- 1Third Hospital of Shanxi Medical University, Shanxi Bethune Hospital, Shanxi Academy of Medical Sciences Tongji Shanxi Hospital, Taiyuan, China
- 2Department of Urology, Shanxi Bethune Hospital, Shanxi Academy of Medical Science, Tongji Shanxi Hospital, Third Hospital of Shanxi Medical University, Taiyuan, China
Background: A common pathophysiological association between lipid metabolism and sex hormone levels has been revealed in recent research. The atherogenic index of plasma (AIP) is the marker currently used to evaluate metabolism. The purpose of this research was to discover the relationship between the AIP and testosterone deficiency (TD) in a nationwide representative population.
Methods: Data from the National Health and Nutrition Examination Survey (NHANES) database from 2011 to 2016 were utilized in this cross-sectional research. The formula, lg [TG (mmol/L)/HDL-C(mmol/L)], was applied to determine the AIP. Total serum testosterone levels were used to define TD. Our researcher utilized smoothed curve fitting and multivariate logistic or linear regression analysis to inspect the relationship between AIP and TD among adult males. The consistency of these results was examined in various population subgroups.
Results: In total, 1,198 individuals (28.6%) were stratified into the TD group. We observed statistically significant differences (P values < 0.05) in the TD population for all variables. After correcting for potential confounders, our researchers discovered a strong positive relationship between the AIP and the probability of developing TD. With each additional unit of the AIP, the incidence of TD increased by 2.81-fold in adult males. Subgroup analyses showed the correlations for the majority of the subgroups remained stable. However, marital status, CKD, smoking, and alcohol consumption may modify this association.
Conclusions: A higher AIP is correlated with a lower level of testosterone in adult males. This correlation may be altered by factors including marriage, chronic kidney disease, alcohol, and smoking consumption.
1 Background
The main source of testosterone, an important class of sex hormones, is the testes in the male reproductive system, and in particular, the interstitial cells within the testes are responsible for synthesizing and releasing this hormone in large quantities. In addition to this, the body’s adrenal glands and ovaries are also involved in the production of testosterone (1). As a result, testosterone levels in men are generally significantly higher than in women and are essential for maintaining male secondary sexual characteristics and fertility (2). Research has revealed that the highest levels of testosterone production in men are during puberty and early adulthood, followed by a gradual decrease with age. After the age of 40, there is an annual decline of 0.4%–2.6%, and by the age of 80, testosterone concentrations may be 20% to 50% lower in comparison to younger ages (3–5). The role of testosterone is not limited to sex characterization and individual growth and development but is also involved the maintenance of a variety of physiological functions (6). In the United States, approximately 20% to 50% of men suffer from testosterone deficiency (TD) (7), a prevalent health problem that not only affects reproductive health and sexual function, but also leads to psychological and physiological disorders, cognitive dysfunction, muscular atrophy, osteoporosis, and related metabolic disorders such as depression (8–11). As a key regulator of men’s health, testosterone modulates a number of biological processes that are critical to men’s health. Reduced or insufficient serum testosterone levels have been associated with an increased risk of several cardiovascular diseases, such as hypertension, stroke, and coronary heart disease (12–14). In addition, TD has a pivotal role in the development of metabolic diseases, which include dyslipidemia, diabetes, and obesity (15, 16). Research suggests that deficient testosterone levels may be correlated with the incidence and seriousness of these metabolic diseases. Amid the increasing worldwide demographic shift towards an older population, the associated problem of lower testosterone levels has gradually attracted widespread public attention. Epidemiological statistics reveal that the average prevalence of TD in males above the age of 50 is approximately 7%, with the incidence increasing significantly with age (17, 18). Therefore, identifying and establishing biomarkers that can accurately predict changes in testosterone levels is of paramount importance for clinical practice, not only to help prevent a range of physical and mental disorders caused by testosterone deficiency but also to have a significant impact on improving the overall life satisfaction of patients.
Current medical research has found that individuals with abnormal lipid metabolism and obesity are often accompanied by lower testosterone levels, which rebound significantly when these metabolic problems are effectively treated. These findings reveal a strong link between testosterone levels and an individual’s metabolic health status (19–22). The plasma atherosclerotic index (AIP) is a new lipid biomarker that signifies the characteristics and severity of abnormal lipid metabolic procession (23). The AIP is strongly associated with atherosclerotic load and cardiovascular events (24–28). The AIP reflects the ratio of triglyceride (TG) to high-density lipoprotein cholesterol (HDL-C) and represents the dimensions of lipoprotein molecules, which indicates the disease-causing potential and distinctive nature of dyslipidemia more effectively than TG or HDL-C levels by themselves (29, 30). Since testosterone levels are associated with metabolic status and obesity, and the AIP reflects the lipid metabolic status of an individual, abnormalities in the AIP may suggest changes in testosterone levels. However, to our knowledge, the predictive value of the AIP in diagnosing testosterone deficiency among adult individuals remains unclear. Our research aimed to explore the correlation between the AIP and the prevalence of testosterone deficiency among US adults based on a nationally representative sample.
2 Materials and methods
2.1 Data sources
The 2011–2016 National Health and Nutrition Examination Survey (NHANES) datasets were the original information resource for the present analysis. The NHANES database provides comprehensive data on each participant’s laboratory tests, disease information, human examinations, and population characteristics. The reliability of the data in this database stems from the fact that it is collected based on a nationwide representative sample in the United States via a comprehensive investigation, using the most advanced and reliable techniques of information collection and organizational and statistical methods for data analysis. This data is subsequently subjected to additional analysis and utilized to study risk factors for a variety of diseases. A sophisticated multi-stage probabilistic approach was used in this study to ensure the representativeness and accuracy of the samples used. The qualification of human subjects in NHANES was accessible via the National Center for Health Statistics (NCHS) Ethics Review Board and each participant provided signed informed consent.
2.2 Research population
The researchers selected 29,902 participants from NHANES 2011–2016.The researchers requested that the dataset be a usable dataset containing information on TD, and the relevant variables needed to calculate the AIP. The researchers applied the exclusion criterion to the following groups: participants aged <20 years; those lacking sociodemographic data, confounders, and incomplete information on TD; and participants with incomplete data required for the AIP calculation. A total of 4,115 participants with comprehensive information participated in this cross-sectional investigation after the exclusion of participants who met the above criteria (Figure 1). This study applied the WTMEC2YR assessment weights to correct for sampling bias and improve the generalizability of the results.
