- 1Post-Graduate Program in Health Sciences (PPGCS), Federal University of Maranhão (UFMA), São Luis, Brazil
- 2Service of Endocrinology, University Hospital of the Federal University of Maranhao (HUUFMA), São Luis, Brazil
- 3Research Group in Clinical and Molecular Endocrinology and Metabology (ENDOCLIM), Federal University of Maranhão (UFMA), São Luis, Brazil
- 4Clinical Research Center (CEPEC), University Hospital of the Federal University of Maranhao (HUUFMA), São Luís, Brazil
- 5Department of Medicine I, Federal University of Maranhao (UFMA), São Luis, Brazil
Craniopharyngiomas are rare intracranial tumors originating from the Rathke’s pouch, affecting the sellar and parasellar regions. Despite their benign nature, they cause significant morbidity and mortality due to their proximity to vital structures such as the optic pathways and the hypothalamic-pituitary axis, resulting in endocrine, visual, neurological impairment, and hypothalamic syndrome. Classified into adamantinomatous (ACP) and papillary (PCP), these tumors differ in epidemiology, histology, and pathophysiology. ACP, the most common type, presents a bimodal peak incidence between 5 and 15 years of age and 45 and 60 years of age, while PCP is more restricted to adults. Traditional treatments such as surgery and radiotherapy face significant challenges, including high recurrence rates. Intracystic chemotherapy is used in monocystic ACP but with limited efficacy and adverse effects related to toxicity. Recent advances in molecular biology have introduced targeted therapies, such as BRAF and MEK inhibitors, which show potential benefits in craniopharyngioma patients, particularly in the PCP. For ACP, however, therapeutic outcomes remain limited despite advances in molecular understanding, including mutations in the CTNNB1 gene and growth factors. Increasing investigation into the inflammatory microenvironment and immune response of these tumors presents new therapeutic possibilities and promising alternatives for tumor control, such as the use of anti-IL-6R, anti-VEGF agents and immune checkpoints inhibitors. This review aims to synthesize advancements in the pathophysiology of craniopharyngiomas and explore emerging therapeutic implications, focusing on precision medicine approaches for the management of this challenging disease.
1 Introduction
Craniopharyngiomas are rare intracranial epithelial tumors that develop from remnants of Rathke’s pouch, predominantly located in the sellar and parasellar regions (1, 2). Two main histological types are recognized: adamantinomatous craniopharyngioma (ACP) and papillary craniopharyngioma (PCP) (1). These tumors account for 1–3% of all primary intracranial tumors in adults and 5–10% of intracranial tumors in children, with an annual incidence ranging from 0.13 to 2 per 100,000 individuals and no gender predilection (2).
ACP is more common in children and young adults, displaying a bimodal age distribution (5–15 years and 45–60 years) and is frequently associated with somatic mutations in the CTNNB1 gene encoding β-catenin. In contrast, PCP predominantly occurs in adults, especially in the fifth and sixth decades of life, and is associated with BRAF V600E mutations (1, 3).
Although histologically classified as WHO low grade I benign tumors, craniopharyngiomas exhibit complex clinical behavior and significant morbidity and mortality (4). Treatment typically involves a multidisciplinary approach combining surgery and radiotherapy to control the tumor while preserving hypothalamic and pituitary functions. Total resection is preferred when feasible, but subtotal resection followed by radiotherapy is often used due to proximity to critical neurovascular structures, especially in cases of hypothalamic invasion (3, 5). While total resection can provide local control, it is associated with a high risk of morbidity, including hypothalamic and pituitary dysfunction (6). Subtotal resection with radiotherapy offers comparable control rates with fewer complications (7, 8). Various radiotherapy modalities are effective, but may cause side effects like visual deterioration and endocrinopathies, depending on tumor characteristics and patient factors (9, 10). The limited efficacy and considerable side effects of traditional therapies underscore the need for innovative strategies to improve therapeutic outcomes and mitigate adverse effects (11).
In light of the limitations of conventional therapeutic approaches, the use of advanced omics technologies – such as next-generation sequencing (NGS) and single-cell transcriptomics (scRNA-seq) – has emerged as a promising strategy to unravel the molecular complexity of craniopharyngiomas. These approaches provide novel insights for developing more effective and personalized therapies. By enabling the identification of specific molecular targets and stratification of patients based on unique molecular profiles, they hold the potential to optimize treatment strategies and improve clinical outcomes (12, 13).
Recent advances in molecular biology have provided new insights into the genetic basis of craniopharyngiomas. Notably, the discovery of the BRAF V600E mutation in PCP has enabled the development of targeted therapeutic interventions. The use of BRAF inhibitors, such as vemurafenib and dabrafenib, either alone or in combination with MEK inhibitors, has shown efficacy in specific cases. This represents a paradigm shift in treatment, as these therapies offer more precise and less invasive alternatives compared to traditional approaches (14, 15).
Targeted therapies offer multiple advantages in the management of craniopharyngiomas. Unlike conventional methods, which often harm surrounding healthy tissues, these therapies specifically inhibit molecular pathways driving tumor growth. Consequently, they promote significant reductions in tumor size and improved clinical outcomes while minimizing complications and preserving essential neurological and endocrine functions. This highlights their potential for achieving better long-term results (14). Understanding the genetic alterations underlying craniopharyngiomas enables a personalized approach, tailoring treatment to the molecular profile of each patient. Furthermore, positive responses in cases refractory to conventional therapies reinforce the potential of these interventions as rescue options, offering new hope to patients with limited therapeutic alternatives (14, 16, 17).
This review aims to provide a comprehensive analysis of the current understanding of craniopharyngioma pathophysiology, with a particular emphasis on targeted therapies. By compiling and synthesizing available evidence, it seeks to highlight targeted therapies evaluated in craniopharyngioma patients with a pathophysiological basis, identify knowledge gaps, address emerging challenges, and delineate areas requiring further research to guide future directions.
2 Papillary craniopharyngioma
PCPs account for approximately 10% of craniopharyngiomas and almost exclusively occur in adults, predominantly in the fifth and sixth decades of life, with a mean age of 44.7 years (18, 19). These tumors typically present as large masses in the suprasellar region, often located above a preserved infundibulum or in the infundibulo-tuberal region of the third ventricle`s floor. Clinically, patients may present with visual deficits, hormonal alterations, memory impairment, and symptoms related to intracranial hypertension (19).
Macroscopically, PCPs consist of solid or mixed round masses, containing yellowish viscous cysts and rare calcifications. Histologically, these tumors are composed of well-defined neoplastic epithelium with cauliflower-shaped papillary projections, without infiltration into adjacent brain tissue. The histological component of PCPs includes pseudopapillae of mature squamous epithelium and an anastomosing fibrovascular stroma with fine capillaries and scattered immune cells, such as macrophages and neutrophils (18, 20) (Figure 1).
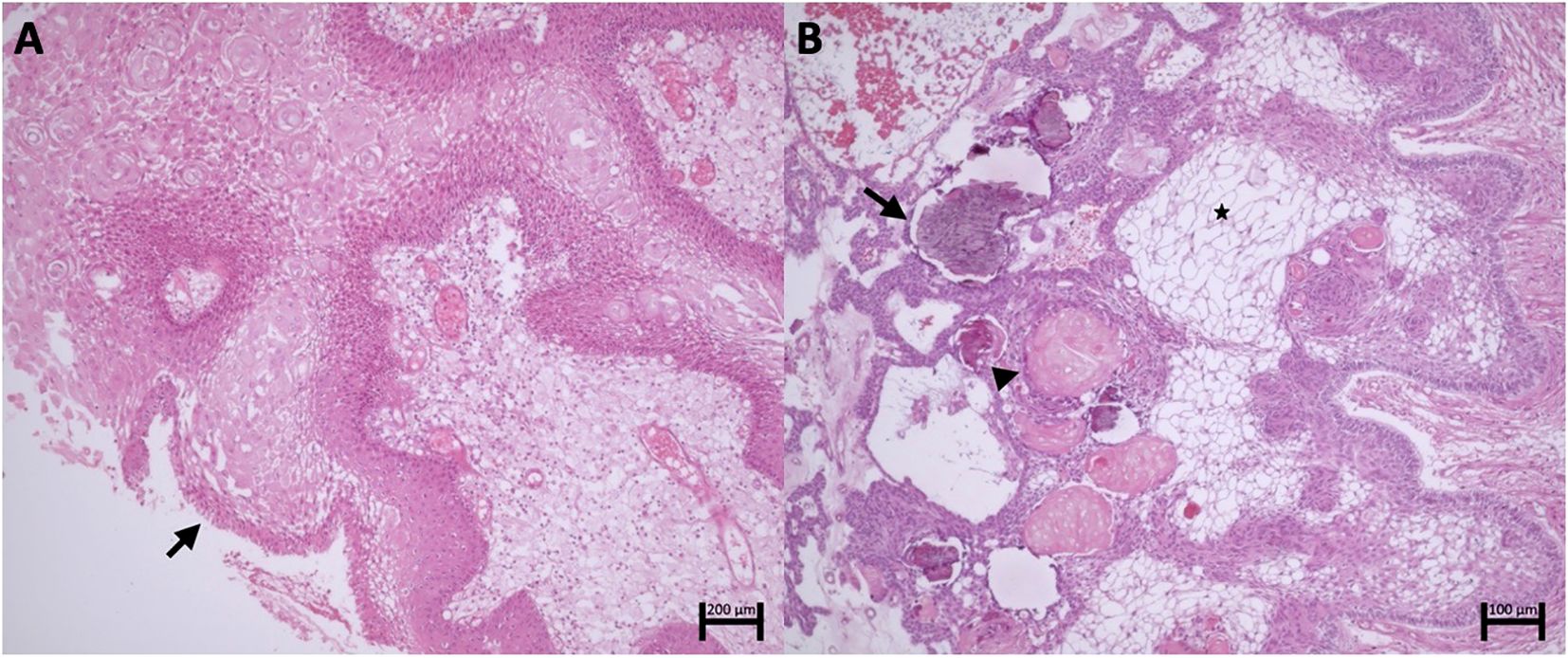
Figure 1. (A) Papillary craniopharyngioma. Fibrovascular connective axes forming papillae lined by well-differentiated squamous epithelium with palisading (arrow). (B) Adamantinomatous Craniopharyngioma. Islands and cords of epithelial cells with palisading delineating the stroma composed of stellate reticulum cells (asterisk). Clusters of anucleated squamous cells (arrowhead) and foci of calcification (arrow).
