- Cellular Immunology, International Centre for Genetic Engineering and Biotechnology, Trieste, Italy
T-cell activation within immunological synapses is a complex process whereby different types of signals are transmitted from antigen-presenting cells to T cells. The molecular strategies developed by T cells to interpret and integrate these signals have been systematically dissected in recent years and are now in large part understood. On the other side of the immune synapse, dendritic cells (DCs) participate actively in synapse formation and maintenance by remodeling of membrane receptors and intracellular content. However, the details of such changes have been only partially characterized. The DCs actin cytoskeleton has been one of the first systems to be identified as playing an important role in T-cell priming and some of the underlying mechanisms have been elucidated. Similarly, the DCs microtubule cytoskeleton undergoes major spatial changes during synapse formation that favor polarization of the DCs subcellular space toward the interacting T cell. Recently, we have begun to investigate the trafficking machinery that controls polarized delivery of endosomal vesicles at the DC–T immune synapse with the aim of understanding the functional relevance of polarized secretion of soluble factors during T-cell priming. Here, we will review the current knowledge of events occurring in DCs during synapse formation and discuss the open questions that still remain unanswered.
Introduction
Once in the lymph node, dendritic cells (DCs) have few hours to transfer the information gathered in the periphery to T cells. Live imaging of the lymph node unveiled that, depending on the inflammatory context and antigen density, T-cell activation can ensue from long-lasting interactions or by collection of signals from subsequent repetitive contacts (1). Recent technologies to visualize recruitment and activation of signaling molecules in vivo within individual DC–T contacts is beginning to shed light on the actual functional meaning of various types of intercellular encounters (2, 3). Nevertheless, our present understanding of the subcellular events underlying informational transfer at the synapse and signal decoding by T cells mostly comes from in vitro studies. As largely discussed in this topic, sophisticated analysis unveiled the mechanism by which the antigen-presenting cell (APC)-derived flow of information is turned into fine tuned T-cell activation. Less is known, instead, about the events that control efficient delivery of antigen-derived signals to T cells. Among APCs, DCs are uniquely potent in their ability to launch adaptive responses. They are composed of a complex network of different subsets that differentially control T-cell functions. The classical CD8α+ with the ontogenetically related tissue resident CD103+ cells and the Cd11b subsets share some general principles, i.e., the ability to migrate from tissue to regional lymph nodes charged with antigens, but are specialized in the activation of CD8+ and CD4+ T lymphocytes, respectively (4). Yet, this paradigm is still evolving and these distinctions may turn out to be less strict.
In the following paragraphs, the events that takes place on the DCs side of the immune synapse (DC-IS) refer mostly to studies conducted using DCs models, i.e., cells differentiated from monocytes in the human system or from bone marrow precursors in the mouse system and unfortunately do not yet take into account such complexity. Still, common themes have emerged that will certainly be translated into more physiological cell types in the near future.
Surface Receptors
To simplify and categorize events occurring at the DC-IS, it is useful to proceed from the surface inward to the cytoplasm. Remodeling of the plasma membrane architecture and redistribution of the surface receptors have been documented in DCs from the earliest instance upon T-cell contact and even before the two cell bodies become adherent. Some conserved mechanisms to support intercellular interactions described in the neuronal synapse operate in DCs and are essential for contact formation, including semaphorins, plexins, and neuropilins (5). Plexin-1A, a receptor for class-3 semaphorins is highly expressed in mature DCs, cluster at the IS, and controls T-cell priming by regulating cytoskeletal remodeling, possibly via Rho activation (6, 7). A second receptor for semaphorins, neuropilin-1, is expressed on both sides of the synapse and can control DC–T encounter during priming and during instruction of regulatory T cells (8, 9). The way DCs interact with T cells is peculiar in terms of global geometry and microdomains organization, as compared with the synapse between B cells and T cells. Unlike B cells, DCs possess a highly dynamic membrane with projections such as veils and ruffles that are increased during the process of maturation (10, 11) and further modify when an approaching T cell is sensed by chemokine receptors (12, 13). In human DCs, a peculiar microvilli-like structure was shown to be the preferential site of association with T cells leading to multiple aggregates of TCR/CD28 