- 1Department of Neurology, Shengjing Hospital of China Medical University, Shenyang, China
- 2Department of Cardiology, Kailuan General Hospital, Tangshan, China
- 3Department of Epidemiology and Biostatistics, School of Public Health, North China University of Science and Technology, Tangshan, China
- 4Department of Epidemiology and Biostatistics, School of Public Health, Peking University, Beijing, China
- 5Department of Internal Medicine, Hebei Medical University, Shijiazhuang, Hebei, China
- 6Department of Epidemiology and Biostatistics, Ministry of Education Key Laboratory of Environment and Health, School of Public Health, Tongji Medical College, Huazhong University of Science and Technology, Wuhan, Hubei, China
Objective: Systolic blood pressure time in target range (SBP-TTR) is an independent risk factor for stroke. We aimed to investigate the associations of SBP-TTR with stroke among participants with or without diabetes using data from the Kailuan study.
Methods: We included 28,591 participants [mean age, 57.5 years; 83.8% men; 23.2% with diabetes] from the Kailuan Study. Cox proportional hazards regression models were used to estimate the hazard ratios (HRs) and 95% confidence intervals (95% CIs) of SBP-TTR on stroke in individuals with and without diabetes.
Results: After a median of 8.7 years follow-up, 2,206 stroke cases occurred. Among participants with diabetes, those with SBP-TTR 75%–100% (HR [95%CI]: 0.64 [0.49, 0.84]) had a lower risk of stroke compared to those with SBP-TTR 0%–25%. Among participants without diabetes, those with SBP-TTR 50%–75% (HR 0.75, 95% CI 0.64–0.88) and 75%–100% (HR [95%CI]: 0.62 [0.52, 0.73]) had a significantly lower risk of stroke. A significant interaction between diabetes status and SBP-TTR was observed (P for interaction = 0.03). Additionally, the restricted cubic spline analysis showed a non-linear relationship between SBP-TTR and stroke risk among participants with diabetes (P for non-linearity = 0.001), and a linear relationship among those without diabetes (P for non-linearity = 0.035).
Conclusion: Higher SBP-TTR was associated with a reduced risk of stroke among participants with or without diabetes. The findings underscore the importance of maintaining blood pressure within the target range to mitigate stroke risk, particularly emphasizing the need for stringent blood pressure control in diabetic patients.
Introduction
Stroke has become a significant global public health concern. In 2019, the global burden of disease identified stroke as the second leading cause of death worldwide, with 12.2 million new cases (1), including 3.94 million in China (2). Hypertension and diabetes are two major risk factors for stroke, and these two conditions often coexist (3–5). Previous evidence showed that 32% of individuals with hypertension were also diagnosed with diabetes (6), while 60%-75% of patients with diabetes also had hypertension among Chinese population (7). Moreover, the presence of both diabetes and hypertension could significantly increase the risk of stroke (8).
Effective blood pressure (BP) management is fundamental to stroke prevention in people with and without diabetes, with antihypertensive therapy demonstrating significant stroke risk reduction (9). However, BP control targets differ between these groups. For individuals without diabetes, BP management primarily focuses on maintaining systolic blood pressure (SBP) between 120 and 140 mmHg to reduce the risk of stroke (10). However, individuals with diabetes exhibit a significantly higher stroke risk, with women being at greater risk than men, particularly for ischemic stroke (11). Consequently, more intensive BP control is generally recommended for individuals with diabetes, yet the optimal target remains controversial. The Action to Control Cardiovascular Risk in Diabetes (ACCORD) trial found that lowering SBP to <120 mmHg, compared to <140 mmHg, did not reduce the rate of a composite outcome of cardiovascular events in individuals with diabetes (12). In contrast, the Blood Pressure Control Target in Diabetes (BPROAD) trial demonstrated that intensive SBP control (<120 mmHg) significantly lowered the risk of major cardiovascular events (13). These findings not only highlight the ongoing debate over optimal BP management in diabetes but also emphasize the need to paying attention to other BP control measures beyond absolute SBP targets.
Additionally, SBP time in target range (SBP-TTR) is a new metric for evaluating blood pressure control, which refers to the proportion of time that SBP remains within the target range. This metric includes both the average BP value and BP variability over a long-term follow-up period, reflecting BP variation over time (14). According to the Systolic Blood Pressure Intervention Trial (SPRINT) study, higher SBP-TTR was associated with a reduced risk of stroke in a dose-dependent manner (15). Similarly, the ACCORD study found that in individuals with diabetes, higher SBP-TTR was also associated with a lower risk of stroke (16). However, both the SPRINT and ACCORD studies were conducted as randomized controlled trials with specific inclusion criteria, which do not fully capture the complexity and variability of real-world clinical settings, where populations are more diverse and management practices differ. Moreover, there remains a gap in understanding whether this benefit varies between participants with diabetes and without diabetes over longer follow-up periods in real-world settings.
To address these gaps, this study aims to investigate the impact of SBP-TTR on the risk of stroke in people with and without diabetes leveraging data from the Kailuan Study, a large, long-term Chinese cohort.
Methods
Study population
The Kailuan Study is a prospective cohort study conducted in Tangshan, China, based on a community population. Detailed descriptions of the study design have been provided in previous articles (17, 18). Since June 2006, the Kailuan General Hospital and its 10 affiliated hospitals have conducted biennial health examination for both current and retired staff members of the Kailuan Group, a large state-owned coal and energy enterprise. This comprehensive assessment was followed by a series of biennial health check-ups and questionnaire-based surveys. This study was approved by the Medical Ethics Committee of Kailuan General Hospital (No. 2018ZX10715005) and adhered strictly to the principles outlined in the Helsinki Declaration. Informed consent was obtained from all participants.