Unlike ACPs, PCPs do not present palisading reticular cells, wet keratin, or collagenous whorls, which are rare and small when present (17). Distinguishing PCPs from other suprasellar masses, such as Rathke’s pouch cysts, can be challenging (1).
In recent years, the genetic characterization of PCPs has advanced significantly. In 2014, the BRAF V600E mutation was identified through exome sequencing in three PCP samples, later confirmed in 36 of 39 additional samples (21, 22). The BRAF V600E mutation constitutively activates the MAPK/ERK pathway, an oncogenic signaling pathway that promotes the proliferation of SOX2+ embryonic cells, transforming them into tumor-initiating cells and stimulating processes such as angiogenesis and apoptosis inhibition (23, 24) (Figure 2).
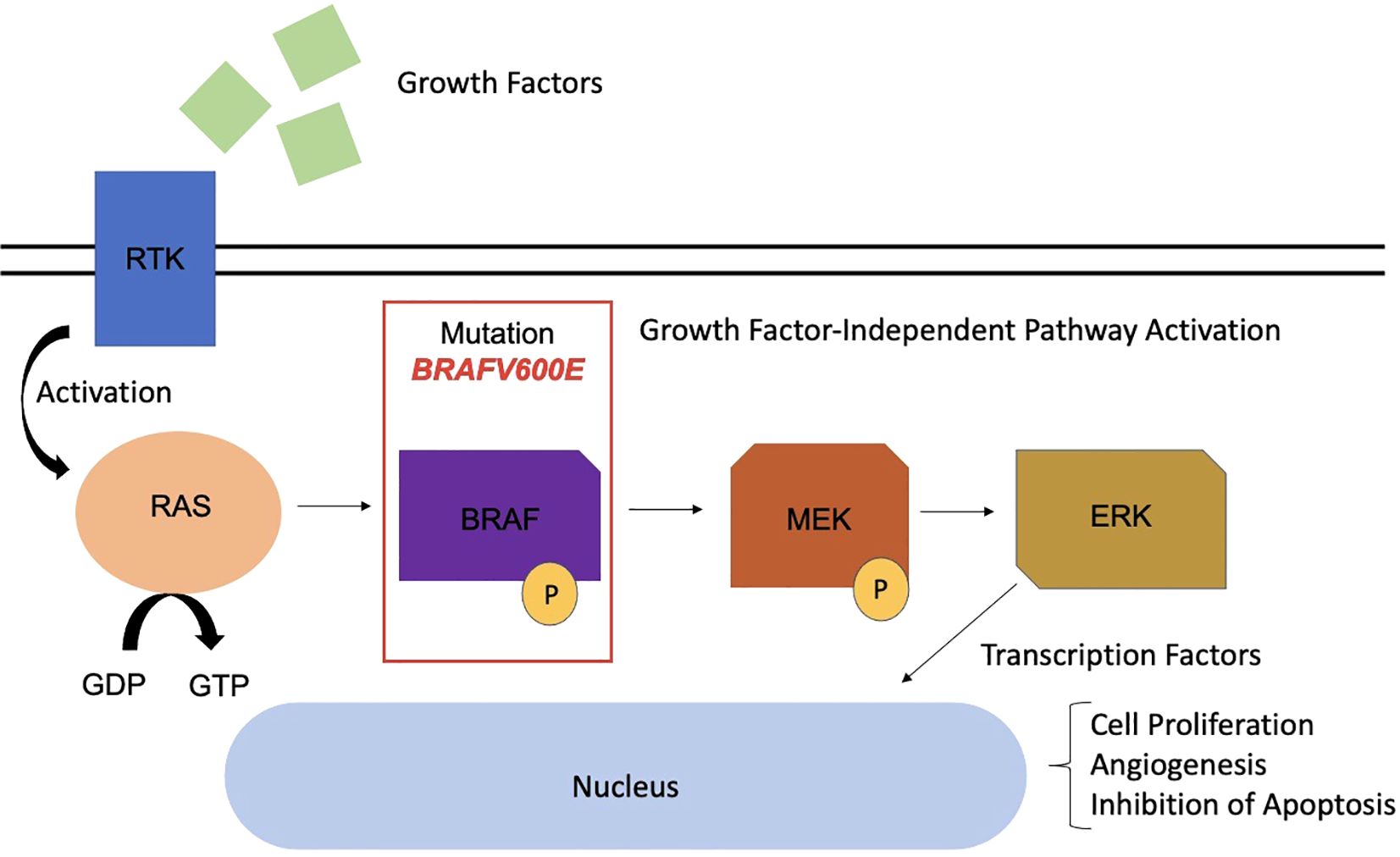
Figure 2. MAPK pathway with presence of BRAF mutation in the pathophysiology of papillary craniopharyngioma. RTK, Receptor tyrosine kinase; RAS, Rat Sarcoma; GDP, Guanosine Diphosphate; GTP, Guanosine Triphosphate; BRAF, B-Rapidly Accelerated Fibrosarcoma; MEK, Mitogen-Activated Protein Kinase; ERK, Extracellular Signal-Regulated Kinase.
The BRAF V600E mutation is identified in 81% to 100% of PCPs, serving as a genetic marker for this type (22, 24). Immunoreactivity for the VE1 antibody using immunohistochemistry confirms the presence of this mutation, while β-catenin remains localized to the cell membranes (21). This mutation is also observed in melanomas, and BRAF and MEK inhibitors such as vemurafenib, dabrafenib, and trametinib have revolutionized the treatment of these neoplasms, with promising results also for PCPs (25) (Figure 3).
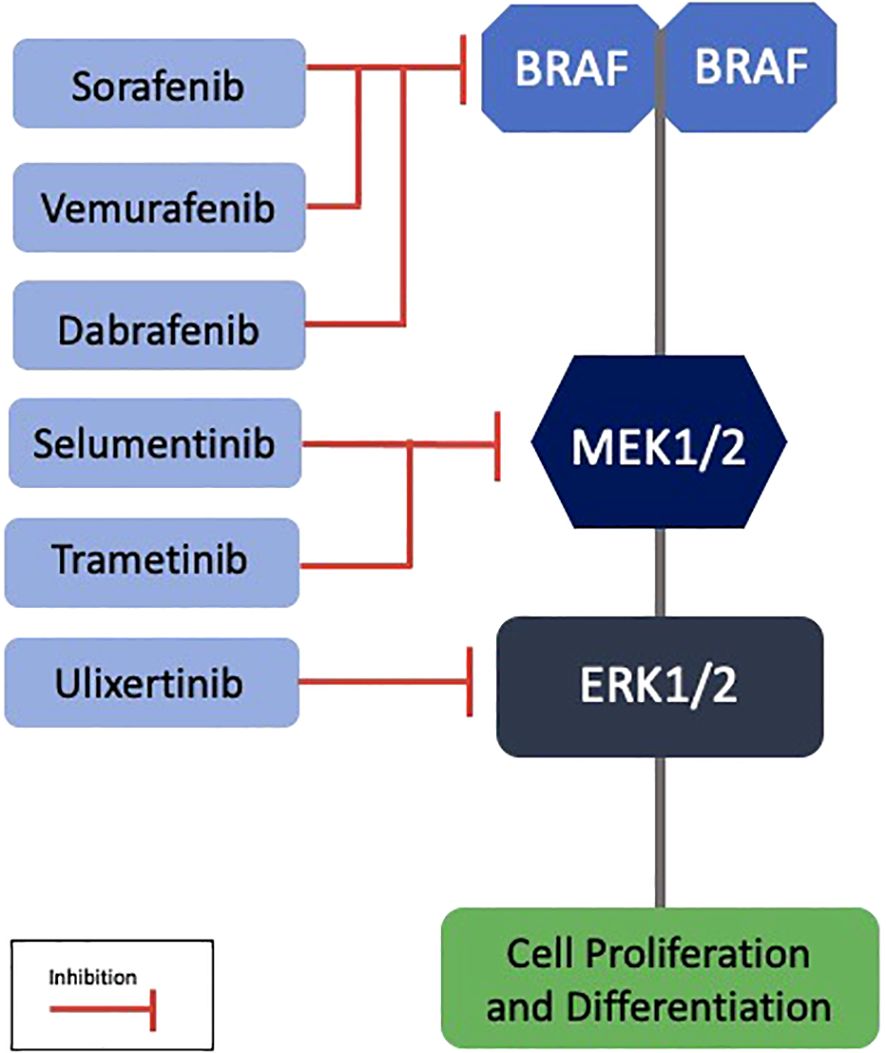
Figure 3. Targeted therapies in the MAPK pathway with potential application in papillary craniopharyngiomas. BRAF, B-Rapidly Accelerated Fibrosarcoma; MEK, Mitogen-Activated Protein Kinase; ERK, Extracellular Signal-Regulated Kinase.
