- 1Department of Radiology, Yueyang Hospital of Integrated Traditional Chinese and Western Medicine, Shanghai University of Traditional Chinese Medicine, Shanghai, China
- 2Department of Clinical Laboratory, Shanghai Fourth People’s Hospital, School of Medicine, Tongji University, Shanghai, China
- 3Department of Gastroenterology, Yueyang Hospital of Integrated Traditional Chinese and Western Medicine, Shanghai University of Traditional Chinese Medicine, Shanghai, China
Objective: To investigate the relationship between circulating receptor activator of nuclear factor-kappa B ligand (RANKL) levels and marrow adipose tissue in postmenopausal females.
Methods: A total of 164 postmenopausal females were included in the study. Serum levels of osteoprotegerin (OPG) and RANKL were measured using ELISA kits. Body composition and bone mineral density (BMD) were assessed using dual-energy X-ray absorptiometry. Complex-based chemical shift imaging-based MRI was employed to evaluate the vertebral marrow proton density fat fraction (PDFF). A multivariate linear regression model was utilized to analyze the predictive effects of PDFF and BMD on circulating levels of OPG and RANKL.
Results: Simple regression analysis showed significant associations among the marrow PDFF, BMD at either site, serum RANKL, and the RANKL/OPG ratio. In multivariate linear regression models, marrow PDFF was found to have a positive correlation (β = 3.15, 95% CI 2.60 to 3.70) and BMD had negative correlations (β = −0.200, 95% CI −0.348 to −0.051 for vertebral BMD; β = −0.383, 95% CI −0.589 to −0.177 for total hip BMD; and β =−0.393, 95% CI −0.598 to −0.188 for femoral neck BMD, all p < 0.01) with circulating soluble RANKL levels after adjusting for age, body mass index, physical activity, total fat mass, android/gynoid ratio, and lean mass. Similar results were observed for the RANKL/OPG ratio. Additionally, multivariate linear regression analyses revealed that marrow PDFF was a significant independent contributor of circulating soluble RANKL (β = 1.34, 95% CI 1.10 to 1.58, p < 0.001) after further controlling for BMD. However, marrow PDFF or BMD had no associations with circulating levels of OPG after adjusting for all potential confounders mentioned above.
Conclusions: Vertebral marrow fat fraction is independently associated with circulating soluble RANKL levels in postmenopausal females.
1 Introduction
Receptor activator of nuclear factor-kappa B ligand (RANKL) and its cognate receptor, activator of nuclear factor kappa B (RANK), are crucial for the differentiation, survival, and activity of osteoclasts (1). RANKL, expressed by osteoblasts and osteocytes, binds to RANK on the membrane of osteoclast progenitors. Besides osteoblasts and osteocytes, other cells such as immune cells and hypertrophic chondrocytes also produce RANKL (2). Osteoprotegerin (OPG) is a soluble decoy receptor for RANKL that inhibits the RANKL-RANK interaction, thereby mediating osteoclastogenesis. The RANK/RANKL/OPG signaling pathways are vital for bone resorption (3). Therefore, measuring the levels of RANKL, OPG, and their ratio can provide insights into bone formation and resorption.
Previous studies have shown that circulating levels of soluble RANKL are elevated in animal models of menopause (4, 5) and in mice with postmenopausal obesity induced by ovariectomy and subsequent high-fat and high-sucrose diet feeding (1). Several observational studies have attempted to determine the relationships between circulating RANKL levels, OPG levels, the RANKL/OPG ratio, and bone mineral density (BMD) (6–11), but the results have been inconsistent. Bone marrow adipose tissue, which constitutes more than 10% of total body fat, has been implicated in various physiological and pathological conditions, including osteoporosis, diabetes, and certain cancers (12–14). Aging is associated with a decline in BMD and a significant increase in marrow adipose tissue. Increased marrow adiposity is linked to decreased osteoblast numbers and bone formation, increased bone resorption, and osteoporotic pathologies characterized by altered bone structure, reduced bone mass, and increased fracture risk (15–17). In animal models, enhanced bone resorption in early-stage type I diabetes has been attributed to RANKL derived from marrow fat rather than from bone tissue itself (18). Recent ex vivo and animal studies have also suggested that adipose lineage cells within the bone marrow express RANKL (19–22). Therefore, bone marrow adipose tissue may be an important factor influencing soluble RANKL levels.
However, little is known about the relationships between bone marrow fat content, serum soluble RANKL levels, and the RANKL/OPG ratio in humans. This is a significant knowledge gap because the interactions between bone marrow fat and the RANKL/OPG system in bone balance are not well understood. Hence, the aim of our study was to investigate the associations between circulating soluble RANKL levels, OPG levels, and the RANKL/OPG ratio with body composition and marrow adipose tissue, as assessed by dual-energy X-ray absorptiometry and complex-based chemical shift imaging-based MRI, in postmenopausal females.
