia is a common complication of NAFLD (133). Cheng et al. (9) found that among 394 NAFLD patients (16. 5% lean), lean NAFLD patients are older, more female and have higher levels of HDL, but lower serum triglyceride and alanine transaminase (ALT) levels in contrast to non-lean patients. Compared with the lean healthy group, lean NAFLD patients showed a higher percentage of hyperlipidemia, age, and an increase in waist circumference, serum triglyceride, LDL-C, and blood glucose levels (9). A study involving 1,305 Chinese residents found that sarcopenic lean NAFLD patients presented a distinct metabolomic profile that is prone to carotid plaque and liver fibrosis, with increased serum valine, small LDL triglyceride and VLDL5 components, and reduced components of HDL4 (134). Park et al. found that higher relative grip strength, one of the indicators of sarcopenia, is associated with lower TG, TC, and LDL-C and higher HDL-C levels (135). These studies suggest that LDL-C, TG and TC as well as HDL-C might be biomarkers of sarcopenic lean NAFLD.
Bile acids (BAs) are amphiphilic steroid molecules synthesized from cholesterol in the liver (136). BAs regulate lipid and glucose metabolism, as well as the synthesis, transport and reabsorption of BAs through bile acid receptors (137). The dysfunctional BA metabolism promotes the accumulation of fat and infiltration of inflammatory cells in the liver (4). Compared with non-lean NAFLD patients, the total BA, primary BA and secondary BA levels are higher in lean NAFLD patients. BA profiling showed that lean NAFLD patients had lower levels of deoxycholic acid (DCA), glycodeoxycholic acid (GCDCA) and goosenodeoxycholic acid (CDCA), and higher levels of glycocholic acid (GCA) (3). Studies reported that serum cholic acid (CA), ursodeoxycholic acid (UDCA) and DCA impair muscle fiber structure and function and induce sarcopenia (138–140). And the level of CA is significantly higher in patients with severe fibrosis compared with those with none/mild fibrosis (3).
Serum uric acid, a metabolite of purines in the liver, is considered to be a predictor of insulin resistance and the severity of liver injury in NAFLD (141). Serum uric acid levels are reported to be higher in lean NAFLD than in healthy controls, but HDL-c levels are in the contrary. Since the ratio of uric acid to HDL-c (UHR) is independently associated with an increased risk of NAFLD, UHR might be a new promising marker for lean NAFLD (142). Some studies have reported a positive association between serum uric acid levels and relative grip strength (143–145). However, a cross-sectional study of 5,247 adults from Korea found a negative association (146). Therefore, the relationship between serum uric acid level and sarcopenia remains controversial and needs further studies to verify the association.
4.7 Altered gut microbiota
The gut microbiome has a significant contribution to digestion, vitamin synthesis and pathogen resistance (147). The disruption of intestinal homeostasis and changes in microbiota are involved in the pathogenesis of NAFLD (147). Recent studies highlight that lean NAFLD subjects have a distinct gut microbiota profile from obese ones (18).
In a study conducted in a Chinese population, lean NAFLD subjects demonstrated a reduced enrichment of Firmicutes, including Lachnospiraceae, Ruminococcaceae, Lactobacillaceae, and an increase in lipopolysaccharide-producing Gram-negative bacteria (148). Chen et al. (3) reported a distinct microbiome profile in a Caucasian population, and found that lean NAFLD patients have an increased proportion of Ruminococcaceae compared to obese NAFLD patients and an increased population of Dorea and decreased populations of Marvinbryantia and Christensellenaceae compared with healthy controls. According to the analysis of liver biopsy proved NASH patients, intestinal permeability and bacterial overgrowth are associated with the severity of steatosis (149). The gut microbiomes affect FXR-mediated signaling pathways in the liver and gut by interacting with BAs, which is associated with the pathogenesis of lean NAFLD (150, 151). Gut microbiome and BA alterations may predispose NAFLD development at a lower BMI, and further studies of microbiome modulation are required to better understand its role as both a potential biomarker and a therapeutic option in lean NAFLD patients (152).
Nikkhah et al. found that the alteration in age-related sarcopenia and liver cirrhosis-induced sarcopenia was a reduction in short-chain fatty acids (SCFAs) -producing bacteria. Lachnospiraceae family, consisting of Lachnospira, Fusicatenibacter, Roseburia, and Lachnoclostridium, is significantly decreased in age-related sarcopenia, while in liver cirrhosis-induced sarcopenia, the alpha diversity of gut microbiota decreased in comparison to the control group (153). The richness of gut microbiota is considerably reduced in sarcopenic patients, and the Firmicutes/Bacteroidetes ratio, Agathobacter, Dorea and Butyrate are decreased, whereas Shigella and Bacteroides are enriched in the gut (154). A case-control study also reported that Phylum Bacteroides is significantly decreased in old-women with sarcopenia, whereas genus Prevotella is increased (155). However, the relationship between the types and richness of gut microbiota in sarcopenia and lean NAFLD is not clear.
5 Strategies for sarcopenia and lean NAFLD
There is a close relationship between the amount of physical activity and mortality, and people with more physical activity have a lower risk of death (156). Lack of physical activity leads to loss of muscle mass and reduced energy expenditure, leading to obesity and hepatic steatosis (157). Chronic inflammation, oxidative stress, and insulin resistance can worsen sarcopenia and NAFLD (158). During exercise, the production of pro-inflammatory cytokines decreased while the anti-inflammatory cytokines and muscle protein synthesis, as well as glucose uptake increased. Physical activity may reduce the risk of sarcopenia progression (159). Studies found that exercise improves metabolic health even without observable weight loss (160, 161).
Circuit training has been reported as an effective way to simultaneously improve both the muscular and cardiovascular systems. Circuit training programs offer a combination of aerobic and resistance exercises, the intensity of which can be adapted to the individual and purpose. In a Korean study on circuit training intervention on elderly women with sarcopenia, the muscle mass and strength, body composition, balance, and pulmonary function in subjects were improved after 12-week circuit exercise training (162). Nutrition supplements also have a significant contribution to the improvement of sarcopenia. A randomized controlled trial shows that the skeletal muscle mass index in the exercise and protein supplementation group is significantly higher than either the exercise-only or protein supplementation-only groups. Simultaneously, the increase in grip strength and gait speed is significantly greater for the exercise and protein supplementation group than for the protein supplementation-only group (163). Other trials also proved that nutrition such as amino acids, vitamin D, or calcium supplements improve muscle mass, strength, and physical function in patients with sarcopenia (164–168). The above evidence indicates that combined intervention of exercise (including aerobic and resistance exercises) and nutrition (such as whey protein, branched-chain amino acids, and vitamin D as well as calcium supplement) has positive effects on sarcopenia, which provide a theoretical basis and therapeutic strategy for the improvement of sarcopenia. Since sarcopenia is associated with some hormones disorder and decreased muscle protein synthesis, some drugs such as sex hormones (e. g. testosterone and 17 beta-estradiol plus cyclic norethisterone acetate) and monoclonal antibodies that stimulate muscle growth (e. g. bimagrumab) have been used to improve sarcopenia (169–171).
Currently, there is no specific drug for the treatment of NAFLD including lean NAFLD. However, some potential medications might be suitable for countering the syndromes or preventing disease progression. Insulin resistance is one of the most critical potential co-pathogenesis of sarcopenia and lean NAFLD (48). Pioglitazone is a PPAR-γ agonist that increases insulin sensitivity, and exerts anti-inflammatory and anti-atherosclerosis effects (172). In the UTHSCSA NASH Phase 4 trial (NCT00994682) (173), the number of patients in the pioglitazone group who recovered from impaired fasting glucose (IFG)/impaired glucose tolerance (IGT) is significantly higher than that in the placebo group, suggesting that pioglitazone has a desirable effect in reducing metabolic risk factors, which can be used as a potential therapeutic agent for lean NAFLD. Dapagliflozin and Empagliflozin, sodium-glucose cotransporter 2 (SGLT-2) inhibitors, are approved by the FDA as oral hypoglycemic agents in patients with T2DM (174). A Phase 2 trial (175) studied the effect of Dapagliflozin on NAFLD patients with T2DM, and showed a significant radiographic improvement in liver fat content compared with placebo. SGLT-2 inhibitors have also found to reduce visceral fat, improve glycemic disorder, and protect the heart, which may have therapeutic potential for lean NAFLD (175). Fenofibrate is a drug for dyslipidemia (176). Abdelmoneim et al. (177) fed experimental mice palm oil and fructose to establish a non-obese NAFLD model, and found that fenofibrate could prevent the occurrence of NAFLD, improve high blood glucose and oxidative stress status, indicating that fenofibrate can be a potential candidate for lean NAFLD.
