- 1Department of Reproductive Medicine, Binzhou Medical University Hospital, Binzhou, China
- 2Department of Obstetrics Medicine, Linquan County People’s Hospital, Fuyang, Anhui, China
- 3Obstetrics and Gynecology of Binzhou Medical University, Binzhou, Shandong, China
Diminished Ovarian Reserve (DOR) is a complex etiology disease that significantly impacts female fertility, endocrine function, and overall health status. In recent years, the incidence of DOR has been increasing, yet therapeutic methods remain relatively limited, particularly for patients with reproductive needs who often require Assisted Reproductive Technology (ART) treatments. Growth Hormone (GH), a peptide hormone secreted by the anterior pituitary, promotes growth in bones, viscera, and multiple organs and systems throughout the body, enhances protein synthesis, and influences fat and mineral metabolism, playing a crucial role in human growth and development. Its levels decrease with the aging of the organism. In recent years, studies have suggested that a decline in growth hormone levels may be one of the causes of decreased ovarian function, leading to the application of GH in assisted reproductive treatments for patients with DOR. An increasing body of research indicates that GH can improve ovarian function through mechanisms such as antioxidant stress, promotion of follicle development, and enhancement of oocyte quality, and it also shows potential to improve endometrial receptivity, making GH a promising safe and effective strategy in ART for DOR patients.
1 Introduction
DOR is characterized by a reduction in the number and/or quality of oocytes, leading to insufficient ovarian function, sex hormone deficiency, and decreased fertility. Auxiliary examinations reveal decreased levels of anti-Mullerian hormone (AMH), reduced antral follicle count (AFC) (AMH < 1.1 ng/ml or AFC < 7), and elevated follicular stimulating hormone (FSH) levels (1). A subset of DOR patients suffer from premature ovarian insufficiency (POI) (2). Studies indicate that the prevalence of DOR in the population is approximately 0.9% to 3%, with incidence rates before the ages of 20, 30, and 40 being 0.01%, 0.1%, and 1%, respectively, showing a trend of increasing prevalence and a shift towards younger ages (3). Among women undergoing assisted reproductive technology treatments, the prevalence of DOR is 19% to 26% (4). The etiology of DOR is complex and not fully understood, but it may be related to aging, autoimmune conditions, genetic factors, iatrogenic damage, lifestyle habits, environmental factors, and psychological influences (1). Compared to other organs, the ovaries show more pronounced age-related changes, with a decrease in follicle number, accelerated follicle atresia, and reduced follicle quality, accompanied by a lower probability of natural pregnancy, making it a significant cause of female infertility (5). Therefore, delaying or intervening in the onset of DOR is crucial for improving female fertility rate. In recent years, clinical research on the application of GH has gradually expanded from pediatric growth and development to adult diseases. Studies have shown that GH can delay ovarian aging and effectively treat DOR patients, increasing the success rate of assisted reproductive technologies (6). This article provides a comprehensive review of the application and research advancements of GH in DOR, POI, and its terminal stage, Premature Ovarian Failure (POF).
2 An overview of GH and its receptor
GH is a polypeptide synthesized and secreted by the acidophilic cells of the anterior pituitary gland, predominantly secreted during nocturnal sleep. Comprising 191 amino acids (7), GH is regulated by positive and negative feedback mechanisms involving growth hormone-releasing hormone (GHRH) and somatostatin (SS), respectively. GHRH, produced by the hypothalamus, stimulates the synthesis and release of GH by the acidophilic cells of the anterior pituitary, while SS, also hypothalamic in origin, inhibits GH secretion through receptor binding on these cells, as shown in Figure 1. In most instances, GH released by the anterior pituitary into the bloodstream promotes the synthesis and secretion of insulin-like growth factor-1 (IGF-1) in the liver, thereby exerting its physiological functions. The primary functions of GH include: (1) Promotion of growth and development: by stimulating the proliferation and differentiation of osteoblasts and chondrocytes, thereby increasing the growth velocity of long bones; (2) Regulation of the body’s material metabolism: facilitating the breakdown of triglycerides in adipose tissue; modulating the activity of lipoprotein lipase and the number of LDL receptors; enhancing protein synthesis and metabolism; increasing the absorption of skeletal minerals, thereby improving bone density and quality; (3) Modulation of physiological functions: augmenting mitochondrial ATP synthesis in muscle cells; adjusting myocardial contractility and skeletal muscle strength; promoting neural synaptic growth and cognitive function improvement; and elevating nitric oxide levels to increase sexual desire (8).
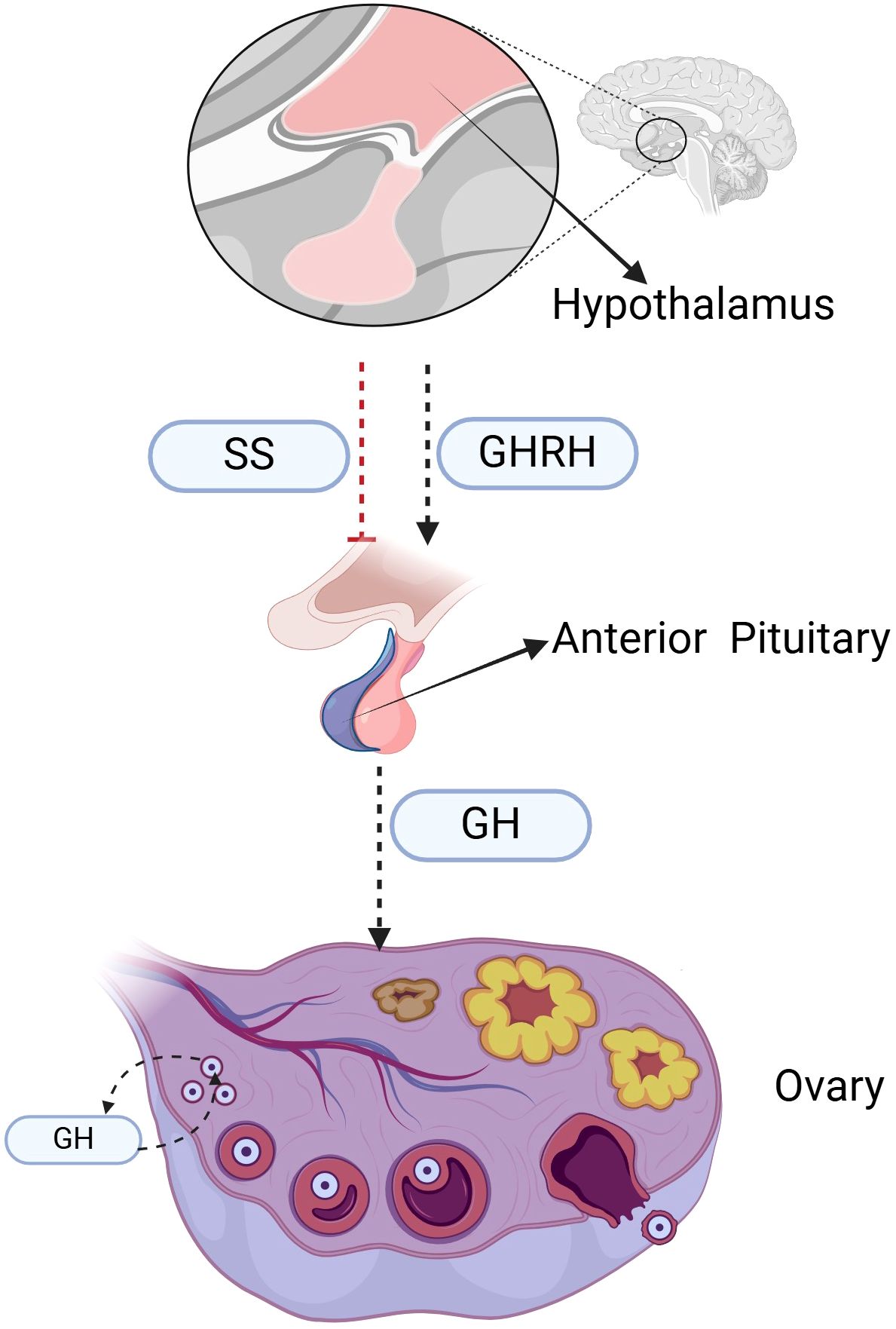
Figure 1. The role of GH in ovarian follicular development within the HPG Axis. The figure illustrates the macroscopic processes in the HPG axis, including the promotion of GH secretion by GHRH, the inhibition of GH secretion by SS, and the role of GH in follicle development. SS, somatostatin; GHRH, growth hormone releasing hormone; GH, growth hormone.
GH molecules can directly interact with two growth hormone receptor (GHR) molecules on the surface of target cells, simultaneously recognizing and binding to form a GH-GHR2 ternary complex, which activates various intracellular signaling proteins (JAK-STAT, MAPK, etc.), promoting mitosis and growth development (9). However, research has found that the primary biological role of pituitary-derived GH in the body is to act on GHRs on the surface of liver cells, stimulating hepatocytes to produce IGFs (IGF-1 and IGF-2) to exert its function. Among them, IGF-1 promotes the growth of bones, viscera, and the whole body, and stimulates cell proliferation; IGF-2 mainly regulates development during the perinatal period. Subsequent studies have shown that not only the pituitary gland can secrete GH, but female reproductive system organs and tissues such as the ovaries can also locally secrete GH (10, 11). Pituitary secretion of GH is pulsatile, while locally produced GH is characterized by continuous low concentration secretion in the locality, with paracrine and autocrine regulatory modes. GHRs have been identified in granulosa cells, theca cells, oocytes, and in testicular tissue, as well as in the glandular cells of the endometrium and decidua during the luteal phase. This distribution suggests that GH plays a significant role in maintaining the function of female reproductive organs and tissues, including the ovaries and uterus (12, 13). Figure 2 illustrates that GH synthesized by the ovary itself is produced on the endoplasmic reticulum (ER) and rapidly binds to GHR within the ER, promoting the maturation of GHR and forming a GH-GHR complex. These complexes then activate the JAK-STAT signaling pathway through the Golgi apparatus system. Once the complex moves to the cell surface, autocrine GH induces the JAK-STAT signaling pathway; however, signal transduction does not occur when GHRs are still within the ER. This process indicates that activation of the JAK-STAT pathway is not necessarily contingent upon binding to the extracellular domain of the cell membrane GHR (14, 15). The majority of patients with DOR are related to increasing age and disease onset. As age advances, there is a decline in the function of the adenohypophysis and ovaries, which inevitably leads to a decrease in reproductive function alongside a reduction in GH levels. This provides a theoretical basis for the therapeutic use of exogenous GH in the treatment of DOR and in the field of assisted reproduction.
