- Department of Anesthesiology, Shanghai Ninth People’s Hospital, Shanghai Jiao Tong University School of Medicine, Shanghai, China
Objectives: Multiple research projects have provided evidence of the correlation between obesity and cognitive impairment. WWI, a novel metric for assessing obesity, has the potential to provide a more precise assessment of muscle and fat mass. This research aimed to investigate the association between WWI and cognitive functioning among elderly individuals residing in the United States.
Methods: This study utilized data from the National Health and Nutrition Examination Survey (NHANES) conducted between 2011 and 2014. Weighted multiple linear regression models, smoothed fitted curves, and generalized weighted models were employed to examine the associations between WWI and cognitive function in linear and nonlinear contexts.
Results: The study included a cohort of 2,764 adult volunteers aged 60 years and older, all with complete data. Upon controlling for all potential confounding variables, our analysis revealed statistically significant negative associations between WWI and the Digit Symbol Substitution Test (DSST) score. Specifically, for each 1-unit increase in WWI, there was a corresponding loss of 3.57 points in the DSST score [-3.57 (-4.31, -2.82)]. The negative correlations between WWI with CERAD total word recall [-0.63 (-0.85, -0.40)], CERAD delayed recall [-0.19 (-0.30, -0.07)], and AFT [-0.65 (-0.94, -0.37)] were significant only in partially adjusted models.
Conclusion: Higher WWI was associated with poorer cognitive function.
1 Background
Cognition refers to the cognitive processes through which the human brain receives, analyses, and internally transforms external information, facilitating knowledge acquisition and application. The cognitive abilities encompassed within this framework consist of memory, language, executive, visuospatial, comprehension judgment and computational (1). Cognitive impairment is the deterioration of one or more cognitive functions, resulting in adverse effects on an individual’s everyday functioning and social abilities. Cognitive impairment is prevalent among the elderly population, as evidenced by a study conducted in the United States, which revealed that approximately 33% of individuals aged 65 and above exhibit symptoms of dementia or mild cognitive impairment (MCI) (2).
Obesity, a burgeoning global problem, not only heightens the risk of cardiovascular disease but also exerts a detrimental influence on the central nervous system and cognitive function (3). A substantial body of data indicates a connection between obesity and moderate cognitive impairment, as well as notable changes in the structure and function of the hippocampus. Moreover, a robust correlation has been established between obesity and the specific dementia subtype known as Alzheimer’s disease (4–6). There was a correlation between central adiposity and suboptimal performance on assessments that evaluate executive functioning and visuomotor skills (7). Typically, the evaluation of obesity involves the utilization of body mass index (BMI) and waist circumference (WC). However, it is worth noting that these measurements do not allow for the differentiation between muscle mass and adipose tissue.
The weight-adjusted waist index (WWI) is a novel measure of obesity proposed by Park et al. This index incorporates body composition variations, including muscle and fat mass, providing a more comprehensive assessment of centripetal obesity (8).
There is existing evidence demonstrating a correlation between obesity and cognitive impairment. However, the relationship between WWI and cognitive function remains unexplored in the literature. Hence, this research aimed to investigate the correlation between WWI and cognitive abilities among the elderly population in the United States, utilizing data obtained from the National Health and Nutrition Examination Survey (NHANES).
2 Methods
2.1 Study population
The National Health and Nutrition Examination Study (NHANES) is a continuous and comprehensive countrywide study that seeks to assess the nutritional and health conditions of the population in the United States (9). The Research Ethics Review Board of the National Center for Health Statistics (NCHS) authorized the study procedure. During the recruitment process, all participants were granted written consent. Written consent was obtained from all participants recruited from the NHANES 2011-2014 at the time of their recruitment. Our analysis included participants with thorough information on cognitive functioning assessments and WWI. Initially, a cohort of 19,931 individuals was enrolled. However, after the removal of participants with missing data on weight (n 965), waist circumference (n 2,120), and cognition function (n 1,4082), our final analysis contained a total of 2,764 eligible participants (Figure 1).
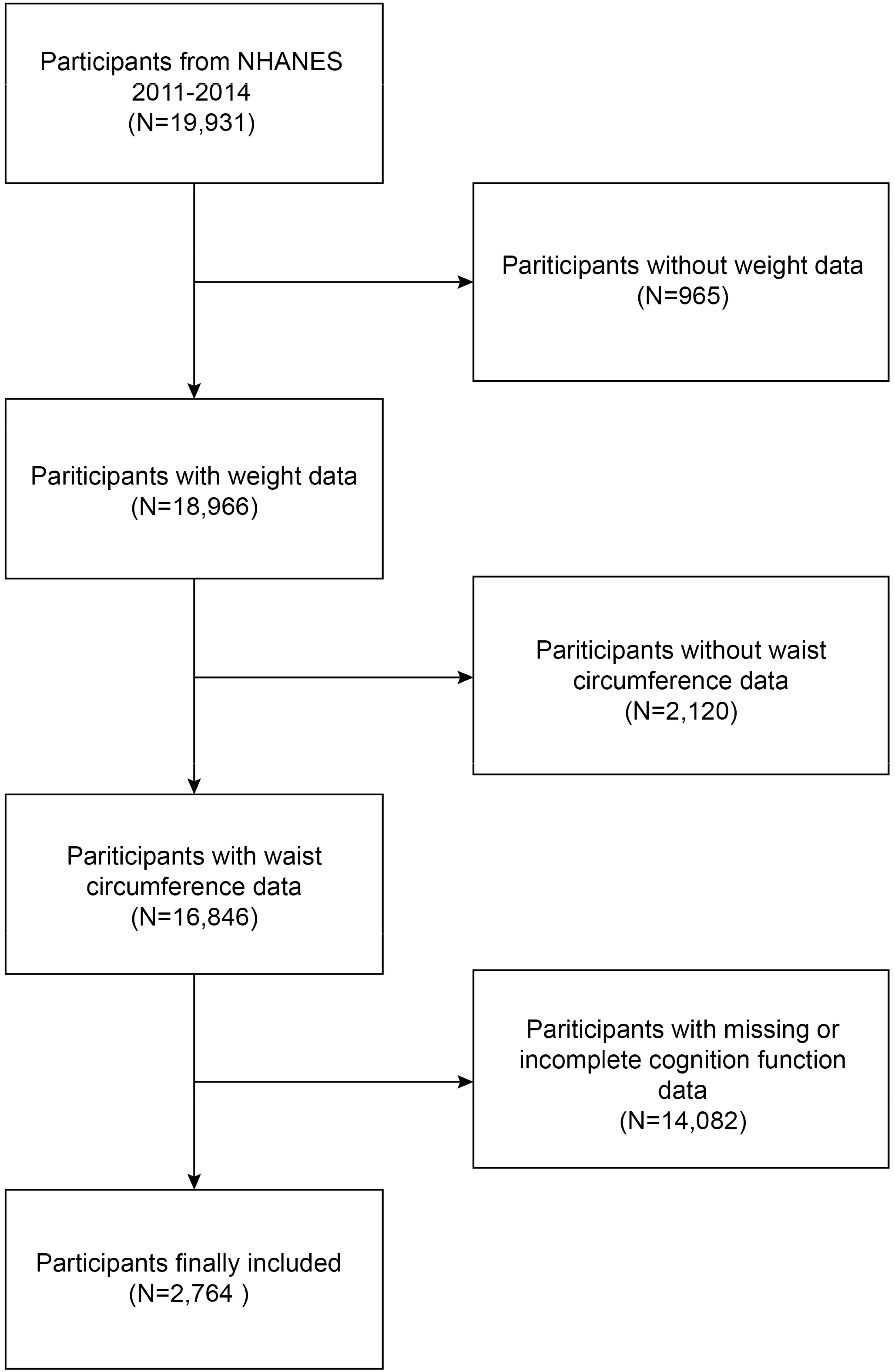
Figure 1 Flow chart of participants selection. NHANES, National Health and Nutrition Examination Survey.
