- Departamento de Inmunología, Instituto de Investigaciones Biomédicas, Universidad Nacional Autónoma de México, Ciudad de México, Mexico
Antibodies participate in defense of the organism from all types of pathogens, including viruses, bacteria, fungi, and protozoa. IgG antibodies recognize their associated antigen via their two Fab portions and are in turn recognized though their Fc portion by specific Fcγ receptors (FcγRs) on the membrane of immune cells. Multiple types and polymorphic variants of FcγR exist. These receptors are expressed in many cells types and are also redundant in inducing cell responses. Crosslinking of FcγR on the surface of leukocytes activates several effector functions aimed toward the destruction of pathogens and the induction of an inflammatory response. In the past few years, new evidence on how the particular IgG subclass and the glycosylation pattern of the antibody modulate the IgG–FcγR interaction has been presented. Despite these advances, our knowledge of what particular effector function is activated in a certain cell and in response to a specific type of FcγR remains very limited today. On one hand, each immune cell could be programmed to perform a particular cell function after FcγR crosslinking. On the other, each FcγR could activate a particular signaling pathway leading to a unique cell response. In this review, I describe the main types of FcγRs and our current view of how particular FcγRs activate various signaling pathways to promote unique leukocyte functions.
Introduction
The first antibodies produced by the adaptive immune response belong to the immunoglobulin M (IgM) class. These antibodies present low affinity for pathogen antigens. However, as the adaptive immune response progresses, antibodies produced are mainly of the IgG class. These antibodies present higher affinity and greater specificity for their particular antigen. Thus, IgG antibodies are involved in protection from all types of pathogens, including viruses, bacteria, fungi, and protozoa (1).
Although, IgG molecules are key for controlling infections, these antibodies usually do not directly damage the microorganisms they recognize. Nowadays, it is well known that leukocytes of the innate immune system are responsible for the protective effects of these antibodies. Some antibodies can directly neutralize toxins or viruses, and activate complement. By binding to a toxin, antibodies prevent the toxin from reaching its receptor on a cell and thus protect the cell. Similarly, by binding to a virus, antibodies inhibit uncoating of the virus and prevent a productive viral infection (2). Antibodies can also activate complement, which is then deposited on pathogens to promote phagocytosis via complement receptors (3, 4), or to induce bacterial lysis via the formation of the membrane attack complex (5).
IgG antibodies recognize their associated antigen via their two Fab (fragment antigen-binding) portions and are in turn recognized though their Fc (fragment crystallizable) portion by specific Fcγ receptors (FcγRs) on the membrane of immune cells (6, 7). Crosslinking of FcγR on the surface of cells activates several effector functions. These effector functions are aimed toward the destruction of pathogens and the induction of an inflammatory response that is beneficial during infections (8). Depending on the cell type, and also on the Fcγ receptor type, these effector functions include phagocytosis, activation of the oxidative burst, cell degranulation, antibody-dependent cell-mediated cytotoxicity (ADCC), and activation of genes for production of cytokines and chemokines (8, 9).
Because FcγR-mediated cell effector functions vary considerably among different leukocytes and types of IgG, it is then of great interest to understand how a certain FcγR is activated to induce a particular cellular function. This knowledge would help us in the future to augment an effective anti-microbial response for example during infections, or to inhibit an exacerbated inflammatory or autoimmune response (10, 11). In addition, it will help us to develop new therapeutic antibodies capable of interacting with certain Fc receptors to induce particular effector cell functions (12). The first level of control is clearly the binding of IgG molecules to FcγRs. In the past few years, the binding of IgG molecules to FcγRs has been examined more carefully, and new evidences on the manner some factors modulate the IgG–FcγR interaction have been described. These factors include the particular IgG subclass (13, 14) and the glycosylation pattern of the antibody (15–17).
Despite these advances on how IgG molecules and FcγRs interact, our knowledge of what particular effector function is activated in a certain cell and in response to a specific type of FcγR remains very limited today. The traditional view has been that each immune cell could be programmed to perform a particular cell function after FcγR crosslinking. Another more recent view is that each FcγR activates a particular signaling pathway leading to a unique cell response. In this review, I describe the main types of FcγRs, and the recent evidence that supports the idea that a specific FcγR induces a unique cell response.
Fcγ Receptors
Fcγ receptors are a family of glycoproteins expressed on the membrane of immune cells, and capable of binding the Fc portion of IgG antibody molecules (9, 14). These receptors can bind to the various IgG subclasses with different affinities (8), and when crosslinked by multivalent antigen-antibody complexes, can induce different cellular responses. In mice, there are three exclusive IgG receptors (mFcγRI, mFcRn, and mTRIM21), and three receptors that can bind both IgG and IgE (mFcγRIIb, mFcγRIII, and mFcγRIV) (18) (Figure 1). The latter dual-specific receptors prefer binding to IgG (affinity is around 2 log higher) that they are usually described as IgG receptors (18). However, interacting with IgE can also induce biological responses (19). All these receptors bind IgG on the membrane of the cells expressing them, except the neonatal FcR (mFcRn) (20, 21) and the cytosolic tripartite motif-containing protein 21 (TRIM21) (22, 23) that bind antibody molecules once internalized. In addition, polymorphisms for mouse Fc receptors have been described. Ly17.1 and Ly17.2 are alleles for mFcγRIIb, and V, T, H are alleles for mFcγRIII (Figure 1). These receptors can also be divided into activating (mFcγRI, mFcγRIII, and FcγRIV) and one inhibitory (mFcγRIIb) receptors (14, 24).
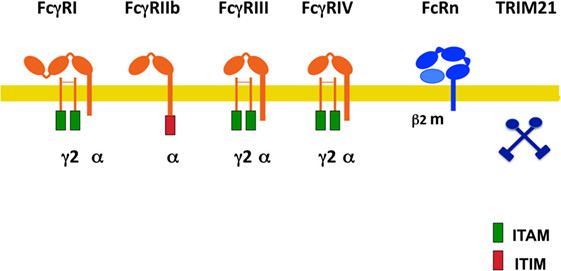
Figure 1. Mouse Fcγ receptors (FcγRs). Schematic illustration of mouse receptors for IgG. FcγRs are shown relative to the cell membrane (yellow line) and together with their respective signaling subunits. γ2, FcR gamma subunit dimer; β2m, beta-2 microglobulin; ITAM, immunoreceptor tyrosine-based activation motif (green rectangle); ITIM, immunoreceptor tyrosine-based inhibition motif (red rectangle); FcRn, neonatal Fc receptor. TRIM21 is a cytosolic receptor.
In humans, also several activating receptors (FcγRI/CD64, FcγRIIa/CD32a, FcγRIIc/CD32c, and FcγRIIIa/CD16a), one inhibitory receptor (FcγRIIb/CD32b), and one glycosylphosphatidylinositol (GPI)-linked receptor, lacking a cytoplasmic tail (FcγRIIIb/CD16b) have been identified (Figure 2) (14, 24–26). These are also described as classical IgG receptors. In addition, non-classical receptors for IgG include two FcR-like receptors, FcRL4/CD307d and FcRL5/CD307e that are homologous to FcγRI, and the receptors hFcRn and hTRIM21. All these receptors, with the exception of FcRL4 (that binds both IgA and IgG) are truly IgG receptors since they do not bind any other class of immunoglobulin (27) (Figure 2).
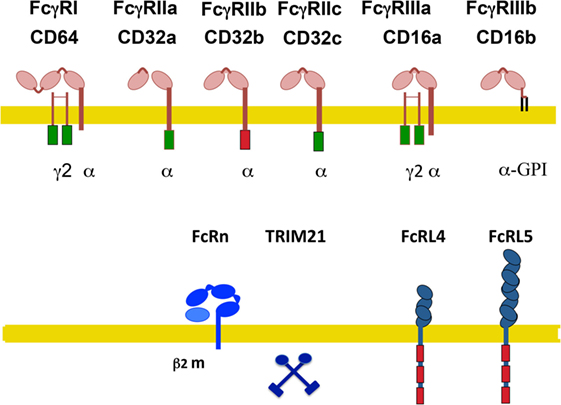
Figure 2. Human Fcγ receptors (FcγRs). Schematic illustration of human receptors for IgG. FcγRs are shown relative to the cell membrane (yellow line) and together with their respective signaling subunits. Upper panel shows the classical FcγR (those containing typical Ig-domains). Lower panel shows the non-classical FcγR. γ2, FcR gamma subunit dimer; β2m, beta-2 microglobulin; ITAM, immunoreceptor tyrosine-based activation motif (green rectangle); ITIM, immunoreceptor tyrosine-based inhibition motif (red rectangle); FcRn, neonatal Fc receptor. TRIM21 is a cytosolic receptor. The FcR-like receptors FcRL4 and FcRL5 are inhibitory receptors that are expressed exclusively on B cells.
FcRL4 and FcRL5 are inhibitory receptors that are expressed exclusively on B cells and downregulate B-cell receptor responses (28, 29). FcRL4 is restricted to a subset of memory B cells (30). The hFcRn is a transport receptor that allows IgG recycling. Expression of hFcRn on vascular endothelial cells and on intestinal epithelial cells permits bidirectional IgG transport, from the circulation into tissues and vice versa. Also, on placental syncytiotrophoblasts, this receptor allows the transport of maternal IgG into the fetus (20). The hFcRn seems also capable of transporting IgG-bound antigens in dendritic cells (31), macrophages (32), and neutrophils (33), thus promoting antigen presentation and modulating immune responses (21). Similarly to the mouse, the cytosolic receptor hTRIM21 is also ubiquitously expressed (23).
FcγRI is a high-affinity receptor, having three Ig-like extracellular domains. It binds mainly monomeric IgG (34). By contrast, FcγRII and FcγRIII are low-affinity receptors, having two Ig-like extracellular domains. They bind only multimeric immune complexes (34, 35).
Activating receptors are associated with a dimer of the common FcRγ chain, which contains an immunoreceptor tyrosine-based activation motif (ITAM) sequence (Figure 2). An ITAM is a conserved signaling motif with the consensus sequence YxxI/Lx(6–12)YxxI/L, where x represents any amino acid (36). Exceptions to this rule are the human FcγRIIa and FcγRIIc, which contain their own ITAM within their cytoplasmic tail. By contrast, the inhibitory receptor FcγRIIb contains an immunoreceptor tyrosine-based inhibition motif (ITIM) within its cytoplasmic tail (Figure 2). An ITIM has the consensus sequence I/V/L/SxYxxL/V (37). FcγRIIb negatively regulates various cell functions including antibody production by the B cell (38), proliferation, degranulation, and phagocytosis in other leukocytes when it is crosslinked with activating FcγRs (37, 39). Most immune cells express both activating and inhibitory FcγRs, hence simultaneous crosslinking establishes a threshold for cell activation (40, 41) that maintains a balanced immune response (42, 43). The GPI-linked receptor FcγRIIIb is expressed mainly on neutrophils and on a subset of basophils (44). It is classified as an activating receptor, although it is not associated with the common FcRγ chain (34). In fact, no other subunits are known to associate with it, and its signaling mechanism remains unknown (4, 14). The human FcγRIIa and FcγRIIIb are exclusive receptors that are not found in other species (24, 45).
