- Department of Respiratory, Inflammation, and Autoimmunity, MedImmune LLC, Gaithersburg, MD, United States
Protein citrullination catalyzed by peptidyl arginine deiminase (PADs) is involved in autoimmune disease pathogenesis, especially in rheumatoid arthritis. Calcium is a key regulator of PAD activity, but under normal physiological conditions it remains uncertain how intracellular calcium levels can be raised to sufficiently high levels to activate these enzymes. In pursuit of trying to identify other factors that influence PAD activity, we identified bicarbonate as a potential regulator of PAD activity. We demonstrate that physiological levels of bicarbonate upregulate citrullination by recombinant PAD2/4 and endogenous PADs in neutrophils. The impact of bicarbonate is independent of calcium and pH. Adding bicarbonate to commercial PAD activity kits could increase assay performance and biological relevance. These results suggest that citrullination activity is regulated by multiple factors including calcium and bicarbonate. We also provide commentary on the current understanding of PAD regulation and future perspective of research in this area.
Introduction
Protein citrullination (or deimination) is the conversion of peptidylarginine to citrulline. This process is mediated by a family of enzymes; peptidyl arginine deiminase (PADs), including five isozymes in human (PAD1-4, PAD6) (1–3). These isozymes are expressed in different tissues and are involved in distinct functions. Among them, PAD2 and PAD4 are expressed mainly in leukocytes, especially enriched in neutrophils and are widely studied in immune dysregulations. Elevated protein citrullination was observed in inflamed joints of patients with rheumatoid arthritis (RA) (4–7). Autoantibodies against citrullinated proteins (ACPA) are present in approximately 70% of patients with RA and are highly diagnostic for the disease (8–10).
Of the five arginine deiminases, only PAD4 contains a nuclear localization sequence and its main physiological function appears to be histone citrullination (11, 12). Citrullination regulates binding of histone 1 to chromatin, alters the gene expression, and regulates stem cell differentiation (13). According to some reports, histone hypercitrullination leads to chromatin decondensation and the formation of neutrophil extracellular trap (NET formation) (12, 14). PAD4 activity also regulates antimicrobial defense in soft tissue infections (14). Increased NET formation is evident and considered pathogenic in systemic lupus erythematosus and ANCA-associated vasculitis (15–20). PAD4 and citrullinated proteins are found in NETs, so, NET formation may be a critical source of citrullinated autoantigen in RA (21–24). Neutrophil hypercitrullination mediated by calcium influx was also found in synovial fluid from patients with RA (25). Consequently, PADs are being increasingly recognized as therapeutic targets (26). Inhibition of PAD activity suppressed pathology in murine models of autoimmune disease (27–30). These effects have been attributed mainly to the inhibition of PAD4 (31).
Recent studies have focused on PAD activity in pathological conditions; however, little is known about the physiological functions of PADs, and how these enzymes are regulated at a biochemical level (32). It is clear that calcium ions are essential for PAD activity. X-ray crystallography has revealed that PAD4 binds to five calcium ions (33). Two calcium ions are in the C-terminal domain of PAD4 at the bottom of the active domain. The binding of those two calcium ions to the acidic concave surface of the C-terminal domain is crucial for recognition of the substrate and PAD activity (33). Another three calcium ions reside on the molecular surface of the PAD4 N-terminal domain, and these ions may stabilize protein structure and are important for the full activation of PAD4 (34). Other than calcium, reduced glutathione (GSH) was recently described as an in vivo PAD co-activator to maintain the reduced state of Cys645 in PAD4 catalytic domain (Cys647 in PAD2) (35). There could well be an oxidation/reduction (redox) balance regulation of PAD activity (36).
Here, we demonstrated that bicarbonate is important for optimal recombinant PAD2/4 activity. Neutrophil histone citrullination and hypercitrullination at physiological calcium concentrations was also regulated by bicarbonate. The impact of bicarbonate on citrullination was observed at different calcium concentrations and was independent of pH. Our work has revealed a previously unknown role of bicarbonate on PAD activity regulation. Based on these findings, we propose that in vivo PAD activity could be under complex regulation by factors including calcium, bicarbonate, and redox.
