- 1Faculty of Medicine of Saint-Etienne, University of Lyon, Saint-Etienne, France
- 2Institut National de la Transfusion Sanguine, Paris, France
- 3Hôpital du Sacré-Coeur, Beirut, Lebanon
- 4Centre de Transfusion Sanguine, Sousse, Tunisia
- 5Faculty of Pharmacy, University of Monastir, Monastir, Tunisia
- 6Etablissement Français du Sang Rhône-Alpes-Auvergne, Saint-Etienne, France
Transfusion of blood cell components is frequent in the therapeutic arsenal; it is globally safe or even very safe. At present, residual clinical manifestations are principally inflammatory in nature. If some rare clinical hazards manifest as acute inflammation symptoms of various origin, most of them linked with conflicting and undesirable biological material accompanying the therapeutic component (infectious pathogen, pathogenic antibody, unwanted antigen, or allergen), the general feature is subtler and less visible, and essentially consists of alloimmunization or febrile non-hemolytic transfusion reaction. The present essay aims to present updates in hematology and immunology that help understand how, when, and why subclinical inflammation underlies alloimmunization and circumstances characteristic of red blood cells and – even more frequently – platelets that contribute inflammatory mediators. Modern transfusion medicine makes sustained efforts to limit such inflammatory hazards; efforts can be successful only if one has a clear view of each element’s role.
Introduction
Historically, inflammation was viewed as the compendium of all four stigmas: “rubor, calor, dolor, and tumor”; this concept fits well with the theory of humors; bloodletting – and surrogates (e.g., leeches and suction cups) – have long been applied to treat, if not cure, inflammation symptoms. As a matter of fact, iron depletion caused by bloodletting happened to alter bacterial growth and ameliorate certain disease conditions, as already observed by Tissot in 1761 (1). The Hippocratic theory of humors was probably the first to introduce the relationship between blood and inflammation, though using wrong descriptors. In its earliest days, transfusion was clearly associated with acute inflammation, though the connection was not acknowledged as such: indeed, the very first reported serious adverse events (SAEs) of “modern” transfusion in the early twentieth century were dual in nature: first, immune-hematological [i.e., antigen–antibody (ABO)] conflicts, and second, blood-borne and blood-transmitted infections, such as syphilis and malaria (2). Both conditions – presenting as very severe – were later on acknowledged as being dominated by cytokine storms and standing for acute inflammatory reactions (often lethal) (3, 4).
The concept of inflammation has been largely revisited by modern internal medicine; series of autoimmune and auto-inflammatory diseases have thus been acknowledged. No organ-specific disorder is actually beyond the scope of the large clinical inflammation spectrum, since a number of neurological disorders (5) – as well as many cardiovascular lesions especially the atheroma plaque deposit (6) – are inflammation stigmas. The causality of inflammation in organ-specific lesions is being questioned, but combinations of genetic predisposition, lifelong hygienic habits, other environmental factors, and infectious triggers are commonly evoked. For decades now, clinical inflammation has not been restricted to acute Hippocratic symptoms and is acknowledged to present as more subtle symptoms of varying degrees.
We believe two major achievements have helped reconsider clinical inflammation as it may apply to transfusion medicine and cell, tissue, and organ transplantation. Neither was intended to apply to this discipline; however, the first is the (re)discovery of the danger signal theory, as proposed by P. Matzinger at the NIAID, NIH, in the 1990s, after its seminal conceptualization by E. Metchnikoff at the Pasteur Institute 100 years earlier. This discovery is basic to immunology and helps reframe the reading of immunology (7). The second is the conceptualization of the microbiota’s role in immunity – initially presented as governing what Ph Sansonetti (at the Pasteur Institute in Paris) called “war and peace” at the mucosal surfaces. This concept helped show that inflammation spans the whole spectrum, from physiology to pathology (8–10). It has since been suggested that healing (e.g., of tissue attrition or organ lesions) is the ultimate step of inflammation (11, 12).
Transfusion and Inflammation: From Bedside to Bench
From the bedside, one can consider two periods in relation to transfusion-related hazards, especially inflammation. The initial period concerns acute symptoms of SAEs: inflammation is observed among other symptoms such as shock. Those accidents were principally reported with reference to their major cause(s): the ABO conflict, transfusion-transmitted bacterial, viral, or parasitic infections, and allergy. In 1983, a novel cause of transfusion-transmitted SAE was described: transfusion-related acute lung injury (TRALI) (13–15). Interestingly, this SAE is ascribed to a dual cause: an Ag/Ab conflict – within the human leukocyte antigen (HLA) or, more rarely but more severely, the human neutrophil antigen (HNA) systems – and an inflammatory layer: sepsis, stress, etc. Besides conflicting Abs (when identified, i.e., in two out of every three cases on average), the principal actors are leukocytes recruited or residing in lung capillaries. The TRALI concept prompted a reinvestigation of SAEs in transfusion and acknowledgment of serious inflammatory cases. This is also true for allergy: though one cannot exclude the possibility of pathogenic IgE transfer, it is rather felt that such an occurrence cannot account for one-third of adverse events (AEs) varying in severity (16, 17). Transfusion allergy is, in general, considered to present like allergy, though it is not believed to have a link with atopy or involve allergens or Abs specific to allergens. It is recognized as one of the most frequent inflammatory consequences of transfusion (18).
In summary, despite this is over-simplistic, one may acknowledge that inflammation symptoms manifested by a transfused patient and in relation with the transfusion process has two principal causes: it is either due to the transfer of pathogenic material collected from the donor or it is due to a conflict between high affinity receptors found on the recipients’ cells or plasma molecules and ligands brought by the transfused component.
The majority of AEs in patients receiving blood (recipients) manifest either allergy or febrile non-hemolytic transfusion reactions (FNHTRs), both being clearly inflammatory conditions (19). Leukocytes transferred with blood were ascribed to as the principal causes of TT inflammation. Systematic “leucoreduction” – often inappropriately, but nevertheless officially, termed “leucodepletion” – was proposed at the start of the millenium by many countries or blood transfusion systems. However, leucoreduction has neither been become recommended nor a mandatory practice for mitigating inflammatory responses but is instead used to limit transfusion-transmitted viral risks as many “serious transfusion-associated viruses” are intracellular. Leucoreduction was principally aimed to reduce the risk of transmitting the Creutzfeldt–Jakob prion (20). Veterans of transfusion medicine very well recall the time when every single transfused patient was “shaking and heating,” manifesting common symptoms that were subsequent to the therapy and introduced as such to patients (when patients happened to receive information). Pre-storage leucoreduction was then acknowledged to have largely improved comfort and safety in patients, suggesting a deleterious role for leukocytes (21, 22). When leucoreduction is performed post-storage (e.g., at the bedside, prior to the infusion of the blood component), inflammatory manifestation is intermediate, largely suggesting that not only leukocytes but also their secreted content play a role in the transfusion inflammation pathophysiology (23).
However, as the transfused patient profile changed, more and more recipients benefited from platelet components (PCs). This major change took place more or less at the same time as the implementation of systematic hemovigilance, and it soon became obvious that PCs – though representing no more than 10% of issued blood components – provide between one-quarter to one-half of reported AEs (24). This means that leukocytes were not the only cells associated with transfusion-associated inflammation.
