- 1School of Traditional Chinese Medicine, Shandong University of Traditional Chinese Medicine, Jinan, China
- 2Department of Pharmacy, Zhongshan Hospital of Traditional Chinese Medicine, Zhong Shan, China
- 3School of Pharmacy, Shandong University of Traditional Chinese Medicine, Jinan, China
- 4College of Foreign Languages, Shandong University of Traditional Chinese Medicine, Jinan, China
- 5Department of Neurosurgery, The Second Affiliated Hospital of Fujian Medical University, Quanzhou, China
- 6Innovation Research Institute of Chinese Medicine, Shandong University of Traditional Chinese Medicine, Jinan, China
Premenstrual syndrome (PMS) occurs recurrently during the luteal phase of a woman’s menstrual cycle and disappears after menstruation ends. It is characterized by abnormal changes in both the body and mood, and in certain cases, severe disruptions in daily life and even suicidal tendencies. Current drugs for treating PMS, such as selective serotonin reuptake inhibitors, do not yield satisfactory results. Orexin, a neuropeptide produced in the lateral hypothalamus, is garnering attention in the treatment of neurological disorders and is believed to modulate the symptoms of PMS. This paper reviews the advancements in research on sleep disturbances, mood changes, and cognitive impairment caused by PMS, and suggests potential pathways for orexin to address these symptoms. Furthermore, it delves into the role of orexin in the molecular mechanisms underlying PMS. Orexin regulates steroid hormones, and the cyclic fluctuations of estrogen and progesterone play a crucial role in the pathogenesis of PMS. Additionally, orexin also modulates the gamma-aminobutyric acid (GABA) system and the inflammatory response involved in coordinating the mechanism of PMS. Unraveling the role of orexin in the pathogenesis of PMS will not only aid in understanding the etiology of PMS but also hold implications for orexin as a novel target for treating PMS.
1 Introduction
PMS is a common issue among women of childbearing age. The American College of Obstetricians and Gynecologists (ACOG), in its guidelines for women’s health, defines PMS as the cyclical onset of affective and somatic symptoms that occur 7 to 14 days before menstruation (luteal phase). Affective symptoms include anxiety, confusion, depression, irritability, and social withdrawal, while somatic symptoms encompass breast tenderness or swelling, headaches, joint or muscle pain, swelling of the extremities, and weight gain, with at least one affective and one somatic symptom (1, 2). Premenstrual dysphoric disorder (PMDD) represents a severe form of PMS. According to the Diagnostic and Statistical Manual of Mental Disorders (DSM), 3-8% of women of childbearing age are diagnosed with PMDD (3). These patients exhibit symptoms such as significant mood swings, subjective difficulties in concentrating, obvious changes in appetite, lethargy, or insomnia. Moreover, their conditions may exacerbate the progression of disorders like major depression, panic disorder, and personality disorders, significantly impacting work, studies, daily social activities, and relationships with others (4, 5). Therefore, it becomes increasingly important to gain a clearer understanding of the neurological aspects of premenstrual depression. Modern medical research has yet to establish a clear and consistent definition of the pathogenesis of PMS. Most experts believe it arises from the interaction of several factors (6), including dysregulation of hormone levels, especially estrogen and progesterone, which forms the primary theory for the development of PMS. Neurotransmitters such as 5-hydroxytryptamine (5-HT), dopamine, glutamate, noradrenaline, and GABA impact the psychological manifestations of the syndrome (7, 8). Abnormal responses to inflammatory factors may also be associated with disease development (9) (Figure 1).
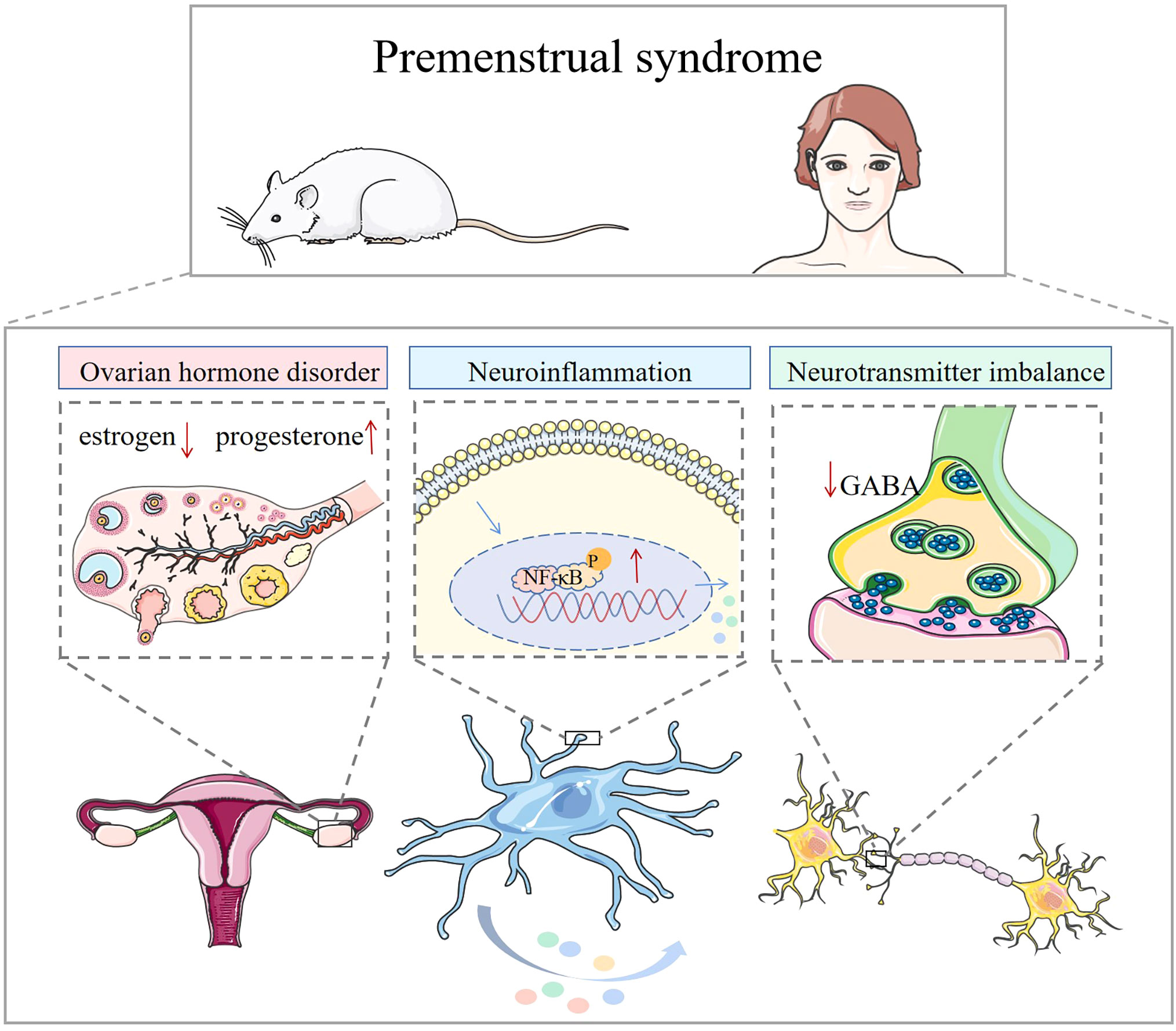
Figure 1 Mechanisms associated with premenstrual syndrome. In animals and humans, ovarian hormone disruption, elevated inflammatory factors, and neurotransmitter imbalance are associated with the development of premenstrual syndrome.
Orexin, also known as hypocretin, derives its name from the Greek word “orexin”, which means ‘appetite’. Sakurai et al. named these neuropeptides ‘orexin’ due to their propensity to promote feeding (10). Based on their similarity to incretins “hypocretin”, de Lecea et al., in 1998 named them “hypocretins” (11). Studies have shown that prepro-hypocretin mRNA is exclusively expressed by neurons in the lateral hypothalamic area (LHA), including the lateral nucleus, posterior nucleus, and dorsomedial nucleus of the hypothalamus (12). Orexin-A and orexin-B are produced from prepro-orexin by enzymatic cleavage, with the former consisting of 33 amino acid residues and two intramolecular disulfide bridges in its N-terminal structural domain, and the latter comprising 28 amino acid residues. Orexin-A and orexin-B share 46% sequence homology (13). Orexin peptides are highly conserved in vertebrates, particularly in mammals: orexin-A has 100% sequence homology, while orexin-B differs by only 1 or 2 amino acids between species (12). Orexin-A exhibits equally high affinity for orexin receptor 1 (OX1R) and orexin receptor 2 (OX2R), while orexin-B demonstrates approximately 10-fold selectivity for OX2R. The orexin neuropeptide system has emerged as a potential new drug target for the treatment of psychiatric disorders (14). Despite orexin’s primary function being the maintenance of wakefulness, animal studies have revealed its influence on emotional, behavioral, and cognitive regulation, encompassing stress response, reward processing, sleep-wake regulation, and feeding (15, 16). This paper examines the relationship between premenstrual syndrome and orexin considering both clinical features and molecular biological mechanisms (Figure 2). This review may establish a theoretical basis for orexin-targeted therapy for PMS/PMDD.