Recombinant PAD2/4 Require Bicarbonate for Optimal Histone Citrullination
To assess inhibitors of PAD activity, various assays are in use, which consist of recombinant enzyme, a simple buffer or tissue culture media (e.g., Tris–HCl pH 7–8, HBSS, RPMI, or DMEM), calcium, a reduction reagent, and an arginine containing substrate. A biochemical or antibody-based approach is then used to detect the citrullinated substrate. One intriguing difference between these citrullination assays is the amount of bicarbonate included in the buffer. Human serum contains 17–29 mM bicarbonate (37), whereas Tris–HCl buffer contains no bicarbonate, HBSS, RPMI, and DMEM media contain 4.17, 23.81, and 44.05 mM of bicarbonate, respectively. Initially, we verified the importance of calcium for PAD activity in a histone H3 citrullination assay containing HBSS (Figure 1A). We next assessed the impact of bicarbonate on PAD4 and PAD2 activity on histone H3 citrullination in DPBS/HEPES (adjusted to pH 7.2) with 0.9 mM calcium. Increased citrullination was detected with both recombinant PAD4 and PAD2 in the presence of low amounts of bicarbonate (Figures 1A,B). The requirement for bicarbonate appeared greater for PAD4 than PAD2 activity, since minimal histone H3 citrullination was observed with PAD4 in the absence of bicarbonate (Figure 1A). PAD2 activity increased with increasing amounts of bicarbonate from 1 to 22 mM (Figure 1B). Since the pH was maintained at a consistent pH 7.2 in these assays, the results could indicate that the impact of bicarbonate on recombinant PAD activity was independent of pH.
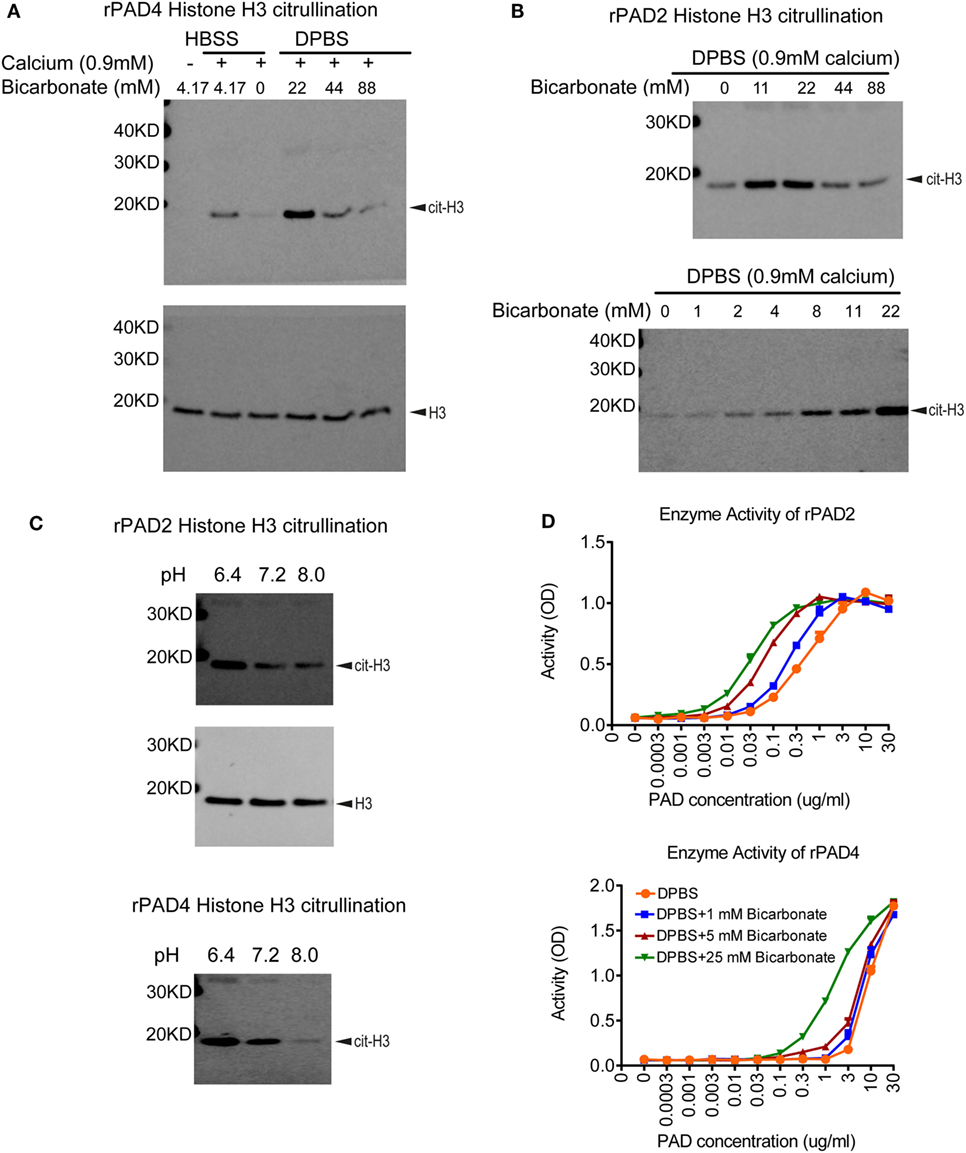
Figure 1. Bicarbonate impacts recombinant protein arginine deiminase (PAD) activity. (A) Histone H3 citrullination by recombinant human PAD4 for 1 h at 37°C with 5% CO2 in HBSS or DPBS with different bicarbonate concentrations. Total histone H3 was used as loading control. (B) Histone H3 citrullination by recombinant human PAD2 for 1 h at 37°C with 