6 Conclusions
Although BMI is within the normal range, lean NAFLD is not a benign state. It can also progress to NASH and fibrosis, and even cirrhosis and hepatocellular carcinoma as obese NAFLD. Lean NAFLD patients even have a higher risk of metabolic syndrome and all-cause mortality in comparison to obese NAFLD patients. Skeletal muscle is a major metabolic organ, and the decline of skeletal muscle quality and quantity leads to a decrease of insulin sensitivity and metabolic disorders. Sarcopenia is commonly coexistent with lean NAFLD, may present in the early stage of liver disease and worsen with the severity of liver disease. Under the background of co-pathogenesis of insulin resistance and chronic inflammation, the interaction between sarcopenia and lean NAFLD forms a vicious circle (Figure 1). Clinical interventions should be considered for patients with lean NAFLD as intensively as for those with obese NAFLD, especially when they have a comorbidity of sarcopenia. The first-line recommendation for lean NAFLD patients with sarcopenia is exercise, nutrition supplements and reduce total calories, carbohydrates, and fat as well as total cholesterol, so as to reduce visceral fat and increase skeletal muscle mass, so as to restore the homeostasis of lipid, glucose, endocrinal and metabolic status. However, there is no study to compare the status of sarcopenia between lean NAFLD and obese NAFLD, or exploring the underlying mechanisms, and more studies are needed to explore the pathophysiology and underly mechanisms of lean NAFLD and sarcopenia, which will assist in treatment and preventive strategies for lean NAFLD.
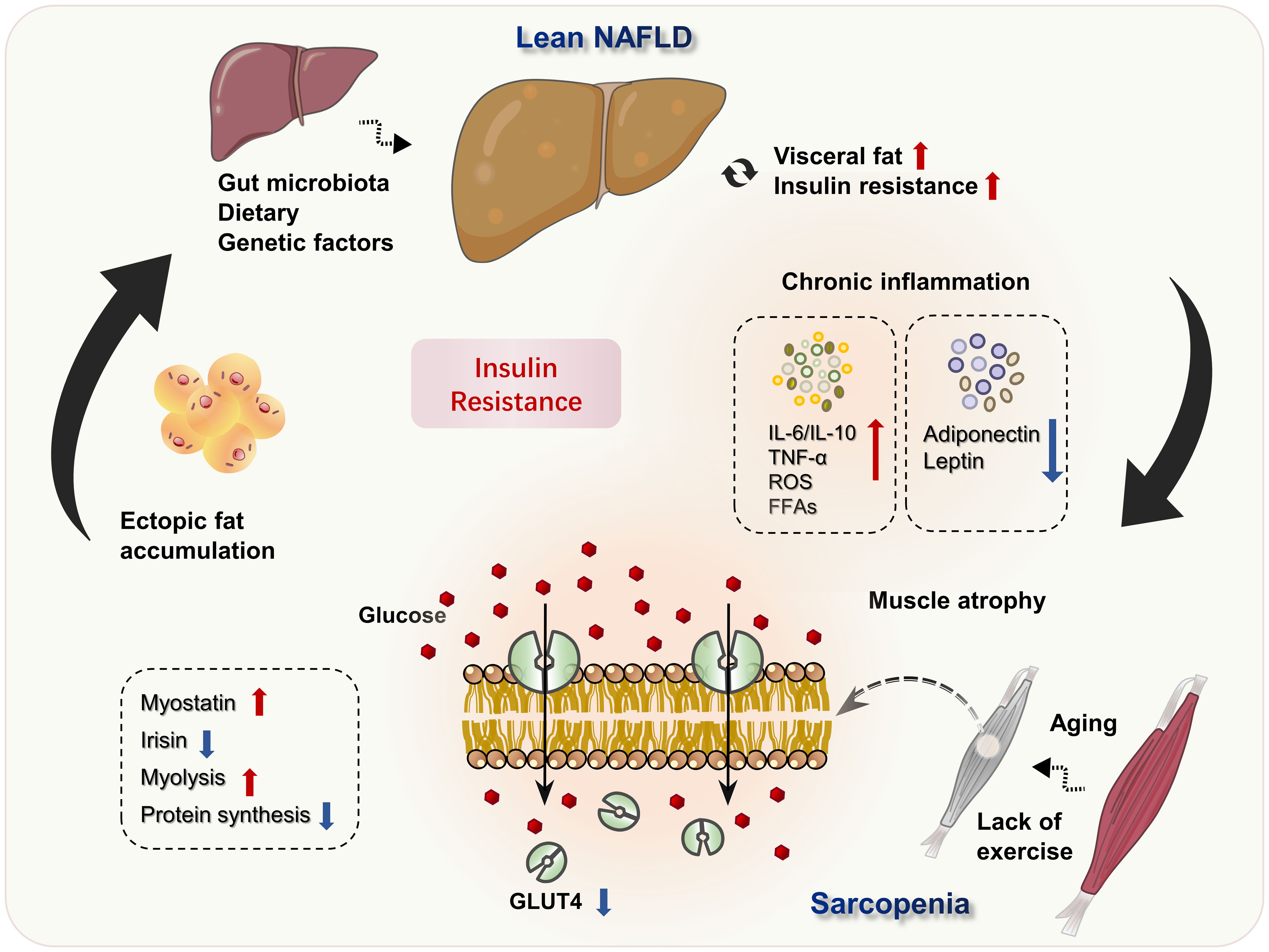
Figure 1 Potential mechanisms of lean NAFLD and sarcopenia. Excessive dietary intake and decreased energy consumption, as well as genetic predisposition and other factors, result in visceral fat accumulation, IR and the development of lean NAFLD. Simultaneously, IR and metabolic inflammatory worsen the living environment of muscle cells, aggravates muscle atrophy, and leads to the occurrence of sarcopenia. Loss of skeletal muscle mass inhibits the GH/IGF-1 axis, resulting in decreased muscle protein synthesis, increased lipolysis. The decreased expression of GLUT4 in sarcopenia, further suppressed glucose utilization and aggravated IR, forming a vicious circle.
Author contributions
LZ conceptualized the manuscript, MC collected the literature and drafted the manuscript, YC revised the manuscript and prepared the figure, LZ and GJ revised the manuscript. All authors edited, revised, and approved the final version of this review.
Funding
This work was supported by Youth Science Discipline Leading Project of Shanghai Municipal Health Commission (No.2022XD023).
Conflict of interest
The authors declare that the research was conducted in the absence of any commercial or financial relationships that could be construed as a potential conflict of interest.
Publisher’s note
All claims expressed in this article are solely those of the authors and do not necessarily represent those of their affiliated organizations, or those of the publisher, the editors and the reviewers. Any product that may be evaluated in this article, or claim that may be made by its manufacturer, is not guaranteed or endorsed by the publisher.