Individuals who underwent the Kailuan health examination for the first time in 2006 or 2008, participated in the 2012 follow-up, and were diagnosed with hypertension at their first examination were included in the present analysis. The observation window for participants enrolled in 2006 was from 2006 to 2012, while for those enrolled in 2008, it was from 2008 to 2012. The exclusion criteria were as follows: (1) participants who died before 2012, (2) participants with missing SBP values in either 2006 or 2012 for those enrolled in 2006, and in either 2008 or 2012 for those enrolled in 2008, (3) participants with history of stroke before 2012, and (4) participants with history of cardiovascular disease before 2012 (Figure 1).
Measurement of BP, and definition of SBP-TTR
BP values were measured between 7:00 and 9:00 am on the day of the physical examination using a standard desktop mercury sphygmomanometer. Participants were instructed to sit quietly for at least 5 minutes before the measurement. Three readings were taken at 1 to 2-minute intervals, and the average of these readings was used. In this study, the target range for SBP was set between 120 and 140 mmHg (15). Hypertension was defined as a self-reported history of hypertension, use of antihypertensive medications, SBP ≥140 mmHg, and/or diastolic blood pressure (DBP) ≥90 mmHg. SBP-TTR was defined as the percentage of time that SBP remains within the target range during the observation window. To estimate this, we employed linear interpolation to impute missing SBP values between observation window. For each pair of adjacent time points, a linear model was fitted to estimate the duration within the target range. The time spent in the target range for each interval was then summed to determine the total duration within the target range across the observation window. Finally, SBP-TTR was calculated using the following formula: (time in target range/observation period) *100% (19). SBP-TTR was divided into four groups: 0%<SBP-TTR ≤ 25%, 25% < SBP-TTR ≤ 50%, 50% < SBP-TTR ≤75%, 75% < SBP-TTR ≤ 100%.
Assessment of stroke
The identification of stroke cases was achieved through meticulous review of medical records (ICD-10 code I60 to I61 for hemorrhagic stroke and I63 for ischemic stroke). All cases were confirmed by professional physicians based on hospitalization records. Participants were followed beginning with the completion of health examinations in 2012 until the occurrence of stroke, death, loss to follow-up, or end of follow-up (December 31, 2020), whichever occurred first.
Assessment of the covariates
Demographic data (age, sex, income), health behaviors (smoking status, drinking status, education, physical exercise), medical history (diabetes, stroke, cardiovascular disease), and medication use (antihypertensive, lipid-lowering, hypoglycemic drugs) of the participants were collected through questionnaires. Methods for assessing relevant biomarkers (total cholesterol [TC], fasting blood glucose [FBG] and serum creatinine) and anthropometric measurements (height, weight, SBP, DBP) were described in previously published literature (20). Body Mass Index (BMI) was calculated as weight in kilograms divided by the square of height in meters (kg/m²). Current smokers were defined as consuming at least one cigarette per day on average over the past year. Current drinkers were defined as consuming at least 100 mL of liquor (alcohol content ≥ 50%) per day on average over the past year. Education levels were categorized into low (illiterate, primary school, or middle school) and high (high school or college/university). Physically active was defined as engaging in physical activity at least three times per week, with each session lasting at least 30 minutes. Estimated glomerular filtration rate (eGFR) was calculated using the Chronic Kidney Disease Epidemiology Collaboration (CKD-EPI) creatinine equation (21). Urine analysis was conducted using the dry chemical method and urine sediment examination. Urinary protein was measured using the semi-quantitative test strip method. Positive proteinuria was defined as urine dipstick reading equal to or more than 1+. Diabetes was defined as FBG ≥7.0 mmol/L, a self-reported physician diagnosis, or the self-reported use of antidiabetic medications (22, 23). Cardiovascular disease was defined as myocardial infarction, heart failure and atrial fibrillation.
Statistical analysis
The differences in baseline characteristics by SBP-TTR Group were examined with ANOVA for continuous variables and x2 test for categorical variables. The cumulative incidence of endpoint events among different groups was calculated using the Kaplan-Meier method, with inter-group comparisons made using the Log-Rank test. A multivariable-adjusted Cox proportional hazards model was employed to analyze the association of SBP-TTR with the risk of stroke and its subtypes in participants with diabetes and without diabetes. The model adjusted for confounders including age, sex (men, women), smoking status (no, current smokers), drinking status (no, current drinkers), education levels (low, high), physically active (yes, no), BMI, eGFR, TC, baseline SBP, and use of antihypertensive and lipid-lowering medications (yes, no). For participants with diabetes, the models were additionally adjusted for glucose-lowering drugs. We also examined the association between each 10% increase in SBP-TTR and the risk of stroke. Interactions between diabetes status and SBP-TTR on stroke were evaluated including multiplicative interaction terms in the model. Restricted cubic spline models were used to examine the dose-response relationship between SBP-TTR and stroke among individuals with diabetes and without diabetes, with three knots placed at 25th, 50th, and 75th percentiles.
Subgroup analyses were performed based on age (≤60 years, >60 years), sex (men, women), smoking status (no or current smokers), drinking status (no or current drinkers), baseline SBP (<140mmHg, ≥140mmHg) and BMI (<25 kg/m², ≥25 kg/m²). Sensitivity analyses were conducted to ensure the robustness of the results. First, individuals with a follow-up period of 2 years or less were excluded to mitigate the potential reverse causality. Second, the SBP target range was narrowed to 110–130 mmHg. Third, we applied a uniform 6-year SBP-TTR observation window for participants enrolled in 2006 or 2008 to assess the robustness of our findings. Fourth, we further adjusted for proteinuria in the full model. Fifth, given the potential inaccuracies of self-reported data (n=251), diabetes was defined solely based on FBG. Sixth, considering the competing risk of death, Fine-Gray models were used to assess the associations of SBP-TTR and stroke.