5% CO2 in DPBS with different bicarbonate concentrations. Results are representative of three independent experiments. (C) Histone H3 citrullination by recombinant human PAD2/4 for 1 h at 37°C with 5% CO2 in DPBS with 0.9 mM calcium under different pH levels in the absence of bicarbonate. Total histone H3 was used as loading control. (D) Recombinant human PAD2/4 activity in DPBS with different bicarbonate concentrations measured by ELISA-based fibrinogen citrullination assay. r2 values of nonlinear regression curve fit were higher than 0.98 for all conditions. Significant increase of PAD activity in DPBS with 25 mM bicarbonate was confirmed with two-way ANOVA analysis (P < 0.0001).
To further confirm the pH-independent effect of bicarbonate and explore the effect of pH on PAD activity, we performed histone H3 citrullination with recombinant enzyme under different pH levels adjusted by HCl and NaOH. Both PAD2 and PAD4 showed higher activity at pH of 6.4 compared to 7.2 and 8.0 (Figure 1C). Our data suggest that, for PAD2/4, their optimal pH for histone citrullination could be at a more acidic condition. Since higher bicarbonate levels would increase pH (without additional adjustment), these results confirm that bicarbonate increases PAD activity independent of pH.
Bicarbonate Improves PAD Activity in ELISA-Based Fibrinogen Citrullination Assays
To verify that the impact of bicarbonate was not limited to histone H3 citrullination or related to the western blot detection method, we examined the impact of bicarbonate on an ELISA-based fibrinogen citrullination assay, again using DPBS/HEPES (adjusted to pH7.2) with 0.9 mM calcium. Bicarbonate significantly increased fibrinogen citrullination by PAD2 and PAD4 in a dose-dependent manner (Figure 1D). These results demonstrate that the impact of bicarbonate on PAD activity was independent of substrates or detection methods and provide additional supportive evidence that bicarbonate regulates recombinant PAD activity.
