- 1School of Medicine, Royal College of Surgeons in Ireland - Medical University of Bahrain, Busaiteen, Bahrain
- 2Research Department, Royal College of Surgeons in Ireland - Medical University of Bahrain, Busaiteen, Bahrain
Iodine is a critical trace element in the human body. It is primarily obtained through dietary sources such as dairy products, seafood, fish, eggs and certain vegetables. Iodine plays an essential role in various bodily functions, most notably in producing the thyroid hormones, triiodothyronine and thyroxine. Additionally, it influences the immune, cardiovascular, reproductive and gastrointestinal systems. Historically, iodine deficiency has been a significant global health issue; however, over the past decade, there has been a rise in iodine excess. This surge has been primarily attributed to inadequate monitoring and over-iodization of salt. Despite the well-documented consequences of iodine deficiency, the ramifications of excessive iodine intake remain underexplored. In view of rising global infertility rates, excess iodine has been linked to significant reproductive health effects. These include decreased sperm count, motility and morphology in males, as well as adverse pregnancy outcomes in females, such as maternal thyroid dysfunction and congenital hypothyroidism. This mini-review aims to collate and analyze current literature pertaining to the effects of iodine excess on reproductive health and shed light on its increasing incidence worldwide. Further research on the biological and clinical effects of iodine excess is required to derive a better understanding of this issue. Given the rising prevalence of iodine excess, it is crucial to raise awareness and implement proactive measures to prevent it from escalating into a major public health crisis in the future.
Introduction
What is iodine
Iodine is a trace element in the human body, found at levels of 15-20 mg in a healthy adult, with the majority (70-80%) contained within the thyroid gland; it is acquired solely through dietary sources (1–7). Iodine is naturally present in foods such as dairy, seaweed, fish, eggs, broccoli, spinach and peas. Iodine plays several roles in the human body, primarily in the synthesis of thyroid hormones such as triiodothyronine (T3) and thyroxine (T4) (1). This element is implicated in thyroid function and exerts profound effects on immune system modulation, the cardiovascular and reproductive systems, and gastrointestinal disorders, among others (1, 8, 9).
Daily recommended intake
The daily recommended intake for iodine according to the World Health Organization (WHO) is different for schoolchildren, at 120 µg, versus adults at 150 µg, with increased levels recommended for pregnant/lactating women at 250 µg (10–12). Historically, iodine deficiency has been a major concern, prompting countries such as the United States and Switzerland to introduce iodized table salt in the 1920s, which helped improve iodine levels (1, 13, 14). By the 1990s, these salt iodization programs were adopted globally, leading to a decrease in the prevalence of iodine deficiency worldwide (1, 13, 14). As of 2019, global iodine deficiency rates were at 2.4% (14).
Search strategy
A literature search was conducted between October 2024 to January 2025 utilizing Google Scholar and PubMed databases. The search employed keywords, including “iodine excess”, “iodine toxicity”, and “iodine overload”, among others. Only articles written in the English language were considered. Articles were included based on relevancy.
From deficiency to excess
Within the past decade, the number of countries with iodine deficiency has reduced from 54 to 30, while those with adequate levels have increased from 67 to 112 (15). Meanwhile, the number of countries with excessive iodine intake (defined as a median urinary iodine level over 300 µg/L) has doubled from 5 to 10: Brazil, Colombia, Somalia, Uganda, Armenia, Benin, Georgia, Honduras, Paraguay, Uruguay (15). Poor monitoring and over-iodization of salt have been the primary factors contributing to excessive iodine levels in these countries (15). The American Thyroid Association (ATA) sets the upper tolerable limit of iodine at 1100 µg and advises against daily intake exceeding 500 µg for children, adults and pregnant or lactating individuals (16). Meanwhile, the Joint Food and Agriculture Organization (FAO)/World Health Organization (WHO) Expert Committee on Food Additives (JECFA) has proposed a maximum upper limit of 1 mg/day of iodine for the general population (10–12). Other organizations such as the former Scientific Committee on Food (SCF) of the European Commission and the UK Expert Group on Vitamins & Minerals (EVM) propose upper tolerable limit values for adults at 600 µg/day and 500 µg/day respectively (17, 18).
It is important to recognize that individuals can differ significantly in terms of their tolerance to iodine intake due to a multitude of factors. Diet plays an important role, as varying amounts and sources of iodine with differing eating patterns contribute to inter-individual variability (19). Furthermore, those with pre-existing thyroid disease may be more susceptible to excessive iodine intake (10). Genetic mutation can additionally impact the normal physiological processes of the thyroid to iodine (20). Consequently, these factors should be considered when interpreting studies.
Although the impact of iodine deficiency is well-studied, iodine excess has received much less attention regarding its effects on human health. The literature contains a paucity of articles assessing the impacts of excess iodine especially in terms of reproductive health; however, some studies have shown excess iodine affects reproductive health and pregnancy by impairing spermatogenesis, hindering infant neurodevelopment, increasing the risk of infertility and contributing to gestational diabetes mellitus (GDM) and hypertensive disorders during pregnancy (HDP) (21–24). In this mini-review, we aim to highlight the impact excess iodine has on the reproductive health of males and females.
The body’s reaction to excessive iodine exposure
Historically, one of the earliest mentions of the reaction of the thyroid gland to acute excessive iodine concentrations was described by Dr. Jan Wolff and Dr. Israel Chaikoff, an observation now known as the Wolff-Chaikoff effect (25, 26). By reducing the synthesis of T3 and T4, this effect acts to block the excessive amounts of iodide in the body from generating large amounts of thyroid hormone (27–29). One proposed mechanism of the Wolff-Chaikoff effect is the production of inhibitory substances that impact thyroid peroxidase, namely intrathyroidal iodoaldehydes, iodolactones or iodolipids (25, 30).
However, the Wolff-Chaikoff effect is transient, usually lasting only one to two days, after which an escape occurs where regular synthesis of thyroid hormones resumes (25, 27, 31). Reduced expression of the sodium-iodide symporter has been theorized to diminish iodide uptake and the subsequent generation of thyroid hormones, thereby maintaining a euthyroid state (25, 27, 31).
Notably, excess amounts of daily iodide ranging from 30 mg to 2 g are typically well tolerated in individuals with normal thyroid glands. Although laboratory alterations are seen, such as a reduction in serum T4 by 25%, T3 by 15% and a 2 mU/L rise in TSH, these values remain within the normal ranges without clinical signs of thyroid dysfunction (32).
A failure of the Wolff-Chaikoff effect can give rise to the Jod-Basedow phenomenon, typically seen in patients with impaired thyroid regulation, including those with thyroid nodules. Rather than becoming hypothyroid, these patients may generate an excessive amount of thyroid hormone (25, 28, 31, 33, 34).