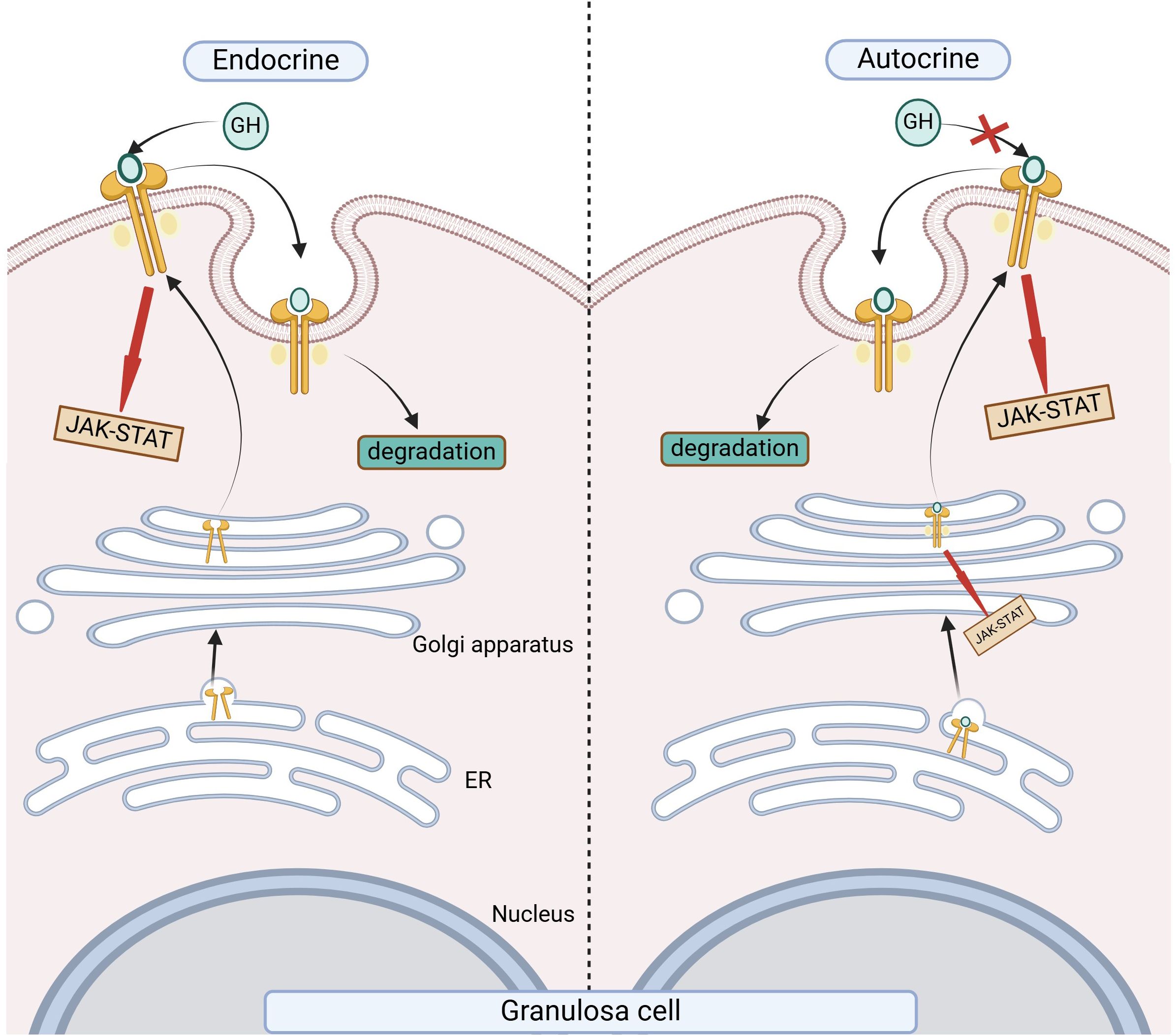
Figure 2. The Process of ovarian autocrine GH secretion. The figure illustrates the comparison between the autocrine and paracrine processes of growth hormone. ER, endoplasmic reticulum.
3 Mechanisms of DOR and the impact of GH on follicles
3.1 Pathophysiological mechanisms underlying the development and progression of DOR
The physiological processes through which the ovaries perform their reproductive functions primarily include follicular growth, maturation, ovulation, and corpus luteum formation. The development of primordial follicles to maturity involves four stages: primordial, primary, secondary, and mature follicles. The size of the primordial follicle pool represents the reserve of fertility potential, with early activation of primordial follicles determining the ovarian reserve function and serving as the foundation for follicular development. The vast majority of primordial follicles remain in a quiescent state, with only a small fraction initiating growth, eventually maturing and ovulating (16). Possible causes of DOR include depletion of the follicle pool, increased follicular atresia, and reduced sensitivity to gonadotropins (Gn), leading to a decline in ovarian function (17). Patients with DOR often exhibit excessive reactive oxygen species (ROS) production in ovarian tissue, leading to an imbalance between oxidation and antioxidation, which results in apoptosis of ovarian granulosa cells, mitochondrial dysfunction, inflammation, and telomere shortening (18). A prospective clinical study by Yan Huang et al. indicates that compared to individuals with normal ovarian reserve (NOR), DOR patients have significantly increased oxidative stress products and inflammatory markers in follicular fluid, along with reduced antioxidant status, suggesting that interventions targeting oxidative stress may potentially improve DOR (19).
3.2 Growth hormone-stimulated activation of primordial follicles and follicular development
The Hypothalamic-Pituitary-Ovarian (HPO) axis regulates the secretion of steroid hormones through Gn, thereby influencing follicular development. Patients with DOR exhibit elevated follicle-stimulating hormone (FSH), decreased estradiol (E2) and GH levels, and impaired follicular development, with GH capable of modulating these changes. Although pituitary-derived Gn are the primary regulators of ovarian steroidogenesis, in vitro studies have shown that GH can also promote follicular development and maturation, as well as ovulation, by binding to receptors on ovarian granulosa cells (GCs) and thecal cells (TC). Additionally, GH indirectly modulates the production of E2 and progesterone (P) by GCs through the stimulation of IGF-1 production in the liver and ovaries (11, 20). In bovine granulosa cells and luteinized human granulosa cells, growth hormone (GH) can increase the production of estradiol (E2) and progesterone, thereby promoting follicular development (15, 21). Additionally, during the early stages of follicular development, the activation of primordial follicles and their transformation into antral follicles is independent of Gn, with this process being modulated by various locally produced growth factors through paracrine or autocrine mechanisms (22). Saccon et al. employed GH-deficient Ames Dwarf (df/df) mice to block GH secretion at the pituitary level, leading to a significant increase in the number of primordial follicles compared to Normal (N/df) mice and wild-type mice. In contrast, the number of follicles in developmental stages and mature follicles markedly decreased. Reduced GH secretion is correlated with an increase in follicular atresia, a condition that can be reversed by the exogenous supplementation of GH. This indicates that GH may be involved in the activation of primordial follicles and plays a pivotal role in stimulating follicular development to the gonadotropin-dependent phase (23). In GHR gene-knockout mice, circulating GH levels are high while IGF-1 levels are low. Histological examination of the ovaries reveals an increase in the number of primordial or primary follicles and a decrease in the number of healthy and growing antral or preovulatory follicles. This suggests that in the absence of GH, follicles remain in the primordial stage (24). A previous animal experiment involved the use of transgenic mice expressing Bovine Growth Hormone (bGH) under the control of the phosphoenolpyruvate carboxykinase (PEPCK) promoter, which were observed alongside untreated normal mice under identical conditions for 15 days. It was found that the ovulation rate in transgenic mice was significantly increased. To further assess the impact of bGH on the ovulation rate in non-transgenic mice, an experimental group of non-transgenic mice was injected with either 0.75 mg bGH per day or 0.30 mg bGH per day (as a single dose or 0.15 mg twice daily) for three days. Compared to the control group, the ovulation rate increased in non-transgenic mice treated with 0.75 mg bGH per day, indicating that GH transgenic expression or administration of GH enhances the ovulation rate in mice (25). Additionally, GH may be essential for late follicular development, as GHR deficiency leads to complete inhibition of dominant follicle development in cattle (26). Furthermore, GH can promote the repair and generation of ovarian blood vessels by stimulating the production of vascular endothelial growth factor 1 (VEGF-1), and a richer blood supply is also a significant factor in promoting follicular growth (27). In summary, GH is indispensable in the growth and development of follicles.
3.3 GH mitigates ovarian oxidative stress, ameliorates mitochondrial dysfunction, and modulates cell apoptosis
Oxidative stress (OS) and apoptosis are closely associated with the development of DOR in busulfan/cyclophosphamide (Bu/Cy)-induced mouse ovary aging models (28). Apoptosis of oocytes and female germline stem cells (FGSCs) directly leads to a reduction in the number of germ cells, decreasing ovarian reserve and reproductive potential in infertility mice treated with Bu/Cy (29). Extensive apoptosis of GCs reduces the number of primordial follicles, impairs the reproductive capacity of FGSCs, and disrupts the metabolic homeostasis of the ovary, thereby damaging ovarian function on pig CGs (30). Studies have indicated that GCs apoptosis is closely related to the depletion of mitochondrial DNA; mitochondrial dysfunction disrupts the normal production of ATP and increases the levels of Reactive Oxygen Species (ROS), leading to pathological changes (31). Further research has found that an increase in ROS can disrupt mitochondrial membrane permeability. Enhanced mitochondrial membrane permeability may be a limiting factor in apoptosis; if it increases, causing mitochondrial matrix swelling, it leads to a decrease in mitochondrial membrane potential and the collapse of the inner membrane potential, a consequent decline in ATP generation, and ultimately, the release of cytochrome C (apoptotic factor) and cell apoptosis. The release of apoptotic factors can further promote an increase in ROS and mitochondrial damage (32). In aged ovaries, an overproduction of ROS leads to cellular structure damage (mitochondria, DNA, proteins, cell membranes, and ER) and cell apoptosis. Therefore, antioxidants may inhibit ovarian OS, improve mitochondrial function and cell apoptosis, thus preventing the occurrence and development of DOR (33). Although GH is not a direct antioxidant, it can intervene in the signaling pathways of cellular defense mechanisms against OS, exerting an antioxidant effect (34).
Wang et al. treated human ovarian granulosa cell line (KGN cells) with cisplatin to establish an in vitro model of GCs apoptosis and mitochondrial dysfunction. Following cisplatin treatment, cell proliferation was significantly inhibited, and the rate of apoptosis increased (P < 0.05). However, treatment with GH rescued cell proliferation (for details, see Figure 3), reduced the rate of apoptosis, decreased the ratio of Bax (pro-apoptotic protein) to Bcl-2 (anti-apoptotic protein) (P < 0.05), significantly lowered levels of abnormal ROS, and increased the levels of Sirt3 and Sod2 (antioxidant components), thereby alleviating OS. Additionally, GH was found to increase the mitochondrial membrane potential and the copy number of mitochondrial DNA (mtDNA) in GCs. This suggests that GH can mitigate OS, enhance mitochondrial function, and protect against cisplatin-induced apoptosis in GCs (35).
