- 1The George Institute for Global Health, Faculty of Medicine, University of New South Wales, Sydney, NSW, Australia
- 2Department of Stroke, The George Institute for Global Health China, Beijing, China
- 3Neurology Department, Shanghai East Hospital, School of Medicine, Tongji University, Shanghai, China
- 4Department of Neurology and Clinical Research Center of Neurological Disease, The Second Affiliated Hospital of SooChow University, Suzhou, China
- 5Department of Cardiovascular Sciences and National Institute for Health and Care Research (NIHR) Leicester Biomedical Research Centre, Leicester, United Kingdom
- 6Westmead Clinical School, University of Sydney, Sydney, NSW, Australia
- 7Department of Public Health, Fukuoka University, Fukuoka, Japan
- 8Facultad de Medicina, Clinica Alemana Universidad del Desarrollo, Santiago, Chile
Background: The effect of renal impairment in patients who receive intravenous thrombolysis for acute ischemic stroke (AIS) is unclear. We aimed to determine the associations of renal impairment and clinical outcomes and any modification of the effect of intensive versus guideline-recommended blood pressure (BP) control in the BP arm of the International Enhanced Control of Hypertension and Thrombolysis Stroke Study (ENCHANTED).
Methods: We conducted a post-hoc analysis of the ENCHANTED BP arm, which involved 2,196 thrombolyzed AIS patients. Logistic regression models were used to define the association between eGFR and clinical outcomes of death, death or major disability [modified Rankin scale (mRS) scores 3–6], and major disability (mRS 3–5) at 90 days.
Results: Of the 2,151 patients with available baseline renal function data (mean age 66.9 years; 38% women), 993 (46.2%), 822 (38.2%), and 336 (15.6%) had normal (eGFR ≥ 90 mL/min/1.73 m2), mildly (60–89), and moderate-to-severely impaired (<60) renal function, respectively. Compared with patients with normal eGFR, mortality was higher in those with moderate-to-severe renal impairment (adjusted odds ratio 1.77, 95% confidence interval 1.05–2.99; p = 0.031 for trend). However, the difference in death or major disability (mRS 3–6) was not significant between groups. There was no heterogeneity in the effect of intensive versus guideline-recommended BP-lowering treatment on death by grades of renal function (p for interaction = 0.545).
Discussion: The presence of moderate-to-severe renal impairment is associated with increased mortality in thrombolyzed patients with AIS. Renal function does not modify the effect of early intensive BP-lowering treatment on death in this patient group.
Introduction
Both stroke and chronic kidney disease (CKD) are common public health concerns that caused 143.2 million disability-adjusted life years (DALYs) and 41.5 million DALYs in 2019, respectively (1), and often occur as comorbidities (2). Renal impairment is an independent risk factor for serious cardiovascular disease, including stroke (3, 4). While studies have reported an association between renal impairment and poor functional outcome in patients with acute ischemic stroke (AIS) (5, 6), data are more limited in those who receive intravenous thrombolysis and BP-lowering treatment where the risk of intracerebral hemorrhage (ICH) and other adverse outcomes may be increased (7–10).
The blood pressure (BP) arm of the International Enhanced Control of Hypertension and Thrombolysis Stroke Study (ENCHANTED) trial showed that intensive BP-lowering treatment did not improve clinical outcomes after AIS, despite reducing the risk of intracranial hemorrhage (11). It is unknown as to whether there is an interaction between intensive BP-lowering treatment and renal function on clinical outcomes in this patient group. On the one hand, intensive BP control may cause potential renal impairment from a decrease in renal perfusion pressure and blood flow, and this may in turn lead to a higher risk of cerebral bleeding and poor functional outcome. Moreover, BP variability may be influenced by renal functional status in relation to therapeutic and psychological resistance (12). On the other hand, intensive BP-lowering treatment may have added benefits in “high-risk” patients with renal impairment who receive intravenous thrombolysis for AIS. Thus, we conducted a post-hoc analysis of the ENCHANTED trial to assess the prognostic significance of renal impairment and its interaction with the randomized treatment on clinical outcomes in thrombolyzed AIS patients.
Methods
Study design
ENCHANTED was an international, multicenter, prospective, quasifactorial, randomized, open-label blinded-endpoint assessed trial, for which the main results have been presented elsewhere (11, 13). For the BP arm, 2,196 eligible patients were randomly assigned (1:1, by means of a central web-based system) within 6 h of stroke onset to receive either intensive [target systolic BP (SBP) 130–140 mmHg within 1 h] or guideline (target SBP <180 mmHg) BP-lowering treatment over 72 h after the commencement of intravenous thrombolysis. The study protocol was approved by the ethics committees of each participating center, and written informed consent was obtained from the patients or an appropriate surrogate. Baseline demographic and clinical characteristics were obtained at the time of hospital presentation. Stroke severity was measured on both the Glasgow Coma Scale (GCS) and the National Institutes of Health Stroke Scale (NIHSS) at baseline, 24 h, and 7 days (or earlier on discharge from the hospital).