2.2 Weight-adjusted-waist index
With a focus on estimating obesity, the WWI is an anthropometric index based on weight and waist circumference. A more excellent WWI score was indicative of a heightened level of obesity. Health technicians who had received specialized training took data on waist circumference (WC) and weight within the Mobile Examination Center (MEC). The WWI index for each participant was calculated by separating the waist circumference (WC) in centimeters by the square root of the weight in kilograms and afterward rounding the resulting value to two decimal points (8). To facilitate our research, WWI was considered a continuous variable, and individuals were classified based on the tertiles of WWI. WWI was intended to serve as an exposure variable in our research.
2.3 Measurement of cognitive function
The Consortium to Establish a Registry for Alzheimer’s Disease (CERAD W-L) evaluates the capacity of individuals to acquire and retain new verbal material in terms of immediate and delayed learning (10, 11). The CERAD W-L protocol comprises three successive learning trials and a delayed recall task. During the three learning trials, participants were provided with instructions to orally articulate a set of 10 words that were not related to each other. Participants tried to recall as many words as they could immediately after the terms were presented. The delayed recall was conducted roughly 10 minutes following the commencement of the word learning trials. Each trial is assigned a maximum score of 10, and the final score for the word list is 40, representing the combined sum of the three tests and the delayed recall.
The Animal Fluency Test assesses verbal category fluency, a fundamental aspect of executive function. Additionally, this test evaluates other abilities related to semantic memory and processing speed (12). With a score awarded for each animal named, participants were instructed to list as many creatures as possible in a minute.
The Digit Symbol Substitution Test (DSST) is a comprehensive assessment tool for evaluating brain health, encompassing various cognitive domains such as visual scanning, processing speed, short-term memory, and sustained attention (13, 14). A paper form is used for the test, and the top of the state has a key with nine numbers and different symbols paired with them. The participants were given a time limit of two minutes to replicate the characters that were associated with the 133 boxes adjacent to the numbers.
2.4 Covariables
The covariates considered in the study encompassed age (measured in years), gender (categorized as male or female), race (including Mexican American, Other Hispanic, Non-Hispanic White, Non-Hispanic Black, and Other races), education level (classified as below high school, high school, or above high school), income-to-poverty ratio (PIR), smoking status (indicated as yes or no), diabetes status (shown as yes or no), stroke status (marked as yes or no), blood pressure status(indicated as yes or no), dyslipidemia status(indicated as yes or no), CVD status(indicated as yes or no) and alcohol use status (categorized as more than or less than 12 drinks per year). The NHANES Survey Methods and Analysis Guide provides complete data on various methods employed for collecting variables (https://www.cdc.gov/nchs/nhanes/AnalyticGuidelines).
2.5 Statistical analysis
R (version 4.2) and EmpowerStats (version 4.1) were used for all analyses. The sample groups were divided into WWI tertiles, and the demographic traits of each group were assessed using the chi-square test and the t-test. We investigated the linear relationships between WWI and cognitive function using weighted multivariate linear regression models. After logistic transformation, the linear trend of the four dimensions of cognitive function variables was examined using the Cochran-Armitage test. The non-linear relationships between WWI and cognitive function were investigated using additional generalized additive models and smoothed curve fits. To investigate the relationship between WWI and cognitive function among different demographic groups, subgroup analysis and interaction tests were implemented. The threshold for statistical significance was a two-tailed P value of 0.05.
3 Results
3.1 Baseline characteristics
The population characteristics are shown in Table 1 in order of WWI tertiles. During the evaluation period, the study included a cohort of 2,764 individuals who were 60 years of age or older. Among this group, 48.95% were identified as male, while 51.05% were identified as female. The average age (SD) and average WWI (SD) of the 2,764 participants were 69.27 (6.74) years and 11.49 (0.71), respectively. WWI tertiles 1–3 ranges were 9.02–11.18, 11.18–11.77, and 11.77–14.79. In contrast to the group exhibiting the lowest WWI, individuals in the group demonstrating the greatest WWI displayed a higher likelihood of being female, belonging to Mexican American or other Hispanic ethnic backgrounds, possessing advanced age, engaging in smoking habits, and exhibiting a greater frequency of diabetes, stroke, and cardiovascular disease, such as hypertension, dyslipidemia, CVD. Additionally, there was a higher probability of individuals having lower levels of educational achievement and household income. Meanwhile, the CERAD-total, CERAD-delayed, Animal Fluency test, DSST scores were lower (Table 1).