Another population of patients benefiting from frequent transfusion episodes, sickle-cell disease patients, led to an important discovery: first, they manifest complex hemolytic reactions that involve activated complement and present as essentially inflammatory (25) and second, they are subjected to the most frequent rate of alloimmunization among tracked cohorts of transfused patients (26). This prompted specialists to also examine the inflammatory potential of stored erythrocytes.
The Major Identified Causes of Transfusion-Associated Inflammation
There is good evidence in favor of an undesirable role for residual leukocytes in transfused patients; blood banks can get rid of such residual leukocytes with a high degree of efficacy by using filtration methods. Leucoreduction is highly recommended by the European Community and the American Association of Blood Banks with a target of no more than 106 residual leukocytes per blood component after filtration (27, 28); most pre-storage methods allow scores of leucoreduction ranging between 2 and 5 × 105 residual leukocytes per component (29). However, clinical observations suggest that other constituents of pre-stored and leucoreduced cellular blood components still lead to some inflammatory manifestations in patients.
To simplify and summarize, pro-inflammatory factors in labile blood components fall into one of the following four categories: (1) infectious pathogens transmitted by blood that cause bacterial sepsis, acute or chronic viral infection, or acute parasitic infection (and often hemolysis); (2) pathogenic, undesirable, Abs (causing hemolysis when encountering target Ags, especially when capable of binding complement; causing TRALI, depending on circumstances or predisposition; causing Reagin-mediated allergy; and causing a number of non-hemolytic situations, now ascribed to FNHTRs); (3) leukocytes and their content, and especially their high loads of pro-inflammatory cytokines, chemokines, and the like, collectively termed biological response modifiers (BRMs); and (4) (pro)inflammatory material linked to platelet and erythrocyte pathophysiology, especially when cellular blood components [PCs and packed red blood cell components (pRBCCs)] are stored over time and undergo so-called storage lesions, which consist of extracellular vesicle emission and the freeing of membrane-bound molecules and intracellular content, either iron (erythrocytes) or BRMs (platelets). It is not unusual that extracellular vesicles are called microparticles.
The first two categories are chiefly inflammatory, involving two principal mechanisms: first, the triggering of a cytokine storm, with broad consequences for all systems, exposing the patient to multivisceral failure and severe central neurologic disorders. Second, if erythrocytes are ultimate targets of the Ab or infectious pathogens, there is acute hemolysis with obvious consequences. This essay will not further discuss such cases. Neither will it discuss the specific case of bacterial contamination of PCs, largely related to the storage temperature of 22 ± 2°C; 18.5 severe cases per million PCs delivered are recorded annually according to the latest French hemovigilance records; and one such case happens to be lethal, on average (30).
The latter two categories reveal that inflammation is not only the result of substantial levels of BRMs secreted by leukocytes, platelets, or lysed erythrocytes but also of products secreted as a consequence of cell–cell encounters after the blood component has been transfused. Cell–cell interactions occur mainly between (i) donor transfused cells and recipient circulating cells and (ii) donor transfused cells and recipient vascular endothelium cells. Transfusion is a dynamic process, but it is often regarded as the passive infusion of therapeutic components (31). This view is misleading and a source of errors for the interpretation of transfusion-associated inflammation.
Models of Transfusion-Associated Inflammation Hit
This section will address three main issues: (1) how transfusion can act as a stress for the recipient, subsequently triggering an immune defense; (2) how blood components can present with varying degrees of stress signals, accompanied by pathogenic storage lesions; and (3) how the stage is set for a recipient’s adaptive immune response to donor cell Ags. These three points parallel three major aspects of the transfusion process or chain: donor-linked characteristics, additional pathogenic steps during blood component production, and recipient-linked characteristics.
Transfusion as a Stress and Donor-Linked Characteristics Account for Recipients’ Inflammatory Symptoms
Transfusion is an unnatural process in the sense that the exchange of body parts between individuals – other than mothers and their embryos or fetuses – is not part of the human evolutionary program. Each individual’s blood has potentially unique biological characteristics. Thus, when foreign cellular material, is tentatively grafted into a recipient, the latter identifies it as foreign and potentially dangerous, even when it has a therapeutic purpose. Indeed, many studies have shown that platelets express a large variety of pathogen sensors, promptly engaged by several kinds of the so-called pathogen-associated molecular pattern (molecules) or PAMPs (if stresses are infectious in nature) or damage-associated molecular pattern (molecules) or DAMPs (if stresses are internal, such as Abs). This has been principally found relative to platelets (32, 33), and similar findings have been reported for erythrocytes (34). Furthermore, donor platelets express HLA class I Ags that differ in general from those of recipients. Donor cells are thus likely to be sensed as foreign by recipients’ circulating and vessel-lining leukocytes, which are prone to signaling this through a pro-inflammatory response, or by vessel endothelial cells. Experimental data suggest that endothelial cells can also signal the detection of foreign material by mounting a pro-inflammatory response (35–38). In general, though it is still difficult to link with certainty a host’s innate inflammatory response with unmanipulated donor cells, the danger theory of innate immunity would largely predict it in transfusion.
Recent data offer newer evidence supporting the hypothesis. First, a large Canadian clinical trial recently reported that age and sex of donors influenced the outcome of transfusion in recipients, more than any other factor (e.g., age of blood or pathology) (39, 40). Although there is now good evidence that there are differences between males and females in pathology and in particular in immune responses to infection or vaccines and inflammation processes, the gender issue has not been specifically addressed satisfactorily in transfusion medicine (41): this is perhaps a path for further investigation.
Our own investigations have shown that donors present great variation in the genes coding for CD40L; CD40L was investigated because platelets are the major purveyors of sCD40L in the body (42), and this BRM influences both innate and adaptive immunity (43). CD40L gene polymorphism was found to influence the presentation of secreted CD40L (44). It has been hypothesized that this genetic characteristic of donors may affect pro-inflammatory secretion of donated platelets in BCs (45, 46). This type of result is plausible as well for other BRMs.
Blood Component Manufacturing and Storage Lesions with Pro-inflammatory Consequences in Recipients
A large body of reviews has documented this topic. We may consider two sets of data for illustrative purposes: one explores the secretory capacity of stored platelets over time or of platelets undergoing stress lesions upon collection, processing, and storage (47–50); the other explores the age of blood – and more precisely, the age of pRBCCs – at delivery. Both data sets incorporate diverse readouts: BRMs, oxidants, free iron, and extracellular vesicles (51–54). Despite such extracellular vesicles are reported to carry pro-inflammatory factors (55), some anti-inflammatory properties of extracellular vesicles have been reported (56), suggesting a fine-tune balance of inflammatory responses in relation of extracellular vesicles, likely depending on their sizes (57), origin, and abundance.