All data analyses were conducted using SAS 9.4 (SAS Institute, Cary, North Carolina) and R 4.2 (R Foundation for Statistical Computing) statistical software. In this study, statistical significance was indicated as p<0.05 (two-sided test).
Results
Baseline characteristics
A total of 28,591 participants were included in the analysis. The majority of participants had an SBP-TTR of 0% to 25% (42.1%), followed by 75% to 100% (24.2%), 25% to 50% (17.3%), and 50% to 75% (16.4%). Table 1 presents the distribution of baseline characteristics across different SBP-TTR groups among participants with and without diabetes. In general, individuals with higher SBP-TTR were younger, and more likely to be men, current smokers, current drinkers, and higher educated. They also tended to have a lower level of BMI, SBP and DBP, and a lower proportion of receiving antihypertensive drugs treatment.
Among our study population, 6,624 individuals had diabetes with an average age of 59.1 ± 9.7 years, of whom 5,458 (82.4%) were men. The mean number of BP measurements was 3.4 times. The remaining 21,967 participants were without diabetes, with an average age of 57.0 ± 11.0 years, and 18,512 (84.3%) were men. Compared to participants without diabetes, those with diabetes tended to be older and were more likely to be women. They also had lower education levels, higher BMI and FBG levels. Additionally, a higher percentage of participants with diabetes were receiving antihypertensive and lipid-lowering drug treatments.
Association between SBP-TTR and stroke
During the mean ± SD follow-up duration of 8.7 ± 1.8 years, we documented 2,206 incident stroke events (2,003 ischemic stroke, and 275 hemorrhagic stroke). The stroke incidence rates per 1,000 person-years across the SBP-TTR categories of 0% to 25%, 25% to 50%, 50% to 75%, and 75% to 100% were 15.35, 14.02, 11.86 and 7.64 for participants with diabetes, and 11.97, 8.37, 6.58, and 4.83, for participants without diabetes (Table 2). Supplementary Figure S1 shows significant differences in the cumulative incidence of stroke among participants with or without diabetes (log-rank test, P< 0.001).
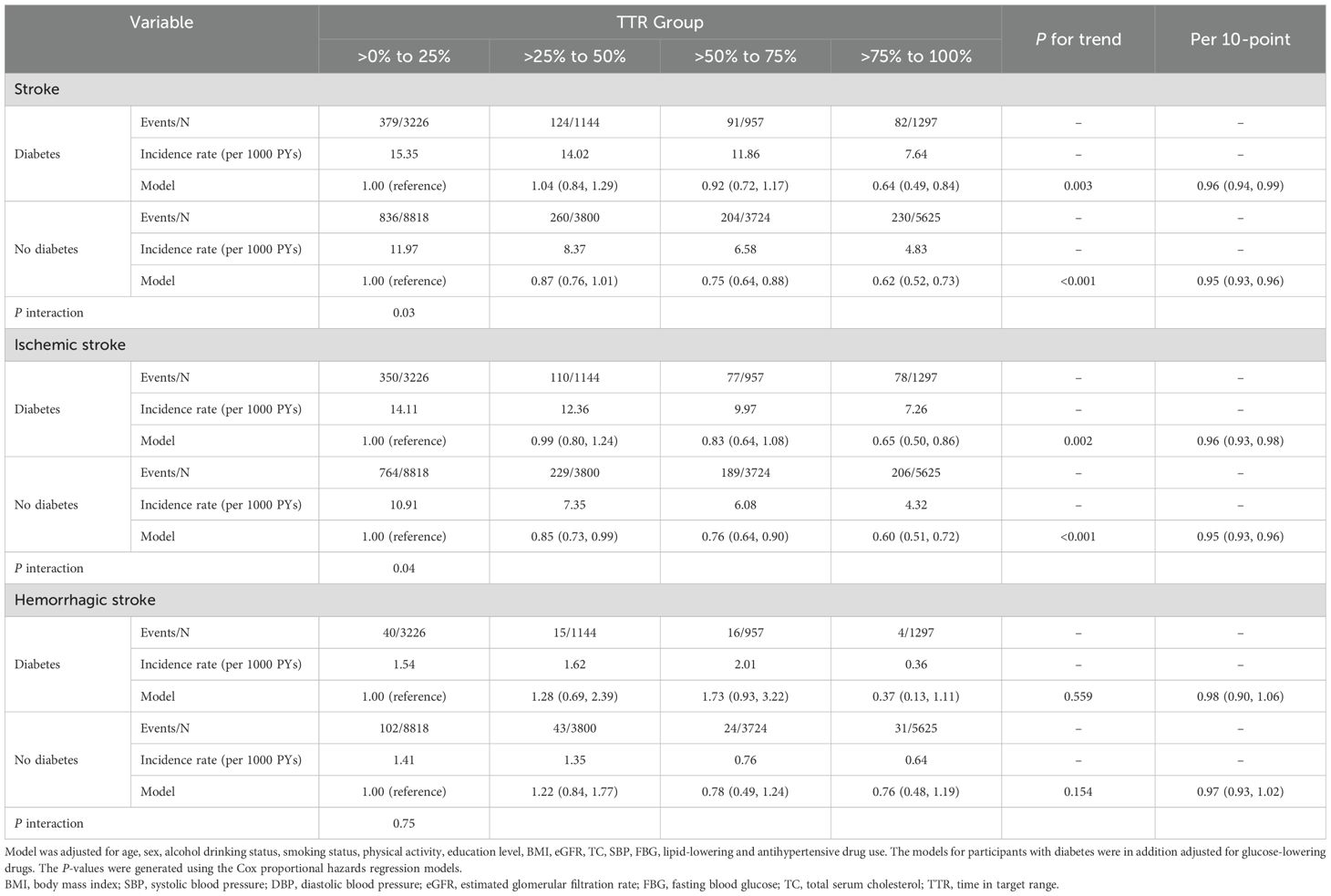
Table 2. HR (95% CI) of stroke according to SBP-TTR in individuals with or without diabetes (n=28,591).