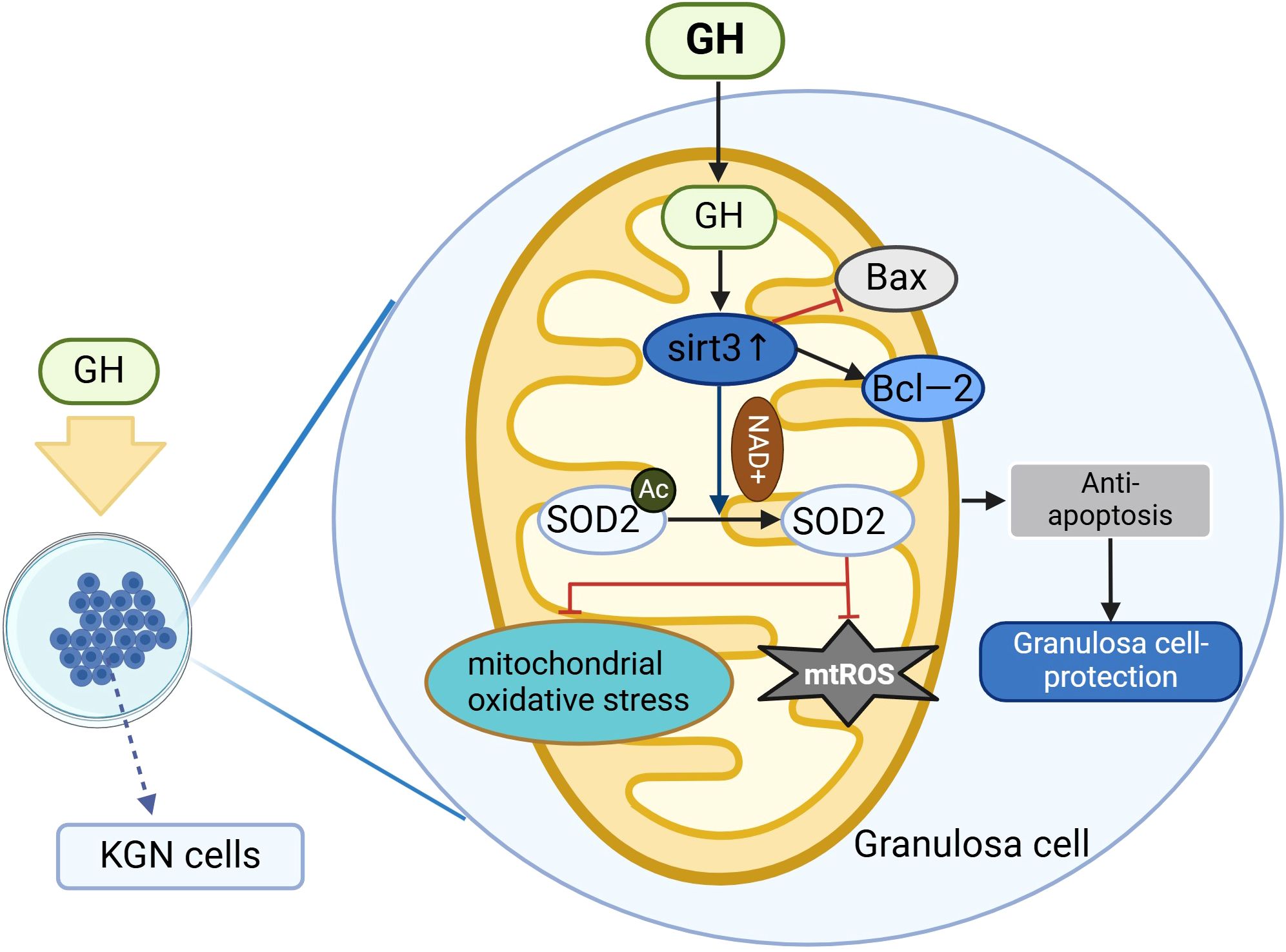
Figure 3. Mechanism of GH protection in GCs. The figure illustrates the regulation of oxidative and antioxidant processes by GH in the mitochondria of KGN cells. Bax, pro-apoptotic protein; Bcl-2, anti-apoptotic protein; mtROS: mitochondrial reactive oxygen species.
Studies have shown that cyclophosphamide (CTX) disrupts the microenvironment necessary for oocyte maturation by inducing GCs apoptosis, leading to sustained ovarian dysfunction and suppression of follicle development (36), as well as inducing the activation of pro-apoptotic genes, the production of ROS, and the depletion of glutathione (37). Feng et al. established a mouse model of DOR with CTX and intervened with recombinant human growth hormone (rhGH). The results indicated that high doses of rhGH increased the number of oocytes retrieved, effectively reduced GCs apoptosis, and mitigated ROS and OS induced by superoxides. Furthermore, rhGH regulated the energy metabolism of oocytes by modulating mitochondrial membrane potential and ATP content, but had no effect on the regulation of mitochondrial DNA (mtDNA) copy number. Single-cell transcriptome analysis revealed that rhGH directly or indirectly promoted the balance between oxidation and antioxidation (38). This suggests that the mechanism of GH treatment for DOR may be related to alleviating OS and mitochondrial damage, thereby maintaining and improving the normal development of follicles.
4 Signaling pathways associated with GH treatment for DOR
The mechanism of action of GH in the treatment of DOR involves multiple signaling pathways, which are crucial for follicular development, oocyte maturation, and the maintenance of ovarian function. The following are the main signaling pathways through which GH affects DOR, collectively regulating the complex biological processes within the ovary. The detailed mechanisms and interactions of specific signaling pathways can be visually presented through Figure 4.
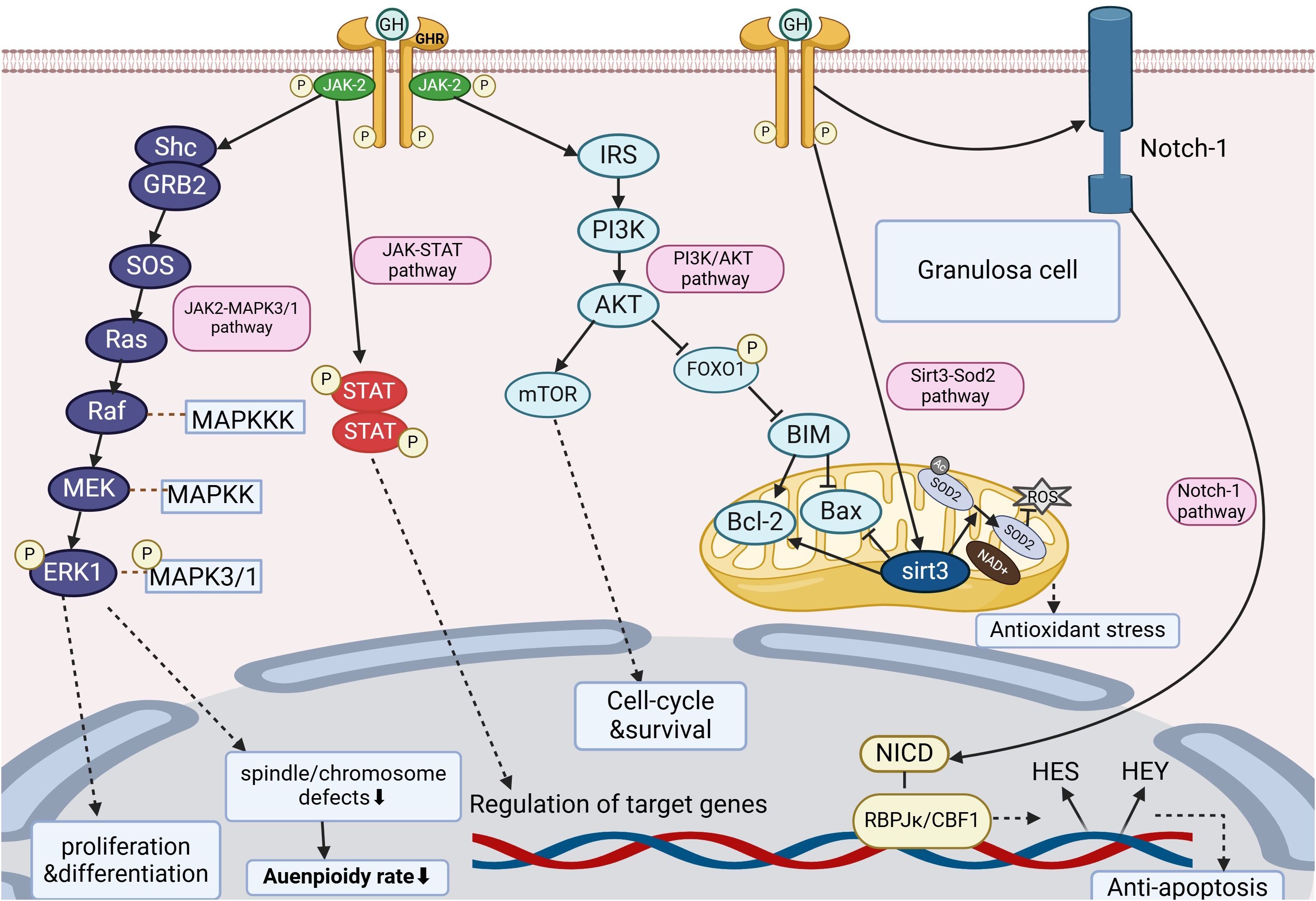
Figure 4. Signaling pathways associated with GH treatment for DOR. The figure illustrates the processes of each signaling pathway in granulosa cells, as well as the corresponding functions they exert.
4.1 JAK2-MAPK3/1 signaling pathway
Yonatan B et al. reported that the MAPK signaling pathway is activated during the resumption of meiosis in oocytes, regulating spindle assembly and cell cycle progression, and mediating the physiological effects of Gn in granulosa cells, promoting cumulus expansion, ovulation, and CL formation. It plays a key role in regulating meiotic processes and oocyte aging (39, 40). In mammalian oocytes, the activity of MAPK3/1 is crucial for the progression of the first meiotic division and maintaining the arrest at Metaphase II (MII) (41). Furthermore, increased expression of MAPK3/1 also promotes microtubule organization and spindle assembly during oocyte meiotic maturation (42, 43). Janus kinase 2 (JAK2), a member of the Janus kinase family, plays an important role in signal transduction of growth hormone (44). Additionally, another study pointed out that in mouse oocytes and early cleavage stage embryos, JAK2 is located at chromosome 9p24. Treatment of oocytes with the selective JAK2 inhibitor CEP-33779 was found to significantly reduce the efficiency of oocyte maturation, revealing that JAK2 has a critical role in the maturation process of oocytes (45).