Although the effect of iodine on thyroid pathophysiology is well described, the role that excess iodine has independent of thyroid hormone in regard to infertility has not been well documented (35). Infertility, defined as a couple’s inability to conceive after one year of regular unprotected intercourse, is a significant health issue impacting 50-80 million individuals worldwide (36–38). Infertility can be subcategorized into primary and secondary infertility, the former being when a woman has never conceived, and the latter being when a woman has had at least one successful previous conception but now has inability to conceive according to the WHO (39–41). Categories of infertility include ovulatory dysfunction, tubal occlusion, diminished ovarian reserve, endometriosis, uterine and male factors (42). Furthermore, lifestyle and environmental factors such as sexual violence, sexually transmitted diseases, stress and nutritional insufficiency among many others have also been associated with reduced fertility (43). An identifiable cause can be found in 85% of infertile couples, whereas the remaining 15% are classified as unexplained infertility (42, 44). Furthermore, infertility rates in men have been rising over the past decades, with a notable 76.9% increase since 1990 (45). Additionally, 20-30% of infertility cases are due to males, while male factors contribute to 50-70% of infertile couples (46). Therefore, it is imperative to highlight the possible role that excess iodine may have on exacerbating this global health crisis (47).
Iodine excess and its impact on male reproductive health
Recent studies have shown that excessive iodine levels correlate with several adverse effects on male reproductive health, particularly with regard to semen quality. A study investigating the association between semen quality and iodine intake in 1,098 fertile Chinese men found that participants with iodine excess, defined as a urinary iodine concentration (UIC) of ≥ 200 µg/L, exhibited a 5% higher semen volume, 26% lower semen concentration, 20% fewer semen counts, and a 1.48 month longer time to pregnancy in comparison to those with an optimal iodine intake (100 ≤ UIC < 200µg/L) (48). Although these findings were statistically significant, there was no significant effect on sperm motility (48). A strength of this paper is its relatively large multi-province sample of 1,098 couples, enhancing external validity; however, a limitation is its cross-sectional design which does not allow definitive establishment of causality. Furthermore, its sample inclusion of only fertile men, excluding infertile men, may underestimate the true effect of iodine on semen quality.
Another study conducted in Spain analyzing 96 couples with a median UIC of 97.6 µg/L undergoing infertility evaluation found a higher rate of altered spermatozoa morphology and a lower motile sperm count in those with higher semen iodine levels and urinary iodine levels respectively (35). Moreover, males who had not been able to achieve pregnancy with their partner for more than three years had a higher urinary iodine level than those trying for fewer than three years (35). In contrast to the previously mentioned study, this paper observed no association in certain semen characteristics such as volume, total sperm count and percentage of spermatozoa with progressive motility, after adjusting for age, smoking and BMI (35). Interestingly, the urinary iodine level was negatively correlated with the total motile sperm count (35). Moreover, males with abnormal sperm morphology possessed a higher median semen iodine level (35). Additionally, those who reported consuming iodized salt had significantly higher median semen iodine levels (16.5 µg/L) compared to those who consumed non-iodized salt (11.7 µg/L). A strength of this study is that it was the first study to measure semen iodine concentration in association with semen quality; a limitation is the small sample size. Although a causal relationship could not be established within the study due to cross-sectional design, the findings highlight the need for additional clinical research to investigate whether excess dietary iodine impacts spermatozoal morphology and other parameters, which could affect fertility.
The hypothalamic-pituitary-thyroid axis is key in reproductive health, especially in the context of iodine excess. Since iodine excess in certain susceptible individuals can lead to thyroid dysfunction (hypothyroidism or hyperthyroidism) (10). Hypothyroidism in humans can lead to reductions in total serum testosterone, luteinizing hormone (LH), follicle stimulating hormone, sex-hormone binding globulin and alterations in sperm quality (49–52). Similarly, adverse effects on fertility are seen in hyperthyroidism, such as alterations in sex steroid levels and spermatozoal DNA damage and motility abnormalities (53–55). Iodine excess’ effect on fertility is theorized to be a consequence of oxidative stress on the testis (53).
A randomized control trial studying the effects of iodine excess in adult male rats evaluated parameters including testicular morphology, steroidogenic enzyme activity, and sperm count, viability and morphology (56). They found that male rats administered 100 times and 500 times the recommended iodine level saw dose- and duration-dependent impairment of reproductive function compared with controls (56).
Interestingly, the role of iodine in male reproductive health is substantiated by the immunohistochemical confirmation of the sodium-iodide symporter and pendrin (57, 58). One proposed mechanism is reactive oxygen species (ROS) generation following excessive iodine accumulation within the testes, adjudged by an alteration in levels of pro-oxidant and antioxidants (catalase, superoxide dismutase, glutathione peroxidase) as well as increased levels of lipid peroxidation (56). Under normal physiological states, ROS is generated as part of the steriodogenesis process, however, as a result of iodine-induced alteration in antioxidant levels, this leads to the inability of the testes to counteract the harmful effects caused by ROS (56, 59) causing cellular damage to testicular germ cells (56, 60). Furthermore, this leads to a decrease in testosterone synthesis via inhibition of the enzymes Δ5 3β-hydroxysteroid dehydrogenase (HSD) and 17 β-HSD (56). Since testosterone is an important regulator of spermatogenesis, these processes eventually result in functional and structural alterations in the testes (56, 61). Furthermore, prolonged ROS generation disrupts the hypothalamic-pituitary-adrenal axis by upregulating the adrenocortical stress signaling pathway thereby increasing corticosterone. This effect leads to a downstream inhibition of LH release further decreasing testosterone synthesis (56). Moreover, a protracted state of iodine excess can directly precipitate a hypothyroid state, eventually decreasing testosterone levels (56). While this study provides mechanistic insights, it was performed in rats, limiting translational relevance to humans.
Another study by Chakraborty et al. used rat models to evaluate the role of excess iodine in spermatogenesis. Similar to the previous findings, these authors observed a significant reduction in both sperm motility and sperm count (21). Furthermore, molecular investigations revealed that oxidative stress, increased apoptosis, reduced expression of blood-testis barrier (BTB) proteins, decreased regulators of spermatogenesis, and reduced expression of markers related to germ cell proliferation and differentiation all played a role in causing impaired spermatogenesis in the study (21). Ultimately, the mechanisms the authors propose that lead to spermatogenesis impairment is an interplay between cystoskeleton and BTB disruption as well as oxidative stress (21). Another paper confirmed that excess iodine, by inducing ROS generation, led to spermatozoal cell apoptosis thereby affecting male fertility (62).
Although these findings are based on rat models, they provide valuable insight into how iodine may impact human reproductive health (Figure 1). Hence, it is crucial to develop effective strategies to mitigate iodine-induced male infertility to combat the rise in infertility rates over the past decades.