Polymorphisms and Links to Disease Susceptibility
In addition, there are several polymorphisms in the human FcγRII and FcγRIII (46). Two alleles of the gene coding for FcγRIIa generate two isoforms with different aminoacids at position 131. These are known as low-responder (H131) and high-responder (R131) (47). The H131 and R131 isoforms are expressed differentially in Caucasian and Asian people (48). For FcγRIIIa also allelic variants exist expressing either valine or phenylalanine at position 158 (49, 50). Similarly, for FcγRIIIb on neutrophils, two isoforms exist differing at four positions, NA1 (R36 N65 D82 V106) and NA2 (S36 S65 N82 I106) (51), and with different glycosylation patterns (52). These differences affect the capacity of FcγRIIIb to interact with human IgG. Therefore, neutrophils from individuals who are homozygous for the NA1 allele have better phagocytosis of IgG-opsonized targets than do neutrophils from NA2-homozygous individuals (53, 54). Also, a point mutation (A78D) in the NA2 allele generates another FcγRIIIb isoform named SH (55). In addition, the gene for FcγRIIIb may be present in a variable number of gene copies in different individuals. Thus, a single person may express all three FcγRIIIb isoforms (56). Several of these polymorphisms have been associated to autoimmune and infectious diseases. FcγRIIa R131 has been associated to nephropathy (57), bacterial infections (58), and systemic lupus erythematosus (SLE) (57, 59). FcγRIIIa F158 has been associated to SLE (49) and to rheumatoid arthritis (60). FcγRIIIb NA1 has been associated to Wegener granulomatosis (61) and systemic vasculitis (62), while FcγRIIIb NA2 has been associated to SLE in Japanese people (54). These multiple FcγR and their allelic variants vary greatly in their affinity for different IgG classes (35).
Cell Expression of FcγRs
Fcγ receptors are found on many cells of the immune system (34). The expression pattern of these receptors on the different immune cell types has been recently reexamined with support from new FcγR-specific monoclonal antibodies (Table 1). FcγRI is expressed on monocytes, macrophages, dendritic cells (25), and interferon-γ (IFN-γ)-stimulated neutrophils (63) and mast cells (64). FcγRIIa is expressed on macrophages, neutrophils, mast cells, eosinophils, and platelets. FcγRIIb is expressed on B cells (65), basophils (66), tissue macrophages, dendritic cells (65), and on a small fraction of monocytes and neutrophils (67). FcγRIIIa is expressed mostly on NK cells and weakly on monocytes, macrophages, basophils, and mast cells (26, 34). FcγRIIIb is expressed on neutrophils and by a subset of basophils (44). Interestingly, the expression of some of these classical FcγRs has been found on cells other than hematopoietic cells (68). For example, FcγRI expressed on sensory and motor neurons allows uptake of IgG and release of neurotransmitter (69), while FcγRIIb is expressed on hippocampal neurons (70), and also on liver endothelial sinusoidal cells (71). Thus, FcγR-mediated functions may not always be related to immune cells.
It is worth mentioning that FcγR expression is not fixed and can be altered by other factors. For example, Th1-type cytokines such as IFN-γ and the anaphylatoxin C5a upregulate activating FcγRs expression and downregulate FcγRIIb expression (72, 73), whereas Th2-type cytokines, such as interleukin (IL)-4, IL-10, and transforming growth factor-beta upregulate FcγRIIb expression (41, 74).
Soluble FcγRs
Another interesting characteristic of FcγRs is that soluble forms exist. They are generated by enzymatic cleavage of membrane-associated receptors or by alternative splicing of the transmembrane region encoding exons. In the first case, these soluble receptors comprise the extracellular part of the receptor, and in the second case they include the extracellular region linked to the intracytoplasmic part of the receptor. Soluble FcγRs are found in serum (75, 76), human saliva (77), and their levels depend on the immune status of the host (78). Recombinant soluble FcγRs bind mouse and human IgG subclasses with a binding profile identical to the corresponding membrane-associated receptors and present immunomodulatory properties (79). Thus, FcγRs present a dual role in immunity. They are signal transduction units for antibodies during activation of leukocytes, and also function as regulatory molecules when produced in solution.
Soluble forms of FcγRs were first described for the mouse FcγRIIb on activated B cells (80), T cells (81), and on fibroblasts expressing a recombinant form of this receptor (80). In murine macrophages (P388D1 cell line), a soluble form of FcγRIIb was detected in tissue culture supernatants. This soluble receptor corresponded to an mRNA derived from the FcγR gene by splicing exons encoding the transmembrane and intracytoplasmic domains (82). Interestingly, B cells, which do not splice the IC1 exon, do not secrete this soluble FcγRIIb isoform (81, 83). The mouse FcγRIII has also been shown to be released in soluble form from activated NK cells (83), macrophage cell lines (82), and Langerhans cells (84).
In human cells, an mRNA splice form of FcγRII without the transmembrane region was detected by PCR in erythroleukemia (K562) and monocytic (U937) cell lines (47, 76). This soluble isoform of FcRII has been found in serum (85), and can also be released from Langerhans cells (86). In addition, a soluble FcγRIIb produced by proteolytic cleavage of the membrane-bound receptor, is released from activated B cells (87, 88). For human FcγRIII, both isoforms, FcγRIIIa and FcγRIIIb are released by proteolytic cleavage upon NK cell (89, 90) and neutrophil activation (91, 92), respectively, by various stimuli. The soluble FcγRIII is found in serum (92), in synovial fluid and saliva (77). No soluble FcγRI isoform has been reported. However, one human FcγRI gene has a stop codon at the 3′ end of the exon coding for the second extracellular domain. Thus, this gene would code for a predictive soluble low-affinity FcγR. Such a secreted receptor has not been identified (79).
The shedding of FcγRIIIa involves mainly matrix metalloproteinases (93), whereas FcγRIIIb is released by the action of both metalloproteinases and serine proteases (94, 95). Metalloproteinase inhibitors mostly blocked phorbol-12-myristate-13-acetate (PMA)-induced, but not cytochalasin B + fMLF-induced shedding of FcγRIIIb. By contrast, serine protease inhibitors mostly blocked cytochalasin B + fMLF-induced, but not PMA-induced shedding of FcγRIIIb (96). Thus, distinct types of proteolytic enzymes seem to be involved in the stimulus-induced shedding of FcγRIIIb from human neutrophils. Because, inhibitors of metalloproteinase members of the A Disintegrin And Metalloproteinase (ADAM) family appeared most efficient in preventing FcγRIIIb shedding (96), more recently it has been shown that ADAM17 is the primary protease mediating FcγRIIIb cleavage (97). ADAM17 is also involved in releasing FcγRIIIa from activated NK cells (97–100). However, in these cells, membrane-type 6 matrix metalloproteinase may also participate in FcγRIIIa shedding (101). FcγRIII presents a short membrane proximal cleavage region where three separate cleavage sites have been identified at positions alanine195/valine196, valine196/serine197, and threonine198/isoleucine199 (102).
Functions for soluble FcγRs are not completely known. However, because their levels in serum depend on the immune status of the host, these soluble receptors have a potent immunomodulatory role (78). In mouse, activation of the immune system by protein antigens such as ovalbumin and parasitic infections increases the levels of soluble FcγRs in serum (103), and in tumor-bearing animals (75). T cell-produced soluble FcγRs inhibited IgM and IgG production (79), and primary and secondary responses were inhibited by recombinant soluble FcγRII both in vitro (79) and in vivo (81, 83). Also, the intraperitoneally administration of these recombinant soluble receptors inhibited B cell responses induced via the B cell receptor, or B cell proliferation induced by mitogens (104). Purified soluble human FcγRIIIb inhibited IgM and IgG production by peripheral blood leukocytes stimulated with pokeweed mitogen (79, 105).
Despite a clear immunomodulatory role for these soluble FcγRs, a potential function for them in immunological disorders has been difficult to demonstrate. However, several examples exist where soluble FcγRs clearly change in pathological conditions. In patients with paroxysmal nocturnal hemoglobinuria, an acquired defect of hematopoietic stem cells in the synthesis or attachment of GPI-anchored proteins, a reduced expression of FcγRIIIb on neutrophils (106), and a reduced level of soluble FcγRIIIb (91, 92) have been reported. The impact for the deficiency of both membrane and soluble FcγRIIIb on the immunological disorders associated with this disease has not been established (79). In patients with multiple myeloma, a reduction of soluble FcγRIIIb correlated with disease severity (79). This reduction was associated with a slight decrease in circulating neutrophils, but not with a significant defect in soluble FcγRIIIb production by neutrophils, as detected in vitro (107). Treatment of acute immune thrombocytopenic purpura (ITP) with intravenous immunoglobulin (IVIG) induces partial or complete responses, shown by increases in platelet count. The mechanism for this clinical benefit may be the blockade of FcγRs. Platelets sensitized by IgG could not be cleared by cells of the reticuloendothelial system if their FcγRs were blocked with IVIG (108, 109). In the same way, children with ITP, who were treated with intravenous infusions of Fc fragments of IgG, showed rapid increases in platelet counts together with partial or complete responses (110). In addition, an increase in serum soluble FcγRIII concentration correlated with the rise in platelet count (110). Thus, it seems that FcγR blockade is the main mechanism of action of IVIG in ITP. However, other immunoregulatory mechanisms triggered by the presence of increased soluble FcγRIII could also be involved in the clinical benefit observed during ITP treatment (110). Also, in human immunodeficiency virus (HIV)-infected patients, a reduction of soluble FcγRIII levels in serum was reported. The reduction of soluble receptor correlated with a reduction of CD4+ T cells (111). Although, no specific changes in the number of NK cells expressing FcγRIIIa were found in this study, recently it has been proposed that NK cell activation during HIV infection leads to profound decreases in FcγRIIIa expression on NK cells (112). These results suggest that NK cell activation-induced FcγR cleavage may result in the soluble FcγRIII that associates with HIV disease progression, further suggesting a linkage between chronic NK cell activation and HIV disease progression (112).
More research on the role of these soluble FcγRs in various immunological and inflammatory disorders is needed, in order to fully understand their effects on the immune response and to use them in novel therapeutic approaches.
IgG Binding Affinity for FcγRs
As described above, there is one high-affinity Fcγ receptor, FcγRI (CD64), and two groups of low-affinity FcγRs, FcγRII and FcγRIII. The FcγRII group includes FcγRIIa, FcγRIIc, and FcγRIIb (CD32a, CD32c, and CD32b), while the FcγRIII group includes FcγRIIIa and FcγRIIIb (CD16a and CD16b). This means that a single IgG molecule cannot bind to most FcγRs. On the contrary, antigen-antibody (immune) complexes promote many low-affinity interactions between FcγR and IgG. In consequence, only immune complexes are able to induce the crosslinking of FcγR on the membrane of immune cells leading to the various antibody-mediated cell functions (Tables 2 and 3).