Measures
Non-invasive BP monitoring was undertaken using an automated device applied to the non-hemiparetic arm (or right arm in the case of coma or tetraparesis), with the patient resting supine for at least 3 min in accordance with a standard protocol. Following thrombolysis, BP measurements were recorded every 15 min for 1 h, hourly from 1 to 6 h, and 6-hourly from 6 to 24 h. Thereafter, BP was recorded twice daily for 1 week (or until hospital discharge or death, if earlier). An admission blood test was conducted before the thrombolysis. Estimated glomerular filtration rate (eGFR) was calculated using the Chronic Kidney Disease–Epidemiology Collaboration equation (14). Renal function was classified into three stages: G1, normal (eGFR ≥ 90 mL/min/1.73 m2); G2, mild impairment (eGFR 60–89); and G3, moderate-to-severe impairment (eGFR < 60) (15).
Clinical outcomes were death, major disability [modified Rankin scale (mRS) scores 3–5], death or major disability (mRS scores 3–6), and physical function (ordinal shift in mRS scores) at 90 days. The key safety outcome was ICH according to centrally adjudicated brain images in association with symptoms: symptomatic intracerebral hemorrhage (sICH) was ICH associated with substantial neurological deterioration or death, according to the Safe Implementation of Thrombolysis in Stroke-Monitoring Study (SITS-MOST) definition of a large or remote parenchymal ICH (type 2, defined as >30% of the infarcted area affected by hemorrhage with mass effect or extension outside the infarct) combined with neurological deterioration (≥4 points on the NIHSS) or leading to death within 24–36 h (16). Other criteria for sICH were also used in this study.
Statistical analysis
Demographic and baseline clinical characteristics were presented as mean with standard deviation (SD) or median with interquartile range (IQR) for continuous variables and numbers with percentages for categorical variables. Baseline characteristics across the grades of renal function were compared using the chi-square test, analysis of variance, or the Kruskal–Wallis test, as appropriate. The repeated measure linear mixed model was conducted to compare SBP observed in various grades over 7 days by treatment group, adjusting for baseline SBP. Associations between renal function and the efficacy and safety outcomes were estimated using logistic regression models: model 1 as univariate; model 2 with adjustment for minimal variables of age and sex; and model 3 as fully adjusted for variables of age, sex, time from stroke onset to randomization (hour), ethnicity, GCS score, NIHSS score, hypertension, coronary artery disease, diabetes mellitus, atrial fibrillation (AF), other heart disease, hypercholesterolemia, use of glucose-lowering treatment, smoking status, baseline SBP, baseline diastolic BP, premorbid mRS (0 or 1), premorbid use of aspirin, and randomized treatment (intensive vs. guideline-recommended BP-lowering treatment) as recorded at baseline. Logistic regression models were also used to compare the randomized treatment effect on the efficacy and safety outcomes across the three grades of renal function by adding an interaction term to the models. We also use eGFR as a continuous variable in logistic regression models to comprehensively estimate the relationship between eGFR and the outcomes of death, treatment effects, and sICH. Restricted cubic spline analysis was performed to examine the effect patterns, with 60 mL/min/1.73 m2 as the reference point. We conducted fractional polynomial analysis for the interaction effect between randomized treatment and continuous eGFR. Cause-specific mortality at 90 days was also assessed. Heterogeneity for the effect of renal function on mortality across three demography subgroups was assessed through tests for interaction, including age, sex, and ethnicity. As missing data for renal function accounted for less than 10% of the full dataset, we did not conduct imputation.
Data are reported with odds ratios (OR) and 95% confidence intervals (CI). Two-sided p-values were reported with p <0.05 considered as statistically significant. Data analyses were performed with SAS version 9.3 (SAS Institute): Cary, North Carolina, USA.R Foundation for Statistical Computing: Vienna, Austria.
Results
Overall, 2,151 patients (mean age 66.9 years; 38.0% women) with available baseline renal function data were involved in this analysis, as outlined in Supplementary Figure 1. Baseline median eGFR was 86.9 (IQR 68.6–108.6) mL/min/1.73 m2, with 993 (46.2%), 822 (38.2%), and 336 (15.6%) patients identified as normal (G1), mild impairment (G2), and moderate-to-severe impairment (G3) of renal function. Demographic and clinical characteristics by baseline renal function category are outlined in Table 1. Patients with decreased eGFR were older, had more severe neurological impairment, and had greater frequency of hypertension, coronary artery disease, other heart disease, AF, diabetes mellitus, hypercholesterolemia, use of antiplatelet and glucose-lowering agents, and premorbid symptoms.
Supplementary Figure 2 depicts SBP management profiles in patients with different baseline renal functions, overall and by randomized treatment groups. Mean SBP within 7 days was higher in patients with moderate-to-severe impairment of eGFR (G3) than in the other two groups (p < 0.0001), in both BP-lowering groups.