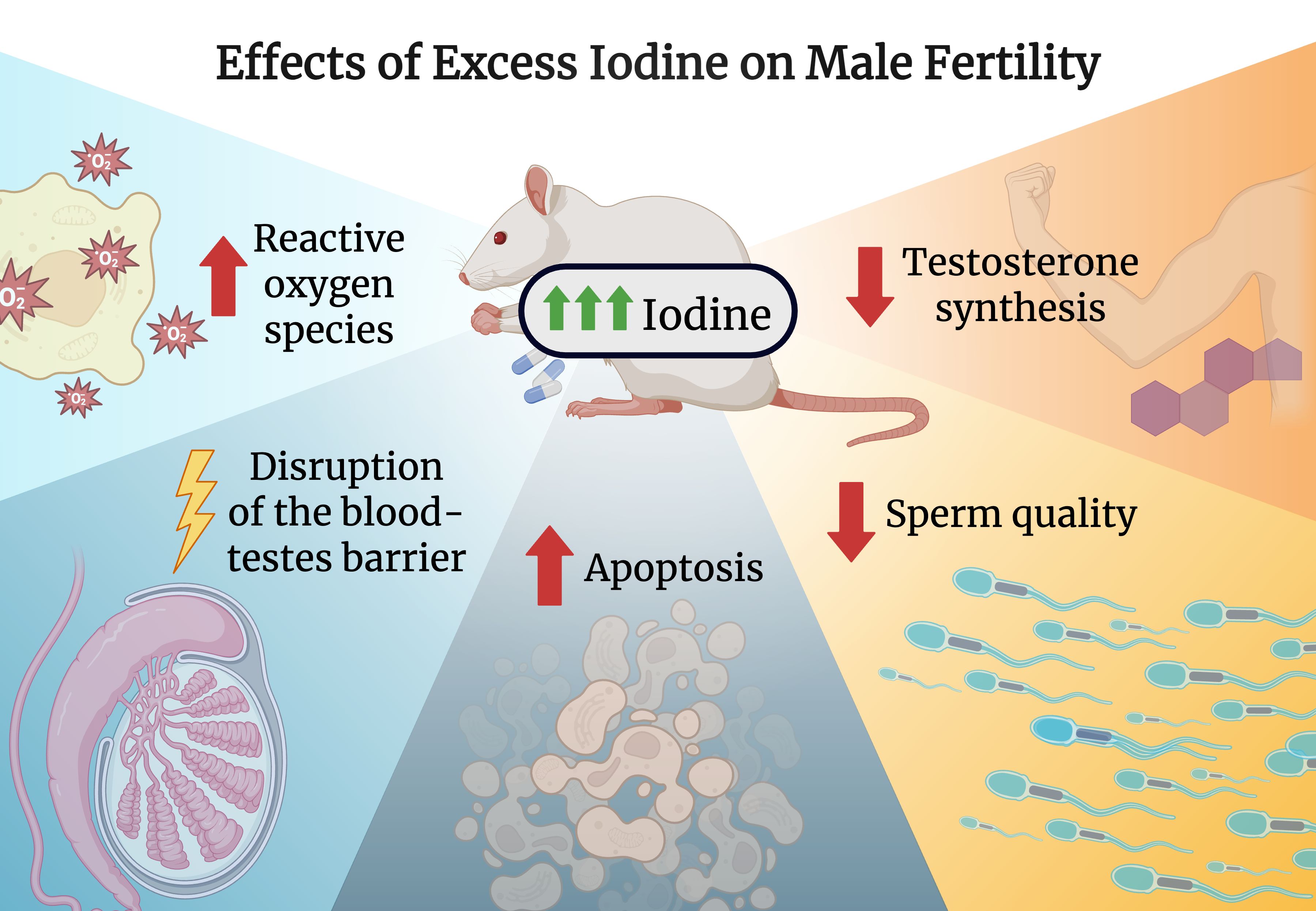
Figure 1. Effects of excess iodine on male fertility. Created with biorender.com.
Iodine excess and its impact on female reproductive health
Iodine excess and female fertility
Unexplained infertility (UI) is the diagnosis given when a couple has been actively trying to conceive for more than a year unsuccessfully, and medical testing cannot identify a cause for infertility (44). For women who have low fertility, hysterosalpingography (HSG) is historically the most common first-line diagnostic modality to assess the uterine cavity and tubal patency (63). However, a study using an oil-soluble contrast medium (OSCM) in conjunction with HSG caused iodine excess, leading to the development of subclinical hypothyroidism in approximately 40% of women, and later-onset hyperthyroidism in 5% of women (64). Additionally, women who conceive successfully after HSG surgery may be at risk of excess iodine, as previous studies showed that women who underwent HSG before pregnancy remained in a prolonged state of high iodine throughout the gestational and postpartum periods (65). Moreover, another study confirmed persistently high levels of iodine after OSCM HSG, and iodine excess did not correlate to improved fertility. However, it was noted that 30% of their population was iodine deficient, and the OSCM HSG corrected that deficiency, which aided in improving fertility for those patients (66). Thus, it is essential to recognize the impact of iodine excess on differing populations, as baseline iodine levels have the potential to influence outcomes of OSCM HSG.
Research on the impact of excess iodine on female reproductive physiology is limited. However, a study by Mahapatra et al. provides some insights. Rats were separated into three groups were given varying levels of iodine in the form of potassium iodide for an extended period of time, and demonstrated that extended exposure to iodine in excess exerted a biphasic mode of action, causing either a hypofunctioning or a hyperfunctioning ovary, with a fertility index of zero at both doses (67). Furthermore, a study evaluating ovarian and uterine histological changes following prolonged iodine excess demonstrated an alteration in the structure and number of ovarian follicles and corpus luteums (68). In addition, uterine changes were also observed which further contributed to the negative alteration of female rat reproductive function (68). Despite current findings, further research is essential to gain a comprehensive understanding of the effects of excess iodine on ovarian structure and function.
Iodine excess and pregnancy
The physiological demands of pregnancy necessitate an approximately 50% increase in iodine requirements, rendering this population vulnerable to iodine level imbalances (69). A median UIC below 100 μg/L for nonpregnant women and children defines an iodine-deficient population (70). For pregnant women, the WHO defines UIC levels of 150–249 μg/L as adequate iodine status, UIC values below 150 μg/L indicating iodine deficiency, levels between 250 and 500 μg/L being classified as more than adequate, and values exceeding 500 μg/L considered to be iodine excess (70). Iodine deficiency may be exacerbated by avoiding iodine-rich foods, stemming from concerns about heavy metal contamination in these foods, and managing nausea and vomiting (71), so iodine supplementation has become popular. However, pregnant women are at risk of iodine excess due to the intake of water, food, nutritional supplements or additional medications (10). A new study challenges the current WHO recommendation by suggesting a lower limit for iodine intake during pregnancy (72).
