- 1Department of Orthopaedics, Tongde Hospital of Zhejiang Province, Hangzhou, China
- 2Department of Ultrasound Medicine, The 903rd Hospital of The People's Liberation Army, Hangzhou, Jiangsu, China
Osteoarthritis (OA), the most prevalent joint disorder associated with aging, is characterized by impaired extracellular matrix (ECM) synthesis and the degradation of articular cartilage. It is influenced by various factors, including aging and mechanical stress (such as traumatic injury). Increasing evidence suggests that alterations in cartilage stiffness occur during OA progression, particularly at its onset. This review comprehensively examines how aging and mechanical stress contribute to ECM stiffening, a precursor to irreversible cartilage degradation. We also discuss how increased matrix stiffness disrupts the homeostatic balance between chondrocyte catabolism and anabolism and the mechanotransduction pathways involved in cartilage stiffening. Furthermore, the potential of cartilage engineering to target the stiffness of synthetic materials is explored as a promising approach to advancing cartilage repair and regeneration in OA. A deeper understanding of this research area may not only lead to more innovative strategies for early OA detection and diagnosis but also offer novel insights into OA treatment and prognosis.
Introduction
Osteoarthritis (OA) is a complex degenerative condition influenced by factors such as aging, obesity, and trauma. OA begins at the molecular level and progressively disrupts the structure and function of articular cartilage, ultimately leading to irreversible damage (1). While some medications can alleviate inflammation and pain associated with OA, few have shown efficacy in halting or reversing the disease’s progression. This challenge is further exacerbated by the limited regenerative capacity of cartilage, leading to a shortage of clinically effective interventions for OA.
Matrix stiffness significantly influences cell proliferation, phenotype, and differentiation among the physical changes observed in OA (2). Research shows that matrix stiffness directly affects chondrocyte behavior and phenotype, particularly in laboratory conditions (3). The stiffening of the extracellular matrix (ECM) is a critical factor in cartilage aging and a major contributor to the development of knee OA (4). However, the precise molecular and cellular mechanisms underlying age-related changes in biomechanical properties remain largely unexplored. Furthermore, there is a notable gap in research focused on strategies targeting the downstream cellular response to cartilage matrix stiffness in OA treatment. This ongoing lack of effective therapeutic targets continues to challenge clinicians, often resulting in further cartilage degeneration or the need for total joint replacement (5, 6).
The upstream molecular mechanisms triggering cartilage matrix stiffening
Understanding the upstream molecular mechanisms that lead to cartilage matrix stiffening is essential for grasping the progression of OA. Alterations in ECM composition and chondrocyte behavior, driven by aging and mechanical stress, are key factors. These changes disrupt the balance between catabolic and anabolic processes in cartilage, leading to increased stiffness, a precursor to cartilage degradation and symptomatic OA.
Ageing-related cartilage matrix stiffening
Changes in ECM proteins
As articular cartilage ages, it undergoes substantial structural, compositional, and mechanical changes that lead to increased extracellular matrix (ECM) stiffness (7). In both mice and humans, this age-related stiffness is observed to be two to three times greater than that in younger adults (8). Changes in ECM proteins, such as collagen and proteoglycans, drive nonenzymatic collagen cross-linking and shorten aggrecan molecules (4, 9). Excessive collagen cross-linking increases cartilage stiffness, making it more brittle and susceptible to fatigue failure (10). The degradation of aggrecan further exacerbates this process by reducing sugar side chains and the ability to bind water (11).
Advanced glycation end-products
Additionally, elevated levels of advanced glycation end-products (AGEs) have been associated with decreased anabolic activity, which promotes further nonenzymatic collagen cross-linking, thereby increasing ECM stiffness (12). AGEs and their receptors in articular tissue provide valuable insights into the biochemical and biomechanical changes that contribute to OA pathogenesis (13). The accumulation of AGEs is linked to increased cellular senescence, which reduces chondrocyte metabolic and biosynthetic functions, promoting cartilage degeneration and OA progression (13). Studies have shown that the upregulation of AGEs in aging joints accelerates matrix stiffening through collagen cross-linking and the loss of glycosaminoglycans (GAGs) (4). In vitro experiments with threose have demonstrated increased AGE cross-linking in cartilage, leading to enhanced collagen network stiffness and contributing to age-related collagen degradation and increased susceptibility to damage (10).
Trauma-related cartilage matrix stiffening
Mechanical stress
Mechanical stress resulting from joint instability and injury is another significant risk factor for the development of osteoarthritis (OA). Traumatic events can accelerate the onset of OA, suggesting that matrix cross-linking in post-traumatic OA (PTOA) can occur independently of the advanced glycation end-products (AGEs) accumulation typically seen in age-related OA. While spontaneous OA is more common in older individuals, PTOA often affects younger adults, leading to irreversible cartilage degradation due to injury. PTOA may arise from direct damage to cartilage or trauma to the joint.