2 Methods
2.1 Study participants
The study, conducted between March 2019 and June 2023, recruited 176 postmenopausal females aged 50 to 87 from the community. Participants met the following inclusion criteria: they were ambulatory, aged over 50, had experienced menopause at least one year prior (defined as amenorrhea for at least 12 months after the last menstrual period) (12), and were capable of providing informed consent. The exclusion criteria were comprehensive (1): Presence of other metabolic or inherited bone diseases, such as Paget’s disease, hyperparathyroidism, hypoparathyroidism, or vitamin D deficiency (2); Endocrine disorders impacting bone or fat metabolism, including hyperthyroidism and diabetes mellitus (3); Cardiovascular diseases, chronic liver or kidney diseases, chronic lung diseases, history of malignancy, autoimmune diseases, rheumatic inflammatory diseases, and depressive disorders (4); Current or past use of medications affecting bone marrow metabolism, such as glucocorticoids, bisphosphonates, aromatase inhibitors, anticonvulsant therapy, hormone replacement therapy, and lipid-lowering agents (5); Neurological diseases or cerebrovascular accident sequelae affecting the musculoskeletal system (6); Vitamin D supplementation exceeding 1200 IU daily, alcohol consumption, current smoking, or a body mass index (BMI) outside the range of 19-35 kg/m². Twelve participants were excluded due to metastasis (n = 1), hyperglycemia (n = 5), and silent vertebral body fracture (n = 6), resulting in a final sample of 164 participants.
A standardized physical examination was conducted for all participants, with data recorded on age, menopausal status, body weight, height, drinking habits, alcohol consumption, smoking history, and physical activity. BMI was calculated from body weight and height. Physical activity was defined as moderate activity occurring at least three times per week for at least 30 minutes (23). The research protocol was approved by the institute’s Ethics Committee (no. 2018-075), adhering to the ethical principles of the Declaration of Helsinki and its amendments. Written informed consent was obtained from all participants.
2.2 Laboratory analyses
Morning blood samples for laboratory analysis were collected from all participants between 7:00 and 10:00 AM after an 8-hour overnight fast. Lipid profiles (total cholesterol, triglycerides, high-density lipoprotein cholesterol, and low-density lipoprotein cholesterol) and plasma fasting glucose were analyzed using a chemiluminescence immunoassay system. Enzymatic methods were employed to measure serum calcium, phosphorus, creatinine and uric acid levels.
The estimated glomerular filtration rate was calculated utilizing the Chronic Kidney Disease Epidemiology Collaboration equation. Serum levels of bone turnover biomarkers, including 25-hydroxyvitamin D, parathyroid hormone, β-type I collagen telopeptides, osteocalcin, and N-terminal propeptide of type 1 procollagen, were determined using an electrochemiluminescence immunoassay system (Cobas 8000 e801; Roche Diagnostics, Basel, Switzerland). The intra-assay and inter-assay coefficients of variation (CVs) for these biomarkers ranged from 3.2% to 7.0%. Serum levels of RANKL and OPG were measured by enzyme-linked immunosorbent assay (MULTISCIENCES, Hangzhou, China), with values obtained from standard curves in duplicate according to the manufacturer’s instructions. The intra-assay and inter-assay CVs for soluble RANKL and OPG were less than 8.6% and 7.7%, respectively.
2.3 BMD and body composition measurements
Dual-energy X-ray absorptiometry (Prodigy Lunar; GE Healthcare, Waukesha, WI) was used to determine total and regional bone and soft tissue compositions, including total fat mass, total lean mass, and android and gynoid fat amounts. BMD (g/cm²) was measured at the total hip, femoral neck, and lumbar spine (L1-L4). The CVs for BMD measurements were less than 1.3% for the lumbar spine, 1.8% for the femoral neck, and 1.4% for the total hip. Additionally, the CVs for total lean mass and fat mass were 0.73% and 1.0%, respectively.
2.4 MR image acquisition and data analysis
All imaging was performed on a clinical 3.0-T whole-body MR imager (uMR 780, United Imaging Healthcare). All participants underwent MRI examination of the lumbar spine on the same day as the dual-energy X-ray absorptiometry scan. The interval between laboratory analysis and image acquisitions was within one week. To identify pre-existing abnormalities of the lumbar vertebrae, routine MR imaging of the lumbar spine was performed using sagittal T1-weighted and T2-weighted sequences, as well as a transverse T2-weighted sequence.
To determine the percentage PDFF, which is the ratio of fat to the sum of water and fat, a commercially available 3D spoiled gradient echo pulse sequence called Fat Analysis & Calculation Technique (FACT) was used. The sequence had equidistant echo spacing of 1.1 ms, with the first TE at 1.21 ms. The TR was set to the shortest possible duration for the 6-echo FACT, which is 7.2 ms. A low flip angle of 3° was applied to minimize T1 saturation effects, and a multipeak fat model was used to address the spectral complexity of fat (24). Parallel imaging was employed with a SENSE factor of 2 in the anterior-posterior direction. The total scan time was 17.5 seconds, completed in a single breath-hold. Additional imaging parameters included a receiver bandwidth of 1.3 kHz, a field of view of 40 cm, an acquisition matrix size of 256 x 192, a slice thickness of 3 mm, no interslice gap, and 1 average.
FACT images were transferred to the uWS-MR Advanced Postprocess Workstation (United Imaging Healthcare), where the parametric PDFF maps were automatically generated. The CV of PDFF measured by FACT in the lumbar spine of ten subjects, who were scanned twice on the same day after repositioning, was 2.8%.
2.5 Statistical analysis
Statistical analysis was performed using SPSS (version 27.0; Chicago, IL, USA) and GraphPad Prism 10. Results were expressed as mean ± standard deviation (SD) for continuous variables, median (interquartile range [IQR]) for non-normally distributed continuous variables, and n (%) for categorical variables. The normality of distributions was assessed using the Shapiro-Wilk test. Trends in marrow PDFF across soluble RANKL, OPG, and their ratio quartiles were tested using a polynomial test. Least square mean values of marrow PDFF were compared across quartiles of circulating RANKL, OPG, or the RANKL/OPG ratio, controlling for age, BMI, physical activity (moderate intensity), total fat mass, android/gynoid ratio, and lean mass. Multiple linear regression analysis was conducted to test for linear trends. Pearson’s and Spearman’s correlations were used to investigate bivariate associations between variables for normally and non-normally distributed data, respectively. A multivariate linear regression model was used to investigate the independent effect of BMD (at the lumbar spine, total hip, and femoral neck) on circulating RANKL, OPG, or the RANKL/OPG ratio, adjusting for the potential covariates mentioned above. A similar analysis was conducted to explore the correlation between marrow PDFF and serum RANKL, OPG, and the RANKL/OPG ratio. To avoid multicollinearity in the regression model, tolerance and variance inflation factor (VIF) were evaluated. A tolerance value less than 0.3 and a variance inflation factor value above 2 indicated potential issues with the model (25). The level of significance was set at 0.05.