signaling complexes in T cells, as opposed to the concentrically structured immune synapse formed by B cells (14). A multifocal synapse is frequently formed also in murine T cells interacting with DCs, further strengthening that antigen presentation by DCs is more dynamic than by B cell (14, 15). Mirroring TCR clustering, MHC class-II and costimulatory molecules such as CD86 are recruited at the DC contact area very early during initial DC–T scanning. This recruitment is driven by ICAM-1/3 interactions with LFA-1 via, a mechanism that depends on an intact actin cytoskeleton and the Vav1/Rac1 effectors (16). Delivery of MHC class-II at the plasma membrane depends on long tubules of late endosomal origin that contain MHC–peptide complexes and move directionally toward the contact zone. This process is conserved in murine and human DCs both during antigen presentation and cross-presentation (17, 18) and ensures continuous fueling of TCR ligands in the IS region. Other costimulatory molecules undergo spatial reorganization on the APC. CD40 clusters at the synapse in B cells during delivery of T-cell help and it is stored in intracellular vesicles and rapidly released upon contact with an allogeneic CD4 T cells in rat lymph DCs (19, 20). Mirroring CD40 clustering, CD40L in T cells is recruited at the IS with human DCs and this polarization is important for DCs–T cross-talk and T-cell induced DCs maturation as it triggers IL-12 production by DCs (21). T-cell-induced DC maturation provides an important demonstration of the mutual exchange that occurs between the two cells [reviewed in Ref. (22)]. A further costimulatory molecule CD70, a TNF-family member receptor with critical roles in T-cell priming, is concentrated at the DC-IS. Interestingly, CD70 is found in intracellular compartments that overlap with class-II positive vesicles, and this localization depends on the Invariant chain (Ii), suggesting a shared control of antigen peptides and costimulatory molecules transport to the synaptic area (23, 24). Similarly, ICAM-1 is recycled at the interaction zone through recycling compartments where it intersects MHC class-II molecules (25). ICAM-1 mobility on the DCs membrane is a critical factor controlling activation of T cells. Besides being the main adhesive force to stabilize the interaction, LFA-1 is important to modulate signaling from the TCR. Binding of LFA-1 to its ligand, in fact, enhances signaling for key TCR signal transduction molecules, such as PI3K, PLC-γ, MAP kinases, and SLP-76, reinforcing IL-2 production and T-cell proliferation (26–29). A recent study revealed that cytoskeletal remodeling during DC maturation causes a decrease in the lateral mobility of ICAM-1 in the membrane. Laterally confined ICAM-1 imposes forces on the interacting LFA-1 that promote ligand-dependent activation and increase T-cell priming (30).
Signaling Molecules
Underneath the plasma membrane and concomitantly to redistribution of membrane receptors, several events take place in the cytoplasm of DCs, suggesting that the DC-IS is an active signaling zone. Spinophilin is a PDZ domain-containing protein that is highly expressed in the dendritic spines of neurons where it controls interactions with the underlying cytoskeleton and with membrane trafficking proteins. Spinophilin was shown to be expressed in DCs and to cluster at the DC membrane in antigen-specific conjugates, and its depletion caused a strong inhibition of T-cell priming (31). This study did not address the consequences of spinophilin loss in terms of cytoskeletal proteins distribution and overall cell symmetry. It is likely that spinophilin participates in the network of PDZ domain-containing proteins regulating cell polarity, indicating the importance of asymmetric distribution of functional subdomains for antigen presentation.
Durable presentation of antigens to T cells, either via long-lasting contacts or cumulative interactions, is essential for efficient priming of T cells in lymph nodes (1). The life span of DCs is short, and it is therefore essential to control their fitness when they come to accomplish their ultimate task in the lymph node. Intriguingly, it was demonstrated that synapse formation provides antiapoptotic signals to DCs. Formation of the synapse induces recruitment and activation of the antiapoptotic protein Akt at the DC-IS followed by activation of prosurvival signals, including inhibition of FOXO and activation of NF-κB [(32) and reviewed in Ref. (33)]. Given the extensive remodeling of actin and microtubule cytoskeleton in DCs engaged in synapses (see below), it is likely to expect that other large signaling complexes become selectively recruited and activated at the site of interaction. However, the membrane receptors primarily involved, the composition of these signaling platforms, and the hierarchy of their assembly still remains to be elucidated.
The Actin Cytoskeleton
All synaptic events, from intercellular contact formation and adhesion to signal transduction, are orchestrated by the actin and microtubules cytoskeleton, their upstream regulators and downstream effectors in a continuous feedback loop.