Recent transcriptomic studies, including scRNA-seq have revealed distinct gene expression profiles in PCPs, marked by overexpression of genes associated with the MAPK pathway and Rathke’s pouch development (22, 26). Furthermore, characterization of the tumor microenvironment has revealed an immunosuppressive landscape enriched in regulatory T cells (13). These insights support the use of targeted therapy with BRAF and MEK inhibitors, which have yielded superior response rates in recurrent or unresectable PCPs compared to conventional treatment modalities (27, 28).
Transcriptomic data also reinforce the notion that PCPs are a molecularly homogeneous entity, with lower intratumoral heterogeneity than ACPs (13, 21, 22). Moreover, signals originating from fibroblasts and activating immune responses in neutrophils have been reported, highlighting the critical role of the tumor immune microenvironment in disease pathophysiology and the development of targeted therapies (26).
Recent studies report significant responses to treatment with BRAF and MEK inhibitors in cases of PCPs with the BRAF V600E mutation. In one of the first reported cases, there was an 85% reduction in the solid portions and 81% reduction in the cystic portions of the tumor following treatment with MEK/BRAF inhibitors (29). In a series with six patients, treatment with dabrafenib, trametinib, or vemurafenib, either alone or in combination, resulted in a reduction of 80% to 91% of the tumor masses, allowing for subsequent surgical or radiotherapeutic interventions (25).
The efficacy of these therapies with BRAF and MEK inhibitors has been extensively studied. In a phase II study, 94% of patients treated with the combination of vemurafenib and cobimetinib achieved a durable partial response, with a median tumor volume reduction of 91%. Disease progression-free survival was 87% at 12 months and 58% at 24 months, indicating significant tumor control (30). Dabrafenib, in combination with trametinib, has shown promise as a treatment option for papillary craniopharyngiomas with the BRAFV600E mutation. Studies and case reports suggest that this combination may lead to significant reductions in tumor volume and improvement in clinical symptoms, such as visual deficits and neurological dysfunctions (27, 29, 31). In a cohort study, the combination of dabrafenib and trametinib resulted in a partial response or better in 94% of patients, with an average tumor volume reduction of up to 91.8% in some treatment groups (27). A systematic review highlighted that treatment response may range from 24% to 100% volumetric reduction, with near-complete response observed in many cases (28) (Table 1).
These findings strengthen the potential of targeted therapies in the management of PCPs, especially in patients refractory to traditional treatments. The accurate identification of the genetic mutations involved not only facilitates differential diagnosis but also opens new perspectives for personalized and more effective therapeutic approaches (21).
3 Adamantinomatous craniopharyngioma
ACPs represent 90% of craniopharyngiomas and can affect individuals of any age, showing a bimodal distribution with peaks between 5–15 and 45–60 years (32). The mean age at diagnosis in children under 15 years is 8.8 years (1, 18). Studies have shown no significant differences between pediatric and adult ACPs (24, 33).
These tumors are commonly cystic, with calcifications and cholesterol-rich contents resembling “motor oil” (34). Their irregular margins, with palisading basal layers infiltrating into surrounding tissues in finger-like projections, are surrounded by intense gliosis, complicating surgical identification of planes (35). The heterogeneous tumor epithelium is juxtaposed with stellate reticulum and wet keratin nodules, composed of ghost and squamous cells, often associated with calcifications, cholesterol crystals, and hemosiderin deposits from chronic hemorrhage (1) (Figure 1).
In their pathophysiology, the Sonic Hedgehog (SHH) pathway is highly active, particularly in cell clusters and palisaded basal layers marked by Ki67 positivity (36, 37). The SHH pathway, linked to pituitary embryogenesis, appears to sustain tumor stem cells and promote tumor growth, infiltration, and angiogenesis via autocrine and paracrine mechanisms (38–40).
The pathogenesis of ACPs involves somatic mutations in the CTNNB1 gene, which encodes β-catenin (41). Normally, the Wnt pathway regulates crucial processes like growth and metabolism, with β-catenin localized to cell membranes (42). β-catenin also participates in adhesion complexes with E-cadherin, maintaining cellular architecture (43). Activating mutations in CTNNB1 are reported with prevalence rates ranging from 16% to 100%, depending on sequencing techniques (1, 17, 44). The heterogeneity in the prevalence of CTNNB1 mutations may also reflect the complex cellular composition of the tumor, which includes distinct neoplastic and non-neoplastic subpopulations such as senescent cells, tumor germ cells, and cells with different cytokeratin (CK) expression profiles (12).
Mutations in CTNNB1 affect exon 3, encoding β-catenin’s degradation complex, leading to aberrant nucleocytoplasmic accumulation in 96% of ACPs (39). This accumulation hyperactivates the Wnt pathway, critical for cell proliferation and pituitary embryogenesis, as evidenced by targets like AXIN2, LEF1, and BMP4 (37, 45). In ACPs, β-catenin accumulates in small whorled clusters or near infiltrative edges, acting as signaling centers for cell proliferation and differentiation (45, 46) (Figure 4).
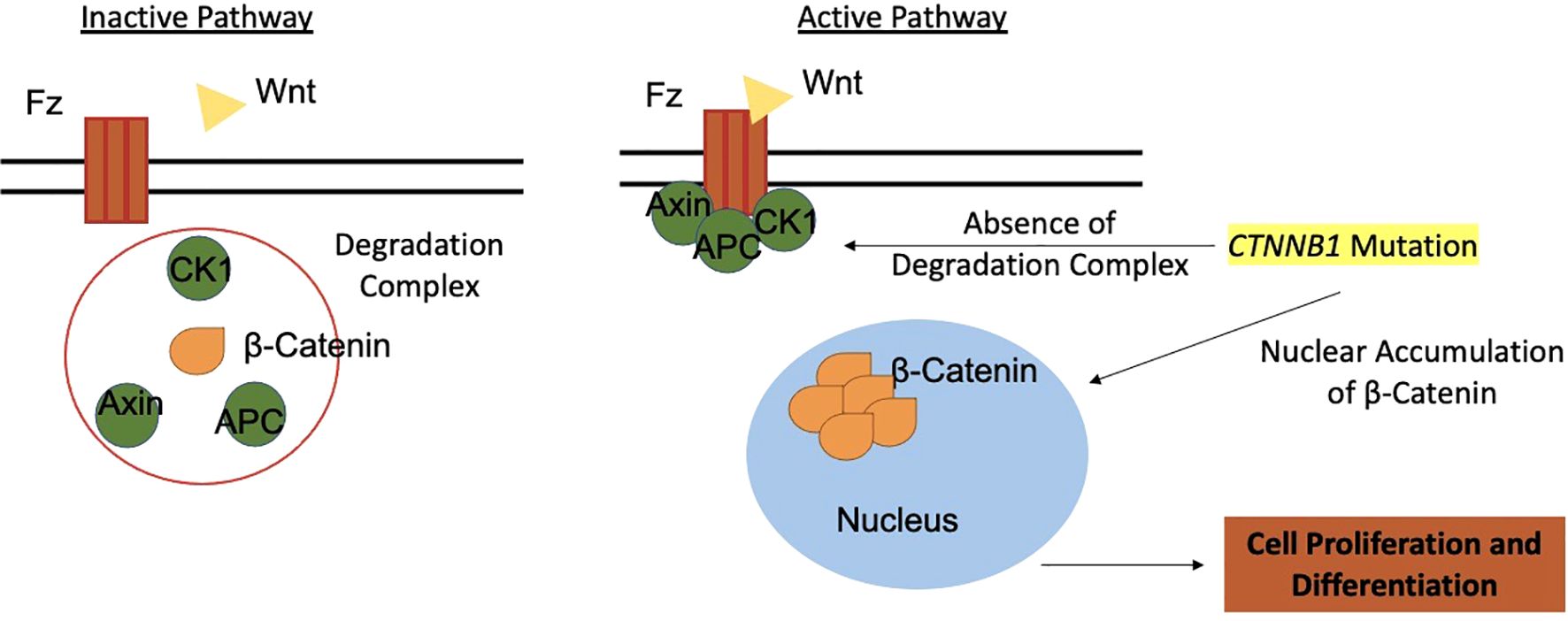
Figure 4. The Wnt pathway and its role in tumorigenesis of adamantinomatous craniopharyngiomas. Wnt, Wingless-Integration site; Fz, Frizzled receptor; CK1, Casein Kinase 1; APC, Antigen-presenting Cell; AXIN, Axin protein.
CTNNB1 mutations are specific to ACPs and absent in other sellar region tumors, such as PCPs (17, 46, 47). However, simultaneous mutations in CTNNB1 and BRAF have been identified in tumors with mixed adamantinomatous and papillary features (48). Wnt pathway hyperactivation correlates with aggressive disease and poorer overall survival rates (49).