3 Results
3.1 Characteristics of the study participants
The demographic data, BMD, body composition, marrow PDFF, and serological characteristics of the study participants are summarized in Table 1. The quartiles for circulating RANKL levels in the cohort were as follows: Quartile 1, <112 pg/mL; Quartile 2, 112–157 pg/mL; Quartile 3, 158–196 pg/mL; and Quartile 4, >196 pg/mL. After adjusting for age, BMI, physical activity (moderate intensity), total fat mass, android/gynoid ratio, and lean mass, the least square mean of marrow PDFF was compared across these quartile groups. As shown in Figure 1, a significant trend in marrow PDFF was observed across the quartiles of circulating RANKL. Similar trends were observed for the RANKL/OPG ratio, but not OPG levels.
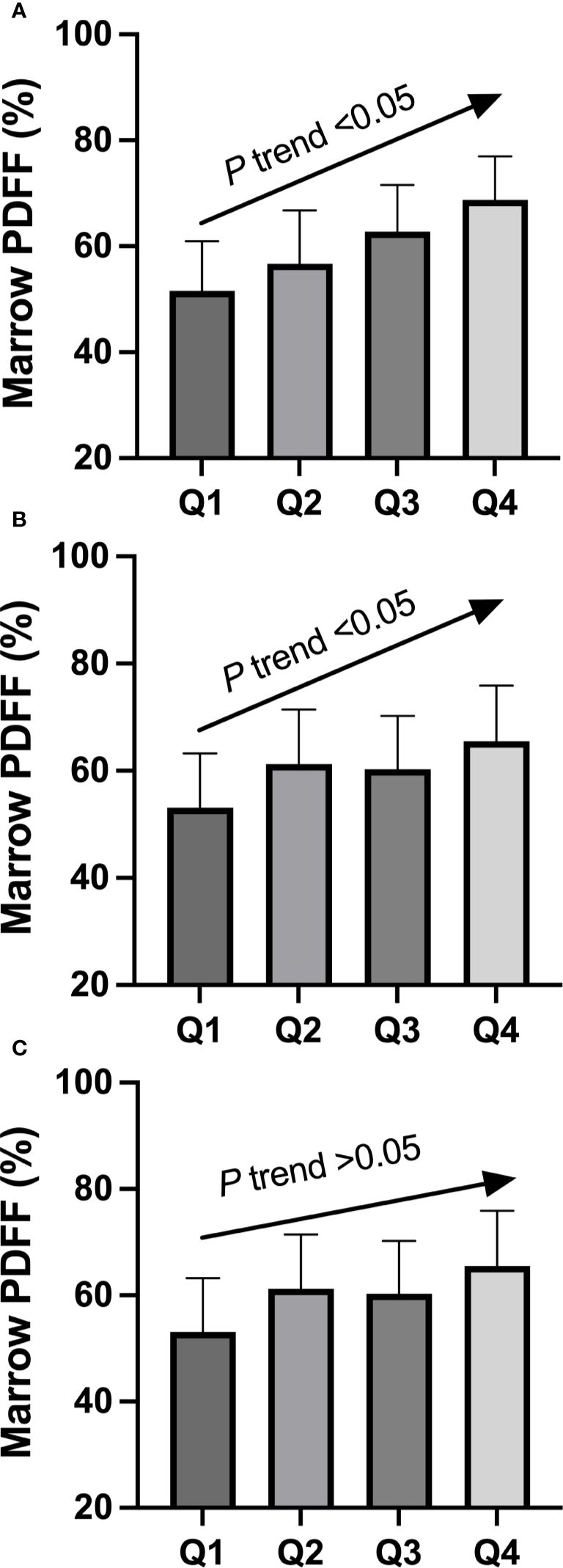
Figure 1. Adjusted mean value of circulating RANKL (A), RANKL/OPG ratio (B), and OPG (C) in participants classified according to quartile groups (Q1–Q4) of marrow fat fraction. Mean values were adjusted for age, BMI, physical activity (moderate intensity), total fat mass, android/gynoid ratio, and lean mass. There was a significant linear trend between marrow fat fraction and circulating RANKL quartile groups, as well as between marrow fat fraction and RANKL/OPG ratio quartile groups. OPG, osteoprotegerin; Q, quartile; RANKL, receptor activator of nuclear factor-kappa B ligand.
3.2 Bivariate correlations of circulating RANKL, OPG levels, and their ratio with BMD and marrow PDFF
Age showed a positive correlation with marrow PDFF (r = 0.436, p <0.001), suggesting it could be a potential confounder. A suggestive correlation was observed between marrow PDFF and β-type I collagen telopeptides (r = 0.142, p =0.070), but no significant correlations were found with the N-terminal propeptide of type I procollagen (r = 0.063, p =0.427), parathyroid hormone (r = 0.071, p =0.369), and osteocalcin (r = 0.122, p =0.120).