Among individuals with diabetes, after adjusting for potential confounders, compared to those with 0%<SBP-TTR ≤ 25%, HRs (95% CIs) for stroke were 1.04 (0.84, 1.29) for 25%<SBP-TTR ≤ 50%, 0.92 (0.72, 1.17) for 50%<SBP-TTR ≤ 75%, and 0.64 (0.49, 0.84) for 75%<SBP-TTR ≤ 100%. In those without diabetes, the HRs (95% CIs) for stroke were 0.87 (0.76, 1.01), 0.75 (0.64, 0.88), and 0.62 (0.52, 0.73), respectively. Moreover, we identified a significant interaction between diabetes and SBP-TTR in the risk of stroke (P for interaction= 0.03). The relationship between SBP-TTR and stroke risk is non-linear among participants with diabetes (P for non-linearity = 0.001), showing maintaining SBP within the target range for a larger percentage of time (beyond 75%) was significantly associated with reduced stroke risk. In contrast, the relationship is linear among participants without diabetes (P for non-linearity = 0.355; Figure 2). Additionally, the protective effect of increased SBP-TTR was consistent when using ischemic stroke as the outcome (P for interaction= 0.04). However, no significant association was observed between SBP-TTR and hemorrhagic stroke events in either group (Table 2).
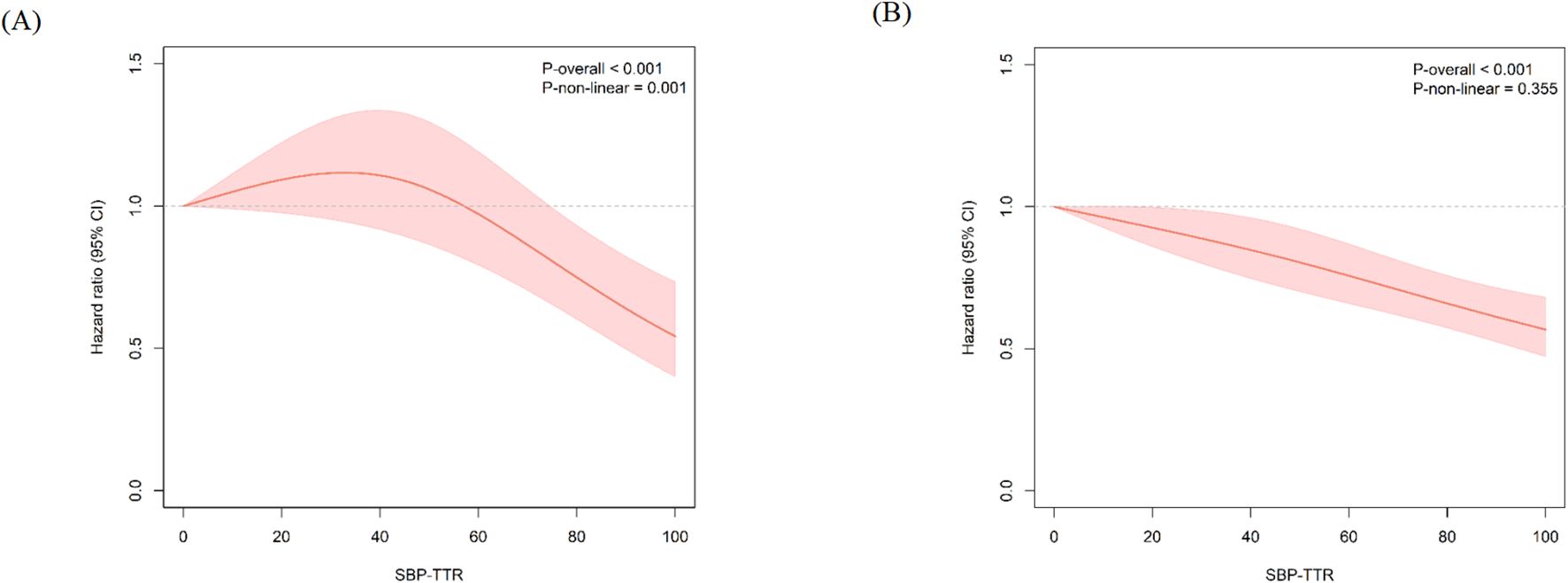
Figure 2. Dose-response association between the SBP-TTR range and risk of stroke using restricted cubic spline. Cox regression models with restricted cubic splines were fitted to the data with SBP-TTR. The solid line represents the point estimate of SBP-TTR correlation with the risk of stroke in individuals with (A) or without (B) diabetes, and the shaded part represents the 95% CI estimate. Covariates in the model include age, sex, alcohol drinking status, smoking status, physical activity, education level, BMI, eGFR, TC, SBP, FBG, lipid-lowering and antihypertensive drug use. The models for participants with diabetes were in addition adjusted for glucose-lowering drugs. BMI, body mass index; SBP, systolic blood pressure; DBP, diastolic blood pressure; eGFR, estimated glomerular filtration rate; FBG, fasting blood glucose; TC, total serum cholesterol; TTR, time in target range.
Secondary analyses and subgroup analyses
Stratified analysis showed that the association between SBP-TTR and risk of stroke was more prominent among the younger adults among participants without diabetes (P for interaction <0.01). Among individuals with diabetes, SBP-TTR exhibited a stronger protective effect in men (P for interaction = 0.05). Additionally, interactions were not significant when stratified by smoking status, alcohol consumption, SBP and BMI in participants with or without diabetes (Supplementary Table S2). The robustness of these findings is further supported by the consistency of results when excluding participants with follow-up durations of less than two years. Additionally, strengthening BP reduction to 110–130 mmHg indicated a stronger association between TTR and stroke risk. When applying a uniform 6-year SBP-TTR observation window for participants enrolled in 2006 or 2008, the results remained consistent with the primary findings. After adjusting for proteinuria in the model, using FBG to define diabetes, and using Fine-Gray models, the results remained consistent with the primary findings (Supplementary Tables S3-S8).