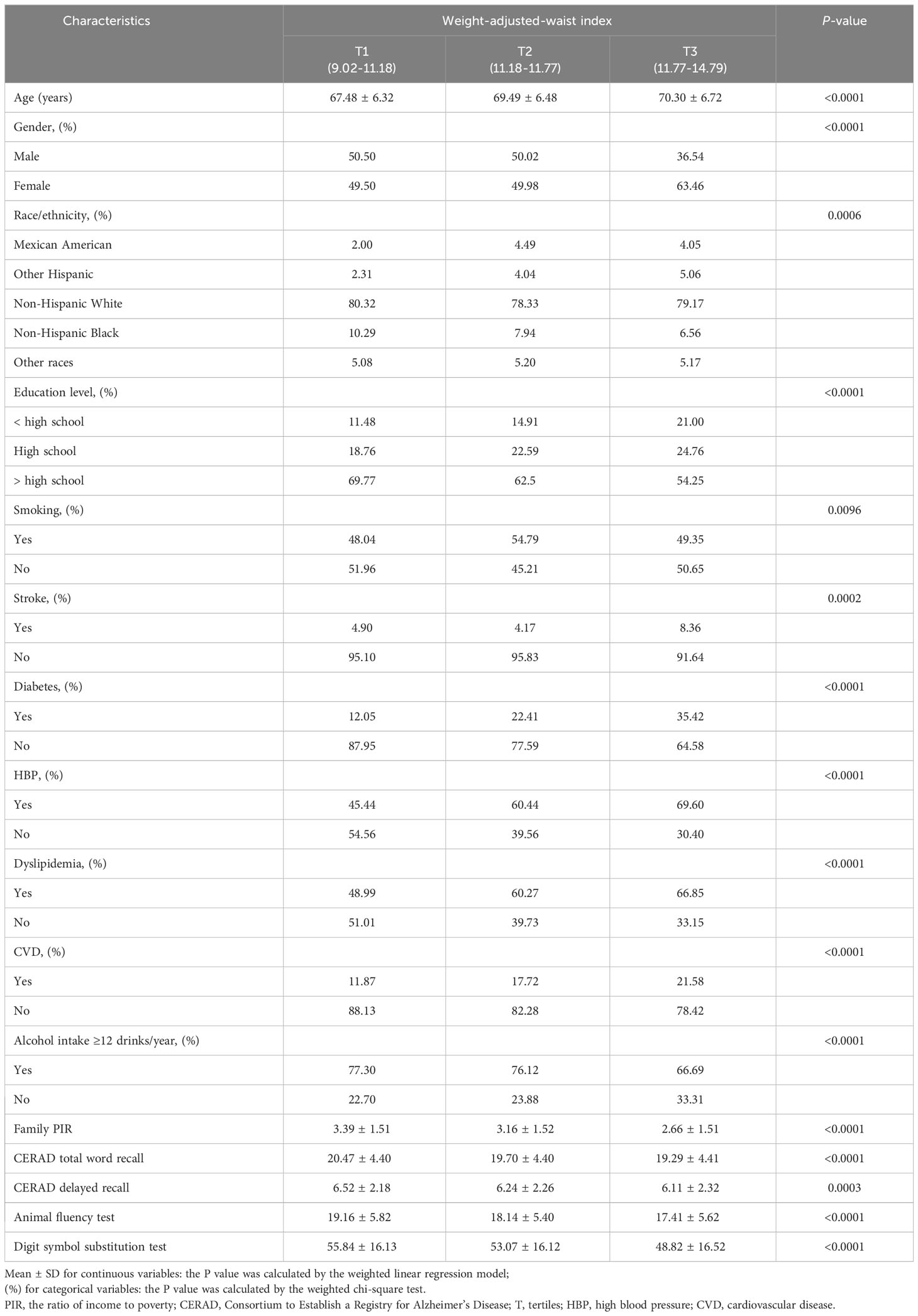
Table 1 Basic characteristics of participants by weight-adjusted-waist index tertiles among U.S. older adults.
3.2 Association between WWI and cognitive function
WWI had a strong negative linear correlation with the Digit symbol substitution test in all three linear regression models. When key demographic factors were adjusted, each unit increase in WWI score was linked to a 3.57-scores decrease in Digit symbol substitution test [-3.57 (-4.31, -2.82)], and a 0.63-scores decrease in CERAD total word recall [-0.63 (-0.85, -0.40)], and a 0.19-scores decrease in CERAD delayed recall [-0.19 (-0.30, -0.07)] and a 0.65-scores decrease in AFT [-0.65 (-0.94, -0.37)], respectively. In the fully adjusted model, the negative correlation of WWI with the Digit symbol substitution test was then 1.34 scores for each increase in WWI score for the Digit symbol substitution test [-1.34 (-2.05, -0.62)]. However, the significant negative linear connection between WWI and CERAD total word recall, CERAD delayed recall, and AFT was not preserved after controlling for all variables (Table 2).
Additionally, from a nonlinear standpoint, the generalized model and smoothed curve fitting results further corroborated the negative association between WWI and the Digit symbol substitution exam (Figure 2).