Excessive iodine during pregnancy can increase serum thyroid-stimulating hormone (TSH) concentrations, negatively impacting maternal thyroid function and potentially leading to further health risks, as seen in Figure 2. Findings from an observational study indicate that pregnant women consuming high-dose iodine supplements (>200 μg/day) may have a higher risk of serum TSH elevation compared to with women whose supplemental iodine intake was lower at <100 μg per day (73). Elevated maternal serum iodide can potentially lead to reduced fetal thyroid hormones, fetal skeletal development, placentation and preterm delivery (74). The ingestion of excess maternal iodine tablets during pregnancy has led to several cases of congenital hypothyroidism being reported (75).
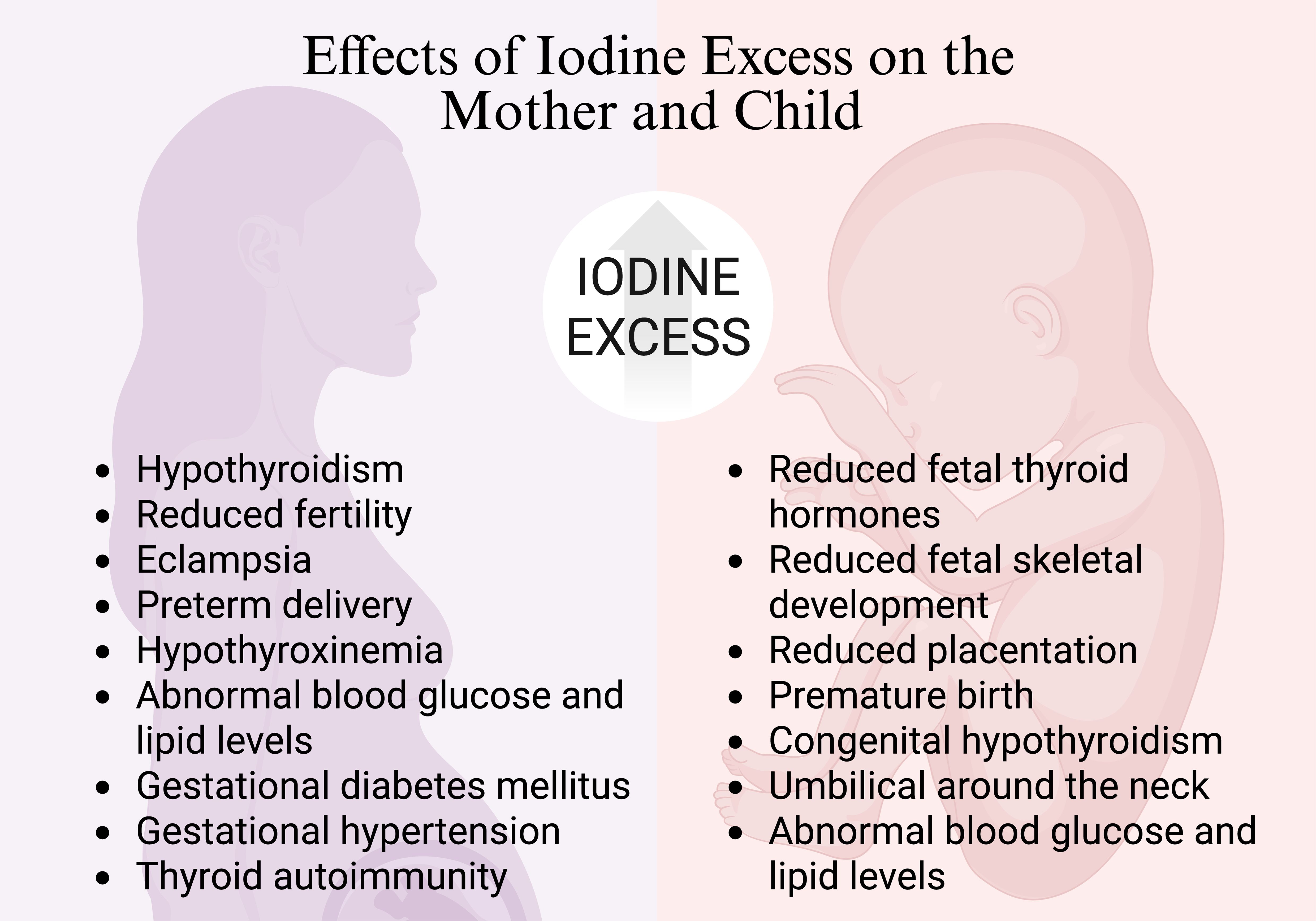
Figure 2. Effects of iodine excess on mother and child. Created with biorender.com.
Beyond these hormonal effects, excessive iodine intake has also been linked to thyroid autoimmunity, which has been associated with increased infertility rates, polycystic ovary syndrome, premature ovarian insufficiency and endometriosis (76, 77). Excess iodine may trigger immune dysregulation, contributing to autoimmune thyroid diseases such as Hashimoto’s thyroiditis and Graves’ disease, both of which have been associated with excess long-term morbidities in children and adolescence (78). Since immune balance is crucial for implantation, ovarian function and maternal-fetal interactions, these findings suggest that iodine-related immune disturbances could contribute to reproductive challenges (79–81). While direct studies on iodine excess and reproductive immunology remain limited, further research is needed to clarify these potential mechanisms.
In addition to supplements, excess iodine may also be due to diet. For mothers who consumed excessive amounts of seaweed during both pregnancy and lactation, hypothyroidism was diagnosed in their offspring (82, 83). A Finnish study revealed a positive association between elevated serum iodide levels and risk of preterm birth (84). Furthermore, iodine excess during pregnancy was found to be linked to a significant increase in the occurrence of adverse mother and fetus outcomes, including eclampsia, umbilical cord wrapping around the neck, abnormal blood glucose and lipid levels (85).
Moreover, a study involving 214 pregnant women determined that those with UIC between 250-499 µg/L exhibited higher rates of GDM (20.3%) compared to the group with UIC 150-249 µg/L (9.7%) (24). Additionally, HDP, encompassing pre-eclampsia and gestational hypertension, occurred with a prevalence of 33.3% in those with UIC ≥ 250 µg/L compared to 4.3% in those with 150-249 µg/L (24). Neonatal growth parameters, such as head circumference, femur length, and estimated fetal weight were inversely associated with a state of iodine excess (86). Additionally, a cross-sectional study of 7,190 pregnant women in China found that both deficient and excessive iodine intake were associated with an increased risk of maternal hypothyroxinemia, hypothyroidism prevalence and thyroid autoimmunity (87). Despite its clinical significance, there are limited studies concentrating specifically on iodine excess during pregnancy, and maternal and neonatal outcomes. However, with the current research, the potential risks associated with even mild iodine excess during pregnancy warrant careful consideration.