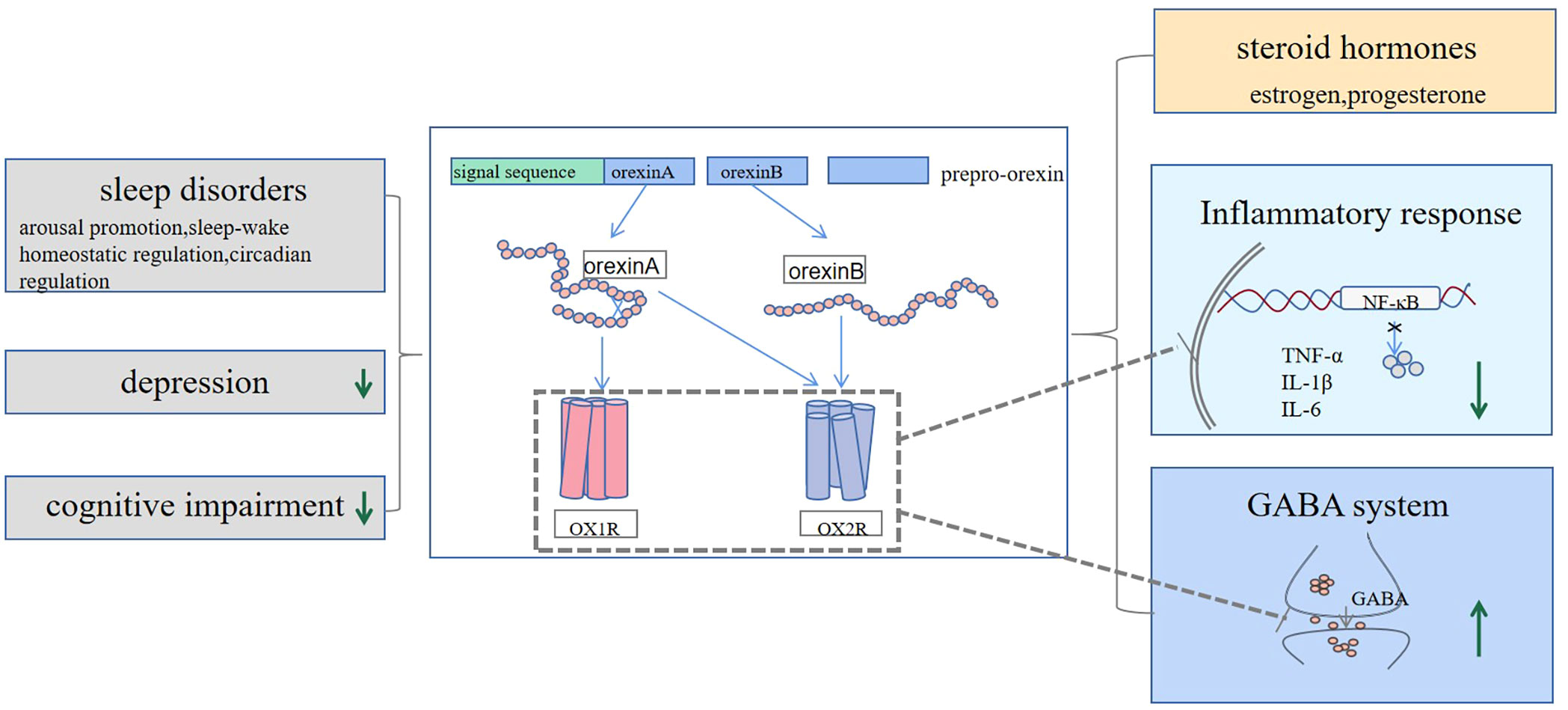
Figure 2 Orexin may have a potential therapeutic effect on PMS symptoms by reducing daytime sleepiness and increasing the duration of rapid eye movement sleep, improving cognitive function, and alleviating depressive mood. Mechanistically, orexin can modulate ovarian hormones, suppress elevated levels of inflammatory factors, and increase the release of GABA neurotransmitters, all of which may contribute to its positive effect on PMS symptoms.
2 Orexin reduced PMS-induced sleep disorders
Sleep disturbances in patients with PMS are typically characterized by subjective sleep disruptions, abnormal sleep electroencephalogram (EEG) patterns, and shifts in sleep-wake rhythm (17–19). In clinical studies, patients with PMS self-reported difficulties with nocturnal awakening and morning fatigue (20), often accompanied by varying levels of sleep deprivation (21). Some patients reported experiencing insomnia, inattention, and fatigue, with greater severity observed in women with PMDD compared to healthy women, especially during the luteal phase as opposed to the follicular phase (22). Conzatti et al. (23) observed in their study that 49.3% of PMS patients experienced excessive daytime sleepiness (EDS) during the luteal phase, with the likelihood of EDS during the follicular phase at 48.8%. In an epidemiological survey, women with PMS exhibited an increased total sleep time as recorded by polysomnography (PSG) and lower peripheral oxygen saturation levels (24). Women with severe PMS also showed significantly increased latency to rapid eye movement (REM) sleep compared to those with no or mild PMS. Additionally, detailed analysis of the sleep EEG revealed subtle differences; when compared with asymptomatic control subjects, women with PMDD demonstrated decreased occurrence of delta waves and increased occurrence and amplitude of theta waveforms in non-REM sleep (25). Furthermore, changes in the circadian rhythm of sleep during the menstrual cycle were observed, with a delayed luteal phase and an earlier follicular phase, in a study involving women with PMS (26). Sleep disorders represent a crucial component of PMS symptoms, warranting more comprehensive investigation and specific treatment measures.
2.1 Clinical studies
Orexin plays a role in promoting wakefulness, regulates sleep-wake homeostasis, and contributes to circadian rhythm control. Orexin signaling peaks during wakefulness and subsequently decreases during slow-wave and REM sleep (27). PSG studies have demonstrated that orexin-A has a stabilizing effect on nocturnal sleep in a large clinical sample of patients with hypersomnia, and different cerebrospinal fluid (CSF) orexin-A levels correlate with nocturnal sleep/wake rhythms (28). Deficiency, loss, and disruption of signaling in orexin neurons can lead to sleep disorders. Research indicates that patients with reduced CSF orexin-A levels showed shortened mean sleep latency (29) and orexin-A deficiency leads to REM sleep disturbances in patients with narcolepsy (30). Narcolepsy type 1 (NT 1) results from the loss of orexin neurons, leading to low levels of orexin neuropeptides in the brain. In a clinical trial investigating this disorder, Evans et al. found that the OX2R agonist danavorexton significantly improved subjective somnolence in NT 1 participants, dose-dependently increased arousal in NT 1 patients, and effectively treated drowsiness symptoms, although the study noted mild side effects associated with the treatment (31). Suvorexant is a novel orexin receptor antagonist that induces and maintains sleep clinically used in treating insomnia. Herring et al. found that patients treated with suvorexant exhibited greater improvements in subjective and objective PSG measures of sleep onset and sleep maintenance compared to the placebo group in a clinical trial for insomnia treatment. However, in assessing drug safety, patients treated with suvorexant presented mild to moderate somnolence (32). Whether it is suvorexant for insomnia or danavorexton for drowsiness, these findings provide evidence for the role of orexin in the treatment of sleep disorders.