To summarize, it is generally reported that longer PC storage is accompanied by greater production of pro-inflammatory cytokines, which make up the majority of anti-inflammatory products (58–62) (Table 1). If leukocytes are still present in the PCs, leukocyte- and platelet-originating cytokines and other BRMs potentiate each other over time (63). Our own group has reported that there is a direct relationship between (i) the secretion of sCD40L [proved to exert a pathogenic effect in certain recipients, together with companion BRMs Ox40L and IL-27 (64, 65)] and the component shelf life; and (ii) between the net amount of sCD40L (alongside potentiating molecules IL-13 or MIP-1α) and the manifestation of an inflammatory AE in the recipient (62). Similar findings exist for mitochondrial DNA (66–68). In addition, it has also been proposed that the techniques used to obtain PCs influence pro-inflammatory reactions, as these techniques do not expose platelets to the same stress (62, 69). Together, these observations strongly suggest the platelet storage lesions have a role in PC induced inflammation and its balance in transfused patients.
The situation for erythrocytes is even more complex. Substantial experimental evidence suggests that the age of erythrocytes, and the subsequent freeing of iron, is directly responsible for inflammation in experimental models, both in vivo and ex vivo/in vitro (70–74). Thus far, however, clinical trials have consistently failed to support this hypothesis (75). It should nevertheless be noted that this is extremely difficult to investigate and that further trials are necessary to resolve the matter (76).
Consequences of Inflammation in Recipients: Manifestations of Adaptive Immunity
There are two main consequences of inflammation in blood component recipients. One – alloimmunization to foreign Ags – is rather clear. The other is transfusion-related immune modulation or TRIM. TRIM is a complex occurrence that involves a number of adaptive immune tools, of which suppressive CD8+ T cells, regulatory T (and probably B) cells, anti-idiotypic T cell clones, along with soluble HLA molecules and other supposed mediators (77–79). It must be made clear that, if the main visible consequence of immunization to foreign Ags is alloimmunization, there is a likely strong T cell immunity; however, it seems difficult to catch it up, and the majority of published works focus on Ab production. One may hypothesize that T cell immunity and TRIM rather explain cases where immunization is not productive in terms of Ab formation. Another consequence of TRIM is perhaps the likely depression of immune surveillance with the report of suspected increase of posttransfusion infections (that are quite well documented) and perhaps malignancies or organ dysfunctions (that are to be ascertained) (80–83).
Alloimmunization remains the most frequently reported AE of transfusion. It is often reported in pathologies where extended matching of red cells is difficult to achieve, for people needing repeated transfusions, such as sickle-cell disease or β-thalassemic patients. Indeed, as there are near 350 Ags on erythrocytes, a perfect match is unlikely. Recipient characteristics such as how well one presents HLA are also considered to have a major impact (84). In addition, residual leukocytes are highly potent immunizers (far more commonly in HLA groups than in HNA groups); platelets are also good immunizers in both HLA class I and HPA groups (85). However, it has been demonstrated that residual leukocytes can influence the global immunization score: as shown in experimental models, stringent but incomplete leucoreduction minimizes alloimmunization, while strict leucoreduction reinforces it, supposedly by erasing the TRIM effect (84).
Yet in spite of several attempts to decipher innate immune mechanisms acting as layers of inflammation that fuel Ag presentation, the details of alloimmunization largely remain a mystery (86).
Last, another consequence of inflammation is the reported enhanced erythrocyte phagocytosis by spleen cells. Inflammation created by excess iron and nitric oxide (NO) freeing – perhaps linked with aged erythrocytes – would aggravate anemia instead of correcting it by bringing Hb/O2 (87). While there is good experimental evidence in favor of this pathophysiology, clinical relevance is not yet ascertained.
From Bench to Bedside: Paths to Improve Patients’ Safety
Pathophysiological studies of platelets reveal that any exposure to stress may have consequences. Depending on the nature of this stress, platelets can mobilize predefined patterns of BRMs (88, 89). Indeed, contrary to what we might expect, given that they are anucleate, platelets do not indiscriminately release granule content through an all-or-nothing mechanism but rather exhibit stress-dictated processes (32, 90–93). This may explain why certain patients having received PCs manifest allergic reactions (where δ-granule BRMs predominate) or FNHTR/inflammation (where α-granule BRMs predominate) (Figure 1; Table 2). A readout in the PC leftover is given by the predominance of either IL-13 or MIP-1α in addition to sCD40L (62). Platelets are extremely reactive cells, and it is almost impossible to not pre-activate them while processing PCs for transfusion purposes. However, it has been made clear that the collection process, i.e., apheresis vs. recovered platelets from whole blood; platelets recovered from platelet-rich plasma vs. from buffy coats; PAS vs. plasma; pathogen reduction/inactivation vs. no additional safety measure; and in the case of apheresis, type of cell separator – and the length of storage are important parameters to control activation (59, 62, 65, 94–97). Despite conflicting data (98), it cannot be ruled out that ABO compatibility vs. identity also affects the outcome of platelet transfusion (99, 100), but probably not by triggering pre-activation. Furthermore, PCs are almost always HLA incompatible, at least for the majority of expressed class I Ags. This has not appeared to be deleterious in terms of clinical outcome – in the PLADO trial (98), for example, but consistent recommendations suggest that refractoriness to platelet transfusion is better addressed with HLA-compatible PCs (97). The HPA case is barely addressed, unless a specific and pathogenic Ab is identified, or in the case of fetal/neonatal maternal incompatibility (101). Serious allergies, allergic reactions, or severe FNHTRs can be addressed – when further PC transfusions are needed – by washing the components. This process is nevertheless tedious as it may itself pre-activate the cells. Alternatively, some teams may absorb pathogenic BRMs on columns when available (102, 103). At present, there are parameters that cannot be controlled (recipients’ genetic characteristics and – to a large extent – donors’ characteristics) and those that can be partly controlled, i.e., manufacturing, ABO matching, and aging of the PCs (Table 3). Most efforts appear to focus on the last three issues to limit inflammation in recipients. Further investigations are needed to evaluate the actual impact of safety measures in PC recipients and determine whether efforts can be made to propose matching procedures that can calculate the most important factors to limit inflammatory responses in patients.
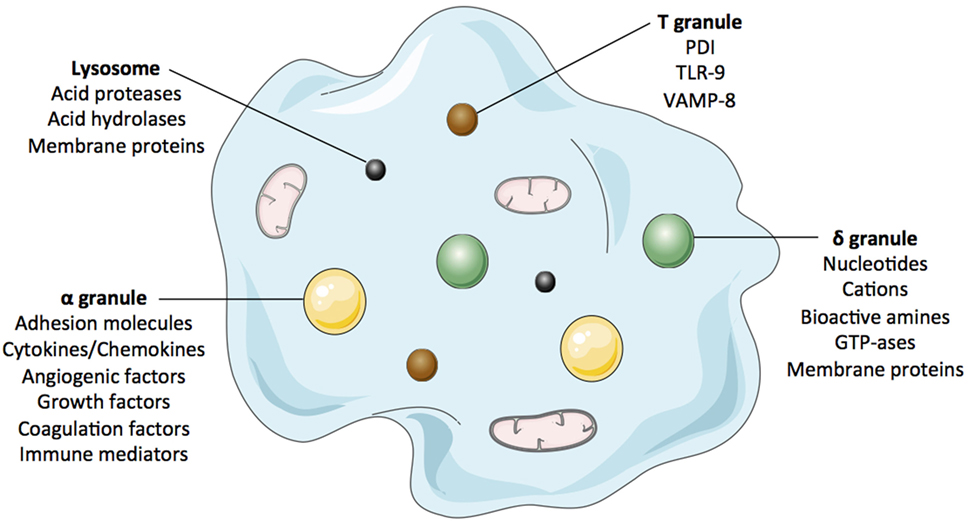
Figure 1. The figure cartoons the platelet’ main granules and their secretory content. Most products that can be released by platelets are listed in complementary Table 2.