Bicarbonate Impacts Neutrophil Histone H3 Citrullination
To determine if bicarbonate impacts endogenous PAD activity, we examined neutrophil citrullination induced by ionomycin-mediated calcium influx. Interestingly, in the presence of calcium, ionomycin-treated neutrophils in DMEM showed much stronger histone H3 citrullination when bicarbonate was present (pH was adjusted to 7.2 with HEPES in all DMEM media, Figure 2A). A previous study suggested that high glucose in diabetes was associated with elevated neutrophil PAD4 expression and NET formation (38). However, we found that added extracellular glucose did not affect ionomycin/calcium-induced citrullination (Figure 2A). This suggests that glucose does not impact PAD activity directly.
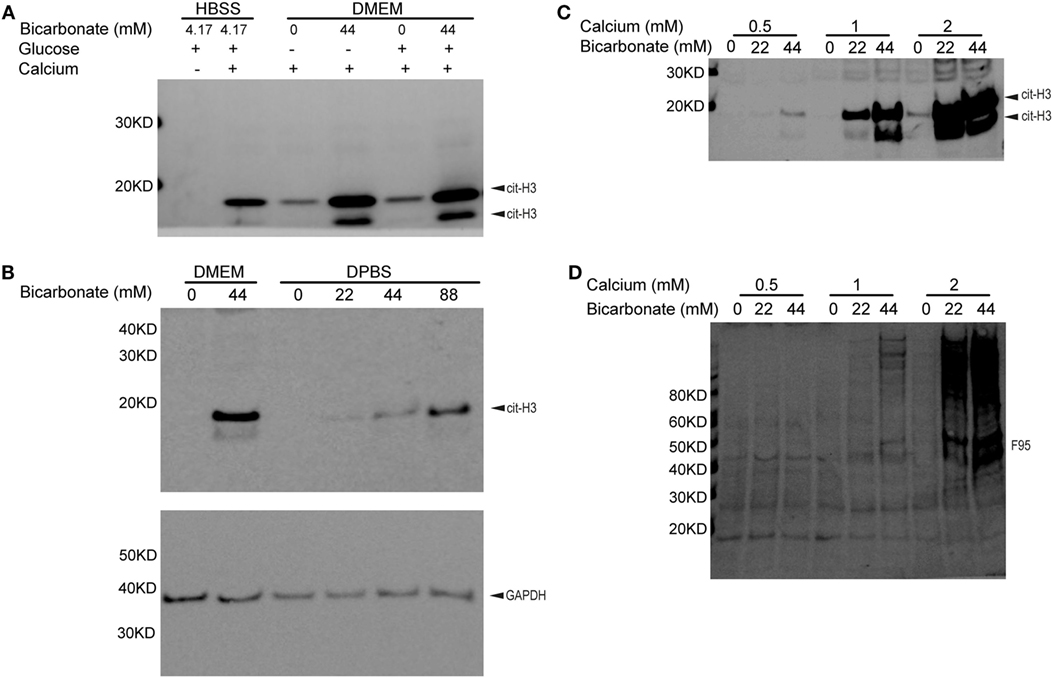
Figure 2. Bicarbonate level impacts neutrophil citrullination. (A) Ionomycin-induced neutrophil histone H3 citrullination with HBSS and DMEM (30 min at 37°C with 5% CO2). (B) Bicarbonate dose–response of neutrophil histone H3 citrullination stimulated by ionomycin (30 min at 37°C with 5% CO2). GAPDH used as loading control. (C) Ionomycin-induced neutrophil histone H3 citrullination with different bicarbonate and calcium levels. (D) Neutrophil hypercitrullination detected by anti-citrulline antibody F95 of panel (C). Results are representative of three different donors.
We confirmed that neutrophil histone citrullination was also increased with bicarbonate in DPBS, a much simple buffer without vitamins, amino acids, and other factors present in tissue culture media (Figure 2B).
Taken together, we conclude that bicarbonate promotes intracellular PAD activity.