2.2 Animal studies
In animal studies, Hung et al. found that the ablation of orexin neurons in the mice brain reduced arousal and resulted in a narcolepsy-like phenotype (33). Murillo-Rodriguez et al. damaged orexin neurons with a neurotoxin in rats and observed that these rats woke up less during the night and had more REM sleep, which is consistent with reduced orexin function (34). Mieda et al. discovered that orexin neuron-ablated mice exhibited narcolepsy, and central administration of orexin-A significantly suppressed cataplectic behavioral arrests and increased wakefulness in these mice (35). Evans et al. (31) found that danavorexton administration increased arousal and reduced arousal fragmentation in orexin/ataxin-3 mice (a narcoleptic mouse model with postnatal hypocretin/orexin cell death) during the active/wakeful period. Furthermore, observations on its pharmacodynamics have informed study design and dose selection for NT 1 cohorts in human studies using intravenous infusion. Additionally, the orexin receptor antagonist suvorexant can promote sleep by blocking OX1R and OX2R (36). Orexin receptor antagonists and agonists provide a novel treatment for sleep disorders, opening new therapeutic avenues in the treatment of PMS/PMDD. The pharmacological role of these drugs in treating sleep disorders in patients with PMS warrants further exploration. These studies offer insights into the treatment of PMS sleep disorders, suggesting that orexin may be a potential target for therapy. However, the distribution and specific mechanisms of orexin and its receptors in PMS/PMDD have not been fully elucidated and may be related to the interaction of orexin with neurotransmitters, such as 5-HT, noradrenaline, acetyl-choline, GABA, and dopamine, etc. Large-scale preclinical and clinical trials are needed to further explore the relevant mechanisms.
3 Orexin improved mood and cognitive impairment
3.1 Orexin improved depression
Patients with PMS and PMDD are characterized by affective disorders, including anxiety and depression, with depressed mood being the main symptom in patients with PMDD (37). Therefore, alleviating mood symptoms is crucial for PMS. The current drugs of choice for the treatment of PMS/PMDD are first-line antidepressants, namely selective serotonin reuptake inhibitors (SSRIs). However, these drugs’ tolerability and dose-dependent nature of adverse effects limit their widespread clinical use (38). In recent years, research on orexin’s ability to alleviate depressive symptoms has been increasingly recognized and validated in preclinical trials (39). Reduced orexin levels are associated with depressed mood in depressed patients (40). Lu et al. also revealed significant gender-related changes in orexin in the hypothalamus of depressed patients, with female patients showing more pronounced orexin immunoreactivity (41). Research and development of drugs targeting orexin signaling is well established (42, 43) and has shown promise in animal experiments. The Orexin system appears to be a promising target for treating the symptoms of PMS/PMDD.
3.1.1 Clinical studies
In humans, changes in orexin levels in the CSF correlate with physical and emotional changes. Orexin levels in peripheral blood cells of patients with major depression were significantly different from those of healthy patients. It was found that orexin-A mRNA expression levels in patients with major depression were negatively correlated with Hamilton Depression Rating Scale scores (used to assess depressive states) (44). Brundin et al. analyzed 66 patients with major depression who attempted suicide with significantly lower CSF orexin-A levels (45). In a clinical observation, Pu et al. found that the orexin promoter showed 61% methylation in 41 patients with depression with cancer and 43% methylation in 7 patients with depression alone, indicating that higher methylation levels were associated with lower orexin levels and depression (46). It is reported that exercise can increase human blood plasma orexin-A levels (47), and Chieffi et al. concluded that exercise also increases hippocampal volume (48), both of which are relevant to the treatment of depression.
3.1.2 Animal studies
Wistar-Kyoto rats (WKY), a genetic model for depression, exhibit increased depressive-like behaviors, that resemble clinical features found in patients with depression. Compared to normal rats, WKY rats show an 18% reduction in the number of orexin-positive neurons and a 15% reduction in the average soma size of orexin-producing cells in the hypothalamus (49). Reduced orexin-A/OX1R mRNA expression and protein content were observed in the lateral hypothalamic region of a rat model of depression (50), and the chronic unpredictable mild stress (CUMS) model of depression reduced OXR2 expression in the ventral hippocampus, thalamus, as well as hypothalamus of rats (51). Basal levels of orexin-A and orexin-B were reduced in the medial prefrontal cortex, voxel nucleus, ventral tegmental area (VTA), and hypothalamus in the social defeat rat model of depression (52). Furthermore, the elimination of OX1R and OX2R by knockdown or pharmacological blockade significantly induced depressive symptoms in experimental mice (53); In contrast, the OX1R receptor antagonist SB334867 effectively suppressed the orexin-A-induced decrease in immobility duration and increase in bromodeoxyuridine (BrdU)-positive cells (a thymidine analog that labels dividing cells in the S-phase of the cell cycle, which accurately reflects cell proliferation) in the hippocampal dentate gyrus (54). These observations provide clear evidence that orexin is a key target for mood regulation, particularly for alleviating depressed mood. Ito et al. found that orexin enhanced hippocampal dentate gyrus BrdU-positive cells in a dose-dependent manner when orexin-A was injected into the ventricles of mice compared to saline controls, thereby inducing an antidepressant-like effect to a certain extent (54). Similarly, orexin-A injection in the VTA brain region of mice showed depression-like behavior significantly ameliorated depressive symptoms and was found to significantly improve depression-like behavior in behavioral tests in mice through optogenetic and chemogenetic activation of orexin terminals in the VTA of CUMS mice (55). This evidence sheds light on the role of orexin in the pathogenesis of depression and suggests that orexin may be a therapeutic target for depression.
3.2 Orexin improved cognitive impairment
In addition to changes in mood, patients with PMDD report impairments in cognitive abilities, including attention, memory, and motor coordination (56). Negative cognitive styles, such as a general negative expectancy for future events and the feeling of having no influence over them, are important factors in the pathogenesis of PMS/PMDD (57). PMDD shares similarities with major depression regarding cognitive vulnerability, and cognitive deficits are well-established in MDD. In a survey of 14 women with PMDD, Reed found that during the luteal phase, women with PMDD exhibited decreased performance on immediate and delayed word recall tasks, immediate and delayed digit recall tasks, and digit symbol replacement tests compared to controls (58). The working memory (WM) system temporally stores and processes information used to guide behavior and includes four subsystems: central execution, visuospatial storage, speech storage, and episode buffering. Yen et al. (59) first demonstrated reduced WM and cognitive control in PMDD patients during premenstrual GO/Nogo tasks, which was relieved during the follicular phase, consistent with abnormal mood and somatic symptoms. Slyepchenko et al. found persistent, subtle WM and selective attention difficulties during the follicular phase of the menstrual cycle in patients with moderate to severe PMS (60). There is also evidence that ovarian hormones are associated with emotional and cognitive processing and affect the cognitive abilities of women with PMDD during the menstrual cycle (61, 62).
Orexin plays an important role in cognitive regulation. It improves cognitive deficits in rats by addressing deficits in neurotransmission (63). Intranasal administration of [Ala11, D-Leu15]-OXB increased c-fos expression (a reliable marker for neural activity) in cortical and basal forebrain areas as well as medial septal cholinergic neurons. Orexin-A also activated a wider range of cortical areas and basal forebrain cholinergic neurons. In addition, intranasal orexin-A significantly reversed the changes produced by sleep deprivation on local brain changes in cerebral metabolic rate for glucose consumption (CMRglc) in rhesus monkeys during delayed match-to-sample task execution more effectively than intravenous injection (64). In orexin/ataxin-3 narcoleptic mice, Yang et al. found that they showed deficits in long-term social memory. Nasal administration of exogenous orexin-A restored social memory to some extent in AT mice, which was associated with enhanced hippocampal synaptic plasticity and cAMP response element binding protein phosphorylation (65). In conclusion, these findings provide strong evidence for the effectiveness of orexin in improving cognitive deficits.