Further studies identified fibroblast growth factors (FGFs), bone morphogenetic proteins (BMPs), and transforming growth factor-β (TGF-β) as mediators of tumor growth in ACPs (39, 45). Activation of the epidermal growth factor receptor (EGFR) stabilizes β-catenin in tumor cells coexpressing fascin, an actin-binding protein linked to adhesion, migration, invasion, and cytoskeletal reorganization. Fascin expression correlates with invasive growth and poor prognosis in ACP patients (50).
Moreover, reports suggest MAPK/ERK pathway activation in ACPs, with FGF, EGF and ERK1/2 expression colocalized with Ki67 in palisaded epithelium. Ex vivo experiments on ACPs treated with the MEK inhibitor trametinib demonstrated increased apoptosis and reduced cell proliferation, highlighting the relevance of this pathway (51).
Markers like CK, particularly CK8 and CK18, are elevated in β-catenin-positive cells, indicating dedifferentiation and tumor progression. Claudin-1 (CLDN1), a tight junction component, is downregulated in β-catenin-positive clusters and finger-like projections, suggesting a role in invasiveness. Claudins also influence cyst formation through fluid accumulation from endothelial leakage, impairing cellular adhesion (52).
Inflammation plays a central role in ACP pathophysiology. Cholesterol crystals from cystic components can stimulate IL-1β secretion, triggering immune activation (53). Spatial transcriptomic analyses have demonstrated that this inflammatory response involves heterogeneous immune cell infiltration, including tumor-associated macrophages (TAMs) with both M1 and M2 polarization. These processes may be modulated by interactions between tumor cells and the lipid-rich microenvironment (13).
Treating ACPs remains challenging, with no clear consensus on the optimal therapeutic approach. Since β-catenin is critical for normal processes, nonspecific inhibitors could harm healthy tissues (54). Therapeutic approaches vary widely, reflecting the complexity of managing ACPs. Interferon-α (INF-α) shows mixed outcomes, ranging from complete response to disease progression (55, 56). Pegylated Interferon-α-2b (INF-peg-α-2b) appears more effective, with studies showing complete responses or significant cystic reduction (57, 58). Other inflammatory mediators like tocilizumab (anti-IL-6R) and bevacizumab (anti-VEGF) are promising, with reports of significant tumor volume reduction after recurrences (59–61). Understanding the role of the MEK/ERK pathway in ACPs may also enable therapeutic targeting. MEK inhibitor binimetinib reduced tumor volume by over 95% after 21 months of treatment (62) (Table 2).
Despite these advances, therapeutic responses remain highly variable. The molecular and cellular heterogeneity of ACPs – revealed through single-cell and spatial transcriptomic approaches – emphasizes the need for individualized treatments tailored to each tumor’s unique profile (63). Integrated multi-omics analyses encompassing genomic, transcriptomic, proteomic, and spatial data, combined with detailed microenvironment characterization, represent a promising strategy for identifying predictive biomarkers, stratifying patients, and developing more precise and effective therapies (12). A deeper understanding of the cellular and molecular crosstalk within the tumor microenvironment—including the roles of senescent cells, tumor germ cells, and macrophage polarization—may ultimately pave the way for innovative therapeutic approaches in ACP treatment (13, 64–67).
4 Inflammatory mediators and immune response
Inflammation plays a significant role in the pathophysiology of craniopharyngiomas, influencing both the biological behavior of the tumor and the clinical prognosis of patients (11, 51). Several inflammatory mediators, including interleukins (IL-6, IL-8, IL-10), tumor necrosis factor (TNF), and chemokines (CXCL12, CXCR4), are implicated in tumor progression and the pathological inflammatory microenvironment of these tumors (68). The presence of a pronounced inflammatory response correlates with a higher incidence of hypopituitarism and a lower likelihood of recurrence-free survival (68). Moreover, β-catenin, frequently mutated in adamantinomatous craniopharyngiomas (ACPs), not only participates in Wnt signaling but also interacts with inflammatory mediators, amplifying cytokine and chemokine production in the tumor microenvironment. This process establishes a vicious cycle wherein inflammation perpetuates cellular proliferation and fosters tumor recurrence (69).
4.1 Interleukins
Interleukins, such as IL-6 and IL-8, are often found in elevated levels within tumor tissues and are associated with the promotion of a pro-tumorigenic microenvironment (70). IL-6 is known for its ability to enhance tumor invasion and angiogenesis while mediating communication between tumor cells and immune cells, such as tumor-associated macrophages, which can increase tumor aggressiveness. This occurs via epithelial-mesenchymal transition (EMT), a process that boosts the migratory capacity of tumor cells, facilitating local invasion (71). Furthermore, elevated levels of IL-6 and its receptor (IL-6R) suggest that this signaling pathway could be a potential therapeutic target, as demonstrated in studies exploring the use of tocilizumab, a monoclonal antibody that blocks IL-6R, to reduce cystic volume in ACP patients (60).
IL-8 also plays a significant role in the tumor microenvironment of craniopharyngiomas, contributing to local invasion and adhesion to surrounding tissues (72). Studies indicate that IL-8, alongside other pro-inflammatory cytokines such as IL-6, is elevated in the plasma of patients and brain tumor tissues (68, 73). IL-8 is further recognized for its pro-tumorigenic functions across various cancers, including promoting angiogenesis, increasing cellular proliferation and survival, and facilitating cell migration (72). Although specific literature on craniopharyngiomas is limited, IL-8’s role in other tumors suggests that it may contribute to tumor progression through similar mechanisms by modulating the tumor microenvironment (68, 74).
Vascular Endothelial Growth Factor (VEGF), a key mediator of angiogenesis, is an essential component in craniopharyngioma pathophysiology, driving the formation of new blood vessels that supply the tumor with nutrients and oxygen (75). It is present in epithelial cells of both ACP and PCP, and microvascular density related to VEGF expression correlates with tumor recurrence, suggesting the prognostic value of angiogenesis extent. VEGF is also linked to cyst formation in craniopharyngiomas, with its expression appearing to correlate with tumor size (76). Regulated by β-catenin and other molecular pathways, VEGF interacts with matrix metalloproteinases (MMPs), such as MMP-9, to remodel the extracellular matrix and facilitate tumor invasion. This remodeling may be critical for craniopharyngioma progression. MMP-1, for instance, can enhance VEGF-2R expression on endothelial cells, promoting cellular proliferation and angiogenesis via intracellular signaling pathways (77). Evidence also suggests that MMPs may induce the production of the anti-apoptotic protein Bcl-2, which regulates tumor growth and recurrence through autocrine and paracrine mechanisms (78). Dysregulation of apoptosis-related genes, including members of the Bcl-2 family, seems to play a significant role in the pathogenesis of pituitary adenomas (79). Although the specific role of MMPs in craniopharyngiomas remains underexplored, their involvement in angiogenesis and VEGF modulation suggests they contribute to tumor pathogenesis.
The interplay between IL-6 and VEGF establishes a pathological axis that amplifies inflammation and vascularization in the tumor microenvironment. IL-6 can upregulate VEGF expression, creating a feedback loop that intensifies these processes (80). This interaction also contributes to resistance to conventional therapies, highlighting the need for integrated approaches to disrupt this cycle.
While studies on anti-IL-6 and anti-VEGF therapies in craniopharyngiomas remain limited, preliminary evidence suggests therapeutic potential. A study reported the combined use of tocilizumab (anti-IL-6R) and bevacizumab (anti-VEGF) in two patients with recurrent cystic ACPs, resulting in significant responses, including reduced cystic tumor burden and tumor stability, suggesting a viable therapeutic alternative (60).
Inflammatory mediators also play a significant role in PCPs, influencing both tumor invasiveness and the immune microenvironment. The use of preoperative inflammatory markers, such as neutrophil counts and the neutrophil-to-lymphocyte ratio, can aid in the differential diagnosis of PCPs from other sellar region tumors, with higher levels observed in PCPs. These tumors exhibit high immune infiltration but with low activity, attributed to extensive neutrophil infiltration that creates an inactive immune microenvironment (81). Furthermore, elevated IL-6 expression is positively correlated with PCP tumor invasion into the hypothalamus, suggesting that these inflammatory mediators could serve as potential therapeutic targets to prevent tumor invasion (82).
4.2 Chemokines
Chemokines from the CXC and CC families play significant roles in craniopharyngioma pathophysiology, particularly in ACP. The CXCL12/CXCR4 axis is specifically implicated in tumor progression. Overexpression of CXCL12 and CXCR4 in ACP promotes tumor cell proliferation, migration, and invasion, primarily via the activation of the PI3K/AKT signaling pathway (83, 84). This pathway is critical in regulating cell growth and survival, indicating that the CXCL12/CXCR4 axis contributes to tumor aggressiveness. These chemokines are also recognized for their capacity to influence the tumor microenvironment by modulating angiogenesis and immune responses, potentially affecting tumor progression (85). Although the impact of other CC and CXC chemokines on craniopharyngiomas is less studied, their roles in other cancers suggest similar contributions to tumor growth and invasion (86).