The correlations between serum RANKL, OPG, RANKL/OPG ratio, and the parameters of interest are shown in Table 2. In terms of BMD, we found an inverse correlation between marrow PDFF and BMD at the lumbar spine (r = −0.462), total hip (r = −0.317), and femoral neck (r = −0.305, all p < 0.001). Serum RANKL levels and RANKL/OPG ratio were found to be negatively correlated with BMD at the lumbar spine, total hip, and femoral neck, and positively associated with marrow PDFF (all p < 0.05) (Figure 2). However, circulating OPG levels were not associated with marrow PDFF or BMD at any skeletal site, except for a mild positive correlation between circulating OPG and lumbar spine BMD.
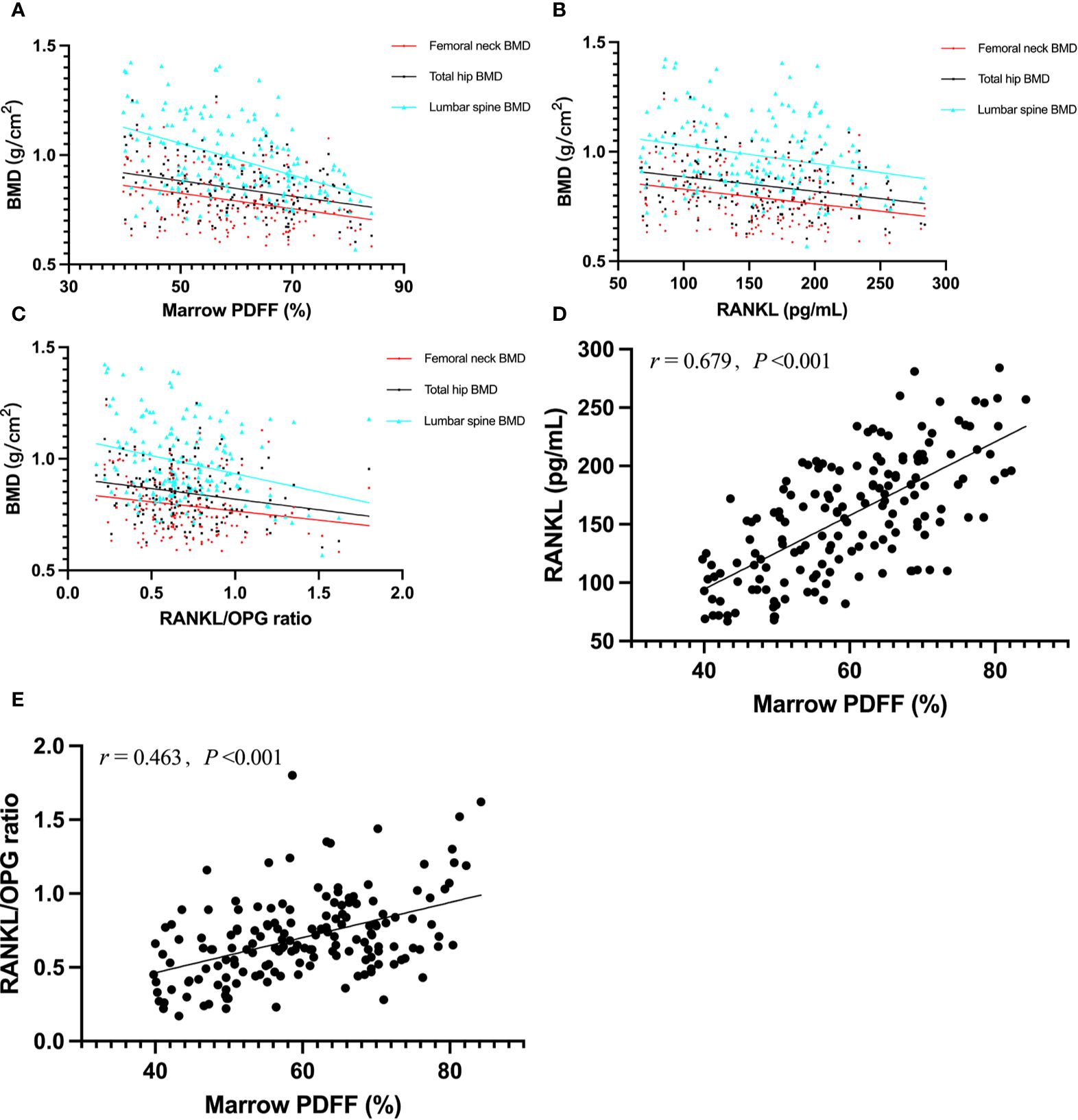
Figure 2. Graphic representation of correlations between marrow PDFF and BMD (A); r = –0.462 for lumbar sipne, r = –0.317 for total hip and r = –0.305 for femoral neck), serum RANKL and BMD (B); r = –0.246 for lumbar sipne, r = –0.278 for total hip and r = –0.269 for femoral neck), RANKL/OPG ratio and BMD (C); r = –0.266 for lumbar sipne, r = –0.221 for total hip and r = –0.183 for femoral neck), marrow PDFF and serum RANKL (D), and marrow PDFF and RANKL/OPG ratio (E). BMD, bone mineral density; PDFF, proton density fat fraction; OPG, osteoprotegerin; RANKL, receptor activator of nuclear factor-kappa B ligand.