There was no statistically significant association of impaired renal function with either death or major disability [mRS scores 3 to 6; adjusted odds ratio (aOR) 1.06, 95% confidence interval (CI) 0.78–1.45; p = 0.940 for trend] or major disability (mRS scores 3 to 5, aOR 1.01, 95% CI 0.72–1.43; p = 0.708 for trend) at 90 days. However, patients with stage G3 had nearly two-fold greater odds of death compared to those with stage G1 (aOR 1.77, 95% CI 1.05–2.99; p = 0.031 for trend) within 90 days (Table 2). There is no multicollinearity in covariates in the above models. A non-linear association between baseline eGFR as a continuous variable and death after adjustment for other variables is shown in Figure 1, and the OR of death was statistically significantly increased where the eGFR was <60 mL/min/1.73 m2.
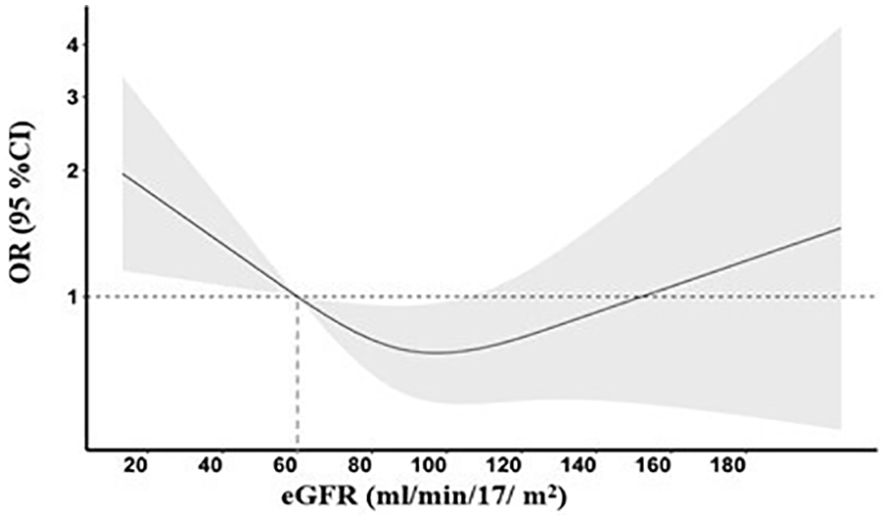
Figure 1. Risk of death across the baseline estimated glomerular filtration rate (eGFR). A restricted cubic spline curve with three knots (53.5, 86.9, and 130.2) illustrating the association of the baseline eGFR with odds ratios (solid line) and 95% confidence intervals (gray area). The model was adjusted for age, sex, time from stroke onset to randomization (hour), ethnicity, GCS score, NIHSS score, hypertension, coronary artery disease, diabetes mellitus, atrial fibrillation, other heart disease, hypercholesterolemia, glucose-lowering treatment, smoker, baseline systolic BP, baseline diastolic BP, premorbid mRS (0 or 1), premorbid use of aspirin, and randomized treatment (intensive vs. guideline-recommended BP-lowering treatment).