2.3 Definition of the exposure variable
The AIP was regarded in the current investigation as an exposure variable, calculated by lg [TG (mg/dL)/HDL-C(mg/dL)]. TG values and HDL-C were assessed using a Cobas 6000 biochemistry instrument. We treated the AIP as both a categorical variable and a continuous variable for correlation studies for the purpose of further investigating the relationship between TD and AIP.
2.4 Definition of the outcome variable
The researchers considered the prevalence of TD and the level of testosterone as the outcome variables. Total testosterone in serum was measured using an isotope dilution liquid chromatography-tandem mass spectrometry (ID-LC-MS/MS) method developed by the Centers for Disease Control and Prevention (CDC) for routine analysis. The total testosterone level for the diagnosis of TD according to the Association of American Urology was <300 ng/dL.
2.5 Covariates
Factors that may have an impact on the association between TD and the AIP were regarded as covariates in this investigation. The covariates considered in this study included the sociodemographic factors of age (≤40, 41–60, or >60 years), educational level (high school graduate/GED or equivalent, college graduate or above, or less than 12th grade), ethnicity (other Hispanic, other races, Mexican American, non-Hispanic Black, or non-Hispanic white), marital condition (living alone, married, or living with partner), and family poverty income ratio (PIR) (≤1.0, 1.1-4.0, or >4.0). The researchers also included the health-related factors of diabetes, sedentary behavior, physical activity, hypertension, hyperlipidemia, chronic kidney disease (CKD), and cardiovascular diseases (CVDs) (all described as yes/no). According to questionnaire information, self-reported disease was also considered, including diabetes. A diagnosis of cardiovascular disease, hypertension, and hyperlipidemia was regarded as the basis of a self-reported history. The definition of cardiovascular disease includes angina, congestive heart failure, stroke, heart attack, and coronary heart disease. A diagnosis of CKD was defined as urinary Albumin-to-Creatinine Ratio (uACR)>30 mg/g or eGFR <60 ml/min/1.73 m². Specialized medical technicians took careful measurements of an individual’s body mass index (BMI) in a Mobile Examination Center. BMI is classified as overweight (25–30 kg/m²), obesity (≥30 kg/m²), or normal (<25 kg/m²). Physical activity is defined as a questionnaire response to moderate recreational activities. According to the responses to the questionnaire, sedentary behavior was defined as more than 360 minutes of sitting or lying down in a day at work, home, or school, while sleep time was excluded. PIR is the measurement of socioeconomic position, described as the rate of income to the United States Census Bureau’s poverty level for a family. Individuals are classified as present smokers, never smokers, or former smokers based on the “Do you personally use cigarette or tobacco?” and “Smoked more than 100 cigarettes in your lifetime” questions. Based on the “Drink at least twelve times in a lifetime or a year” question, the definition of a alcohol drinker includes current, never, and former alcohol drinkers. Laboratory data included albumin, liver enzymes, urea nitrogen, serum creatinine, calcium, phosphorus, sodium, potassium, total bilirubin, uric acid, and creatinine.
2.6 Statistical methods
Continuous and categorical variables were used to characterize participants at baseline. Categorical variables are described by numbers and percentages. Continuous variables are described in terms of mean ± standard deviation. Weighted chi-square tests and t-tests were conducted for comparisons of baseline characteristics among these participants. By assessing the corrected odds ratio (OR), β, and 95% confidence interval (CI), the study conducted a weighted logistic or linear regression model to explore the connections between TD, testosterone levels, and AIP. Three models were used by us with varying degrees of modification for covariates (Model 1, unadjusted for covariates; Model 2, modified for marital status, education, age, ethnicity, and PIR; and Model 3, adjustments for marital status, smoking status, BMI, PIR, education, alcohol consumption, physical activity, age, ethnicity, diabetes, hypertension, CVD, CKD, sedentary behavior, hyperlipidemia, and laboratory test indicators). In all three models, continuous variable AIP was used by our researchers. In addition, the dose-response relationship between AIP and the prevalence of the TD and testosterone level was evaluated by utilizing the restricted cubic spline (RCS) curves. This multiple logistic or linear regression model includes the continuous and categorical models. The AIP was divided into quartiles, and then the linear trends were calculated by considering the median value of every subgroup as a continuous variable. In addition, our researchers conducted subgroup analyses of general information, sedentary behavior, smoking status, disease condition, physical activity, alcohol consumption, and their interactions to examine whether there were different associations between subgroups. All statistical analyses in this investigation were conducted utilizing R 4.3.3 and SPSS 26.0. A bilateral P-value < 0.05 was recognized as statistically significant.
3 Result
3.1 Baseline characteristics of participants
The study recruited 29,902 individuals from NHANES 2011–2016. Figure 1 displays the flowchart for the exclusion and inclusion of individuals. Participants with incomplete information for testosterone were excluded and the remaining 21,592 participants were retained. Participants with incomplete information for age <20 years, sociodemographic data, and covariates were excluded, and the remaining 6,764 participants were retained. In addition, after excluding participants who had incomplete data required for AIP calculations and disease condition, the remaining 4,115 participants were considered in our investigation. The characteristics of the participants involved in this investigation at baseline are presented in Table 1. In total, 1,198 individuals (28.6%) were stratified into the TD group. We observed statistically significant differences (P-value < 0.05) in the TD population for all variables.
3.2 Association between AIP and TD
The crude model revealed the prevalence of TD increased 3.94-fold (OR 4.94, 95%CI 4.05~6.02, P < 0.001) for every unit addition in the AIP. Model 2 was adjusted for sociodemographic indicators and showed a 4.43-fold (OR 5.43, 95%CI 4.41~6.69, P < 0.001) increase in prevalence of TD in adult males. Model 3 was further controlled for disease status, with every unit addition of the AIP significantly increasing the prevalence of TD by 2.81-fold (OR 3.81, 95% CI 3.00~4.82, P < 0.001).
As shown in Table 2, each additional unit of the AIP was also categorized by quartile and compared to the first quartile as the reference. In the crude model, compared with the lowest Q1, the highest Q4 had a 4.36-fold increase in the prevalence of TD (OR 4.36, 95% CI 3.54~5.36). In Model 2, after an adjustment for sociodemographic factors, the risk of TD was increased 4.69-fold (OR 4.69, 95% CI 3.77~5.82) compared with Q1. In Model 3, the incidence was increased 3.13-fold, after the total adjustment (OR 3.13, 95% CI 2.46~3.98).