4 Orexin regulated ovarian hormone levels
4.1 Hormonal fluctuations and PMS
During the menstrual cycle, estrogen levels are low during menstruation, gradually rise during ovulation, and then fall gradually during the luteal phase. On the other hand, progesterone levels remain low throughout the follicular phase, experience a rapid increase after ovulation, and then decrease quickly during the late luteal phase (66). These fluctuations in hormone levels may significantly contribute to mood swings experienced by women. Numerous studies have underscored the impact of estrogen and progesterone on depression, anxiety, and other mood disorders in women, implicating abnormal hormone fluctuations as crucial mechanisms in the development of premenstrual depression (67). Different from healthy women, those with PMS exhibit lower estrogen levels and higher progesterone levels. It has been found that the rapid withdrawal of ovarian hormones is likely pivotal in the pathogenesis of PMDD (68). Women with PMDD have demonstrated lower early luteal estrogen levels compared to controls, and covariate analysis demonstrates that the interaction between early luteal phase estrogen and progesterone levels correlates with PMDD severity (69). Both preclinical and human research have illustrated that the cessation of ovarian steroids (estrogen, progesterone, or both) can trigger emotional, cognitive, and behavioral symptoms associated with suicide in susceptible individuals (70). The link between allelic variants in the estrogen receptor alpha gene and PMDD demonstrated by Huo et al. was the first finding revealing genetic alteration in the background of this disorder. ERα’s involvement in the etiopathogenesis and treatment of PMDD, through its regulation of neurotransmitter system signal transduction, underscores its potential significance (71). In addition, Pakharenko et al. have verified that polymorphic variants in the estrogen receptor 1 (ESR1) A-351G gene can serve as markers for the development of PMS (72). Intriguingly, estrogen-mediated signaling also plays a significant role in the activation of neural circuits associated with social cognition and emotion regulation (62). In conclusion, these studies demonstrate the relevance of ovarian hormones to PMS/PMDD. However, it is important to acknowledge that the production of hormones and their actions in the body involve complex molecular mechanisms; consequently, ovarian hormone therapy is not merely about controlling estrogen and progesterone levels, necessitating further comprehensive investigations.
4.2 Orexin regulated steroid hormones
The transcription and translation of prepro-orexin mRNA take place in the hypothalamic region, a crucial locus for the generation of orexin neurons and the primary origin of the hypothalamic-pituitary-gonadal axis (HPG). Consequently, it is plausible that the regulation of behavioral and stress responses in the hypothalamus may be intricately linked to the coordinated interactions among the E2, progesterone, and orexin systems (73). Orexin neurons play an essential role in the regulation of the HPG axis and sexual behavior (74, 75). Research by Cataldi et al. indicates that both orexin-A and orexin-B diminish progesterone secretion in luteal cells, suggesting a modulatory role for the orexin system within the ovary (76). Notably, both in vivo and in vitro investigations have revealed that orexin-A inhibits luteinizing hormone-releasing hormone (LHRH)-stimulated LH release in the dispersed anterior pituitary of proestrus female rats in a dose-dependent manner (75). The hormonal secretion during the rodent estrous cycle appears to correlate with appropriate nutritional status and alertness. It has been demonstrated that orexin governs luteinizing hormone-releasing steroids, nutritional status, and alertness in an interdependent fashion, thus hinting at a possible coordinating function for orexin throughout the estrous cycle (77). Similarly, Kiezun et al. explored alterations in the estrous cycle and early pregnancy in the pig uterus concerning estrogen E1 and E2 emphasizing the orexin system’s role as a “molecular switch” for estrogen activation (78). In conclusion, this body of evidence suggests that orexin may have therapeutic effects in PMS/PMDD through its modulation of ovarian hormones. However, for PMS, there exists inconsistency in the results pertaining to hormone expression levels despite the increasing recognition of the trend toward lower estrogen levels and higher progesterone. Furthermore, the molecular activity and downstream signaling pathways of orexin in the context of intricate hormonal mechanisms remain largely unelucidated, necessitating comprehensive mechanistic studies.
5 Orexin regulated the neuroimmune system to inhibit the inflammatory response
5.1 Inflammatory mechanisms in PMS/PMDD
Emerging trends in neuroendocrinology indicate a connection between inflammatory processes and both mental and somatic disorders sharing common features with PMS/PMDD. Low-level inflammation has been associated with alterations in mood and pain. In a study investigating the correlation between changes in physical and psychological symptoms and inflammatory markers during the menstrual cycle in normal weight and overweight women. Puder et al. discovered that variations in tumor necrosis factor-α (TNF-α) and high-sensitivity C-reactive protein serum concentrations were associated with physical and psychological symptoms experienced during the menstrual cycle (79). Another investigation focusing on the association between inflammatory markers and the severity of menstrual symptoms and PMS among young women revealed that mean levels of interleukin 4, interleukin 10 (IL-10), interleukin 12, and Interferon-gamma were significantly elevated in women meeting PMS criteria compared to healthy women (80). In a comprehensive review by Hantsoo et al. on the epidemiology and treatment of PMS, it was suggested that inflammatory molecules may contribute to the pathobiology of PMDD (81). Furthermore, in a survey involving 21 healthy women with regular menstrual cycles, plasma IL-10 levels were notably higher in women experiencing PMS compared to their healthy counterparts (82). Cumulatively, these findings provide substantial preliminary evidence supporting a potential link between neuroinflammation and the etiology of PMS (83).
5.2 Orexin inhibited inflammatory response to protect neurons
Orexin neurons play an important role in neuroprotection, primarily through the inhibition of inflammatory responses mediated by OX1R and OX2R (84). Experimental findings demonstrated that diminished orexin signaling plays a crucial role in mediating inflammation-induced drowsiness (85). Clark et al. proposed that the activity of orexin neurons is suppressed by the inflammatory cytokine TNF, which has the capacity to degrade orexin precursor mRNA in a time- and dose-dependent manner (86). In a study by Modi et al., utilizing intranasal orexin in a rat model of cardiac arrest (CA), it was revealed that CA led to increased pro-inflammatory markers across all brain regions. Treatment with orexin-A significantly ameliorated CA-induced neuroinflammatory markers in the hypothalamus, increased the production of OX1R and OX2R, exerted anti-inflammatory effects, and expedited cortical EEG and behavioral recovery (87). Additionally, Zhang et al. found that orexin-A effectively dampened endothelial cell inflammation by inhibiting mitogen-activated protein kinases p38 and nuclear factor kappa-B (NF-κB) inflammatory signaling pathways (88). In an investigation into the molecular mechanisms by which orexin-A reduces cerebral ischemia/reperfusion injury, Xu et al. observed that orexin-A confers neuroprotective effects by inhibiting OX1R-mediated NF-κB, mitogen-activated protein kinase (MAPK)/extracellular signal-regulated kinase, and MAPK/p38 signaling pathways, and by suppressing astrocyte apoptosis, activation, and the generation of inflammatory factors such as interleukin 1β (IL-1β), TNF-α, and interleukin 6 (IL-6) (89). Moreover, examining the physiological function of orexin-A in fibroblast-like synoviocytes, Sun et al. found that orexin-A treatment resulted in reduced secretion of IL-1β, IL-6, and interleukin 8, decreased production of reactive oxygen species, and inhibited TNF-α-induced activation of the NF-κB signaling pathway, thus demonstrating an important role in neuroprotection (84). This body of evidence reveals the antagonistic relationship between orexin and inflammatory cytokines, underscoring the potential of orexin’s anti-inflammatory effect as a promising new target for the treatment of PMS/PMDD.
6 Modulation of the GABA system by orexin
6.1 Involvement of the GABA system in the etiology of PMS
Gamma-aminobutyric acid (GABA) serves as the predominant inhibitory neurotransmitter in the human cortex and plays a pivotal role in regulating salience plasticity, sleep, and mood (90–92). Patients diagnosed with PMS often demonstrate alterations in GABA-α receptor sensitivity and GABA concentration, exhibiting reduced plasma GABA levels during the late luteal phase, particularly in cases of premenstrual anxiety (93). Fluctuations in steroid hormone levels can influence the structure and function of the GABAA receptor (GABAAR), impacting the expression levels of GABAARγ-2 and delta subunits, which are closely associated with the pathophysiology of PMS (94, 95). In a study by Zhang et al., analysis of GABA receptor subunit expression patterns in a rat model simulating clinical PMDD symptoms revealed notable differences in the expression of GABAAR α1, α2, α4, α5, β2, β3, and δ subunits among PMDD rat models with distinct characteristics, suggesting their potential as biomarkers for PMDD pathogenesis (96). Furthermore, experimental findings by Iba et al. indicated that the Japanese herb Inochinohaha White increased the b2-subunit of the GABAA receptor in the amygdala, attenuating anxiety-like behavior in a rat model of progesterone withdrawal through augmentation of the GABAA receptor-mediated signaling pathway (97). Similarly, the Chinese medicinal preparation Shuyu capsule, utilized for treating PMS and comprising active ingredients extracted from four Chinese medicines (bupleurum chinense, white paeony root, radix glycyrrhizae, and rhizoma cyperi), reversed the decrease in glutamate to GABA ratio in the right hippocampus of a rat model of premenstrual depression (98). These investigations provide encouraging evidence for the relationship between the GABA system and PMS, warranting further exploration of GABA as an effective therapeutic target for PMS.