There was no statistically significant interaction between stages of renal function and randomized treatment effect on clinical outcomes, including for an ordinal shift in mRS scores (p for interaction = 0.192) (Figure 2) and others (Table 3). In fractional polynomial analysis, no significant interaction effect on death was shown between continuous eGFR value and randomized treatment. Patients with decreased eGFR at baseline did not increase the risk of death significantly in the intensive BP-lowering group (p for interaction = 0.853) (Supplementary Figure 3).
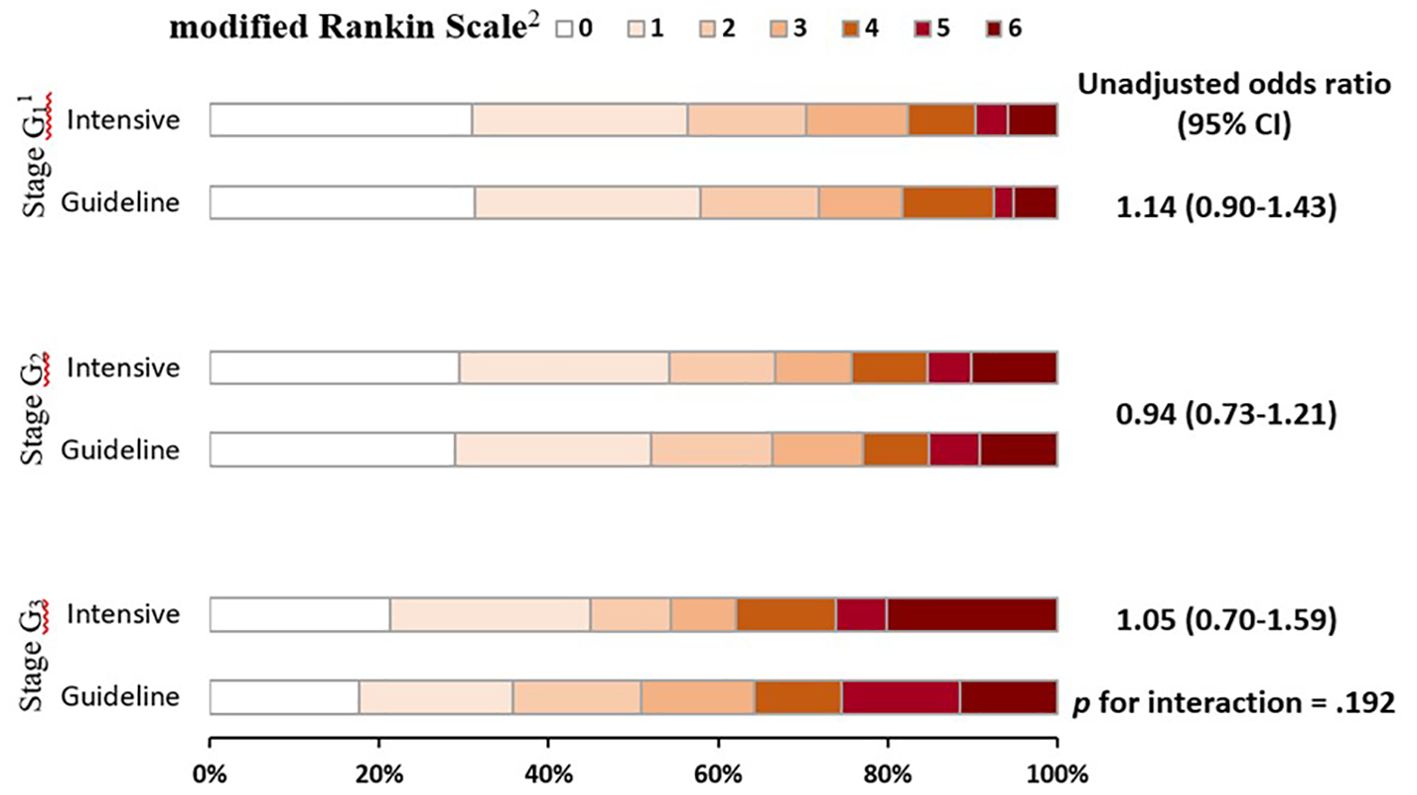
Figure 2. Global functional outcome at 90 days in patients by stages of renal function and randomized treatment. Raw distribution of scores is shown by renal failure stages. 1Scores on the modified Rankin scale range from 0 to 6, with 0 indicating no symptoms, 1 symptoms without clinically significant disability, 2 slight disability, 3 moderate disability, 4 moderately severe disability, 5 severe disability, and 6 death. 2Categories of estimated glomerular filtration rate: stage G1 (≥90 mL/min/1.73 m2), stage G2 (60–89 mL/min/1.73 m2), and stage G3 (<60 mL/min/1.73 m2).
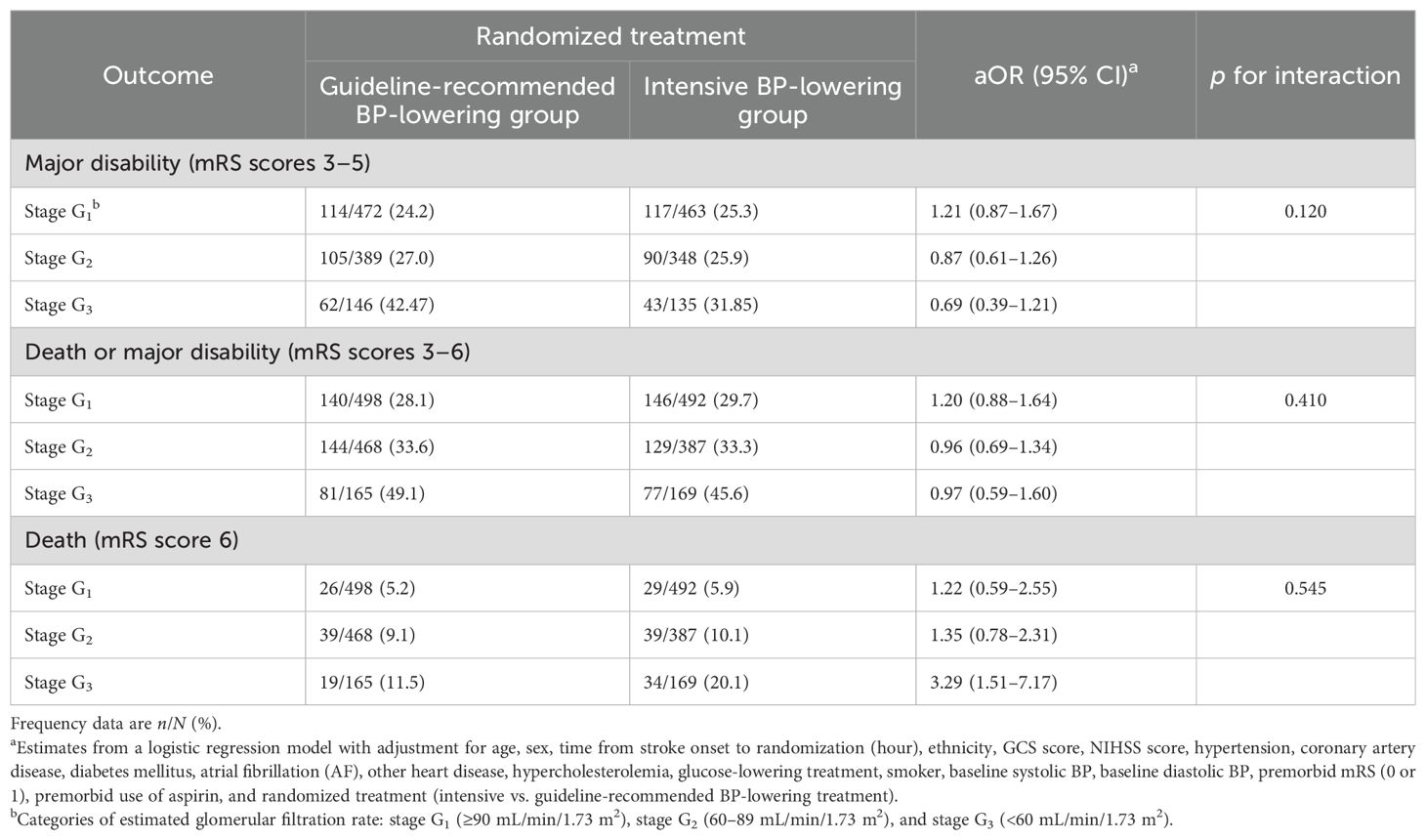
Table 3. Categorical primary outcome at 90 days by stages of renal function and randomized treatment.