Lysyl oxidase secretion
Mechanical stress triggers the extracellular secretion of lysyl oxidase (LOX), an enzyme that significantly increases collagen matrix stiffness (Young’s modulus). Kim et al. identified mechanical stress as a key factor in cartilage matrix stiffening in OA (4). Their in vitro studies showed that LOX secretion enhances collagen matrix stiffness, with LOX-treated chondrocytes exhibiting OA-associated gene expression (4). In vivo, joint instability from medial meniscus destabilization (DMM) elevated LOX levels, which preceded MMP-13 expression and cartilage degradation. These findings suggest that matrix stiffening is an early event in trauma-related OA progression. In contrast, Chery et al. observed a moderate reduction in the modulus of DMM-treated murine cartilage, likely due to differences in study focus: Kim et al. measured the elastic modulus of LOX-CM gel used for culturing chondrocytes, while Chery et al. assessed cartilage tissue modulus three days post-DMM without considering ECM-degrading enzyme activity (14, 15). The immediate ECM degradation following DMM likely explains the reduced modulus observed. Despite these discrepancies, both studies highlight the critical role of ECM micromechanics in early PTOA, emphasizing the detrimental cycle of cartilage degeneration caused by impaired mechanosensitive responses in chondrocytes.
The downstream molecular mechanisms of cartilage matrix stiffening
The downstream molecular and cellular mechanisms that contribute to cartilage matrix stiffening, an early event in both spontaneous OA and PTOA, are not yet fully understood. Research shows that stiff substrates enhance chondrocyte stress fiber formation and alter cellular morphology, highlighting the importance of mechanotransductive signaling in regulating cellular phenotypes in response to matrix stiffness (14, 16). Several key mechanotransduction pathways have been identified in the initiation of OA (Figure 1).
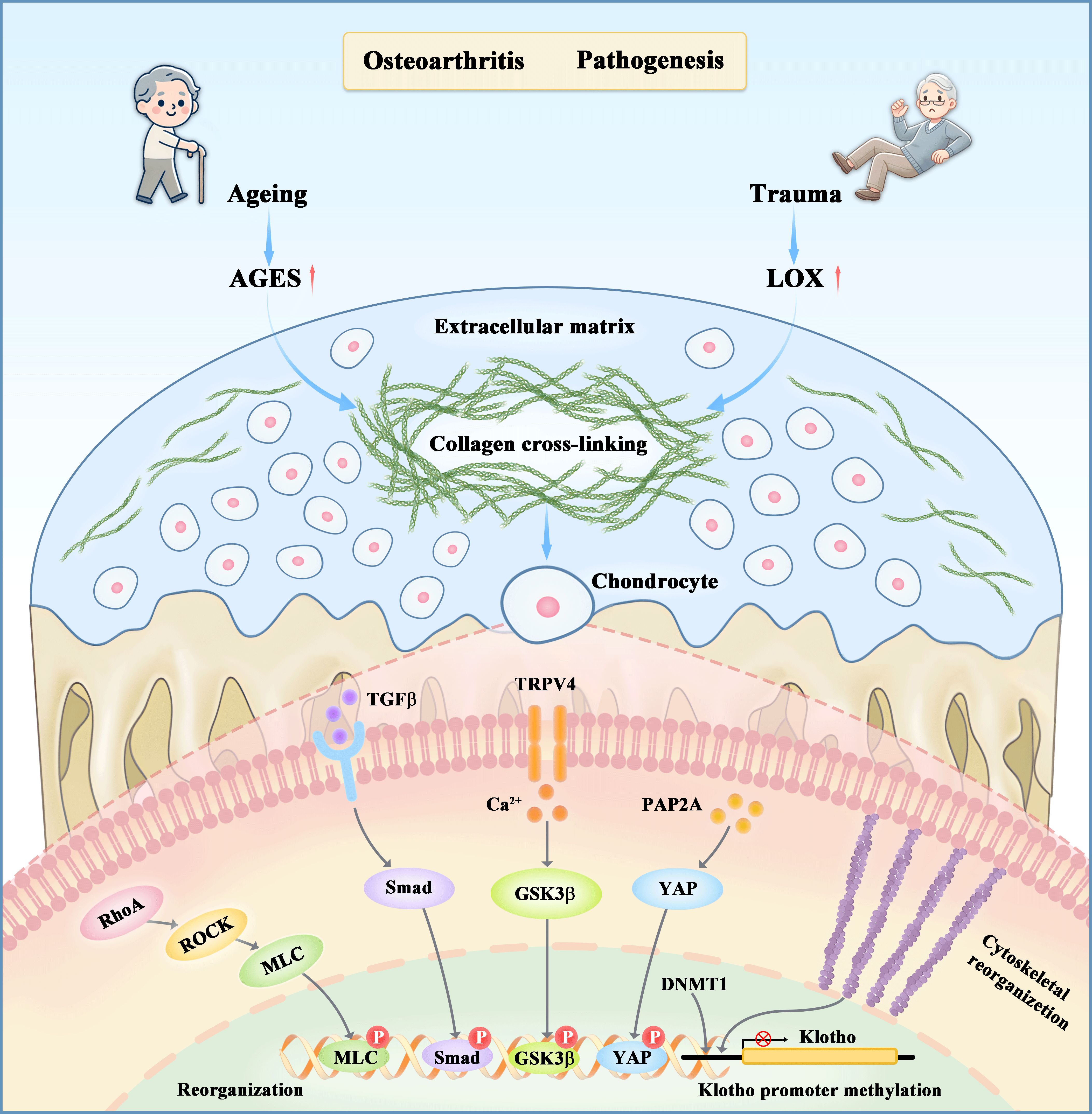
Figure 1. The regulatory effects of matrix stiffness on chondrocyte metabolism through both upstream and downstream mechanisms. Aging and mechanical stress are two primary factors that initiate osteoarthritis by inducing collagen crosslinking in cartilage tissues, resulting in extracellular matrix stiffening. This stiffening disrupts the catabolic-anabolic balance of chondrocyte metabolism through various mechanotransductive pathways, such as RhoA/ROCK, PAP2A/YAP, TGFβ/Smad, and Ca2+ channels TRPV4, as well as epigenetic modifications. Subsequent events include the phosphorylation of YAP, MLC, Smad3, and GSK3β, which activate genes involved in cytoskeleton reorganization, inflammation, and chondrocyte metabolism.