4.3 Immune checkpoint
Immune checkpoints are essential molecules and pathways in the immune system that regulate immune responses, preventing them from becoming destructive to the body’s healthy cells. These include inhibitory receptors and ligands that play a crucial role in modulating T-cell activation and function. Under normal conditions, these checkpoints help maintain immune tolerance and prevent collateral damage during antimicrobial immune responses (87). In the context of cancer, tumor cells can exploit these checkpoints to evade immune destruction. This occurs when proteins on the surface of T-cells, known as immune checkpoint proteins, recognize and bind to partner proteins on cancer cells, sending inhibitory signals that suppress the immune response (88). Therapies that block these checkpoints, known as immune checkpoint inhibitors, have shown significant efficacy in restoring T-cell capability to attack tumor cells, leading to robust tumor regressions (89).
Immune checkpoint inhibitors have demonstrated significant potential in the treatment of various cancer types. Blocking programmed cell death protein 1 (PD-1) and its ligand (PD-L1) with agents such as nivolumab and pembrolizumab has improved survival in cases of melanoma and non-small cell lung adenocarcinoma (90, 91). The availability of these agents, combined with their relatively favorable side effect profiles, has prompted numerous studies investigating their efficacy across various tumor types.
Recent studies have demonstrated PD-L1 expression in the epithelial cells lining cysts and intrinsic PD-1 expression in cell clusters in ACPs with β-catenin overexpression. These clusters play a central role in tumor growth in ACPs through various mechanisms, making PD-1 targeting a promising therapy (92, 93). Two more studies also demonstrated elevated PD-L1 expression in ACPs, revealing this as a potential therapeutic target in craniopharyngiomas (94, 95).
5 Ongoing clinical trials
In recent years, the multi-omic characterization of craniopharyngiomas has provided significant insights into their pathogenesis, driving clinical trials aimed at developing more personalized and lower-risk therapeutic approaches. Ongoing studies with binimetinib (NCT05286788), tocilizumab (NCT05233397), and nivolumab (NCT05465174) for ACP as well as vemurafenib and cobimetinib (NCT03224767) for the PCP, offer promising therapeutic alternatives and may help define optimal patient profiles (96) (Figure 5) (Table 3). However, some challenges still remain regarding the optimal timing for introducing these treatments—whether as neoadjuvant therapy to reduce tumor volume before surgery or as adjuvant therapy to minimize recurrence and improve disease control—as well as the appropriate duration of treatment. Furthermore, uncertainties persist regarding their use as monotherapy, in combination with other agents, or in conjunction with surgery and radiotherapy, underscoring the need for further studies to refine the management of these rare tumors.
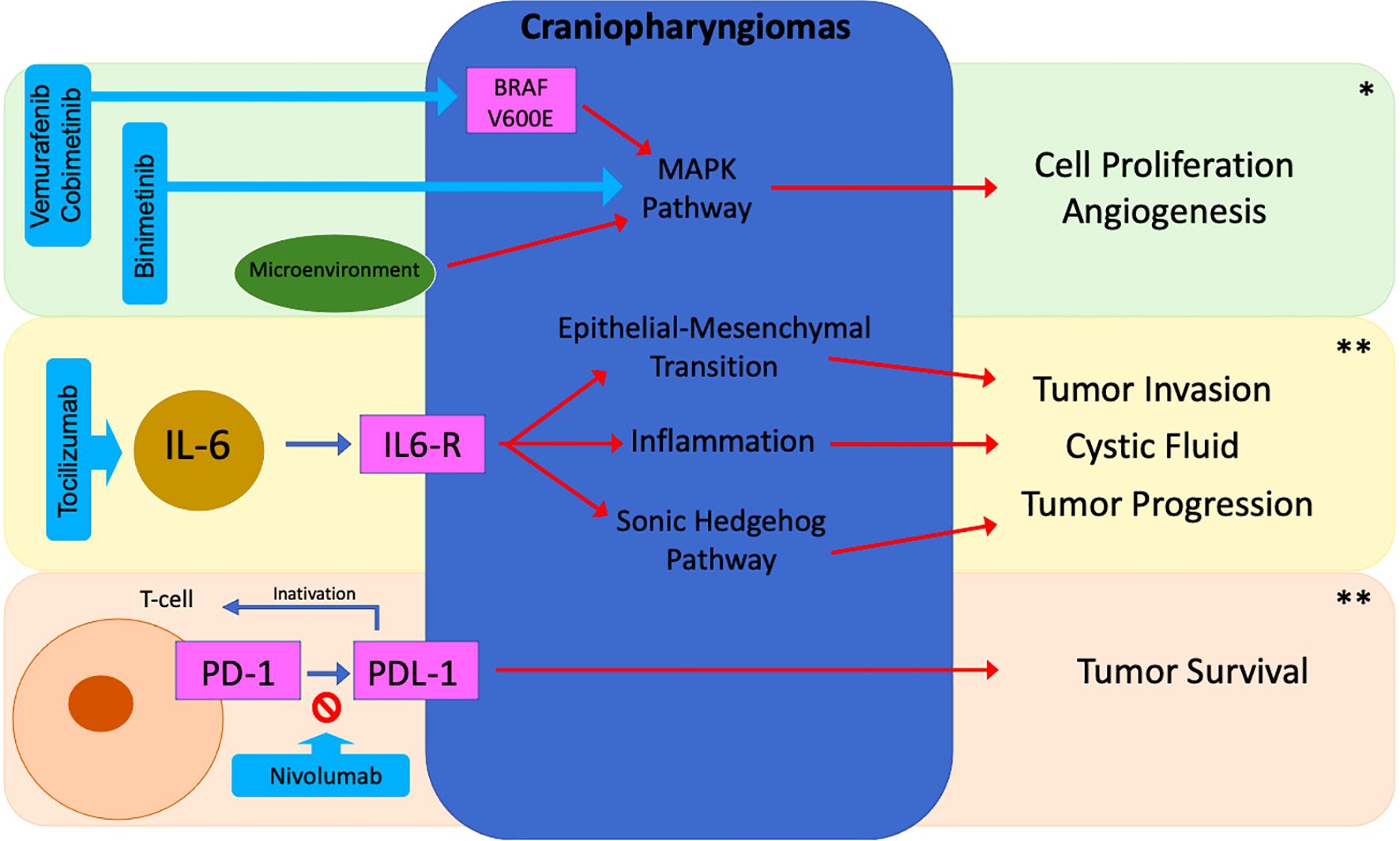
Figure 5. Potential targeted therapies in craniopharyngiomas. *ACP/PCP; **ACP; BRAF, B-Rapidly Accelerated Fibrosarcoma; IL-6, Interleukin 6; PD-1, Programmed Cell Death Protein 1; PD-L1, Programmed Cell Death Protein Ligand 1.
6 Conclusion
Recent advances in understanding the pathophysiology of craniopharyngiomas provide a promising foundation for developing more effective therapeutic strategies. Given the limitations of conventional approaches, targeted therapies, such as BRAF and MEK inhibitors for the PCP, have shown encouraging results. Recently, the growing understanding of the inflammatory behavior and immune response of these tumors has highlighted the therapeutic potential of anti-IL-6R and anti-VEGF agents and immune checkpoints inhibitors, signaling a promising future for the application of precision medicine in this field in both, APC and PCP.
Significant gaps still remain, particularly in managing more aggressive or resistant tumors. Robust clinical trials and interinstitutional collaborations are crucial to validate these therapies on a larger scale and standardize treatment protocols. Additionally, identifying predictive biomarkers and elucidating molecular interactions within the tumor microenvironment may offer new therapeutic opportunities, improve prognostic outcomes, and reduce the morbidity and mortality associated with craniopharyngiomas.
Author contributions
CP: Conceptualization, Writing – original draft, Writing – review & editing. GN: Writing – original draft, Writing – review & editing. SD: Writing – original draft, Writing – review & editing. MF: Conceptualization, Writing – original draft, Writing – review & editing.
Funding
The author(s) declare that financial support was received for the research and/or publication of this article. This study was supported by CNPq (Conselho Nacional de Pesquisa – Finance code 406304/2024-6) and CAPES (Coordenacao de Aperfeiçoamento de Pessoal de Nível Superior - Finance code 001).
Conflict of interest
The authors declare that the research was conducted in the absence of any commercial or financial relationships that could be construed as a potential conflict of interest.
Generative AI statement
The author(s) declare that no Generative AI was used in the creation of this manuscript.
Publisher’s note
All claims expressed in this article are solely those of the authors and do not necessarily represent those of their affiliated organizations, or those of the publisher, the editors and the reviewers. Any product that may be evaluated in this article, or claim that may be made by its manufacturer, is not guaranteed or endorsed by the publisher.