Soon after the discovery of the supramolecular activation cluster in T cells, researchers discovered that DCs actively participate in synapse formation by polarizing the actin cytoskeleton, unlike B cells. The causal link was strong as F-actin pharmacological inhibitors inhibited the T-cell priming capacity of DCs (34). Actin remodeling is controlled by a set of proteins that promote actin nucleation and polymerization, in turn controlled by Rho GTPases. Studies to decipher the molecular pathways involved in the control of actin remodeling in DCs showed, at first, an involvement of the actin-bundling protein, fascin. This protein is highly expressed selectively in DCs, upregulated during maturation, and necessary for DCs full antigen-presenting function (35). Few years later, we found that one essential mediators of actin remodeling during synapse formation in DCs is the small GTPases, Rac. Genetic deletion of Rac1 and 2 rendered DCs unable to establish productive interaction with T cells, leading to extremely inefficient T-cell activation (36). Two main classes of actin nucleating factors, formins and WASp family, involved, respectively, in the formation of long actin polymer elongation and branched actin networks were both shown to play a role in DCs migration and interaction with T cells. Deletion of mDia, a formin family member, inhibits DCs migration to LNs and decreases the capacity to establish long contacts with T cells and to induce their activation (37). WASp, expressed selectively in hematopoietic cells and target of mutations in Wiskott–Aldrich syndrome (38), controls various DCs functions, including the capacity to form stable interactions with T cells and the stability and structure of the synapse. In vivo two-photon microscopy analysis in the 3D environment of the lymph node showed decreased contact time between WASp null DCs and CD8+ T cells. Closer inspection in vitro revealed a defect in accomplishing the complete cycle of movements required to establish a full contact. As a consequence, WASp null conventional DCs often undergo repetitive contacts of short duration, resulting in decreased T-cell proliferation and IFN-γ production (39). Similarly, CD4 T cells are inefficiently primed by WASp null DCs in vivo and in vitro (40). In this last work, Ca2+ signaling and clustering of signaling molecules were shown to be decreased in T cells interacting with mutant cells, indicating that failure to sustain proximal events represents an important contribution to the overall immunodeficiency in WAS. Recently, a novel cell-free system to mimic the T-cell surface was used to address the role of WASp at the DC-IS with increased resolution. The results show that a spatially organized structure containing MHC-II, ICAM-1, and actin forms at the DC-IS, and it is stabilized in a WASp- and Arp2/3-dependent manner (41). It would be interesting to investigate whether WASp is involved in controlling the lateral mobility of ICAM-1, a parameter recently emerged to be critically during antigen presentation by DCs (30). Interestingly, the function of WASp goes beyond the control of motility and signaling at the IS as it also acts in tuning innate responses by toll-like receptors in plasmacytoid DCs (42).
The role of actin and actin regulatory proteins involved in synapse formation in DCs is summarized in Figure 1. Despite these insights, the triggers and the players of actin remodeling during synapse formation in DCs are not yet understood in sufficient details. As shown in a recent elegant study, DCs switch between different actin nucleating machineries during the process of migration and antigen uptake (43). What type of actin machinery prevails during antigen presentation at the IS and how the switch to this third DCs’ specific function is achieved remains an interesting open question.
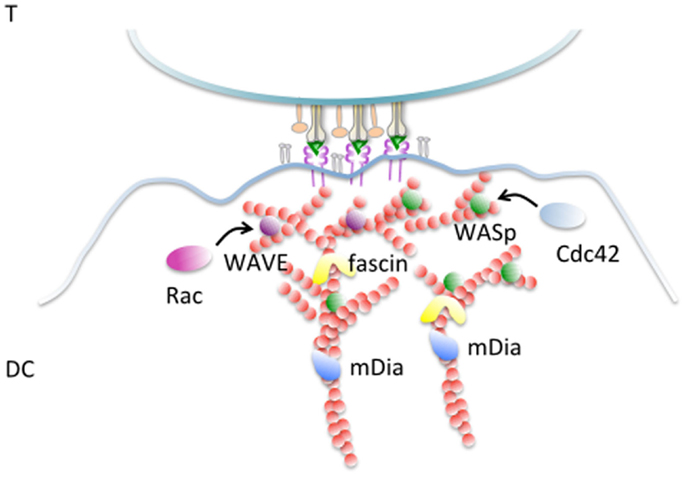
Figure 1. Actin remodeling at the DC-IS. F-actin filaments become polarized and cluster enriched at the junction with an antigen-specific T cells. Fascin, an actin-bundling protein implicated in extension of membrane protrusion and development of dendrites is enriched in this area and controls the capacity of DCs to activate T cells. The Rho GTPase Rac is required for synapse formation and T-cell activation, likely via WAVE-mediated actin remodeling. WASp is a further activator of the Arp2/3 complex that is essential to support the antigen-presenting activities of DCs by promoting actin branching, thereby stabilizing the synaptic structure. WASp activation is mediated by cdc42 that is also found enriched at the DC-IS. The formin family mDia, involved in elongation of long actin filaments is important to establish and stabilize DC–T contacts and to support T-cell activation.