The Role of Particular IgG Subclass
Because, there are four subclasses of IgG (IgG1, IgG2a, IgG2b, and IgG3 in mice; and IgG1, IgG2, IgG3, and IgG4 in humans) (113), different kinds of immune complexes exist. It has been observed in many in vivo studies that the different IgG subclasses indeed can activate different cell responses. For example, in mice, IgG2b was better at eliminating B cells (114) and T cell lymphomas (115) than IgG1. Also, anti-erythrocyte antibodies of IgG2a and IgG2b subclasses were better in mediating phagocytosis of opsonized erythrocytes than antibodies of IgG1 and IgG3 subclasses (116, 117). In addition, IgG2a could induce a more severe glomerular inflammation than IgG2b, and in turn IgG2b could do it better than IgG1 (118).
All these reports confirmed that different IgG subclasses mediate different cellular responses in vivo and have suggested that these different cellular activities result from crosslinking different FcγRs. In consequence, a great interest exists for determining which type of IgG binds to which FcγR and what particular receptor is involved in mediating a certain cellular function. In humans, it was shown that most FcγRs bind primarily IgG1 and IgG3 over the other subclasses of IgG (Table 2). Similarly, in mice it was shown that IgG1 binds only to mFcγRIII, while IgG2a binds to all types of activating FcγR. IgG2b binds to mFcγRIII and mFcγRIV. IgG3 does not seem to bind significantly to any of the FcγR (14, 24, 117, 119) (Table 3).
In agreement with these data, IgG1 activity was lost in mice deficient in mFcγRIII (117, 120). For IgG2a and IgG2b, however, the correlation with particular FcγRs is not as simple. In some model systems, the activity of these IgG classes was lost in mFcγRIII-deficient mice, while it was not in others (13). Therefore, it seems clearly established that different IgG subclasses mediate different cellular responses by crosslinking different FcγRs. However, the mechanism used to generate this IgG–FcγR selectivity is not completely understood.
Obviously, this selectivity depends mainly on the affinities of different IgG subclasses to particular FcγRs. For this reason, detailed studies to measure the affinities of IgG classes to the various FcγRs have been conducted both for mice FcγRs (117) and more recently for all human FcγRs (35). Through these studies, it was found that murine IgG1 has higher affinity for the inhibitory FcγRIIb than for the activating mFcγRIII. By contrast, murine IgG2a and IgG2b have higher affinity for the activating mFcγRIV than for the inhibitory mFcγRIIb. These results suggest that for IgG1 a high threshold for activation exists, while for IgG2a a lower threshold for activation is present, and also help explain why in most in vivo responses IgG2a antibodies seem to be much more potent and effective (121, 122). In the case of humans, it was found that IgG1 and IgG3 bind to all FcγRs. IgG2 binds mainly to FcγRIIa (H131 isoform) and FcγRIIIa (V158 isoform), but not to FcγRIIIb (35). IgG4 binds to many FcγRs (35). Thus, it is clear that different IgG subclasses engage different FcγRs depending on the relative affinity of these receptors for a particular IgG class (24).
The Role of Antibody Glycosylation Pattern
All IgG antibodies have one carbohydrate (sugar) side chain added to asparagine 297 (Asp297) in their Fc portion. This N-glycosylated carbohydrate side chain is important for IgG function (123) and its deletion leads to poor binding to FcγRs (124). The N-glycans attached to the Fc portion of the IgG molecule are heterogeneous in their sugar composition (15). The heterogeneous pattern of glycosylation may contain sugar residues such as galactose, fucose, and sialic acid in straight or branching patterns (16). This heterogeneous pattern may also change with age and disease (125). For example, terminal galactose and sialic acid residues were reduced in active autoimmune disease (126, 127), while they were increased during pregnancy (128, 129). These changes in the glycosylation pattern seem to regulate IgG activity (130).
Many IgG antibodies present a fucose residue linked to an N-acetylglucosamine residue (131). The absence of this fucose residue increased the binding affinity of antibodies to human FcγRIIIa and its mouse ortholog mFcγRIV (132). Together with the increased receptor binding, these IgG antibodies also augmented ADCC activity against various tumor cells (119, 132, 133). These observations have lead to producing recombinant IgG antibodies with low fucose levels in order to increase their ADCC activity. Several of these antibodies are now in clinical trials to test their therapeutic potential (134).
IgG antibodies also have sugar side chain often terminating with sialic acid residues (135). High levels of terminal sialic acid correlate with very low affinity for FcγRs and also with reduced ADCC activity (127, 136). These sialic acid-rich antibodies were also found to preferentially bind other cellular receptors different from FcγRs. Specific ICAM-3 grabbing non-integrin-related 1 and its human ortholog dendritic cell specific ICAM-3 grabbing non-integrin were identified as receptors for sialic acid-rich IgG (137). Hence, terminal sialic acid can modify IgG activity by promoting less binding to FcγRs and more binding to other novel (type II) antibody receptors (17, 138).
Fc Receptor Signaling
All activating FcγR containing ITAM sequences seem to signal in a similar way at least at the first signaling step. After crosslinking of activating FcγRs, Src family kinases, such as Fyn, Lck, or Lyn, get activated followed by activation of Syk (spleen tyrosine kinase) family kinases. These kinases phosphorylate tyrosines within the ITAM. Phosphorylated tyrosines then become docking sites for Syk, which in turn phosphorylates multiple substrates leading to different cell responses (4, 14, 139). The Ras pathway can be activated through phosphorylation of Sos. This leads to activation of Ras, which in turn phosphorylates Raf, leading to activation of MAPK/ERK kinase (MEK) and extracellular signal-regulated kinase (ERK). This pathway is associated with activation of transcription factors such as AP-1, nuclear factor of activated T cells (NFAT), and NF-κB that control cytokine production and expression of cell survival proteins (Figure 3). Syk can also induce activation of phosphatidylinositol-3 kinase, which produces phosphatidylinositol 3,4,5-trisphosphate (PIP3). This phospholipid recruits pleckstrin homology domain-expressing proteins such as Bruton’s tyrosine kinase and other Tec family kinases involved in activation of small GTPases, such as Rho and Rac that are required for cytoskeleton remodeling. These small GTPases also impinge in activation of MEK and c-Jun N-terminal kinases, leading to nuclear factor activation (Figure 3). PIP3 also recruits phospholipase Cγ, which in turn generates diacylglycerol (DAG) and inositol triphosphate (IP3). DAG activates PKC (protein kinase C), an important serine/threonine kinase that can lead to activation of the MAP kinases ERK and p38 (Figure 3). IP3 induces release of intracellular calcium from the endoplasmic reticulum. Calcium regulates several proteins such as calmodulin and calcineurin, which are important for activation of some nuclear factors like NFAT (Figure 3). Activation of different nuclear factors induces expression of cytokines important for inflammation and immune regulation, such as IL-2, IL-6, IL-8, IL-10, tumor necrosis factor α (TNF-α), and IFN-γ (140–142) (Figure 3).
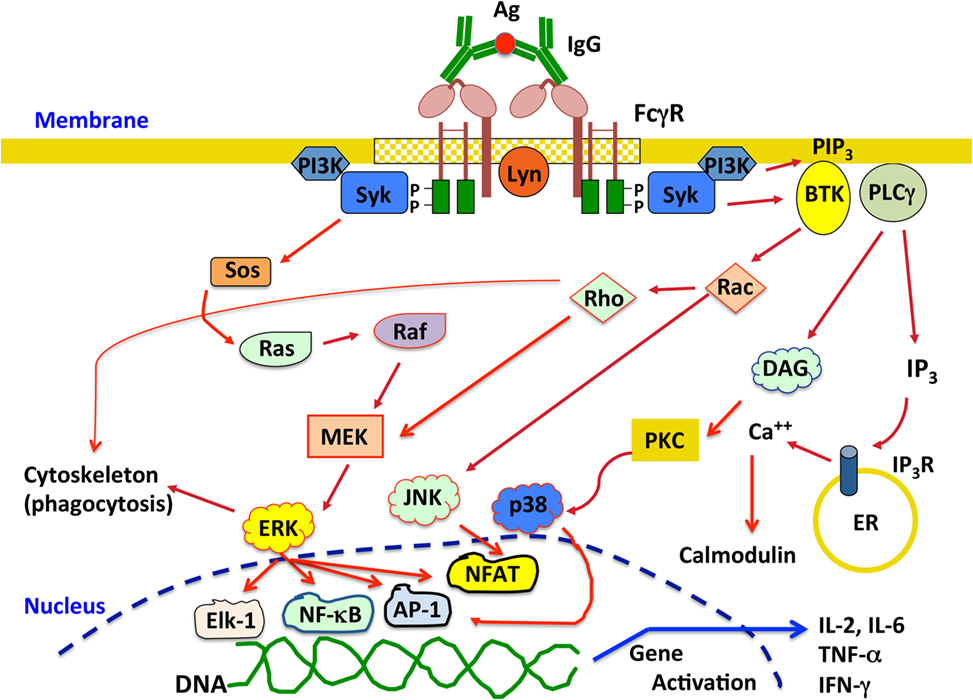
Figure 3. Overview of the signaling pathways activated upon crosslinking activating Fcγ receptors (FcγRs). Engagement of activating FcγRs by IgG immune complexes induces receptor crosslinking and phosphorylation of tyrosine residues in the immunoreceptor tyrosine-based activation motif domains by Src family kinases, for example, Lyn. Phosphorylated tyrosines then become docking sites for Syk, which in turn phosphorylates multiple substrates leading to different signaling pathways that ultimately activate various cell responses. See text for details. P represents a phosphate group; Ag, antigen; Syk, spleen tyrosine kinase; MEK, MAPK/ERK kinase; ERK, extracellular signal-regulated kinase; PI3K, phosphatidylinositol-3 kinase; PIP3, phosphatidylinositol 3,4,5-trisphosphate; BTK, Bruton’s tyrosine kinase; JNK, c-Jun N-terminal kinase; PLCγ, phospholipase Cγ; DAG, diacylglycerol; IP3, inositol triphosphate; PKC, protein kinase C; ER, endoplasmic reticulum; NFAT, nuclear factor of activated T cells; IL-2, interleukin-2; IL-6, interleukin-6; TNF-α, tumor necrosis factor α; IFN-γ, interferon-γ.
The signal transduction pathways activated by FcγRs binding to high avidity immune complexes, induce multiple cell responses including phagocytosis, respiratory burst, cytokine and chemokine production, and antibody-dependent cell-mediated cytotoxicity (ADCC) (14, 18, 41). The particular signaling molecules activated to initiate each cell response are not clearly defined in part because every cell has more than one type of FcγR and all receptors can bind more than one type of IgG. Thus, it is not clear whether each receptor leads to a particular response or the average signaling from various receptors activates a predetermined cell response. As discussed later in more detail, recent research is beginning to shade light into this issue.
Each FcγR Leads to Unique Cellular Responses
As discussed above, it is now clear that different IgG subclasses engage different FcγRs to induce particular cellular responses in vivo. However, the data published so far does not explain what cell function is activated in response to a particular type of Fcγ receptor. We can think of at least two mechanisms to generate this IgG–FcγR response selectivity: in one, each immune cell is already programmed to perform a particular cell function after FcγR crosslinking, independently of the receptor used. This does not seem likely because as mentioned before, each type of immune cell can give different responses depending on the class of IgG and also on the conditions the cell encounters (such as inflammation, etc.) (42, 72). In the second mechanism, each FcγR activates a particular signaling pathway leading to a unique cell response. This mechanism is supported by recent reports where individual FcγR were crosslinked on human neutrophils (143–147).