3.3 Vertebral marrow fat fraction is independently associated with circulating soluble RANKL levels
Multiple regression analysis was performed to assess the influences of BMD and PDFF on serum RANKL, OPG, and the RANKL/OPG ratio, with results presented in Table 3. The analysis showed that marrow PDFF and BMD were significant contributing factors to serum soluble RANKL levels (β = 3.15, 95% CI 2.60 to 3.70 for PDFF; β = −0.200, 95% CI −0.348 to −0.051 for vertebral BMD; β = −0.383, 95% CI −0.589 to −0.177 for total hip BMD; and β =−0.393, 95% CI −0.598 to −0.188 for femoral neck BMD, all p < 0.01) in postmenopausal females, after adjusting for age, BMI, physical activity (moderate intensity), total fat mass, android/gynoid ratio, and lean mass. This association remained significant for marrow PDFF even after additional adjustment for BMD (β = 1.34, 95% CI 1.10 to 1.58, p < 0.001). Similar results were obtained for the RANKL/OPG ratio. Conversely, marrow PDFF and BMD did not significantly affect OPG levels (Table 3).
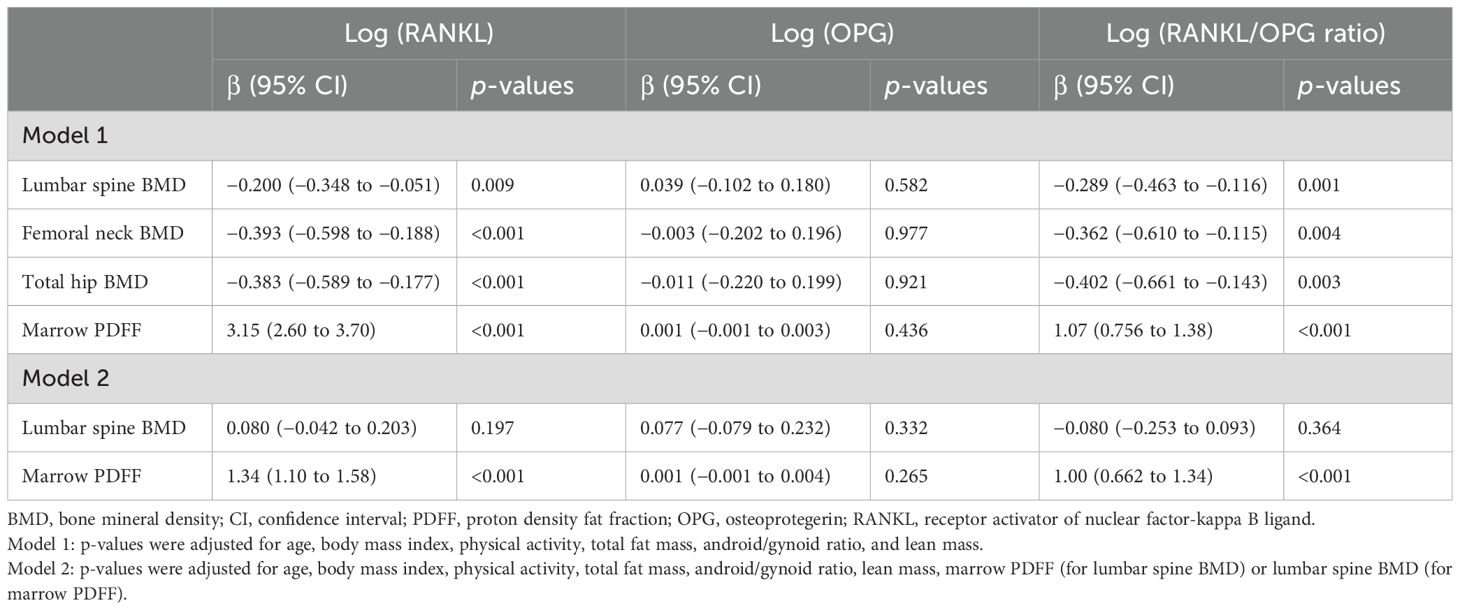
Table 3. Multiple linear regression analysis: Marrow PDFF, BMD at the lumber spine, total hip, and femoral neck, respectively as dependent parameters.
4 Discussion
In this study, multiple regression analysis was used to assess the influence of BMD at various skeletal sites on RANKL, OPG, and the RANKL/OPG ratio. The fingings revealed that BMD significantly impacted circulating soluble RANKL but not OPG in postmenopausal females, which aligns partially with existing literature. Previous research has shown inconsistent effects of circulating RANKL and OPG on serum markers of bone turnover and BMD. For instance, some studies have reported elevated levels of soluble RANKL in ovariectomized mice (1), whereas others found no difference in RANKL levels between premenopausal and postmenopausal females, despite higher RANKL expression in mesenchymal stem cells of postmenopausal females (9), or between individuals with normal and low bone mass (8). Additionally, some studies have indicated a significant negative association between serum RANKL and BMD (11). Similarly, the relationship between circulating OPG levels and BMD has shown mixed results in various studies of postmenopausal females (6–8, 10, 11, 26). Interestingly, Nabipour et al. (10) found that serum levels of RANKL and OPG were independent determinants of BMD. However, Mezquita-Raya et al. (26) reported that OPG, but not RANKL, correlated with vertebral fractures and osteoporosis in postmenopausal females. In that study, serum RANKL concentrations were undetectable in a substantial proportion of women (54.9%). Among the detectable levels, most were exceedingly low, likely representing assay noise. Eghbali-Fatourechi et al. (9) corroborated our findings, suggesting that the upregulation of RANKL on bone marrow cells is a crucial factor in the increased bone resorption associated with estrogen deficiency. These discrepancies may be attributed to differences in study design, sample size, methodology, statistical analysis, and other unknown factors. They might also suggest that serum RANKL and OPG are not reflective of the biological effects of these molecules at the tissue level.