References
1. Chan KE, Koh TJL, Tang ASP, Quek J, Yong JN, Tay P, et al. Global prevalence and clinical characteristics of metabolic-associated fatty liver disease: a meta-analysis and systematic review of 10 739 607 individuals. J Clin Endocrinol Metab (2022) 107(9):2691–700. doi: 10.1210/clinem/dgac321
2. Wong VW-S, Wong GL-H, Woo J, Abrigo JM, Chan CK-M, Shu SS-T, et al. Impact of the new definition of metabolic associated fatty liver disease on the epidemiology of the disease. Clin Gastroenterol Hepatol (2021) 19(10):2161–71. doi: 10.1016/j.cgh.2020.10.046
3. Chen F, Esmaili S, Rogers GB, Bugianesi E, Petta S, Marchesini G, et al. Lean nafld: a distinct entity shaped by differential metabolic adaptation. Hepatology (2020) 71(4):1213–27. doi: 10.1002/hep.30908
4. Maier S, Wieland A, Cree-Green M, Nadeau K, Sullivan S, Lanaspa MA, et al. Lean nafld: an underrecognized and challenging disorder in medicine. Rev Endocr Metab Disord (2021) 22(2):351–66. doi: 10.1007/s11154-020-09621-1
5. Kumar R, Mohan S. Non-alcoholic fatty liver disease in lean subjects: characteristics and implications. J Clin Transl Hepatol (2017) 5(3):216–23. doi: 10.14218/JCTH.2016.00068
6. Patel AH, Peddu D, Amin S, Elsaid MI, Minacapelli CD, Chandler TM, et al. Nonalcoholic fatty liver disease in Lean/Nonobese and obese individuals: a comprehensive review on prevalence, pathogenesis, clinical outcomes, and treatment. J Clin Transl Hepatol (2023) 11(2):502–15. doi: 10.14218/JCTH.2022.00204
7. Riazi K, Azhari H, Charette JH, Underwood FE, King JA, Afshar EE, et al. The prevalence and incidence of nafld worldwide: a systematic review and meta-analysis. Lancet Gastroenterol Hepatol (2022) 7(9):851–61. doi: 10.1016/S2468-1253(22)00165-0
8. Ye Q, Zou B, Yeo YH, Li J, Huang DQ, Wu Y, et al. Global prevalence, incidence, and outcomes of non-obese or lean non-alcoholic fatty liver disease: a systematic review and meta-analysis. Lancet Gastroenterol Hepatol (2020) 5(8):739–52. doi: 10.1016/S2468-1253(20)30077-7
9. Cheng YM, Kao JH, Wang CC. The metabolic profiles and body composition of lean metabolic associated fatty liver disease. Hepatol Int (2021) 15(2):405–12. doi: 10.1007/s12072-021-10147-0
10. Younossi Z, Anstee QM, Marietti M, Hardy T, Henry L, Eslam M, et al. Global burden of nafld and Nash: trends, predictions, risk factors and prevention. Nat Rev Gastroenterol Hepatol (2018) 15(1):11–20. doi: 10.1038/nrgastro.2017.109
11. Eslam M CF, George J. Nafld in lean asians. Clin Liver Dis (Hoboken) (2021) 16(6):240–3. doi: 10.1002/cld.930
12. Younes R, Bugianesi E. Nash In lean individuals. Semin Liver Dis (2019) 39(1):86–95. doi: 10.1055/s-0038-1677517
13. Kim D, Kim WR. Nonobese fatty liver disease. Clin Gastroenterol Hepatol (2017) 15(4):474–85. doi: 10.1016/j.cgh.2016.08.028
14. Kallwitz ER, Daviglus ML, Allison MA, Emory KT, Zhao L, Kuniholm MH, et al. Prevalence of suspected nonalcoholic fatty liver disease in Hispanic/Latino individuals differs by heritage. Clin Gastroenterol Hepatol (2015) 13(3):569–76. doi: 10.1016/j.cgh.2014.08.037
15. Gerber L, Otgonsuren M, Mishra A, Escheik C, Birerdinc A, Stepanova M, et al. Non-alcoholic fatty liver disease (Nafld) is associated with low level of physical activity: a population-based study. Aliment Pharmacol Ther (2012) 36(8):772–81. doi: 10.1111/apt.12038
16. Hallsworth K, Thoma C, Moore S, Ploetz T, Anstee QM, Taylor R, et al. Non-alcoholic fatty liver disease is associated with higher levels of objectively measured sedentary behaviour and lower levels of physical activity than matched healthy controls. Frontline Gastroenterol (2015) 6(1):44–51. doi: 10.1136/flgastro-2014-100432
17. Keating SE, George J, Johnson NA. The benefits of exercise for patients with non-alcoholic fatty liver disease. Expert Rev Gastroenterol Hepatol (2015) 9(10):1247–50. doi: 10.1586/17474124.2015.1075392
18. Kuchay MS, Martinez-Montoro JI, Choudhary NS, Fernandez-Garcia JC, Ramos-Molina B. Non-alcoholic fatty liver disease in lean and non-obese individuals: current and future challenges. Biomedicines (2021) 9(10):1346. doi: 10.3390/biomedicines9101346
19. Parth SP, Ameet R, Anil M, Dipak P. A. study and comparison of metabolic profile of lean and obese subjects with non alcoholic fatty liver disease. J Assoc Physicians India (2020) 68(8):51–4.
20. Ha J, Yim SY, Karagozian R. Mortality and liver-related events in lean versus non-lean nonalcoholic fatty liver disease: a systematic review and meta-analysis. Clin Gastroenterol Hepatol (2022) S1542-3565(22). doi: 10.1016/j.cgh.2022.11.019
21. Lan Y, Lu Y, Li J, Hu S, Chen S, Wang Y, et al. Outcomes of subjects who are lean, overweight or obese with nonalcoholic fatty liver disease: a cohort study in China. Hepatol Commun (2022) 6(12):3393–405. doi: 10.1002/hep4.2081
22. Leung JC, Loong TC, Wei JL, Wong GL, Chan AW, Choi PC, et al. Histological severity and clinical outcomes of nonalcoholic fatty liver disease in nonobese patients. Hepatology (2017) 65(1):54–64. doi: 10.1002/hep.28697
23. Niriella MA, Kasturiratne A, Pathmeswaran A, De Silva ST, Perera KR, Subasinghe S, et al. Lean non-alcoholic fatty liver disease (Lean nafld): characteristics, metabolic outcomes and risk factors from a 7-year prospective, community cohort study from Sri Lanka. Hepatol Int (2019) 13(3):314–22. doi: 10.1007/s12072-018-9916-4
24. Feng RN, Du SS, Wang C, Li YC, Liu LY, Guo FC, et al. Lean-Non-Alcoholic fatty liver disease increases risk for metabolic disorders in a normal weight Chinese population. World J Gastroenterol (2014) 20(47):17932–40. doi: 10.3748/wjg.v20.i47.17932
25. Nabi O, Lapidus N, Boursier J, de Ledinghen V, Petit JM, Kab S, et al. Lean individuals with nafld have more severe liver disease and poorer clinical outcomes (Nash-Co study). Hepatology (2023). doi: 10.1097/HEP.0000000000000329
26. van der Poorten D, Milner KL, Hui J, Hodge A, Trenell MI, Kench JG, et al. Visceral fat: a key mediator of steatohepatitis in metabolic liver disease. Hepatology (2008) 48(2):449–57. doi: 10.1002/hep.22350
27. Ahadi M, Molooghi K, Masoudifar N, Namdar AB, Vossoughinia H, Farzanehfar M. A review of non-alcoholic fatty liver disease in non-obese and lean individuals. J Gastroenterol Hepatol (2021) 36(6):1497–507. doi: 10.1111/jgh.15353
28. Drolet R, Belanger C, Fortier M, Huot C, Mailloux J, Legare D, et al. Fat depot-specific impact of visceral obesity on adipocyte adiponectin release in women. Obes (Silver Spring) (2009) 17(3):424–30. doi: 10.1038/oby.2008.555
29. Motoshima H, Wu X, Sinha MK, Hardy VE, Rosato EL, Barbot DJ, et al. Differential regulation of adiponectin secretion from cultured human omental and subcutaneous adipocytes: effects of insulin and rosiglitazone. J Clin Endocrinol Metab (2002) 87(12):5662–7. doi: 10.1210/jc.2002-020635
30. Yasutake K, Nakamuta M, Shima Y, Ohyama A, Masuda K, Haruta N, et al. Nutritional investigation of non-obese patients with non-alcoholic fatty liver disease: the significance of dietary cholesterol. Scand J Gastroenterol (2009) 44(4):471–7. doi: 10.1080/00365520802588133