Luo et al. ingeniously combined in vivo and in vitro studies to investigate the effects of GH on the quality of oocytes in aged mice and its potential link to the MAPK3/1 pathway. They selected 8-month-old aged mice as subjects and administered GH at a dose of 1.6 mg/kg via daily intraperitoneal injection for 8 weeks to simulate a long-term in vivo environment of GH supplementation. Concurrently, they collected germinal vesicle (GV) stage oocytes from aged mice and treated them with GH during the in vitro simulation of oocyte maturation. These experiments not only revealed that the decrease in p-MAPK3/1 levels in oocytes of aged mice is closely associated with an increase in aneuploidy rates but also suggested that elevating MAPK3/1 activity could be an effective strategy for improving oocyte quality. Furthermore, they found that GH supplementation significantly increased p-MAPK3/1 levels in aged oocytes and correspondingly reduced aneuploidy rates. This finding provides strong evidence for the application of GH in improving oocyte quality. Additionally, GH was able to partially reverse spindle/chromosome defects induced by MAPK3/1 inhibition, thereby enhancing the developmental potential of aged oocytes. The data from this study confirmed that the enhancing effect of GH on p-MAPK3/1 is closely related to the increased expression of p-JAK2 (46). In summary, Luo et al.’s research revealed the important role of the MAPK3/1 pathway in the reduction of aneuploidy in aging oocytes mediated by GH and emphasized the potential value of the JAK2-MAPK3/1 pathway in improving the quality of aged oocytes (46). These findings provide new directions for future research and hold promise for more effective treatment options for DOR patients.
4.2 Notch-1 signaling pathway
The Notch signaling pathway is an extremely important and complex network that regulates cell fate, proliferation, differentiation, and apoptosis, thereby playing a key role in tissue development, maintenance of adult homeostasis, and self-renewal of stem cells (47, 48). The Notch proteins are a family of receptors that regulate cell fate. These Notch receptors are activated upon direct contact with ligands on adjacent cells (48, 49). In mammals, there are four Notch receptors (NOTCH1-NOTCH4) and five ligands (Jagged-1 and -2, and Delta-like proteins 1, 3, and 4) (48, 50). NOTCH receptors possess extracellular, transmembrane, and intracellular domains (48, 49). RBPJκ is a key transcription factor in the canonical Notch signaling pathway, acting downstream of all four Notch receptors (49–51). Within the nucleus, the NICD (Notch receptor’s intracellular domain) forms a transcription-activating complex with RBPJκ/CBF1, playing a central role in the Notch signaling pathway (49–51). Activating the transcription of downstream target genes, such as the primarily activated target genes including HES (hairy/enhancer of split) and HEY (Hey-hairy/enhancer-of-split related with YRPW motif family members), which are basic helix-loop-helix (bHLH) family transcription factors. These transcription factors further promote the expression of downstream genes, affecting cell proliferation and inhibiting cell differentiation (51, 52). Additionally, it has been reported that the overexpression of Notch-1 inhibits apoptosis in various types of human cancers, indicating its potential as a therapeutic target for inhibiting cell apoptosis (49–52).
Liu et al. established a POF mouse model using CTX and observed changes in ovarian weight, hormone secretion, and the number of normal follicles in mice treated with recombinant murine growth hormone (rmGH). After treatment with rmGH, there was a significant improvement in the pathological condition of ovarian tissue, a reduction in damage to ovarian granulosa cells, a decrease in the number of atretic follicles, and a significant increase in the number of mature oocytes. Enzyme-linked immunosorbent assays indicated that the plasma E2 levels in rmGH-treated mice increased over time, while plasma FSH levels decreased. The study analyzed the expression levels of genes related to the Notch-1 signaling pathway in mouse ovarian granulosa cells using RT-qPCR and found that medium and high doses of rmGH activated the Notch-1 signaling pathway in the ovarian granulosa cells of POF mice; immunohistochemical staining for Notch1 signaling pathway factors (Notch1, CBF1, and HES1) was positive in the WT, medium dose, and high dose groups, but not in the low dose or saline-treated groups (53). This suggests that GH promotes ovarian tissue repair and regeneration, E2 release, and oocyte maturation by activating the expression of Notch-1 signaling pathway factors in the ovarian tissue of POF mice.
4.3 PI3K/AKT signaling pathway
The PI3K-AKT pathway is a critical intracellular signal transduction pathway that responds to extracellular signals and regulates key biological processes such as cell metabolism, proliferation, survival, migration, and angiogenesis. Within this pathway, the PI3K/AKT/mTOR signaling axis is closely associated with the autophagy process in granulosa cells. Specifically, activated phosphoinositol-3 kinase (PI3K) catalyzes the phosphorylation of the metabolite PIP2 into PIP3, which then activates AKT. Recent studies on humans and mice have confirmed that the PI3K/Akt signaling plays an essential role in the regulation of GCs growth and apoptosis during follicular development (54, 55). The forkhead box O (FOXO) family of transcription factors are downstream targets of PI3K/Akt. Phosphorylation of FOXOs by p-Akt inhibits the transcriptional function of FOXOs and contributes to cell survival, growth, and proliferation (56). As a member of the FOXO family, FOXO1 plays a key role in upregulating the expression of downstream pro-apoptotic genes. FOXO1 promotes the activation of the mitochondrial pathway by upregulating Bax and downregulating Bcl-2 expression, thereby mediating GCs apoptosis (57, 58).
GH, by activating the PI3K/Akt signaling pathway, can significantly reduce cell apoptosis induced by OS, an effect that has been confirmed in various cell types, including cardiomyocytes and skeletal muscle cells (59, 60). Therefore, GH shows broad application prospects in the treatment of OS-related pathological processes (60). It is worth mentioning that the GH receptor is not only expressed in various muscle cells but also in human GCs and oocytes, providing a basis for the role of GH in the reproductive system. GH improves oocyte quality and outcomes of in vitro fertilization (IVF) by enhancing mitochondrial function (61). An animal experiment by Liu et al. also demonstrated that GH treatment could promote GCs proliferation by reducing OS through the PI3K/Akt signaling pathway. These results indicate that GH treatment can affect ovarian activity conducive to follicle and oocyte development. The aforementioned research findings provide strong theoretical support and practical basis for the targeted treatment of GH in DOR with respect to this signaling pathway (62).
4.4 Sirt3-Sod2 signaling pathway
Sirtuins are a class of nicotinamide adenine dinucleotide-dependent deacetylases that participate in numerous key biological processes by reducing the acetylation levels of proteins within cells. Activation of the Sirtuins signaling pathway not only delays the activation of primordial follicles and follicular atresia but also promotes the assembly of the meiotic spindle and chromosome segregation. Additionally, Sirtuins are involved in oocyte DNA damage repair, counteract oxidative stress damage in oocytes, and promote mitochondrial biogenesis. Therefore, Sirtuins are potential targets for the treatment of POF. The Sirtuins family includes multiple members from SIRT1 to SIRT7, each with different subcellular localizations and enzymatic activities, thus playing diverse biological functions in cells (63). Sirtuin 3 (Sirt3), as an important member of the Sirtuins family, is primarily localized in the mitochondria and possesses NAD+-dependent protein deacetylase characteristics. Sirt3 plays an indispensable role in maintaining mitochondrial function and stability. Notably, Sirt3 has a significant impact on maintaining the homeostasis of ROS within the mitochondria (64). In addition to directly reducing ROS production, Sirt3 can also remove the acetylation level of manganese superoxide dismutase (Sod2). Sod2 is activated after deacetylation, further promoting the detoxification of ROS and inhibiting mitochondrial oxidative stress (65).
Wang et al. established an in vitro apoptosis model of granulosa cells using cisplatin and observed cell proliferation, apoptosis, ROS, mitochondrial membrane potential, and mtDNA copy number after GH administration. They found that GH could promote the survival and proliferation of granulosa cells and alleviate cisplatin-induced apoptosis in these cells. GH, through the Sirt3-Sod2 pathway, activated Bcl-2 and downregulated Bax, thereby significantly reducing abnormal ROS levels, increasing the degree of mitochondrial membrane potential depolarization, and mtDNA copy number. This study demonstrated that GH protects ovarian granulosa cells from cisplatin-induced apoptosis and enhances mitochondrial function through the Sirt3-Sod2 pathway, reducing OS and apoptosis (35), providing new theoretical basis and therapeutic strategies for the treatment of DOR.
5 Impact of GH on oocytes and pregnancy
5.1 GH enhances oocyte quality
Oocyte maturation must undergo a series of processes, including nuclear and cytoplasmic maturation, to complete the transition from a gamete to an embryo. This process also involves epigenetics, including post-transcriptional modifications such as gene expression regulation, cell differentiation, and disease occurrence (66). Nuclear maturation encompasses a series of processes from germinal vesicle breakdown, resumption of meiosis, to the release of the first polar body, which reverses the arrest at Prophase I (Pro I) of meiosis and drives meiosis to progress to MII. Cytoplasmic maturation includes the redistribution of organelles, cytoskeletal dynamics, and molecular maturation, which prepares the oocyte for activation and pre-implantation development (67).
GH has beneficial effects on nuclear maturation and oocyte quality. Numerous animal experimental studies have shown that exogenous administration of GH can promote the nuclear maturation process of cumulus-oocyte complexes in various species (horses, dogs, cattle, sheep) (68–71). Previous research has found that GH promotes cell proliferation, inhibits apoptosis, and suppresses the synthesis of connexin 43, thereby promoting cumulus cell proliferation and oocyte maturation. It can also enhance oocyte quality by upregulating oocyte receptors and increasing mitochondrial activity (72, 73). Additionally, GH has shown a promoting effect on cytoplasmic maturation; adding GH to in vitro culture systems can significantly increase cortical distribution in equine and bovine oocytes (74, 75). Furthermore, adding IGF-1 to the culture medium of immature oocytes during in vitro culture can increase the number of mature oocytes (76) and reduce the number of abnormal oocytes (77). The study by Scheffler et al. demonstrated that the levels of GH and IGF-1 in the follicular fluid of normal oocytes, as well as the fertilization rates, were higher than those in abnormal oocytes, indicating a positive correlation between the GH/IGF-1 content in follicular fluid and oocyte quality (78). This further confirms that the exogenous use of GH and IGF-1 can enhance oocyte quality.