Discussion
Using a large cohort study of Chinese, we found that a higher SBP-TTR was associated with a lower risk of stroke in both participants with diabetes and without diabetes, with significant interactions suggesting a stronger protective effect in individuals without diabetes. Similar patterns were found for ischemic stroke but not for hemorrhagic stroke. Additionally, among individuals with diabetes, the protective effect of SBP-TTR was more pronounced in men than in women.
Hypertension stands as the foremost risk factor for stroke (24, 25), with prior studies primarily focusing on evaluating and monitoring the efficacy of BP management through single BP measurements or mean BP values. SBP is influenced by multiple factors and internal regulatory mechanisms, existing within a dynamic equilibrium. Therefore, it is imperative that we adopt a more comprehensive approach to consider the temporal variations in BP. Compared to traditional indicators, SBP-TTR better reflects the dynamic fluctuations of BP, and accounts for changes in BP trends over a period, thereby more accurately assessing the efficacy of BP management. Previous studies have indicated that higher SBP-TTR are associated with lower risk of cardiovascular disease (15, 26, 27). However, whether different SBP-TTR targets are needed for participants with diabetes is limited.
In agreement with previous studies, our data showed that a higher SBP-TTR was associated with a lower risk of stroke in both participants with diabetes and without diabetes, yet none of which has further stratified the analysis based on diabetes status to explore whether the protective effects exhibit any differences. Previous studies have widely recognized BP control as established strategy for reducing the risk of stroke (28). Per 10 mm Hg reduction in SBP significantly reduced the risk of stroke by an estimated 27% (29). The UK Prospective Diabetes Study (UKPDS) results showed that strict BP control in participants with diabetes and hypertension can reduce the risk of stroke by 44% (30). SBP-TTR serves as an indicator for assessing long-term BP management. Buckley et al.’s study results demonstrated a significant relationship between SBP-TTR and cardiovascular risk in individuals with diabetes and without diabetes (31). Additionally, SPRINT study and ACCORD study, which focused on individuals with hypertension alone and those with hypertension and diabetes, respectively, also demonstrated that increased SBP-TTR was associated with a reduced risk of stroke (15, 16).
Moreover, our data further demonstrated that the protective effect of SBP-TTR was stronger in individuals without diabetes. Among individuals with diabetes, SBP-TTR needs to be maintained above 75% to achieve a notable protective effect against stroke. A similar result was found in a meta-analysis of 51 randomized trials, which showed that antihypertensive treatment can reduce cardiovascular risk in participants with diabetes, although the effects were weaker than those without diabetes (32). Of note, the present study implied that a linear association between SBP-TTR and risk of stroke in people without diabetes, consistent with prior studies (15, 31). Conversely, among those with diabetes, this association deviates from linearity. This divergence is potentially attributable to the observation that individuals with diabetes and SBP-TTR exceeding 75% exhibit significantly lower baseline SBP and FBG levels compared to those with SBP-TTR below 75%. Additionally, diabetes may induce comorbidities such as vascular damage and autonomic neuropathy, which present challenges in BP management (33, 34). This study also found a significant protective effect of SBP-TTR in male but not female participants with diabetes. This sex difference may be attributed to greater arterial stiffness, more pronounced microvascular dysfunction, and a higher stroke risk in women with diabetes, which could attenuate the protective impact of BP control (35, 36). Our finding has several important clinical implications. First, it highlights the need for different strategies in BP management for participants with and without diabetes. For patients with diabetes, the goal should be to maintain SBP-TTR above 75% to significantly reduce the risk of stroke. Second, since SBP-TTR reflects long-term BP control and variability, clinicians should focus more on long-term monitoring rather than single BP measurements. This implies that regular BP monitoring and adjustments to treatment plans based on BP trends over time are essential in daily clinical practice. Finally, patients with diabetes should be treated as a high-risk group for more stringent BP control. Clinicians should stratify patients based on their individual risk factors and implement personalized and precise BP management strategies to enhance clinical outcomes.
Diabetes and hypertension frequently coexist, sharing considerable overlap in their etiologies and mechanisms, with a bidirectional pathogenic relationship (5, 37). Hypertension usually occurs before diabetes and the population base of individuals with hypertension is much larger than that of individuals with both hypertension and diabetes (37, 38). Therefore, strategies to improve SBP-TTR levels in individuals with hypertension can not only reduce the risk of diabetes but also lower the risk of stroke, achieving dual health benefits and greater societal impact. Significantly extending SBP-TTR is essential for achieving a marked protective effect against stroke among participants with diabetes. Furthermore, our findings demonstrated that regardless of whether the baseline SBP was <140 mmHg or ≥140 mmHg, higher SBP-TTR levels were associated with lower stroke risk in both groups. Proactive BP management to extend SBP-TTR should be pursued regardless of an individual’s BP levels to reduce the risk of stroke. Additionally, we further categorized stroke into ischemic and hemorrhagic subtypes, observed that SBP-TTR was significantly associated with ischemic stroke but not with hemorrhagic stroke. This may be attributed to the relatively small number of hemorrhagic stroke cases in our study and the fact that SBP-TTR primarily reflects long-term BP control rather than acute fluctuations. Given that hemorrhagic stroke is more closely associated with sudden and extreme BP elevations, this could explain the lack of a significant association. This highlights the necessity of considering the distinct pathologies and underlying mechanisms of different stroke types in public health strategies and clinical practice. Personalized prevention and management approaches should be adopted for different stroke subtypes to achieve optimal cost-effectiveness.