31. Wiedmer P, Jung T, Castro JP, Pomatto LCD, Sun PY, Davies KJA, et al. Sarcopenia - molecular mechanisms and open questions. Ageing Res Rev (2021) 65:101200. doi: 10.1016/j.arr.2020.101200
32. Bauer J, Morley JE, Schols A, Ferrucci L, Cruz-Jentoft AJ, Dent E, et al. Sarcopenia: a time for action. an scwd position paper. J Cachexia Sarcopenia Muscle (2019) 10(5):956–61. doi: 10.1002/jcsm.12483
33. Meza-Junco J M-LA, Baracos VE, Prado CM, Bain VG, Beaumont C, Esfandiari N, et al. Sarcopenia as a prognostic index of nutritional status in concurrent cirrhosis and hepatocellular carcinoma. J Clin Gastroenterol (2013) 47(10):861–70. doi: 10.1097/MCG.0b013e318293a825
34. Guillet C BY. Insulin resistance: a contributing factor to age-related muscle mass loss? Diabetes Metab (2005) 31 Spec No 2:5S20–5S6. doi: 10.1016/S1262-3636(05)73648-X
35. Lee YH, Kim SU, Song K, Park JY, Kim DY, Ahn SH, et al. Sarcopenia is associated with significant liver fibrosis independently of obesity and insulin resistance in nonalcoholic fatty liver disease: nationwide surveys (Knhanes 2008-2011). Hepatology (2016) 63(3):776–86. doi: 10.1002/hep.28376
36. Kim D, Wijarnpreecha K, Sandhu KK, Cholankeril G, Ahmed A. Sarcopenia in nonalcoholic fatty liver disease and all-cause and cause-specific mortality in the united states. Liver Int (2021) 41(8):1832–40. doi: 10.1111/liv.14852
37. Chung GE, Kim MJ, Yim JY, Kim JS, Yoon JW. Sarcopenia is significantly associated with presence and severity of nonalcoholic fatty liver disease. J Obes Metab Syndr (2019) 28(2):129–38. doi: 10.7570/jomes.2019.28.2.129
38. Golabi P, Gerber L, Paik JM, Deshpande R, de Avila L, Younossi ZM. Contribution of sarcopenia and physical inactivity to mortality in people with non-alcoholic fatty liver disease. JHEP Rep (2020) 2(6):100171. doi: 10.1016/j.jhepr.2020.100171
39. Petta S, Ciminnisi S, Di Marco V, Cabibi D, Camma C, Licata A, et al. Sarcopenia is associated with severe liver fibrosis in patients with non-alcoholic fatty liver disease. Aliment Pharmacol Ther (2017) 45(4):510–8. doi: 10.1111/apt.13889
40. Zhai Y, Xiao Q, Miao J. The relationship between nafld and sarcopenia in elderly patients. Can J Gastroenterol Hepatol (2018) 2018:5016091. doi: 10.1155/2018/5016091
41. Wijarnpreecha K, Kim D, Raymond P, Scribani M, Ahmed A. Associations between sarcopenia and nonalcoholic fatty liver disease and advanced fibrosis in the USA. Eur J Gastroenterol Hepatol (2019) 31(9):1121–8. doi: 10.1097/MEG.0000000000001397
42. Hsieh YC, Joo SK, Koo BK, Lin HC, Kim W. Muscle alterations are independently associated with significant fibrosis in patients with nonalcoholic fatty liver disease. Liver Int (2021) 41(3):494–504. doi: 10.1111/liv.14719
43. Hsieh YC, Joo SK, Koo BK, Lin HC, Lee DH, Chang MS, et al. Myosteatosis, but not sarcopenia, predisposes nafld subjects to early steatohepatitis and fibrosis progression. Clin Gastroenterol Hepatol (2023) 21(2):388–97 e10. doi: 10.1016/j.cgh.2022.01.020
44. Harring M, Golabi P, Paik JM, Shah D, Racila A, Cable R, et al. Sarcopenia among patients with nonalcoholic fatty liver disease (Nafld) is associated with advanced fibrosis. Clin Gastroenterol Hepatol (2023) S1542–3565. doi: 10.1016/j.cgh.2023.02.013
45. Cruz-Jentoft AJ, Bahat G, Bauer J, Boirie Y, Bruyere O, Cederholm T, et al. Sarcopenia: revised European consensus on definition and diagnosis. Age Ageing (2019) 48(1):16–31. doi: 10.1093/ageing/afy169
46. Hsu CS, Kao JH. Sarcopenia and chronic liver diseases. Expert Rev Gastroenterol Hepatol (2018) 12(12):1229–44. doi: 10.1080/17474124.2018.1534586
47. Younossi ZM, Koenig AB, Abdelatif D, Fazel Y, Henry L, Wymer M. Global epidemiology of nonalcoholic fatty liver disease-Meta-Analytic assessment of prevalence, incidence, and outcomes. Hepatology (2016) 64(1):73–84. doi: 10.1002/hep.28431
48. Zhang H, Lin S, Gao T, Zhong F, Cai J, Sun Y, et al. Association between sarcopenia and metabolic syndrome in middle-aged and older non-obese adults: a systematic review and meta-analysis. Nutrients (2018) 10(3):364. doi: 10.3390/nu10030364
49. Li AA KD, Ahmed A. Association of sarcopenia and nafld an overeview. Clin Liver Dis (Hoboken) (2020) 16(2):73–6. doi: 10.1002/cld.900
50. Zhang X, He Z, Si Q, Hu X, Yang L, Gu X, et al. The association of sarcopenia and visceral obesity with lean nonalcoholic fatty liver disease in Chinese patients with type 2 diabetes mellitus. J Diabetes Res (2022) 2022:2229139. doi: 10.1155/2022/2229139
51. Joo SK, Kim W. Interaction between sarcopenia and nonalcoholic fatty liver disease. Clin Mol Hepatol (2023) 29(Suppl):S68–78. doi: 10.3350/cmh.2022.0358
52. Harimoto N, Shirabe K, Yamashita YI, Ikegami T, Yoshizumi T, Soejima Y, et al. Sarcopenia as a predictor of prognosis in patients following hepatectomy for hepatocellular carcinoma. Br J Surg (2013) 100(11):1523–30. doi: 10.1002/bjs.9258
53. Alam S, Jahid Hasan M, Khan MAS, Alam M, Hasan N. Effect of weight reduction on histological activity and fibrosis of lean nonalcoholic steatohepatitis patient. J Transl Int Med (2019) 7(3):106–14. doi: 10.2478/jtim-2019-0023
54. Muriel P, Cardoso-Lezama I, Vargas-Pozada EE, Ramos-Tovar E. Mechanisms of non-alcoholic fatty liver disease development in normal-weight individuals. Eur J Gastroenterol Hepatol (2023) 35(5):521–9. doi: 10.1097/MEG.0000000000002530
55. Li C, Guo P, Okekunle AP, Ji X, Huang M, Qi J, et al. Lean non-alcoholic fatty liver disease patients had comparable total caloric, carbohydrate, protein, fat, iron, sleep duration and overtime work as obese non-alcoholic fatty liver disease patients. J Gastroenterol Hepatol (2019) 34(1):256–62. doi: 10.1111/jgh.14360
56. Honarvar B, Bagheri Lankarani K, Keshani P, Rafiee T. Dietary determinants of non-alcoholic fatty liver disease in lean and non-lean adult patients: a population-based study in Shiraz, southern Iran. Hepatitis Monthly (2017) 17(4). doi: 10.5812/hepatmon.44962
57. Younossi ZM, Stepanova M, Negro F, Hallaji S, Younossi Y, Lam B, et al. Nonalcoholic fatty liver disease in lean individuals in the united states. Med (Baltimore) (2012) 91(6):319–27. doi: 10.1097/MD.0b013e3182779d49
58. Cohen JC, Horton JD, Hobbs HH. Human fatty liver disease: old questions and new insights. Science (2011) 332(6037):1519–23. doi: 10.1126/science.1204265
59. Koester AM, Geiser A, Bowman PRT, van de Linde S, Gadegaard N, Bryant NJ, et al. Glut4 translocation and dispersal operate in multiple cell types and are negatively correlated with cell size in adipocytes. Sci Rep (2022) 12(1):20535. doi: 10.1038/s41598-022-24736-y
60. Furuzono S, Kubota T, Taura J, Konishi M, Naito A, Tsutsui M, et al. A xanthene derivative, Ds20060511, attenuates glucose intolerance by inducing skeletal muscle-specific Glut4 translocation in mice. Commun Biol (2021) 4(1):994. doi: 10.1038/s42003-021-02491-6
61. Zaid H, Antonescu CN, Randhawa VK, Klip A. Insulin action on glucose transporters through molecular switches, tracks and tethers. Biochem J (2008) 413(2):201–15. doi: 10.1042/BJ20080723
62. Antonescu CN, Foti M, Sauvonnet N, Klip A. Ready, set, internalize: mechanisms and regulation of Glut4 endocytosis. Biosci Rep (2009) 29(1):1–11. doi: 10.1042/BSR20080105