5.2 GH improves embryo quality and increases pregnancy rates
A large-scale foreign clinical survey indicates that the incidence of DOR is approximately 19%-26% (4). DOR is one of the significant causes leading to the decline in the success rate of ART. The quality of oocytes and embryos is closely related to clinical pregnancy rates (79). During the early development of embryos, mRNAs, proteins, and mitochondria derived from oocytes are key factors determining implantation (80). Experimental results show that GH has an improving effect on oocyte mitochondrial function, cytoplasmic maturation, and nuclear maturation; therefore, exogenous supplementation of GH can increase the number of mature oocytes obtained, thereby improving embryo quality (81, 82). Previous research found that injecting IGF-1 into the ovaries of cows significantly increased the number of inner cell masses (ICM) during the transition from morula to blastocyst, suggesting that IGF-1 may regulate the development of early embryos (83). Regarding embryo quality, studies on follicular fluid hormones indicate that high levels of GH in follicular fluid are positively correlated with several parameters of embryo implantation potential, including cleavage rate, embryo morphology, embryo development speed, chromosomal abnormalities, zona pellucida factors, and embryo culture environment (84). A randomized controlled trial (RCT) using a long protocol of GnRH agonist showed that GH adjuvant therapy can improve the number and quality of oocytes retrieved, mature oocytes (MII), fertilized oocytes, and the number and quality of embryos for transfer and cryopreservation (85), indicating that GH can not only improve oocyte quality but also embryo quality. A recent meta-analysis including 10 RCTs showed that when the GH dosage was 3IU-5IU, GH was associated with an increase in clinical pregnancy rates (RR=1.63, 95%CI [1.31, 2.03], P<0.0001) (86). An RCT conducted by Li et al. recruited 158 patients who had at least one IVF cycle failure due to a lack of high-quality embryos, using GH 3IU daily, and the results showed that GH supplementation increased implantation rates, pregnancy rates, and live birth rates (87). All the above research results indicate that GH can improve pregnancy rates in DOR patients and increase the success rate of ART.
5.3 GH promotion of ovarian angiogenesis and maintenance of corpus luteum function
The ovarian arteries originate from the branches of the abdominal aorta. Although the primary sources of blood supply to the ovaries remain unchanged throughout development, a complex network composed of initially nonfunctional small arteries derived from the ovarian arteries and newly formed capillaries continuously evolves to support the functions of follicular development, ovulation, and the formation of the corpus luteum (CL). Since puberty, the processes of folliculogenesis, ovulation, and the formation and maintenance of CL function are critically dependent on angiogenesis. The ability of the ovaries to maintain normal physiological functions is not only due to Gn but also related to the intervention of GH, which promotes the generation of ovarian blood vessels, thereby further promoting CL development and maintaining CL function (88). In addition, the supportive role of GH on CL function is also demonstrated by its ability to induce the production of P and exert anti-apoptotic effects (89). Studies have shown that during the early stages of bovine CL, the secretion of prostaglandin F2α and P by luteinized granulosa cells is increased through the exogenous addition of GH and other factors, thus maintaining CL function (90).
6 Use and dosage of GH in the assisted reproductive process for DOR
Several studies have explored adjuvant therapies that improve ovarian function and increase the success rate of pregnancy. A meta-analysis has indicated that DHEA (dehydroepiandrosterone) and Coenzyme Q10 are two additional adjuvant therapies that improve the likelihood of pregnancy, producing better clinical outcomes in terms of pregnancy success rates and the dosage of Gn required for ovulation induction compared to the control group (91). In 2016, an RCT by Kotb et al., based on the Bologna criteria for defining POR (poor ovarian reserve) patients, showed that DHEA supplementation could improve ovarian responsiveness, reduce the number of days of ovarian stimulation and the total dose of Gn, increase the number of oocytes retrieved, and improve fertilization rates, implantation rates, clinical pregnancy rates, and ongoing pregnancy rates (92). However, an RCT by Kara et al. in 2014 did not find positive results from DHEA supplementation (93). In 2015, a study by Ben-Meir et al. found that in aged mice, ovarian responsiveness was significantly improved during COH (controlled ovarian hyperstimulation) after pretreatment with Coenzyme Q10 (94). However, research on Coenzyme Q10 in human assisted reproduction is still relatively limited.
In 1988, Homburg et al. were the first to utilize GH to promote ovulation, aiming to enhance ovarian response to Gn and thereby increase the number of retrieved oocytes (95). As GH can improve ovarian sensitivity to Gn, increase the number of recruitable follicles, and improve the rate of high-quality embryos, it has been used as an adjunctive therapy during the ovulation induction phase in ART for over three decades (96). With a deeper understanding of the mechanisms of GH action, in recent years, numerous studies have begun to focus more on the application of GH in ART for specific patient populations, particularly those with DOR. He et al.’s study observed that adjunctive GH therapy altered the metabolic profile in follicular fluid of DOR patients, particularly increasing the level of the antioxidant metabolite itaconic acid (97). Zhang et al.’s study, by comparing the impact of different durations of GH pretreatment on the number of retrieved oocytes, found that GH pretreatment could improve treatment outcomes in DOR patients (98). Studies by Chen et al. and Dogan et al. both indicated that GH pretreatment could significantly increase the number of retrieved oocytes and improve embryo quality (99, 100). The study by Haydaredeoğlu et al., unlike most clinical studies using GH alone, performed DHEA and testosterone combined with GH pretreatment, and the results showed that it may increase androgen levels in the ovaries, resulting in a slowdown in the growth of (initial preantral) IP follicles, which may promote the development of more sinus follicles and make maturation more uniform, significantly increasing the number of follicles, recovered oocytes, MII oocytes, and fertilization rates in DOR patients (101). In terms of adjuvant therapy and drug combination, the current research is still limited, and we look forward to more breakthroughs in future research. Further details on the use and dosage of GH in the aforementioned studies are summarized in Table 1. However, the aforementioned clinical studies are mostly small-scale or single-center studies, which may be subject to bias and thus have certain limitations. Therefore, there is an urgent need to conduct larger-scale, multicenter randomized controlled trials (RCTs) to further validate the efficacy of GH therapy. Such trials are crucial for providing more robust and generalizable evidence for its clinical application.
In the treatment of patients with DOR using GH, dosage and timing of administration are crucial. Multiple studies have shown that in ART, patients with poor ovarian response (POR) who receive 4 to 8 IU of GH daily during the follicular phase exhibit increased endometrial thickness, reduced gonadotropin requirements, and a higher number of mature oocytes compared to the control group (102). During the treatment period, patients should strictly follow medical advice for regular medication use and avoid arbitrary interruptions to ensure efficacy and safety. Dosage regimens need to be individually adjusted for different populations. For patients with DOR who have fertility needs, poor embryo quality, thin endometrium, and recurrent implantation failure, initiating GH treatment three months in advance can regulate follicle development from the preantral stage to maturity, thereby improving oocyte quality (103). For older patients with POR, a study involving women over 40 undergoing IVF demonstrated that appropriately increasing the GH dosage and extending the pretreatment period can improve pregnancy outcomes in patients with thin endometrium undergoing frozen embryo transfer (104). For patients with POR and recurrent implantation failure, the use of GH during follicle stimulation can mitigate the decline in ART efficiency due to aging, including improvements in the number of oocytes retrieved, fertilization rate, embryo quality, implantation rate, pregnancy rate, and live birth rate (6). In contrast, relatively younger patients (under 40 years old) with relatively better ovarian reserve can use a lower dose of 2 U/d and start pretreatment four weeks before ovulation induction (98). In summary, when treating patients with DOR using GH, the dosage and timing should be individually adjusted according to the specific circumstances of the patient to achieve the best therapeutic effect.
7 Potential adverse reactions and safety of GH therapy
Previous studies have indicated that long-term, high-dose use of GH in the treatment of DOR may trigger adverse reactions and potential risks across multiple systems. Specifically, GH can induce insulin resistance and glucose-lipid metabolic disorders by antagonizing insulin action, thereby increasing the risk of new-onset diabetes (105). Moreover, excessive use of GH may lead to cardiovascular complications such as acromegaly, myocardial hypertrophy, and hypertension through its effects on endothelial dysfunction, atherosclerosis formation, oxidative stress, and metabolic homeostasis (106).Although the direct association between GH and tumorigenesis has not been established, GH may increase the risk of tumor recurrence or proliferation (107). For example, one study found that over half of the tumor cells in 61% of cervical cancer patients express nuclear GHR, and high levels of nuclear GHR may act as a promoter of cervical cancer cell progression (108). However, another review summarizing the published papers to date concluded that GH use is safe in terms of tumor recurrence (109).Furthermore, excessive GH can also disrupt the stability of the reproductive endocrine axis. A study focusing on premenopausal women with acromegaly revealed that 64% of these women of childbearing age had low levels of AMH, suggesting that excessive GH may lead to a decrease in ovarian reserve (110).In summary, strict adherence to individualized principles is required in clinical applications, along with continuous monitoring of side effects and safety after medication use, to ensure the safety and efficacy of treatment.
8 Summary and outlook
The application of GH therapy in DOR has made significant progress, offering new therapeutic hope for many patients. DOR is a common reproductive disorder characterized by a reduction in the number and quality of ovarian follicles, leading to a decline in female fertility. As an important hormone for growth and metabolism regulation, GH promotes follicular development and improves oocyte quality through mechanisms such as the activation of the PI3K/Akt, Sirt3-Sod2, Notch-1, and JAK2-MAPK3/1 signaling pathways, thereby improving the fertility prognosis of DOR patients. Numerous clinical trials have confirmed the effectiveness of GH therapy for DOR in previous studies. GH can stimulate ovarian angiogenesis, improve the follicular microenvironment, and thereby promote follicular growth and development. Additionally, GH protects follicles from oxidative stress and other damages by regulating apoptotic processes, maintaining the health of the ovaries. However, issues regarding the optimal dosage, timing, and duration of GH therapy for DOR still require further exploration. Additionally, further investigation is required regarding the indications, contraindications, and potential adverse effects of GH therapy. Previous studies have indicated that long-term, high-dose use of GH may trigger adverse reactions and potential risks across multiple systems, including insulin resistance, cardiovascular complications, and potential impacts on tumor recurrence. Therefore, strict adherence to individualized principles is required in clinical applications, along with continuous monitoring of side effects and safety after medication use, to ensure the safety and efficacy of treatment. With the continuous advancement of technologies such as genomics and proteomics, we can gain a deeper understanding of the pathogenesis of DOR, thus providing more precise and personalized treatment plans for GH therapy. We anticipate that further basic and clinical research will validate and refine the methods and strategies for GH treatment of DOR.
Author contributions
PH: Writing – original draft. HX: Writing – original draft. YY: Data curation, Investigation, Writing – original draft. ZW: Data curation, Investigation, Writing – original draft. JY: Data curation, Investigation, Writing – original draft. LH: Writing – review & editing. DZ: Supervision, Writing – review & editing.
Funding
The author(s) declare that financial support was received for the research and/or publication of this article. Natural Science Foundation of Shandong Province (NO. ZR2023MH222); Medical and Health Science and Technology Program of Shandong Province (NO. 202405030716); Rongxiang Xu’s Regenerative Medicine Development Program Project of Binzhou Medical University (BY20 20XRX07).