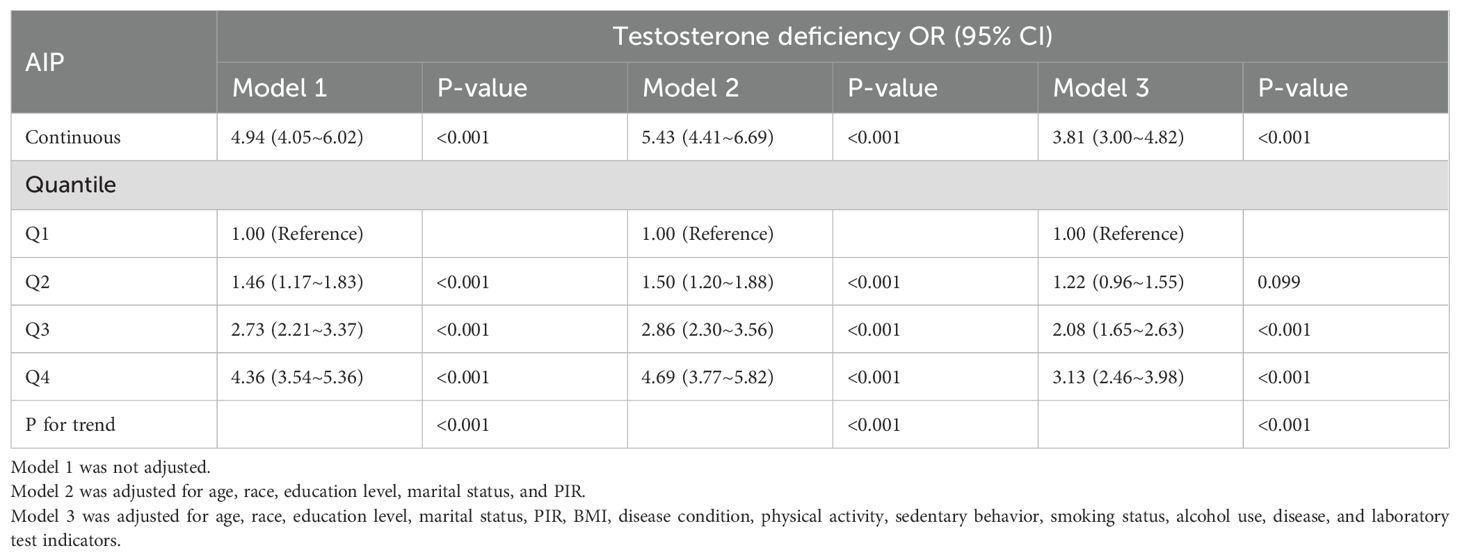
Table 2. Weighted regression models and trend tests elucidating the association between AIP and testosterone deficiency.
3.3 Association between AIP and total testosterone
This crude model showed that total testosterone decreases by 154.07 (β =-154.07, 95% CI -168.56~-139.58, P<0.001) for every unit increase in the AIP. Model 2 was adjusted for sociodemographic factors and showed a 155.89 decrease in total testosterone (β =-155.89, 95%CI -170.83~-140.96, P<0.001). Model 3 further controlled for disease status, with every unit addition of the AIP significantly increasing the total testosterone by 111.97 (β = -111.97, 95% CI -127.89~-96.06, P<0.001).
As shown in Table 3, the total testosterone in the Q4 group decreased by 104.78 compared with the Q1 group under the same conditions (β = -104.78, 95% CI -120.89~-88.66, P <0.001). In all models, a significant dose-response trend was found with the increase in the AIP (P for trend < 0.001). In Figure 2, the constructed RCS curves show the dose-response relationship between AIP and TD or testosterone levels.
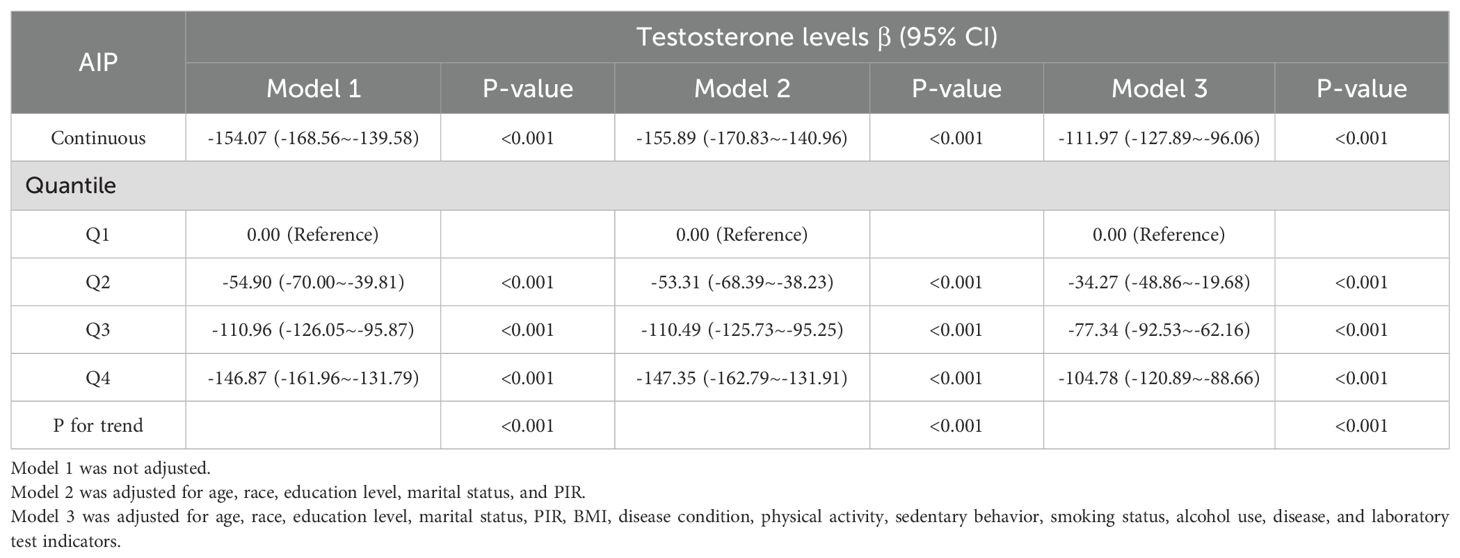
Table 3. Weighted regression models and trend tests elucidating the association between AIP and testosterone levels.