63. Kalyani RR, Corriere M, Ferrucci L. Age-related and disease-related muscle loss: the effect of diabetes, obesity, and other diseases. Lancet Diabetes Endocrinol (2014) 2(10):819–29. doi: 10.1016/S2213-8587(14)70034-8
64. Sugimoto K, Tabara Y, Ikegami H, Takata Y, Kamide K, Ikezoe T, et al. Hyperglycemia in non-obese patients with type 2 diabetes is associated with low muscle mass: the multicenter study for clarifying evidence for sarcopenia in patients with diabetes mellitus. J Diabetes Investig (2019) 10(6):1471–9. doi: 10.1111/jdi.13070
65. Gaster M SP, Beck-Nielsen H, Schrøder HD, Handberg A. Glut4 is reduced in slow muscle fibers of type 2 diabetic patients: is insulin resistance in type 2 diabetes a slow, type 1 fiber disease? Diabetes (2001) 50(6):1324–9. doi: 10.2337/diabetes.50.6.1324
66. DeFronzo RA, Tripathy D. Skeletal muscle insulin resistance is the primary defect in type 2 diabetes. Diabetes Care (2009) 32 Suppl 2(Suppl 2):S157–63. doi: 10.2337/dc09-S302
67. Cappellari GG, Semolic A, Zanetti M, Vinci P, Ius M, Guarnieri G, et al. Sarcopenic obesity in free-living older adults detected by the espen-easo consensus diagnostic algorithm: validation in an Italian cohort and predictive value of insulin resistance and altered plasma ghrelin profile. Metabolism (2023), 155595. doi: 10.1016/j.metabol.2023.155595
68. Chung GE, Oh S, Ahn D-W, Kim SH, Jung YJ, Kim JW, et al. Effects of additive interactions among obesity, visceral adiposity, and sarcopenia on nonalcoholic fatty liver disease. Sci Rep (2023) 13(1):3628. doi: 10.1038/s41598-023-30833-3
69. Kawada T. Insulin resistance and sarcopenia are closely related to metabolic syndrome in Male and female adolescents. Public Health Nutr (2016) 19(8):1528. doi: 10.1017/S1368980015002475
70. Nelson ME, Madsen S, Cooke KC, Fritzen AM, Thorius IH, Masson SWC, et al. Systems-level analysis of insulin action in mouse strains provides insight into tissue- and pathway-specific interactions that drive insulin resistance. Cell Metab (2022) 34(2):227–39. doi: 10.1016/j.cmet.2021.12.013
71. Al-Muzafar HM, Alshehri FS, Amin KA. The role of pioglitazone in antioxidant, anti-inflammatory, and insulin sensitivity in a high fat-carbohydrate diet-induced rat model of insulin resistance. Braz J Med Biol Res (2021) 54(8):e10782. doi: 10.1590/1414-431X2020e10782
72. Cao C, Koh H-CE, Van Vliet S, Patterson BW, Reeds DN, Laforest R, et al. Increased plasma fatty acid clearance, not fatty acid concentration, is associated with muscle insulin resistance in people with obesity. Metabolism (2022) 132:155216. doi: 10.1016/j.metabol.2022.155216
73. Bhat N, Mani A. Dysregulation of lipid and glucose metabolism in nonalcoholic fatty liver disease. Nutrients (2023) 15(10):2323. doi: 10.3390/nu15102323
74. Cui C, Han S, Shen X, He H, Chen Y, Zhao J, et al. Islr regulates skeletal muscle atrophy Via Igf1-Pi3k/Akt-Foxo signaling pathway. Cell Tissue Res (2020) 381(3):479–92. doi: 10.1007/s00441-020-03251-4
75. Metz M, Beghini M, Wolf P, Pfleger L, Hackl M, Bastian M, et al. Leptin increases hepatic triglyceride export Via a vagal mechanism in humans. Cell Metab (2022) 34(11):1719–31. doi: 10.1016/j.cmet.2022.09.020
76. Smith GI, Shankaran M, Yoshino M, Schweitzer GG, Chondronikola M, Beals JW, et al. Insulin resistance drives hepatic De novo lipogenesis in nonalcoholic fatty liver disease. J Clin Invest (2020) 130(3):1453–60. doi: 10.1172/JCI134165
77. Seko T, Akasaka H, Koyama M, Himuro N, Saitoh S, Miura T, et al. Preserved lower limb muscle mass prevents insulin resistance development in nondiabetic older adults. J Am Med Directors Assoc (2023) 24(3):376–81. doi: 10.1016/j.jamda.2022.12.002
78. Ahmad SS, Ahmad K, Lee EJ, Lee YH, Choi I. Implications of insulin-like growth factor-1 in skeletal muscle and various diseases. Cells (2020) 9(8):1773. doi: 10.3390/cells9081773
79. Sakai H, Asami M, Naito H, Kitora S, Suzuki Y, Miyauchi Y, et al. Exogenous insulin-like growth factor 1 attenuates cisplatin-induced muscle atrophy in mice. J Cachexia Sarcopenia Muscle (2021) 12(6):1570–81. doi: 10.1002/jcsm.12760
80. Hata S, Mori H, Yasuda T, Irie Y, Yamamoto T, Umayahara Y, et al. A low serum igf-1 is correlated with sarcopenia in subjects with type 1 diabetes mellitus: findings from a Post-hoc analysis of the idiamond study. Diabetes Res Clin Pract (2021) 179:108998. doi: 10.1016/j.diabres.2021.108998
81. Scott D TT, Skinner E, Clark RA, Levinger P, Haines TP, Sanders KM, et al. Associations of calf inter- and intra-muscular adipose tissue with cardiometabolic health and physical function in community-dwelling older adults. J Musculoskelet Neuronal Interact (2015) 15(4):350–7.
82. Lin Y-A, Li Y-R, Chang Y-C, Hsu M-C, Chen S-T. Activation of igf-1 pathway and suppression of atrophy related genes are involved in epimedium extract (Icariin) promoted C2c12 myotube hypertrophy. Sci Rep (2021) 11(1):10790. doi: 10.1038/s41598-021-89039-0
83. Takahashi Y, Iida K, Takahashi K, Yoshioka S, Fukuoka H, Takeno R, et al. Growth hormone reverses nonalcoholic steatohepatitis in a patient with adult growth hormone deficiency. Gastroenterology (2007) 132(3):938–43. doi: 10.1053/j.gastro.2006.12.024
84. Lonardo A, Loria P, Leonardi F, Ganazzi D, Carulli N. Growth hormone plasma levels in nonalcoholic fatty liver disease. Am J Gastroenterol (2002) 97(4):1071–2. doi: 10.1111/j.1572-0241.2002.05641.x
85. Ichikawa T, Hamasaki K, Ishikawa H, Ejima E, Eguchi K, Nakao K. Non-alcoholic steatohepatitis and hepatic steatosis in patients with adult onset growth hormone deficiency. Gut (2003) 52(6):914. doi: 10.1136/gut.52.6.914
86. Koo BK, Kim D, Joo SK, Kim JH, Chang MS, Kim BG, et al. Sarcopenia is an independent risk factor for non-alcoholic steatohepatitis and significant fibrosis. J Hepatol (2017) 66(1):123–31. doi: 10.1016/j.jhep.2016.08.019
87. Harwood HJ Jr. The adipocyte as an endocrine organ in the regulation of metabolic homeostasis. Neuropharmacology (2012) 63(1):57–75. doi: 10.1016/j.neuropharm.2011.12.010
88. Tilg H, Moschen AR. Adipocytokines: mediators linking adipose tissue, inflammation and immunity. Nat Rev Immunol (2006) 6(10):772–83. doi: 10.1038/nri1937
89. Pellegrinelli V RC, Rodriguez-Cuenca S, Albert V, Edom-Vovard F, Vidal-Puig A, Clément K, et al. Human adipocytes induce inflammation and atrophy in muscle cells during obesity. Diabetes (2015) 64(9):3121–34. doi: 10.2337/db14-0796
90. Lim JP, Leung BP, Ding YY, Tay L, Ismail NH, Yeo A, et al. Monocyte chemoattractant protein-1: a proinflammatory cytokine elevated in sarcopenic obesity. Clin Interv Aging (2015) 10:605–9. doi: 10.2147/CIA.S78901
91. Straub LG, Scherer PE. Insulin sensitive human adipocytes for in vitro studies. Nat Rev Endocrinol (2022) 18(10):591–2. doi: 10.1038/s41574-022-00727-x
92. Sun L, Fu J, Mu Z, Duan X, Chan P, Xiu S. Association between body fat and sarcopenia in older adults with type 2 diabetes mellitus: a cross-sectional study. Front In Endocrinol (2023) 14:1094075. doi: 10.3389/fendo.2023.1094075