RhoA/ROCK signaling
The Ras homolog gene family member A (RhoA) and its downstream effector, Rho-associated protein kinase (ROCK), play crucial roles in various cellular processes, particularly in the pathogenesis of osteoarthritis (OA). Aberrant activation of the RhoA/ROCK pathway is strongly associated with OA development. This pathway is vital for regulating stress fiber formation and cytoskeletal reorganization within chondrocytes, both of which are key contributors to OA progression (17).
Recent studies have highlighted the differential behavior of chondrocytes in two-dimensional (2D) versus three-dimensional (3D) environments. In 2D cultures, increased matrix stiffness leads to abnormal RhoA/ROCK signaling, resulting in enhanced stress fiber formation and elevated catabolic activity. Kim et al. demonstrated that increased matrix stiffness activates the RhoA/ROCK pathway, leading to increased chondrocyte catabolism and decreased anabolism. Activation of the RhoA-ROCK-MLC axis drives these molecular changes, and inhibiting this axis can effectively prevent the stiffness-induced imbalance between catabolic and anabolic activities (4). Appleton et al. further found that stress fiber formation and contractility, driven by RhoA/ROCK signaling, negatively impact chondrocyte phenotype by altering Sox9 expression and disrupting actin organization. They proposed that targeting the RhoA/ROCK pathway could mitigate cartilage degeneration, promote cartilage maintenance, and support repair processes (18). Additionally, Liang et al. (19) revealed that leptin activates the RhoA/ROCK pathway, leading to significant cytoskeletal reorganization in chondrocytes. This reorganization alters cell morphology, causing changes such as flattening, elongation, and hypertrophy, and promotes the formation of stress fibers. These morphological and functional changes can disrupt normal cellular activities, shifting chondrocyte metabolism from anabolic (building up) to catabolic (breaking down) processes. However, in 3D environments, the effects of stiffness on RhoA/ROCK signaling can be attenuated or even reversed, with chondrocytes exhibiting less catabolic behavior in stiffer matrices. This suggests that the dimensionality of the culture system significantly influences the cellular response to mechanical cues, which is crucial for interpreting in vitro findings in the context of in vivo tissue environments.
TGFβ/SMAD3 signaling
Transforming growth factor-beta (TGFβ) is a versatile cytokine that plays a critical role in regulating cell proliferation, differentiation, and extracellular matrix production. Upon binding to its receptors on the cell surface, TGFβ initiates a signaling cascade that results in the phosphorylation and activation of SMAD3. Once activated, SMAD3 translocates to the nucleus, where it regulates gene expression related to chondrocyte metabolism. Research has shown that matrix stiffness can modulate the TGFβ/SMAD3 signaling pathway, significantly influencing chondrocyte metabolic responses (20, 21). For example, recent studies have demonstrated that chondrocytes cultured on substrates with a specific stiffness (0.5 MPa) exhibit enhanced differentiation. This is evidenced by increased deposition of proteoglycans and elevated expression levels of chondrogenic markers such as Sox9, Col2α1, and aggrecan. This process is mediated by the stiffness-sensitive induction of TGFβ1, which promotes SMAD3 phosphorylation, its nuclear localization, and subsequent transcriptional activity. These findings highlight the synergistic interaction between extracellular matrix (ECM) stiffness and TGFβ, revealing how chondrocytes integrate both physical and biochemical signals to coordinate their responses within the microenvironment (22).
YAP signaling
Yes-associated protein (YAP) is a well-characterized mechanotransducer that plays a crucial role in transmitting mechanical signals from surrounding cells to the nucleus (23). In articular cartilage, a load-bearing tissue, mechanical forces such as pressure, tensile stress, and fluid shear stress are mediated through the Hippo/YAP pathway (24, 25). Zhang et al. discovered that in stiffer microenvironments, YAP mediates chondrocyte catabolism by promoting YAP dephosphorylation and its translocation to the nucleus. Both in vivo and in vitro experiments have shown that increased nuclear YAP levels are associated with reduced cartilage matrix deposition in osteoarthritis (OA), particularly in cases where the microenvironment is stiffer (26). Further studies have demonstrated that genetic deletion of YAP or pharmacological inhibition of its nuclear translocation can enhance chondrocyte anabolism in stiff scaffolds, leading to reduced cartilage degradation in OA animal models. Similarly, Zhong et al. identified a clear correlation between substrate stiffness and changes in chondrocyte phenotype, with YAP localization shifting in response to these changes. Specifically, nuclear YAP levels were significantly lower on softer substrates, which coincided with an increase in Col2α1 expression compared to stiffer substrates (27). Recent evidence highlights the crosstalk between the TGFβ/SMAD3 and YAP signaling pathways in osteoarthritis (OA). Runx1 has been identified as a key regulator that coordinates multiple signaling pathways, including TGFβ/Smad, Hippo/YAP, and Wnt/β-catenin (28). It integrates these pathways to regulate articular cartilage homeostasis and contribute to cartilage degradation in OA (29). Specifically, Runx1 influences the expression of YAP and p-Smad2/3, which are critical mediators in these signaling pathways. Loss of Runx1 in cartilage leads to a marked reduction in YAP protein levels and p-Smad2/3 expression. This suggests that Runx1 is essential for maintaining the activity of both the TGFβ and Hippo/YAP pathways. In the absence of Runx1, the function of these pathways is impaired, resulting in cartilage degradation and osteophyte formation in OA. Notably, the TGFβ pathway plays a pivotal role in maintaining cartilage integrity by regulating the phosphorylation of Smad2/3, a key step in its activation (30).