This is among the first large-scale prospective studies investigating the impact of SBP-TTR on stroke in participants with and without diabetes. Other strengths of this study include its large sample size, relatively long follow-up duration, and standardized BP measurements. However, our study has several limitations. First, the study participants were employees of a specific corporation, and predominantly men, which may limit the generalizability of the findings to other populations with different social backgrounds. Second, we diagnosed diabetes using FBG level, self-reported disease and therapy history, without assessing oral glucose tolerance tests or levels of hemoglobin A1c, which may underestimate the incidence of diabetes. Third, our study used office BP measurements to assess SBP-TTR, rather than home or ambulatory BP monitoring, which could introduce bias due to phenomena such as white-coat hypertension or masked hypertension. Fourth, our study did not differentiate between ischemic stroke subtypes, which may have differing underlying mechanisms and risk factor associations. Future research should further explore these subtypes, particularly lacunar infarction, as hypertension and diabetes are its primary risk factors (39). Fifth, A potential limitation of our study is that BP was measured every two years, which may not fully capture short-term fluctuations. However, SBP-TTR was estimated using linear interpolation, consistent with prior studies. Given the large-scale design and extended follow-up period of this real-world study, conducting more frequent BP measurements would have been challenging. Despite this limitation, our findings provide important evidence on the impact of SBP-TTR on stroke risk. Finally, as this study is observational in nature, causality between SBP-TTR and the risk of stroke cannot be established.
In conclusion, the Kailuan study highlights the critical role of SBP-TTR in reducing stroke risk. While stringent BP control is particularly important for individuals with diabetes, our findings also indicate that a higher SBP-TTR is associated with greater protective effects in individuals without diabetes. This underscores the importance of optimal BP control for all individuals, regardless of diabetes status. These results have significant implications for clinical practice, suggesting that tailored BP management strategies that maximize SBP-TTR could reduce the risk of stroke across different risk groups. Future research is required to validate our findings and elucidate the exact mechanisms underlying the association between SBP-TTR and stroke risk in individuals with and without diabetes.
Data availability statement
The original contributions presented in the study are included in the article/Supplementary Material. Further inquiries can be directed to the corresponding author/s.
Ethics statement
The studies involving humans were approved by the Ethics Committee of Kailuan General Hospital. The studies were conducted in accordance with the local legislation and institutional requirements. The participants provided their written informed consent to participate in this study.
Author contributions
YuY: Data curation, Writing – original draft, Writing – review & editing. SW: Methodology, Writing – original draft, Writing – review & editing. JZ: Methodology, Validation, Writing – original draft. YaL: Methodology, Software, Writing – original draft. MY: Investigation, Software, Writing – original draft. ZH: Investigation, Writing – original draft. SC: Data curation, Investigation, Writing – original draft. GW: Data curation, Investigation, Writing – original draft. YoX: Investigation, Writing – original draft. YW: Investigation, Writing – original draft. YuL: Investigation, Writing – original draft. JD: Investigation, Writing – original draft. TG: Methodology, Supervision, Writing – original draft, Writing – review & editing. HZ: Funding acquisition, Methodology, Writing – original draft, Writing – review & editing.
Funding
The author(s) declare that financial support was received for the research and/or publication of this article. The Liaoning Province Key Research and Development Joint Project (grant number: 2020JH 2/10300154).
Acknowledgments
We thank all the members of the Kailuan Study for their contribution and the participants who contributed their data.
Conflict of interest
The authors declare that the research was conducted in the absence of any commercial or financial relationships that could be construed as a potential conflict of interest.
The author(s) declared that they were an editorial board member of Frontiers, at the time of submission. This had no impact on the peer review process and the final decision.
Generative AI statement
The author(s) declare that no Generative AI was used in the creation of this manuscript.
Publisher’s note
All claims expressed in this article are solely those of the authors and do not necessarily represent those of their affiliated organizations, or those of the publisher, the editors and the reviewers. Any product that may be evaluated in this article, or claim that may be made by its manufacturer, is not guaranteed or endorsed by the publisher.
Supplementary material
The Supplementary Material for this article can be found online at: https://www.frontiersin.org/articles/10.3389/fendo.2025.1537343/full#supplementary-material
Abbreviations
ACCORD, action to control cardiovascular risk in diabetes-blood pressure; BPROAD, Blood Pressure Control Target in Diabetes trial; BMI, body mass index; CI, confidence interval; DBP, diastolic blood pressure; eGFR, estimated glomerular filtration rate; FBG, fasting blood glucose; HR, hazard ratio; SBP, systolic blood pressure; SD, standard deviation; SPRINT, systolic blood pressure intervention trial; TC, total serum cholesterol; TTR, time in target range.