Bicarbonate Impacts Neutrophil Citrullination at Different Calcium Levels
Since high levels of calcium are critical for PAD activity, we sought to determine if bicarbonate could promote citrullination independent of calcium concentrations. Bicarbonate increased neutrophil histone H3 citrullination (Figure 2C) and hyper citrullination (Figure 2D) at three different calcium concentrations from 0.5 to 2 mM. pH was adjusted to 7.2 with HEPES in DPBS and DMEM used. These results demonstrate that bicarbonate promotes intracellular citrullination at sub-physiological and physiological levels of serum calcium. Although there is a requirement for calcium for citrullination, bicarbonate independently increases PAD activity.
Discussion
Current research on PAD biology has focused on the role of citrullination in disease pathogenesis and efforts to inhibit them. Little progress has been made on how PADs are activated and their functions under non-pathogenic conditions. The only essential factor known for PAD enzyme activity is calcium (1), although a reducing environment is also required to prevent oxidation of cysteine in the catalytic domain (36). We show for the first time that bicarbonate also has a profound impact on the activity of recombinant PAD enzymes and intracellular citrullination in neutrophils. The increased PAD activity promoted by bicarbonate is independent of its pH buffering capacity, and it acts independently of calcium and glucose. Therefore, bicarbonate may function as an important regulator of PAD activity.
Bicarbonate is the main buffering component in blood and an important in vivo signaling molecule (37, 39). Human serum contains 17–29 mM bicarbonate, which maintains serum pH levels at 7.4 (37). Bicarbonate and CO2 concentrations are also essential in maintaining optimal tissue culture growth conditions (40). Because of its important role in pH buffering, it is essential to separate the impact of pH and bicarbonate in biological functions. To focus on the impact of bicarbonate only in citrullination, we used HEPES to adjust all media pH to 7.2, then added different concentrations of bicarbonate. These results were consistent with different media such as DMEM, RPMI (data not shown), and DPBS that contained different inorganic ions and other weak acid and base-buffering pairs. The effect of bicarbonate on citrullination was consistent across different media and buffers, further demonstrated that the impact was indeed due to bicarbonate, independent of pH, and not from many other components in the tissue culture media.
Interestingly, we observed that recombinant histone H3 citrullination was increased by both recombinant PAD2 and PAD4 at a mildly acidic pH of 6.4. This further confirmed the impact of bicarbonate was independent of pH since higher bicarbonate leads to more alkaline pH levels. Previous studies with a different substrate—Benzoyl l-arginine ethyl ester (BAEE) showed optimal pH of 7.6 for PAD4 (41). This difference is likely due to the different substrates used in experiments, as BAEE, unlike histone, is a small molecule substrate.
Leppkes et al. first discovered that pancreatic juice could induce NET formation (42). Bicarbonate is a main component in pancreatic juice and was found to increase NET formation through histone citrullination. Later studies have shown that bicarbonate in media could impact NET formation through pH (43, 44). We limited the stimulation time in neutrophil citrullination assays to 30 min to focus on PAD enzymatic activity rather than downstream NET formation. The impact of bicarbonate on PAD2 and PAD4 activity was independent of pH and substrate. It remains possible that other pathways involved with NET formation may be impacted by bicarbonate through increased pH.
We showed that bicarbonate greatly impacted citrullination in biochemical assays with recombinant PADs and in neutrophils. The profound interest in PADs has led to an increase in different citrullination assays (45, 46). Those assays consider calcium levels and the reducing environment. All PADs require high calcium levels to be active (1), and the PAD catalytic domain contains a critical cysteine, which requires a reducing environment to maintain proper enzymatic function (35). Bicarbonate is a common but sometimes overlooked component in tissue culture media, which is necessary for optimal PAD activity. Our work suggests that a reconfiguration of assay buffers is needed to reflect the biology of citrullination.
More importantly, our study has indicated that the regulation of PAD activity is rather more complicated than previously known. Both bicarbonate and calcium can act as physiological signaling molecules. Remarkably, there are few examples of how bicarbonate regulates enzyme activity. A well-characterized example of this would be soluble adenylyl cyclase (sAC) (47). sAC is the only intracellular adenylyl cyclase that produces cAMP in response to calcium or bicarbonate signals (48, 49). sAC is conserved from cyanobacteria to mammals, with a wide range of functions including neuron activation to spermatozoa (50, 51). Intriguingly, the crystal structure showed that bicarbonate binds adjacent to Arg176 in human sAC, which acts as a switch that enables formation of the catalytic cation sites (52, 53). It is interesting to speculate that PADs could function by a similar process to sAC with citrullination regulated as a potential secondary messenger as seen with cAMP. Future crystallography studies are needed to understand whether bicarbonate impacts PAD structure, and how it could regulate citrullination activity.