Human neutrophils express only two FcγRs, FcγRIIa and FcγRIIIb (45). These receptors are different in the way they are anchored to the cell membrane. FcγRIIa has a typical transmembrane and cytoplasmic tail containing an ITAM for signaling. By contrast, FcγRIIIb is a GPI-linked receptor, lacking a cytoplasmic tail, and its signaling mechanism remains unknown. The first report suggesting that these receptors could initiate distinct cellular responses came out over 20 years ago. It was reported that both FcγRs were capable of signaling, but while FcγRIIIb induced actin polymerization in a Ca2+-dependent manner, FcγRIIa did not (148). This pioneer work did not manage to maintain the idea of one receptor one response. However with time other reports have provided new evidence that supports this idea. For example, it was later reported that FcγRIIa, but not FcγRIIIb could induce an increase in L-selectin expression (149). Based on this, it was suggested that FcγRIIIb-mediated activation of circulating neutrophils could lead to a proadhesive phenotype (149). Supporting this view, it was also found that after selective engagement of each receptor with specific monoclonal antibodies, FcγRIIIb, but not FcγRIIa, was able to activate β1 integrins (143). This activation was not due to an increase in integrin expression but rather to an increase in binding affinity for integrin ligands such as fibronectin (143). By contrast, when the major cell response of neutrophils, arguably phagocytosis (150, 151), was examined with receptor specific opsonized beads, FcγRIIa was the predominant FcγR mediating this response. FcγRIIIb contribution to phagocytosis was minimal (145). Thus, at least in human neutrophils each Fcγ receptor is used to activate unique cell responses. FcγRIIa induces mainly phagocytosis, while FcγRIIIb promotes an adhesive phenotype via activation of β1 integrins (Figure 4).
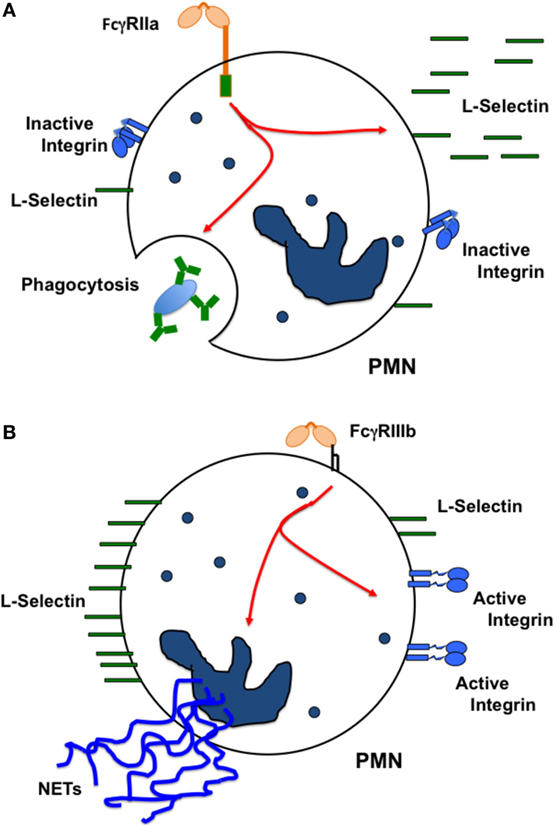
Figure 4. Each FcγR induces particular cellular responses. In human neutrophils (PMN), (A) FcγRIIa causes L-selectin shedding from the cell membrane, and also activates efficient phagocytosis. By contrast, (B) FcγRIIIb does not cause L-selectin shedding, but stimulates activation of β1 integrins promoting in this way a proadhesive phenotype. FcγRIIIb also induces formation of neutrophil extracellular traps (NETs). The oval represents an IgG-opsonized particle.
In addition, it was recently found that FcγRIIIb signaling to the neutrophil nucleus was much more efficient than FcγRIIa signaling. FcγRIIIb, but not FcγRIIa, promoted a robust increase in phosphorylated ERK in the nucleus, and also efficient phosphorylation of the nuclear factor Elk-1 (144) (Figure 5). Interestingly, FcγRIIa also induced phosphorylation of ERK in the cytosol (144, 152), but this active ERK seems to function mainly in enhancing phagocytosis and not in nuclear signaling (Figure 5). An important point still unresolved is the actual FcγRIIIb signaling pathway. For FcγRIIa, the FcγR signaling pathway resembles the classical ITAM-mediated pathway (4, 14, 139, 153) (Figure 3), while for FcγRIIIb, the signaling pathway remains a mystery and further research is needed in this area (Figure 5).
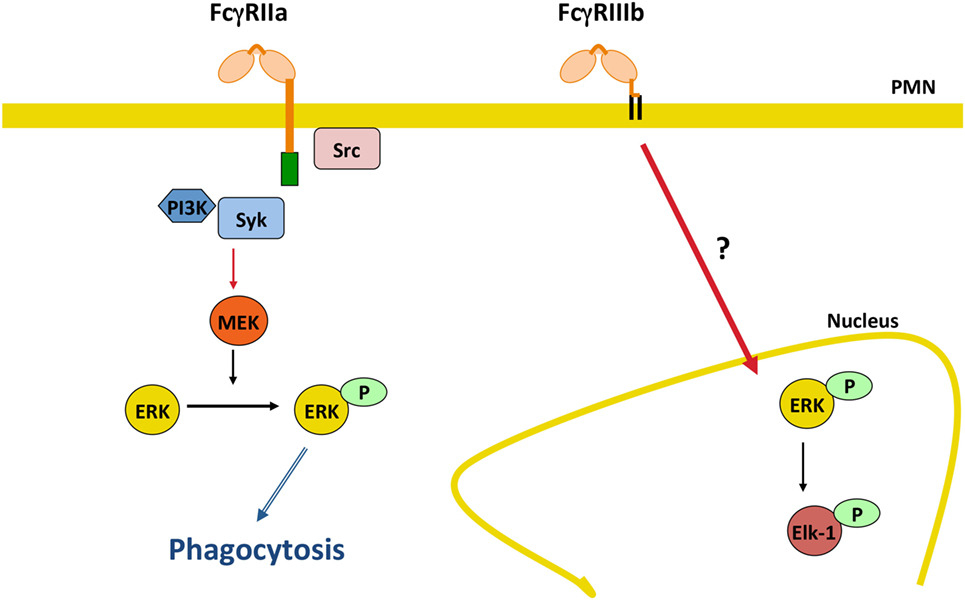
Figure 5. FcγRIIIb initiates signaling pathways to the nucleus. In human neutrophils (PMN), FcγRIIa activates the classical immunoreceptor tyrosine-based activation motif-mediated signaling pathway leading to efficient phagocytosis. By contrast, FcγRIIIb induces a robust increase in phosphorylated ERK in the nucleus, and also efficient phosphorylation of the nuclear factor Elk-1. The FcγRIIIb signaling pathway remains a mystery and further future research is needed in this area. P represents a phosphate group; Syk, spleen tyrosine kinase; PI3K, phosphatidylinositol-3 kinase; MEK, ERK kinase; ERK, extracellular signal-regulated kinase.
Another important cellular function of neutrophils to kill microbes is the formation of neutrophil extracellular traps (NETs) (154, 155). These structures are induced by several pathogens, including virus, bacteria, fungi, and parasites (156). Also, pro-inflammatory stimuli such as lipopolysaccharide, TNF-α, and PMA are efficient inducers of NETs (157). Because, antigen-antibody complexes are also capable of inducing NET formation (158, 159), it was clear that FcγRs were involved in NET formation. Recently, it was reported that FcγRIIIb is the receptor responsible for NET formation in response to immobilized immune complexes (160). In this study, NET formation induced by immobilized immune complexes was blocked by antibodies against FcγRIIIb, but not by antibodies against FcγRIIa (160), indicating that solely FcγRIIIb mediates NET release. Moreover, by direct crosslinking of each type of FcγR with specific monoclonal antibodies it was also confirmed that only FcγRIIIb is capable of inducing NET formation (147, 161). Although, the initial signaling mechanism for FcγRIIIb remains unknown, the signaling pathway for this cell response has been shown to involve the Syk and TAK1 kinases, as well as the MEK/ERK cascade (Figure 6) (161). Because FcγRIIIb is a GPI-linked receptor it is not clear how it can connect to the ERK pathway. However, it is known that GPI-linked proteins concentrate in lipid rafts on the cell membrane. In these rafts many signaling molecules such as Src family tyrosine kinases concentrate, and it is possible that FcγRIIIb upon ligand binding can connect somehow with these kinases and activate Syk. A possible mechanism is the binding of the receptor, within the lipid rafts, to a putative ITAM-containing molecule (151). After Syk activation, a signaling molecular complex can be organized leading to activation of other kinases such as TAK1 (Figure 6). Many steps are still unknown and future research will help in elucidate this signaling pathway.
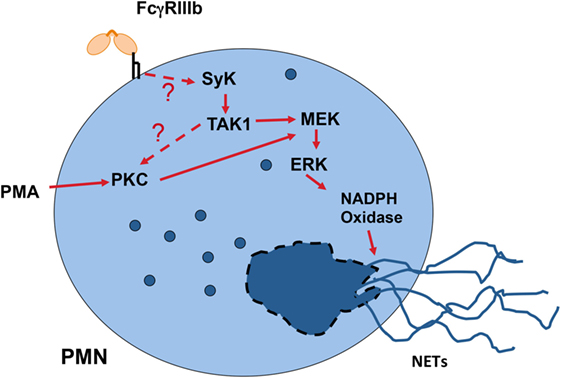
Figure 6. Model for FcγRIIIb signaling to induce neutrophil extracellular trap (NET) formation. In human neutrophils (PMN), crosslinking FcγRIIIb results in activation of Syk (spleen tyrosine kinase) and TAK1 (transforming growth factor-β-activated kinase 1). These kinases lead to activation of PKC (protein kinase C) and the MEK/ERK signaling pathway to generate the production of reactive oxygen species via NADPH oxidase and to induce NETs formation. The question marks indicate that the mechanism for FcγRIIIb-induced Syk activation is not known, and that it is not clear whether TAK1 functions upstream of PKC. Model based on Alemán et al. (161).
Taken together, these reports strongly support the hypothesis that each FcγR is capable of initiating particular signaling pathways that lead to unique cell responses. This information would certainly be very helpful in the future for controlling some of the cellular responses in clinical settings. For example, during a strong infection efficient phagocytosis may be desirable. Considering that IgG2 displays a stronger binding to FcγRIIa than to FcγRIIIb (35) (Table 2), one could predict that antibodies of the IgG2 subclass would be much better at inducing phagocytosis by neutrophils. Thus, inducing the production of IgG2 antibodies against certain pathogens, would very likely improve the phagocytosis response against them. Following the same idea, new monoclonal antibodies against tumors have been developed for recognition of malignant cells. Because on NK cells the only activating Fcγ receptor is FcγRIIIa, finding antibodies with better binding (higher affinity) to FcγRIIIa should improve the activation of ADCC. Indeed, this has been shown to be the case for several anti-tumor antibodies (12, 162, 163). This means that, when we know what is the particular cellular response initiated by each FcγR on an immune cell, we could find ways to improve the IgG binding interaction and enhance the response, or vice versa to block the IgG binding interaction and in consequence inhibit the response.