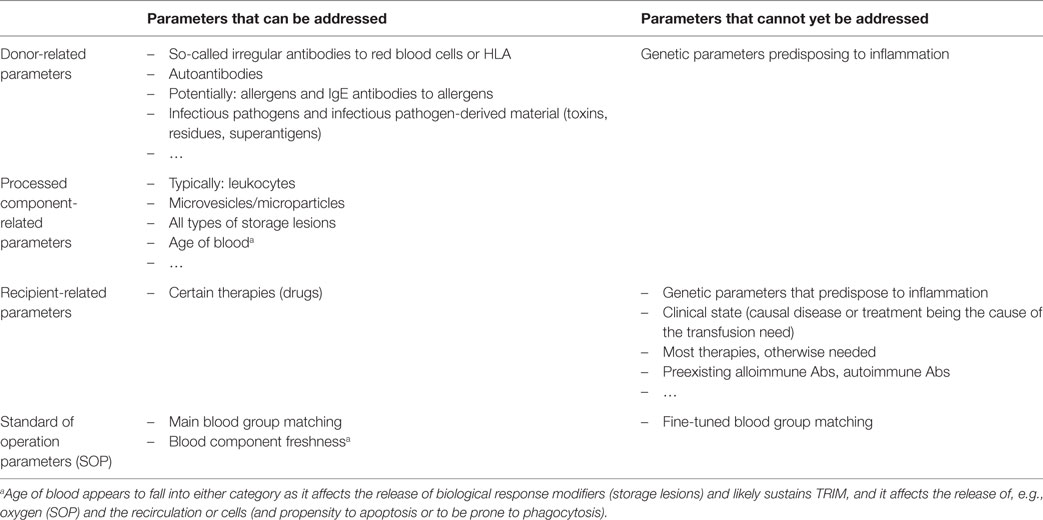
Table 3. Examples of preventable and not yet preventable causes of inflammation in transfusion medicine.
For pRBCCs, the appropriate strategy may be very simple or very complex. In theory, it should be simple if one considers that the enemy is alloimmunization, which concerns inflammation. To limit the risk of immunization, an improvement of blood group Ag matching would be ideal; however, considering the volume of blood components to be issued to millions of recipients, this is simply not achievable on a routine basis. Efforts are being made to facilitate matching for at-risk recipients, such as those routinely receiving transfusions, though success varies according to specific needs of ethnic groups transfused outside their native region, where erythrocyte Ag group distribution differs from their own. Further, if inflammation fuels alloimmunization, some genetic control of responders vs. non-responders – or more precisely, good vs. bad HLA presenters – seems to prevail: this has been observed for certain blood group Ags and is very likely true for all others (77, 104). The situation is more complex than for platelets because the triggers of inflammation are less clearly identified. The age of blood is a likely but unproven factor, and the effects of storage lesions and erythrocyte Ag alloimmunization (105). Various teams have provided indirect evidence after examining whether RBC collection can stress RBCs and subsequently stress endothelial cells exposed to such RBCs (at least in ex vivo/in vitro models) (106, 107). Here again, until more direct evidence becomes available, one may heed protocols that minimize stress to donors’ RBCs and subsequently to recipients’ vascular endothelium. Accordingly, some authors recommend not overexposing cellular blood components to radiation unless absolutely required as this may increase storage lesions (54, 108, 109). Closer examination is needed to determine the extent to which irradiation of BCs favors alloimmunization. Similar caution has been suggested for pathogen inactivation/reduction technologies, but there are conflicting claims in favor of reduction or alloimmunization based on impairment of indirect Ag presentation (84). This too calls for further investigation.
Conclusion
Transfusion is an old therapy, though it is not obsolete. In fact, it is quite modern if seen as cell therapy or biotherapy (110). It is very commonly used and is nowadays associated with few nosocomial AEs. Moreover, not all AEs are truly nosocomial as some are in fact linked to characteristics of recipients that can neither be dampened nor counteracted by matching blood components. When transfusion is associated with AEs, most can be related to an inflammatory state, which is either obvious (allergy, FNHTR, hypotension) or ascribed to such a state by current knowledge (alloimmunization). Indeed, transfusion-transmitted infection has become a rarity, and novel means are regularly applied to further minimize their occurrence. Means of decreasing the occurrence of transfusion-associated inflammation have received less attention and care, though they should be our new focus, to help patients, secure resources, and limit indirect costs. Platelet pathophysiology owes a lot to transfusion medicine: many of the major discoveries in this field were made by researchers who, questioning the role of PC transfusion in AEs (and occasionally SAEs), attempted to solve questions about platelet activation and secretion. This review has not considered the other side of the coin with respect to platelets and their role in the inflammation process. Namely, in addition to being the source of many pro-inflammatory BRMs, platelets also produce healing factors (the terminus of physiological inflammation) that may also be used as therapeutic tools (111).
Author Contributions
OG drafted the manuscript; all other contributors contributed illustrations, discussion, and critical review, along with the production of original data supporting the synthesis as a review article.
Conflict of Interest Statement
The authors declare no competing financial interests and no conflicts of interest regarding this study.
The reviewer PS declared a shared affiliation, though no other collaboration, with one of the authors FC to the handling Editor, who ensured that the process nevertheless met the standards of a fair and objective review.
Acknowledgments
The authors wish to thank Prof. Bruno Pozzetto, Prof. Thomas Bourlet, Prof. Philippe Berthelot, Dr. Hind Hamzeh-Cognasse, Lyon/Saint-Etienne, and Prof. Saloua Jemni-Yacoub, Monastir, for their excellent input, as well as Charles-Antoine Arthaud, Marie-Ange Eyraud, and Jocelyne Fagan for invaluable technical support. They would also like to express their gratitude to Dr. Julien Berthet, Dr. Sandrine Lafarge, Dr. Kim Ahn Nguyen, and Mr. Adrien Chabert for their excellent work. The authors further acknowledge support from University Jean-Monnet of Saint-Etienne, University of Lyon, Région Rhône-Alpes-Auvergne, Foundation Erasmus Mundus Al-Idrisi, Etablissement Français du Sang Rhône-Alpes-Auvergne, Institut National de la Transfusion Sanguine, Agence Nationale du Médicament et des Produits de Santé, Association Recherche-Tranfusion, and Association Les Amis de Rémi.