6.2 Orexin regulates PMS symptoms through the GABA system
GABA and glutamate neurons are distributed in the reticular core of the brain, where they play a crucial role in regulating cortical activity and behavior during the wake-sleep state. These in turn are modulated by acetylcholine, noradrenaline, dopamine, serotonin, histamine, orexin, and the melanin-concentrating hormone neurons (99). The interplay between orexin and GABA neurotransmission is particularly significant in sleep and mood regulation. Ji et al. found that orexin neurons have direct projections to GABA neurons in the ventral pallidum, enabling them to modulate GABA depolarization via OX1R and OX2R. Knockdown or pharmacological blockade of orexin receptors led to a decrease in peak current in GABA neuron cells. Furthermore, exogenous orexin was found to activate GABA neurons via OX1R and OX2R thereby ameliorating depressive-like behavior in rats (46). Moreover, postsynaptic OX2R activation has been shown to stimulate Na/Ca2 exchange currents in GABA neurons, resulting in heightened membrane depolarization, increased firing rates, and augmented GABA release (100). Similarly, Brevig et al. demonstrated that intracerebral pontine reticular formation injection of orexin-A in rats led to an increase in consolidated wakefulness through the activation of GABAA receptors and OX1R, ultimately promoting arousal (101). Orexin has been found to enhance neuronal plasticity via its modulation of GABA neuron release, exerting selective influence on sleep/wake control within the brain (102), and contributing to the regulation of mood changes. This evidence suggests that the activation of GABA by orexin may represent a potential target for the treatment of PMS/PMDD.
7 Conclusion
In summary, orexin may play a pivotal role in the pathomechanism of PMS, thus showing therapeutic potential to ameliorate symptoms of PMS. Orexin improves sleep disorder, suppresses depression, and improves cognitive deficits, all of which are crucial components of the pathologic expression of PMS/PMDD. Additionally, orexin may also be beneficial in improving hormonal abnormalities, inhibiting neuroinflammation, and modulating GABA neurotransmission, as well as it exerts neuroprotective effects. Therefore, orexin may be a potential target for PMS/PMDD treatment.
The physiological effects of orexin in PMS/PMDD remain unexplored and orexin receptor antagonists have not been used in the clinical treatment of PMS/PMDD. Research on the use of orexin receptor antagonists or agonists in sleep disorders presents promising potential as a therapeutic agent, warranting further investigation and exploration. This review laid the groundwork for future studies examining orexin’s role and application in PMS. Ultimately, orexin holds promise as a therapeutic target for PMS, and the development of related drugs may offer novel treatments for PMS/PMDD in the future, through direct or indirect modulation of the orexin system.
Author contributions
PD: Writing – original draft. WD: Writing – original draft. MS: Writing – original draft. SW: Writing – original draft. YM: Writing – review & editing. TZ: Writing – review & editing. FZ: Writing – review & editing. PS: Writing – review & editing.
Funding
The author(s) declare financial support was received for the research, authorship, and/or publication of this article. This study was supported by the National Natural Science Foundation of China (NSFC, Nos. 81874419, 81673719, 82205290, and 81303074), National Health Commission of the People’s Republic of China (No. 2017ZX09301064 and 2017ZX09301064002), Natural Science Foundation of Shandong Province (Nos. ZR2020MB108 and ZR2019MH063), Central Government Guided Local Science and Technology Development Project (22-1-3-11-zyyd-nsh-3), Shandong Major Innovation Project (2022CXGC020514), Shandong Province Integrated Traditional Chinese and Western Medicine. Depression Prevention and Treatment Project (NO.YXH2019ZXY006) and the Youth Research and Innovation Team of TCM-Based Neuroimmune Pharmacology, University of Shandong Traditional Chinese Medicine (No. 22202112).
Conflict of interest
The authors declare that the research was conducted in the absence of any commercial or financial relationships that could be construed as a potential conflict of interest.
Publisher’s note
All claims expressed in this article are solely those of the authors and do not necessarily represent those of their affiliated organizations, or those of the publisher, the editors and the reviewers. Any product that may be evaluated in this article, or claim that may be made by its manufacturer, is not guaranteed or endorsed by the publisher.
References
1. Hofmeister S, Bodden S. Premenstrual syndrome and premenstrual dysphoric disorder. Am Fam Physician (2016) 94(3):236–40.
2. American College of Obstetricians and Gynecologists. Guidelines for Women’s Health Care: A Resource Manual. 4th ed. Washington, DC: American College of Obstetricians and Gynecologists (2014) p. 607–13.
3. Halbreich U, Borenstein J, Pearlstein T, Kahn LS. The prevalence, impairment, impact, and burden of premenstrual dysphoric disorder (PMS/PMDD). Psychoneuroendocrinology (2003) 28 Suppl 3:1–23. doi: 10.1016/s0306-4530(03)00098-2
4. Mishra S, Elliott H, Marwaha R. Premenstrual Dysphoric Disorder. In: StatPearls. Treasure Island (FL: StatPearls Publishing (2023).
5. Heinemann LA, Minh TD, Heinemann K, Lindemann M, Filonenko A. Intercountry assessment of the impact of severe premenstrual disorders on work and daily activities. Health Care Women Int (2012) 33(2):109–24. doi: 10.1080/07399332.2011.610530
6. Tiranini L, Nappi RE. Recent advances in understanding/management of premenstrual dysphoric disorder/premenstrual syndrome. Fac Rev (2022) 11:11. doi: 10.12703/r/11-11
7. Muraki Y, Yamanaka A, Tsujino N, Kilduff TS, Goto K, Sakurai T. Serotonergic regulation of the orexin/hypocretin neurons through the 5-HT1A receptor. J Neurosci (2004) 24(32):7159–66. doi: 10.1523/JNEUROSCI.1027-04.2004
8. Winsky-Sommerer R, Yamanaka A, Diano S, Borok E, Roberts AJ, Sakurai T, et al. Interaction between the corticotropin-releasing factor system and hypocretins (orexins): a novel circuit mediating stress response. J Neurosci (2004) 24(50):11439–48. doi: 10.1523/JNEUROSCI.3459-04.2004
9. Gold EB, Wells C, Rasor MO. The Association of Inflammation with Premenstrual symptoms. J Womens Health (Larchmt) (2016) 25(9):865–74. doi: 10.1089/jwh.2015.5529
10. Sakurai T, Amemiya A, Ishii M, Matsuzaki I, Chemelli RM, Tanaka H, et al. Orexins and orexin receptors: a family of hypothalamic neuropeptides and G protein-coupled receptors that regulate feeding behavior. Cell (1998) 92(4):573–85. doi: 10.1016/s0092-8674(00)80949-6
11. de Lecea L, Kilduff TS, Peyron C, Gao X, Foye PE, Danielson PE, et al. The hypocretins: hypothalamus-specific peptides with neuroexcitatory activity. Proc Natl Acad Sci U S A (1998) 95(1):322–7. doi: 10.1073/pnas.95.1.322