Endocrine-disrupting chemicals and thyroid function
According to the Endocrine Society, Endocrine-disrupting chemicals (EDCs) are defined as a mixture of chemicals or an exogenous chemical that can interfere with any aspect of hormone action (88). They include a wide range of compounds such as fungicides, plasticizers, pesticides and industrial chemicals, among others (89). Certain EDCs, namely thiocyanate and perchlorate, affect thyroid metabolism by inhibiting the sodium-iodide symporter (90, 91). Additionally, compounds such as flame retardants may disrupt thyroid physiology.
A recent 2024 review examining flame retardants and thyroid function revealed conflicting evidence, with some studies finding a positive association with TSH while others noted a negative one (92). Similar relationships were found between flame retardants and total T3 and total T4 (92). The authors emphasize the need for long-term studies to more conclusively determine the effects flame retardants have on thyroid function (92).
As EDCs affect thyroid physiology, they can potentially impact iodine levels, making them a factor to consider in infertility. As a result, further research into the long-term effects of EDCs on thyroid function, iodine levels and reproductive health is crucial for mitigating possible future fertility challenges worldwide.
Conclusion
Excess iodine intake is an emerging concern with potential implications for reproductive health. While iodine is essential for thyroid function and overall well-being, an imbalance, whether deficiency or excess, can lead to significant health consequences. This review highlights evidence suggesting that excessive iodine exposure may impair male and female reproductive health by altering semen quality, disrupting hormonal balance, and contributing to conditions such as infertility, GDM, and HDP. Given the rising number of countries experiencing excessive iodine intake due to over-iodization and poor monitoring, it is crucial to implement strategies that ensure a balanced intake. Future research should further investigate the underlying mechanisms and long-term reproductive outcomes associated with iodine excess to refine public health recommendations. By addressing these gaps, we can develop more precise dietary guidelines and policies to safeguard reproductive health while maintaining optimal iodine nutrition.
Author contributions
AiK: Conceptualization, Formal analysis, Visualization, Writing – original draft, Writing – review & editing. AhK: Conceptualization, Formal analysis, Methodology, Visualization, Writing – original draft, Writing – review & editing. SN: Conceptualization, Formal analysis, Investigation, Visualization, Writing – original draft, Writing – review & editing. HH: Conceptualization, Formal analysis, Methodology, Visualization, Writing – original draft, Writing – review & editing. AB: Conceptualization, Project administration, Supervision, Writing – review & editing.
Funding
The author(s) declare that no financial support was received for the research and/or publication of this article.
Conflict of interest
The authors declare that the research was conducted in the absence of any commercial or financial relationships that could be construed as a potential conflict of interest.
Generative AI statement
The author(s) declare that no Generative AI was used in the creation of this manuscript.
Publisher’s note
All claims expressed in this article are solely those of the authors and do not necessarily represent those of their affiliated organizations, or those of the publisher, the editors and the reviewers. Any product that may be evaluated in this article, or claim that may be made by its manufacturer, is not guaranteed or endorsed by the publisher.
References
1. Hatch-McChesney A, Lieberman HR. Iodine and iodine deficiency: A comprehensive review of a re-emerging issue. Nutrients. (2022) 14:3474. doi: 10.3390/nu14173474
2. Ahad F, Ganie SA. Iodine, Iodine metabolism and Iodine deficiency disorders revisited. Indian J Endocrinol Metab. (2010) 14:13–7.
3. Pehrsson PR, Roseland JM, Patterson KY, Phillips KM, Spungen JH, Andrews KW, et al. Iodine in foods and dietary supplements: A collaborative database developed by NIH, FDA and USDA. J Food Compos Anal. (2022) 109:104369. doi: 10.1016/j.jfca.2021.104369
4. Lee K, Shin D, Cho M, Song W. Food group intakes as determinants of iodine status among US adult population. Nutrients. (2016) 8:325. doi: 10.3390/nu8060325
5. Krela-Kaźmierczak I, Czarnywojtek A, Skoracka K, Rychter AM, Ratajczak AE, Szymczak-Tomczak A, et al. Is there an ideal diet to protect against iodine deficiency? Nutrients. (2021) 13:513. doi: 10.3390/nu13020513
6. Carlsen MH, Andersen LF, Dahl L, Norberg N, Hjartåker A. New iodine food composition database and updated calculations of iodine intake among Norwegians. Nutrients. (2018) 10:930. doi: 10.3390/nu10070930
7. Zimmermann MB. Present Knowledge in Nutrition Vol. 1. Amsterdam, The Netherlands: Elsevier (2020).
8. Aceves C, Mendieta I, Anguiano B, Delgado-González E. Molecular iodine has extrathyroidal effects as an antioxidant, differentiator, and immunomodulator. Int J Mol Sci. (2021) 22:1228. doi: 10.3390/ijms22031228
9. Bilal MY, Dambaeva S, Kwak-Kim J, Gilman-Sachs A, Beaman KD. A role for iodide and thyroglobulin in modulating the function of human immune cells. Front Immunol. (2017) 8:1573. doi: 10.3389/fimmu.2017.01573
10. Farebrother J, Zimmermann MB, Andersson M. Excess iodine intake: sources, assessment, and effects on thyroid function. Ann N Y Acad Sci. (2019) 1446:44–65. doi: 10.1111/nyas.2019.1446.issue-1
11. Joint FAO/WHO Expert Committee on Food Additives, International Program on Chemical Safety. Toxicological evaluation of certain food additives and contaminants. In: WHO Food additives series. Cambridge University Press, Cambridge; New York (1989). 362 p.
12. World Health Organization, Food and Agriculture Organization of the United Nations. Vitamin and mineral requirements in human nutrition. 2nd ed. Geneva: Rome: World Health Organization; FAO (2004). 341 p.
13. Leung AM, Braverman LE, Pearce EN. History of U.S. Iodine fortification and supplementation. Nutrients. (2012) 4:1740–6. doi: 10.3390/nu4111740
14. GBD 2019 Diseases and Injuries Collaborators. Iodine deficiency—Level 3 cause. Lancet. (2019). 396. Available online at: https://www.thelancet.com/pb-assets/Lancet/gbd/summaries/diseases/iodine-deficiency.pdf (Accessed November 11, 2024).
15. Zimmermann MB. Iodine deficiency and excess in children: worldwide status in 2013. Endocr Pract. (2013) 19:839–46. doi: 10.4158/EP13180.RA
16. Leung AM, Avram AM, Brenner AV, Duntas LH, Ehrenkranz J, Hennessey JV, et al. Potential risks of excess iodine ingestion and exposure: statement by the American thyroid association public health committee. Thyroid. (2015) 25:145–6. doi: 10.1089/thy.2014.0331
17. Scientific Committee on Food. Opinion of the scientific committee on food on the tolerable upper intake level of iodine(2002). Available online at: https://ec.europa.eu/food/fs/sc/scf/out146_en.pdf:~:text=iodide%2Fday,the%20ULs%20for%20children%20were (Accessed March 8, 2025).