References
1. Garraud O, Tissot JD. Bloodletting for non-medical reasons: what about safety and quality? Transfus Med (2015) 25(6):424–5. doi:10.1111/tme.12234
2. Alter HJ, Klein HG. The hazards of blood transfusion in historical perspective. Blood (2008) 112(7):2617–26. doi:10.1182/blood-2008-07-077370
3. Higgins SJ, Kain KC, Liles WC. Immunopathogenesis of falciparum malaria: implications for adjunctive therapy in the management of severe and cerebral malaria. Expert Rev Anti Infect Ther (2011) 9(9):803–19. doi:10.1586/eri.11.96
4. Sazama K. Transfusion errors: scope of the problem, consequences, and solutions. Curr Hematol Rep (2003) 2(6):518–21.
5. Shabab T, Khanabdali R, Moghadamtousi SZ, Kadir HA, Mohan G. Neuroinflammation pathways: a general review. Int J Neurosci (2016) 1–10. doi:10.1080/00207454.2016.1212854
6. Gregersen I, Holm S, Dahl TB, Halvorsen B, Aukrust P. A focus on inflammation as a major risk factor for atherosclerotic cardiovascular diseases. Expert Rev Cardiovasc Ther (2016) 14(3):391–403. doi:10.1586/14779072.2016.1128828
7. Matzinger P. Tolerance, danger and the extended family. Annu Rev Immunol (1994) 12:991–1045. doi:10.1146/annurev.iy.12.040194.005015
8. Sansonetti PJ. War and peace at the intestinal epithelial surface: an integrated view of bacterial commensalism versus bacterial pathogenicity. J Pediatr Gastroenterol Nutr (2008) 46(Suppl 1):E6–7. doi:10.1097/01.mpg.0000313819.96520.27
9. Thaiss CA, Zmora N, Levy M, Elinav E. The microbiome and innate immunity. Nature (2016) 535(7610):65–74. doi:10.1038/nature18847
10. Honda K, Littman DR. The microbiota in adaptive immune homeostasis and disease. Nature (2016) 535(7610):75–84. doi:10.1038/nature18848
11. Martin P, Nunan R. Cellular and molecular mechanisms of repair in acute and chronic healing. Br J Dermatol (2015) 173(2):370–8. doi:10.1111/bjd.13954
12. Portou MJ, Baker D, Abraham D, Tsui J. The innate immune system, toll-like receptors and dermal wound healing: a review. Vascul Pharmacol (2015) 71:31–6. doi:10.1016/j.vph.2015.02.007
13. Popovsky MA, Abel MD, Moore SB. Transfusion-related acute lung injury associated with passive transfer of antileukocyte antibodies. Am Rev Respir Dis (1983) 128(1):185–9. doi:10.1164/arrd.1983.128.1.185
14. Vlaar AP, Juffermans NP. Transfusion-related acute lung injury: a clinical review. Lancet (2013) 382(9896):984–94. doi:10.1016/S0140-6736(12)62197-7
15. Tariket S, Sut C, Hamzeh-Cognasse H, Laradi S, Pozzetto B, Garraud O, et al. Transfusion-related acute lung injury: transfusion, platelets and biological response modifiers. Expert Rev Hematol (2016) 9(5):497–508. doi:10.1586/17474086.2016.1152177
16. Hirayama F. Current understanding of allergic transfusion reactions: incidence, pathogenesis, laboratory tests, prevention and treatment. Br J Haematol (2013) 160(4):434–44. doi:10.1111/bjh.12150
17. Bolton-Maggs PH, Cohen H. Serious Hazards of Transfusion (SHOT) haemovigilance and progress is improving transfusion safety. Br J Haematol (2013) 163(3):303–14. doi:10.1111/bjh.12547
18. Hendrickson JE, Hillyer CD. Noninfectious serious hazards of transfusion. Anesth Analg (2009) 108(3):759–69. doi:10.1213/ane.0b013e3181930a6e
19. Marti-Carvajal AJ, Sola I, Gonzalez LE, Leon de Gonzalez G, Rodriguez-Malagon N. Pharmacological interventions for the prevention of allergic and febrile non-haemolytic transfusion reactions. Cochrane Database Syst Rev (2010) 6:CD007539. doi:10.1002/14651858.CD007539.pub2
20. Douet JY, Bujdoso R, Andreoletti O. Leukoreduction and blood-borne vCJD transmission risk. Curr Opin Hematol (2015) 22(1):36–40. doi:10.1097/MOH.0000000000000101
21. Rajesh K, Harsh S, Amarjit K. Effects of prestorage leukoreduction on the rate of febrile nonhemolytic transfusion reactions to red blood cells in a tertiary care hospital. Ann Med Health Sci Res (2015) 5(3):185–8. doi:10.4103/2141-9248.157498
22. Bassuni WY, Blajchman MA, Al-Moshary MA. Why implement universal leukoreduction? Hematol Oncol Stem Cell Ther (2008) 1(2):106–23. doi:10.1016/S1658-3876(08)50042-2
23. Pagano MB, Ness PM, Chajewski OS, King KE, Wu Y, Tobian AA. Hypotensive transfusion reactions in the era of prestorage leukoreduction. Transfusion (2015) 55(7):1668–74. doi:10.1111/trf.13047
24. Politis C, Wiersum JC, Richardson C, Robillard P, Jorgensen J, Renaudier P, et al. The international haemovigilance network database for the surveillance of adverse reactions and events in donors and recipients of blood components: technical issues and results. Vox Sang (2016) 111:409–17. doi:10.1111/vox.12447
25. Gardner K, Hoppe C, Mijovic A, Thein SL. How we treat delayed haemolytic transfusion reactions in patients with sickle cell disease. Br J Haematol (2015) 170(6):745–56. doi:10.1111/bjh.13494
26. Yazdanbakhsh K. Mechanisms of sickle cell alloimmunization. Transfus Clin Biol (2015) 22(3):178–81. doi:10.1016/j.tracli.2015.05.005
27. EDQM. European Directorate for the Quality of Medicines & HealthCare – Guide to the Preparation, Use and Quality Assurance of Blood Components. 18th ed. Strasbourg: Council of Europe (2015).
29. Masse M. Universal leukoreduction of cellular and plasma components: process control and performance of the leukoreduction process. Transfus Clin Biol (2001) 8(3):297–302. doi:10.1016/S1246-7820(01)00119-7
30. Lafeuillade B, Eb F, Ounougnhene N, Petermann R, Daurat G, Huygue G, et al. Residual risk and retrospective analysis of transfusion-transmitted bacterial infection reported by the French national hemovigilance network from 2000 to 2008. Transfusion (2015) 55(3):636–46. doi:10.1111/trf.12883
31. Stolla M, Refaai MA, Heal JM, Spinelli SL, Garraud O, Phipps RP, et al. Platelet transfusion – the new immunology of an old therapy. Front Immunol (2015) 6:28. doi:10.3389/fimmu.2015.00028
32. Garraud O, Cognasse F. Are platelets cells? And if yes, are they immune cells? Front Immunol (2015) 6:article70. doi:10.3389/fimmu.2015.00070
33. Cognasse F, Nguyen KA, Damien P, McNicol A, Pozzetto B, Hamzeh-Cognasse H, et al. The inflammatory role of platelets via their TLRs and siglec receptors. Front Immunol (2015) 6:article83. doi:10.3389/fimmu.2015.00083
34. Mendonca R, Silveira AA, Conran N. Red cell DAMPs and inflammation. Inflamm Res (2016) 65(9):665–78. doi:10.1007/s00011-016-0955-9
35. Kaplanski G, Farnarier C, Kaplanski S, Porat R, Shapiro L, Bongrand P, et al. Interleukin-1 induces interleukin-8 secretion from endothelial cells by a juxtacrine mechanism. Blood (1994) 84(12):4242–8.