Conflict of interest
The authors declare that the research was conducted in the absence of any commercial or financial relationships that could be construed as a potential conflict of interest.
Generative AI statement
The author(s) declare that no Generative AI was used in the creation of this manuscript.
Publisher’s note
All claims expressed in this article are solely those of the authors and do not necessarily represent those of their affiliated organizations, or those of the publisher, the editors and the reviewers. Any product that may be evaluated in this article, or claim that may be made by its manufacturer, is not guaranteed or endorsed by the publisher.
References
1. Zhu Q, Li Y, Ma J, Ma H, Liang X. Potential factors result in diminished ovarian reserve: a comprehensive review. J Ovarian Res. (2023) 16:208. doi: 10.1186/s13048-023-01296-x
2. Pastore LM, Christianson MS, Stelling J, Kearns WG, Segars JH. Reproductive ovarian testing and the alphabet soup of diagnoses: DOR, POI, POF, POR, and FOR. J Assist Reprod Genet. (2018) 35:17–23. doi: 10.1007/s10815-017-1058-4
3. Fassnacht W, Mempel A, Strowitzki T, Vogt P. Premature ovarian failure (POF) syndrome: towards the molecular clinical analysis of its genetic complexity. Curr Med Chem. (2006) 13:1397–410. doi: 10.2174/092986706776872943
4. Devine K, Mumford SL, Wu M, DeCherney AH, Hill MJ, Propst A. Diminished ovarian reserve in the United States assisted reproductive technology population: diagnostic trends among 181,536 cycles from the Society for Assisted Reproductive Technology Clinic Outcomes Reporting System. Fertil Steril. (2015) 104:612–19.e3. doi: 10.1016/j.fertnstert.2015.05.017
5. Han L, Tian H, Guo X, Zhang L. Regulation of ovarian function by growth hormone: Potential intervention of ovarian aging. Front Endocrinol. (2023) 13:1072313. doi: 10.3389/fendo.2022.1072313
6. Chang C-W, Sung Y-W, Hsueh Y-W, Chen Y-Y, Ho M, Hsu H-C, et al. Growth hormone in fertility and infertility: Mechanisms of action and clinical applications. Front Endocrinol. (2022) 13:1040503. doi: 10.3389/fendo.2022.1040503
7. Yovich JL, Regan SLP, Zaidi S, Keane KN. The concept of growth hormone deficiency affecting clinical prognosis in IVF. Front Endocrinol. (2019) 10:650. doi: 10.3389/fendo.2019.00650
8. Nicholls AR, Holt RIG. Growth hormone and insulin-like growth factor-1. Front Horm Res. (2016) 47:101–14. doi: 10.1159/000445173
9. Lin S, Li C, Li C, Zhang X. Growth hormone receptor mutations related to individual dwarfism. Int J Mol Sci. (2018) 19:1433. doi: 10.3390/ijms19051433
10. Magon N, Agrawal S, Malik S, Babu K. Growth hormone in the management of female infertility. Indian J Endocrinol Metab. (2011) 15:246. doi: 10.4103/2230-8210.84876
11. Sirotkin AV. Control of reproductive processes by growth hormone: extra- and intracellular mechanisms. Vet J. (2005) 170:307–17. doi: 10.1016/j.tvjl.2004.05.014
12. Brunet-Dunand SE, Vouyovitch C, Araneda S, Pandey V, Vidal LJ-P, Print C, et al. Autocrine human growth hormone promotes tumor angiogenesis in mammary carcinoma. Endocrinology. (2009) 150:1341–52. doi: 2020071613130074500
13. Wajnrajch MP. Physiological and pathological growth hormone secretion. J Pediatr Endocrinol Metab. (2005) 18:325–38. doi: 10.1515/jpem.2005.18.4.325
14. van den Eijnden MJ, Strous GJ. Autocrine growth hormone: effects on growth hormone receptor trafficking and signaling. Mol Endocrinol Baltim Md. (2007) 21:2832–46. doi: 10.1210/me.2007-0092
15. Hull KL, Harvey S. Growth hormone and reproduction: a review of endocrine and autocrine/paracrine interactions. Int J Endocrinol. (2014) 2014:1–24. doi: 10.1155/2014/234014
16. Laisk T, Tšuiko O, Jatsenko T, Hõrak P, Otala M, Lahdenperä M, et al. Demographic and evolutionary trends in ovarian function and aging. Hum Reprod Update. (2018) 25:34–50. doi: 10.1093/humupd/dmy031
17. Wang J, Pan X, Zhou J, Li X, Sun Y, Wang L. Advances in understanding the effect and mechanism of dehydroepiandrosterone on diminished ovarian reserve. Drug Discovery Ther. (2023) 17:87–94. doi: 10.5582/ddt.2022.01109
18. Liang J, Gao Y, Feng Z, Zhang B, Na Z, Li D. Reactive oxygen species and ovarian diseases: Antioxidant strategies. Redox Biol. (2023) 62:102659. doi: 10.1016/j.redox.2023.102659
19. Huang Y, Cheng Y, Zhang M, Xia Y, Chen X, Xian Y, et al. Oxidative stress and inflammatory markers in ovarian follicular fluid of women with diminished ovarian reserve during in vitro fertilization. J Ovarian Res. (2023) 16:206. doi: 10.1186/s13048-023-01293-0
20. Hull K, Harvey S. Growth hormone: roles in female reproduction. J Endocrinol. (2001) 168:1–23. doi: 10.1677/joe.0.1680001
21. Doldi N, Bassan M, Bonzi V, Ferrari A. Effects of growth hormone and growth hormone-releasing hormone on steroid synthesis in cultured human luteinizing granulosa cells. Gynecol Endocrinol. (1996) 10:101–8. doi: 10.3109/09513599609097899
22. Kezele P. Cell-cell interactions in primordial follicle assembly and development. Front Biosci. (2002) 7:d1990. doi: 10.2741/kezele
23. Saccon TD, Moreira F, Cruz LA, Mondadori RG, Fang Y, Barros CC, et al. Ovarian aging and the activation of the primordial follicle reserve in the long-lived Ames dwarf and the short-lived bGH transgenic mice. Mol Cell Endocrinol. (2017) 455:23–32. doi: 10.1016/j.mce.2016.10.015
24. Dosouto C, Calaf J, Polo A, Haahr T, Humaidan P. Growth hormone and reproduction: lessons learned from animal models and clinical trials. Front Endocrinol. (2019) 10:404. doi: 10.3389/fendo.2019.00404
25. Cecim M, Kerr J, Bartke A. Effects of bovine growth hormone (bGH) transgene expression or bGH treatment on reproductive functions in female mice. Biol Reprod. (1995) 52:1144–8. doi: 10.1095/biolreprod52.5.1144
26. Chase CC, Kirby CJ, Hammond AC, Olson TA, Lucy MC. Patterns of ovarian growth and development in cattle with a growth hormone receptor deficiency. J Anim Sci. (1998) 76:212. doi: 10.2527/1998.761212x
27. Kaczmarek MM, Schams D, Ziecik AJ. Role of vascular endothelial growth factor in ovarian physiology - an overview. Reprod Biol. (2005) 5:111–36.
28. Wu M, Ma L, Xue L, Ye W, Lu Z, Li X, et al. Resveratrol alleviates chemotherapy-induced oogonial stem cell apoptosis and ovarian aging in mice. Aging. (2019) 11:1030–44. doi: 10.18632/aging.101808
29. Jiang Y, Zhu D, Liu W, Qin Q, Fang Z, Pan Z. Hedgehog pathway inhibition causes primary follicle atresia and decreases female germline stem cell proliferation capacity or stemness. Stem Cell Res Ther. (2019) 10:198. doi: 10.1186/s13287-019-1299-5
30. Palmerini MG, Nottola SA, Tunjung WAS, Kadowaki A, Bianchi S, Cecconi S, et al. EGF-FSH supplementation reduces apoptosis of pig granulosa cells in co-culture with cumulus-oocyte complexes. Biochem Biophys Res Commun. (2016) 481:159–64. doi: 10.1016/j.bbrc.2016.10.151
31. Hsueh AJ, Eisenhauer K, Chun SY, Hsu SY, Billig H. Gonadal cell apoptosis. Recent Prog Horm Res. (1996) 51:433–455; discussion 455-456.