References
1. Feigin VL, BAS, Johnson CO, Roth GA, Bisignano C, Abady GG. Global, regional, and national burden of stroke and its risk factors, 1990-2019: a systematic analysis for the Global Burden of Disease Study 2019. Lancet Neurol. (2021) 20:795–820. doi: 10.1016/s1474-4422(21)00252-0
2. Ma Q, Li R, Wang L, Yin P, Wang Y, Yan C, et al. Temporal trend and attributable risk factors of stroke burden in China, 1990-2019: an analysis for the Global Burden of Disease Study 2019. Lancet Public Health. (2021) 6:e897–906. doi: 10.1016/S2468-2667(21)00228-0
3. Benjamin EJ, Blaha MJ, Chiuve SE, Cushman M, Das SR, Deo R, et al. Heart disease and stroke statistics-2017 update: A report from the American heart association. Circulation. (2017) 135:e146–603. doi: 10.1161/CIR.0000000000000485
4. Kalyani CPC RR, Cherrington AL, Coustan DR, de Boer IH, Feldman H. Cardiovascular disease and risk management. Diabetes Care. (2017) 40:S75–s87. doi: 10.2337/dc17-S012
5. Sun D, Zhou T, Heianza Y, Li X, Fan M, Fonseca VA, et al. Type 2 diabetes and hypertension. Circ Res. (2019) 124:930–7. doi: 10.1161/CIRCRESAHA.118.314487
6. Huang XB, Tang WW, Liu Y, Hu R, Ouyang LY, Liu JX, et al. Prevalence of diabetes and unrecognized diabetes in hypertensive patients aged 40 to 79 years in southwest China. PloS One. (2017) 12:e0170250. doi: 10.1371/journal.pone.0170250
7. Zhang YQ, Li Y, Dong YG, Wu YH, Bian R, Li JH, et al. A nationwide assessment of blood pressure control and the associated factors in Chinese type 2 diabetes mellitus patients. J Clin Hypertens (Greenwich). (2019) 21:1654–63. doi: 10.1111/jch.v21.11
8. Hu G, Sarti C, Jousilahti P, Peltonen M, Qiao Q, Antikainen R, et al. The impact of history of hypertension and type 2 diabetes at baseline on the incidence of stroke and stroke mortality. Stroke. (2005) 36:2538–43. doi: 10.1161/01.STR.0000190894.30964.75
9. Gorelick PB, Whelton PK, Sorond F, Carey RM. Blood pressure management in stroke. Hypertension. (2020) 76:1688–95. doi: 10.1161/HYPERTENSIONAHA.120.14653
10. Mancia G, Kreutz R, Brunström M, Burnier M, Grassi G, Januszewicz A, et al. 2023 ESH Guidelines for the management of arterial hypertension The Task Force for the management of arterial hypertension of the European Society of Hypertension: Endorsed by the International Society of Hypertension (ISH) and the European Renal Association (ERA). J Hypertens. (2023) 41:1874–2071. doi: 10.1097/HJH.0000000000003480
11. Arboix A, Milian M, Oliveres M, García-Eroles L, Massons J. Impact of female gender on prognosis in type 2 diabetic patients with ischemic stroke. Eur Neurol. (2006) 56:6–12. doi: 10.1159/000094249
12. Cushman WC, Evans GW, Byington RP, Goff DC Jr., Grimm RH Jr., Cutler JA, et al. Effects of intensive blood-pressure control in type 2 diabetes mellitus. N Engl J Med. (2010) 362:1575–85. doi: 10.1056/NEJMoa1001286
13. Bi Y, Li M, Liu Y, Li T, Lu J, Duan P, et al. Intensive blood-pressure control in patients with type 2 diabetes. N Engl J Med. (2024) 392(12):1155-67. doi: 10.1056/NEJMoa2412006
14. Bakris G, Sternlicht H. Time in therapeutic range: redefining “Optimal” Blood pressure control. J Am Coll Cardiol. (2021) 77:1300–1. doi: 10.1016/j.jacc.2021.01.019
15. Fatani N, Dixon DL, Van Tassell BW, Fanikos J, Buckley LF. Systolic blood pressure time in target range and cardiovascular outcomes in patients with hypertension. J Am Coll Cardiol. (2021) 77:1290–9. doi: 10.1016/j.jacc.2021.01.014
16. Cheng Y, Wang D, Yang Y, Miao Y, Shen WL, Tian J, et al. Diastolic and systolic blood pressure time in target range as a cardiovascular risk marker in patients with type 2 diabetes: A post hoc analysis of ACCORD BP trial. Diabetes Res Clin Pract. (2023) 203:110831. doi: 10.1016/j.diabres.2023.110831
17. Wang A, Tian X, Zuo Y, Chen S, Meng X, Wu S, et al. Change in triglyceride-glucose index predicts the risk of cardiovascular disease in the general population: a prospective cohort study. Cardiovasc Diabetol. (2021) 20:113. doi: 10.1186/s12933-021-01305-7
18. Huo Z, Huang Z, Feng J, Li J, Chen S, Wang G, et al. Life’s Essential 8 and heart failure among patients with chronic kidney disease: the Kailuan Cohort Study. Eur J Prev Cardiol. (2024) 31:824–31. doi: 10.1093/eurjpc/zwad398
19. Schmitt L, Speckman J, Ansell J. Quality assessment of anticoagulation dose management: comparative evaluation of measures of time-in-therapeutic range. J Thromb Thrombol. (2003) 15:213–6. doi: 10.1023/B:THRO.0000011377.78585.63
20. Jin C, Chen S, Vaidya A, Wu Y, Wu Z, Hu FB, et al. Longitudinal change in fasting blood glucose and myocardial infarction risk in a population without diabetes. Diabetes Care. (2017) 40:1565–72. doi: 10.2337/dc17-0610
21. Levey AS, Stevens LA, Schmid CH, Zhang YL, Castro AF 3rd, Feldman HI, et al. A new equation to estimate glomerular filtration rate. Ann Intern Med. (2009) 150:604–12. doi: 10.7326/0003-4819-150-9-200905050-00006
22. Sattar N, Rawshani A, Franzén S, Rawshani A, Svensson AM, Rosengren A, et al. Age at diagnosis of type 2 diabetes mellitus and associations with cardiovascular and mortality risks. Circulation. (2019) 139:2228–37. doi: 10.1161/CIRCULATIONAHA.118.037885