18. Expert Group on Vitamins and Minerals. Safe upper levels for vitamins and minerals. Food Standards Agency. (2003) 203–212. https://cot.food.gov.uk/sites/default/files/cot/vitmin2003.pdf (Accessed March 8, 2025).
19. Fuse Y, Tsukada N, Urakawa Y, Yokoyama J, Matsuzaki M, Shishiba Y, et al. Studies on urinary excretion and variability of dietary iodine in healthy Japanese adults. Endocr J. (2022) 69:427–40. doi: 10.1507/endocrj.EJ21-0486
20. Kwak MJ. Clinical genetics of defects in thyroid hormone synthesis. Ann Pediatr Endocrinol Metab. (2018) 23:169–75. doi: 10.6065/apem.2018.23.4.169
21. Chakraborty A, Singh V, Singh K, Rajender S. Excess iodine impairs spermatogenesis by inducing oxidative stress and perturbing the blood testis barrier. Reprod Toxicol. (2020) :96:128–40. doi: 10.1016/j.reprotox.2020.06.012
22. Wu W, Chen Y, Guo W, Zhang K, Chen W, Fu M, et al. The relationship between iodine excess and thyroid function during pregnancy and infantile neurodevelopment at 18–24 months. J Nutr. (2023) 153:2320–7. doi: 10.1016/j.tjnut.2023.05.012
23. Mathews DM, Johnson NP, Sim RG, O’Sullivan S, Peart JM, Hofman PL. Iodine and fertility: do we know enough? Hum Reprod. (2021) 36:265–74. doi: 10.1093/humrep/deaa312
24. Silva De Morais N, Ayres Saraiva D, Corcino C, Berbara T, Schtscherbyna A, Moreira K, et al. Consequences of iodine deficiency and excess in pregnancy and neonatal outcomes: A prospective cohort study in Rio de Janeiro, Brazil. Thyroid. (2020) 30:1792–801. doi: 10.1089/thy.2019.0462
25. Sohn SY, Inoue K, Rhee CM, Leung AM. Risks of iodine excess. Endocr Rev. (2024) 45:bnae019. doi: 10.1210/endrev/bnae019
26. Wolff J, Chaikoff IL. Plasma inorganic iodide as a homeostatic regulator of thyroid function. J Biol Chem. (1948) 174:555–64. doi: 10.1016/S0021-9258(18)57335-X
27. Markou K, Georgopoulos N, Kyriazopoulou V, Vagenakis AG. Iodine-induced hypothyroidism. Thyroid. (2001) 11:501–10. doi: 10.1089/105072501300176462
28. Rodriguez-Diaz E, Pearce EN. Iodine status and supplementation before, during, and after pregnancy. Best Pract Res Clin Endocrinol Metab. (2020) 34:101430. doi: 10.1016/j.beem.2020.101430
29. Salvatore D, Davies TF, Schlumberger MJ, Hay ID, Larsen PR. Thyroid physiology and diagnostic evaluation of patients with thyroid disorders. In: Williams Textbook of Endocrinology. Amsterdam, Netherlands: Elsevier (2016). p. 333–68. Available at: https://linkinghub.elsevier.com/retrieve/pii/B9780323297387000113 (Accessed December 16, 2024).
30. Raben MS. The paradoxical effects of thiocyanate and of thyrotropin on the organic binding of iodine by the thyroid in the presence of large amounts of iodide. Endocrinology. (1949) 45:296–304. doi: 10.1210/endo-45-3-296
31. Leung AM, Braverman LE. Consequences of excess iodine. Nat Rev Endocrinol. (2014) 10:136–42. doi: 10.1038/nrendo.2013.251
32. Bürgi H. Iodine excess. Best Pract Res Clin Endocrinol Metab. (2010) 24:107–15. doi: 10.1016/j.beem.2009.08.010
33. Rose HR, Zulfiqar H, Anastasopoulou C. Jod-basedow syndrome. In: StatPearls. StatPearls Publishing, Treasure Island (FL (2024). Available at: http://www.ncbi.nlm.nih.gov/books/NBK544277/.
34. Inoue K, Guo R, Lee ML, Ebrahimi R, Neverova NV, Currier JW, et al. Iodine-induced hyperthyroidism and long-term risks of incident atrial fibrillation and flutter. J Clin Endocrinol Metab. (2023) 108:e956–62. doi: 10.1210/clinem/dgad250
35. Partal-Lorente AB, Maldonado-Ezequiel V, Martinez-Navarro L, Herrera-Contreras I, Gutierrez-Repiso C, García-Fuentes E, et al. Iodine is associated to semen quality in men who undergo consultations for infertility. Reprod Toxicol. (2017) 73:1–7. doi: 10.1016/j.reprotox.2017.07.020
36. Babakhanzadeh E, Nazari M, Ghasemifar S, Khodadadian A. Some of the factors involved in male infertility: A prospective review. Int J Gen Med. (2020) 13:29–41. doi: 10.2147/IJGM.S241099
37. Briceag I, Costache A, Purcarea VL, Cergan R, Dumitru M, Briceag I, et al. Fallopian tubes–literature review of anatomy and etiology in female infertility. J Med Life. (2015) 8:129–31.