32. Shimizu S, Eguchi Y, Kamiike W, Funahashi Y, Mignon A, Lacronique V, et al. Bcl-2 prevents apoptotic mitochondrial dysfunction by regulating proton flux. Proc Natl Acad Sci. (1998) 95:1455–9. doi: 10.1073/pnas.95.4.1455
33. Wang S, He G, Chen M, Zuo T, Xu W, Liu X. The role of antioxidant enzymes in the ovaries. Oxid Med Cell Longev. (2017) 2017:1–14. doi: 10.1155/2017/4371714
34. Tesarik J, Galán-Lázaro M, Mendoza-Tesarik R. Ovarian aging: molecular mechanisms and medical management. Int J Mol Sci. (2021) 22:1371. doi: 10.3390/ijms22031371
35. Wang J, Wu J, Zhang Y, Zhang J, Xu W, Wu C, et al. Growth hormone protects against ovarian granulosa cell apoptosis: Alleviation oxidative stress and enhancement mitochondrial function. Reprod Biol. (2021) 21:100504. doi: 10.1016/j.repbio.2021.100504
36. Song D, Zhong Y, Qian C, Zou Q, Ou J, Shi Y, et al. Human umbilical cord mesenchymal stem cells therapy in cyclophosphamide-induced premature ovarian failure rat model. BioMed Res Int. (2016) 2016:2517514. doi: 10.1155/2016/2517514
37. Tsai-Turton M, Luong BT, Tan Y, Luderer U. Cyclophosphamide-induced apoptosis in COV434 human granulosa cells involves oxidative stress and glutathione depletion. Toxicol Sci. (2007) 98:216–30. doi: 10.1093/toxsci/kfm087
38. Feng P, Xie Q, Liu Z, Guo Z, Tang R, Yu Q. Study on the reparative effect of PEGylated growth hormone on ovarian parameters and mitochondrial function of oocytes from rats with premature ovarian insufficiency. Front Cell Dev Biol. (2021) 9:649005. doi: 10.3389/fcell.2021.649005
39. A H, F R, L N, B T, T Yb. Oocyte aging is controlled by mitogen-activated protein kinase signaling. Aging Cell. (2021) 20:e13386. doi: 10.1111/acel.13386
40. Achache H, Laurent L, Hecker-Mimoun Y, Ishtayeh H, Rappaport Y, Kroizer E, et al. Progression of meiosis is coordinated by the level and location of MAPK activation via OGR-2 in caenorhabditis elegans. Genetics. (2019) 212:213–29. doi: 10.1534/genetics.119.302080
41. Cui W, Zhang J, Lian H-Y, Wang H-L, Miao D-Q, Zhang C-X, et al. Roles of MAPK and spindle assembly checkpoint in spontaneous activation and MIII arrest of rat oocytes. PLoS One. (2012) 7:e32044. doi: 10.1371/journal.pone.0032044
42. Verlhac MH, de Pennart H, Maro B, Cobb MH, Clarke HJ. MAP kinase becomes stably activated at metaphase and is associated with microtubule-organizing centers during meiotic maturation of mouse oocytes. Dev Biol. (1993) 158:330–40. doi: 10.1006/dbio.1993.1192
43. Verlhac MH, Kubiak JZ, Clarke HJ, Maro B. Microtubule and chromatin behavior follow MAP kinase activity but not MPF activity during meiosis in mouse oocytes. Dev Camb Engl. (1994) 120:1017–25. doi: 10.1242/dev.120.4.1017
44. Strous GJ, Almeida ADS, Putters J, Schantl J, Sedek M, Slotman JA, et al. Growth hormone receptor regulation in cancer and chronic diseases. Front Endocrinol. (2020) 11:597573. doi: 10.3389/fendo.2020.597573
45. Wu C, Li R, Luo H, Xu M, Zhang X. JAK2 inhibitor CEP-33779 prevents mouse oocyte maturation in vitro. Biosci Rep. (2017) 37:BSR20170642. doi: 10.1042/BSR20170642
46. Luo Y-Y, Zeng X, Zhu L, Li C, Xie J, Dong Q, et al. Growth hormone reduces aneuploidy and improves oocytes quality by JAK2-MAPK3/1 pathway in aged mice. J Transl Med. (2023) 21:426. doi: 10.1186/s12967-023-04296-z
47. Subramaniam D, Ponnurangam S, Ramamoorthy P, Standing D, Battafarano RJ, Anant S, et al. Curcumin induces cell death in esophageal cancer cells through modulating Notch signaling. PLoS One. (2012) 7:e30590. doi: 10.1371/journal.pone.0030590
48. Yao L, Kan EM, Kaur C, Dheen ST, Hao A, Lu J, et al. Notch-1 signaling regulates microglia activation via NF-κB pathway after hypoxic exposure in vivo and in vitro. PLoS One. (2013) 8:e78439. doi: 10.1371/journal.pone.0078439
49. Ji X, Wang Z, Geamanu A, Goja A, Sarkar FH, Gupta SV. Delta-tocotrienol suppresses Notch-1 pathway by upregulating miR-34a in nonsmall cell lung cancer cells. Int J Cancer. (2012) 131:2668–77. doi: 10.1002/ijc.27549
50. Zhang H, Hilton MJ, Anolik JH, Welle SL, Zhao C, Yao Z, et al. NOTCH inhibits osteoblast formation in inflammatory arthritis via noncanonical NF-κB. J Clin Invest. (2014) 124:3200–14. doi: 10.1172/JCI68901
51. Chen G, Qiu Y, Sun L, Yu M, Wang W, Xiao W, et al. The jagged-2/notch-1/hes-1 pathway is involved in intestinal epithelium regeneration after intestinal ischemia-reperfusion injury. PLoS One. (2013) 8:e76274. doi: 10.1371/journal.pone.0076274
52. Wang Z, Azmi AS, Ahmad A, Banerjee S, Wang S, Sarkar FH, et al. TW-37, a small-molecule inhibitor of Bcl-2, inhibits cell growth and induces apoptosis in pancreatic cancer: involvement of Notch-1 signaling pathway. Cancer Res. (2009) 69:2757–65. doi: 10.1158/0008-5472.CAN-08-3060
53. Liu TE, Wang S, Zhang L, Guo L, Yu Z, Chen C, et al. Growth hormone treatment of premature ovarian failure in a mouse model via stimulation of the Notch-1 signaling pathway. Exp Ther Med. (2016) 12:215–21. doi: 10.3892/etm.2016.3326
54. Hu C-L, Cowan RG, Harman RM, Quirk SM. Cell cycle progression and activation of Akt kinase are required for insulin-like growth factor I-mediated suppression of apoptosis in granulosa cells. Mol Endocrinol Baltim Md. (2004) 18:326–38. doi: 10.1210/me.2003-0178
55. John GB, Shidler MJ, Besmer P, Castrillon DH. Kit signaling via PI3K promotes ovarian follicle maturation but is dispensable for primordial follicle activation. Dev Biol. (2009) 331:292–9. doi: 10.1016/j.ydbio.2009.05.546
56. Zhang X, Tang N, Hadden TJ, Rishi AK. Akt, FoxO and regulation of apoptosis. Biochim Biophys Acta. (2011) 1813:1978–86. doi: 10.1016/j.bbamcr.2011.03.010
57. Shen M, Lin F, Zhang J, Tang Y, Chen W-K, Liu H. Involvement of the up-regulated FoxO1 expression in follicular granulosa cell apoptosis induced by oxidative stress. J Biol Chem. (2012) 287:25727–40. doi: 10.1074/jbc.M112.349902
58. Zhu L, Yuan H, Guo C, Lu Y, Deng S, Yang Y, et al. Zearalenone induces apoptosis and necrosis in porcine granulosa cells via a caspase-3- and caspase-9-dependent mitochondrial signaling pathway. J Cell Physiol. (2012) 227:1814–20. doi: 10.1002/jcp.22906
59. Granata R, Trovato L, Gallo MP, Destefanis S, Settanni F, Scarlatti F, et al. Growth hormone-releasing hormone promotes survival of cardiac myocytes in vitro and protects against ischaemia-reperfusion injury in rat heart. Cardiovasc Res. (2009) 83:303–12. doi: 10.1093/cvr/cvp090
60. Perrini S, Laviola L, Carreira MC, Cignarelli A, Natalicchio A, Giorgino F. The GH/IGF1 axis and signaling pathways in the muscle and bone: mechanisms underlying age-related skeletal muscle wasting and osteoporosis. J Endocrinol. (2010) 205:201–10. doi: 10.1677/JOE-09-0431
61. Kolibianakis EM, Venetis CA, Diedrich K, Tarlatzis BC, Griesinger G. Addition of growth hormone to gonadotrophins in ovarian stimulation of poor responders treated by in-vitro fertilization: a systematic review and meta-analysis. Hum Reprod Update. (2009) 15:613–22. doi: 10.1093/humupd/dmp026
62. Liu K, Zhang L, Qi Q, Li J, Yan F, Hou J. Growth hormone treatment improves the development of follicles and oocytes in prepubertal lambs. J Ovarian Res. (2023) 16:132. doi: 10.1186/s13048-023-01209-y
63. Vassilopoulos A, Fritz KS, Petersen DR, Gius D. The human sirtuin family: evolutionary divergences and functions. Hum Genomics. (2011) 5:485. doi: 10.1186/1479-7364-5-5-485
64. Liu X, Zhang L, Wang P, Li X, Qiu D, Li L, et al. Sirt3-dependent deacetylation of SOD2 plays a protective role against oxidative stress in oocytes from diabetic mice. Cell Cycle Georget Tex. (2017) 16:1302–8. doi: 10.1080/15384101.2017.1320004
65. Chen Y, Fu LL, Wen X, Wang XY, Liu J, Cheng Y, et al. Sirtuin-3 (SIRT3), a therapeutic target with oncogenic and tumor-suppressive function in cancer. Cell Death Dis. (2014) 5:e1047. doi: 10.1038/cddis.2014.14
66. Heikinheimo O, Gibbons WE. The molecular mechanisms of oocyte maturation and early embryonic development are unveiling new insights into reproductive medicine. Mol Hum Reprod. (1998) 4:745–56. doi: 10.1093/molehr/4.8.745
67. Eppig J. Coordination of nuclear and cytoplasmic oocyte maturation in eutherian mammals. Reprod Fertil Dev. (1996) 8:485. doi: 10.1071/rd9960485
68. Pereira GR, Lorenzo PL, Carneiro GF, Ball BA, Gonçalves PBD, Pegoraro LMC, et al. The effect of growth hormone (GH) and insulin-like growth factor-I (IGF-I) on in vitro maturation of equine oocytes. Zygote. (2012) 20:353–60. doi: 10.1017/S0967199411000335
69. Chigioni S, Secchi C, Borromeo V, Modina S, Beretta MS, Luvoni GC. Effects of growth hormone on oocyte in vitro maturation and its localization in the canine cumulus-oocyte complexes. Vet Res Commun. (2008) 32:131–4. doi: 10.1007/s11259-008-9098-y
70. Mtango NR, Varisanga MD, Dong YJ, Rajamahendran R, Suzuki T. Growth factors and growth hormone enhance in vitro embryo production and post-thaw survival of vitrified bovine blastocysts. Theriogenology. (2003) 59:1393–402. doi: 10.1016/s0093-691x(02)01163-9
71. Shirazi A, Shams-Esfandabadi N, Ahmadi E, Heidari B. Effects of growth hormone on nuclear maturation of ovine oocytes and subsequent embryo development. Reprod Domest Anim. (2010) 45:530–6. doi: 10.1111/j.1439-0531.2008.01290.x
72. Kölle S, Stojkovic M, Boie G, Wolf E, Sinowatz F. Growth hormone-related effects on apoptosis, mitosis, and expression of connexin 43 in bovine in vitro maturation cumulus-oocyte complexes. Biol Reprod. (2003) 68:1584–9. doi: 10.1095/biolreprod.102.010264
73. Songsasen N, Yu I, Leibo SP. Nuclear maturation of canine oocytes cultured in protein-free media. Mol Reprod Dev. (2002) 62:407–15. doi: 10.1002/mrd.10130
74. Pereira GR, Lorenzo PL, Carneiro GF, Ball BA, Bilodeau-Goeseels S, Kastelic J, et al. The involvement of growth hormone in equine oocyte maturation, receptor localization and steroid production by cumulus-oocyte complexes in vitro. Res Vet Sci. (2013) 95:667–74. doi: 10.1016/j.rvsc.2013.06.024
75. Nyholt De Prada JK, VandeVoort CA. Growth hormone and in vitro maturation of rhesus macaque oocytes and subsequent embryo development. J Assist Reprod Genet. (2008) 25:145–58. doi: 10.1007/s10815-008-9208-3
76. Gómez E, Tarín JJ, Pellicer A. Oocyte maturation in humans: the role of gonadotropins and growth factors. Fertil Steril. (1993) 60:40–6.