Patients with decreased eGFR had a higher risk of sICH according to the SITS-MOST and other definitions (Supplementary Table 1). A series of splines display the non-linear relationship between baseline continuous eGFR (eGFR 60 mL/min/1.73 m2 as the reference) and sICH. For eGFR <60 mL/min/1.73 m2, the OR for sICH increased with decreased baseline eGFR (Supplementary Figure 4). Patients with decreased eGFR had a higher risk of death caused by the direct effects of acute ischemic stroke and non-vascular reasons (Supplementary Table 2). There was no significant interaction between renal impairment and the three subgroups on mortality (Figure 3). However, we found that the risk of mortality was significantly higher in the Asian subgroup than in the non-Asian subgroup. In the Asian group, stage G2 had nearly two-fold greater odds of death compared to those with stage G1 (aOR 1.79, 95% CI 1.08–2.98) and stage G3 (more than two-fold) (aOR 2.36, 95% CI 1.25–4.49) (Figure 3).
Discussion
In this post-hoc analysis of the ENCHANTED BP arm, moderate-to-severe impairment of eGFR was adversely associated with mortality and risk of sICH but not with functional outcome in thrombolyzed patients with AIS. We also found that patients with moderate-to-severe renal impairment maintained a higher SBP compared to those with normal or mild renal impairment, regardless of the randomized treatment allocation. There was no evidence of heterogeneity in the effect of intensive BP-lowering treatment on death according to different renal function groups.
Renal dysfunction was proven to increase mortality in critical illnesses including AIS (8, 17–20). Our study further adds to this knowledge by defining the association specifically in thrombolyzed AIS and is consistent with an earlier analysis of the ENCHANTED alteplase-dose arm and other studies (8, 21). The relationship between eGFR and mortality was non-linear, and the odds of death were statistically significantly higher in patients whose baseline eGFR was less than 60 mL/min/1.73 m2. Potential explanations are that patients with renal impairment exhibit other organ dysfunction, which increases the risk of death (22). We did not find any statistically significant association between renal impairment and disability, as observed in other studies (23, 24), but ICH was higher in those patients with pre-existing renal impairment, which may have potentiated effects on the coagulation system and morphologically changed blood vessel walls through interactions between the endothelium and platelets (25, 26). Patients with renal impairment have varying degrees of systematic inflammation, which also influences the risk of bleeding (27), while dialysis treatment is known to produce a hypocoagulable state and exacerbate the likelihood of ICH (28). Thus, weighing up the potential risks and benefits of thrombolysis treatment in patients with chronic kidney disease can be challenging.
In our study, patients with severe renal impairment had more hypertension and higher baseline BP and maintained higher BP over the subsequent 7 days compared to those with normal or mildly reduced renal function, which potentially reflects the complex interaction between renal function and response to BP-lowering treatment in AIS, as shown elsewhere (12). Renal impairment is a common cause of hypertension, and there was a higher frequency of history of hypertension at baseline in the G3 compared to the other two groups (29). The higher mean SBP achieved within 6 h raises the possibility that renal impairment contributes to the therapeutic resistance to antihypertensive agents through such mechanisms as increased sympathetic activity, alterations in the renin–angiotensin–aldosterone system, vascular aging and atherosclerosis, and sodium dysregulation from reduced functioning nephrons and lowered glomerular filtration rate (29, 30). Of course, clinicians may have also altered the intensity of their BP-lowering treatment from concerns about inducing renal impairment in such high-risk patients (31).
There was no heterogeneity in the BP-lowering treatment effect on death across different stages of renal impairment. Although the rate of death appeared higher in the intensive BP-lowering group with decreased eGFR, this finding lacked precision due to small numbers. This might be a reminder for clinicians to be cautious about the use of intensive BP-lowering treatment in patients with known renal impairment, but more data are needed to confirm this finding. Subgroup analysis results of ethnicity found that renal impairment might lead to a higher risk of mortality in Asians. As there was no heterogeneity, more studies are needed in the future to confirm it.