Polarity and Secretion on the APC Side of the Immune Synapse
Polarity is a highly conserved mechanism to spatially organize different functions within a cell, common to many processes, including the regulation of immune cells functions. The importance of polarity is well characterized in T cells where centrosome polarization in CTLs and T-helper cells is a fairly well-understood mechanism to optimize release of cytotoxic granules and cytokines, respectively (44–46). In B cells, centrosome polarization at the synapse is a mechanism to orient the secretion of lysosomes and to promote the extraction of immobilized BCR ligands (47). B cells were shown to polarize also in the context of a B–T cell synapse with both the centrosome and the Golgi apparatus reoriented toward the interaction zone (48).
Many evidences exist that cell polarity and polarized membrane trafficking establishes also in DCs upon formation of antigen-specific conjugates. For instance, tubules of MHC class-II emanating in the direction of the interacting T cell indicate that remodeling of membrane trafficking is induced by antigen-specific contacts (17). The ability to polarize the tubules is acquired upon signals from toll-like receptors and depends on microtubules integrity (49, 50). DCs contacting NK cells were shown to polarize cytokines in the synaptic cleft, further suggesting directional vesicular transport (51, 52). Indeed, it was formally demonstrated that DC, following induction by a microbial maturation stimuli, polarizes the microtubule-organizing center (MTOC) toward the DC–T interface (53). This process depends on cdc42 as genetic deletion of this key polarity protein hampers the ability of DCs to reposition the centrosome underneath the synaptic membrane. Cdc42 is indeed highly enriched at the contact zone in DCs indicating its possible role as a WASp activator as well. Vesicles carrying newly synthesized interleukin 12, a key mediator of T-cell priming, clustered around the Golgi and were rapidly repositioned at the DC-IS, resulting in polarized secretion and local induction of IL-12-dependent signaling in T cells (53) (Figure 2). Thus, similarly to what have been shown in T cells, polarized transport of soluble mediators is a fundamental process that contributes to the antigen-presenting properties of DCs as ablation of cdc42-mediated polarization inhibits T-cell proliferation (53). The notion that the so-called signal 3 (proinflammatory cytokines) can be locally coupled to antigenic and costimulatory signals, and it can be delivered in cis may have important consequences for the fate imprinted to different T cells that interact sequentially with the same APC.
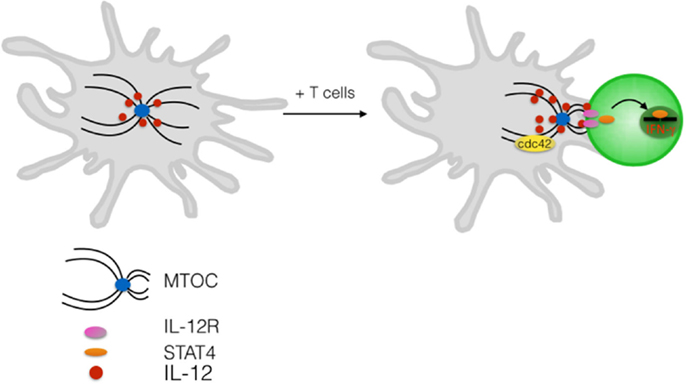
Figure 2. Polarized delivery of cytokine at the DC-IS. Upon contact with antigen-specific T cells DCs reorient the centrosome toward the interacting T cell. Polarization depends on the Rho GTPase cdc42. Newly synthesized IL-12 is contained in intracellular vesicles that cluster in the Golgi area and are readily redistribute at the IS upon antigen-specific contact formation. Release of IL-12 in the synaptic cleft induces activation of STAT4 and triggering of IFN-γ in the interacting T cell.