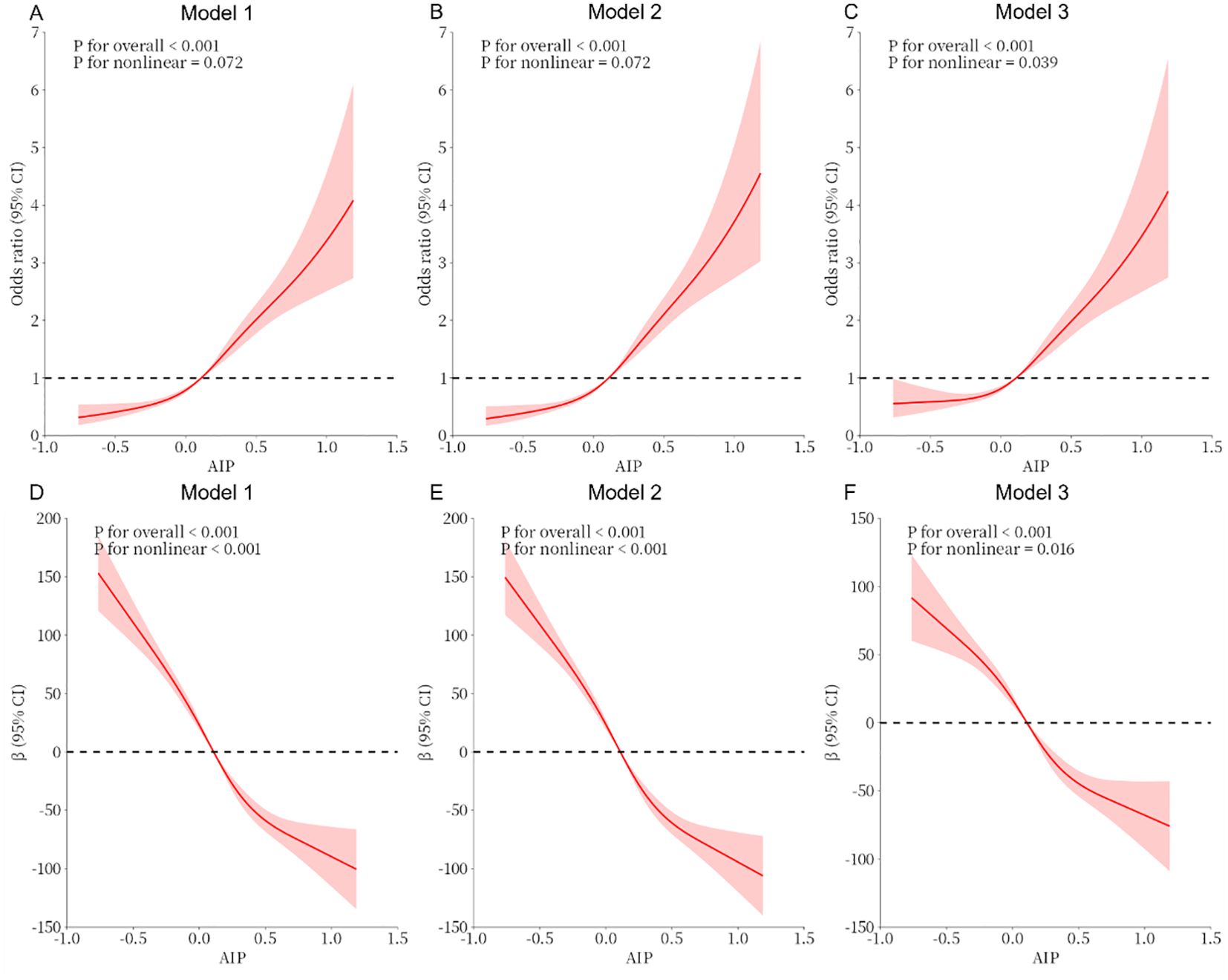
Figure 2. (A–C) Analysis of the measured response relationship between AIP and testosterone deficiency; (D–F) Analysis of the measured response relationship between AIP and testosterone levels. Model 1 was not adjusted. Model 2 was adjusted for age, race, education level, marital status, and PIR. Model 3 was adjusted for age, race, education level, marital status, PIR, BMI, disease condition, physical activity, sedentary behavior, smoking status, alcohol use, disease, and laboratory test indicators. The solid red line indicates the odds ratio or β and the red shaded area indicates the 95% confidence interval (CI).
3.4 Subgroup analysis
Analyzed by weighted logistic regression, the relationship between the incidence of TD and AIP for each layered indicator is reported in Table 4. For each unit addition in the AIP, the incidence of TD increased 3.73-fold (OR 4.74, 95% CI 3.57 ~ 6.29) in participants married and living with a partner. However, for participants living alone, the incidence of TD increased by 76% (OR 1.76, 95% CI 1.14 ~ 2.71). In addition, we observed significant changes in CKD. The tiered analysis of disease status showed a 2.91-fold increase for participants without CKD (OR 3.91, 95% CI 2.99 ~ 5.11) and a 1.33-fold increased risk for individuals with hypertension (OR 2.33, 95% CI 1.44 ~ 3.79). Regarding smoking and alcohol consumption, the risk was 1.95-fold increased for participants who currently smoked (OR 1.95, 95% CI 1.17 ~ 3.24) and 3.29-fold increased for participants who currently consumed alcohol (OR 3.29, 95% CI 2.56 ~ 4.22). The results of the stratified analysis remained reliably stable between the remaining groups except for marital status, CKD, smoking, and alcohol consumption.
4 Discussion
Our investigation primarily investigated the relevance between the AIP and the prevalence of TD in adult males using the NHANES database. The research involved in a sample size of 4,115 individuals. Weighted multifactorial logistic regression analysis revealed, after all covariates were controlled, a positive and statistically significant relationship between the prevalence of TD and the AIP in adult males. Furthermore, the RCS curves revealed a non-linear positive correlation between TD and the AIP in adult males. Additionally, the prevalence of TD progressively increased with the increasing AIP quartiles in adult males. The subgroup analyses revealed that the relationship between AIP and TD might be altered because of marital status, CKD, smoking, and alcohol consumption. Future research should also explore the complex interplay between the AIP, testosterone, and comorbid conditions such as chronic kidney disease and diabetes mellitus, where dyslipidemia and endocrine dysfunction may create synergistic detrimental effects on male health outcomes.