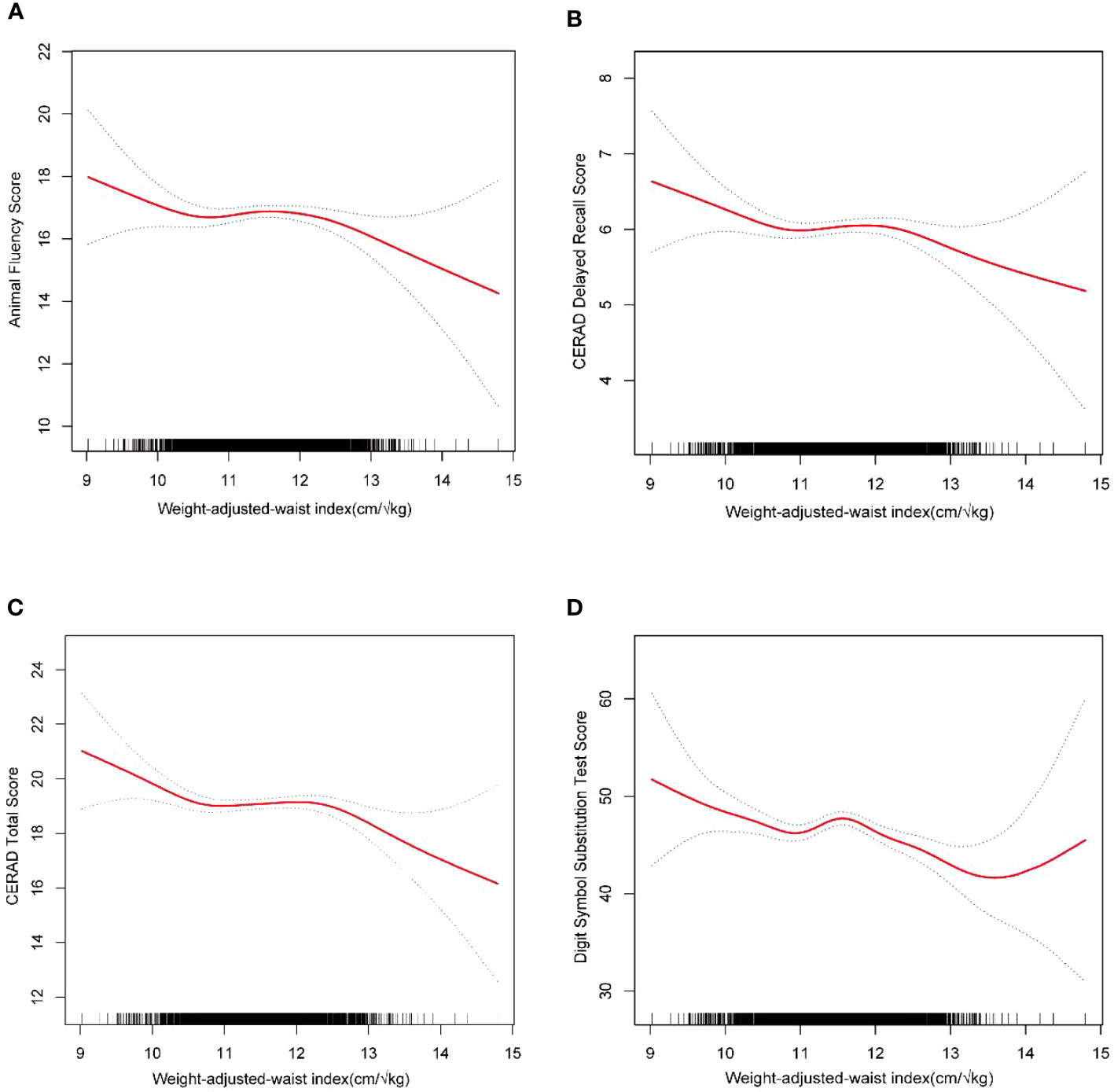
Figure 2 The nonlinear associations between WWI and Digit symbol substitution test. The solid red line represents the smooth curve fit between variables. Blue bands represent the 95% of confidence interval from the fit. (A) WWI and Animal fluent test; (B) WWI and CERAD delayed recall score; (C) WWI and CERAD total score; (D) WWI and Digit symbol substitution test.
To assess the uniformity of the association between WWI and cognitive function throughout the general population and to discover potential differences among particular population groups, we carried out subgroup analyses and interaction tests, categorized by age, gender, race, stroke status, diabetes status, HBP status, dyslipidemia status and CVD status (Table 3). The findings of this study indicate a notable disparity in the relationship between WWI and cognitive function among individuals who have suffered a stroke. Specifically, participants who have experienced a stroke [-4.37 (-7.42, -1.32)] have a stronger negative correlation between WWI with cognitive function than those without stroke [-1.18 (-1.91, -0.45)]. In the other groupings, the association between WWI and cognitive function persisted (P for interaction > 0.05).
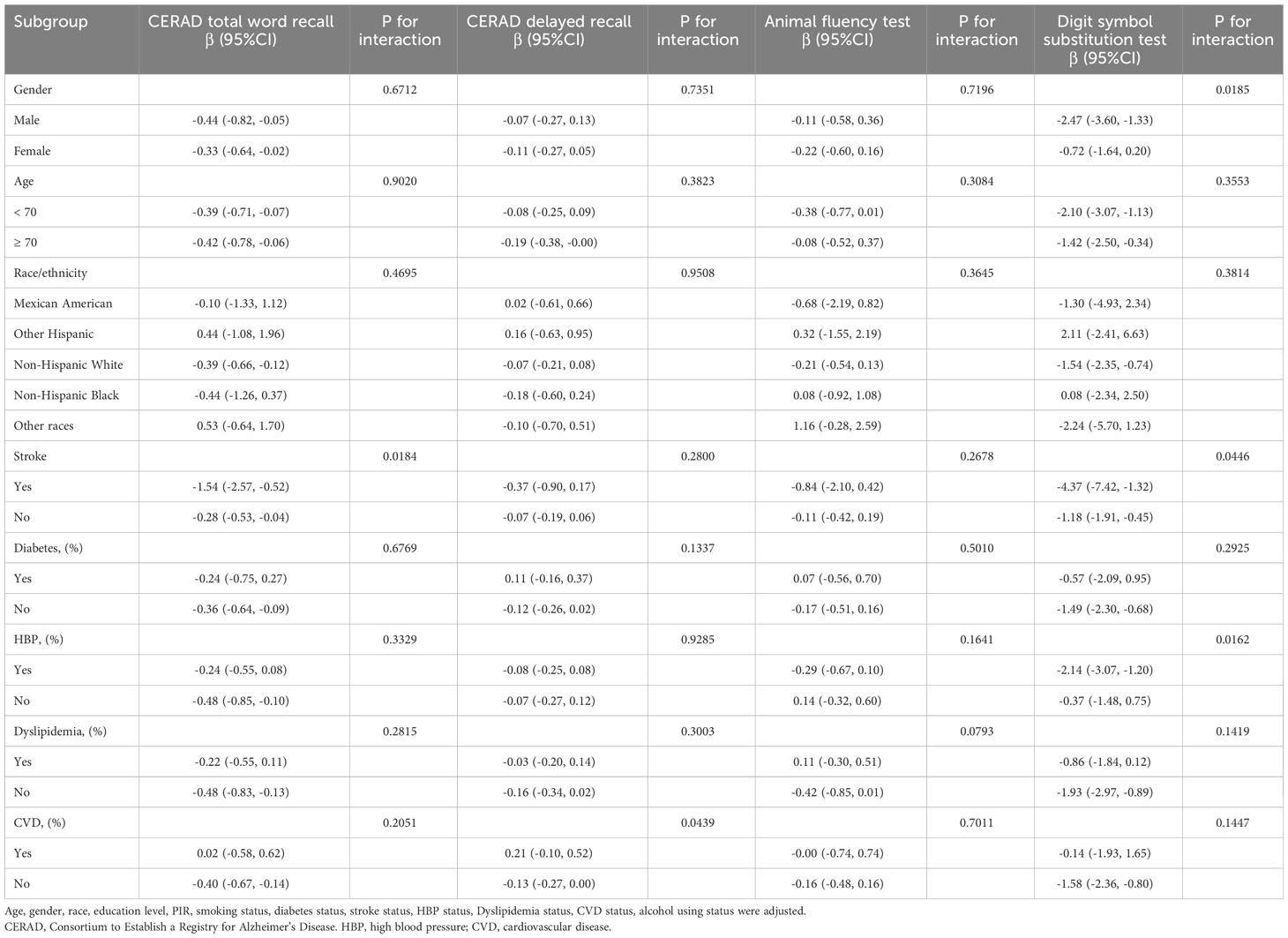
Table 3 Subgroup analysis of the association between weight-adjusted-waist index with cognitive function.