77. Yu Y, Yan J, Li M, Yan L, Zhao Y, Lian Y, et al. Effects of combined epidermal growth factor, brain-derived neurotrophic factor and insulin-like growth factor-1 on human oocyte maturation and early fertilized and cloned embryo development. Hum Reprod. (2012) 27:2146–59. doi: 10.1093/humrep/des099
78. Scheffler F, Vandecandelaere A, Soyez M, Bosquet D, Lefranc E, Copin H, et al. Follicular GH and IGF1 levels are associated with oocyte cohort quality: A pilot study. Front Endocrinol. (2021) 12:793621. doi: 10.3389/fendo.2021.793621
79. Oron G, Son W-Y, Buckett W, Tulandi T, Holzer H. The association between embryo quality and perinatal outcome of singletons born after single embryo transfers: a pilot study. Hum Reprod. (2014) 29:1444–51. doi: 10.1093/humrep/deu079
80. Gosden R. Oogenesis as a foundation for embryogenesis. Mol Cell Endocrinol. (2002) 186:149–53. doi: 10.1016/s0303-7207(01)00683-9
81. Weall BM, Al-Samerria S, Conceicao J, Yovich JL, Almahbobi G. A direct action for GH in improvement of oocyte quality in poor-responder patients. Reproduction. (2015) 149:147–54. doi: 10.1530/REP-14-0494
82. Izadyar F, Zhao J, Van Tol HTA, Colenbrander B, Bevers MM. Messenger RNA expression and protein localization of growth hormone in bovine ovarian tissue and in cumulus oocyte complexes (COCs) during in vitro maturation. Mol Reprod Dev. (1999) 53:398–406. doi: 10.1002/(SICI)1098-2795(199908)53:4<398::AID-MRD5>3.0.CO;2-I
83. Velazquez MA, Hadeler K-G, Herrmann D, Kues WA, Rémy B, Beckers J-F, et al. In vivo oocyte IGF-1 priming increases inner cell mass proliferation of in vitro-formed bovine blastocysts. Theriogenology. (2012) 78:517–27. doi: 10.1016/j.theriogenology.2012.02.034
84. Mendoza C. Relationship between fertilization results after intracytoplasmic sperm injection, and intrafollicular steroid, pituitary hormone and cytokine concentrations. Hum Reprod. (1999) 14:628–35. doi: 10.1093/humrep/14.3.628
85. Dakhly DMR, Bassiouny YA, Bayoumi YA, Hassan MA, Gouda HM, Hassan AA. The addition of growth hormone adjuvant therapy to the long down regulation protocol in poor responders undergoing in vitro fertilization: Randomized control trial. Eur J Obstet Gynecol Reprod Biol. (2018) 228:161–5. doi: 10.1016/j.ejogrb.2018.06.035
86. Lin G, Zhong X, Li S, Xu L. Clinical evidence of growth hormone for infertile women with diminished ovarian reserve undergoing IVF: a systematic review and meta-analysis. Front Endocrinol. (2023) 14:1215755. doi: 10.3389/fendo.2023.1215755
87. Li J, Chen Q, Wang J, Huang G, Ye H. Does growth hormone supplementation improve oocyte competence and IVF outcomes in patients with poor embryonic development? A randomized controlled trial. BMC Pregnancy Childbirth. (2020) 20:310. doi: 10.1186/s12884-020-03004-9
88. Devesa J, Caicedo D. The role of growth hormone on ovarian functioning and ovarian angiogenesis. Front Endocrinol. (2019) 10:450. doi: 10.3389/fendo.2019.00450
89. Schams D, Berisha B. Regulation of corpus luteum function in cattle–an overview. Reprod Domest Anim. (2004) 39:241–51. doi: 10.1111/j.1439-0531.2004.00509.x
90. Kobayashi S, Miyamoto A, Berisha B, Schams D. Growth hormone, but not luteinizing hormone, acts with luteal peptides on prostaglandin F2alpha and progesterone secretion by bovine corpora lutea. vitro Prostaglandins Other Lipid Mediat. (2001) 63:79–92. doi: 10.1016/s0090-6980(00)00099-x
91. Zhang Y, Zhang C, Shu J, Guo J, Chang H-M, Leung PCK, et al. Adjuvant treatment strategies in ovarian stimulation for poor responders undergoing IVF: a systematic review and network meta-analysis. Hum Reprod Update. (2020) 26:247–63. doi: 10.1093/humupd/dmz046
92. Kotb MMM, Hassan AMA, AwadAllah AMA. Does dehydroepiandrosterone improve pregnancy rate in women undergoing IVF/ICSI with expected poor ovarian response according to the Bologna criteria? A randomized controlled trial. Eur J Obstet Gynecol Reprod Biol. (2016) 200:11–5. doi: 10.1016/j.ejogrb.2016.02.009
93. Kara M, Aydin T, Aran T, Turktekin N, Ozdemir B. Does dehydroepiandrosterone supplementation really affect IVF-ICSI outcome in women with poor ovarian reserve? Eur J Obstet Gynecol Reprod Biol. (2014) 173:63–5. doi: 10.1016/j.ejogrb.2013.11.008
94. Ben-Meir A, Burstein E, Borrego-Alvarez A, Chong J, Wong E, Yavorska T, et al. Coenzyme Q10 restores oocyte mitochondrial function and fertility during reproductive aging. Aging Cell. (2015) 14:887–95. doi: 10.1111/acel.12368
95. Homburg R, Eshel A, Abdalla HI, Jacobs HS. Growth hormone facilitates ovulation induction by gonadotrophins. Clin Endocrinol (Oxf). (1988) 29:113–7. doi: 10.1111/j.1365-2265.1988.tb00252.x
96. Liu F-T, Hu K-L, Li R. Effects of growth hormone supplementation on poor ovarian responders in assisted reproductive technology: a systematic review and meta-analysis. Reprod Sci. (2021) 28:936–48. doi: 10.1007/s43032-020-00298-0
97. He F, Wang F, Yang Y, Yuan Z, Sun C, Zou H, et al. The effect of growth hormone on the metabolome of follicular fluid in patients with diminished ovarian reserve. Reprod Biol Endocrinol RBE. (2023) 21:21. doi: 10.1186/s12958-023-01073-x
98. Zhang Y, Liu L, Xu A, Jin Y, Tong X, Zhou F, et al. Effect of different growth hormone pretreatment times in assisted reproductive therapy for patients with diminished ovarian reserve: A retrospective pilot cohort study. Med (Baltimore). (2024) 103:e39645. doi: 10.1097/MD.0000000000039645
99. Chen Y, Tao L, Lin Y, Li X, Ma C. Outcomes of in vitro fertilization-embryo transfer in women with diminished ovarian reserve after growth hormone pretreatment. Gynecol Endocrinol Off J Int Soc Gynecol Endocrinol. (2020) 36:955–8. doi: 10.1080/09513590.2020.1737005
100. Dogan S, Cicek OSY, Demir M, Yalcinkaya L, Sertel E. The effect of growth hormone adjuvant therapy on assisted reproductive technologies outcomes in patients with diminished ovarian reserve or poor ovarian response. J Gynecol Obstet Hum Reprod. (2021) 50:101982. doi: 10.1016/j.jogoh.2020.101982
101. Haydardedeoğlu B, Işık AZ, Bulgan Kılıçdağ E. The combination of dehydroepiandrosterone, transdermal testosterone, and growth hormone as an adjuvant therapy in assisted reproductive technology cycles in patients aged below 40 years with diminished ovarian reserve. Turk J Obstet Gynecol. (2015) 12:60–5. doi: 10.4274/tjod.32656
102. Xu Z, Tong W, Yang Z, Zhang H, Chen X. Comparative efficacy of different growth hormone supplementation protocols in improving clinical outcomes in women with poor ovarian response undergoing assisted reproductive therapy: a network meta-analysis. Sci Rep. (2024) 14:3377. doi: 10.1038/s41598-024-53780-z
103. Sood A, Mohiyiddeen G, Ahmad G, Fitzgerald C, Watson A, Mohiyiddeen L. Growth hormone for in vitro fertilisation (IVF). Cochrane Database Syst Rev. (2021) 2021:CD000099. doi: 10.1002/14651858.CD000099.pub4
104. Lan K-C, Lin P-Y, Chang Y-C, Chen Y-J, Tsai Y-R, Ismaeil Mohamed IS, et al. Growth hormone supplementation may improve the pregnancy rate and endometrial receptivity among women aged more than 40 years undergoing in vitro fertilization. BioMed J. (2019) 42:411–6. doi: 10.1016/j.bj.2019.05.003
105. Kim S-H, Park M-J. Effects of growth hormone on glucose metabolism and insulin resistance in human. Ann Pediatr Endocrinol Metab. (2017) 22:145–52. doi: 10.6065/apem.2017.22.3.145
106. Wolters TLC, Netea MG, Riksen NP, Hermus ARMM, Netea-Maier RT. Acromegaly, inflammation and cardiovascular disease: a review. Rev Endocr Metab Disord. (2020) 21:547–68. doi: 10.1007/s11154-020-09560-x
107. Harvey S, Martínez-Moreno CG, Luna M, Arámburo C. Autocrine/paracrine roles of extrapituitary growth hormone and prolactin in health and disease: An overview. Gen Comp Endocrinol. (2015) 220:103–11. doi: 10.1016/j.ygcen.2014.11.004
108. Dehari R, Nakamura Y, Okamoto N, Nakayama H. Increased nuclear expression of growth hormone receptor in uterine cervical neoplasms of women under 40 years old. Tohoku J Exp Med. (2008) 216:165–72. doi: 10.1620/tjem.216.165
109. Spaziani M, Tarantino C, Tahani N, Gianfrilli D, Sbardella E, Isidori AM, et al. Clinical, diagnostic, and therapeutic aspects of growth hormone deficiency during the transition period: review of the literature. Front Endocrinol. (2021) 12:634288. doi: 10.3389/fendo.2021.634288
Keywords: diminished ovarian reserve, growth hormone, oxidative stress, signaling pathway, reactive oxygen species
Citation: Han P, Xu H, Yuan Y, Wen Z, Yang J, Han L and Zhang D (2025) The role of growth hormone in assisted reproductive technology for patients with diminished ovarian reserve: from signaling pathways to clinical applications. Front. Endocrinol. 16:1551126. doi: 10.3389/fendo.2025.1551126
Received: 24 December 2024; Accepted: 31 March 2025;
Published: 17 April 2025.
Edited by:
Michio Kitajima, Takagi Hospital, JapanCopyright © 2025 Han, Xu, Yuan, Wen, Yang, Han and Zhang. This is an open-access article distributed under the terms of the Creative Commons Attribution License (CC BY). The use, distribution or reproduction in other forums is permitted, provided the original author(s) and the copyright owner(s) are credited and that the original publication in this journal is cited, in accordance with accepted academic practice. No use, distribution or reproduction is permitted which does not comply with these terms.
*Correspondence: Lei Han, aGFubGVpbW1AMTI2LmNvbQ==; Dongmei Zhang, YnlmeXpkbUAxMjYuY29t
†These authors have contributed equally to this work