The positive correlation of the AIP with the incidence of TD varied among certain subgroups. Different marital statuses may lead to different psychological and physiological stresses that may affect the stability of testosterone levels. It has been revealed that men living with a partner have lower testosterone levels compared to unmarried men (31), a result that leads to an unstably positive synergistic relationship between the atherosclerosis index and testosterone deficiency across marital status. Meanwhile, a broad spectrum of studies has underscored that smoking has an effect on sperm density, viability, vigor, and morphology; seminal plasma zinc concentration; and sperm DNA damage in men, with the sperm DNA fracture index being higher in smoking than in non-smoking groups and sperm DNA fragmentation being increased. This suggests that smoking may affect testosterone synthesis and sperm quality by increasing DNA damage (32). Alcohol consumption may lead to abnormal liver function and affect the metabolism of sex hormone-binding globulin, which in turn affects the bioavailability of testosterone (33). In summary, smoking and alcohol consumption may combine to destabilize the association between AIP and testosterone deficiency by affecting the endocrine system, increasing oxidative stress, and affecting liver function. In addition, changes in renal function directly affect hormone metabolism and excretion, which may influence testosterone levels and lead to unstable outcomes. These findings raise important clinical questions about whether targeted interventions such as dietary modifications, lifestyle changes, or pharmacologic lipid-lowering therapies could serve as adjunct strategies for managing androgen deficiency in metabolic syndrome populations, a hypothesis requiring verification through randomized controlled trials. Similarly, lifestyle interventions that reduce visceral adiposity and insulin resistance could ameliorate both the AIP and testosterone deficiency through shared metabolic pathways. Randomized controlled trials are urgently needed to evaluate whether AIP-lowering strategies serve as adjunct therapies for managing androgen deficiency in high-risk populations, particularly those with metabolic syndrome or CKD.
An array of investigations have revealed that TD is a common condition. It has a significant influence on the quality of life and healthy condition of the patient, as well as a remarkable influence on the psychological wellbeing of individuals. Nevertheless, the underlying pathological processes of TD remain incompletely understood. Although the occurrence of TD in the adults has remained at the same level in recent years, the overall incidence and public health impact of the condition remains quite significant given the rising population size, which may indirectly reflect trends in the development of TD in other countries around the globe. This exacerbates the strain on healthcare systems and increases explicit and implicit medical costs connected to the condition. In addition, TD is connected to a variety of chronic diseases, including cardiovascular and disease kidney disease, further increasing the need for public health interventions. An elevated AIP is a key indicator of lipid metabolite interactions and is associated with the incidence of TD, a relationship that may involve multiple mechanisms. We introduced the AIP, which combines triglyceride to HDL-C ratios, and concluded that it more accurately reflects an individual’s metabolic health status.
Several mechanisms may explain the positive relationship between the incidence of TD and AIP in adults with diabetes. These include several aspects such as dyslipidemia, insulin resistance, inflammatory response, and hepatic lipid metabolism (34–38). Initially, research demonstrated that testosterone significantly contributes to the regulation of lipid metabolism in male individuals. Serum testosterone levels were positively correlated with HDL-C and inversely related to low-density lipoprotein cholesterol (LDL-C), triglycerides, and total cholesterol (39, 40). Correlation studies indicate that higher levels of testosterone in the blood are associated with increased levels of HDL-C, while they are inversely related to the concentrations of low-density lipoprotein cholesterol (LDL-C), triglycerides, and total cholesterol.
This implies that endogenous testosterone may be a favorable factor in maintaining the balance of lipid metabolism in men. When testosterone levels are low, this balance is disrupted, resulting in increased levels of TG and non-HDL-C and decreased levels of HDL-C. This implies that testosterone deficiency may contribute to an elevated AIP by affecting lipid levels, specifically by increasing harmful lipid components (TG and non-HDL-C) and decreasing beneficial lipid components (HDL-C) (41). At the same time, testosterone deficiency results in a compromised increase of HDL-C triggered by its conversion to estradiol by aromatase, which also leads to an increased AIP (42). Furthermore, evidence has demonstrated that retinol-binding protein 4 (RBP4) is crucial for managing insulin resistance. Notably, in a murine model exhibiting diminished testosterone activity, there was a marked increase in RBP4 protein concentrations, potentially linking it to the onset of insulin resistance (43–45). Furthermore, insulin resistance could contribute to the activation of the fatty acid synthesis pathway in adipocytes, which promotes fatty acid synthesis and fat accumulation while inhibiting fatty acid oxidation, leading to dyslipidemia (46), which in turn affects AIP levels. In addition, research has demonstrated a strong correlation between testosterone deficiency and increased levels of inflammatory indicators, including high-sensitivity C-reactive protein, interleukin-6, and tumor necrosis factor-alpha (47). This implies that TD may be associated with increased levels of inflammation in the body, while the AIP is dependent on the logarithm of the ratio of plasma TG to HDL-C, which reflects the size of oxidized LDL particles in serum. Inflammatory factors can affect lipid metabolism (48), particularly by increasing the levels of oxidized low-density lipoprotein (oxLDL), which may lead to elevated AIP levels. Ultimately, testosterone may alter the genetic expression linked to the formation and release of lipoproteins, including the scavenger receptor B1 (SR-B1). Hepatic lipase expression is upregulated in hepatocytes. This enzyme catalyzes the breakdown of phospholipids on HDL-C particles and facilitates the selective uptake of HDL-C via SR-B1, which promotes retrograde cholesterol transport and exerts an antiatherosclerotic effect (49), thus leading to the correlation between an elevated AIP and testosterone deficiency. The biological mechanisms underlying this association warrant further investigation, particularly regarding how altered lipid metabolism may impact cell function, steroidogenic enzyme activity, or androgen receptor signaling through inflammatory or oxidative stress pathways.