36. Daub K, Langer H, Seizer P, Stellos K, May AE, Goyal P, et al. Platelets induce differentiation of human CD34+ progenitor cells into foam cells and endothelial cells. FASEB J (2006) 20(14):2559–61. doi:10.1096/fj.06-6265fje
37. Lovren F, Verma S. Evolving role of microparticles in the pathophysiology of endothelial dysfunction. Clin Chem (2013) 59(8):1166–74. doi:10.1373/clinchem.2012.199711
38. Xie RF, Hu P, Wang ZC, Yang J, Yang YM, Gao L, et al. Platelet-derived microparticles induce polymorphonuclear leukocyte-mediated damage of human pulmonary microvascular endothelial cells. Transfusion (2015) 55(5):1051–7. doi:10.1111/trf.12952
39. Chasse M, McIntyre L, English SW, Tinmouth A, Knoll G, Wolfe D, et al. Effect of blood donor characteristics on transfusion outcomes: a systematic review and meta-analysis. Transfus Med Rev (2016) 30(2):69–80. doi:10.1016/j.tmrv.2016.01.002
40. Chasse M, Tinmouth A, English SW, Acker JP, Wilson K, Knoll G, et al. Association of blood donor age and sex with recipient survival after red blood cell transfusion. JAMA Intern Med (2016) 176(9):1307–14. doi:10.1001/jamainternmed.2016.3324
41. Fischer J, Jung N, Robinson N, Lehmann C. Sex differences in immune responses to infectious diseases. Infection (2015) 43(4):399–403. doi:10.1007/s15010-015-0791-9
42. Andre P, Nannizzi-Alaimo L, Prasad SK, Phillips DR. Platelet-derived CD40L: the switch-hitting player of cardiovascular disease. Circulation (2002) 106(8):896–9. doi:10.1161/01.CIR.0000028962.04520.01
43. Aloui C, Prigent A, Sut C, Tariket S, Hamzeh-Cognasse H, Pozzetto B, et al. The signaling role of CD40 ligand in platelet biology and in platelet component transfusion. Int J Mol Sci (2014) 15(12):22342–64. doi:10.3390/ijms151222342
44. Mälarstig A, Lindahl B, Wallentin L, Siegbahn A. Soluble CD40L levels are regulated by the -3459 A>G polymorphism and predict myocardial infarction and the efficacy of antithrombotic treatment in non-ST elevation acute coronary syndrome. Arterioscler Thromb Vasc Biol (2006) 26(7):1667–73. doi:10.1161/01.ATV.0000222908.78873.36
45. Aloui C, Sut C, Prigent A, Fagan J, Cognasse F, Granados-Herbepin V, et al. Are polymorphisms of the immunoregulatory factor CD40LG implicated in acute transfusion reactions? Sci Rep (2014) 4:7239. doi:10.1038/srep07239
46. Aloui C, Prigent A, Tariket S, Sut C, Fagan J, Cognasse F, et al. Levels of human platelet-derived soluble CD40 ligand depend on haplotypes of CD40LG-CD40-ITGA2. Sci Rep (2016) 6:24715. doi:10.1038/srep24715
47. Capocelli KE, Dumont LJ. Novel platelet storage conditions: additive solutions, gas, and cold. Curr Opin Hematol (2014) 21(6):491–6. doi:10.1097/MOH.0000000000000081
48. Thon JN, Schubert P, Devine DV. Platelet storage lesion: a new understanding from a proteomic perspective. Transfus Med Rev (2008) 22(4):268–79. doi:10.1016/j.tmrv.2008.05.004
49. Devine DV, Serrano K. The platelet storage lesion. Clin Lab Med (2010) 30(2):475–87. doi:10.1016/j.cll.2010.02.002
50. Ohto H, Nollet KE. Overview on platelet preservation: better controls over storage lesion. Transfus Apher Sci (2011) 44(3):321–5. doi:10.1016/j.transci.2011.03.008
51. Hod EA, Spitalnik SL. Stored red blood cell transfusions: iron, inflammation, immunity, and infection. Transfus Clin Biol (2012) 19(3):84–9. doi:10.1016/j.tracli.2012.04.001
52. Hess JR. Measures of stored red blood cell quality. Vox Sang (2014) 107(1):1–9. doi:10.1111/vox.12130
53. Liu C, Liu X, Janes J, Stapley R, Patel RP, Gladwin MT, et al. Mechanism of faster NO scavenging by older stored red blood cells. Redox biol (2014) 2:211–9. doi:10.1016/j.redox.2013.12.014
54. Adams F, Bellairs G, Bird AR, Oguntibeju OO. Biochemical storage lesions occurring in nonirradiated and irradiated red blood cells: a brief review. Biomed Res Int (2015) 2015:968302. doi:10.1155/2015/968302
55. Lee H, Zhang D, Zhu Z, Dela Cruz CS, Jin Y. Epithelial cell-derived microvesicles activate macrophages and promote inflammation via microvesicle-containing microRNAs. Sci Rep (2016) 6:35250. doi:10.1038/srep35250
56. Burnouf T, Chou ML, Goubran H, Cognasse F, Garraud O, Seghatchian J. An overview of the role of microparticles/microvesicles in blood components: are they clinically beneficial or harmful? Transfus Apher Sci (2015) 53(2):137–45. doi:10.1016/j.transci.2015.10.010
57. Boilard E, Duchez AC, Brisson A. The diversity of platelet microparticles. Curr Opin Hematol (2015) 22(5):437–44. doi:10.1097/MOH.0000000000000166
58. Stack G, Snyder EL. Cytokine generation in stored platelet concentrates. Transfusion (1994) 34(1):20–5. doi:10.1046/j.1537-2995.1994.34194098597.x
59. Cognasse F, Boussoulade F, Chavarin P, Acquart S, Fabrigli P, Lamy B, et al. Release of potential immunomodulatory factors during platelet storage. Transfusion (2006) 46(7):1184–9. doi:10.1111/j.1537-2995.2006.00869.x
60. Seghatchian J. Platelet storage lesion: an update on the impact of various leukoreduction processes on the biological response modifiers. Transfus Apher Sci (2006) 34(1):125–30. doi:10.1016/j.transci.2005.09.026
61. Sahler J, Spinelli S, Phipps R, Blumberg N. CD40 ligand (CD154) involvement in platelet transfusion reactions. Transfus Clin Biol (2012) 19(3):98–103. doi:10.1016/j.tracli.2012.02.003
62. Nguyen KA, Hamzeh-Cognasse H, Sebban M, Fromont E, Chavarin P, Absi L, et al. A computerized prediction model of hazardous inflammatory platelet transfusion outcomes. PLoS One (2014) 9(5):e97082. doi:10.1371/journal.pone.0097082
63. Aloui C, Chakroun T, Prigent A, Jemni-Yacoub S, Cognasse F, Laradi S, et al. Leukocyte cytokines dominate over platelet cytokines overtime in non-leukoreduced platelet components. Blood Transfus (2016) 8:1–10. doi:10.2450/2016.0076-16
64. Hamzeh-Cognasse H, Damien P, Nguyen KA, Arthaud CA, Eyraud MA, Chavarin P, et al. Immune-reactive soluble OX40 ligand, soluble CD40 ligand, and interleukin-27 are simultaneously oversecreted in platelet components associated with acute transfusion reactions. Transfusion (2014) 54(3):613–25. doi:10.1111/trf.12378