4 Discussion
This study aimed to investigate the association between a newly developed indicator of obesity, the Weight-to-Waist Index (WWI), and cognitive function in a sample of 2764 older individuals in the United States. According to our findings, a higher WWI is substantially linked to more severe cognitive impairment. The study found a negative correlation between higher levels of WWI and lower scores on the Digit Symbol Substitution Test (DSST), widely recognized as a reliable measure of overall cognitive function. This association was observed consistently across various subgroups, suggesting that higher levels of WWI are linked to poorer executive function and processing speed. The results of this study indicate that WWI could potentially serve as a reliable measure for evaluating the correlation between obesity and cognitive impairment.
To the extent of our current understanding, this is a pioneering study to evaluate the correlation between WWI and cognitive function, highlighting the negative correlation between higher WWI levels and worsening cognitive function. Obesity has been found to have a detrimental effect on cognitive performance in earlier investigations. Greater BMI was linked to worse cognitive scores, and greater BMI at baseline was also linked to higher levels of cognitive decline at follow-up, according to the prospective cohort research of 2223 middle-aged adults conducted by M Cournot et al. (15). Another cross-sectional study involving 3179 American adults over the age of 60 discovered that those who met the criteria for the metabolic syndrome—abdominal obesity, high triglycerides, and low HDL cholesterol—showed 2.39 fewer points on the CERAD delayed recall test than those who did not [Beta = 2.39, SE = 0.46, 4.32 (0.49) vs. 6.71 (0.30)] (16). In our research’s raw and adjusted models, we found a negative linear correlation between WWI and lower DSST scores. The sensitivity analysis using WWI as a tertile also showed a dose-response association between WWI and DSST score. The non-linear relationship perspective further substantiates the negative correlation between WWI and DSST.
Obesity is a state caused by excessive accumulation of body fat. The link between obesity and cognitive dysfunction can be attributed to the metabolic consequences of visceral fat, the metabolic syndrome, including insulin resistance, dyslipidemia, and hypertension (17). The most likely diseases linked to cognitive impairment and neuroinflammation are insulin resistance and hyper-inflammation (18, 19). Adipose tissue releases pro-inflammatory factors (TNF-α, IL-1β, IL-6, MCP1) and inflammation-associated proteins (C-reactive protein, CRP), and these mediators contribute to systemic inflammation (20). Several studies have elaborated on the correlation between systemic inflammation and neuronal degeneration (21, 22). Most of these inflammatory mediators and insulin resistance contribute to mitochondrial malfunction, oxidative stress, and apoptosis, inducing neuronal loss (23, 24). The interplay of inflammatory cells, inflammatory factors, and microglia activation can lead to damage in the hippocampal region and impairment of cognitive learning (25). Gang Ma et al. used liver ischemia-reperfusion as a model to investigate whether ribonuclease could attenuate postoperative cognitive dysfunction and found that surgical trauma considerably enhanced the expression of inflammatory mediators IL-1 and IL-6 in the serum and hippocampus of rats (26). Insulin resistance usually occurs due to increased visceral obesity in excess, and the relationship between insulin resistance and impaired cognitive function may be linked to insulin-degrading enzymes (IDE). These enzymes are involved in the metabolism of both insulin and β-amyloid (27, 28). The deposition of beta-amyloid in the brain is hypothesized to be among the initial, observable indications in the advancement of Alzheimer’s disease, and it is linked to cognitive deterioration, neurodegeneration, and impaired synaptic function (29–31). According to several research, the relationship between obesity and worse cognitive function may involve structural and functional modifications to the cortex and subcortex. Cognitive domains, including working memory, verbal memory, processing speed, and fluid intelligence, demonstrate observable impacts (32–34). In a study conducted by M. E. Levine et al., it was shown that individuals aged 70 years and above who had sarcopenia and obesity, either independently or simultaneously, experienced a decline in cognitive function compared to older adults who did not have sarcopenia or obesity (35).