α-Klotho signaling
A recent systematic review has highlighted that elevated inflammation, increased senescence, and impaired autophagy are common factors associated with age-induced knee osteoarthritis (OA) in mice (31), all of which are downstream of α-Klotho (32, 33). Moreover, studies have demonstrated that α-Klotho overexpression can delay cartilage degeneration in a post-traumatic OA (PTOA) model. Earlier in vitro and in vivo investigations have suggested that α-Klotho overexpression can alleviate chondrocyte dysfunction and cartilage degeneration in a PTOA model (34). Additionally, a separate study has shown that combining α-Klotho overexpression with soluble TGF-β supplementation can enhance chondrocyte health and cartilage integrity in a chemically-induced OA model (35). Iijima et al. (36) found that young chondrocytes cultured on rigid substrates exhibited aging characteristics compared to those cultured on flexible substrates, as evidenced by decreased levels of type II collagen and aggrecan. This change coincided with a reduction in α-Klotho protein levels. In contrast, aged chondrocytes cultured on flexible substrates showed elevated levels of type II collagen and α-Klotho compared to those cultured on rigid substrates.
Calcium signaling
Recent studies have examined the influence of extracellular microenvironment stiffness on intracellular Ca2+ signaling in chondrocytes and its implications for osteoarthritis (OA) progression. The TRPV4 ion channel, which facilitates the passage of calcium ions, plays a pivotal role in regulating mechanical stress in cartilage. Alterations in TRPV4 expression have been found to affect OA progression differently depending on age and traumatic injury (37). Additionally, ECM viscoelasticity has been shown to modulate TRPV4 activity in healthy chondrocytes, leading to a decrease in intracellular Ca2+ in fast-relaxation matrices and an increase in intracellular Ca2+ in low-relaxation matrices (38). Similarly, Zhang et al. (3) demonstrated that different substrate stiffness levels induce distinct dynamic deformations in chondrocytes during cell swelling and recovery processes. Specifically, chondrocytes exhibit accelerated swelling on soft substrates but slower recovery compared to stiff substrates when subjected to hypo-osmotic challenges. Their research also revealed that stiff substrates intensify cytosolic Ca2+ oscillations in chondrocytes under iso-osmotic conditions. Soft substrates notably influence Ca2+ oscillations during cell swelling, while stiff substrates enhance cytosolic Ca2+ oscillations during cell recovery. Moreover, the TRPV4 channel contributes to chondrocyte perception of substrate stiffness by modulating Ca2+ signaling in a manner dependent on substrate stiffness.
Clinically relevant evidence on cartilage ECM stiffening
Although no clinical trials have yet been conducted on the role of cartilage matrix stiffness in the pathogenesis of OA, experimental findings based on human samples have already demonstrated that cartilage matrix stiffness can accelerate chondrocyte senescence and promote cartilage degradation. Fu et al. (39) demonstrated that human OA cartilage exhibits increased matrix stiffness compared to normal cartilage. This stiffening was positively correlated with the degree of cartilage damage, supporting the hypothesis that matrix stiffness is a critical factor in OA progression. In human samples, senescent chondrocytes exhibited higher expression of markers such as p16INK4a, p21, and p53. These findings suggest a strong association between ECM stiffness and the senescence phenotype in chondrocytes, further linking matrix stiffness to cartilage degeneration in OA. Liu et al. (40) found valuable insights into the mechanistic relationship between matrix stiffness, chondrocyte behavior, and the progression of intervertebral disc degeneration in humans. Human cartilage endplate (CEP) samples from 48 patients undergoing spinal fusion surgery were analyzed. The matrix stiffness of the CEPs increased with the degeneration grade, from mild (Grade 2) to severe (Grade 6), and was positively correlated with the degree of IDD. As the degeneration progressed, the elastic modulus of the matrix increased, indicating stiffer matrices in more degenerated CEPs. This change in matrix stiffness was linked to collagen disarrangement and the degeneration of cartilage in these human samples.