The strengths of our study include the prospective design with high rates of follow-up, treatment compliance, and rigorous assessment of outcomes in a relatively large sample of patients with a broad range of characteristics. Our analyses included the use of restricted cubic spline models and various sensitive analyses to describe the relationship between eGFR and outcomes. However, our study has limitations that include the dataset pertaining to a clinical trial population where most patients were of Asian ethnicity where the CKD-EPI equation, which was derived from North American and European populations, may have measurement bias. Selection bias may have also led to the exclusion of patients with severe AIS where the risks of intensive BP-lowering treatment were perceived to be high, thus further limiting the generalizability of our findings. Furthermore, the use of baseline/admission eGFR as the exposure marker may be misleading as serum creatinine is prone to elevation by acute illness (32), and the definitions for renal impairment normally require persistence of eGFR <60 mL/min per 1.73 m2 for at least 3 months in the absence of a reversible condition (33). Finally, the ENCHANTED trial did not demonstrate an improvement in functional outcomes, primarily due to the minimal difference in mean SBP between the intensive BP-lowering group and the control group. Consequently, a post-hoc analysis might inherently possess limitations in addressing the research question of this article.
Conclusion
In summary, our analysis of the ENCHANTED BP arm clinical trial dataset has shown that renal impairment predicts higher mortality in thrombolyzed AIS patients. Patients with reduced eGFR might also have a higher risk of sICH. Uncertainty persists as to whether intensive BP-lowering treatment confers benefits over guideline-recommended treatment in AIS patients with renal impairment.
Data availability statement
The raw data supporting the conclusions of this article will be made available by the authors, without undue reservation.
Ethics statement
The studies involving humans were approved by http://www.clinicaltrials.gov. Unique identifier: NCT01422616. The studies were conducted in accordance with the local legislation and institutional requirements. The participants provided their written informed consent to participate in this study.
Author contributions
XR: Writing – original draft. CC: Formal analysis, Writing – review & editing. YZ: Formal analysis, Writing – review & editing. QL: Data curation, Writing – review & editing. XW: Writing – review & editing. SY: Formal analysis, Writing – review & editing. MO: Formal analysis, Writing – review & editing. TR: Resources, Writing – review & editing. RL: Formal analysis, Writing – review & editing. HA: Supervision, Writing – review & editing. XC: Formal analysis, Writing – review & editing. JC: Resources, Writing – review & editing. CA: Supervision, Writing – review & editing. LS: Supervision, Writing – review & editing.
Funding
The author(s) declare financial support was received for the research, authorship, and/or publication of this article. The main funding for the ENCHANTED trial was from the National Health and Medical Research Council (NHMRC) of Australia. Additional funding was from the Stroke Association (United Kingdom), the National Council for Scientific and Technological Development (Brazil; CNPQ: 467322/2014-7, 402388/2013-5), and the Ministry for Health, Welfare and Family Affairs (Republic of Korea; HI14C1985).
Acknowledgments
The authors thank all the talented ENCHANTED investigators and coordinators.
Conflict of interest
The authors declare that the research was conducted in the absence of any commercial or financial relationships that could be construed as a potential conflict of interest.
Publisher’s note
All claims expressed in this article are solely those of the authors and do not necessarily represent those of their affiliated organizations, or those of the publisher, the editors and the reviewers. Any product that may be evaluated in this article, or claim that may be made by its manufacturer, is not guaranteed or endorsed by the publisher.
Supplementary material
The Supplementary Material for this article can be found online at: https://www.frontiersin.org/articles/10.3389/fendo.2024.1341902/full#supplementary-material
References
1. Institute for Health Metrics and Evaluation, University of Washington. GBD results. Available online at: https://vizhub.healthdata.org/gbd-results/ (Accessed 23 February 2023).