WWI is an anthropometric index that evaluates elevated body fat mass and indicates reduced muscle mass. The study conducted by Kim et al. revealed a positive correlation between WWI and abdominal fat measures, while a negative correlation was observed between WWI and abdominal muscle mass values (36). In comparison to BMI and WC, WWI provides a more accurate measurement of natural obesity because BMI cannot differentiate between muscle mass and fat mass. A cross-sectional study involving 10,289 Chinese participants with hypertension showed a linear positive association between WWI and dementia (odds ratio [OR], 1.45; 95% CI: 1.35, 1.56), a negative association with MMSE scores (β, -1.09; 95% confidence interval [CI]. -1.24, -0.94). There was a dose-response relationship between WWI and MMSE scores and dementia. In daily clinical practice, WWI can function as a simple and reliable tool for assessing the risk of dementia (37). According to previous research, SIRT1 is substantially downregulated in the hippocampal neurons of mice with cognitive impairment (38). The anti-aging gene Silent information regulation 1 (SIRT1) regulates glycolipid metabolism, inflammatory response, cell death and apoptosis, oxidative stress, and tumor formation. SIRT1 inhibits apoptosis mediated by p53 by dephosphorylating the Lys382 residue of the p53 protein. SIRT1 and p53 may interact to modulate adipocytokines and immune responses, which may be crucial for NAFLD, obesity, and neurodegenerative diseases. Repression of SIRT1 in conjunction with other anti-aging genes, including klotho, p66Shc, and FOXO1/FOXO3a, results in aberrant regulation of glucose, lipid, and amyloid metabolism, which is associated with programmed cell death in the liver and brain. Neurons in a brain with inhibited SIRT1 may undergo premature programmed cell death accompanied by altered astrocyte-neuron interactions, thereby accelerating brain aging (39–42). The WWI may detect and diagnose early-onset anti-aging gene inactivation in the US population, which has implications for the development of obesity, diabetes, and cognitive impairment.
The findings from the subgroup analysis revealed that the association between WWI and cognitive function exhibited variability among different subgroups of stroke patients. Cognitive impairment is a prevalent complication following a stroke, affecting around 60% of those who have survived a stroke within the first year. Moreover, the incidence of cognitive impairment tends to be significantly higher in the immediate aftermath of a stroke (43, 44). 44% of stroke patients admitted to hospitals reported general cognitive impairment, according to data compiled by the Stroke and Cognition Consortium (STROKOG) from 13 studies conducted in 8 different countries (45). Based on longitudinal research, it has been observed that approximately 20% of individuals who have experienced a stroke either initially or previously are susceptible to developing early-onset post-stroke dementia (PSD). Furthermore, the prevalence of late-onset PSD ranges from 4.4% to 23.9% (46).
One of the notable aspects of this study is its comprehensive analysis of the linear and nonlinear correlations between WWI and four distinct cognitive function assessment tools. This approach provides valuable insights into the consistency of the relationship between WWI and cognitive function, offering a multifaceted perspective on the subject matter. Studies have shown that American older adults aged 50 years and older report higher severity of subjective cognitive impairment than Chinese older adults, indicating that the US older individuals may be more sensitive to cognitive impairment when compared with other populations (47). Furthermore, our study incorporated a total of 2,764 individuals who were selected as nationally representative participants based on specific criteria. The ample sample size enabled us to conduct subgroup analyses, thereby facilitating the examination of the relationship between WWI and cognitive function across various populations. This approach enhances the statistical robustness and credibility of our findings. The cross-sectional design of this study limits our ability to demonstrate a causal link between WWI and cognitive performance. The significant adverse effects of obesity on cognitive abilities such processing speed, sustained attention, and working memory, as well as the presence of confounding factors, may be responsible for the observed disparity in the correlation between WWI and the CERAD test, the AFT, and the AFT compared to the DSST. The results in the fully adjusted model may also be inaccurate because the variables linked to WWI and cognitive performance were too vast for us to account for all potential confounding factors.
5 Conclusion
Our study suggests that a higher WWI might be linked to poorer cognitive function, potentially predicting cognitive dysfunction in individuals aged 60 years and above in the United States. Further investigation is necessary to substantiate the validity of our findings.
Data availability statement
The original contributions presented in the study are included in the article/supplementary material. further inquiries can be directed to the corresponding author.
Ethics statement
The studies involving humans were approved by Research Ethics Review Board of the National Center for Health Statistics (NCHS). The studies were conducted in accordance with the local legislation and institutional requirements. The participants provided their written informed consent to participate in this study.
Author contributions
X-tH: Conceptualization, Data curation, Formal Analysis, Investigation, Methodology, Project administration, Resources, Software, Supervision, Validation, Visualization, Writing – original draft. XL: Conceptualization, Data curation, Formal Analysis, Investigation, Methodology, Project administration, Software, Writing – original draft. HJ: Conceptualization, Funding acquisition, Investigation, Methodology, Resources, Software, Supervision, Validation, Writing – review & editing.
Funding
The author(s) declare financial support was received for the research, authorship, and/or publication of this article. This work did not receive any specific grant from any funding agency in the public, commercial, or not-for-profit sector.
Conflict of interest
The authors declare that the research was conducted in the absence of any commercial or financial relationships that could be construed as a potential conflict of interest.
Publisher’s note
All claims expressed in this article are solely those of the authors and do not necessarily represent those of their affiliated organizations, or those of the publisher, the editors and the reviewers. Any product that may be evaluated in this article, or claim that may be made by its manufacturer, is not guaranteed or endorsed by the publisher.
Abbreviations
WWI, Weight-adjusted waist index; NHANES, National Health and Nutrition Examination Survey; NCHS, National Center for Health Statistics; CRP, C-reactive protein; CERAD E-L, Consortium to Establish a Registry for Alzheimer’s Disease; AFT, Animal Fluency Test; DSST, Digit Symbol Substitution Test; BMI, Body Mass Index; WC, Waist Circumference; HBP, High Blood Pressure; CVD, Cardiovascular Disease.
References
1. Eshkoor SA, Hamid TA, Mun CY, Ng CK. Mild cognitive impairment and its management in older people. Clin Interv Aging (2015) 10:687–93. doi: 10.2147/cia.S73922
2. Shahnawaz Z, Reppermund S, Brodaty H, Crawford JD, Draper B, Trollor JN, et al. Prevalence and characteristics of depression in mild cognitive impairment: the Sydney Memory and Ageing Study. Acta Psychiatr Scand (2013) 127(5):394–402. doi: 10.1111/acps.12008
3. Miller AA, Spencer SJ. Obesity and neuroinflammation: a pathway to cognitive impairment. Brain Behav Immun (2014) 42:10–21. doi: 10.1016/j.bbi.2014.04.001
4. Jagust W, Harvey D, Mungas D, Haan M. Central obesity and the aging brain. Arch Neurol (2005) 62(10):1545–8. doi: 10.1001/archneur.62.10.1545
5. Anstey KJ, Cherbuin N, Budge M, Young J. Body mass index in midlife and late-life as a risk factor for dementia: a meta-analysis of prospective studies. Obes Rev (2011) 12(5):e426–37. doi: 10.1111/j.1467-789X.2010.00825.x
6. Arnold SE, Arvanitakis Z, Macauley-Rambach SL, Koenig AM, Wang HY, Ahima RS, et al. Brain insulin resistance in type 2 diabetes and Alzheimer disease: concepts and conundrums. Nat Rev Neurol (2018) 14(3):168–81. doi: 10.1038/nrneurol.2017.185
7. Wolf PA, Beiser A, Elias MF, Au R, Vasan RS, Seshadri S. Relation of obesity to cognitive function: importance of central obesity and synergistic influence of concomitant hypertension. The Framingham Heart Study. Curr Alzheimer Res (2007) 4(2):111–6. doi: 10.2174/156720507780362263
8. Park Y, Kim NH, Kwon TY, Kim SG. A novel adiposity index as an integrated predictor of cardiometabolic disease morbidity and mortality. Sci Rep (2018) 8(1):16753. doi: 10.1038/s41598-018-35073-4
9. Curtin LR, Mohadjer LK, Dohrmann SM, Montaquila JM, Kruszan-Moran D, Mirel LB, et al. The national health and nutrition examination survey: sample design, 1999-2006. Vital Health Stat (2012) 2(155):1–39.