The age-related increase in fatty marrow typically surpasses the reduction in cellular marrow, likely because fatty marrow progressively replaces both hematopoietic tissue and bone (27). Recent studies have highlighted the role of marrow adipose tissue in regulating energy metabolism and bone homeostasis. Excessive fat content in the marrow is associated with metabolic dysfunction, disrupting the balance among osteoblast, osteoclast, and adipocyte activity that maintains bone mass. Increased marrow fat content often correlates with decreased bone mass, as observed in previous aging studies (12, 28) and confirmed in our study of postmenopausal females. In our study, no significant correlation was found between vertebral marrow PDFF and bone turnover biomarkers, such as parathyroid hormone, β-type I collagen telopeptides, osteocalcin, and the N-terminal propeptide of type 1 procollagen. These results align with earlier studies indicating that vitamin D, calcium, and parathyroid hormone levels do not correlate with vertebral marrow fat content in postmenopausal women with type 2 diabetes mellitus, patients with chronic kidney disease, or healthy controls (29–31).
Regarding marrow adipose tissue, the relationship between circulating soluble RANKL and marrow fat content aligned with our expectations. One key finding was that marrow adipose tissue was a determinant of variation in circulating soluble RANKL in postmenopausal females. This suggests that the secretion of soluble RANKL is, to some extent, specific to marrow adipose tissue and that serum concentrations may reflect intracellular concentrations or activity within the bone microenvironment. Increased marrow adipose tissue is often accompanied by bone mass loss due to elevated bone resorption. A prior study analyzed RANKL gene expressions in peripheral fat depots, including inguinal, epididymal, and interscapular adipose depots. Compared to marrow fat tissue, RANKL expression was virtually undetectable in these peripheral adipose depots (32), indicating the capability of marrow fat tissue, but not other adipose depots, to secrete RANKL. Primary human bone marrow adipocytes play a favorable role in osteoclast differentiation and function by expressing the pro-osteoclastogenic factor RANKL (33). In murine studies, aging significantly increased stromal/osteoblastic cell-induced osteoclastogenesis. Marrow adipocytes with osteogenic and adipogenic features can secrete RANKL and impact bone resorption. During marrow adipogenesis, RANKL expression was induced through the action of C/EBPβ and/or C/EBPδ, and RANKL-positive pre-adipocytes increased in the bone marrow of aged mice, concomitantly with a downregulation of osteoprotegerin (22). Interestingly, the lack of soluble RANKL did not influence bone loss caused by estrogen deficiency (4).
This study has several limitations. Firstly, the cross-sectional design limits our ability to establish direct causal relationships between variables. Secondly, the findings may not be generalizable to younger age groups or men, as the study focused solely on postmenopausal females. Thirdly, despite the statistical analysis accounting for numerous covariates, certain unconsidered factors, such as dietary influences or comorbidities, cannot be completely ruled out. Lastly, the measurement of circulating RANKL levels may not fully reflect tissue levels. Further investigation is needed to determine the direct link between marrow fat content and soluble RANKL using experimental studies.
5 Conclusion
In this observed sample, marrow fat content was negatively correlated with BMD at various skeletal sites in postmenopausal females, and marrow adipose tissue was identified as a determinant of variation in circulating soluble RANKL. The potential direct link between marrow fat content and soluble RANKL should be further explored through experimental studies. Additional research is necessary to clarify whether the increased bone resorption observed in pathological conditions like osteoporosis is driven by RANKL originating from marrow adipose tissue rather than from the bone tissue itself.
Data availability statement
The raw data supporting the conclusions of this article will be made available by the authors, without undue reservation.
Ethics statement
The studies involving humans were approved by Institutional Review Board of Yueyang Hospital of Integrated Traditional Chinese and Western Medicine. The studies were conducted in accordance with the local legislation and institutional requirements. Written informed consent for participation in this study was provided by the participants’ legal guardians/next of kin.
Author contributions
XL: Writing – original draft, Writing – review & editing, Investigation. XZ: Writing – original draft, Writing – review & editing, Methodology. LL: Methodology, Writing – original draft, Writing – review & editing, Software. RX: Writing – original draft, Writing – review & editing, Data curation, Formal analysis. YinW: Formal analysis, Writing – original draft, Writing – review & editing. SC: Writing – original draft, Writing – review & editing. YW: Conceptualization, Formal analysis, Writing – review & editing, Writing – original draft. PL: Formal analysis, Writing – original draft, Writing – review & editing, Conceptualization, Validation. GL: Validation, Writing – review & editing, Conceptualization, Supervision, Writing – original draft.
Funding
The author(s) declare financial support was received for the research, authorship, and/or publication of this article. This study was funded by the National Natural Science Foundation of China (82474604).
Acknowledgments
The authors would like to thank Drs. Bin Wang and Rongrong Huang for their invaluable assistance with data collection and technical support.
Conflict of interest
The authors declare that the research was conducted in the absence of any commercial or financial relationships that could be construed as a potential conflict of interest.
Publisher’s note
All claims expressed in this article are solely those of the authors and do not necessarily represent those of their affiliated organizations, or those of the publisher, the editors and the reviewers. Any product that may be evaluated in this article, or claim that may be made by its manufacturer, is not guaranteed or endorsed by the publisher.
Abbreviations
BMD, bone mineral density; BMI, body mass index; CV, coefficient of variation; OPG, osteoprotegerin; PDFF, proton density fat fraction; RANKL, receptor activator of nuclear factor-kappa B ligand; ROI, regions of interest; SD, standard deviation.