Conclusion
Fcγ receptors expressed in many immune cells are capable of activating different cellular responses important not only for controlling microbial infections but also for regulating immunity. Different subclasses of IgG antibodies bind the various FcγRs with different affinities. These FcγRs are expressed on a wide variety of leukocytes and are capable of activating when crosslinked with immune complexes, different cellular responses of great importance for host defense and for immune regulation. Recent evidence suggests that a specific Fcγ receptor activates particular cell responses. At least for the human neutrophil it is clear that FcγRIIa activates efficient phagocytosis, while FcγRIIIb signals to the nucleus for nuclear factor activation and NETs formation. Therefore, in principle, a particular cell response could be induced or inhibited by engaging or blocking the corresponding FcγR. For example, using IgG2 antibodies a better phagocytosis response should be generated in neutrophils. Because, FcγRs are responsible not only of initiating anti-microbial responses, but also of controlling the intensity of the immune response, there is growing interest in revealing what specific Fcγ receptor activates a particular cell response. Information similar to the one described for neutrophil FcγRs on other immune cells, such as monocytes or dendritic cells, is not available. We will certainly see in the near future much more research in this area.
Author Contributions
CR conceived the issues which formed the content of the manuscript and wrote the manuscript.
Conflict of Interest Statement
The author declares that the research was conducted in the absence of any commercial or financial relationships that could be construed as a potential conflict of interest.
Funding
Research in the authors’ laboratory was supported by grant 254434 from Consejo Nacional de Ciencia y Tecnología, Mexico.
References
1. Ballow M. Historical perspectives in the diagnosis and treatment of primary immune deficiencies. Clin Rev Allergy Immunol (2014) 46:101–3. doi:10.1007/s12016-013-8384-9
2. Hangartner L, Zinkernagel RM, Hengartner H. Antiviral antibody responses: the two extremes of a wide spectrum. Nat Rev Immunol (2006) 6:231–43. doi:10.1038/nri1783
3. Tohyama Y, Yamamura H. Complement-mediated phagocytosis – the role of Syk. IUBMB Life (2006) 58:304–8. doi:10.1080/15216540600746377
4. Rosales C. Fc receptor and integrin signaling in phagocytes. Signal Transduct (2007) 7:386–401. doi:10.1002/sita.200700141
5. Heyman B. Regulation of antibody responses via antibodies, complement, and Fc receptors. Annu Rev Immunol (2000) 18:709–37. doi:10.1146/annurev.immunol.18.1.709
6. Nimmerjahn F, Ravetch J. Antibodies, Fc receptors and cancer. Curr Opin Immunol (2007) 19:239–45. doi:10.1016/j.coi.2007.01.005
7. Powell MS, Hogarth PM. Fc receptors. Adv Exp Med Biol (2008) 640:22–34. doi:10.1007/978-0-387-09789-3_3
8. Rosales C, Uribe-Querol E. Antibody – Fc receptor interactions in antimicrobial functions. Curr Immunol Rev (2013) 9:44–55. doi:10.2174/1573395511309010006
9. Nimmerjahn F, Ravetch JV. FcγRs in health and disease. Curr Top Microbiol Immunol (2011) 350:105–25. doi:10.1007/82_2010_86
10. Sondermann P. The FcγR/IgG interaction as target for the treatment of autoimmune diseases. J Clin Immunol (2016) 36:95–9. doi:10.1007/s10875-016-0272-7
11. Yu X, Lazarus AH. Targeting FcγRs to treat antibody-dependent autoimmunity. Autoimmun Rev (2016) 15:510–2. doi:10.1016/j.autrev.2016.02.006
12. Sondermann P, Szymkowski DE. Harnessing Fc receptor biology in the design of therapeutic antibodies. Curr Opin Immunol (2016) 40:78–87. doi:10.1016/j.coi.2016.03.005
13. Nimmerjahn F, Ravetch J. Fcγ receptors: old friends and new family members. Immunity (2006) 24:19–28. doi:10.1016/j.immuni.2005.11.010
14. Rosales C, Uribe-Querol E. Fc receptors: cell activators of antibody functions. Adv Biosci Biotechnol (2013) 4:21–33. doi:10.4236/abb.2013.44A004
15. Arnold J, Wormald M, Sim R, Rudd P, Dwek R. The impact of glycosylation on the biological function and structure of human immunoglobulins. Annu Rev Immunol (2007) 25:21–50. doi:10.1146/annurev.immunol.25.022106.141702
16. Raju T. Terminal sugars of Fc glycans influence antibody effector functions of IgGs. Curr Opin Immunol (2008) 20:471–8. doi:10.1016/j.coi.2008.06.007
17. Anthony RM, Wermeling F, Ravetch JV. Novel roles of the IgG Fc glycan. Ann N Y Acad Sci (2012) 1253:170–80. doi:10.1111/j.1749-6632.2011.06305.x
18. Bruhns P. Properties of mouse and human IgG receptors and their contribution to disease models. Blood (2012) 119:5640–9. doi:10.1182/blood-2012-01-380121
19. Mancardi D, Iannascoli B, Hoos S, England P, Daëron M, Bruhns P. FcγRIV is a mouse IgE receptor that resembles macrophage FcεRI in humans and promotes IgE-induced lung inflammation. J Clin Invest (2008) 118:3738–50. doi:10.1172/JCI36452
20. Roopenian DC, Akilesh S. FcRn: the neonatal Fc receptor comes of age. Nat Rev Immunol (2007) 7:715–25. doi:10.1038/nri2155
21. Stapleton NM, Einarsdóttir HK, Stemerding AM, Vidarsson G. The multiple facets of FcRn in immunity. Immunol Rev (2015) 268:253–68. doi:10.1111/imr.12331
22. Mallery DL, McEwan WA, Bidgood SR, Towers GJ, Johnson CM, James LC. Antibodies mediate intracellular immunity through tripartite motif-containing 21 (TRIM21). Proc Natl Acad Sci U S A (2010) 107:9985–19990. doi:10.1073/pnas.1014074107
23. Foss S, Watkinson R, Sandlie I, James LC, Andersen JT. TRIM21: a cytosolic Fc receptor with broad antibody isotype specificity. Immunol Rev (2015) 268:328–39. doi:10.1111/imr.12363
24. Bruhns P, Jönsson F. Mouse and human FcR effector functions. Immunol Rev (2015) 268:25–51. doi:10.1111/imr.12350
25. Daëron M. Fc receptor biology. Annu Rev Immunol (1997) 15:203–34. doi:10.1146/annurev.immunol.15.1.203
26. Ravetch JV, Bolland S. IgG Fc receptors. Annu Rev Immunol (2001) 19:275–90. doi:10.1146/annurev.immunol.19.1.275
27. Wilson TJ, Fuchs A, Colonna M. Cutting edge: human FcRL4 and FcRL5 are receptors for IgA and IgG. J Immunol (2012) 188:4741–5. doi:10.4049/jimmunol.1102651
28. Hatzivassiliou G, Miller I, Takizawa J, Palanisamy N, Rao PH, Iida S, et al. IRTA1 and IRTA2, novel immunoglobulin superfamily receptors expressed in B cells and involved in chromosome 1q21 abnormalities in B cell malignancy. Immunity (2001) 14:277–89. doi:10.1016/S1074-7613(01)00109-1
29. Falini B, Tiacci E, Pucciarini A, Bigerna B, Kurth J, Hatzivassiliou G, et al. Expression of the IRTA1 receptor identifies intraepithelial and subepithelial marginal zone B cells of the mucosa-associated lymphoid tissue (MALT). Blood (2003) 102:3684–92. doi:10.1182/blood-2003-03-0750
30. Ehrhardt GR, Hsu JT, Gartland L, Leu CM, Zhang S, Davis RS, et al. Expression of the immunoregulatory molecule FcRH4 defines a distinctive tissue-based population of memory B cells. J Exp Med (2005) 202:783–91. doi:10.1084/jem.20050879
31. Yoshida M, Claypool SM, Wagner JS, Mizoguchi E, Mizoguchi A, Roopenian DC, et al. Human neonatal Fc receptor mediates transport of IgG into luminal secretions for delivery of antigens to mucosal dendritic cells. Immunity (2004) 20:769–83. doi:10.1016/j.immuni.2004.05.007
32. Zhu X, Meng G, Dickinson BL, Li X, Mizoguchi E, Miao L, et al. MHC class I-related neonatal Fc receptor for IgG is functionally expressed in monocytes, intestinal macrophages, and dendritic cells. J Immunol (2001) 166:3266–76. doi:10.4049/jimmunol.166.5.3266
33. Vidarsson G, Stemerding AM, Stapleton NM, Spliethoff SE, Janssen H, Rebers FE, et al. FcRn: an IgG receptor on phagocytes with a novel role in phagocytosis. Blood (2006) 108:3573–9. doi:10.1182/blood-2006-05-024539
34. Ravetch JV. Fc receptors. 5th ed. In: Paul WE, editor. Fundamental Immunology. Philadelphia, PA: Lippincott Williams and Wilkins (2003). p. 631–84.