10. Fillenbaum GG, Mohs R. CERAD (Consortium to establish a registry for alzheimer's disease) neuropsychology assessment battery: 35 years and counting. J Alzheimers Dis (2023) 93(1):1–27. doi: 10.3233/jad-230026
11. Paajanen T, Hänninen T, Tunnard C, Hallikainen M, Mecocci P, Sobow T, et al. CERAD neuropsychological compound scores are accurate in detecting prodromal alzheimer's disease: a prospective AddNeuroMed study. J Alzheimers Dis (2014) 39(3):679–90. doi: 10.3233/jad-122110
13. Proust-Lima C, Amieva H, Dartigues JF, Jacqmin-Gadda H. Sensitivity of four psychometric tests to measure cognitive changes in brain aging-population-based studies. Am J Epidemiol (2007) 165(3):344–50. doi: 10.1093/aje/kwk017
14. Bienias JL, Beckett LA, Bennett DA, Wilson RS, Evans DA. Design of the Chicago health and aging project (CHAP). J Alzheimers Dis (2003) 5(5):349–55. doi: 10.3233/jad-2003-5501
15. Cournot M, Marquié JC, Ansiau D, Martinaud C, Fonds H, Ferrières J, et al. Relation between body mass index and cognitive function in healthy middle-aged men and women. Neurology (2006) 67(7):1208–14. doi: 10.1212/01.wnl.0000238082.13860.50
16. Díaz-Camargo E, Hernández-Lalinde J, Sánchez-Rubio M, Chaparro-Suárez Y, Álvarez-Caicedo L, Fierro-Zarate A, et al. NHANES 2011-2014 reveals decreased cognitive performance in U.S. Older adults with metabolic syndrome combinations. Int J Environ Res Public Health (2023) 20(7):5257. doi: 10.3390/ijerph20075257
17. Kouvari M, D'Cunha NM, Travica N, Sergi D, Zec M, Marx W, et al. Metabolic syndrome, cognitive impairment and the role of diet: A narrative review. Nutrients (2022) 14(2). doi: 10.3390/nu14020333
18. Barber TM, Kyrou I, Randeva HS, Weickert MO. Mechanisms of insulin resistance at the crossroad of obesity with associated metabolic abnormalities and cognitive dysfunction. Int J Mol Sci (2021) 22(2):546. doi: 10.3390/ijms22020546
19. Monteiro R, Azevedo I. Chronic inflammation in obesity and the metabolic syndrome. Mediators Inflamm (2010) 2010. doi: 10.1155/2010/289645
20. Dermitzaki E, Liapakis G, Androulidaki A, Venihaki M, Melissas J, Tsatsanis C, et al. Corticotrophin-Releasing Factor (CRF) and the urocortins are potent regulators of the inflammatory phenotype of human and mouse white adipocytes and the differentiation of mouse 3T3L1 pre-adipocytes. PloS One (2014) 9(5):e97060. doi: 10.1371/journal.pone.0097060
21. Crispino M, Trinchese G, Penna E, Cimmino F, Catapano A, Villano I, et al. Interplay between peripheral and central inflammation in obesity-promoted disorders: the impact on synaptic mitochondrial functions. Int J Mol Sci (2020) 21(17). doi: 10.3390/ijms21175964
22. Qin L, Wu X, Block ML, Liu Y, Breese GR, Hong J-S, et al. Systemic LPS causes chronic neuroinflammation and progressive neurodegeneration. Glia (2007) 55(5):453–62. doi: 10.1002/glia.20467
23. Manucha W, Ritchie B, Ferder L. Hypertension and insulin resistance: implications of mitochondrial dysfunction. Curr Hypertens Rep (2015) 17(1):504. doi: 10.1007/s11906-014-0504-2
24. Kleinridders A, Cai W, Cappellucci L, Ghazarian A, Collins WR, Vienberg SG, et al. Insulin resistance in brain alters dopamine turnover and causes behavioral disorders. Proc Natl Acad Sci USA (2015) 112(11):3463–8. doi: 10.1073/pnas.1500877112
25. Feng X, Valdearcos M, Uchida Y, Lutrin D, Maze M, Koliwad SK. Microglia mediate postoperative hippocampal inflammation and cognitive decline in mice. JCI Insight (2017) 2(7):e91229. doi: 10.1172/jci.insight.91229
26. Ma G, Chen C, Jiang H, Qiu Y, Li Y, Li X, et al. Ribonuclease attenuates hepatic ischemia reperfusion induced cognitive impairment through the inhibition of inflammatory cytokines in aged mice. BioMed Pharmacother (2017) 90:62–8. doi: 10.1016/j.biopha.2017.02.094
27. Jha NK, Jha SK, Kumar D, Kejriwal N, Sharma R, Ambasta RK, et al. Impact of insulin degrading enzyme and neprilysin in alzheimer's disease biology: characterization of putative cognates for therapeutic applications. J Alzheimers Dis (2015) 48(4):891–917. doi: 10.3233/jad-150379
28. Su F, Shu H, Ye Q, Wang Z, Xie C, Yuan B, et al. Brain insulin resistance deteriorates cognition by altering the topological features of brain networks. NeuroImage Clin (2017) 13:280–87. doi: 10.1016/j.nicl.2016.12.009
29. Knopman DS, Amieva H, Petersen RC, Chételat G, Holtzman DM, Hyman BT, et al. Alzheimer disease. Nat Rev Dis Primers (2021) 7(1):33. doi: 10.1038/s41572-021-00269-y
30. Huber CM, Yee C, May T, Dhanala A, Mitchell CS. Cognitive decline in preclinical alzheimer's disease: amyloid-beta versus tauopathy. J Alzheimers Dis (2018) 61(1):265–81. doi: 10.3233/jad-170490