65. Hamzeh-Cognasse H, Laradi S, Osselaer JC, Cognasse F, Garraud O. Amotosalen-HCl-UVA pathogen reduction does not alter poststorage metabolism of soluble CD40 ligand, Ox40 ligand and interkeukin-27, the cytokines that generally associate with serious adverse events. Vox Sang (2015) 108(2):205–7. doi:10.1111/vox.12203
66. Boudreau LH, Duchez AC, Cloutier N, Soulet D, Martin N, Bollinger J, et al. Platelets release mitochondria serving as substrate for bactericidal group IIA-secreted phospholipase A2 to promote inflammation. Blood (2014) 124(14):2173–83. doi:10.1182/blood-2014-05-573543
67. Cognasse F, Aloui C, Anh Nguyen K, Hamzeh-Cognasse H, Fagan J, Arthaud CA, et al. Platelet components associated with adverse reactions: predictive value of mitochondrial DNA relative to biological response modifiers. Transfusion (2016) 56(2):497–504. doi:10.1111/trf.13373
68. Yasui K, Matsuyama N, Kuroishi A, Tani Y, Furuta RA, Hirayama F. Mitochondrial damage-associated molecular patterns as potential proinflammatory mediators in post-platelet transfusion adverse effects. Transfusion (2016) 56(5):1201–12. doi:10.1111/trf.13535
69. Nguyen KA, Chavarin P, Arthaud CA, Cognasse F, Garraud O. Do manual and automated processes with distinct additive solutions affect whole blood-derived platelet components differently? Blood Transfus (2013) 11(1):152–3. doi:10.2450/2012.0010-12
70. Godbey EA, Hod EA. The outsider adverse event in transfusion: inflammation. Presse Med (2016) 45(7–8 Pt 2):e325–9. doi:10.1016/j.lpm.2016.06.025
71. Spitalnik SL, Francis RO. Red blood cell components: meeting the quantitative and qualitative transfusion needs. Presse Med (2016) 45(7–8 Pt 2):e281–8. doi:10.1016/j.lpm.2016.06.019
72. Hod EA, Zhang N, Sokol SA, Wojczyk BS, Francis RO, Ansaldi D, et al. Transfusion of red blood cells after prolonged storage produces harmful effects that are mediated by iron and inflammation. Blood (2010) 115(21):4284–92. doi:10.1182/blood-2009-10-245001
73. Zimring JC, Spitalnik SL. Pathobiology of transfusion reactions. Annu Rev Pathol (2015) 10:83–110. doi:10.1146/annurev-pathol-012414-040318
74. Gibb DR, Calabro S, Liu D, Tormey CA, Spitalnik SL, Zimring JC, et al. The Nlrp3 inflammasome does not regulate alloimmunization to transfused red blood cells in mice. EBioMedicine (2016) 9:77–86. doi:10.1016/j.ebiom.2016.06.008
75. Lacroix J, Hébert PC, Fergusson DA, Tinmouth A, Cook DJ, Marshall JC, et al. Age of transfused blood in critically ill adults. N Engl J Med (2015) 372(15):1410–8. doi:10.1056/NEJMoa1500704
76. Garraud O. Do we need [more] clinical trials in transfusion medicine and hemotherapy? Transfus Apher Sci (2016) 55(2):262–3. doi:10.1016/j.transci.2016.09.001
77. Bilgin YM, van de Watering LM, Brand A. Clinical effects of leucoreduction of blood transfusions. Neth J Med (2011) 69(10):441–50.
78. Refaai MA, Blumberg N. Transfusion immunomodulation from a clinical perspective: an update. Expert Rev Hematol (2013) 6(6):653–63. doi:10.1586/17474086.2013.850026
79. Abe Y, Urakami H, Ostanin D, Zibari G, Hayashida T, Kitagawa Y, et al. Induction of Foxp3-expressing regulatory T-cells by donor blood transfusion is required for tolerance to rat liver allografts. PLoS One (2009) 4(11):e7840. doi:10.1371/journal.pone.0007840
80. Karrowni W, Vora AN, Dai D, Wojdyla D, Dakik H, Rao SV. Blood transfusion and the risk of acute kidney injury among patients with acute coronary syndrome undergoing percutaneous coronary intervention. Circ Cardiovasc Interv (2016) 9(9):e003279. doi:10.1161/CIRCINTERVENTIONS.115.003279
81. Chalfin HJ, Liu JJ, Gandhi N, Feng Z, Johnson D, Netto GJ, et al. Blood transfusion is associated with increased perioperative morbidity and adverse oncologic outcomes in bladder cancer patients receiving neoadjuvant chemotherapy and radical cystectomy. Ann Surg Oncol (2016) 23(8):2715–22. doi:10.1245/s10434-016-5193-4
82. Shander A, Lobel GP, Javidroozi M. Transfusion practices and infectious risks. Expert Rev Hematol (2016) 9(6):597–605. doi:10.1586/17474086.2016.1164593
83. Muszynski JA, Spinella PC, Cholette JM, Acker JP, Hall MW, Juffermans NP, et al. Transfusion-related immunomodulation: review of the literature and implications for pediatric critical illness. Transfusion (2016). doi:10.1111/trf.13855
84. Pavenski K, Freedman J, Semple JW. HLA alloimmunization against platelet transfusions: pathophysiology, significance, prevention and management. Tissue Antigens (2012) 79(4):237–45. doi:10.1111/j.1399-0039.2012.01852.x
85. Rozman P. Platelet antigens. The role of human platelet alloantigens (HPA) in blood transfusion and transplantation. Transpl Immunol (2002) 10(2–3):165–81. doi:10.1016/S0966-3274(02)00063-1
86. Hod EA, Spitalnik SL. Harmful effects of transfusion of older stored red blood cells: iron and inflammation. Transfusion (2011) 51(4):881–5. doi:10.1111/j.1537-2995.2011.03096.x
87. Hendrickson JE, Chadwick TE, Roback JD, Hillyer CD, Zimring JC. Inflammation enhances consumption and presentation of transfused RBC antigens by dendritic cells. Blood (2007) 110(7):2736–43. doi:10.1182/blood-2007-03-083105
88. Berthet J, Damien P, Hamzeh-Cognasse H, Pozzetto B, Garraud O, Cognasse F. Toll-like receptor 4 signal transduction in platelets: novel pathways. Br J Haematol (2010) 151(1):89–92. doi:10.1111/j.1365-2141.2010.08292.x
89. Berthet J, Damien P, Hamzeh-Cognasse H, Arthaud CA, Eyraud MA, Zeni F, et al. Human platelets can discriminate between various bacterial LPS isoforms via TLR4 signaling and differential cytokine secretion. Clin Immunol (2012) 145(3):189–200. doi:10.1016/j.clim.2012.09.004
90. Cognasse F, Garraud O, Pozzetto B, Laradi S, Hamzeh-Cognasse H. How can non-nucleated platelets be so smart? J Thromb Haemost (2016) 14(4):794–6. doi:10.1111/jth.13262