35. Bruhns P, Iannascoli B, England P, Mancardi D, Fernandez N, Jorieux S, et al. Specificity and affinity of human Fcγ receptors and their polymorphic variants for human IgG subclasses. Blood (2009) 113:3716–25. doi:10.1182/blood-2008-09-179754
36. Underhill DM, Goodridge HS. The many faces of ITAMs. Trends Immunol (2007) 28:66–73. doi:10.1016/j.it.2006.12.004
37. Daëron M, Lesourne R. Negative signaling in Fc receptor complexes. Adv Immunol (2006) 89:39–86. doi:10.1016/S0065-2776(05)89002-9
38. Stefanescu RN, Olferiev M, Liu Y, Pricop L. Inhibitory Fcγ receptors: from gene to disease. J Clin Immnol (2004) 24:315–26. doi:10.1023/B:JOCI.0000029105.47772.04
39. Baerenwaldt A, Nimmerjahn F. Immune regulation: FcγRIIB – regulating the balance between protective and autoreactive immune responses. Immunol Cell Biol (2008) 86:482–4. doi:10.1038/icb.2008.34
40. Boruchov A, Heller G, Veri M, Bonvini E, Ravetch J, Young J. Activating and inhibitory IgG Fc receptors on human DCs mediate opposing functions. J Clin Invest (2005) 115:2914–23. doi:10.1172/JCI24772
41. Nimmerjahn F, Ravetch J. Fcγ receptors as regulators of immune responses. Nat Rev Immunol (2008) 8:34–47. doi:10.1038/nri2206
42. Nimmerjahn F, Ravetch JV. Antibody-mediated modulation of immune responses. Immunol Rev (2010) 236:265–75. doi:10.1111/j.1600-065X.2010.00910.x
43. Lehmann B, Schwab I, Böhm S, Lux A, Biburger M, Nimmerjahn F. FcγRIIB: a modulator of cell activation and humoral tolerance. Expert Rev Clin Immunol (2012) 8:243–54. doi:10.1586/eci.12.5
44. Meknache N, Jönsson F, Laurent J, Guinnepain MT, Daëron M. Human basophils express the glycosylphosphatidylinositol-anchored low-affinity IgG receptor FcγRIIIB (CD16B). J Immunol (2009) 182:2542–50. doi:10.4049/jimmunol.0801665
45. Willcocks LC, Smith KG, Clatworthy MR. Low-affinity Fcγ receptors, autoimmunity and infection. Expert Rev Mol Med (2009) 11:e24. doi:10.1017/S1462399409001161
46. van Sorge NM, van der Pol WL, van de Winkel JG. FcγR polymorphisms: implications for function, disease susceptibility and immunotherapy. Tissue Antigens (2003) 61:189–202. doi:10.1034/j.1399-0039.2003.00037.x
47. Warmerdam PA, van de Winkel JG, Gosselin EJ, Capel PJ. Molecular basis for a polymorphism of human Fcγ receptor II (CD32). J Exp Med (1990) 172:19–25. doi:10.1084/jem.172.1.19
48. Osborne JM, Chacko GW, Brandt JT, Anderson CL. Ethnic variation in frequency of an allelic polymorphism of human FcγRIIA determined with allele specific oligonucleotide probes. J Immunol Methods (1994) 173:207–17. doi:10.1016/0022-1759(94)90299-2
49. Wu J, Edberg JC, Redecha PB, Bansal V, Guyre PM, Coleman K, et al. A novel polymorphism of FcγRIIIa (CD16) alters receptor function and predisposes to autoimmune disease. J Clin Invest (1997) 100:1059–70. doi:10.1172/JCI119616
50. Koene HR, Kleijer M, Swaak AJG, Sullivan KE, Bijl M, Petri MA, et al. The FcγRIIIA-158F allele is a risk factor for systemic lupus erythematosus. Arthritis Rheum (1998) 41:1813–8. doi:10.1002/1529-0131(199810)41:10<1813::AID-ART13>3.3.CO;2-Y
51. Huizinga TWJ, Kleijer M, Tetteroo PAT, Roos D, von dem Borne AE. Biallelic neutrophil Na-antigen system is associated with a polymorphism on the phospho-inositol-linked Fcγ receptor III (CD16). Blood (1990) 75:213–7.
52. Ory PA, Clark MR, Kwoh EE, Clarkson SB, Goldstein IM. Sequences of complementary DNAs that encode the NA1 and NA2 forms of Fc receptor III on human neutrophils. J Clin Invest (1989) 84:1688–91. doi:10.1172/JCI114350
53. Salmon JE, Edberg JC, Kimberly RP. Fcγ receptor III on human neutrophils. Allelic variants have functionally distinct capacities. J Clin Invest (1990) 85:1287–95. doi:10.1172/JCI114566
54. Hatta Y, Tsuchiya N, Ohashi J, Matsushita M, Fujiwara K, Hagiwara K, et al. Association of Fcγ receptor IIIB, but not of Fcγ receptor IIA and IIIA, polymorphisms with systemic lupus erythematosus in Japanese. Genes Immun (1999) 1:53–60. doi:10.1038/sj.gene.6363639
55. Bux J, Stein E-L, Bierling P, Fromont P, Clay M, Stroncek D, et al. Characterization of a new alloantigen (SH) on the human neutrophil Fcγ receptor IIIB. Blood (1997) 89:1027–34.
56. Aitman TJ, Dong R, Vyse TJ, Norsworthy PJ, Johnson MD, Smith J, et al. Copy number polymorphism in Fcgr3 predisposes to glomerulonephritis in rats and humans. Nature (2006) 439:851–5. doi:10.1038/nature04489
57. Duits AJ, Bootsma H, Derksen RH, Spronk PE, Kater L, Kallenberg CG, et al. Skewed distribution of IgG Fc receptor IIa (CD32) polymorphism is associated with renal disease in systemic lupus erythematosus patients. Arthritis Rheum (1995) 38:1832–6. doi:10.1002/art.1780381217
58. Sanders LA, van de Winkel JG, Rijkers GT, Voorhorst-Ogink MM, de Haas M, Capel PJ, et al. Fcγ receptor IIa (CD32) heterogeneity in patients with recurrent bacterial respiratory tract infections. J Infect Dis (1994) 170:854–61. doi:10.1093/infdis/170.4.854
59. Lehrnbecher T, Foster CB, Zhu S, Leitman SF, Goldin LR, Huppi K, et al. Variant genotypes of the low-affinity Fcγ receptors in two control populations and a review of low-affinity Fcγ receptor polymorphisms in control and disease populations. Blood (1999) 94:4220–32.
60. Nieto A, Caliz R, Pascual M, Mataran L, Garcia S, Martin J. Involvement of Fcγ receptor IIIA genotypes in susceptibility to rheumatoid arthritis. Arthritis Rheum (2000) 43:735–9. doi:10.1002/1529-0131(200004)43:4<735::AID-ANR3>3.0.CO;2-Q
61. Edberg JC, Wainstein E, Wu J, Csernok E, Sneller MC, Hoffman GS, et al. Analysis of FcγRII gene polymorphisms in Wegener’s granulomatosis. Exp Clin Immunogenet (1997) 14:183–95.
62. Tse WY, Abadeh S, Jefferis R, Savage CO, Adu D. Neutrophil FcγRIIIb allelic polymorphism in anti-neutrophil cytoplasmic antibody (ANCA)-positive systemic vasculitis. Clin Exp Immunol (2000) 119:574–7. doi:10.1046/j.1365-2249.2000.01182.x
63. Ravetch JV, Kinet JP. Fc receptors. Annu Rev Immunol (1991) 9:457–92. doi:10.1146/annurev.iy.09.040191.002325
64. Okayama Y, Kirshenbaum AS, Metcalfe DD. Expression of a functional high-affinity IgG receptor, FcγRI, on human mast cells: up-regulation by IFN-γ. J Immunol (2000) 164:4332–9. doi:10.4049/jimmunol.164.8.4332
65. Veri MC, Gorlatov S, Li H, Burke S, Johnson S, Stavenhagen J, et al. Monoclonal antibodies capable of discriminating the human inhibitory Fcγ-receptor IIB (CD32B) from the activating Fcγ-receptor IIA (CD32A): biochemical, biological and functional characterization. Immunology (2007) 121:392–404. doi:10.1111/j.1365-2567.2007.02588.x
66. Cassard L, Jönsson F, Arnaud S, Daëron M. Fcγ receptors inhibit mouse and human basophil activation. J Immunol (2012) 189:2995–3006. doi:10.4049/jimmunol.1200968
67. Magnusson SE, Engström M, Jacob U, Ulfgren AK, Kleinau S. High synovial expression of the inhibitory FcγRIIb in rheumatoid arthritis. Arthritis Res Ther (2007) 9:R51. doi:10.1186/ar2206
68. Anderson CL, Ganesan LP, Robinson JM. The biology of the classical Fcγ receptors in non-hematopoietic cells. Immunol Rev (2015) 268:236–40. doi:10.1111/imr.12335
69. Mohamed HA, Mosier DR, Zou LL, Siklós L, Alexianu ME, Engelhardt JI, et al. Immunoglobulin Fcγ receptor promotes immunoglobulin uptake, immunoglobulin-mediated calcium increase, and neurotransmitter release in motor neurons. J Neurosci Res (2002) 69:110–6. doi:10.1002/jnr.10271
70. Nakamura K, Hirai H, Torashima T, Miyazaki T, Tsurui H, Xiu Y, et al. CD3 and immunoglobulin G Fc receptor regulate cerebellar functions. Mol Cell Biol (2007) 27:5128–34. doi:10.1128/MCB.01072-06
71. Ganesan LP, Kim J, Wu Y, Mohanty S, Phillips GS, Birmingham DJ, et al. FcγRIIb on liver sinusoidal endothelium clears small immune complexes. J Immunol (2012) 189:4981–8. doi:10.4049/jimmunol.1202017
72. Pricop L, Redecha P, Teillaud J-L, Frey J, Fridman WH, Sautès-Fridman C, et al. Differential modulation of stimulatory and inhibitory Fcγ receptors on human monocytes by Th1 and Th2 cytokines. J Immunol (2001) 166:531–7. doi:10.4049/jimmunol.166.1.531
73. Schmidt R, Gessner J. Fc receptors and their interaction with complement in autoimmunity. Immunol Lett (2005) 100:56–67. doi:10.1016/j.imlet.2005.06.022
74. Tridandapani S, Wardrop R, Baran CP, Wang Y, Opalek JM, Caligiuri MA, et al. TGF-β1 suppresses myeloid Fcγ receptor function by regulating the expression and function of the common γ-subunit. J Immunol (2003) 170:4572–7. doi:10.4049/jimmunol.170.9.4572
75. Lynch A, Tartour E, Teillaud JL, Asselain B, Fridman WH, Sautès C. Increased levels of soluble low-affinity Fc gamma receptors (IgG-binding factors) in the sera of tumour-bearing mice. Clin Exp Immunol (1992) 87:208–14. doi:10.1111/j.1365-2249.1992.tb02976.x
76. Rappaport EF, Cassel DL, Walterhouse DO, McKenzie SE, Surrey S, Keller MA, et al. A soluble form of the human Fc receptor Fc gamma RIIA: cloning, transcript analysis and detection. Exp Hematol (1993) 21:689–96.
77. Sautès C, Teillaud C, Mazières N, Tartour E, Bouchard C, Galinha A, et al. Soluble Fc gamma R (sFc gamma R): detection in biological fluids and production of a murine recombinant sFc gamma R biologically active in vitro and in vivo. Immunobiology (1992) 185:207–21. doi:10.1016/S0171-2985(11)80642-X
78. Teillaud JL, Bouchard C, Astier A, Teillaud C, Tartour E, Michon J, et al. Natural and recombinant soluble low-affinity Fc gamma R: detection, purification, and functional activities. Immunomethods (1994) 4:48–64. doi:10.1006/immu.1994.1007
79. Fridman WH, Teillaud JL, Bouchard C, Teillaud C, Astier A, Tartour E, et al. Soluble Fc gamma receptors. J Leukoc Biol (1993) 54:504–12.