31. Öhrfelt A, Benedet AL, Ashton NJ, Kvartsberg H, Vandijck M, Weiner MW, et al. Association of CSF GAP-43 with the rate of cognitive decline and progression to dementia in amyloid-positive individuals. Neurology (2023) 100(3):e275–e85. doi: 10.1212/wnl.0000000000201417
32. Morys F, Dadar M, Dagher A. Association between midlife obesity and its metabolic consequences, cerebrovascular disease, and cognitive decline. J Clin Endocrinol Metab (2021) 106(10):e4260–74. doi: 10.1210/clinem/dgab135
33. Wu X, Palaniyappan L, Yu G, Zhang K, Seidlitz J, Liu Z, et al. Morphometric dis-similarity between cortical and subcortical areas underlies cognitive function and psychiatric symptomatology: a preadolescence study from ABCD. Mol Psychiatry (2023) 28(3):1146–58. doi: 10.1038/s41380-022-01896-x
34. Nakai T, Nishimoto S. Representations and decodability of diverse cognitive functions are preserved across the human cortex, cerebellum, and subcortex. Commun Biol (2022) 5(1):1245. doi: 10.1038/s42003-022-04221-y
35. Levine ME, Crimmins EM. Sarcopenic obesity and cognitive functioning: the mediating roles of insulin resistance and inflammation? Curr Gerontol Geriatr Res (2012) 2012:826398. doi: 10.1155/2012/826398
36. Kim JY, Choi J, Vella CA, Criqui MH, Allison MA, Kim NH. Associations between weight-adjusted waist index and abdominal fat and muscle mass: multi-ethnic study of atherosclerosis. Diabetes Metab J (2022) 46(5):747–55. doi: 10.4093/dmj.2021.0294
37. Zhou W, Xie Y, Yu L, Yu C, Bao H, Cheng X. Positive association between weight-adjusted-waist index and dementia in the Chinese population with hypertension: a cross-sectional study. BMC Psychiatry (2023) 23(1):519. doi: 10.1186/s12888-023-05027-w
38. Shu J, Huang X, Liao Q, Wang J, Zhou Y, Chen Y, et al. Sevoflurane improves hemorrhagic shock and resuscitation-induced cognitive impairments and mitochondrial dysfunctions through SIRT1-mediated autophagy. Oxid Med Cell Longev (2022) 2022:9771743. doi: 10.1155/2022/9771743
39. Martins IJ. Anti-aging genes improve appetite regulation and reverse cell senescence and apoptosis in global populations. Adv Aging Res (2016) 5(1):9–26. doi: 10.4236/aar.2016.51002
40. James MI. Single gene inactivation with implications to diabetes and multiple organ dysfunction syndrome. J Clin Epigenet (2017) 03(03). doi: 10.21767/2472-1158.100058
41. Martins I. Nutrition therapy regulates caffeine metabolism with relevance to NAFLD and induction of type 3 diabetes. J Diabetes Metab Disord (2017) 4:019. doi: 10.24966/DMD-201X/100019
42. Martins IJ. Insulin therapy and autoimmune disease with relevance to non alchoholic fatty liver disease. Nonalcoholic fatty liver disease - an update. IntechOpen (2018), 1–14. doi: 10.5772/intechopen.81297
43. Lee M, Saver JL, Hong KS, Wu Y-L, Liu HC, Rao NM, et al. Cognitive impairment and risk of future stroke: a systematic review and meta-analysis. Cmaj (2014) 186(14):E536–46. doi: 10.1503/cmaj.140147
44. El Husseini N, Katzan IL, Rost NS, Blake ML, Byun E, Pendlebury ST, et al. Cognitive impairment after ischemic and hemorrhagic stroke: A scientific statement from the American Heart Association/American Stroke Association. Stroke (2023) 54(6):e272–91. doi: 10.1161/str.0000000000000430
45. Lo JW, Crawford JD, Desmond DW, Godefroy O, Jokinen H, Mahinrad S, et al. Profile of and risk factors for poststroke cognitive impairment in diverse ethnoregional groups. Neurology (2019) 93(24):e2257–e71. doi: 10.1212/wnl.0000000000008612
46. Mok VCT, Lam BYK, Wang Z, Liu W, Au L, Leung EYL, et al. Delayed-onset dementia after stroke or transient ischemic attack. Alzheimers Dement (2016) 12(11):1167–76. doi: 10.1016/j.jalz.2016.05.007
Keywords: a population-based study, weight-adjusted waist index, cognitive function, NHANES, obesity, older
Citation: Huang X-t, Lv X and Jiang H (2023) The weight-adjusted-waist index and cognitive impairment among U.S. older adults: a population-based study. Front. Endocrinol. 14:1276212. doi: 10.3389/fendo.2023.1276212
Received: 11 August 2023; Accepted: 29 September 2023;
Published: 08 November 2023.
Edited by:
Dave Bridges, University of Michigan, United StatesReviewed by:
Ian James Martins, University of Western Australia, AustraliaAlpna Tyagi, University of Colorado Anschutz Medical Campus, United States
Copyright © 2023 Huang, Lv and Jiang. This is an open-access article distributed under the terms of the Creative Commons Attribution License (CC BY). The use, distribution or reproduction in other forums is permitted, provided the original author(s) and the copyright owner(s) are credited and that the original publication in this journal is cited, in accordance with accepted academic practice. No use, distribution or reproduction is permitted which does not comply with these terms.
*Correspondence: Hong Jiang, ZHJfaG9uZ2ppYW5nQDE2My5jb20=; Xiang Lv, bXprbHZ4aWFuZ0AxNjMuY29t