References
1. Mori K, Mizokami A, Sano T, Mukai S, Hiura F, Ayukawa Y, et al. RANKL elevation activates the NIK/NF-kappaB pathway, inducing obesity in ovariectomized mice. J Endocrinol. (2022) 254:27–36. doi: 10.1530/JOE-21-0424
2. Ono T, Hayashi M, Sasaki F, Nakashima T. RANKL biology: bone metabolism, the immune system, and beyond. Inflammation Regener. (2020) 40:2. doi: 10.1186/s41232-019-0111-3
3. Hussein R, Aboukhamis I. The association of serum RANKL levels with disease activity and hematological parameters in Syrian patients with rheumatoid arthritis. Biochem Biophys Rep. (2022) 32:101373. doi: 10.1016/j.bbrep.2022.101373
4. Xiong J, Cawley K, Piemontese M, Fujiwara Y, Zhao H, Goellner JJ, et al. Soluble RANKL contributes to osteoclast formation in adult mice but not ovariectomy-induced bone loss. Nat Commun. (2018) 9:2909. doi: 10.1038/s41467-018-05244-y
5. Wen B, Zhao L, Zhao H, Wang X. Liraglutide exerts a bone-protective effect in ovariectomized rats with streptozotocin-induced diabetes by inhibiting osteoclastogenesis. Exp Ther Med. (2018) 15:5077–83. doi: 10.3892/etm.2018.6043
6. Rogers A, Saleh G, Hannon RA, Greenfield D, Eastell R. Circulating estradiol and osteoprotegerin as determinants of bone turnover and bone density in postmenopausal women. J Clin Endocrinol Metab. (2002) 87:4470–5. doi: 10.1210/jc.2002-020396
7. Kudlacek S, Schneider B, Woloszczuk W, Pietschmann P, Willvonseder R. Serum levels of osteoprotegerin increase with age in a healthy adult population. Bone. (2003) 32:681–6. doi: 10.1016/s8756-3282(03)00090-5
8. Amer OE, Wani K, Ansari MGA, Alnaami AM, Aljohani N, Abdi S, et al. Associations of bone mineral density with RANKL and osteoprotegerin in arab postmenopausal women: A cross-sectional study. Medicina (Kaunas). (2022) 58(8):976. doi: 10.3390/medicina58080976
9. Eghbali-Fatourechi G, Khosla S, Sanyal A, Boyle WJ, Lacey DL, Riggs BL. Role of RANK ligand in mediating increased bone resorption in early postmenopausal women. J Clin Invest. (2003) 111:1221–30. doi: 10.1172/JCI17215
10. Nabipour I, Larijani B, Vahdat K, Assadi M, Jafari SM, Ahmadi E, et al. Relationships among serum receptor of nuclear factor-kappaB ligand, osteoprotegerin, high-sensitivity C-reactive protein, and bone mineral density in postmenopausal women: osteoimmunity versus osteoinflammatory. Menopause. (2009) 16:950–5. doi: 10.1097/gme.0b013e3181a181b8
11. Stern A, Laughlin GA, Bergstrom J, Barrett-Connor E. The sex-specific association of serum osteoprotegerin and receptor activator of nuclear factor kappaB legend with bone mineral density in older adults: the Rancho Bernardo study. Eur J Endocrinol. (2007) 156:555–62. doi: 10.1530/EJE-06-0753
12. Li G, Xu Z, Li X, Zuo X, Chang S, Wu D, et al. Adding marrow R2 * to proton density fat fraction improves the discrimination of osteopenia and osteoporosis in postmenopausal women assessed with 3D FACT sequence. Menopause. (2021) 28:800–6. doi: 10.1097/GME.0000000000001799
13. Mukherjee A, Chiang CY, Daifotis HA, Nieman KM, Fahrmann JF, Lastra RR, et al. Adipocyte-induced FABP4 expression in ovarian cancer cells promotes metastasis and mediates carboplatin resistance. Cancer Res. (2020) 80:1748–61. doi: 10.1158/0008-5472.CAN-19-1999
14. Shu JB, Kim TY. Bone marrow adiposity in diabetes and clinical interventions. Curr Opin Endocrinol Diabetes Obes. (2022) 29:303–9. doi: 10.1097/MED.0000000000000741
15. Watt J, Schlezinger JJ. Structurally-diverse, PPARgamma-activating environmental toxicants induce adipogenesis and suppress osteogenesis in bone marrow mesenchymal stromal cells. Toxicology. (2015) 331:66–77. doi: 10.1016/j.tox.2015.03.006
16. Li G, Xu Z, Hou L, Li X, Li X, Yuan W, et al. Differential effects of bisphenol A diglicydyl ether on bone quality and marrow adiposity in ovary-intact and ovariectomized rats. Am J Physiol Endocrinol Metab. (2016) 311:E922–7. doi: 10.1152/ajpendo.00267.2016
17. Chen L, Ma R, Luo P, Shi D, Shi X, Nian H, et al. Effects of total flavonoids of epimedium on bone marrow adipose tissue in ovariectomized rats. Front Endocrinol (Lausanne). (2022) 13:900816. doi: 10.3389/fendo.2022.900816
18. Yang J, Chen S, Zong Z, Yang L, Liu D, Bao Q, et al. The increase in bone resorption in early-stage type I diabetic mice is induced by RANKL secreted by increased bone marrow adipocytes. Biochem Biophys Res Commun. (2020) 525:433–9. doi: 10.1016/j.bbrc.2020.02.079
19. Yu W, Zhong L, Yao L, Wei Y, Gui T, Li Z, et al. Bone marrow adipogenic lineage precursors promote osteoclastogenesis in bone remodeling and pathologic bone loss. J Clin Invest. (2021) 131(2):e140214. doi: 10.1172/JCI140214