These findings raise the question of the link between microtubules and membrane trafficking proteins that support the directional transport of mediators en route to exocytosis. In macrophages and in T cells, members of the SNARE and Rab family were shown to control selectively directional secretion of various cytokines (44, 54). In DCs, we have recently discovered that the SNARE VAMP-7 plays a key role in the intracellular trafficking and secretion of newly synthesized IL-12. VAMP-7 not only controls the multidirectional transport of the cytokine toward the plasma membrane but, most importantly, it is absolutely required for clustering and secretion of IL-12 at the DC-IS (55). These findings reveal an usual transport route via late endosomes and represent the first insight into the molecular mechanism that orchestrate trafficking and secretion of soluble factors in DCs.
Conclusion
Both synaptic partners contribute to the successful outcome of the intercellular interaction between an activated DCs and a naive T cell, i.e., T-cell activation.
It is becoming obvious that a complex cross-talk between the two cells exists and that understanding T-cell activation cannot do without a deep understanding of the DCs synapse. Further efforts are envisaged in at least two directions. The first is the systematic dissection of the receptors and the downstream effectors operating sequentially in DCs and their functional significance. This goal will be achieved, thanks to the recent development of cell-free system to reconstitute the T-cell membrane. Second, to increase physiological relevance, future studies should take into account the complexity of the DCs system, which is composed of various DCs subsets that are most likely equipped with different tools to transmit information to their partners.
Author Contributions
The author confirms being the sole contributor of this work and approved it for publication.
Conflict of Interest Statement
The author declares that the research was conducted in the absence of any commercial or financial relationships that could be construed as a potential conflict of interest.
Acknowledgments
The author wishes to thank Italian Telethon and Associazione Italiana Ricerca Cancro grants IG9076 to the PI for the support on the work referenced in this review.
References
1. Moreau HD, Bousso P. Visualizing how T cells collect activation signals in vivo. Curr Opin Immunol (2014) 26:56–62. doi: 10.1016/j.coi.2013.10.013
2. Marangoni F, Murooka TT, Manzo T, Kim EY, Carrizosa E, Elpek NM, et al. The transcription factor NFAT exhibits signal memory during serial T cell interactions with antigen-presenting cells. Immunity (2013) 38:237–49. doi:10.1016/j.immuni.2012.09.012
3. Lodygin D, Odoardi F, Schlager C, Korner H, Kitz A, Nosov M, et al. A combination of fluorescent NFAT and H2B sensors uncovers dynamics of T cell activation in real time during CNS autoimmunity. Nat Med (2013) 19:784–90. doi:10.1038/nm.3182
4. Merad M, Sathe P, Helft J, Miller J, Mortha A. The dendritic cell lineage: ontogeny and function of dendritic cells and their subsets in the steady state and the inflamed setting. Annu Rev Immunol (2013) 31:563–604. doi:10.1146/annurev-immunol-020711-074950
5. Dustin ML, Colman DR. Neural and immunological synaptic relations. Science (2002) 298:785–9. doi:10.1126/science.1076386
6. Eun SY, O’Connor BP, Wong AW, van Deventer HW, Taxman DJ, Reed W, et al. Cutting edge: rho activation and actin polarization are dependent on plexin-A1 in dendritic cells. J Immunol (2006) 177:4271–5. doi:10.4049/jimmunol.177.7.4271
7. Wong AW, Brickey WJ, Taxman DJ, van Deventer HW, Reed W, Gao JX, et al. CIITA-regulated plexin-A1 affects T-cell-dendritic cell interactions. Nat Immunol (2003) 4:891–8. doi:10.1038/ni960