2. Toyoda K. Cerebrorenal interaction and stroke. Contrib Nephrol. (2013) 179:1–6. doi: 10.1159/000346944
3. Mahmoodi BK, Matsushita K, Woodward M, Woodward, Blankestijn PJ, Cirillo M, et al. Associations of kidney disease measures with mortality and end-stage renal disease in individuals with and without hypertension: a meta-analysis. Lancet. (2012) 380:1649–61. doi: 10.1016/s0140-6736(12)61272-0
4. Lee M, Saver JL, Chang KH, Liao HW, Chang SC, Ovbiagele B, et al. Low glomerular filtration rate and risk of stroke: meta-analysis. BMJ. (2010) 341:c4249. doi: 10.1136/bmj.c4249
5. Wang X, Wang Y, Wang C, Zhao X, Xian Y, Wang D, et al. Association between estimated glomerular filtration rate and clinical outcomes in patients with acute ischaemic stroke: results from China National Stroke Registry. Age Ageing. (2014) 43:839–45. doi: 10.1093/ageing/afu090
6. Hayden D, McCarthy C, Akijian L, Callaly E, Ní Chróinín D, Horgan G, et al. Renal dysfunction and chronic kidney disease in ischemic stroke and transient ischemic attack: A population-based study. Int J Stroke. (2017) 12:761–9. doi: 10.1177/1747493017701148
7. Hao Z, Yang C, Liu M, Liu M, Wu Bo. Renal dysfunction and thrombolytic therapy in patients with acute ischemic stroke: a systematic review and meta-analysis. Med (Baltimore). (2014) 93:e286. doi: 10.1097/MD.0000000000000286
8. Carr SJ, Wang X, Olavarria VV, Lavados PM, Rodriguez JA, Kim JS, et al. Influence of renal impairment on outcome for thrombolysis-treated acute ischemic stroke: ENCHANTED (Enhanced control of hypertension and thrombolysis stroke study) Post Hoc analysis. Stroke. (2017) 48:2605–9. doi: 10.1161/strokeaha.117.017808
9. Hsieh CY, Lin HJ, Sung SF, Hsieh HC, Lai EC, Chen CH. Is renal dysfunction associated with adverse stroke outcome after thrombolytic therapy? Cerebrovasc Dis. (2014) 37:51–6. doi: 10.1159/000356348
10. Agrawal V, Rai B, Fellows J, McCullough PA. In-hospital outcomes with thrombolytic therapy in patients with renal dysfunction presenting with acute ischaemic stroke. Nephrol Dialysis Transplant. (2010) 25:1150–7. doi: 10.1093/ndt/gfp619
11. Anderson CS, Huang Y, Lindley RI, Chen X, Arima H, Chen G, et al. Intensive blood pressure reduction with intravenous thrombolysis therapy for acute ischaemic stroke (ENCHANTED): an international, randomised, open-label, blinded-endpoint, phase 3 trial. Lancet. (2019) 393:877–88. doi: 10.1016/S0140-6736(19)31624-1
12. Fukuda-Doi M, Yamamoto H, Koga M, Doi Y, Qureshi AI, Yoshimura S, et al. Impact of renal impairment on intensive blood-pressure-lowering therapy and outcomes in intracerebral hemorrhage: results from ATACH-2. Neurology. (2021) 97:e913–21. doi: 10.1212/WNL.0000000000012442
13. Anderson CS, Robinson T, Lindley RI, Arima H, Lavados PM, Lee TH, et al. Low-dose versus standard-dose intravenous alteplase in acute ischemic stroke. N Engl J Med. (2016) 374:2313–23. doi: 10.1056/nejmc1801548
14. Levey AS, Stevens LA, Schmid CH, Zhang YL, Castro AF 3rd, Feldman HI, et al. A new equation to estimate glomerular filtration rate. Ann Intern Med. (2009) 150:604–12. doi: 10.7326/0003-4819-150-9-200905050-00006
15. Levey AS, Becker C, Inker LA. Glomerular filtration rate and albuminuria for detection and staging of acute and chronic kidney disease in adults: a systematic review. JAMA. (2015) 313:837–46. doi: 10.1001/jama.2015.0602
16. Wahlgren N, Ahmed N, Eriksson N, Aichner F, Bluhmki E, Dávalos A, et al. Multivariable analysis of outcome predictors and adjustment of main outcome results to baseline data profile in randomized controlled trials: Safe Implementation of Thrombolysis in Stroke-MOnitoring STudy (SITS-MOST). Stroke. (2008) 39:3316–22. doi: 10.1001/jama.2015.0602
17. Tsagalis G, Akrivos T, Alevizaki M, Manios E, Stamatellopoulos K, Laggouranis A, et al. Renal dysfunction in acute stroke: an independent predictor of long-term all combined vascular events and overall mortality. Nephrol Dial Transplant. (2009) 24:194–200. doi: 10.1093/ndt/gfn471
18. Ani C, Ovbiagele B. Relation of baseline presence and severity of renal disease to long-term mortality in persons with known stroke. J Neurol Sci. (2010) 288:123–8. doi: 10.1016/j.jns.2009.09.020
19. Fabjan TH, Hojs R. Ischemic stroke: the impact of renal dysfunction on 1-year mortality. Wien Klin Wochenschr. (2015) 127 Suppl 5:S175–180. doi: 10.1007/s00508-015-0705-y
20. Gensicke H, Zinkstok SM, Roos YB, Seiffge DJ, Ringleb P, Artto V, et al. IV thrombolysis and renal function. Neurology. (2013) 81:1780–8. doi: 10.1212/01.wnl.0000435550.83200.9e