References
1. Müller HL, Merchant TE, Warmuth-Metz M, Martinez-Barbera JP, Puget S. Craniopharyngioma. Nat Rev Dis Primer. (2019) 5:75. doi: 10.1038/s41572-019-0125-9
2. Alboqami MN, Khalid S Albaiahy A, Bukhari BH, Alkhaibary A, Alharbi A, Khairy S, et al. Craniopharyngioma: A comprehensive review of the clinical presentation, radiological findings, management, and future Perspective. Heliyo. (2024) 10. doi: 10.1016/j.heliyon.2024.e32112
3. Piloni M, Gagliardi F, Bailo M, Losa M, Boari N, Spina A, et al. Craniopharyngioma in pediatrics and adults. Adv Exp Med Bio. (2023) 1405:299–329. doi: 10.1007/978-3-031-23705-8_11
4. Louis DN, Perry A, Wesseling P, Brat DJ, Cree IA, Figarella-Branger D, et al. The 2021 WHO classification of tumors of the Central Nervous System: A summary. Neuro Onco. (2021) 23:1231–51. doi: 10.1093/neuonc/noab106
5. Henderson F, Schwartz TH. Update on management of craniopharyngiomas. J Neuroonco. (2022) 156:97–108. doi: 10.1007/s11060-021-03906-4
6. Müller HL. Consequences of craniopharyngioma surgery in children. J Clin Endocrinol Meta. (2011) 96:1981–91. doi: 10.1210/jc.2011-0174
7. Poiset SJ, Song A, In Yoon H, Huang J, Jain S, Palmer JD, et al. Long-term outcomes of surgery and radiation treatment for adult patients with craniopharyngioma. World Neurosur. (2024) 187:e852–9. doi: 10.1016/j.wneu.2024.04.177
8. Khriguian J, Tolba M, Khosrow-Khavar F, Kordlouie S, Guiot MC, Abdulkarim B, et al. Is postoperative radiotherapy needed in the management of adult craniopharyngiomas? Can J Neurol Sc. (2023) 50:428–34. doi: 10.1017/cjn.2022.28
9. Harary PM, Rajaram S, Hori YS, Park DJ, Chang SD. The spectrum of radiation therapy options for craniopharyngioma: a systematic review. J Neuroonco. (2025). doi: 10.1007/s11060-025-05001-4
10. Rutenberg MS, Holtzman AL, Indelicato DJ, Huh S, Rao D, Fiester PJ, et al. Disease control after radiotherapy for adult craniopharyngioma: Clinical outcomes from a large single-institution series. J Neuroonco. (2022) 157:425–33. doi: 10.1007/s11060-022-03983-z
11. Whelan R, Hengartner A, Folzenlogen Z, Prince E, Hankinson TC. Adamantinomatous craniopharyngioma in the molecular age and the potential of targeted therapies: a review. Childs Nerv Sys. (2020) 36:1635–42. doi: 10.1007/s00381-020-04677-5
12. An W, Li S, An Y, Lin Z. Molecular subtypes of adamantinomatous craniopharyngiomas. Neuro Onco. (2025). doi: 10.1093/neuonc/noaf030
13. Matsuda T, Kono T, Taki Y, Sakuma I, Fujimoto M, Hashimoto N, et al. Deciphering craniopharyngioma subtypes: Single-cell analysis of tumor microenvironment and immune networks. iScienc. (2024) 27:111068. doi: 10.1016/j.isci.2024.111068
14. Reyes M, Taghvaei M, Yu S, Sathe A, Collopy S, Prashant GN, et al. Targeted therapy in the management of modern craniopharyngiomas. Front Biosci (Landmark Ed. (2022) 27:136. doi: 10.31083/j.fbl2704136
15. Calvanese F, Jacquesson T, Manet R, Vasiljevic A, Lasolle H, Ducray F, et al. Neoadjuvant B-RAF and MEK inhibitor targeted therapy for adult Papillary Craniopharyngiomas: A new treatment paradigm. Front Endocrinol (Lausanne. (2022) 13:882381. doi: 10.3389/fendo.2022.882381
16. Hengartner AC, Prince E, Vijmasi T, Hankinson TC. Adamantinomatous craniopharyngioma: moving toward targeted therapies. Neurosurg Focu. (2020) 48:E7. doi: 10.3171/2019.10.FOCUS19705
17. Larkin S, Karavitaki N. Recent advances in molecular pathology of craniopharyngioma. F1000Re. (2017) 6:1202. doi: 10.12688/f1000research.11549.1
18. Karavitaki N, Wass JAH. Craniopharyngiomas. Endocrinol Metab Clin North A. (2008) 37:173–93,ix–x. doi: 10.1016/j.ecl.2007.10.012
19. Jannelli G, Calvanese F, Paun L, Raverot G, Jouanneau E. Current advances in papillary craniopharyngioma: State-of-the-art therapies and overview of the literature. Brain Sc. (2023) 13. doi: 10.3390/brainsci13030515
20. Larkin SJ, Ansorge O. Pathology and pathogenesis of craniopharyngiomas. Pituitar. (2013) 16:9–17. doi: 10.1007/s11102-012-0418-4
21. Brastianos PK, Santagata S. ENDOCRINE TUMORS: BRAF V600E mutations in papillary craniopharyngioma. Eur J Endocrino. (2016) 174:R139–44. doi: 10.1530/EJE-15-0957
22. Brastianos PK, Taylor-Weiner A, Manley PE, Jones RT, Dias-Santagata D, Thorner AR, et al. Exome sequencing identifies BRAF mutations in papillary craniopharyngiomas. Nat Gene. (2014) 46:161–5. doi: 10.1038/ng.2868
23. Haston S, Pozzi S, Carreno G, Manshaei S, Panousopoulos L, Gonzalez-Meljem JM, et al. MAPK pathway control of stem cell proliferation and differentiation in the embryonic pituitary provides insights into the pathogenesis of papillary craniopharyngioma. Developmen. (2017) 144:2141–52. doi: 10.1242/dev.150490
24. Hölsken A, Sill M, Merkle J, Schweizer L, Buchfelder M, Flitsch J, et al. Adamantinomatous and papillary craniopharyngiomas are characterized by distinct epigenomic as well as mutational and transcriptomic profiles. Acta Neuropathol Commu. (2016) 4:20. doi: 10.1186/s40478-016-0287-6
25. Juratli TA, Jones PS, Wang N, Subramanian M, Aylwin SJB, Odia Y, et al. Targeted treatment of papillary craniopharyngiomas harboring BRAF V600E mutations. Cance. (2019) 125:2910–4. doi: 10.1002/cncr.32197
26. Zhu X, Jia Y, Zhao Z, Zhang X, Zhao Y, Gui S, et al. Cell signaling communication within papillary craniopharyngioma revealed by an integrated analysis of single-cell RNA sequencing and bulk RNA sequencing. J Transl Me. (2025) 23:124. doi: 10.1186/s12967-025-06149-3
27. De Alcubierre D, Gkasdaris G, Mordrel M, Joncour A, Briet C, Almairac F, et al. BRAF and MEK inhibitor targeted therapy in papillary craniopharyngiomas: a cohort study. Eur J Endocrino. (2024) 191:251–61. doi: 10.1093/ejendo/lvae091
28. Cossu G, Ramsay DSC, Daniel RT, El Cadhi A, Kerherve L, Morlaix E, et al. Update on neoadjuvant and adjuvant BRAF inhibitors in papillary craniopharyngioma: A systematic review. Cancers (Basel. (2024) 16. doi: 10.3390/cancers16203479
29. Brastianos PK, Shankar GM, Gill CM, Taylor-Weiner A, Nayyar N, Panka DJ, et al. Dramatic response of BRAF V600E mutant papillary craniopharyngioma to targeted therapy. J Natl Cancer Ins. (2016) 108:djv310. doi: 10.1093/jnci/djv310
30. Brastianos PK, Twohy E, Geyer S, Gerstner ER, Kaufmann TJ, Tabrizi S, et al. BRAF–MEK inhibition in newly diagnosed papillary Craniopharyngiomas. New Engl J Medicin. (2023) 389:118–26. doi: 10.1056/NEJMoa2213329
31. Fasano M, Della Corte CM, Caterino M, Pirozzi M, Rauso R, Troiani T, et al. Dramatic therapeutic response to dabrafenib plus trametinib in BRAF V600E mutated papillary craniopharyngiomas: A case report and literature review. Front Med (Lausann. (2021) 8:652005. doi: 10.3389/fmed.2021.652005
32. Momin AA, Recinos MA, Cioffi G, Patil N, Soni P, Almeida JP, et al. Descriptive epidemiology of craniopharyngiomas in the United States. Pituitar. (2021) 24:517–22. doi: 10.1007/s11102-021-01127-6
33. Prince E, Whelan R, Donson A, Staulcup S, Hengartner A, Vijmasi T, et al. Transcriptional analyses of adult and pediatric adamantinomatous craniopharyngioma reveals similar expression signatures regarding potential therapeutic targets. Acta Neuropathol Commu. (2020) 8:68. doi: 10.1186/s40478-020-00939-0
34. Petito CK, DeGirolami U, Earle KM. Craniopharyngiomas: a clinical and pathological review. Cance. (1976) 37:1944–52. doi: 10.1002/1097-0142(197604)37:4<1944::aid-cncr2820370446>3.0.co
35. Prabhu VC, Brown HG. The pathogenesis of craniopharyngiomas. Childs Nerv Sys. (2005) 21:622–7. doi: 10.1007/s00381-005-1190-9
36. Gomes DC, Jamra SA, Leal LF, Colli LM, Campanini ML, Oliveira RS, et al. Sonic Hedgehog pathway is upregulated in adamantinomatous craniopharyngiomas. Eur J Endocrino. (2015) 172:603–8. Available at: https://www.embase.com/search/results?subaction=viewrecord&id=L604241794&from=export (Accessed January 5, 2025).