38. Kumar N, Singh AK. Trends of male factor infertility, an important cause of infertility: A review of literature. J Hum Reprod Sci. (2015) 8:191–6. doi: 10.4103/0974-1208.170370
39. Benksim A, Elkhoudri N, Addi RA, Baali A, Cherkaoui M. Difference between primary and secondary infertility in Morocco: frequencies and associated factors. Int J Fertil Steril. (2018) 12:142–6. doi: 10.22074/ijfs.2018.5188
40. James SL, Abate D, Abate KH, Abay SM, Abbafati C, Abbasi N, et al. Global, regional, and national incidence, prevalence, and years lived with disability for 354 diseases and injuries for 195 countries and territories, 1990–2017: a systematic analysis for the Global Burden of Disease Study 2017. Lancet. (2018) 392:1789–858. doi: 10.1016/S0140-6736(18)32279-7
41. Tabong PTN, Adongo PB. Infertility and childlessness: a qualitative study of the experiences of infertile couples in Northern Ghana. BMC Pregnancy Childbirth. (2013) 13:72. doi: 10.1186/1471-2393-13-72
42. Carson SA, Kallen AN. Diagnosis and management of infertility: A review. JAMA. (2021) 326:65–76. doi: 10.1001/jama.2021.4788
43. Bala R, Singh V, Rajender S, Singh K. Environment, lifestyle, and female infertility. Reprod Sci. (2021) 28:617–38. doi: 10.1007/s43032-020-00279-3
44. Gelbaya TA, Potdar N, Jeve YB, Nardo LG. Definition and epidemiology of unexplained infertility. Obstet Gynecol Surv. (2014) 69:109–15. doi: 10.1097/OGX.0000000000000043
45. Huang B, Wang Z, Kong Y, Jin M, Ma L. Global, regional and national burden of male infertility in 204 countries and territories between 1990 and 2019: an analysis of global burden of disease study. BMC Public Health. (2023) 23:2195. doi: 10.1186/s12889-023-16793-3
46. Stavros S, Potiris A, Molopodi E, Mavrogianni D, Zikopoulos A, Louis K, et al. Sperm DNA fragmentation: unraveling its imperative impact on male infertility based on recent evidence. Int J Mol Sci. (2024) 25:10167. doi: 10.3390/ijms251810167
47. De Jonge C, Barratt CLR. The present crisis in male reproductive health: an urgent need for a political, social, and research roadmap. Andrology. (2019) 7:762–8. doi: 10.1111/andr.12673
48. Sun Y, Chen C, Liu GG, Wang M, Shi C, Yu G, et al. The association between iodine intake and semen quality among fertile men in China. BMC Public Health. (2020) 20:461. doi: 10.1186/s12889-020-08547-2
49. Krajewska-Kulak E, Sengupta P. Thyroid function in male infertility. Front Endocrinol. (2013) 4:174. doi: 10.3389/fendo.2013.00174
50. Krassas GE, Perros P. Thyroid disease and male reproductive function. J Endocrinol Invest. (2003) 26:372–80. doi: 10.1007/BF03345187
51. Hernández JJC, García JMM, García Diez LC. Primary hypothyroidism and human spermatogenesis. Arch Androl. (1990) 25:21–7. doi: 10.3109/01485019008987590
52. Krassas GE, Papadopoulou F, Tziomalos K, Zeginiadou T, Pontikides N. Hypothyroidism has an adverse effect on human spermatogenesis: A prospective, controlled study. Thyroid. (2008) 18:1255–9. doi: 10.1089/thy.2008.0257
53. Mintziori G, Kita M, Duntas L, Goulis DG. Consequences of hyperthyroidism in male and female fertility: pathophysiology and current management. J Endocrinol Invest. (2016) 39:849–53. doi: 10.1007/s40618-016-0452-6
54. Krassas GE, Pontikides N. Male reproductive function in relation with thyroid alterations. Best Pract Res Clin Endocrinol Metab. (2004) 18:183–95. doi: 10.1016/j.beem.2004.03.003
55. Krassas GE, Poppe K, Glinoer D. Thyroid function and human reproductive health. Endocr Rev. (2010) 31:702–55. doi: 10.1210/er.2009-0041
56. Chakraborty A, Mandal J, Mondal C, Sinha S, Chandra AK. Effect of excess iodine on oxidative stress markers, steroidogenic—Enzyme activities, testicular morphology, and functions in adult male rats. Biol Trace Elem Res. (2016) 172:380–94. doi: 10.1007/s12011-015-0581-3
57. Chakraborty A. Excess iodine exposure: An emerging area of concern for male reproductive physiology in the post-salt iodization era. Asian Pac J Reprod. (2021) 10:102–12. doi: 10.4103/2305-0500.316622
58. Lacroix L, Mian C, Caillou B, Talbot M, Filetti S, Schlumberger M, et al. Na(+)/I(-) symporter and Pendred syndrome gene and protein expressions in human extra-thyroidal tissues. Eur J Endocrinol. (2001) 144:297–302. doi: 10.1530/eje.0.1440297
59. Chandra AK, Ghosh R, Chatterjee A, Sarkar M. Effects of vanadate on male rat reproductive tract histology, oxidative stress markers and androgenic enzyme activities. J Inorg Biochem. (2007) 101:944–56. doi: 10.1016/j.jinorgbio.2007.03.003
60. Joanta A, Filip A, Clichici S, Andrei S, Cluj-Napoca Romania CNR. Iodide excess exerts oxidative stress in some target tissues of the thyroid hormones. Acta Physiol Hung. (2006) 93:347–59. doi: 10.1556/APhysiol.93.2006.4.11
61. Smith LB, Walker WH. The regulation of spermatogenesis by androgens. Semin Cell Dev Biol. (2014) 30:2–13. doi: 10.1016/j.semcdb.2014.02.012
62. Chandra AK, Chakraborty A. Influence of iodine in excess on seminiferous tubular structure and epididymal sperm character in male rats. Environ Toxicol. (2017) 32:1823–35. doi: 10.1002/tox.22405
63. Foroozanfard F, Sadat Z. Diagnostic value of hysterosalpingography and laparoscopy for tubal patency in infertile women. Nurs Midwifery Stud. (2013) 1:188–92. doi: 10.5812/nms.10661
64. Mathews DM, Peart JM, Sim RG, Johnson NP, O’Sullivan S, Derraik JGB, et al. The SELFI study: iodine excess and thyroid dysfunction in women undergoing oil-soluble contrast hysterosalpingography. J Clin Endocrinol Metab. (2022) 107:3252–60. doi: 10.1210/clinem/dgac546
65. Kaneshige T, Arata N, Harada S, Ohashi T, Sato S, Umehara N, et al. Changes in serum iodine concentration, urinary iodine excretion and thyroid function after hysterosalpingography using an oil-soluble iodinated contrast medium (Lipiodol). J Clin Endocrinol Metab. (2015) 100:E469–72. doi: 10.1210/jc.2014-2731
66. Mathews DM, Peart JM, Sim RG, Johnson NP, O’Sullivan S, Derraik JGB, et al. Iodine and other factors associated with fertility outcome following oil-soluble contrast medium hysterosalpingography: a prospective cohort study. Front Endocrinol. (2024) 15:1257888. doi: 10.3389/fendo.2024.1257888
67. Mahapatra D, Chandra AK. Biphasic action of iodine in excess at different doses on ovary in adult rats. J Trace Elem Med Biol. (2017) 39:210–20. doi: 10.1016/j.jtemb.2016.10.006
68. Mahapatra D, Chattopadhyay S, Chandra AK. Iodine in excess: impact on ovarian and uterine histology in adult rats(2017). Available online at: https://api.semanticscholar.org/CorpusID:43930845 (Accessed January 26, 2025).
69. Velasco I, Bath S, Rayman M. Iodine as essential nutrient during the first 1000 days of life. Nutrients. (2018) 10:290. doi: 10.3390/nu10030290
70. World Health Organization. Iodine status worldwide: WHO global database on iodine deficiency(2004). Available online at: https://iris.who.int/bitstream/handle/10665/43010/9241592001.pdf (Accessed January 17, 2025).
71. NHS. Foods to avoid in pregnancy. Available online at: https://www.nhs.uk/pregnancy/keeping-well/foods-to-avoid/ (Accessed January 17, 2025).