93. Irazoki A, Martinez-Vicente M, Aparicio P, Aris C, Alibakhshi E, Rubio-Valera M, et al. Coordination of mitochondrial and lysosomal homeostasis mitigates inflammation and muscle atrophy during aging. Aging Cell (2022) 21(4):e13583. doi: 10.1111/acel.13583
94. Rom O, Reznick AZ. The role of E3 ubiquitin-ligases murf-1 and mafbx in loss of skeletal muscle mass. Free Radic Biol Med (2016) 98:218–30. doi: 10.1016/j.freeradbiomed.2015.12.031
95. Wang Z, Bo H, Song Y, Li C, Zhang Y. Mitochondrial ros produced by skeletal muscle mitochondria promote the decisive signal for uprmt activation. BioMed Res Int (2022) 2022:7436577. doi: 10.1155/2022/7436577
96. Passos JF, Saretzki G, Ahmed S, Nelson G, Richter T, Peters H, et al. Mitochondrial dysfunction accounts for the stochastic heterogeneity in telomere-dependent senescence. PloS Biol (2007) 5(5):e110. doi: 10.1371/journal.pbio.0050110
97. Urbina-Varela R CN, Videla LA, Del Campo A. Impact of mitophagy and mitochondrial unfolded protein response as new adaptive mechanisms underlying old pathologies: sarcopenia and non-alcoholic fatty liver disease. Int J Mol Sci (2020) 21(20):7704. doi: 10.3390/ijms21207704
98. Oh S, Choi CH, Lee B-J, Park J-H, Son K-H, Byun K. Fermented oyster extract attenuated dexamethasone-induced muscle atrophy by decreasing oxidative stress. Molecules (2021) 26(23):7128. doi: 10.3390/molecules26237128
99. Singh H, Kim D, Kim E, Bemben MG, Anderson M, Seo DI, et al. Jump test performance and sarcopenia status in men and women, 55 to 75 years of age. J Geriatr Phys Ther (2014) 37(2):76–82. doi: 10.1519/JPT.0b013e3182a51b11
100. Dufour AB, Hannan MT, Murabito JM, Kiel DP, McLean RR. Sarcopenia definitions considering body size and fat mass are associated with mobility limitations: the framingham study. J Gerontol A Biol Sci Med Sci (2013) 68(2):168–74. doi: 10.1093/gerona/gls109
101. Ryan AS, Li G. Adipose and skeletal muscle expression of adiponectin and liver receptor homolog-1 with weight loss and aerobic exercise. J Endocr Soc (2022) 6(8):bvac095. doi: 10.1210/jendso/bvac095
102. Tanyanskiy DA, Shavva VS, Dizhe EB, Oleinikova GN, Lizunov AV, Nekrasova EV, et al. Adiponectin stimulates apolipoprotein a-1 gene expression in Hepg2 cells Via ampk, pparα, and lxrs signaling mechanisms. Biochem (Mosc) (2022) 87(11):1252–9. doi: 10.1134/S0006297922110049
103. Song N, Xu H, Wu S, Luo S, Xu J, Zhao Q, et al. Synergistic activation of ampk by Adipor1/2 agonist and inhibitor of edps-ebp interaction recover nafld through enhancing mitochondrial function in mice. Acta Pharm Sin B (2023) 13(2):542–58. doi: 10.1016/j.apsb.2022.10.003
104. Wood CL, van ‘t Hof R, Dillon S, Straub V, Wong SC, Ahmed SF, et al. Combined growth hormone and insulin-like growth factor-1 rescues growth retardation in glucocorticoid-treated mdxmice but does not prevent osteopenia. J Endocrinol (2022) 253(2):63–74. doi: 10.1530/JOE-21-0388
105. Spradlin RA, Vassilakos G, Matheny MK, Jones NC, Goldman JL, Lei H, et al. Deletion of muscle Igf1 exacerbates disuse atrophy weakness in mice. J Appl Physiol (Bethesda Md: 1985) (2021) 131(3):881–94. doi: 10.1152/japplphysiol.00090.2021
106. Cabrera D, Ruiz A, Cabello-Verrugio C, Brandan E, Estrada L, Pizarro M, et al. Diet-induced nonalcoholic fatty liver disease is associated with sarcopenia and decreased serum insulin-like growth factor-1. Dig Dis Sci (2016) 61(11):3190–8. doi: 10.1007/s10620-016-4285-0
107. Kosmalski M, Drzewoski J, Szymczak-Pajor I, Zieleniak A, Mikolajczyk-Solinska M, Kasznicki J, et al. Irisin is related to non-alcoholic fatty liver disease (Nafld). Biomedicines (2022) 10(9):2253. doi: 10.3390/biomedicines10092253
108. Lee HJ, Lee JO, Kim N, Kim JK, Kim HI, Lee YW, et al. Irisin, a novel myokine, regulates glucose uptake in skeletal muscle cells Via ampk. Mol Endocrinol (2015) 29(6):873–81. doi: 10.1210/me.2014-1353
109. Qiu S, Wang Q, Cai X, Sun Z, Wu T. Circulating irisin in nonalcoholic fatty liver disease: an updated meta-analysis. Endokrynol Pol (2023) 74(1):47–54. doi: 10.5603/EP.a2022.0067
110. Namjou B, Lingren T, Huang Y, Parameswaran S, Cobb BL, Stanaway IB, et al. Gwas and enrichment analyses of non-alcoholic fatty liver disease identify new trait-associated genes and pathways across emerge network. BMC Med (2019) 17(1):135. doi: 10.1186/s12916-019-1364-z
111. Feldman A, Eder SK, Felder TK, Kedenko L, Paulweber B, Stadlmayr A, et al. Clinical and metabolic characterization of lean Caucasian subjects with non-alcoholic fatty liver. Am J Gastroenterol (2017) 112(1):102–10. doi: 10.1038/ajg.2016.318
112. Wijarnpreecha K, Scribani M, Raymond P, Harnois DM, Keaveny AP, Ahmed A, et al. Pnpla3 gene polymorphism and liver- and extrahepatic cancer-related mortality in the united states. Clin Gastroenterol Hepatol (2021) 19(5):1064–6. doi: 10.1016/j.cgh.2020.04.058
113. Li J, Wu N, Yang Y, Zhai X, Yuan F, Zhang F, et al. Unique genetic variants of lean nonalcoholic fatty liver disease: a retrospective cohort study. BMC Endocr Disord (2023) 23(1):11. doi: 10.1186/s12902-022-01234-w
114. Wu N, Zhai X, Yuan F, Li J, Yu N, Zhang F, et al. Fasting glucose mediates the influence of genetic variants of Sod2 gene on lean non-alcoholic fatty liver disease. Front Genet (2022) 13:970854. doi: 10.3389/fgene.2022.970854
115. Bale G, Vishnubhotla RV, Mitnala S, Sharma M, Padaki RN, Pawar SC, et al. Whole-exome sequencing identifies a variant in phosphatidylethanolamine n-methyltransferase gene to be associated with lean-nonalcoholic fatty liver disease. J Clin Exp Hepatol (2019) 9(5):561–8. doi: 10.1016/j.jceh.2019.02.001
116. Klimentidis YC, Bea JW, Thompson P, Klimecki WT, Hu C, Wu G, et al. Genetic variant in Acvr2b is associated with lean mass. Med Sci Sports Exerc (2016) 48(7):1270–5. doi: 10.1249/MSS.0000000000000889
117. Roth SM. Genetic aspects of skeletal muscle strength and mass with relevance to sarcopenia. Bonekey Rep (2012) 1:58. doi: 10.1038/bonekey.2012.58
118. Di Renzo L, Sarlo F, Petramala L, Iacopino L, Monteleone G, Colica C, et al. Association between -308 g/a tnf-α polymorphism and appendicular skeletal muscle mass index as a marker of sarcopenia in normal weight obese syndrome. Dis Markers (2013) 35(6):615–23. doi: 10.1155/2013/983424
119. Yahagi N, Shimano H, Matsuzaka T, Sekiya M, Najima Y, Okazaki S, et al. P53 involvement in the pathogenesis of fatty liver disease. J Biol Chem (2004) 279(20):20571–5. doi: 10.1074/jbc.M400884200
120. Porrello A, Cerone MA, Coen S, Gurtner A, Fontemaggi G, Cimino L, et al. P53 regulates myogenesis by triggering the differentiation activity of prb. J Cell Biol (2000) 151(6):1295–304. doi: 10.1083/jcb.151.6.1295
121. Di Renzo L, Gratteri S, Sarlo F, Cabibbo A, Colica C, De Lorenzo A. Individually tailored screening of susceptibility to sarcopenia using P53 codon 72 polymorphism, phenotypes, and conventional risk factors. Dis Markers (2014) 2014:743634. doi: 10.1155/2014/743634
122. Heffernan SM, Stebbings GK, Kilduff LP, Erskine RM, Day SH, Morse CI, et al. Fat mass and obesity associated (Fto) gene influences skeletal muscle phenotypes in non-resistance trained males and elite rugby playing position. BMC Genet (2017) 18(1):4. doi: 10.1186/s12863-017-0470-1