According to studies in recent years, the current rate of TD in the global population continues to rise, affecting people’s physical and mental health in various ways. Lipid metabolism is closely associated with TD problems. Our investigation confirms a novel demonstration of a significant relationship between the AIP and the occurrence of TD in adult males, which has significant implications for the diagnosis and treatment of TD. This research exhibits several strengths. Based on the NHANES database, the investigation is primarily a cross-sectional investigation to research the correlation between the AIP and TD in adult males. At the same time, our study is representative because it contains basic data from a large number of respondents in the US. However, it is worth noting that we still have some limitations. First, some of the information in the NHANES database was obtained through self-reporting by the respondents, including disease information. Self-reporting bias could lead to imprecise answers, which may affect the accuracy of the results. Second, this investigation could not establish a direct sequential association between TD and the AIP in adult males, but only inferred a correlation, due to it being a cross-sectional study. Longitudinal studies tracking lipid profiles and hormonal changes over time are needed to establish temporal relationships and clarify potential causal pathways. The diagnosis of testosterone deficiency relied solely on a single serum testosterone measurement without clinical symptom assessment. Diurnal variation in testosterone levels and the absence of symptom data may have introduced misclassification bias. Future studies should incorporate repeated measures, standardized morning blood draws, and validated symptom questionnaires. In conclusion, this study suggests there is a positive significant relationship between the AIP and the risk of TD in adult males.
5 Conclusion
The research revealed a positive significant relationship between the AIP and TD in American adult males. In adult males, a higher AIP was correlated with a higher occurrence of TD. This finding suggests that lipid metabolism may be an influencing factor for TD. The clinical importance of our research is that assessing the AIP may help identify those at higher risk for TD. Incorporation of the AIP into common clinical assessment could contribute to early detection of TD and direct personalized care strategies.
Data availability statement
The original contributions presented in the study are included in the article/supplementary material. Further inquiries can be directed to the corresponding author.
Ethics statement
The NCHS Research Ethics Review Board approved the NHANES study protocol, and participants provided written informed consent at enrollment (https://www.cdc.gov/nchs/nhanes/irba98.htm). The studies were conducted in accordance with the local legislation and institutional requirements. Written informed consent for participation was not required from the participants or the participants’ legal guardians/next of kin in accordance with the national legislation and institutional requirements.
Author contributions
YT: Conceptualization, Data curation, Formal analysis, Investigation, Methodology, Project administration, Software, Writing – original draft, Writing – review & editing. BC: Conceptualization, Data curation, Formal analysis, Investigation, Methodology, Project administration, Software, Writing – original draft, Writing – review & editing. YK: Conceptualization, Data curation, Formal analysis, Investigation, Methodology, Project administration, Software, Writing – original draft, Writing – review & editing. JS: Conceptualization, Data curation, Funding acquisition, Investigation, Methodology, Project administration, Resources, Validation, Visualization, Writing – original draft, Writing – review & editing.
Funding
The author(s) declare that no financial support was received for the research and/or publication of this article.
Conflict of interest
The authors declare that the research was conducted in the absence of any commercial or financial relationships that could be construed as a potential conflict of interest.
Generative AI statement
The author(s) declare that no Generative AI was used in the creation of this manuscript.
Publisher’s note
All claims expressed in this article are solely those of the authors and do not necessarily represent those of their affiliated organizations, or those of the publisher, the editors and the reviewers. Any product that may be evaluated in this article, or claim that may be made by its manufacturer, is not guaranteed or endorsed by the publisher.
Abbreviations
NHANES, National Health and Nutrition Examination Survey; AIP, Atherogenic index of plasma; TD, Testosterone deficiency; TG, Triglyceride; HDL-C, High-density lipoprotein cholesterol; LDL-C, Low-density lipoprotein cholesterol; CKD, Chronic kidney disease; CVD, Cardiovascular diseases; NCHS, National Center for Health Statistics; ID-LC-MS/MS, Liquid chromatography tandem mass spectrometry; PIR, Poverty income ratio; eGFR, Estimated Glomerular Filtration Rate; uACR, Urine Albumin-to-Creatinine Ratio; BMI, Body Mass Index; RCS, Restricted cubic spline; ALT, Alanine aminotransferase; AST, Aspartate aminotransferase; ALP, Alkaline Phosphatase; BUN, Blood Urea Nitrogen; OR, Odds Ratio; CI, Confidence Interval; RBP4, Retinol-binding protein 4; OxLDL, Oxidized low-density lipoprotein; SR-B1. Scavenger receptor B1.