8. Sarris M, Andersen KG, Randow F, Mayr L, Betz AG. Neuropilin-1 expression on regulatory T cells enhances their interactions with dendritic cells during antigen recognition. Immunity (2008) 28:402–13. doi:10.1016/j.immuni.2008.01.012
9. Tordjman R, Lepelletier Y, Lemarchandel V, Cambot M, Gaulard P, Hermine O, et al. A neuronal receptor, neuropilin-1, is essential for the initiation of the primary immune response. Nat Immunol (2002) 3:477–82. doi:10.1038/ni789
10. Watts C, Zaru R, Prescott AR, Wallin RP, West MA. Proximal effects of toll-like receptor activation in dendritic cells. Curr Opin Immunol (2007) 19:73–8. doi:10.1016/j.coi.2006.11.014
11. West MA, Wallin RP, Matthews SP, Svensson HG, Zaru R, Ljunggren HG, et al. Enhanced dendritic cell antigen capture via toll-like receptor-induced actin remodeling. Science (2004) 305:1153–7. doi:10.1126/science.1099153
12. Benvenuti F, Lagaudriere-Gesbert C, Grandjean I, Jancic C, Hivroz C, Trautmann A, et al. Dendritic cell maturation controls adhesion, synapse formation, and the duration of the interactions with naive T lymphocytes. J Immunol (2004) 172:292–301. doi:10.4049/jimmunol.172.1.292
13. Miller MJ, Hejazi AS, Wei SH, Cahalan MD, Parker I. T cell repertoire scanning is promoted by dynamic dendritic cell behavior and random T cell motility in the lymph node. Proc Natl Acad Sci U S A (2004) 101:998–1003. doi:10.1073/pnas.0306407101
14. Fisher PJ, Bulur PA, Vuk-Pavlovic S, Prendergast FG, Dietz AB. Dendritic cell microvilli: a novel membrane structure associated with the multifocal synapse and T-cell clustering. Blood (2008) 112:5037–45. doi:10.1182/blood-2008-04-149526
15. Brossard C, Feuillet V, Schmitt A, Randriamampita C, Romao M, Raposo G, et al. Multifocal structure of the T cell – dendritic cell synapse. Eur J Immunol (2005) 35:1741–53. doi:10.1002/eji.200425857
16. de la Fuente H, Mittelbrunn M, Sanchez-Martin L, Vicente-Manzanares M, Lamana A, Pardi R, et al. Synaptic clusters of MHC class II molecules induced on DCs by adhesion molecule-mediated initial T-cell scanning. Mol Biol Cell (2005) 16:3314–22. doi:10.1091/mbc.E05-01-0005
17. Boes M, Cerny J, Massol R, Op den Brouw M, Kirchhausen T, Chen J, et al. T-cell engagement of dendritic cells rapidly rearranges MHC class II transport. Nature (2002) 418:983–8. doi:10.1038/nature01004
18. Compeer EB, Flinsenberg TW, Boon L, Hoekstra ME, Boes M. Tubulation of endosomal structures in human dendritic cells by toll-like receptor ligation and lymphocyte contact accompanies antigen cross-presentation. J Biol Chem (2014) 289:520–8. doi:10.1074/jbc.M113.511147
19. Boisvert J, Edmondson S, Krummel MF. Immunological synapse formation licenses CD40-CD40L accumulations at T-APC contact sites. J Immunol (2004) 173:3647–52. doi:10.4049/jimmunol.173.6.3647
20. Foster N, Turnbull EL, Macpherson G. Migrating lymph dendritic cells contain intracellular CD40 that is mobilized to the immunological synapse during interactions with antigen-specific T lymphocytes. J Immunol (2012) 189:5632–7. doi:10.4049/jimmunol.1202010
21. Miro F, Nobile C, Blanchard N, Lind M, Filipe-Santos O, Fieschi C, et al. T cell-dependent activation of dendritic cells requires IL-12 and IFN-gamma signaling in T cells. J Immunol (2006) 177:3625–34. doi:10.4049/jimmunol.177.6.3625
22. Hivroz C, Chemin K, Tourret M, Bohineust A. Crosstalk between T lymphocytes and dendritic cells. Crit Rev Immunol (2012) 32:139–55. doi:10.1615/CritRevImmunol.v32.i2.30
23. Keller AM, Groothuis TA, Veraar EA, Marsman M, Maillette de Buy Wenniger L, Janssen H, et al. Costimulatory ligand CD70 is delivered to the immunological synapse by shared intracellular trafficking with MHC class II molecules. Proc Natl Acad Sci U S A (2007) 104:5989–94. doi:10.1073/pnas.0700946104
24. Zwart W, Peperzak V, de Vries E, Keller AM, van der Horst G, Veraar EA, et al. The invariant chain transports TNF family member CD70 to MHC class II compartments in dendritic cells. J Cell Sci (2010) 123:3817–27. doi:10.1242/jcs.068510