Matrix stiffness as a promising microenvironmental factor in cartilage engineering
Matrix-assisted autologous chondrocyte implantation (MACI) has garnered considerable attention for its potential to treat articular cartilage lesions in osteoarthritis (OA), as well as its ability to improve biomimetic substrate designs that promote extracellular matrix (ECM) deposition by transplanted chondrocytes (41). By cultivating chondrocytes in vitro within scaffolds of varying stiffness, researchers have developed a reliable method to study the impact of matrix stiffness on chondrocyte metabolism. Various studies have explored the role of scaffold stiffness in regulating cytoskeletal organization, metabolic activity, and chondrocyte proliferation on these substrates (42–44). Shojarazavi et al. (45) developed an injectable hydrogel based on alginate and cartilage ECM, enhanced with silk fibroin nanofibers (SFN), to improve cartilage tissue engineering. By optimizing SFN concentrations, they achieved a targeted mechanical stiffness of 102.97 ± 4.75 kPa. Cell viability assessments and measurements of glycosaminoglycan (GAG) and collagen II content revealed that this hydrogel effectively mimicked the natural cartilage environment, highlighting its significant potential. The morphology of chondrocytes is intricately controlled by the formation and organization of their cytoskeletal fibers (46). Chondrocytes are sensitive to the stiffness of their surrounding scaffolds, which induces structural adjustments in their cytoskeletons to align with the mechanical properties of their microenvironment (47). Studies using atomic force microscopy (AFM) to evaluate the mechanical properties of scaffolds have shown that stiffer matrices lead to increased elastic modulus and other viscoelastic parameters of chondrocytes. Furthermore, research has indicated that chondrocytes cultured on hydrogels with higher Young’s modulus exhibit a rise in cellular stiffness (48). These insights are valuable for optimizing the design of scaffolds for cartilage repair, which could further enhance outcomes for OA regeneration.
Prolotherapy for ECM and cartilage regeneration
Prolotherapy, particularly dextrose prolotherapy, has shown potential in promoting tissue regeneration by stimulating local inflammatory responses and collagen deposition (49). Studies have suggested that dextrose injections can stimulate the production of growth factors, such as platelet-derived growth factor, transforming growth factor-β, and basic fibroblast growth factor, which help repair damaged cartilage and surrounding tissues (50, 51). While the precise mechanisms of prolotherapy remain debated, its potential in ECM and cartilage regeneration is promising, with multiple studies highlighting its efficacy in pain reduction and improved function for OA patients (52, 53). Further research and inclusion of such treatments in clinical practice could enhance current therapies for cartilage regeneration.
Conclusions
Osteoarthritis (OA) is a prevalent knee joint disorder worldwide, with age, obesity, and trauma recognized as primary risk factors. While medications exist to reduce inflammation and manage OA-associated pain, few have demonstrated efficacy in halting or improving disease progression. The limited success of non-surgical OA treatments can be attributed, at least in part, to gaps in our understanding of the translational relevance of preclinical OA models and an incomplete understanding of the molecular mechanisms underlying disease pathogenesis. This review analyzes recent developments in understanding how changes in cartilage stiffness contribute to OA progression. Aging and mechanical strain, which are associated with joint instability and trauma—primary risk factors for initiating OA—have been identified as sources of matrix collagen crosslinking, leading to cartilage stiffness. The relevant mechanotransductive signaling pathways following cartilage stiffening are discussed, along with their involvement in chondrocyte anabolism and catabolism. Although cartilage ECM stiffening is an early molecular event in OA, current methods for detecting the degree of cartilage matrix sclerosis are limited to laboratory techniques, such as nano-AFM microscopy. There is currently a lack of effective clinical detection tools, which further hinders its clinical translation. It is hoped that increased attention and understanding in this research area will lead to the development of more innovative methods for early OA detection and diagnosis, as well as promising treatment and prognosis strategies.
Author contributions
KH: Writing – original draft, Writing – review & editing. HC: Writing – original draft, Writing – review & editing.
Funding
The author(s) declare that financial support was received for the research and/or publication of this article. The study was supported by Zhejiang Provincial Natural Science Foundation (grant number LY23H060002) and the National Natural Science Foundation of China (grant number 81871792). No benefits in any form have been, or will be, received from a commercial party related directly, or indirectly, to the subject of this study.
Conflict of interest
The authors declare that the research was conducted in the absence of any commercial or financial relationships that could be construed as a potential conflict of interest.
Generative AI statement
The author(s) declare that no Generative AI was used in the creation of this manuscript.
Publisher’s note
All claims expressed in this article are solely those of the authors and do not necessarily represent those of their affiliated organizations, or those of the publisher, the editors and the reviewers. Any product that may be evaluated in this article, or claim that may be made by its manufacturer, is not guaranteed or endorsed by the publisher.
Abbreviations
OA, Osteoarthritis; ECM, Extracellular Matrix; AGE, Advanced Glycation End-products; PTOA, Post-traumatic Osteoarthritis; LOX, Lysyl Oxidase; DMM, Destabilization of the Medial Meniscus; RhoA, Ras homolog gene family, member A; ROCK, Rho-associated protein kinase; TGFβ, Transforming Growth Factor-beta; YAP, Yes-associated protein; TRPV4, Transient Receptor Potential Vanilloid 4; MACI, Matrix-assisted autologous chondrocyte implantation; SFN, Silk Fibroin Nanofibers; AFM, Atomic Force Microscopy; GAG, Glycosaminoglycans.