123. Khayyat YM. Lean non-alcoholic fatty liver disease and associated metabolic disturbance: a Saudi Arabian cross-sectional study. Physiol Rep (2021) 9(14):e14949. doi: 10.14814/phy2.14949
124. Delmonico MJ, Kostek MC, Doldo NA, Hand BD, Walsh S, Conway JM, et al. Alpha-Actinin-3 (Actn3) R577x polymorphism influences knee extensor peak power response to strength training in older men and women. Journals Gerontology Ser A Biol Sci Med Sci (2007) 62(2):206–12. doi: 10.1093/gerona/62.2.206
125. Hopkinson NS, Li KW, Kehoe A, Humphries SE, Roughton M, Moxham J, et al. Vitamin d receptor genotypes influence quadriceps strength in chronic obstructive pulmonary disease. Am J Clin Nutr (2008) 87(2):385–90. doi: 10.1093/ajcn/87.2.385
126. Kikuchi N, Yoshida S, S-k M, Lee K, Sakamaki-Sunaga M, Okamoto T, et al. The Actn3 R577x genotype is associated with muscle function in a Japanese population. Appl Physiol Nutr Metab (2015) 40(4):316–22. doi: 10.1139/apnm-2014-0346
127. Kostek MC, Delmonico MJ, Reichel JB, Roth SM, Douglass L, Ferrell RE, et al. Muscle strength response to strength training is influenced by insulin-like growth factor 1 genotype in older adults. J Appl Physiol (Bethesda Md: 1985) (2005) 98(6):2147–54. doi: 10.1152/japplphysiol.00817.2004
128. Li C-I, Li T-C, Liao L-N, Liu C-S, Yang C-W, Lin C-H, et al. Joint effect of gene-physical activity and the interactions among crp, tnf-α, and lta polymorphisms on serum crp, tnf-α levels, and handgrip strength in community-dwelling elders in Taiwan - tchs-e. Age (Dordrecht Netherlands) (2016) 38(2):46. doi: 10.1007/s11357-016-9909-y
129. Lima RM, Leite TKM, Pereira RW, Rabelo HT, Roth SM, Oliveira RJ. Ace and Actn3 genotypes in older women: muscular phenotypes. Int J Sports Med (2011) 32(1):66–72. doi: 10.1055/s-0030-1267229
130. Xia Z, Man Q, Li L, Song P, Jia S, Song S, et al. Vitamin d receptor gene polymorphisms modify the association of serum 25-hydroxyvitamin d levels with handgrip strength in the elderly in northern China. Nutr (Burbank Los Angeles County Calif) (2019) 57:202–7. doi: 10.1016/j.nut.2018.05.025
131. Aneni EC, Bittencourt MS, Teng C, Cainzos-Achirica M, Osondu CU, Soliman A, et al. The risk of cardiometabolic disorders in lean non-alcoholic fatty liver disease: a longitudinal study. Am J Prev Cardiol (2020) 4:100097. doi: 10.1016/j.ajpc.2020.100097
132. Kim Y, Han E, Lee JS, Lee HW, Kim BK, Kim MK, et al. Cardiovascular risk is elevated in lean subjects with nonalcoholic fatty liver disease. Gut Liver (2022) 16(2):290–9. doi: 10.5009/gnl210084
133. Deprince A, Haas JT, Staels B. Dysregulated lipid metabolism links nafld to cardiovascular disease. Mol Metab (2020) 42:101092. doi: 10.1016/j.molmet.2020.101092
134. Zhu X, Huang Q, Ma S, Chen L, Wu Q, Wu L, et al. Presence of sarcopenia identifies a special group of lean nafld in middle-aged and older people. Hepatol Int (2023) 17(2):313–25. doi: 10.1007/s12072-022-10439-z
135. Park D, Lee D-C, Kim Y. Relationship between relative grip strength and serum total cholesterol, high-density lipoprotein cholesterol, low-density lipoprotein cholesterol, and triglyceride levels in Korean middle-aged or older adults: a panel regression model. Metab Syndr Relat Disord (2022) 20(9):517–23. doi: 10.1089/met.2022.0027
136. Al-Khaifi A, Rudling M, Angelin B. An fxr agonist reduces bile acid synthesis independently of increases in Fgf19 in healthy volunteers. Gastroenterology (2018) 155(4):1012–6. doi: 10.1053/j.gastro.2018.06.038
137. Arab JP, Karpen SJ, Dawson PA, Arrese M, Trauner M. Bile acids and nonalcoholic fatty liver disease: molecular insights and therapeutic perspectives. Hepatology (2017) 65(1):350–62. doi: 10.1002/hep.28709
138. Tacchi F, Orozco-Aguilar J, Valero-Breton M, Cabello-Verrugio C. Bile acids alter the autophagy and mitogenesis in skeletal muscle cells. Adv Exp Med Biol (2023) 1408:183–99. doi: 10.1007/978-3-031-26163-3_10
139. Abrigo J, Olguín H, Gutierrez D, Tacchi F, Arrese M, Cabrera D, et al. Bile acids induce alterations in mitochondrial function in skeletal muscle fibers. Antioxidants (Basel) (2022) 11(9):1706. doi: 10.3390/antiox11091706
140. Orozco-Aguilar J, Tacchi F, Aguirre F, Valero-Breton M, Castro-Sepulveda M, Simon F, et al. Ursodeoxycholic acid induces sarcopenia associated with decreased protein synthesis and autophagic flux. Biol Res (2023) 56(1):28. doi: 10.1186/s40659-023-00431-8
141. Eshraghian A, Nikeghbalian S, Geramizadeh B, Kazemi K, Shamsaeefar A, Malek-Hosseini SA. Characterization of biopsy proven non-alcoholic fatty liver disease in healthy non-obese and lean population of living liver donors: the impact of uric acid. Clin Res Hepatol Gastroenterol (2020) 44(4):572–8. doi: 10.1016/j.clinre.2019.09.002
142. Zhang YN, Wang QQ, Chen YS, Shen C, Xu CF. Association between serum uric acid to hdl-cholesterol ratio and nonalcoholic fatty liver disease in lean Chinese adults. Int J Endocrinol (2020) 2020:5953461. doi: 10.1155/2020/5953461
143. Papadopoulou SK, Voulgaridou G, Kondyli FS, Drakaki M, Sianidou K, Andrianopoulou R, et al. Nutritional and nutrition-related biomarkers as prognostic factors of sarcopenia, and their role in disease progression. Diseases (2022) 10(3):42. doi: 10.3390/diseases10030042
144. Liu X, Chen X, Hu F, Xia X, Hou L, Zhang G, et al. Higher uric acid serum levels are associated with sarcopenia in West China: a cross-sectional study. BMC Geriatr (2022) 22(1):121. doi: 10.1186/s12877-022-02817-x
145. Molino-Lova R, Sofi F, Pasquini G, Vannetti F, Del Ry S, Vassalle C, et al. Higher uric acid serum levels are associated with better muscle function in the oldest old: results from the mugello study. Eur J Intern Med (2017) 41:39–43. doi: 10.1016/j.ejim.2017.03.014
146. Yi D, Lee MJ, Khang AR, Kang YH. Association between serum uric acid and relative hand grip strength in comparison with metabolic syndrome components. Osteoporos Sarcopenia (2022) 8(4):158–64. doi: 10.1016/j.afos.2022.10.001
147. Schnabl B, Brenner DA. Interactions between the intestinal microbiome and liver diseases. Gastroenterology (2014) 146(6):1513–24. doi: 10.1053/j.gastro.2014.01.020
148. Wang B, Jiang X, Cao M, Ge J, Bao Q, Tang L, et al. Altered fecal microbiota correlates with liver biochemistry in nonobese patients with non-alcoholic fatty liver disease. Sci Rep (2016) 6:32002. doi: 10.1038/srep32002
149. Miele L, Valenza V, La Torre G, Montalto M, Cammarota G, Ricci R, et al. Increased intestinal permeability and tight junction alterations in nonalcoholic fatty liver disease. Hepatology (2009) 49(6):1877–87. doi: 10.1002/hep.22848
150. Alberto González-Regueiro J, Moreno-Castañeda L, Uribe M, Carlos Chávez-Tapia N. The role of bile acids in glucose metabolism and their relation with diabetes. Ann Hepatol (2017) 16:S15–20. doi: 10.5604/01.3001.0010.5494
151. Jiao N, Baker SS, Chapa-Rodriguez A, Liu W, Nugent CA, Tsompana M, et al. Suppressed hepatic bile acid signalling despite elevated production of primary and secondary bile acids in nafld. Gut (2018) 67(10):1881–91. doi: 10.1136/gutjnl-2017-314307