91. Mantovani A, Garlanda C. Platelet-macrophage partnership in innate immunity and inflammation. Nat Immunol (2013) 14(8):768–70. doi:10.1038/ni.2666
92. Kapur R, Zufferey A, Boilard E, Semple JW. Nouvelle cuisine: platelets served with inflammation. J Immunol (2015) 194(12):5579–87. doi:10.4049/jimmunol.1500259
93. Semple JW, Italiano JE Jr, Freedman J. Platelets and the immune continuum. Nat Rev Immunol (2011) 11(4):264–74. doi:10.1038/nri2956
94. Cognasse F, Osselaer JC, Payrat JM, Chavarin P, Corash L, Garraud O. Release of immune modulation factors from platelet concentrates during storage after photochemical pathogen inactivation treatment. Transfusion (2008) 48(5):809–13. doi:10.1111/j.1537-2995.2008.01655.x
95. Cognasse F, Hamzeh-Cognasse H, Lafarge S, Acquart S, Chavarin P, Courbil R, et al. Donor platelets stored for at least 3 days can elicit activation marker expression by the recipient’s blood mononuclear cells: an in vitro study. Transfusion (2009) 49(1):91–8. doi:10.1111/j.1537-2995.2008.01931.x
96. Chavarin P, Cognasse F, Argaud C, Vidal M, De Putter C, Boussoulade F, et al. In vitro assessment of apheresis and pooled buffy coat platelet components suspended in plasma and SSP+ photochemically treated with amotosalen and UVA for pathogen inactivation (INTERCEPT Blood System). Vox Sang (2011) 100(2):247–9. doi:10.1111/j.1423-0410.2010.01389.x
97. Garraud O, Cognasse F, Tissot JD, Chavarin P, Laperche S, Morel P, et al. Improving platelet transfusion safety: biomedical and technical considerations. Blood Transfus (2016) 14(2):109–22. doi:10.2450/2015.0042-15
98. Kaufman RM, Assmann SF, Triulzi DJ, Strauss RG, Ness P, Granger S, et al. Transfusion-related adverse events in the Platelet Dose study. Transfusion (2015) 55(1):144–53. doi:10.1111/trf.12791
99. Zaffuto BJ, Conley GW, Connolly GC, Henrichs KF, Francis CW, Heal JM, et al. ABO-immune complex formation and impact on platelet function, red cell structural integrity and haemostasis: an in vitro model of ABO non-identical transfusion. Vox Sang (2016) 110(3):219–26. doi:10.1111/vox.12354
100. Blumberg N, Refaai M, Heal J. ABO matching of platelet transfusions – “Start Making Sense”. “As we get older, and stop making sense. ” – The Talking Heads (1984). Blood Transfus (2015) 13(3):347–50. doi:10.2450/2015.0001-15
101. Brojer E, Husebekk A, Debska M, Uhrynowska M, Guz K, Orzinska A, et al. Fetal/Neonatal alloimmune thrombocytopenia: pathogenesis, diagnostics and prevention. Arch Immunol Ther Exp (Warsz) (2016) 64(4):279–90. doi:10.1007/s00005-015-0371-9
102. Cholette JM, Henrichs KF, Alfieris GM, Powers KS, Phipps R, Spinelli SL, et al. Washing red blood cells and platelets transfused in cardiac surgery reduces postoperative inflammation and number of transfusions: results of a prospective, randomized, controlled clinical trial. Pediatr Crit Care Med (2012) 13(3):290–9. doi:10.1097/PCC.0b013e31822f173c
103. Tanaka S, Hayashi T, Tani Y, Hirayama F. Removal of biological response modifiers associated with platelet transfusion reactions by columns containing adsorption beads. Transfusion (2014) 54(7):1790–7. doi:10.1111/trf.12542
104. Alexander PE, Barty R, Fei Y, Vandvik PO, Pai M, Siemieniuk RA, et al. Transfusion of fresher vs older red blood cells in hospitalized patients: a systematic review and meta-analysis. Blood (2016) 127(4):400–10. doi:10.1182/blood-2015-09-670950
105. Kormoczi GF, Mayr WR. Responder individuality in red blood cell alloimmunization. Transfus Med Hemother (2014) 41(6):446–51. doi:10.1159/000369179
106. Cognasse F, Garraud O, Hamzeh-Cognasse H, Damien P, Nguyen KA, Pozzetto B, et al. Investigative in vitro study about red blood cell concentrate processing and storage. Am J Respir Crit Care Med (2013) 187(2):216–7. doi:10.1164/ajrccm.187.2.216
107. Radwanski K, Garraud O, Cognasse F, Hamzeh-Cognasse H, Payrat JM, Min K. The effects of red blood cell preparation method on in vitro markers of red blood cell aging and inflammatory response. Transfusion (2013) 53(12):3128–38. doi:10.1111/trf.12143
108. Gehrie EA, Dunbar NM. Modifications to blood components: when to use them and what is the evidence? Hematol Oncol Clin North Am (2016) 30(3):653–63. doi:10.1016/j.hoc.2016.01.007
109. Muench MO, Heitman JW, Inglis H, Fomin ME, Marschner S, Goodrich RP, et al. Reduced alloimmunization in mice following repeated transfusion with pathogen-reduced platelets. Transfusion (2016) 56(6):1419–29. doi:10.1111/trf.13579
110. Garraud O, Guillevin L. Towards a “Nouvelle Vague” therapy? Presse Med (2016) 45(7–8 Pt 2):e243–5. doi:10.1016/j.lpm.2016.06.015
Keywords: inflammation, transfusion, allergy, blood components, leukocytes, platelets, erythrocytes, alloimmunization
Citation: Garraud O, Tariket S, Sut C, Haddad A, Aloui C, Chakroun T, Laradi S and Cognasse F (2016) Transfusion as an Inflammation Hit: Knowns and Unknowns. Front. Immunol. 7:534. doi: 10.3389/fimmu.2016.00534
Received: 31 August 2016; Accepted: 11 November 2016;
Published: 29 November 2016
Edited by:
Fulvio D’Acquisto, Queen Mary University of London, UKReviewed by:
Philippe Saas, Etablissement Français du Sang Bourgogne Franche-Comté, FranceAngela Ianaro, University of Naples Federico II, Italy
Philip Norris, Blood Systems, USA
Copyright: © 2016 Garraud, Tariket, Sut, Haddad, Aloui, Chakroun, Laradi and Cognasse. This is an open-access article distributed under the terms of the Creative Commons Attribution License (CC BY). The use, distribution or reproduction in other forums is permitted, provided the original author(s) or licensor are credited and that the original publication in this journal is cited, in accordance with accepted academic practice. No use, distribution or reproduction is permitted which does not comply with these terms.
*Correspondence: Olivier Garraud, b2dhcnJhdWRAaW50cy5mcg==