72. Lee SY, Pearce EN. Iodine intake in pregnancy—even a little excess is too much. Nat Rev Endocrinol. (2015) 11:260–1. doi: 10.1038/nrendo.2015.28
73. Rebagliato M, Murcia M, Espada M, Álvarez-Pedrerol M, Bolúmar F, Vioque J, et al. Iodine intake and maternal thyroid function during pregnancy. Epidemiology. (2010) 21:62–9. doi: 10.1097/EDE.0b013e3181c1592b
74. Pearce EN, Lazarus JH, Moreno-Reyes R, Zimmermann MB. Consequences of iodine deficiency and excess in pregnant women: an overview of current knowns and unknowns. Am J Clin Nutr. (2016) 104:918S–23S. doi: 10.3945/ajcn.115.110429
75. Connelly KJ, Boston BA, Pearce EN, Sesser D, Snyder D, Braverman LE, et al. Congenital hypothyroidism caused by excess prenatal maternal iodine ingestion. J Pediatr. (2012) 161:760–2. doi: 10.1016/j.jpeds.2012.05.057
76. Kalarani IB, Veerabathiran R. Impact of iodine intake on the pathogenesis of autoimmune thyroid disease in children and adults. Ann Pediatr Endocrinol Metab. (2022) 27:256–64. doi: 10.6065/apem.2244186.093
77. Tańska K, Gietka-Czernel M, Glinicki P, Kozakowski J. Thyroid autoimmunity and its negative impact on female fertility and maternal pregnancy outcomes. Front Endocrinol. (2023) 13:1049665. doi: 10.3389/fendo.2022.1049665
78. Jølving LR, Nielsen J, Kesmodel US, Nielsen R, Norgard BM, Beck-Nielsen SS. Chronic diseases in the children of women with maternal thyroid dysfunction: a nationwide cohort study. Clin Epidemiol. (2018) 10:1381–90. doi: 10.2147/CLEP.S167128
79. Dai M, Xu Y, Gong G, Zhang Y. Roles of immune microenvironment in the female reproductive maintenance and regulation: novel insights into the crosstalk of immune cells. Front Immunol. (2023) 14:1109122. doi: 10.3389/fimmu.2023.1109122
80. Lee S, Yoo I, Cheon Y, Choi E, Kim S, Ka H. Function of immune cells and effector molecules of the innate immune system in the establishment and maintenance of pregnancy in mammals — A review. Anim Biosci. (2024) 37:1821–33. doi: 10.5713/ab.24.0257
81. Abrams ET, Miller EM. The roles of the immune system in Women’s reproduction: Evolutionary constraints and life history trade-offs. Am J Phys Anthropol. (2011) 146:134–54. doi: 10.1002/ajpa.v146.53s
82. Nishiyama S, Mikeda T, Okada T, Nakamura K, Kotani T, Hishinuma A. Transient hypothyroidism or persistent hyperthyrotropinemia in neonates born to mothers with excessive iodine intake. Thyroid. (2004) 14:1077–83. doi: 10.1089/thy.2004.14.1077
83. Emder PJ, Jack MM. Iodine-induced neonatal hypothyroidism secondary to maternal seaweed consumption: A common practice in some Asian cultures to promote breast milk supply. J Paediatr Child Health. (2011) 47:750–2. doi: 10.1111/j.1440-1754.2010.01972.x
84. Purdue-Smithe AC, Männistö T, Bell GA, Mumford SL, Liu A, Kannan K, et al. The joint role of thyroid function and iodine status on risk of preterm birth and small for gestational age: A population-based nested case-control study of finnish women. Nutrients. (2019) 11:2573. doi: 10.3390/nu11112573
85. Li S, Zha H, Cui Y, Sun L, Yu L, Yuan Q. The relationship between excessive iodine during pregnancy and adverse pregnancy complications. Endocrine. (2024) 88:203–210. doi: 10.1007/s12020-024-04134-2
86. Chen R, Li Q, Cui W, Wang X, Gao Q, Zhong C, et al. Maternal iodine insufficiency and excess are associated with adverse effects on fetal growth: A prospective cohort study in Wuhan, China. J Nutr. (2018) 148:1814–20. doi: 10.1093/jn/nxy182
87. Shi X, Han C, Li C, Mao J, Wang W, Xie X, et al. Optimal and safe upper limits of iodine intake for early pregnancy in iodine-sufficient regions: A cross-sectional study of 7190 pregnant women in China. J Clin Endocrinol Metab. (2015) 100:1630–8. doi: 10.1210/jc.2014-3704
88. Zoeller RT, Brown TR, Doan LL, Gore AC, Skakkebaek NE, Soto AM, et al. Endocrine-disrupting chemicals and public health protection: A statement of principles from the endocrine society. Endocrinology. (2012) 153:4097–110. doi: 10.1210/en.2012-1422
89. Yilmaz B, Terekeci H, Sandal S, Kelestimur F. Endocrine disrupting chemicals: exposure, effects on human health, mechanism of action, models for testing and strategies for prevention. Rev Endocr Metab Disord. (2020) 21:127–47. doi: 10.1007/s11154-019-09521-z
90. Kabir ER, Rahman MS, Rahman I. A review on endocrine disruptors and their possible impacts on human health. Environ Toxicol Pharmacol. (2015) 40:241–58. doi: 10.1016/j.etap.2015.06.009
91. Lauretta R, Sansone A, Sansone M, Romanelli F, Appetecchia M. Endocrine disrupting chemicals: effects on endocrine glands. Front Endocrinol. (2019) 10:178. doi: 10.3389/fendo.2019.00178
Keywords: iodine, iodine excess, thyroid, thyroid hormones, reproductive health, infertility
Citation: Khudair A, Khudair A, Niinuma SA, Habib H and Butler AE (2025) From deficiency to excess: the impact of iodine excess on reproductive health. Front. Endocrinol. 16:1568059. doi: 10.3389/fendo.2025.1568059
Received: 28 January 2025; Accepted: 10 April 2025;
Published: 30 April 2025.
Edited by:
Joao Dts Anselmo, Hospital do Divino Espírito Santo, PortugalReviewed by:
Lucile Butruille, Muséum National d’Histoire Naturelle, FranceCopyright © 2025 Khudair, Khudair, Niinuma, Habib and Butler. This is an open-access article distributed under the terms of the Creative Commons Attribution License (CC BY). The use, distribution or reproduction in other forums is permitted, provided the original author(s) and the copyright owner(s) are credited and that the original publication in this journal is cited, in accordance with accepted academic practice. No use, distribution or reproduction is permitted which does not comply with these terms.
*Correspondence: Alexandra E. Butler, YWViOTEwMTFAZ21haWwuY29t; YWJ1dGxlckByY3NpLmNvbQ==
†These authors have contributed equally to this work and share first authorship