References
1. De Oliveira DH, Fighera TM, Bianchet LC, Kulak CA, Kulak J. Androgens and bone. MINERVA Endocrinol. (2012) 37:305–14.
2. Gagliano-Juca T, Basaria S. Testosterone replacement therapy and cardiovascular risk. Nat Rev Cardiol. (2019) 16:555–74. doi: 10.1038/s41569-019-0211-4
3. Lokeshwar SD, Patel P, Fantus RJ, Halpern J, Chang C, Kargi AY, et al. Decline in serum testosterone levels among adolescent and young adult men in the USA. Eur Urol Focus. (2021) 7:886–9. doi: 10.1016/j.euf.2020.02.006
4. Mei Y, Chen Y, Wang X, Xu R, Feng X. The relationship between remnant cholesterol and the risk of testosterone deficiency in US adults: a cross-sectional study based on the NHANES database. Front Endocrinol (Lausanne). (2024) 15:1458193. doi: 10.3389/fendo.2024.1458193
5. Liu CC, Wu WJ, Lee YC, Wang CJ, Ke HL, Li WM, et al. The prevalence of and risk factors for androgen deficiency in aging Taiwanese men. J SEX Med. (2009) 6:936–46. doi: 10.1111/j.1743-6109.2008.01171.x
6. Xiao Y, Yin S, Cui J, Bai Y, Yang Z, Wang J, et al. Association between the prevalence rates of circadian syndrome and testosterone deficiency in US males: data from NHANES (2011-2016). Front Nutr. (2023) 10:1137668. doi: 10.3389/fnut.2023.1137668
7. Kwong J, Krakowsky Y, Grober E. Testosterone deficiency: A review and comparison of current guidelines. J SEX Med. (2019) 16:812–20. doi: 10.1016/j.jsxm.2019.03.262
8. Suarez MM, Navarro PA, Lopez RB, Sanchez GP, Lage GJ, Mate HA, et al. Treatment of complicated diverticular disease. Our experience. Rev Esp Enferm Apar Dig. (1988) 73:191–6. doi: 10.1097/01.pra.0000358315.88931.fc
9. DiFrancesco S, Lamers F, Riese H, Merikangas KR, Beekman A, van Hemert AM, et al. Sleep, circadian rhythm, and physical activity patterns in depressive and anxiety disorders: A 2-week ambulatory assessment study. DEPRESS Anxiety. (2019) 36:975–86. doi: 10.1002/da.v36.10
10. Kruljac M, Finnbogadottir H, Bobjer J, Giraldi A, Fugl-Meyer K, Giwercman A. Symptoms of sexual dysfunction among men from infertile couples: prevalence and association with testosterone deficiency. ANDROLOGY-US. (2020) 8:160–5. doi: 10.1111/andr.12678
11. Patel P, Shiff B, Kohn TP, Ramasamy R. Impaired sleep is associated with low testosterone in US adult males: results from the National Health and Nutrition Examination Survey. World J UROL. (2019) 37:1449–53. doi: 10.1007/s00345-018-2485-2
12. Gencer B, Bonomi M, Adorni MP, Sirtori CR, Mach F, Ruscica M. Cardiovascular risk and testosterone - from subclinical atherosclerosis to lipoprotein function to heart failure. Rev Endocr Metab Disord. (2021) 22:257–74. doi: 10.1007/s11154-021-09628-2
13. Gagliano-Juca T, Alvarez M, Basaria S. The medicalization of testosterone: reinventing the elixir of life. Rev Endocr Metab Disord. (2022) 23:1275–84. doi: 10.1007/s11154-022-09751-8
14. Di Lodovico E, Facondo P, Delbarba A, Pezzaioli LC, Maffezzoni F, Cappelli C, et al. Testosterone, hypogonadism, and heart failure. Circ Heart Fail. (2022) 15:e8755. doi: 10.1161/CIRCHEARTFAILURE.121.008755
15. Newman CB. Effects of endocrine disorders on lipids and lipoproteins. Best Pract Res Clin Endocrinol Metab. (2023) 37:101667. doi: 10.1016/j.beem.2022.101667
16. Wittert G, Grossmann M. Obesity, type 2 diabetes, and testosterone in ageing men. Rev Endocr Metab Disord. (2022) 23:1233–42. doi: 10.1007/s11154-022-09746-5
17. Li C, Xu J. Negative correlation between metabolic score for insulin resistance index and testosterone in male adults. DIABETOL Metab Syndr. (2024) 16:113. doi: 10.1186/s13098-024-01353-5
18. Kizer KW, Golden JA. Lipoid pneumonitis in a commercial abalone diver. Undersea BioMed Res. (1987) 14:545–52. doi: 10.1056/NEJMoa0911101
19. Liu X, He Y, Zhang Q, Zeng T, Zhang J, Min J, et al. Catch-up fat in male adults induces low testosterone and consequently promotes metabolic abnormalities and cognitive impairment. ANDROLOGY-US. (2022) 10:871–84. doi: 10.1111/andr.13177
20. Rigon FA, Ronsoni MF, Hohl A, van de Sande-Lee S. Effects of bariatric surgery in male obesity-associated hypogonadism. OBES Surg. (2019) 29:2115–25. doi: 10.1007/s11695-019-03829-0
21. De Maddalena C, Vodo S, Petroni A, Aloisi AM. Impact of testosterone on body fat composition. J Cell Physiol. (2012) 227:3744–8. doi: 10.1002/jcp.v227.12
22. Grandys M, Majerczak J, Zapart-Bukowska J, Duda K, Kulpa JK, Zoladz JA. Lowered serum testosterone concentration is associated with enhanced inflammation and worsened lipid profile in men. Front Endocrinol (Lausanne). (2021) 12:735638. doi: 10.3389/fendo.2021.735638
23. Dobiasova M, Frohlich J. The plasma parameter log (TG/HDL-C) as an atherogenic index: correlation with lipoprotein particle size and esterification rate in apoB-lipoprotein-depleted plasma (FER(HDL)). Clin Biochem. (2001) 34:583–8. doi: 10.1016/s0009-9120(01)00263-6
24. Bora K, Pathak MS, Borah P, Hussain MI, Das D. Association of the apolipoprotein A-I gene polymorphisms with cardiovascular disease risk factors and atherogenic indices in patients from Assam, northeast India. Balkan J Med Genet. (2017) 20:59–70. doi: 10.1515/bjmg-2017-0002
25. Won KB, Heo R, Park HB, Lee BK, Lin FY, Hadamitzky M, et al. Atherogenic index of plasma and the risk of rapid progression of coronary atherosclerosis beyond traditional risk factors. ATHEROSCLEROSIS. (2021) 324:46–51. doi: 10.1016/j.atherosclerosis.2021.03.009
26. Li YW, Kao TW, Chang PK, Chen WL, Wu LW. Atherogenic index of plasma as predictors for metabolic syndrome, hypertension and diabetes mellitus in Taiwan citizens: a 9-year longitudinal study. Sci Rep. (2021) 11:9900. doi: 10.1038/s41598-021-89307-z
27. Zhu X, Yu L, Zhou H, Ma Q, Zhou X, Lei T, et al. Atherogenic index of plasma is a novel and better biomarker associated with obesity: a population-based cross-sectional study in China. Lipids Health Dis. (2018) 17:37. doi: 10.1186/s12944-018-0686-8
28. Guo Q, Zhou S, Feng X, Yang J, Qiao J, Zhao Y, et al. The sensibility of the new blood lipid indicator–atherogenic index of plasma (AIP) in menopausal women with coronary artery disease. Lipids Health Dis. (2020) 19:27. doi: 10.1186/s12944-020-01208-8