References
1. Yao Q, Wu Q, Lin Z, Zhang S, Tan L, Zheng W, et al. Osteoarthritis: pathogenic signaling pathways and therapeutic targets. Signal Transduct Target Ther. (2023) 8:56. doi: 10.1038/s41392-023-01330-w
2. Wang X, Xu Z, Shen Z, Li M, Guo J, Wang Y, et al. Matrix stiffness regulates osteoclast fate through integrin-dependent mechanotransduction. Bioact Mater. (2023) 27:138–53. doi: 10.1016/j.bioactmat.2023.03.014
3. Zhang M, Zhang L, Yang Y, Wang J, Wang L, Li W, et al. Substrate stiffness-dependent regulatory volume decrease and calcium signaling in chondrocytes. Acta Biochim Biophys Sin (Shanghai). (2022) 54:113–25. doi: 10.3724/abbs.2021008
4. Kim JH, Jeon J, Shin M, Kang D, Park S, Han Y, et al. Matrix cross-linking-mediated mechanotransduction promotes posttraumatic osteoarthritis. Proc Natl Acad Sci U S A. (2015) 112:9424–9. doi: 10.1073/pnas.1505700112
5. Hoveidaei AH, Abbasi-Kangevari M, Bagheri-Hariri S, Zaree F, Farrokhpour H, Seddighi N, et al. Patient satisfaction following robotic unicompartmental knee arthroplasty: A systematic review and meta-analysis. Technol Health Care. (2024) 32(5):3625–34. doi: 10.3233/THC-231216
6. Beckers G, Liesman T, Zuiderbaan HA, Postma R, Mullins J, Smith E, et al. Ten flaws of systematic mechanical alignment total knee arthroplasty. J Arthroplasty. (2024) 39:591–9. doi: 10.1016/j.arth.2023.11.023
7. Huynh J, Nishimura N, Rana K, Barzilai N, Cohen P, Martos-Moreno GA, et al. Age-related intimal stiffening enhances endothelial permeability and leukocyte transmigration. Sci Transl Med. (2011) 3:112ra122. doi: 10.1126/scitranslmed.3002761
8. Selman M, Pardo A. Fibroageing: An ageing pathological feature driven by dysregulated extracellular matrix-cell mechanobiology. Ageing Res Rev. (2021) 70:101393. doi: 10.1016/j.arr.2021.101393
9. DeGroot J, Verzijl N, Wenting-van Wijk MJ, Bank RA, Lafeber FP, Bijlsma JW, et al. Accumulation of advanced glycation end products as a molecular mechanism for aging as a risk factor in osteoarthritis. Arthritis Rheumatol. (2004) 50:1207–15. doi: 10.1002/art.20170
10. Verzijl N, DeGroot J, Thorpe SR, Bank RA, Shaw JN, Lyons TJ, et al. Crosslinking by advanced glycation end products increases the stiffness of the collagen network in human articular cartilage: a possible mechanism through which age is a risk factor for osteoarthritis. Arthritis Rheumatol. (2002) 46:114–23. doi: 10.1002/1529-0131(200201)46:1<114::AID-ART10025>3.0.CO;2-P
11. Ohnishi T, Iwasaki N, Sudo H, Sugimoto Y, Takeda S, Chiba K, et al. Causes of and molecular targets for the treatment of intervertebral disc degeneration: A review. Cells. (2022) 11(3):394. doi: 10.3390/cells11030394
12. Wang L, Jiang Y, Zhao C, Wu D, Cao L, Wang X, et al. The effects of advanced glycation end-products on skin and potential anti-glycation strategies. Exp Dermatol. (2024) 33:e15065. doi: 10.1111/exd.15065
13. Song J, Zhu H, Zeng X, Li Y, Liu Z, Chen H, et al. Alteration in cartilage matrix stiffness as an indicator and modulator of osteoarthritis. Biosci Rep. (2024) 44(1):BSR20231730. doi: 10.1042/BSR20231730
14. Danalache M, Klein TJ, Sieber SA, Neumann AJ, Notbohm J, Petersen A, et al. Changes in stiffness and biochemical composition of the pericellular matrix as a function of spatial chondrocyte organisation in osteoarthritic cartilage. Osteoarthritis Cartilage. (2019) 27:823–32. doi: 10.1016/j.joca.2019.01.008
15. Danalache M, Neumann AJ, Klein TJ, Sieber SA, Notbohm J, Petersen A, et al. Assessment of biomechanical properties of the extracellular and pericellular matrix and their interconnection throughout the course of osteoarthritis. J Biomech. (2019) 97:109409. doi: 10.1016/j.jbiomech.2019.109409
16. Zhao Z, Li Y, Wang M, Li H, Su Y, Tang W, et al. Mechanotransduction pathways in the regulation of cartilage chondrocyte homoeostasis. J Cell Mol Med. (2020) 24:5408–19. doi: 10.1111/jcmm.v24.10
17. Deng Z, Li Y, Liu H, Deng X, He X, Tang X, et al. RhoA/ROCK pathway: implication in osteoarthritis and therapeutic targets. Am J Transl Res. (2019) 11:5324–31.