21. Naganuma M, Koga M, Shiokawa Y, Nakagawara J, Furui E, Kimura K, et al. Reduced estimated glomerular filtration rate is associated with stroke outcome after intravenous rt-PA: the Stroke Acute Management with Urgent Risk-Factor Assessment and Improvement (SAMURAI) rt-PA registry. Cerebrovasc Dis. (2011) 31:123–9. doi: 10.1159/000321516
22. Lu R, Kiernan MC, Murray A, Rosner MH, Ronco C. Kidney-brain crosstalk in the acute and chronic setting. Nat Rev Nephrol. (2015) 11:707–19. doi: 10.1038/nrneph.2015.131
23. Hao Z, Wu B, Lin S, Kong FY, Tao WD, Wang DR, et al. Association between renal function and clinical outcome in patients with acute stroke. Eur Neurol. (2010) 63:237–42. doi: 10.1159/000285165
24. Kumai Y, Kamouchi M, Hata J, Ago T, Kitayama J, Nakane H, et al. Proteinuria and clinical outcomes after ischemic stroke. Neurology. (2012) 78:1909–15. doi: 10.1212/WNL.0b013e318259e110
25. Berger PB, Best PJ, Topol EJ, White J, DiBattiste PM, Chan AW, et al. The relation of renal function to ischemic and bleeding outcomes with 2 different glycoprotein IIb/IIIa inhibitors: The do Tirofiban and ReoPro Give Similar Ffficacy Outcome (TARGET) trial. Am Heart J. (2005) 149:869–75. doi: 10.1016/j.ahj.2004.12.002
26. Lutz J, Menke J, Sollinger D, Schinzel H, Thürmel K. Haemostasis in chronic kidney disease. Nephrol Dialysis Transplant January. (2014) 29:29–40. doi: 10.1093/ndt/gft209
27. Mezzano D, Pais EO, Aranda E, Panes O, Downey P, Ortiz M, et al. Inflammation, not hyperhomocysteinemia, is related to oxidative stress and hemostatic and endothelial dysfunction in uremia. Kidney Int. (2001) 60:844–1850. doi: 10.1046/j.1523-1755.2001.00998.x
28. Aguilera A, Sánchez-Tomero JA, Bajo MA, Ruiz-Caravaca ML, Alvarez V, del Peso G, et al. Malnutrition-inflammation syndrome is associated with endothelial dysfunction in peritoneal dialysis patients. Adv Perit Dial. (2003) 19:240–5.
29. Hamrahian SM, Falkner B. Hypertension in chronic kidney disease. Adv Exp Med Biol. (2017) 956:307–25. doi: 10.1007/5584_2016_84
30. Briet M, Boutouyrie P, Laurent S, London GM. Arterial stiffness and pulse pressure in CKD and ESRD. Kidney Int. (2012) 82:388–400. doi: 10.1038/ki.2012.131
31. Wright JT Jr, Agodoa L, Contreras G, Greene T, Douglas JG, Lash J, et al. Successful blood pressure control in the African American Study of Kidney Disease and Hypertension. Arch Intern Med. (2002) 162:1636–43. doi: 10.1001/archinte.162.14.1636
32. Stevens LA, Coresh J, Greene T, Levey AS. Assessing kidney function–measured and estimated glomerular filtration rate. N Engl J Med. (2006) 354:2473–83. doi: 10.1056/NEJMra054415
Keywords: renal function, thrombolysis, acute ischemic stroke, clinical outcome, blood pressure
Citation: Ren X, Chen C, Wang X, Li Q, Zhao Y, You S, Ouyang M, Robinson T, Lindley RI, Arima H, Chen X, Chalmers J, Anderson CS and Song L (2024) Influence of renal function on blood pressure control and outcome in thrombolyzed patients after acute ischemic stroke: post-hoc analysis of the ENCHANTED trial. Front. Endocrinol. 15:1341902. doi: 10.3389/fendo.2024.1341902
Received: 22 November 2023; Accepted: 11 November 2024;
Published: 09 December 2024.
Edited by:
Houwei Du, Fujian Medical University Union Hospital, ChinaReviewed by:
Eileen Hennrikus, Penn State Milton S. Hershey Medical Center, United StatesYajun Cheng, West China Hospital of Sichuan University, China
Copyright © 2024 Ren, Chen, Wang, Li, Zhao, You, Ouyang, Robinson, Lindley, Arima, Chen, Chalmers, Anderson and Song. This is an open-access article distributed under the terms of the Creative Commons Attribution License (CC BY). The use, distribution or reproduction in other forums is permitted, provided the original author(s) and the copyright owner(s) are credited and that the original publication in this journal is cited, in accordance with accepted academic practice. No use, distribution or reproduction is permitted which does not comply with these terms.
*Correspondence: Craig S. Anderson, Y2FuZGVyc29uQGdlb3JnZWluc3RpdHV0ZS5vcmcuYXU=; Lili Song, bHNvbmdAZ2VvcmdlaW5zdGl0dXRlLm9yZy5jbg==
†Present addresses: Craig S. Anderson, Institute of Science and Technology for Brain-Inspired Intelligence, Fudan University, Shanghai, China
The George Institute for Global Health, University of New South Wales, Sydney, NSW, Australia
Neurology Department, Royal Prince Alfred Hospital, Sydney, NSW, Australia
Key Laboratory of Computational Neuroscience and Brain-Inspired Intelligence (Fudan University), Ministry of Education, Shanghai, China
Lili Song, Institute of Science and Technology for Brain-Inspired Intelligence, Fudan University, Shanghai, China
The George Institute for Global Health, University of New South Wales, Sydney, NSW, Australia
Key Laboratory of Computational Neuroscience and Brain-Inspired Intelligence (Fudan University), Ministry of Education, Shanghai, China