152. Phipps M, Wattacheril J. Non-alcoholic fatty liver disease (Nafld) in non-obese individuals. Frontline Gastroenterol (2020) 11(6):478–83. doi: 10.1136/flgastro-2018-101119
153. Nikkhah A, Ejtahed H-S, Ettehad Marvasti F, Taghavi M, Pakmehr A, Hajipour F, et al. The critical role of gut microbiota dysbiosis in skeletal muscle wasting: a systematic review. J Appl Microbiol (2023) 134(1):lxac014. doi: 10.1093/jambio/lxac014
154. Yan X, Xie R, Ding L, Cheng X, Xu J, Lin L, et al. Relationships between sarcopenia, nutrient intake, and gut microbiota in Chinese community-dwelling older women. Arch Gerontol Geriatr (2023) 113:105063. doi: 10.1016/j.archger.2023.105063
155. Wang Z, Xu X, Deji Y, Gao S, Wu C, Song Q, et al. Bifidobacterium as a potential biomarker of sarcopenia in elderly women. Nutrients (2023) 15(5):1266. doi: 10.3390/nu15051266
156. Brinkmann C, Hof H, Gysan D-B, Albus C, Millentrup S, Bjarnason-Wehrens B, et al. Lifestyle intervention reduces risk score for cardiovascular mortality in company employees with pre-diabetes or diabetes mellitus - a secondary analysis of the preford randomized controlled trial with 3 years of follow-up. Front In Endocrinol (2023) 14:1106334. doi: 10.3389/fendo.2023.1106334
157. Biolo G, Cederholm T, Muscaritoli M. Muscle contractile and metabolic dysfunction is a common feature of sarcopenia of aging and chronic diseases: from sarcopenic obesity to cachexia. Clin Nutr (2014) 33(5):737–48. doi: 10.1016/j.clnu.2014.03.007
158. Wong VW-S, Wong GL-H, Chan RS-M, Shu SS-T, Cheung BH-K, Li LS, et al. Beneficial effects of lifestyle intervention in non-obese patients with non-alcoholic fatty liver disease. J Hepatol (2018) 69(6):1349–56. doi: 10.1016/j.jhep.2018.08.011
159. Steffl M, Bohannon RW, Sontakova L, Tufano JJ, Shiells K, Holmerova I. Relationship between sarcopenia and physical activity in older people: a systematic review and meta-analysis. Clin Interv Aging (2017) 12:835–45. doi: 10.2147/CIA.S132940
160. Joo SK KW. Interaction between sarcopenia and nafld. Clin Mol Hepatol (2023) 29Suppl:S68-S78. doi: 10.3350/cmh.2022.0358
161. Johnson NA, Sachinwalla T, Walton DW, Smith K, Armstrong A, Thompson MW, et al. Aerobic exercise training reduces hepatic and visceral lipids in obese individuals without weight loss. Hepatology (2009) 50(4):1105–12. doi: 10.1002/hep.23129
162. Jung WS, Kim YY, Park HY. Circuit training improvements in Korean women with sarcopenia. Percept Mot Skills (2019) 126(5):828–42. doi: 10.1177/0031512519860637
163. Mori H, Tokuda Y. Effect of whey protein supplementation after resistance exercise on the muscle mass and physical function of healthy older women: a randomized controlled trial. Geriatr Gerontol Int (2018) 18(9):1398–404. doi: 10.1111/ggi.13499
164. Takeuchi I, Yoshimura Y, Shimazu S, Jeong S, Yamaga M, Koga H. Effects of branched-chain amino acids and vitamin d supplementation on physical function, muscle mass and strength, and nutritional status in sarcopenic older adults undergoing hospital-based rehabilitation: a multicenter randomized controlled trial. Geriatr Gerontol Int (2019) 19(1):12–7. doi: 10.1111/ggi.13547
165. Tokuda Y, Mori H. Essential amino acid and tea catechin supplementation after resistance exercise improves skeletal muscle mass in older adults with sarcopenia: an open-label, pilot, randomized controlled trial. J Am Nutr Assoc (2023) 42(3):255–62. doi: 10.1080/07315724.2022.2025546
166. Wang Z, Xu X, Gao S, Wu C, Song Q, Shi Z, et al. Effects of Internet-based nutrition and exercise interventions on the prevention and treatment of sarcopenia in the elderly. Nutrients (2022). 14(12):2458. doi: 10.3390/nu14122458
167. Yoshimura Y, Bise T, Shimazu S, Tanoue M, Tomioka Y, Araki M, et al. Effects of a leucine-enriched amino acid supplement on muscle mass, muscle strength, and physical function in post-stroke patients with sarcopenia: a randomized controlled trial. Nutrition (2019) 58:1–6. doi: 10.1016/j.nut.2018.05.028
168. Zhu LY, Chan R, Kwok T, Cheng KC, Ha A, Woo J. Effects of exercise and nutrition supplementation in community-dwelling older Chinese people with sarcopenia: a randomized controlled trial. Age Ageing (2019) 48(2):220–8. doi: 10.1093/ageing/afy179
169. Urban RJ, Dillon EL, Choudhary S, Zhao Y, Horstman AM, Tilton RG, et al. Translational studies in older men using testosterone to treat sarcopenia. Trans Am Clin Climatol Assoc (2014) 125:27–42.
170. Rooks D, Praestgaard J, Hariry S, Laurent D, Petricoul O, Perry RG, et al. Treatment of sarcopenia with bimagrumab: results from a phase ii, randomized, controlled, proof-of-Concept study. J Am Geriatr Soc (2017) 65(9):1988–95. doi: 10.1111/jgs.14927
171. Sørensen MB, Rosenfalck AM, Højgaard L, Ottesen B. Obesity and sarcopenia after menopause are reversed by sex hormone replacement therapy. Obes Res (2001) 9(10):622–6. doi: 10.1038/oby.2001.81
172. Tyagi S, Gupta P, Saini AS, Kaushal C, Sharma S. The peroxisome proliferator-activated receptor: a family of nuclear receptors role in various diseases. J Adv Pharm Technol Res (2011) 2(4):236–40. doi: 10.4103/2231-4040.90879
173. Bril F, Kalavalapalli S, Clark VC, Lomonaco R, Soldevila-Pico C, Liu IC, et al. Response to pioglitazone in patients with nonalcoholic steatohepatitis with vs without type 2 diabetes. Clin Gastroenterol Hepatol (2018) 16(4):558–66 e2. doi: 10.1016/j.cgh.2017.12.001
174. Daniel S Hsia OG, Cefalu WT. An update on Sglt2 inhibitors for the treatment of diabetes mellitus. Curr Opin Endocrinol Diabetes Obes (2017) 24(1):73–9. doi: 10.1097/MED
175. Phrueksotsai S, Pinyopornpanish K, Euathrongchit J, Leerapun A, Phrommintikul A, Buranapin S, et al. The effects of dapagliflozin on hepatic and visceral fat in type 2 diabetes patients with non-alcoholic fatty liver disease. J Gastroenterol Hepatol (2021) 36(10):2952–9. doi: 10.1111/jgh.15580
176. Fruchart JC BHJ, Leitersdorf E. Consensus for the use of fibrates in the treatment of dyslipoproteinemia and coronary heart disease. Fibrate Consensus Group Am J Cardiol (1998) 81(7):912–7. doi: 10.1016/s0002-9149(98)00010-1
Keywords: lean non-alcoholic fatty liver disease, sarcopenia, insulin resistance, visceral obesity, metabolic inflammation
Citation: Chen M, Cao Y, Ji G and Zhang L (2023) Lean nonalcoholic fatty liver disease and sarcopenia. Front. Endocrinol. 14:1217249. doi: 10.3389/fendo.2023.1217249
Received: 05 May 2023; Accepted: 13 June 2023;
Published: 23 June 2023.
Edited by:
Elena Piccinin, University of Bari Aldo Moro, ItalyReviewed by:
Teresa Rubio-Tomás, University of Crete, GreeceMoris Sangineto, University of Foggia, Italy
Copyright © 2023 Chen, Cao, Ji and Zhang. This is an open-access article distributed under the terms of the Creative Commons Attribution License (CC BY). The use, distribution or reproduction in other forums is permitted, provided the original author(s) and the copyright owner(s) are credited and that the original publication in this journal is cited, in accordance with accepted academic practice. No use, distribution or reproduction is permitted which does not comply with these terms.
*Correspondence: Li Zhang, emhhbmdsaS5obEAxNjMuY29t