80. Sautès C, Varin N, Teillaud C, Daëron M, Even J, Hogarth PM, et al. Soluble Fc γ receptors II (Fc γ RII) are generated by cleavage of membrane Fc γ RII. Eur J Immunol (1991) 21:231–4. doi:10.1002/eji.1830210135
81. Sautès C, Mazières N, Galinha A, Tartour E, Bonnerot C, Amigorena S, et al. Murine soluble Fc gamma receptors/IgG-binding factors (IgG-BF): analysis of the relation to Fc gamma RII and production of milligram quantities of biologically active recombinant IgG-BF. Immunol Res (1992) 11:181–90. doi:10.1007/BF02919125
82. Tartour E, de la Salle H, de la Salle C, Teillaud C, Camoin L, Galinha A, et al. Identification, in mouse macrophages and in serum, of a soluble receptor for the Fc portion of IgG (Fc gamma R) encoded by an alternatively spliced transcript of the Fc gamma RII gene. Int Immunol (1993) 5:859–68. doi:10.1093/intimm/5.8.859
83. Fridman WH, Bonnerot C, Daeron M, Amigorena S, Teillaud JL, Sautes C. Structural bases of Fc gamma receptor functions. Immunol Rev (1992) 125:49–76. doi:10.1111/j.1600-065X.1992.tb00625.x
84. Esposito-Farese ME, Sautès C, de la Salle H, Latour S, Bieber T, de la Salle C, et al. Membrane and soluble Fc gamma RII/III modulate the antigen-presenting capacity of murine dendritic epidermal Langerhans cells for IgG-complexed antigens. J Immunol (1995) 155:1725–36.
85. Astier A, de la Salle H, Moncuit J, Freund M, Cazenave JP, Fridman WH, et al. Detection and quantification of secreted soluble Fc gamma RIIA in human sera by an enzyme-linked immunosorbent assay. J Immunol Methods (1993) 166:1–10. doi:10.1016/0022-1759(93)90323-Y
86. de la Salle C, Esposito-Farese ME, Bieber T, Moncuit J, Morales M, Wollenberg A, et al. Release of soluble Fc gamma RII/CD32 molecules by human Langerhans cells: a subtle balance between shedding and secretion? J Invest Dermatol (1992) 99:15S–7S. doi:10.1111/1523-1747.ep12668250
87. Sármay G, Rozsnyay Z, Gergely J. Fc gamma RII expression and release on resting and activated human B lymphocytes. Mol Immunol (1990) 27:1195–200. doi:10.1016/0161-5890(90)90022-R
88. Gergely J, Sarmay G. B-cell activation-induced phosphorylation of Fc gamma RII: a possible prerequisite of proteolytic receptor release. Immunol Rev (1992) 125:5–19. doi:10.1111/j.1600-065X.1992.tb00622.x
89. Lanier LL, Phillips JH, Testi R. Membrane anchoring and spontaneous release of CD16 (FcR III) by natural killer cells and granulocytes. Eur J Immunol (1989) 19:775–8. doi:10.1002/eji.1830190431
90. Harrison D, Phillips JH, Lanier LL. Involvement of a metalloprotease in spontaneous and phorbol ester-induced release of natural killer cell-associated Fc gamma RIII (CD16-II). J Immunol (1991) 147:3459–65.
91. Huizinga TW, van der Schoot CE, Jost C, Klaassen R, Kleijer M, von dem Borne AE, et al. The PI-linked receptor FcRIII is released on stimulation of neutrophils. Nature (1988) 333:667–9. doi:10.1038/333667a0
92. Huizinga TW, de Haas M, Kleijer M, Nuijens JH, Roos D, von dem Borne AE. Soluble Fc gamma receptor III in human plasma originates from release by neutrophils. J Clin Invest (1990) 86:416–23. doi:10.1172/JCI114727
93. Mota G, Moldovan I, Calugaru A, Hirt M, Kozma E, Galatiuc C, et al. Interaction of human immunoglobulin G with CD16 on natural killer cells: ligand clearance, FcgammaRIIIA turnover and effects of metalloproteinases on FcgammaRIIIA-mediated binding, signal transduction and killing. Scand J Immunol (2004) 59:278–84. doi:10.1111/j.0300-9475.2004.01398.x
94. Bazil V, Strominger JL. Metalloprotease and serine protease are involved in cleavage of CD43, CD44, and CD16 from stimulated human granulocytes. Induction of cleavage of L-selectin via CD16. J Immunol (1994) 152:1314–22.
95. Galon J, Moldovan I, Galinha A, Provost-Marloie MA, Kaudewitz H, Roman-Roman S, et al. Identification of the cleavage site involved in production of plasma soluble Fc gamma receptor type III (CD16). Eur J Immunol (1998) 28:2101–7. doi:10.1002/(SICI)1521-4141(199807)28:07<2101::AID-IMMU2101>3.0.CO;2-W
96. Middelhoven PJ, Van Buul JD, Hordijk PL, Roos D. Different proteolytic mechanisms involved in Fc gamma RIIIb shedding from human neutrophils. Clin Exp Immunol (2001) 125:169–75. doi:10.1046/j.1365-2249.2001.01548.x
97. Wang Y, Wu J, Newton R, Bahaie NS, Long C, Walcheck B. ADAM17 cleaves CD16b (FcγRIIIb) in human neutrophils. Biochim Biophys Acta (2013) 1833:680–5. doi:10.1016/j.bbamcr.2012.11.027
98. Romee R, Foley B, Lenvik T, Wang Y, Zhang B, Ankarlo D, et al. NK cell CD16 surface expression and function is regulated by a disintegrin and metalloprotease-17 (ADAM17). Blood (2013) 121:3599–608. doi:10.1182/blood-2012-04-425397
99. Wiernik A, Foley B, Zhang B, Verneris MR, Warlick E, Gleason MK, et al. Targeting natural killer cells to acute myeloid leukemia in vitro with a CD16 x 33 bispecific killer cell engager and ADAM17 inhibition. Clin Cancer Res (2013) 19:3844–55. doi:10.1158/1078-0432.CCR-13-0505
100. Lajoie L, Congy-Jolivet N, Bolzec A, Gouilleux-Gruart V, Sicard E, Sung HC, et al. ADAM17-mediated shedding of FcγRIIIA on human NK cells: identification of the cleavage site and relationship with activation. J Immunol (2014) 192:741–51. doi:10.4049/jimmunol.1301024
101. Peruzzi G, Femnou L, Gil-Krzewska A, Borrego F, Weck J, Krzewski K, et al. Membrane-type 6 matrix metalloproteinase regulates the activation-induced downmodulation of CD16 in human primary NK cells. J Immunol (2013) 191:1883–94. doi:10.4049/jimmunol.1300313
102. Jing Y, Ni Z, Wu J, Higgins L, Markowski TW, Kaufman DS, et al. Identification of an ADAM17 cleavage region in human CD16 (FcγRIII) and the engineering of a non-cleavable version of the receptor in NK cells. PLoS One (2015) 10:e0121788. doi:10.1371/journal.pone.0121788
103. Daëron M, Sautès C, Bonnerot C, Blank U, Varin N, Even J, et al. Murine type II Fc gamma receptors and IgG-binding factors. Chem Immunol (1989) 47:21–78. doi:10.1159/000318912
104. Bouchard C, Galinha A, Tartour E, Fridman WH, Sautès C. A transforming growth factor beta-like immunosuppressive factor in immunoglobulin G-binding factor. J Exp Med (1995) 182:1717–26. doi:10.1084/jem.182.6.1717
105. Teillaud C, Galon J, Zilber MT, Mazières N, Spagnoli R, Kurrle R, et al. Soluble CD16 binds peripheral blood mononuclear cells and inhibits pokeweed-mitogen-induced responses. Blood (1993) 82:3081–90.
106. Selvaraj P, Rosse WF, Silber R, Springer TA. The major Fc receptor in blood has a phosphatidylinositol anchor and is deficient in paroxysmal nocturnal haemoglobinuria. Nature (1988) 333:565–7. doi:10.1038/333565a0
107. Moldovan I, Galon J, Maridonneau-Parini I, Roman Roman S, Mathiot C, Fridman WH, et al. Regulation of production of soluble Fc gamma receptors type III in normal and pathological conditions. Immunol Lett (1999) 68:125–34. doi:10.1016/S0165-2478(99)00041-3
108. Baerenwaldt A, Biburger M, Nimmerjahn F. Mechanisms of action of intravenous immunoglobulins. Expert Rev Clin Immunol (2010) 6:425–34. doi:10.1586/eci.10.9
109. Jolles S, Kaveri SV, Orange J. Intravenous immunoglobulins. Current understanding and future directions. Clin Exp Immunol (2011) 158:68–70. doi:10.1111/j.1365-2249.2009.04029.x
110. Debré M, Bonnet MC, Fridman WH, Carosella E, Philippe N, Reinert P, et al. Infusion of Fc gamma fragments for treatment of children with acute immune thrombocytopenic purpura. Lancet (1993) 342:945–9. doi:10.1016/0140-6736(93)92000-J
111. Khayat D, Soubrane C, Andrieu JM, Visonneau S, Eme D, Tourani JM, et al. Changes of soluble CD16 levels in serum of HIV-infected patients: correlation with clinical and biologic prognostic factors. J Infect Dis (1990) 161:430–5. doi:10.1093/infdis/161.3.430
112. Tang CC, Isitman G, Bruneau J, Tremblay C, Bernard NF, Kent SJ, et al. Phenotypical and functional profiles of natural killer cells exhibiting matrix metalloproteinase-mediated CD16 cleavage after anti-HIV antibody-dependent activation. Clin Exp Immunol (2015) 181:275–85. doi:10.1111/cei.12593
113. Schroeder HW Jr, Cavacini L. Structure and function of immunoglobulins. J Allergy Clin Immunol (2010) 125:S41–52. doi:10.1016/j.jaci.2009.09.046
114. Uchida J, Hamaguchi Y, Oliver J, Ravetch J, Poe J, Haas K, et al. The innate mononuclear phagocyte network depletes B lymphocytes through Fc receptor-dependent mechanisms during anti-CD20 antibody immunotherapy. J Exp Med (2004) 199:1659–69. doi:10.1084/jem.20040119
115. Lambert S, Okada C, Levy R. TCR vaccines against a murine T cell lymphoma: a primary role for antibodies of the IgG2c class in tumor protection. J Immunol (2004) 172:929–36. doi:10.4049/jimmunol.172.2.929
116. Fossati-Jimack L, Ioan-Facsinay A, Reininger L, Chicheportiche Y, Watanabe N, Saito T, et al. Markedly different pathogenicity of four immunoglobulin G isotype-switch variants of an anti-erythrocyte autoantibody is based on their capacity to interact in vivo with the low-affinity Fcγ receptor III. J Exp Med (2000) 191:1293–302. doi:10.1084/jem.191.8.1293
117. Nimmerjahn F, Ravetch J. Divergent immunoglobulin G subclass activity through selective Fc receptor binding. Science (2005) 310:1510–2. doi:10.1126/science.1118948
118. Giorgini A, Brown HJ, Lock HR, Nimmerjahn F, Ravetch JV, Verbeek JS, et al. FcγRIII and FcγRIV are indispensable for acute glomerular inflammation induced by switch variant monoclonal antibodies. J Immunol (2008) 181:8745–52. doi:10.4049/jimmunol.181.12.8745
119. Nimmerjahn F, Bruhns P, Horiuchi K, Ravetch J. FcγRIV: a novel FcR with distinct IgG subclass specificity. Immunity (2005) 23:41–51. doi:10.1016/j.immuni.2005.05.010
120. Meyer D, Schiller C, Westermann J, Izui S, Hazenbos W, Verbeek J, et al. FcγRIII (CD16)-deficient mice show IgG isotype-dependent protection to experimental autoimmune hemolytic anemia. Blood (1998) 92:3997–4002.