12. Jacobson LH, Hoyer D, de Lecea L. Hypocretins (orexins): The ultimate translational neuropeptides. J Intern Med (2022) 291(5):533–56. doi: 10.1111/joim.13406
13. Tsunematsu T, Yamanaka A. The role of orexin/hypocretin in the central nervous system and peripheral tissues. Vitam Horm (2012) 89:19–33. doi: 10.1016/B978-0-12-394623-2.00002-0
14. Pizza F, Magnani M, Indrio C, Plazzi G. The hypocretin system and psychiatric disorders. Curr Psychiatry Rep (2014) 16(2):433. doi: 10.1007/s11920-013-0433-9
15. Li J, Hu Z, de Lecea L. The hypocretins/orexins: integrators of multiple physiological functions. Br J Pharmacol (2014) 171(2):332–50. doi: 10.1111/bph.12415
16. Tsujino N, Sakurai T. Orexin/hypocretin: a neuropeptide at the interface of sleep, energy homeostasis, and reward system. Pharmacol Rev (2009) 61(2):162–76. doi: 10.1124/pr.109.001321
17. Baker FC, Sassoon SA, Kahan T, Palaniappan L, Nicholas CL, Trinder J, et al. Perceived poor sleep quality in the absence of polysomnographic sleep disturbance in women with severe premenstrual syndrome. J Sleep Res (2012) 21(5):535–45. doi: 10.1111/j.1365-2869.2012.01007.x
18. Gupta R, Lahan V, Bansal S. Subjective sleep problems in young women suffering from premenstrual dysphoric disorder. N Am J Med Sci (2012) 4(11):593–5. doi: 10.4103/1947-2714.103326
19. Parry BL, Mostofi N, LeVeau B, Nahum HC, Golshan S, Laughlin GA, et al. Sleep EEG studies during early and late partial sleep deprivation in premenstrual dysphoric disorder and normal control subjects. Psychiatry Res (1999) 85(2):127–43. doi: 10.1016/s0165-1781(98)00128-0
20. Mauri M, Reid RL, MacLean AW. Sleep in the premenstrual phase: a self-report study of PMS patients and normal controls. Acta Psychiatr Scand (1988) 78(1):82–6. doi: 10.1111/j.1600-0447.1988.tb06304.x
21. Parry BL, Javeed S, Laughlin GA, Hauger R, Clopton P. Cortisol circadian rhythms during the menstrual cycle and with sleep deprivation in premenstrual dysphoric disorder and normal control subjects. Biol Psychiatry (2000) 48(9):920–31. doi: 10.1016/s0006-3223(00)00876-3
22. Lin PC, Ko CH, Lin YJ, Yen JY. Insomnia, inattention and fatigue symptoms of women with premenstrual dysphoric disorder. Int J Environ Res Public Health (2021) 18(12):6192. doi: 10.3390/ijerph18126192
23. Conzatti M, Perez AV, Maciel RF, De Castro DH, Sbaraini M, Wender MCO. Sleep quality and excessive daytime sleepiness in women with Premenstrual Syndrome. Gynecol Endocrinol (2021) 37(10):945–9. doi: 10.1080/09513590.2021.1968820
24. Nicolau ZFM, Bezerra AG, Polesel DN, Andersen ML, Bittencourt L, Tufik S, et al. Premenstrual syndrome and sleep disturbances: Results from the Sao Paulo Epidemiologic Sleep Study. Psychiatry Res (2018) 264:427–31. doi: 10.1016/j.psychres.2018.04.008
25. Baker FC, Kahan TL, Trinder J, Colrain IM. Sleep quality and the sleep electroencephalogram in women with severe premenstrual syndrome. Sleep (2007) 30(10):1283–91. doi: 10.1093/sleep/30.10.1283
26. Shinohara K, Uchiyama M, Okawa M, Saito K, Kawaguchi M, Funabashi T, et al. Menstrual changes in sleep, rectal temperature and melatonin rhythms in a subject with premenstrual syndrome. Neurosci Lett (2000) 281(2-3):159–62. doi: 10.1016/s0304-3940(00)00826-0
27. Lee MG, Hassani OK, Jones BE. Discharge of identified orexin/hypocretin neurons across the sleep-waking cycle. J Neurosci (2005) 25(28):6716–20. doi: 10.1523/JNEUROSCI.1887-05.2005
28. Barateau L, Lopez R, Chenini S, Rassu AL, Scholz S, Lotierzo M, et al. Association of CSF orexin-A levels and nocturnal sleep stability in patients with hypersomnolence. Neurology (2020) 95(21):e2900–11. doi: 10.1212/WNL.0000000000010743
29. Baumann CR, Khatami R, Werth E, Bassetti CL. Hypocretin (orexin) deficiency predicts severe objective excessive daytime sleepiness in narcolepsy with cataplexy. J Neurol Neurosurg Psychiatry (2006) 77(3):402–4. doi: 10.1136/jnnp.2005.067207
30. Knudsen S, Gammeltoft S, Jennum PJ. Rapid eye movement sleep behaviour disorder in patients with narcolepsy is associated with hypocretin-1 deficiency. Brain (2010) 133(Pt 2):568–79. doi: 10.1093/brain/awp320
31. Evans R, Kimura H, Alexander R, Davies CH, Faessel H, Hartman DS, et al. Orexin 2 receptor-selective agonist danavorexton improves narcolepsy phenotype in a mouse model and in human patients. Proc Natl Acad Sci U S A. (2022) 119(35):e2207531119. doi: 10.1073/pnas.2207531119
32. Herring WJ, Connor KM, Ivgy-May N, Snyder E, Liu K, Snavely DB, et al. Suvorexant in patients with insomnia: results from two 3-month randomized controlled clinical trials. Biol Psychiatry (2016) 79(2):136–48. doi: 10.1016/j.biopsych.2014.10.003
33. Hung CJ, Ono D, Kilduff TS, Yamanaka A. Dual orexin and MCH neuron-ablated mice display severe sleep attacks and cataplexy. Elife (2020) 9:e54275. doi: 10.7554/eLife.54275
34. Murillo-Rodriguez E, Liu M, Blanco-Centurion C, Shiromani PJ. Effects of hypocretin (orexin) neuronal loss on sleep and extracellular adenosine levels in the rat basal forebrain. Eur J Neurosci (2008) 28(6):1191–8. doi: 10.1111/j.1460-9568.2008.06424.x
35. Mieda M, Willie JT, Hara J, Sinton CM, Sakurai T, Yanagisawa M. Orexin peptides prevent cataplexy and improve wakefulness in an orexin neuron-ablated model of narcolepsy in mice. Proc Natl Acad Sci U S A (2004) 101(13):4649–54. doi: 10.1073/pnas.0400590101
36. Kaushik MK, Aritake K, Cherasse Y, Imanishi A, Kanbayashi T, Urade Y, et al. Induction of narcolepsy-like symptoms by orexin receptor antagonists in mice. Sleep (2021) 44(8):zsab043. doi: 10.1093/sleep/zsab043
37. American Psychiatric Association. Diagnostic and statistical manual of mental disorders (DSM-5) . Available at: https://www.psychiatry.org/psychiatrists/practice/dsm (Accessed October 1, 2022).
38. Marjoribanks J, Brown J, O’Brien PM, Wyatt K. Selective serotonin reuptake inhibitors for premenstrual syndrome. Cochrane. Database Syst Rev (2013) 2013(6):CD001396. doi: 10.1002/14651858.CD001396.pub3
39. Nollet M, Leman S. Role of orexin in the pathophysiology of depression: potential for pharmacological intervention. CNS Drugs (2013) 27(6):411–22. doi: 10.1007/s40263-013-0064-z
40. Khairuddin S, Aquili L, Heng BC, Hoo TLC, Wong KH, Lim LW. Dysregulation of the orexinergic system: A potential neuropeptide target in depression. Neurosci Biobehav Rev (2020) 118:384–96. doi: 10.1016/j.neubiorev.2020.07.040
41. Lu J, Zhao J, Balesar R, Fronczek R, Zhu QB, Wu XY, et al. Sexually dimorphic changes of hypocretin (Orexin) in depression. EBioMedicine (2017) 18:311–9. doi: 10.1016/j.ebiom.2017.03.043
42. Gotter AL, Roecker AJ, Hargreaves R, Coleman PJ, Winrow CJ, Renger JJ. Orexin receptors as therapeutic drug targets. Prog Brain Res (2012) 198:163–88. doi: 10.1016/B978-0-444-59489-1.00010-0