37. Gaston-Massuet C, Andoniadou CL, Signore M, Jayakody SA, Charolidi N, Kyeyune R, et al. Increased Wingless (Wnt) signaling in pituitary progenitor/stem cells gives rise to pituitary tumors in mice and humans. Proc Natl Acad Sci U S A. (2011) 108:11482–7. doi: 10.1073/pnas.1101553108
38. Carreno G, Boult JKR, Apps J, Gonzalez-Meljem JM, Haston S, Guiho R, et al. SHH pathway inhibition is protumourigenic in adamantinomatous craniopharyngioma. Endocr Relat Cancer. (2019) 26:355–66. doi: 10.1530/ERC-18-0538
39. Müller HL, Martinez-Barbera JP. Adamantinomatous craniopharyngioma: genomics, radiologic findings, clinical, and prognosis. Contemp Endocrinology. (2019), 41–70. Available at: https://www.embase.com/search/results?subaction=viewrecord&id=L627371563&from=export (Accessed January 5, 2025).
40. Garcia-Lavandeira M, Saez C, Diaz-Rodriguez E, Perez-Romero S, Senra A, Dieguez C, et al. Craniopharyngiomas express embryonic stem cell markers (SOX2, OCT4, KLF4, and SOX9) as pituitary stem cells but do not coexpress RET/GFRA3 receptors. J Clin Endocrinol Meta. (2012) 97. doi: 10.1210/jc.2011-2187
41. Campanini ML, Colli LM, Paixao BMC, Cabral TPF, Amaral FC, MaChado HR, et al. CTNNB1 gene mutations, pituitary transcription factors, and microRNA expression involvement in the pathogenesis of adamantinomatous craniopharyngiomas. Horm Cancer. (2010) 1:187–96. Available at: https://www.embase.com/search/results?subaction=viewrecord&id=L51134991&from=export (Accessed January 5, 2025).
42. Preda V, Larkin SJ, Karavitaki N, Ansorge O, Grossman AB. The wnt signalling cascade and the adherens junction complex in craniopharyngioma tumorigenesis. Endocr Patho. (2015) 26:1–8. doi: 10.1007/s12022-014-9341-8
43. Huber AH, Weis WI. The structure of the beta-catenin/E-cadherin complex and the molecular basis of diverse ligand recognition by beta-catenin. Cell. (2001) 105:391–402. doi: 10.1016/s0092-8674(01)00330-0
44. Apps JR, Stache C, Gonzalez-Meljem JM, Gutteridge A, Chalker J, Jacques TS, et al. CTNNB1 mutations are clonal in adamantinomatous craniopharyngioma. Neuropathol Appl Neurobio. (2020) 46:510–4. doi: 10.1111/nan.12613
45. Andoniadou CL, Gaston-Massuet C, Reddy R, Schneider RP, Blasco MA, Le Tissier P, et al. Identification of novel pathways involved in the pathogenesis of human adamantinomatous craniopharyngioma. Acta Neuropathol. (2012) 124:259–71. doi: 10.1007/s00401-012-0957-9
46. Martinez-Barbera JP. Molecular and cellular pathogenesis of adamantinomatous craniopharyngioma. Neuropathol Appl Neurobio. (2015) 41:721–32. doi: 10.1111/nan.12226
47. Buslei R, Nolde M, Hofmann B, Meissner S, Eyupoglu IY, Siebzehnrübl F, et al. Common mutations of beta-catenin in adamantinomatous craniopharyngiomas but not in other tumours originating from the sellar region. Acta Neuropathol. (2005) 109:589–97. doi: 10.1007/s00401-005-1004-x
48. Prieto R, Pascual JM. Can tissue biomarkers reliably predict the biological behavior of craniopharyngiomas? A comprehensive overview. Pituitary. (2018) 21:431–42. doi: 10.1007/s11102-018-0890-6
49. Li Z, Xu J, Huang S, You C. Aberrant membranous expression of β-catenin predicts poor prognosis in patients with craniopharyngioma. Ann Diagn Patho. (2015) 19:403–8. doi: 10.1016/j.anndiagpath.2015.10.002
50. Hölsken A, Gebhardt M, Buchfelder M, Fahlbusch R, Blümcke I, Buslei R. EGFR signaling regulates tumor cell migration in craniopharyngiomas. Clin Cancer Re. (2011) 17:4367–77. doi: 10.1158/1078-0432.CCR-10-2811
51. Apps JR, Carreno G, Gonzalez-Meljem JM, Haston S, Guiho R, Cooper JE, et al. Tumour compartment transcriptomics demonstrates the activation of inflammatory and odontogenic programmes in human adamantinomatous craniopharyngioma and identifies the MAPK/ERK pathway as a novel therapeutic target. Acta Neuropathol. (2018) 135:757–77. doi: 10.1007/s00401-018-1830-2
52. Stache C, Hölsken A, Fahlbusch R, Flitsch J, Schlaffer SM, Buchfelder M, et al. Tight junction protein claudin-1 is differentially expressed in craniopharyngioma subtypes and indicates invasive tumor growth. Neuro Onco. (2014) 16:256–64. doi: 10.1093/neuonc/not195
53. Burghaus S, Hölsken A, Buchfelder M, Fahlbusch R, Riederer BM, Hans V, et al. A tumor-specific cellular environment at the brain invasion border of adamantinomatous craniopharyngiomas. Virchows Archiv. (2010) 456:287–300. doi: 10.1007/s00428-009-0873-0
54. de Pellegars-Malhortie A, Picque Lasorsa L, Mazard T, Granier F, Prévostel C. Why is Wnt/β-catenin not yet targeted in routine cancer care? Pharmaceuticals (Basel). (2024) 17:949. doi: 10.3390/ph17070949
55. Agosti E, Zeppieri M, Antonietti S, Piazza A, Ius T, Fontanella MM, et al. Advancing craniopharyngioma management: A systematic review of current targeted therapies and future perspectives. Int J Mol Sc. (2024) 25. doi: 10.3390/ijms25020723
56. Jakacki RI, Cohen BH, Jamison C, Mathews VP, Arenson E, Longee DC, et al. Phase II evaluation of interferon-alpha-2a for progressive or recurrent craniopharyngiomas. J Neurosurg. (2000) 92:255–60. Available at: https://thejns.org/view/journals/j-neurosurg/92/2/article-p255.xml (Accessed January 5, 2025).
57. Yeung JT, Pollack IF, Panigrahy A, Jakacki RI. Pegylated interferon-α-2b for children with recurrent craniopharyngioma. J Neurosurg Pediat. (2012) 10:498–503. Available at: https://thejns.org/pediatrics/view/journals/j-neurosurg-pediatr/10/6/article-p498.xml (Accessed January 5, 2025).
58. Goldman S, Pollack IF, Jakacki RI, Billups CA, Poussaint TY, Adesina AM, et al. Phase II study of peginterferon alpha-2b for patients with unresectable or recurrent craniopharyngiomas: a Pediatric Brain Tumor Consortium report. Neuro Onco. (2020) 22:1696–704. doi: 10.1093/neuonc/noaa119
59. de Vos-Kerkhof E, Buis DR, Lequin MH, Bennebroek CA, Aronica E, Hulleman E, et al. Tocilizumab for the fifth progression of cystic childhood craniopharyngioma-a case report. Front Endocrinol (Lausanne). (2023) 14:1225734. doi: 10.3389/fendo.2023.1225734
60. Grob S, Mirsky DM, Donson AM, Dahl N, Foreman NK, Hoffman LM, et al. Targeting IL-6 is a potential treatment for primary cystic craniopharyngioma. Front Onco. (2019) 9:791. doi: 10.3389/fonc.2019.00791
61. De Rosa A, Calvanese F, Ducray F, Vasiljevic A, Manet R, Raverot G, et al. First evidence of anti-VEGF efficacy in an adult case of adamantinomatous craniopharyngioma: Case report and illustrative review. Ann Endocrinol (Paris). (2023) 84:727–33. doi: 10.1016/j.ando.2023.10.003
62. Patel K, Allen J, Zagzag D, Wisoff J, Radmanesh A, Gindin T, et al. Radiologic response to MEK inhibition in a patient with a WNT-activated craniopharyngioma. Pediatr Blood Cancer. (2021) 68:e28753. doi: 10.1002/pbc.28753
63. Jiang Y, Yang J, Liang R, Zan X, Fan R, Shan B, et al. Single-cell RNA sequencing highlights intratumor heterogeneity and intercellular network featured in adamantinomatous craniopharyngioma. Sci Ad. (2023) 9:eadc8933. doi: 10.1126/sciadv.adc8933
64. Chen Y, Liu X, Ainiwan Y, Li M, Pan J, Chen Y, et al. Axl as a potential therapeutic target for adamantinomatous craniopharyngiomas: Based on single nucleus RNA-seq and spatial transcriptome profiling. Cancer Let. (2024) 592:216905. doi: 10.1016/j.canlet.2024.216905
65. Prince EW, Apps JR, Jeang J, Chee K, Medlin S, Jackson EM, et al. Unraveling the complexity of the senescence-associated secretory phenotype in adamantinomatous craniopharyngioma using multimodal machine learning analysis. Neuro Onco. (2024) 26:1109–23. doi: 10.1093/neuonc/noae015
66. Xu C, Wu J, Ye J, Si Y, Zhang J, Wu B, et al. Multiomics integration-based immunological characterizations of adamantinomatous craniopharyngioma in relation to keratinization. Cell Death Di. (2024) 15:439. doi: 10.1038/s41419-024-06840-1