18. Appleton CT, Pitelka V, Henry JL, Beier F. Rho/ROCK and MEK/ERK activation by transforming growth factor-alpha induces articular cartilage degradation. Lab Invest. (2010) 90:20–30. doi: 10.1038/labinvest.2009.111
19. Liang J, Wang J, Huang J, Du X, Song Y, Xia C, et al. Leptin-mediated cytoskeletal remodeling in chondrocytes occurs via the RhoA/ROCK pathway. J Orthop Res. (2011) 29:369–74. doi: 10.1002/jor.21257
20. Vincent TL. Targeting mechanotransduction pathways in osteoarthritis: a focus on the pericellular matrix. Curr Opin Pharmacol. (2013) 13:449–54. doi: 10.1016/j.coph.2013.01.010
21. Massague J. TGFbeta signalling in context. Nat Rev Mol Cell Biol. (2012) 13:616–30. doi: 10.1038/nrm3434
22. Allen JL, Cooke ME, Alliston T. ECM stiffness primes the TGFbeta pathway to promote chondrocyte differentiation. Mol Biol Cell. (2012) 23:3731–42. doi: 10.1091/mbc.e12-03-0172
23. Cuahtecontzi DR, Gatica J, Tellez-Romero J, Vazquez-Ramirez A, Lopez-Reyes M, Gonzalez I, et al. Mechanotransducive surfaces for enhanced cell osteogenesis, a review. Biomater Adv. (2024) 160:213861. doi: 10.1016/j.bioadv.2024.213861
24. Halder G, Dupont S, Piccolo S. Transduction of mechanical and cytoskeletal cues by YAP and TAZ. Nat Rev Mol Cell Biol. (2012) 13:591–600. doi: 10.1038/nrm3416
25. Zhong W, Tian K, Zheng X, Li L, Yin B, Yang X, et al. Mesenchymal stem cell and chondrocyte fates in a multishear microdevice are regulated by Yes-associated protein. Stem Cells Dev. (2013) 22:2083–93. doi: 10.1089/scd.2012.0685
26. Zhang X, Chen X, Zhang S, Zheng X, Liu H, Wei Y, et al. Targeting downstream subcellular YAP activity as a function of matrix stiffness with Verteporfin-encapsulated chitosan microsphere attenuates osteoarthritis. Biomater. (2020) 232:119724. doi: 10.1016/j.biomaterials.2019.119724
27. Zhong W, Tian K, Zheng X, Li L, Yin B, Yang X, et al. YAP-mediated regulation of the chondrogenic phenotype in response to matrix elasticity. J Mol Histol. (2013) 44:587–95. doi: 10.1007/s10735-013-9502-y
28. Tang CY, Wu M, Zhao D, Edwards D, McVicar A, Luo Y, et al. Runx1 is a central regulator of osteogenesis for bone homeostasis by orchestrating BMP and WNT signaling pathways. PloS Genet. (2021) 17:e1009233. doi: 10.1371/journal.pgen.1009233
29. Yano F, Ohba S, Murahashi Y, Tanaka S, Saito T, Chung UI. Runx1 contributes to articular cartilage maintenance by enhancement of cartilage matrix production and suppression of hypertrophic differentiation. Sci Rep. (2019) 9:7666. doi: 10.1038/s41598-019-43948-3
30. Zhang Y, Zuo T, McVicar A, Yang HL, Li YP, Chen W. Runx1 is a key regulator of articular cartilage homeostasis by orchestrating YAP, TGFβ, and Wnt signaling in articular cartilage formation and osteoarthritis. Bone Res. (2022) 10:63. doi: 10.1038/s41413-022-00231-y
31. Iijima H, Yasuhara R, Onodera T, Kishimoto K, Mochizuki T, Mikami Y, et al. Meta-analysis integrated with multi-omics data analysis to elucidate pathogenic mechanisms of age-related knee osteoarthritis in mice. J Gerontol A Biol Sci Med Sci. (2022) 77:1321–34. doi: 10.1093/gerona/glab386
32. Zhou H, Li Y, Wang Y, Dong H, Chen S, Zhang J, et al. Klotho as potential autophagy regulator and therapeutic target. Front Pharmacol. (2021) 12:755366. doi: 10.3389/fphar.2021.755366
33. Lim SW, Jin L, Luo K, Jin J, Yang CW. Klotho enhances FoxO3-mediated manganese superoxide dismutase expression by negatively regulating PI3K/AKT pathway during tacrolimus-induced oxidative stress. Cell Death Dis. (2017) 8:e2972. doi: 10.1038/cddis.2017.365
34. Chuchana P, Vignais ML, De Fraipont F, Arnaud J, Chevallet M, Muller L, et al. Secreted alpha-Klotho maintains cartilage tissue homeostasis by repressing NOS2 and ZIP8-MMP13 catabolic axis. Aging (Albany NY). (2018) 10:1442–53. doi: 10.18632/aging.101481
35. Martinez-Redondo P, Malandrino A, Schneider S, Cortes R, Paz JC, Martinez-Diaz S, et al. alphaKLOTHO and sTGFbetaR2 treatment counteract the osteoarthritic phenotype developed in a rat model. Protein Cell. (2020) 11:219–26. doi: 10.1007/s13238-019-00685-7
36. Iijima H, Yasuhara R, Onodera T, Kishimoto K, Mikami Y, Tamamura R, et al. Age-related matrix stiffening epigenetically regulates alpha-Klotho expression and compromises chondrocyte integrity. Nat Commun. (2023) 14:18. doi: 10.1038/s41467-022-35359-2
37. Agarwal P, Schulz JN, Blumbach K, Andreasson K, Ruivo-Myers A, Albro MB, et al. A dysfunctional TRPV4-GSK3beta pathway prevents osteoarthritic chondrocytes from sensing changes in extracellular matrix viscoelasticity. Nat BioMed Eng. (2021) 5:1472–84. doi: 10.1038/s41551-021-00691-3