43. Xu TR, Yang Y, Ward R, Gao L, Liu Y. Orexin receptors: multi-functional therapeutic targets for sleeping disorders, eating disorders, drug addiction, cancers and other physiological disorders. Cell Signal (2013) 25(12):2413–23. doi: 10.1016/j.cellsig.2013.07.025
44. Rotter A, Asemann R, Decker A, Kornhuber J, Biermann T. Orexin expression and promoter-methylation in peripheral blood of patients suffering from major depressive disorder. J Affect Disord (2011) 131(1-3):186–92. doi: 10.1016/j.jad.2010.12.004
45. Brundin L, Björkqvist M, Petersén A, Träskman-Bendz L. Reduced orexin levels in the cerebrospinal fluid of suicidal patients with major depressive disorder. Eur Neuropsychopharmacol (2007) 17(9):573–9. doi: 10.1016/j.euroneuro.2007.01.005
46. Pu C, Tian S, He S, Chen W, He Y, Ren H, et al. Depression and stress levels increase risk of liver cancer through epigenetic downregulation of hypocretin. Genes Dis (2020) 9(4):1024–37. doi: 10.1016/j.gendis.2020.11.013
47. Messina G, Di Bernardo G, Viggiano A, De Luca V, Monda V, Messina A, et al. Exercise increases the level of plasma orexin A in humans. J Basic Clin Physiol Pharmacol (2016) 27(6):611–6. doi: 10.1515/jbcpp-2015-0133
48. Chieffi S, Messina G, Villano I, Messina A, Esposito M, Monda V, et al. Exercise influence on hippocampal function: possible involvement of orexin-A. Front Physiol (2017) 8:85. doi: 10.3389/fphys.2017.00085
49. Allard JS, Tizabi Y, Shaffery JP, Trouth CO, Manaye K. Stereological analysis of the hypothalamic hypocretin/orexin neurons in an animal model of depression. Neuropeptides (2004) 38(5):311–5. doi: 10.1016/j.npep.2004.06.004
50. Hou Y, Liu Y, Liu C, Yan Z, Ma Q, Chen J, et al. Xiaoyaosan regulates depression-related behaviors with physical symptoms by modulating Orexin A/OxR1 in the hypothalamus. Anat Rec (Hoboken). (2020) 303(8):2144–53. doi: 10.1002/ar.24386
51. Nollet M, Gaillard P, Minier F, Tanti A, Belzung C, Leman S. Activation of orexin neurons in dorsomedial/perifornical hypothalamus and antidepressant reversal in a rodent model of depression. Neuropharmacology (2011) 61(1-2):336–46. doi: 10.1016/j.neuropharm.2011.04.022
52. Nocjar C, Zhang J, Feng P, Panksepp J. The social defeat animal model of depression shows diminished levels of orexin in mesocortical regions of the dopamine system, and of dynorphin and orexin in the hypothalamus. Neuroscience (2012) 218:138–53. doi: 10.1016/j.neuroscience.2012.05.033
53. Ji MJ, Zhang XY, Chen Z, Wang JJ, Zhu JN. Orexin prevents depressive-like behavior by promoting stress resilience. Mol Psychiatry (2019) 24(2):282–93. doi: 10.1038/s41380-018-0127-0
54. Ito N, Yabe T, Gamo Y, Nagai T, Oikawa T, Yamada H, et al. I.c.v. administration of orexin-A induces an antidepressive-like effect through hippocampal cell proliferation. Neuroscience (2008) 157(4):720–32. doi: 10.1016/j.neuroscience.2008.09.042
55. Song Y, Li J, Li H, Cai M, Miao D. The role of ventral tegmental area orexinergic afferents in depressive-like behavior in a chronic unpredictable mild stress (CUMS) mouse model. Biochem Biophys Res Commun (2021) 579:22–8. doi: 10.1016/j.bbrc.2021.09.062
56. Lin PC, Ko CH, Yen JY. Early and late luteal executive function, cognitive and somatic symptoms, and emotional regulation of women with premenstrual dysphoric disorder. J Pers Med (2022) 12(5):819. doi: 10.3390/jpm12050819
57. Śliwerski A, Bielawska-Batorowicz E. Negative cognitive styles as risk factors for the occurrence of PMS and PMDD. J Reprod Infant Psychol (2019) 37(3):322–37. doi: 10.1080/02646838.2018.1543943
58. Reed SC, Levin FR, Evans SM. Changes in mood, cognitive performance and appetite in the late luteal and follicular phases of the menstrual cycle in women with and without PMDD (premenstrual dysphoric disorder). Horm Behav (2008) 54(1):185–93. doi: 10.1016/j.yhbeh.2008.02.018
59. Yen JY, Tu HP, Chen CS, Yen CF, Long CY, Ko CH. The effect of serotonin 1A receptor polymorphism on the cognitive function of premenstrual dysphoric disorder [published correction appears in Eur Arch Psychiatry Clin Neurosci. Eur Arch Psychiatry Clin Neurosci (2014) 264(8):729–39. doi: 10.1007/s00406-013-0466-4
60. Slyepchenko A, Lokuge S, Nicholls B, Steiner M, Hall GB, Soares CN, et al. Subtle persistent working memory and selective attention deficits in women with premenstrual syndrome. Psychiatry Res (2017) 249:354–62. doi: 10.1016/j.psychres.2017.01.031
61. Thompson K, Sergejew A, Kulkarni J. Estrogen affects cognition in women with psychosis. Psychiatry Res (2000) 94(3):201–9. doi: 10.1016/s0165-1781(00)00161-x
62. Toffoletto S, Lanzenberger R, Gingnell M, Sundström-Poromaa I, Comasco E. Emotional and cognitive functional imaging of estrogen and progesterone effects in the female human brain: a systematic review. Psychoneuroendocrinology (2014) 50:28–52. doi: 10.1016/j.psyneuen.2014.07.025
63. Calva CB, Fadel JR. Intranasal administration of orexin peptides: Mechanisms and therapeutic potential for age-related cognitive dysfunction. Brain Res (2020) 1731:145921. doi: 10.1016/j.brainres.2018.08.024
64. Deadwyler SA, Porrino L, Siegel JM, Hampson RE. Systemic and nasal delivery of orexin-A (Hypocretin-1) reduces the effects of sleep deprivation on cognitive performance in nonhuman primates. J Neurosci (2007) 27(52):14239–47. doi: 10.1523/JNEUROSCI.3878-07.2007
65. Yang L, Zou B, Xiong X, Pascual C, Xie J, Malik A, et al. Hypocretin/orexin neurons contribute to hippocampus-dependent social memory and synaptic plasticity in mice. J Neurosci (2013) 33(12):5275–84. doi: 10.1523/JNEUROSCI.3200-12.2013
66. Mihm M, Gangooly S, Muttukrishna S. The normal menstrual cycle in women. Anim Reprod Sci (2011) 124(3-4):229–36. doi: 10.1016/j.anireprosci.2010.08.030
68. Smith SS, Ruderman Y, Frye C, Homanics G, Yuan M. Steroid withdrawal in the mouse results in anxiogenic effects of 3alpha,5beta-THP: a possible model of premenstrual dysphoric disorder. Psychopharmacol (Berl) (2006) 186(3):323–33. doi: 10.1007/s00213-005-0168-3
69. Yen JY, Lin HC, Lin PC, Liu TL, Long CY, Ko CH. Early- and late-luteal-phase estrogen and progesterone levels of women with premenstrual dysphoric disorder. Int J Environ Res Public Health (2019) 16(22):4352. doi: 10.3390/ijerph16224352
70. Eisenlohr-Moul TA, Bowers SM, Prinstein MJ, Schmalenberger KM, Walsh EC, Young SL, et al. Effects of acute estradiol and progesterone on perimenstrual exacerbation of suicidal ideation and related symptoms: a crossover randomized controlled trial. Transl Psychiatry (2022) 12(1):528. doi: 10.1038/s41398-022-02294-1
71. Huo L, Straub RE, Roca C, Schmidt PJ, Shi K, Vakkalanka R, et al. Risk for premenstrual dysphoric disorder is associated with genetic variation in ESR1, the estrogen receptor alpha gene. Biol Psychiatry (2007) 62(8):925–33. doi: 10.1016/j.biopsych.2006.12.019
72. Pakharenko L. Effect of estrogen receptor gene ESR1 polymorphism on development of premenstrual syndrome. Georgian Med News (2014) 235):37–41.