23. Fox CS, Golden SH, Anderson C, Bray GA, Burke LE, de Boer IH, et al. Update on prevention of cardiovascular disease in adults with type 2 diabetes mellitus in light of recent evidence: A scientific statement from the American heart association and the American diabetes association. Diabetes Care. (2015) 38:1777–803. doi: 10.2337/dci15-0012
24. Lawes CM, Vander Hoorn S, Rodgers A. Global burden of blood-pressure-related disease, 2001. Lancet. (2008) 371:1513–8. doi: 10.1016/S0140-6736(08)60655-8
25. Lawes CM, Rodgers A, Bennett DA, Parag V, Suh I, Ueshima H, et al. Blood pressure and cardiovascular disease in the Asia Pacific region. J Hypertens. (2003) 21:707–16. doi: 10.1097/00004872-200304000-00013
26. Lin Z, Xiao Z, Chen W, Xu W, Huang C, Xie J, et al. Association of long-term time in target range for systolic blood pressure with cardiovascular risk in the elderly: a Chinese veteran cohort study. Eur J Prev Cardiol. (2023) 30:969–77. doi: 10.1093/eurjpc/zwad083
27. Chung SC, Pujades-Rodriguez M, Duyx B, Denaxas SC, Pasea L, Hingorani A, et al. Time spent at blood pressure target and the risk of death and cardiovascular diseases. PloS One. (2018) 13:e0202359. doi: 10.1371/journal.pone.0202359
28. Gaciong Z, Siński M, Lewandowski J. Blood pressure control and primary prevention of stroke: summary of the recent clinical trial data and meta-analyses. Curr Hypertens Rep. (2013) 15:559–74. doi: 10.1007/s11906-013-0401-0
29. Ettehad D, Emdin CA, Kiran A, Anderson SG, Callender T, Emberson J, et al. Blood pressure lowering for prevention of cardiovascular disease and death: a systematic review and meta-analysis. Lancet. (2016) 387:957–67. doi: 10.1016/S0140-6736(15)01225-8
30. Turner RH R, Stratton I, Cull C, Frighi V, Manley S, et al. Tight blood pressure control and risk of macrovascular and microvascular complications in type 2 diabetes: UKPDS 38. UK Prospective Diabetes Study Group. Bmj. (1998) 317:703–13.
31. Buckley LF, Baker WL, Van Tassell BW, Cohen JB, Alkhezi O, Bress AP, et al. Systolic blood pressure time in target range and major adverse kidney and cardiovascular events. Hypertension. (2023) 80:305–13. doi: 10.1161/HYPERTENSIONAHA.122.20141
32. Nazarzadeh M, Bidel Z, Canoy D, Copland E, Bennett DA, Dehghan A, et al. Blood pressure-lowering treatment for prevention of major cardiovascular diseases in people with and without type 2 diabetes: an individual participant-level data meta-analysis. Lancet Diabetes Endocrinol. (2022) 10:645–54. doi: 10.1016/S2213-8587(22)00172-3
33. Eckel RH, Bornfeldt KE, Goldberg IJ. Cardiovascular disease in diabetes, beyond glucose. Cell Metab. (2021) 33:1519–45. doi: 10.1016/j.cmet.2021.07.001
34. Mosenzon O, Cheng AY, Rabinstein AA, Sacco S. Diabetes and stroke: what are the connections? J Stroke. (2023) 25:26–38. doi: 10.5853/jos.2022.02306
35. Angoulvant D, Ducluzeau PH, Renoult-Pierre P, Fauchier G, Herbert J, Semaan C, et al. Impact of gender on relative rates of cardiovascular events in patients with diabetes. Diabetes Metab. (2021) 47:101226. doi: 10.1016/j.diabet.2021.101226
36. Wannamethee SG, Papacosta O, Lawlor DA, Whincup PH, Lowe GD, Ebrahim S, et al. Do women exhibit greater differences in established and novel risk factors between diabetes and non-diabetes than men? The British Regional Heart Study and British Women’s Heart Health Study. Diabetologia. (2012) 55:80–7. doi: 10.1007/s00125-011-2284-4
37. Ohishi M. Hypertension with diabetes mellitus: physiology and pathology. Hypertens Res. (2018) 41:389–93. doi: 10.1038/s41440-018-0034-4
38. Sun H, Saeedi P, Karuranga S, Pinkepank M, Ogurtsova K, Duncan BB, et al. IDF Diabetes Atlas: Global, regional and country-level diabetes prevalence estimates for 2021 and projections for 2045. Diabetes Res Clin Pract. (2022) 183:109119. doi: 10.1016/j.diabres.2021.109119
Keywords: diabetes, Kailuan study, stroke, systolic blood pressure, time in target range
Citation: Yang Y, Wu S, Zhang J, Liu Y, Yin M, Huo Z, Chen S, Wang G, Xiao Y, Wang Y, Li Y, Deng J, Geng T and Zhang H (2025) Time in target range for systolic blood pressure and stroke in people with and without diabetes: the Kailuan prospective cohort study. Front. Endocrinol. 16:1537343. doi: 10.3389/fendo.2025.1537343
Received: 30 November 2024; Accepted: 22 April 2025;
Published: 14 May 2025.
Edited by:
Åke Sjöholm, Gävle Hospital, SwedenReviewed by:
Elisa Gouvêa Bogossian, Université libre de Bruxelles, BelgiumGiuseppe Lisco, University of Bari Aldo Moro, Italy
Adria Arboix, Sacred Heart University Hospital, Spain
Copyright © 2025 Yang, Wu, Zhang, Liu, Yin, Huo, Chen, Wang, Xiao, Wang, Li, Deng, Geng and Zhang. This is an open-access article distributed under the terms of the Creative Commons Attribution License (CC BY). The use, distribution or reproduction in other forums is permitted, provided the original author(s) and the copyright owner(s) are credited and that the original publication in this journal is cited, in accordance with accepted academic practice. No use, distribution or reproduction is permitted which does not comply with these terms.
*Correspondence: Tingting Geng, Z2VuZ190aW5ndGluZ0BodXN0LmVkdS5jbg==; Hong Zhang, aG9uZ3poYW5nQGNtdS5lZHUuY24=
†These authors have contributed equally to this work