121. Concetti F, Napolioni V. Insights into the role of Fc gamma receptors (FcγRs) genetic variations in monoclonal antibody-based anti-cancer therapy. Recent Pat Anticancer Drug Discov (2010) 5:97–204. doi:10.2174/157489210791760490
122. Kim JM, Ashkenazi A. Fcγ receptors enable anticancer action of proapoptotic and immune-modulatory antibodies. J Exp Med (2013) 210:1647–51. doi:10.1084/jem.20131625
123. Wright A, Morrison SL. Effect of glycosylation on antibody function: implications for genetic engineering. Trends Biotechnol (1997) 15:26–32. doi:10.1016/S0167-7799(96)10062-7
124. Shields R, Namenuk A, Hong K, Meng Y, Rae J, Briggs J, et al. High resolution mapping of the binding site on human IgG1 for FcγRI, FcγRII, FcγRIII, and FcRn and design of IgG1 variants with improved binding to the FcγR. J Biol Chem (2001) 276:6591–604. doi:10.1074/jbc.M009483200
125. Yamada E, Tsukamoto Y, Sasaki R, Yagyu K, Takahashi N. Structural changes in immunoglobulin G oligosaccharides with age in healthy human serum. Glucoconj J (1997) 14:401–5. doi:10.1023/A:1018582930906
126. Mizuochi T, Hamako J, Nose M, Titani K. Structural changes in the oligosaccharide chains of IgG in autoimmune MRL/Mp-lpr/lpr mice. J Immunol (1990) 145:1794–8.
127. Kaneko Y, Nimmerjahn F, Ravetch J. Anti-inflammatory activity of immunoglobulin G resulting from Fc sialylation. Science (2006) 313:670–3. doi:10.1126/science.1129594
128. Rook G, Steele J, Brealey R, Whyte A, Isenberg D, Sumar N, et al. Changes in IgG glycoform levels are associated with remission of arthritis during pregnancy. J Autoimmun (1991) 4:779–94. doi:10.1016/0896-8411(91)90173-A
129. van de Geijn F, Wuhrer M, Selman M, Willemsen S, de Man Y, Deelder A, et al. Immunoglobulin G galactosylation and sialylation are associated with pregnancy-induced improvement of rheumatoid arthritis and the postpartum flare: results from a large prospective cohort study. Arthritis Res Ther (2009) 11:R193. doi:10.1186/ar2892
130. Jefferis R. Glycosylation as a strategy to improve antibody-based therapeutics. Nat Rev Drug Discov (2009) 8:226–34. doi:10.1038/nrd2804
131. Mizuochi T, Taniguchi T, Shimitzu A, Kobata A. Structural and numerical variations of the carbohydrate moiety of immunoglobulin G. J Immunol (1982) 129:2016–20.
132. Shields R, Lai J, Keck R, O’Connell L, Hong K, Meng Y, et al. Lack of fucose on human IgG1 N-linked oligosaccharide improves binding to human FcγRIII and antibody-dependent cellular toxicity. J Biol Chem (2002) 277:26733–40. doi:10.1074/jbc.M202069200
133. Shinkawa T, Nakamura K, Yamane N, Shoji-Hosaka E, Kanda Y, Sakurada M, et al. The absence of fucose but not the presence of galactose or bisecting N-acetylglucosamine of human IgG1 complex-type oligosaccharides shows the critical role of enhancing antibody-dependent cellular cytotoxicity. J Biol Chem (2003) 278:3466–73. doi:10.1074/jbc.M210665200
134. Kubota T, Niwa R, Satoh M, Akinaga S, Shitara K, Hanai N. Engineered therapeutic antibodies with improved effector functions. Cancer Sci (2009) 100:1566–72. doi:10.1111/j.1349-7006.2009.01222.x
135. Varki A. “Unusual” modifications and variations of vertebrate oligosaccharides: are we missing the flowers from the trees? Glycobiology (1996) 6:707–10. doi:10.1093/glycob/6.7.707
136. Scallon B, Tam S, McCarthy S, Cai A, Raju T. Higher levels of sialylated Fc glycans in immunoglobulin G molecules can adversely impact functionality. Mol Immunol (2007) 44:1524–32. doi:10.1016/j.molimm.2006.09.005
137. Anthony R, Nimmerjahn F, Ashline D, Reinhold V, Paulson J, Ravetch J. Recapitulation of IVIG anti-inflammatory activity with a recombinant IgG Fc. Science (2008) 320:373–6. doi:10.1126/science.1154315
138. Anthony RM, Ravetch JV. A novel role for the IgG Fc glycan: the anti-inflammatory activity of sialylated IgG Fcs. J Clin Immnol (2010) 30:S9–14. doi:10.1007/s108575-010-9405-6
139. Bournazos S, Ravetch JV. Fcγ receptor pathways during active and passive immunization. Immunol Rev (2015) 268:88–103. doi:10.1111/imr.12343
140. Sánchez-Mejorada G, Rosales C. Signal transduction by immunoglobulin Fc receptors. J Leukoc Biol (1998) 63:521–33.
141. Mócsai A. Diverse novel functions of neutrophils in immunity, inflammation, and beyond. J Exp Med (2013) 210:1283–99. doi:10.1084/jem.20122220
142. Uribe-Querol E, Rosales C. Neutrophils in cancer: two sides of the same coin. J Immunol Res (2015) 2015:983698. doi:10.1155/2015/983698
143. Ortiz-Stern A, Rosales C. FcgRIIIB stimulation promotes b1 integrin activation in human neutrophils. J Leukoc Biol (2005) 77:787–99. doi:10.1189/jlb.0504310
144. García-García E, Nieto-Castañeda G, Ruiz-Saldaña M, Mora N, Rosales C. FcγRIIA and FcγRIIIB mediate nuclear factor activation through separate signaling pathways in human neutrophils. J Immunol (2009) 182:4547–56. doi:10.4049/jimmunol.0801468
145. Rivas-Fuentes S, García-García E, Nieto-Castañeda G, Rosales C. Fcγ receptors exhibit different phagocytosis potential in human neutrophils. Cell Immunol (2010) 263:114–21. doi:10.1016/j.cellimm.2010.03.006
146. García-García E, Uribe-Querol E, Rosales C. A simple and efficient method to detect nuclear factor activation in human neutrophils by flow cytometry. J Vis Exp (2013) 74:e50410. doi:10.3791/50410
147. Alemán OR, Mora N, Cortes-Vieyra R, Uribe-Querol E, Rosales C. Differential use of human neutrophil Fcγ receptors for inducing neutrophil extracellular trap formation. J Immunol Res (2016) 2016:142643. doi:10.1155/2016/2908034
148. Salmon JE, Browle NL, Edberg JC, Kimberly RP. Fcg receptor III induces actin polymerization in human neutrophils and primes phagocytosis mediated by Fcg receptor II. J Immunol (1991) 146:997–1004.
149. Kocher M, Siegel ME, Edberg JC, Kimberly RP. Cross-linking of Fcg receptor IIa and Fcg receptor IIIb induces different proadhesive phenotypes on human neutrophils. J Immunol (1997) 159:3940–8.
150. García-García E, Rosales C. Signal transduction during Fc receptor-mediated phagocytosis. J Leukoc Biol (2002) 72:1092–108.
151. García-García E, Rosales C. Fc receptor signaling in leukocytes: role in host defense and immune regulation. Curr Immunol Rev (2009) 5:227–42. doi:10.2174/157339509788921229
152. Coxon PY, Rane MJ, Powell DW, Klein JB, McLeish KR. Differential mitogen-activated protein kinase stimulation by Fc gamma receptor IIa and Fc gamma receptor IIIb determines the activation phenotype of human neutrophils. J Immunol (2000) 164:6530–7. doi:10.4049/jimmunol.164.12.6530
153. van Egmond M, Vidarsson G, Bakema JE. Cross-talk between pathogen recognizing toll-like receptors and immunoglobulin Fc receptors in immunity. Immunol Rev (2015) 268:311–27. doi:10.1111/imr.12333
154. Brinkmann V, Zychlinsky A. Beneficial suicide: why neutrophils die to make NETs. Nat Rev Microbiol (2007) 5:577–82. doi:10.1038/nrmicro1710
155. Yipp BG, Kubes P. NETosis: how vital is it? Blood (2013) 122:2784–94. doi:10.1182/blood-2013-04-457671
156. Branzk N, Papayannopoulos V. Molecular mechanisms regulating NETosis in infection and disease. Semin Immunopathol (2013) 35:513–30. doi:10.1007/s00281-013-0384-6
157. Brinkmann V, Zychlinsky A. Neutrophil extracellular traps: is immunity the second function of chromatin? J Cell Biol (2012) 198:773–83. doi:10.1083/jcb.201203170
158. Chen K, Nishi H, Travers R, Tsuboi N, Martinod K, Wagner DD, et al. Endocytosis of soluble immune complexes leads to their clearance by FcγRIIIB but induces neutrophil extracellular traps via FcγRIIA in vivo. Blood (2012) 120:4421–31. doi:10.1182/blood-2011-12-401133
159. Short KR, von Köckritz-Blickwede M, Langereis JD, Chew KY, Job ER, Armitage CW, et al. Antibodies mediate formation of neutrophil extracellular traps in the middle ear and facilitate secondary pneumococcal otitis media. Infect Immun (2014) 82:364–70. doi:10.1128/IAI.01104-13
160. Behnen M, Leschczyk C, Möller S, Batel T, Klinger M, Solbach W, et al. Immobilized immune complexes induce neutrophil extracellular trap release by human neutrophil granulocytes via FcγRIIIB and Mac-1. J Immunol (2014) 193:1954–65. doi:10.4049/jimmunol.1400478
161. Alemán OR, Mora N, Cortes-Vieyra R, Uribe-Querol E, Rosales C. Transforming growth factor-β-activated kinase 1 is required for human FcγRIIIb-induced neutrophil extracellular trap formation. Front Immunol (2016) 7:277. doi:10.3389/fimmu.2016.00277
162. Eccles SA. Monoclonal antibodies targeting cancer: ‘magic bullets’ or just the trigger? Breast Cancer Res (2001) 3:86–90. doi:10.1186/bcr276
Keywords: immunoglobulin, antibody, phagocytosis, neutrophil, ERK, NF-κB, integrin
Citation: Rosales C (2017) Fcγ Receptor Heterogeneity in Leukocyte Functional Responses. Front. Immunol. 8:280. doi: 10.3389/fimmu.2017.00280
Received: 13 October 2016; Accepted: 27 February 2017;
Published: 20 March 2017
Edited by:
Luigi Daniele Notarangelo, Harvard Medical School, USAReviewed by:
Luz Pamela Blanco, National Institute of Health, USAMasato Kubo, Tokyo University of Science, Japan
Copyright: © 2017 Rosales. This is an open-access article distributed under the terms of the Creative Commons Attribution License (CC BY). The use, distribution or reproduction in other forums is permitted, provided the original author(s) or licensor are credited and that the original publication in this journal is cited, in accordance with accepted academic practice. No use, distribution or reproduction is permitted which does not comply with these terms.
*Correspondence: Carlos Rosales, Y2Fyb3NhbEB1bmFtLm14