73. Kim HJJ, Dickie SA, Laprairie RB. Estradiol-dependent hypocretinergic/orexinergic behaviors throughout the estrous cycle. Psychopharmacol (Berl). (2023) 240(1):15–25. doi: 10.1007/s00213-022-06296-1
74. Silveyra P, Cataldi NI, Lux-Lantos VA, Libertun C. Role of orexins in the hypothalamic-pituitary-ovarian relationships. Acta Physiol (Oxf) (2010) 198(3):355–60. doi: 10.1111/j.1748-1716.2009.02049.x
75. Russell SH, Small CJ, Kennedy AR, Stanley SA, Seth A, Murphy KG, et al. Orexin A interactions in the hypothalamo-pituitary gonadal axis. Endocrinology (2001) 142(12):5294–302. doi: 10.1210/endo.142.12.8558
76. Cataldi NI, Lux-Lantos VA, Libertun C. Effects of orexins A and B on expression of orexin receptors and progesterone release in luteal and granulosa ovarian cells. Regul Pept (2012) 178(1-3):56–63. doi: 10.1016/j.regpep.2012.06.008
77. Porkka-Heiskanen T, Kalinchuk A, Alanko L, Huhtaniemi I, Stenberg D. Orexin A and B levels in the hypothalamus of female rats: the effects of the estrous cycle and age. Eur J Endocrinol (2004) 150(5):737–42. doi: 10.1530/eje.0.1500737
78. Kiezun M, Dobrzyn K, Rytelewska E, Kisielewska K, Gudelska M, Szeszko K, et al. The effect of estrone and estradiol on the expression of the orexin/hypocretin system in the porcine uterus during early pregnancy. Domest Anim Endocrinol (2019) 68:11–24. doi: 10.1016/j.domaniend.2018.12.008
79. Puder JJ, Blum CA, Mueller B, De Geyter Ch, Dye L, Keller U. Menstrual cycle symptoms are associated with changes in low-grade inflammation. Eur J Clin Invest (2006) 36(1):58–64. doi: 10.1111/j.1365-2362.2006.01591.x
80. Bertone-Johnson ER, Ronnenberg AG, Houghton SC, Nobles C, Zagarins SE, Takashima-Uebelhoer BB, et al. Association of inflammation markers with menstrual symptom severity and premenstrual syndrome in young women. Hum Reprod (2014) 29(9):1987–94. doi: 10.1093/humrep/deu170
81. Hantsoo L, Epperson CN. Premenstrual dysphoric disorder: epidemiology and treatment. Curr Psychiatry Rep (2015) 17(11):87. doi: 10.1007/s11920-015-0628-3
82. Yama K, Asari Y, Ono A, Machida M, Miura J. Plasma interleukin-10 levels are altered in women with severe premenstrual syndrome: A preliminary study. Womens Health Rep (New Rochelle) (2020) 1(1):73–9. doi: 10.1089/whr.2019.0010
83. Bannister E. There is increasing evidence to suggest that brain inflammation could play a key role in the aetiology of psychiatric illness. Could inflammation be a cause of the premenstrual syndromes PMS and PMDD? Post Reprod Health (2019) 25(3):157–61. doi: 10.1177/2053369119875386
84. Sun M, Wang W, Li Q, Yuan T, Weng W. Orexin A may suppress inflammatory response in fibroblast-like synoviocytes. BioMed Pharmacother (2018) 107:763–8. doi: 10.1016/j.biopha.2018.07.159
85. Grossberg AJ, Zhu X, Leinninger GM, Levasseur PR, Braun TP, Myers MG, et al. Inflammation-induced lethargy is mediated by suppression of orexin neuron activity. J Neurosci (2011) 31(31):11376–86. doi: 10.1523/JNEUROSCI.2311-11.2011
86. Clark IA, Vissel B. Inflammation-sleep interface in brain disease: TNF, insulin, orexin. J Neuroinflammation (2014) 11:51. doi: 10.1186/1742-2094-11-51
87. Modi HR, Wang Q, Gd S, Sherman D, Greenwald E, Savonenko AV, et al. Intranasal post-cardiac arrest treatment with orexin-A facilitates arousal from coma and ameliorates neuroinflammation. PloS One (2017) 12(9):e0182707. doi: 10.1371/journal.pone.0182707
88. Zhang H, Liang B, Li T, Zhou Y, Shang D, Du Z. Orexin A Suppresses Oxidized LDL Induced Endothelial Cell Inflammation via MAPK p38 and NF-κB Signaling Pathway. IUBMB Life (2018) 70(10):961–8. doi: 10.1002/iub.1890
89. Xu D, Kong T, Shao Z, Liu M, Zhang R, Zhang S, et al. Orexin-A alleviates astrocytic apoptosis and inflammation via inhibiting OX1R-mediated NF-κB and MAPK signaling pathways in cerebral ischemia/reperfusion injury. Biochim Biophys Acta Mol Basis Dis (2021) 1867(11):166230. doi: 10.1016/j.bbadis.2021.166230
90. Tritsch NX, Granger AJ, Sabatini BL. Mechanisms and functions of GABA co-release. Nat Rev Neurosci (2016) 17(3):139–45. doi: 10.1038/nrn.2015.21
91. Taylor M, Bhagwagar Z, Cowen PJ, Sharp T. GABA and mood disorders. Psychol Med (2003) 33(3):387–93. doi: 10.1017/s0033291702006876
92. Heaney CF, Kinney JW. Role of GABA(B) receptors in learning and memory and neurological disorders. Neurosci Biobehav Rev (2016) 63:1–28. doi: 10.1016/j.neubiorev.2016.01.007
93. Halbreich U, Petty F, Yonkers K, Kramer GL, Rush AJ, Bibi KW. Low plasma gamma-aminobutyric acid levels during the late luteal phase of women with premenstrual dysphoric disorder. Am J Psychiatry (1996) 153(5):718–20. doi: 10.1176/ajp.153.5.718
94. Maguire J, Mody I. Steroid hormone fluctuations and GABA(A)R plasticity. Psychoneuroendocrinology (2009) 34 Suppl 1(Suppl 1):S84–90. doi: 10.1016/j.psyneuen.2009.06.019
95. MacKenzie G, Maguire J. The role of ovarian hormone-derived neurosteroids on the regulation of GABAA receptors in affective disorders. Psychopharmacol (Berl) (2014) 231(17):3333–42. doi: 10.1007/s00213-013-3423-z
96. Zhang H, Gao Z, Sun Y, Lu T, Wang Z, Gao D, et al. Profiling GABA(A) receptor subunit expression in the hippocampus of PMDD rat models based on TCM theories. Mol Neurobiol (2023) 60(8):4418–28. doi: 10.1007/s12035-023-03354-3
97. Iba H, Watanabe T, Motomura S, Harada K, Uesugi H, Shibahara T, et al. A Japanese herbal medicine attenuates anxiety-like behavior through GABAA receptor and brain-derived neurotrophic factor expression in a rat model of premenstrual syndrome. J Pharmacol Sci (2021) 145(1):140–9. doi: 10.1016/j.jphs.2020.11.003
98. Gao X, Sun P, Qiao M, Wei S, Xue L, Zhang H. Shu−Yu capsule, a Traditional Chinese Medicine formulation, attenuates premenstrual syndrome depression induced by chronic stress constraint. Mol Med Rep (2014) 10(6):2942–8. doi: 10.3892/mmr.2014.2599
99. Jones BE. Arousal and sleep circuits. Neuropsychopharmacology (2020) 45(1):6–20. doi: 10.1038/s41386-019-0444-2
100. Burdakov D, Liss B, Ashcroft FM. Orexin excites GABAergic neurons of the arcuate nucleus by activating the sodium–calcium exchanger. J Neurosci (2003) 23(12):4951–7. doi: 10.1523/JNEUROSCI.23-12-04951.2003
101. Brevig HN, Watson CJ, Lydic R, Baghdoyan HA. Hypocretin and GABA interact in the pontine reticular formation to increase wakefulness. Sleep (2010) 33(10):1285–93. doi: 10.1093/sleep/33.10.1285
Keywords: premenstrual syndrome, orexin, symptoms, mechanism, new target
Citation: Dong P, Dai W, Su M, Wang S, Ma Y, Zhao T, Zheng F and Sun P (2024) The potential role of the orexin system in premenstrual syndrome. Front. Endocrinol. 14:1266806. doi: 10.3389/fendo.2023.1266806
Received: 28 July 2023; Accepted: 29 December 2023;
Published: 16 January 2024.
Edited by:
Nils Lambrecht, United States Department of Veterans Affairs, United StatesReviewed by:
Szilvia Vas, University of Cambridge, United KingdomRichard Leandro Spinieli, Beth Israel Lahey Health, United States
Copyright © 2024 Dong, Dai, Su, Wang, Ma, Zhao, Zheng and Sun. This is an open-access article distributed under the terms of the Creative Commons Attribution License (CC BY). The use, distribution or reproduction in other forums is permitted, provided the original author(s) and the copyright owner(s) are credited and that the original publication in this journal is cited, in accordance with accepted academic practice. No use, distribution or reproduction is permitted which does not comply with these terms.
*Correspondence: Peng Sun, c3VucGVuZ0BzZHV0Y20uZWR1LmNu; Yuexiang Ma, bXl4MTAwOEAxMjYuY29t; Tingting Zhao, dHR6MTAxMUAxMjYuY29t; Feng Zheng, ZHIuZmVuZ3poZW5nQGdtYWlsLmNvbQ==
†Lead contact, further information and requests for resources and reagents should bedirected to and will be fulfilled by Peng Sun,c3VucGVuZ0BzZHV0Y20uZWR1LmNu