20. Hu Y, Li X, Zhi X, Cong W, Huang B, Chen H, et al. RANKL from bone marrow adipose lineage cells promotes osteoclast formation and bone loss. EMBO Rep. (2021) 22:e52481. doi: 10.15252/embr.202152481
21. Goto H, Osaki M, Fukushima T, Sakamoto K, Hozumi A, Baba H, et al. Human bone marrow adipocytes support dexamethasone-induced osteoclast differentiation and function through RANKL expression. BioMed Res. (2011) 32:37–44. doi: 10.2220/biomedres.32.37
22. Takeshita S, Fumoto T, Naoe Y, Ikeda K. Age-related marrow adipogenesis is linked to increased expression of RANKL. J Biol Chem. (2014) 289:16699–710. doi: 10.1074/jbc.M114.547919
23. Ahn SH, Seo DH, Kim SH, Nam MS, Hong S. The relationship between fatty liver index and bone mineral density in Koreans: KNHANES 2010-2011. Osteoporos Int. (2018) 29:181–90. doi: 10.1007/s00198-017-4257-z
24. Li G, Xu Z, Wang Y, Jiang L, Chang S, Yuan W, et al. Association of serum ferritin with marrow iron concentration using a three-dimension fat analysis & calculation technique sequence in postmenopausal women. J Comput Assist Tomogr. (2022) 46:464–9. doi: 10.1097/RCT.0000000000001296
25. Shao HD, Li GW, Liu Y, Qiu YY, Yao JH, Tang GY. Contributions of fat mass and fat distribution to hip bone strength in healthy postmenopausal Chinese women. J Bone Miner Metab. (2015) 33:507–15. doi: 10.1007/s00774-014-0613-7
26. Mezquita-Raya P, de la Higuera M, Garcia DF, Alonso G, Ruiz-Requena ME, de Dios Luna J, et al. The contribution of serum osteoprotegerin to bone mass and vertebral fractures in postmenopausal women. Osteoporos Int. (2005) 16:1368–74. doi: 10.1007/s00198-005-1844-1
27. Roldan-Valadez E, Piña-Jimenez C, Favila R, Rios C. Gender and age groups interactions in the quantification of bone marrow fat content in lumbar spine using 3T MR spectroscopy: a multivariate analysis of covariance (Mancova). Eur J Radiol. (2013) 82:e697–702. doi: 10.1016/j.ejrad.2013.07.012
28. Woods GN, Ewing SK, Schafer AL, Gudnason V, Sigurdsson S, Lang T, et al. Saturated and unsaturated bone marrow lipids have distinct effects on bone density and fracture risk in older adults. J Bone Miner Res. (2022) 37:700–10. doi: 10.1002/jbmr.4504
29. Moorthi RN, Fadel W, Eckert GJ, Ponsler-Sipes K, Moe SM, Lin C. Bone marrow fat is increased in chronic kidney disease by magnetic resonance spectroscopy. Osteoporos Int. (2015) 26:1801–7. doi: 10.1007/s00198-015-3064-7
30. Borelli C, Vergara D, Guglielmi R, Aucella F, Testini V, Guglielmi G. Assessment of bone marrow fat by 3-Tesla magnetic resonance spectroscopy in patients with chronic kidney disease. Quant Imaging Med Surg. (2023) 13:7432–43. doi: 10.21037/qims-23-530
31. Baum T, Yap SP, Karampinos DC, Nardo L, Kuo D, Burghardt AJ, et al. Does vertebral bone marrow fat content correlate with abdominal adipose tissue, lumbar spine bone mineral density, and blood biomarkers in women with type 2 diabetes mellitus? J Magn Reson Imaging. (2012) 35:117–24. doi: 10.1002/jmri.22757
32. Fan Y, Hanai JI, Le PT, Bi R, Maridas D, DeMambro V, et al. Parathyroid hormone directs bone marrow mesenchymal cell fate. Cell Metab. (2017) 25:661–72. doi: 10.1016/j.cmet.2017.01.001
Keywords: menopause, marrow adipose tissue, RANKL, bone mineral density, osteoprotegerin
Citation: Li X, Zuo X, Lu L, Xu R, Wang Y, Chang S, Wang Y, Luo P and Li G (2024) Vertebral marrow fat fraction is associated with circulating RANKL in postmenopausal females. Front. Endocrinol. 15:1442046. doi: 10.3389/fendo.2024.1442046
Received: 01 June 2024; Accepted: 29 August 2024;
Published: 16 September 2024.
Edited by:
Zhousheng Xiao, University of Tennessee Health Science Center (UTHSC), United StatesReviewed by:
Hongyan Wei, Shanghai Guanghua Hospital of Integrated Traditional Chinese and Western Medicine, ChinaChangjun Li, Central South University, China
Copyright © 2024 Li, Zuo, Lu, Xu, Wang, Chang, Wang, Luo and Li. This is an open-access article distributed under the terms of the Creative Commons Attribution License (CC BY). The use, distribution or reproduction in other forums is permitted, provided the original author(s) and the copyright owner(s) are credited and that the original publication in this journal is cited, in accordance with accepted academic practice. No use, distribution or reproduction is permitted which does not comply with these terms.
*Correspondence: Peng Luo, eXlsdW9wZW5nQDE2My5jb20=; Guanwu Li, Z3Vhbnd1LmxpQHNodXRjbS5lZHUuY24=
†These authors have contributed equally to this work and share first authorship