29. Alifu J, Xiang L, Zhang W, Qi P, Chen H, Liu L, et al. Association between the atherogenic index of plasma and adverse long-term prognosis in patients diagnosed with chronic coronary syndrome. Cardiovasc DIABETOL. (2023) 22:255. doi: 10.1186/s12933-023-01989-z
30. Fernandez-Macias JC, Ochoa-Martinez AC, Varela-Silva JA, Perez-Maldonado IN. Atherogenic index of plasma: novel predictive biomarker for cardiovascular illnesses. Arch Med Res. (2019) 50:285–94. doi: 10.1016/j.arcmed.2019.08.009
31. Gettler LT, Sarma MS, Gengo RG, Oka RC, McKenna JJ. Adiposity, CVD risk factors and testosterone: Variation by partnering status and residence with children in US men. Evol Med Public Health. (2017) 2017:67–80. doi: 10.1093/emph/eox005
32. Zhao J, Leung J, Lin SL, Mary SC. Cigarette smoking and testosterone in men and women: A systematic review and meta-analysis of observational studies. Prev Med. (2016) 85:1–10. doi: 10.1016/j.ypmed.2015.12.021
33. Smith SJ, Lopresti AL, Fairchild TJ. The effects of alcohol on testosterone synthesis in men: a review. Expert Rev Endocrinol Metab. (2023) 18:155–66. doi: 10.1080/17446651.2023.2184797
34. Shin HR, Song S, Cho JA, Ly SY. Atherogenic index of plasma and its association with risk factors of coronary artery disease and nutrient intake in Korean adult men: the 2013–2014 KNHANES. NUTRIENTS. (2022) 14. doi: 10.3390/nu14051071
35. Samimi S, Rajabzadeh S, Rabizadeh S, Nakhjavani M, Nakhaei P, Avanaki FA, et al. Atherogenic index of plasma is an independent predictor of metabolic-associated fatty liver disease in patients with type 2 diabetes. Eur J Med Res. (2022) 27:112. doi: 10.1186/s40001-022-00731-x
36. Zhu XW, Deng FY, Lei SF. Meta-analysis of Atherogenic Index of Plasma and other lipid parameters in relation to risk of type 2 diabetes mellitus. PRIM Care Diabetes. (2015) 9:60–7. doi: 10.1016/j.pcd.2014.03.007
37. Shi Y, Wen M. Sex-specific differences in the effect of the atherogenic index of plasma on prediabetes and diabetes in the NHANES 2011–2018 population. Cardiovasc DIABETOL. (2023) 22:19. doi: 10.1186/s12933-023-01740-8
38. Duan SJ, Ren ZY, Zheng T, Peng HY, Niu ZH, Xia H, et al. Atherogenic index of plasma combined with waist circumference and body mass index to predict metabolic-associated fatty liver disease. World J Gastroenterol. (2022) 28:5364–79. doi: 10.3748/wjg.v28.i36.5364
39. Chung TH, Kwon YJ, Lee YJ. High triglyceride to HDL cholesterol ratio is associated with low testosterone and sex hormone-binding globulin levels in Middle-aged and elderly men. Aging MALE. (2020) 23:93–7. doi: 10.1080/13685538.2018.1501015
40. Davis SR, Azene ZN, Tonkin AM, Woods RL, McNeil JJ, Islam RM. Higher testosterone is associated with higher HDL-cholesterol and lower triglyceride concentrations in older women: an observational study. CLIMACTERIC. (2024) 27:282–8. doi: 10.1080/13697137.2024.2310530
41. Asscheman H, Gooren LJ, Megens JA, Nauta J, Kloosterboer HJ, Eikelboom F. Serum testosterone level is the major determinant of the male-female differences in serum levels of high-density lipoprotein (HDL) cholesterol and HDL2 cholesterol. METABOLISM. (1994) 43:935–9. doi: 10.1016/0026-0495(94)90170-8
42. Semmens J, Rouse I, Beilin LJ, Masarei JR. Relationship of plasma HDL-cholesterol to testosterone, estradiol, and sex-hormone-binding globulin levels in men and women. METABOLISM. (1983) 32:428–32. doi: 10.1016/0026-0495(83)90002-1
43. Liu C, Zhou XR, Ye MY, Xu XQ, Zhang YW, Liu H, et al. RBP4 is associated with insulin resistance in hyperuricemia-induced rats and patients with hyperuricemia. Front Endocrinol (Lausanne). (2021) 12:653819. doi: 10.3389/fendo.2021.653819
44. Fan J, Hu J. Retinol binding protein 4 and type 2 diabetes: from insulin resistance to pancreatic beta-cell function. ENDOCRINE. (2024) 85:1020–34. doi: 10.1007/s12020-024-03777-5
45. Guducu N, Gormus U, Kavak ZN, Isci H, Yigiter AB, Dunder I. Retinol-binding protein 4 is elevated and is associated with free testosterone and TSH in postmenopausal women. J Endocrinol Invest. (2013) 36:831–4. doi: 10.3275/8948
46. Yin B, Wu Z, Xia Y, Xiao S, Chen L, Li Y. Non-linear association of atherogenic index of plasma with insulin resistance and type 2 diabetes: a cross-sectional study. Cardiovasc DIABETOL. (2023) 22:157. doi: 10.1186/s12933-023-01886-5
47. Mohamad NV, Wong SK, Wan HW, Jolly JJ, Nur-Farhana MF, Ima-Nirwana S, et al. The relationship between circulating testosterone and inflammatory cytokines in men. Aging MALE. (2019) 22:129–40. doi: 10.1080/13685538.2018.1482487
48. Feingold KR, Grunfeld C. The effect of inflammation and infection on lipids and lipoproteins. (2000).
Keywords: AIP, testosterone deficiency, lipid metabolism, population-based studies, NHANES
Citation: Tai Y, Chen B, Kong Y and Shang J (2025) Association between the atherogenic index of plasma and testosterone deficiency in American adults: a cross-sectional study from NHANES 2011–2016. Front. Endocrinol. 16:1531221. doi: 10.3389/fendo.2025.1531221
Received: 20 November 2024; Accepted: 10 April 2025;
Published: 08 May 2025.
Edited by:
Zhice Xu, Wuxi Maternity and Child Health Care Hospital, ChinaReviewed by:
Roshan Kumar Mahat, Dharanidhar Medical College and Hospital, IndiaSarah Salama, King Salman International University, Egypt
Copyright © 2025 Tai, Chen, Kong and Shang. This is an open-access article distributed under the terms of the Creative Commons Attribution License (CC BY). The use, distribution or reproduction in other forums is permitted, provided the original author(s) and the copyright owner(s) are credited and that the original publication in this journal is cited, in accordance with accepted academic practice. No use, distribution or reproduction is permitted which does not comply with these terms.
*Correspondence: Jiwen Shang, U2p3MTM5QDEyNi5jb20=
†These authors have contributed equally to this work
‡ORCID: Jiwen Shang, orcid.org/0000-0003-2971-4683