25. Jo JH, Kwon MS, Choi HO, Oh HM, Kim HJ, Jun CD. Recycling and LFA-1-dependent trafficking of ICAM-1 to the immunological synapse. J Cell Biochem (2010) 111:1125–37. doi:10.1002/jcb.22798
26. Li D, Molldrem JJ, Ma Q. LFA-1 regulates CD8+ T cell activation via T cell receptor-mediated and LFA-1-mediated Erk1/2 signal pathways. J Biol Chem (2009) 284:21001–10. doi:10.1074/jbc.M109.002865
27. Ni HT, Deeths MJ, Mescher MF. LFA-1-mediated costimulation of CD8+ T cell proliferation requires phosphatidylinositol 3-kinase activity. J Immunol (2001) 166:6523–9. doi:10.4049/jimmunol.166.11.6523
28. Perez OD, Mitchell D, Jager GC, South S, Murriel C, McBride J, et al. Leukocyte functional antigen 1 lowers T cell activation thresholds and signaling through cytohesin-1 and Jun-activating binding protein 1. Nat Immunol (2003) 4:1083–92. doi:10.1038/ni984
29. Wang H, Wei B, Bismuth G, Rudd CE. SLP-76-ADAP adaptor module regulates LFA-1 mediated costimulation and T cell motility. Proc Natl Acad Sci U S A (2009) 106:12436–41. doi:10.1073/pnas.0900510106
30. Comrie WA, Li S, Boyle S, Burkhardt JK. The dendritic cell cytoskeleton promotes T cell adhesion and activation by constraining ICAM-1 mobility. J Cell Biol (2015) 208:457–73. doi:10.1083/jcb.201406120
31. Bloom O, Unternaehrer JJ, Jiang A, Shin JS, Delamarre L, Allen P, et al. Spinophilin participates in information transfer at immunological synapses. J Cell Biol (2008) 181:203–11. doi:10.1083/jcb.200711149
32. Riol-Blanco L, Delgado-Martin C, Sanchez-Sanchez N, Alonso CL, Gutierrez-Lopez MD, Del Hoyo GM, et al. Immunological synapse formation inhibits, via NF-kappaB and FOXO1, the apoptosis of dendritic cells. Nat Immunol (2009) 10:753–60. doi:10.1038/ni.1750
33. Rodriguez-Fernandez JL, Riol-Blanco L, Delgado-Martin C. What is the function of the dendritic cell side of the immunological synapse? Sci Signal (2010) 3:re2. doi:10.1126/scisignal.3105re2
34. Al-Alwan MM, Rowden G, Lee TD, West KA. The dendritic cell cytoskeleton is critical for the formation of the immunological synapse. J Immunol (2001) 166:1452–6. doi:10.4049/jimmunol.166.3.1452
35. Al-Alwan MM, Rowden G, Lee TD, West KA. Fascin is involved in the antigen presentation activity of mature dendritic cells. J Immunol (2001) 166:338–45. doi:10.4049/jimmunol.166.1.338
36. Benvenuti F, Hugues S, Walmsley M, Ruf S, Fetler L, Popoff M, et al. Requirement of Rac1 and Rac2 expression by mature dendritic cells for T cell priming. Science (2004) 305:1150–3. doi:10.1126/science.1099159
37. Tanizaki H, Egawa G, Inaba K, Honda T, Nakajima S, Moniaga CS, et al. Rho-mDia1 pathway is required for adhesion, migration, and T-cell stimulation in dendritic cells. Blood (2010) 116:5875–84. doi:10.1182/blood-2010-01-264150
38. Thrasher AJ, Burns SO. WASP: a key immunological multitasker. Nat Rev Immunol (2010) 10:182–92. doi:10.1038/nri2724
39. Pulecio J, Tagliani E, Scholer A, Prete F, Fetler L, Burrone OR, et al. Expression of Wiskott-Aldrich syndrome protein in dendritic cells regulates synapse formation and activation of naive CD8+ T cells. J Immunol (2008) 181:1135–42. doi:10.4049/jimmunol.181.2.1135
40. Bouma G, Mendoza-Naranjo A, Blundell MP, de Falco E, Parsley KL, Burns SO, et al. Cytoskeletal remodeling mediated by WASp in dendritic cells is necessary for normal immune synapse formation and T-cell priming. Blood (2011) 118:2492–501. doi:10.1182/blood-2011-03-340265
41. Malinova D, Fritzsche M, Nowosad CR, Armer H, Munro PM, Blundell MP, et al. WASp-dependent actin cytoskeleton stability at the dendritic cell immunological synapse is required for extensive, functional T cell contacts. J Leukoc Biol (2015). doi:10.1189/jlb.2A0215-050RR