67. Wang X, Lin J, Liu H, Zhao C, Tu Z, Xu D, et al. Single-cell and spatial sequencing identifies senescent and germinal tumor cells in adamantinomatous craniopharyngiomas. Cell Biosc. (2024) 14:112. doi: 10.1186/s13578-024-01299-1
68. Peng J, Yang L, Pan J, Wang C, Nie J, Liu Y, et al. Clinical features and prognosis of pediatric infradiaphragmatic craniopharyngioma relative to the tumor inflammatory response. Pediatr Re. (2021) 89:1119–25. doi: 10.1038/s41390-020-1013-4
69. Donson AM, Apps J, Griesinger AM, Amani V, Witt DA, Anderson RCE, et al. Molecular analyses reveal inflammatory mediators in the solid component and cyst fluid of human adamantinomatous craniopharyngioma. J Neuropathol Exp Neuro. (2017) 76:779–88. doi: 10.1093/jnen/nlx061
70. Qu X, Tang Y, Hua S. Immunological approaches towards cancer and inflammation: A cross talk. Front Immunol. (2018) 9:563. doi: 10.3389/fimmu.2018.00563
71. Zhou J, Zhang C, Pan J, Chen L, Qi ST. Interleukin-6 induces an epithelial-mesenchymal transition phenotype in human adamantinomatous craniopharyngioma cells and promotes tumor cell migration. Mol Med Re. (2017) 15:4123–31. doi: 10.3892/mmr.2017.6538
72. Fousek K, Horn LA, Palena C. Interleukin-8: A chemokine at the intersection of cancer plasticity, angiogenesis, and immune suppression. Pharmacol The. (2021) 219:107692. doi: 10.1016/j.pharmthera.2020.107692
73. Albulescu R, Codrici E, Popescu ID, Mihai S, Necula LG, Petrescu D, et al. Cytokine patterns in brain tumour progression. Mediators Inflam. (2013) 2013:979748. doi: 10.1155/2013/979748
74. Zhao H, Wu L, Yan G, Chen Y, Zhou M, Wu Y, et al. Inflammation and tumor progression: signaling pathways and targeted intervention. Signal Transduct Target The. (2021) 6:263. doi: 10.1038/s41392-021-00658-5
75. Vidal S, Kovacs K, Lloyd RV, Meyer FB, Scheithauer BW. Angiogenesis in patients with craniopharyngiomas: correlation with treatment and outcome. Cancer. (2002) 94:738–45. doi: 10.1002/cncr.10281
76. Vaquero J, Zurita M, de Oya S, Coca S, Morales C, Salas C. Expression of vascular permeability factor in craniopharyngioma. J Neurosurg. (1999) 91:831–4. doi: 10.3171/jns.1999.91.5.0831
77. Mazor R, Alsaigh T, Shaked H, Altshuler AE, Pocock ES, Kistler EB, et al. Matrix metalloproteinase-1-mediated up-regulation of vascular endothelial growth factor-2 in endothelial cells. J Biol Che. (2013) 288:598–607. doi: 10.1074/jbc.M112.417451
78. Yu CF, Chen FH, Lu MH, Hong JH, Chiang CS. Dual roles of tumour cells-derived matrix metalloproteinase 2 on brain tumour growth and invasion. Br J Cancer. (2017) 117:1828–36. doi: 10.1038/bjc.2017.362
79. Facundo AN, Magalhães M, Nascimento GC, Azulay RS, Santos RM, Freitas LA, et al. The expression of VDACs and Bcl2 family genes in pituitary adenomas: clinical correlations and postsurgical outcomes. Front Endocrinol (Lausanne). (2024) 15:1481050. doi: 10.3389/fendo.2024.1481050
80. Loeffler S, Fayard B, Weis J, Weissenberger J. Interleukin-6 induces transcriptional activation of vascular endothelial growth factor (VEGF) in astrocytes in vivo and regulates VEGF promoter activity in glioblastoma cells via direct interaction between STAT3 and Sp1. Int J Cancer J Int du cancer. (2005) 115:202–13. doi: 10.1002/ijc.20871
81. Chen M, Zheng SH, Yang M, Chen ZH, Li ST. The diagnostic value of preoperative inflammatory markers in craniopharyngioma: a multicenter cohort study. J Neurooncol. (2018) 138:113–22. doi: 10.1007/s11060-018-2776-x
82. Jia Y, Ma L, Cai K, Zhang B, Wu W, Xiao Y, et al. Immune infiltration in aggressive papillary craniopharyngioma: High infiltration but low action. Front Immuno. (2022) 13:995655. doi: 10.3389/fimmu.2022.995655
83. Yin X, Liu Z, Zhu P, Wang Y, Ren Q, Chen H, et al. CXCL12/CXCR4 promotes proliferation, migration, and invasion of adamantinomatous craniopharyngiomas via PI3K/AKT signal pathway. J Cell Bioche. (2019) 120:9724–36. doi: 10.1002/jcb.28253
84. Gong J, Zhang HL, Xing SS, Li CD, Ma ZY, Jia G, et al. High expression levels of CXCL12 and CXCR4 predict recurrence of adamantinomatous craniopharyngiomas in children. Cancer Biomarkers. (2014) 14:241–51. doi: 10.3233/CBM-140397
85. Keeley EC, Mehrad B, Strieter RM. Chemokines as mediators of tumor angiogenesis and neovascularization. Exp Cell Re. (2011) 317:685–90. doi: 10.1016/j.yexcr.2010.10.020
86. Bikfalvi A, Billottet C. The CC and CXC chemokines: major regulators of tumor progression and the tumor microenvironment. Am J Physiol Cell Physio. (2020) 318:C542–54. doi: 10.1152/ajpcell.00378.2019
87. Banday AH, Abdalla M. Immune checkpoint inhibitors: Recent clinical advances and future prospects. Curr Med Che. (2023) 30:3215–37. doi: 10.2174/0929867329666220819115849
88. Qiu J, Cheng Z, Jiang Z, Gan L, Zhang Z, Xie Z. Immunomodulatory precision: A narrative review exploring the critical role of immune checkpoint inhibitors in cancer treatment. Int J Mol Sc. (2024) 25:5490. doi: 10.3390/ijms25105490
89. Ribas A, Wolchok JD. Cancer immunotherapy using checkpoint blockade. Science. (2018) 359:1350–5. doi: 10.1126/science.aar4060
90. Garon EB, Rizvi NA, Hui R, Leighl N, Balmanoukian AS, Eder JP, et al. Pembrolizumab for the treatment of non–small-cell lung cancer. N Engl J Me. (2015) 372:2018–28. doi: 10.1056/nejmoa1501824
91. Robert C, Long GV, Brady B, Dutriaux C, Maio M, Mortier L, et al. Nivolumab in previously untreated melanoma without BRAF mutation. N Engl J Me. (2015) 372:320–30. doi: 10.1056/NEJMoa1412082
92. Goschzik T, Gessi M, Dreschmann V, Gebhardt U, Wang L, Yamaguchi S, et al. Genomic alterations of adamantinomatous and papillary craniopharyngioma. J Neuropathol Exp Neuro. (2017) 76:126–34. doi: 10.1093/jnen/nlw116
93. Martinez-Barbera JP, Andoniadou CL. Concise review: Paracrine role of stem cells in pituitary tumors: A focus on adamantinomatous craniopharyngioma. Stem Cell. (2016) 34:268–76. doi: 10.1002/stem.2267
94. Coy S, Rashid R, Lin JR, Du Z, Donson AM, Hankinson TC, et al. Multiplexed immunofluorescence reveals potential PD-1/PD-L1 pathway vulnerabilities in craniopharyngioma. Neuro Onco. (2018) 20:1101–12. doi: 10.1093/neuonc/noy035
95. Topalian SL, Hodi FS, Brahmer JR, Gettinger SN, Smith DC, McDermott DF, et al. Safety, activity, and immune correlates of anti-PD-1 antibody in cancer. N Engl J Me. (2012) 366:2443–54. doi: 10.1056/NEJMoa1200690
96. Joshi N, Mueller S, Kline C. Current clinical trials for craniopharyngiomas: what’s on the horizon? J Neuroonco. (2025) 172:281–8. doi: 10.1007/s11060-024-04899-6
Keywords: craniopharyngioma, adamantinomatous craniopharyngioma, papillary craniopharyngioma, target therapies, precision medicine
Citation: Pires de Oliveira Neto C, Nascimento GC, Damianse SdSP and Faria MdS (2025) Recent advances in craniopharyngioma pathophysiology and emerging therapeutic approaches. Front. Endocrinol. 16:1562942. doi: 10.3389/fendo.2025.1562942
Received: 18 January 2025; Accepted: 28 April 2025;
Published: 13 May 2025.
Edited by:
Andrea Glezer, University of São Paulo, BrazilReviewed by:
Simona Galoiu, Carol Davila University of Medicine and Pharmacy, RomaniaClarissa Silva Martins, Federal University of Mato Grosso do Sul, Brazil
Copyright © 2025 Pires de Oliveira Neto, Nascimento, Damianse and Faria. This is an open-access article distributed under the terms of the Creative Commons Attribution License (CC BY). The use, distribution or reproduction in other forums is permitted, provided the original author(s) and the copyright owner(s) are credited and that the original publication in this journal is cited, in accordance with accepted academic practice. No use, distribution or reproduction is permitted which does not comply with these terms.
*Correspondence: Manuel dos Santos Faria, bWZhcmlhMTk0OUBnbWFpbC5jb20=