38. Du G, Liu X, Zhao S, Zhao Y, Sun Z, Zhao Z, et al. The potential role of mechanosensitive ion channels in substrate stiffness-regulated Ca(2+) response in chondrocytes. Connect Tissue Res. (2022) 63:453–62. doi: 10.1080/03008207.2021.2007902
39. Fu B, Shen J, Zou X, Sun N, Zhang Z, Liu Z, et al. Matrix stiffening promotes chondrocyte senescence and the osteoarthritis development through downregulating HDAC3. Bone Res. (2024) 12:32. doi: 10.1038/s41413-024-00333-9
40. Liu MH, Sun C, Yao Y, Fan X, Liu H, Cui YH, et al. Matrix stiffness promotes cartilage endplate chondrocyte calcification in disc degeneration via miR-20a targeting ANKH expression. Sci Rep. (2016) 6:25401. doi: 10.1038/srep25401
41. Yuan X, Wang JY, Meng T, Ma XW, Li H, Li K. Role of hydrogels in osteoarthritis: A comprehensive review. Int J Rheum Dis. (2023) 26:2390–401. doi: 10.1111/1756-185X.14968
42. Du G, Liu X, Zhao Y, Sun Z, Zhao Z, Zhao S, et al. Development of alginate-collagen interpenetrating network for osteoarthritic cartilage by in situ softening. Int J Biol Macromol. (2024) 266:131259. doi: 10.1016/j.ijbiomac.2024.131259
43. Zhu J, Sun L, Zhang H, Wang Y, Guo W, Li Y, et al. Mesenchymal stromal cells modulate YAP by verteporfin to mimic cartilage development and construct cartilage organoids based on decellularized matrix scaffolds. J Mater Chem B. (2023) 11:7442–53. doi: 10.1039/D3TB01114C
44. Xu J, Wang Q, Wang C, Zhang Y, Wei Z, Wu Z, et al. The association between genetic polymorphisms of matrix metalloproteinases and knee osteoarthritis: A systematic review and meta-analysis. Int J Rheum Dis. (2024) 27:e15123. doi: 10.1111/1756-185X.15123
45. Shojarazavi N, Pasbakhsh P, Mohamadyar-Toupkanlou F, Moallem SA, Akbarzadeh R. Alginate/cartilage extracellular matrix-based injectable interpenetrating polymer network hydrogel for cartilage tissue engineering. J Biomater Appl. (2021) 36:803–17. doi: 10.1177/08853282211024020
46. Duan M, Zang X, Zhuang Y, Yang X, Liu X, Liu L, et al. Stiffened fibre-like microenvironment based on patterned equidistant micropillars directs chondrocyte hypertrophy. Mater Today Bio. (2023) 20:100682. doi: 10.1016/j.mtbio.2023.100682
47. Schuh E, Kramer J, Rohwedel J, Notbohm H, Muller R, Goecke T, et al. The influence of matrix elasticity on chondrocyte behavior in 3D. J Tissue Eng Regener Med. (2012) 6:e31–42. doi: 10.1002/term.v6.10
48. Chen C, Tambe DT, Deng L, Yang L. Substrate stiffness together with soluble factors affects chondrocyte mechanoresponses. ACS Appl Mater Interfaces. (2014) 6:16106–16. doi: 10.1021/am504135b
49. Waluyo Y, Artika SR, Wahyuni IN, Gunawan AMAK, Zainal ATF. Efficacy of prolotherapy for osteoarthritis: A systematic review. J Rehabil Med. (2023) 55:jrm00372. doi: 10.2340/jrm.v55.2572
50. Hsu C, Vu K, Borg-Stein J. Prolotherapy: A narrative review of mechanisms, techniques, and protocols, and evidence for common musculoskeletal conditions. Phys Med Rehabil Clin N Am. (2023) 34:165–80. doi: 10.1016/j.pmr.2022.08.011
51. Siadat AH, Isseroff RR. Prolotherapy: potential for the treatment of chronic wounds? Adv Wound Care (New Rochelle). (2019) 8:160–7. doi: 10.1089/wound.2018.0866
52. Mohamadi Jahromi LS, Dashtimakan H, Roshanzamir S, Dabbaghmanesh A, Parvin R. Effects of intra-articular versus peri-articular dextrose prolotherapy in knee osteoarthritis: A clinical trial study. Turk J Phys Med Rehabil. (2024) 70:327–34. doi: 10.5606/tftrd.2024.12937
Keywords: osteoarthritis, cartilage, matrix stiffness, signaling pathways, chondrocytes
Citation: Huang K and Cai H (2025) Matrix stiffness in osteoarthritis: from mechanism introduction to biomaterial-based therapies. Front. Endocrinol. 16:1571502. doi: 10.3389/fendo.2025.1571502
Received: 05 February 2025; Accepted: 15 April 2025;
Published: 08 May 2025.
Edited by:
James Cheng-Chung Wei, Chung Shan Medical University Hospital, TaiwanReviewed by:
Hy Lin, Chung Shan Medical University Hospital, TaiwanJing Yang, Second Hospital of Hebei Medical University, China
Copyright © 2025 Huang and Cai. This is an open-access article distributed under the terms of the Creative Commons Attribution License (CC BY). The use, distribution or reproduction in other forums is permitted, provided the original author(s) and the copyright owner(s) are credited and that the original publication in this journal is cited, in accordance with accepted academic practice. No use, distribution or reproduction is permitted which does not comply with these terms.
*Correspondence: Kai Huang, aHpodWFuZ2tAMTYzLmNvbQ==