42. Prete F, Catucci M, Labrada M, Gobessi S, Castiello MC, Bonomi E, et al. Wiskott-Aldrich syndrome protein-mediated actin dynamics control type-I interferon production in plasmacytoid dendritic cells. J Exp Med (2013) 210:355–74. doi:10.1084/jem.20120363
43. Vargas P, Maiuri P, Bretou M, Saez PJ, Pierobon P, Maurin M, et al. Innate control of actin nucleation determines two distinct migration behaviours in dendritic cells. Nat Cell Biol (2016) 18:43–53. doi:10.1038/ncb3284
44. Huse M, Lillemeier BF, Kuhns MS, Chen DS, Davis MM. T cells use two directionally distinct pathways for cytokine secretion. Nat Immunol (2006) 7:247–55. doi:10.1038/ni1304
45. Jenkins MR, Griffiths GM. The synapse and cytolytic machinery of cytotoxic T cells. Curr Opin Immunol (2010) 22:308–13. doi:10.1016/j.coi.2010.02.008
46. Martin-Cofreces NB, Robles-Valero J, Cabrero JR, Mittelbrunn M, Gordon-Alonso M, Sung CH, et al. MTOC translocation modulates IS formation and controls sustained T cell signaling. J Cell Biol (2008) 182:951–62. doi:10.1083/jcb.200801014
47. Yuseff MI, Reversat A, Lankar D, Diaz J, Fanget I, Pierobon P, et al. Polarized secretion of lysosomes at the B cell synapse couples antigen extraction to processing and presentation. Immunity (2011) 35:361–74. doi:10.1016/j.immuni.2011.07.008
48. Duchez S, Rodrigues M, Bertrand F, Valitutti S. Reciprocal polarization of T and B cells at the immunological synapse. J Immunol (2011) 187:4571–80. doi:10.4049/jimmunol.1100600
49. Boes M, Bertho N, Cerny J, Op den Brouw M, Kirchhausen T, Ploegh H. T cells induce extended class II MHC compartments in dendritic cells in a toll-like receptor-dependent manner. J Immunol (2003) 171:4081–8. doi:10.4049/jimmunol.171.8.4081
50. Vyas JM, Kim YM, Artavanis-Tsakonas K, Love JC, Van der Veen AG, Ploegh HL. Tubulation of class II MHC compartments is microtubule dependent and involves multiple endolysosomal membrane proteins in primary dendritic cells. J Immunol (2007) 178:7199–210. doi:10.4049/jimmunol.178.11.7199
51. Borg C, Jalil A, Laderach D, Maruyama K, Wakasugi H, Charrier S, et al. NK cell activation by dendritic cells (DCs) requires the formation of a synapse leading to IL-12 polarization in DCs. Blood (2004) 104:3267–75. doi:10.1182/blood-2004-01-0380
52. Semino C, Angelini G, Poggi A, Rubartelli A. NK/iDC interaction results in IL-18 secretion by DCs at the synaptic cleft followed by NK cell activation and release of the DC maturation factor HMGB1. Blood (2005) 106:609–16. doi:10.1182/blood-2004-10-3906
53. Pulecio J, Petrovic J, Prete F, Chiaruttini G, Lennon-Dumenil AM, Desdouets C, et al. Cdc42-mediated MTOC polarization in dendritic cells controls targeted delivery of cytokines at the immune synapse. J Exp Med (2010) 207:2719–32. doi:10.1084/jem.20100007
54. Stow JL, Manderson AP, Murray RZ. SNAREing immunity: the role of SNAREs in the immune system. Nat Rev Immunol (2006) 6:919–29. doi:10.1038/nri1980
Keywords: immune synapse, dendritic cells, actin cytoskeleton, polarized secretion, antigen presentation
Citation: Benvenuti F (2016) The Dendritic Cell Synapse: A Life Dedicated to T Cell Activation. Front. Immunol. 7:70. doi: 10.3389/fimmu.2016.00070
Received: 17 December 2015; Accepted: 15 February 2016;
Published: 07 March 2016
Edited by:
Andres Alcover, Institut Pasteur, FranceReviewed by:
José Luis Rodríguez-Fernández, Consejo Superior de Investigaciones Científicas, SpainAnne Hosmalin, Cochin Institute, France
Copyright: © 2016 Benvenuti. This is an open-access article distributed under the terms of the Creative Commons Attribution License (CC BY). The use, distribution or reproduction in other forums is permitted, provided the original author(s) or licensor are credited and that the original publication in this journal is cited, in accordance with accepted academic practice. No use, distribution or reproduction is permitted which does not comply with these terms.
*Correspondence: Federica Benvenuti, YmVudmVudXRAaWNnZWIub3Jn