In conclusion, we demonstrate that bicarbonate is a potential regulator of PAD activity. PADs could well be under a complex web of regulating factors including calcium and bicarbonate, responding to different in vivo signals through specific citrullination events. Dissecting those regulating signals, citrullination events and functional consequences could unlock a new level understanding of biology far beyond autoimmunity.
Perspective on Future PAD Biology
Despite the focus on PADs and citrullination in the context of autoimmune diseases, there remains much to learn about the normal physiological functions of PADs. We recently reported that human neutrophils express active PAD4 on the surface and secrete active PAD2 (54). The role of extracellular PADs remains undefined but may modulate extracellular protein functions and fine tune inflammation. Within the cell, nuclear-located PAD4 could also regulate gene expression in concert with other histone modification enzymes (13, 55, 56). These physiological functions would require a precise regulation of PAD activity.
Although we showed that bicarbonate can impact citrullination independent of calcium and pH, there are still important gaps in our understanding of PAD regulation. For example, the millimolar concentrations of calcium required for PAD activation do not normally exist intracellularly even following cellular activation (25, 54, 57). So, how can PAD function inside a cell with nanomolar level intracellular calcium? It is plausible that high calcium concentration may exist in localized regions/specific organelles within a cell where PADs could be active. Alternatively, PADs could have other intracellular binding partners or co-factors that lower the calcium threshold for activation. Indeed, it has been shown that anti-PAD3/4 autoantibodies from patients with RA can increase PAD activity in low calcium conditions (58, 59). This calcium paradox for PAD activity is critical for our understanding of intracellular PAD activity, is largely overlooked, and requires further investigation.
Remarkably, intracellular protein citrullination is mostly observed with large calcium influx under membrane lytic conditions (25, 57, 60, 61). Detection of intracellular citrullination under normal healthy conditions is challenging. This may be a consequence of the limited citrullination detection methods and their low sensitivity. Improvements in technology may enable better assessment of intracellular citrullination. Alternatively, we should also consider the possibility that PADs may have functions other than citrullination. It is notable that besides the cysteine present at the catalytic domain (35, 36), PAD2 and PAD4 both exhibit in excess of 10 free surface exposed cysteines. This remarkably unusual characteristic must have some physiological relevance, whether this is related to binding substrate, or some other function such as acting as scavenger for ROS, remains to be determined. In any case, it is clear that there remains much to learn about the regulation and function of PADs in health and disease, and future research efforts will inevitably unravel new and exciting biology.
Materials and Methods
Human Donors and Neutrophil Isolation
Blood from healthy volunteers was obtained with informed consent under MedImmune, LLC’s blood donation program, and studies using human cells were performed in accordance with the Institutional Review Board guidelines. Neutrophils were isolated from heparin anticoagulated blood on a discontinuous Ficoll gradient as previously described (62).
Antibodies and Reagents
Antibodies for detection of citrullinated histone H3 (R2 citrullination, Ab176843) and total histone H3 (Ab24834) were from Abcam (Cambridge, MA, USA). Anti-citrullinated fibrinogen antibody (20B2) was from ModiQuest (Oss, Netherlands). Antibody for detection of pan citrullination, F95 was from EMD Millipore (Billerica, MA, USA).
Recombinant human PAD2 and recombinant human PAD4 were generated in-house. Recombinant human histone H3 was from Cayman Chemicals (Ann Arbor, MI, USA).
DMEM without bicarbonate was from Agilent. DMEM with bicarbonate, RPMI, DPBS with 0.9mM calcium, HBSS, HEPES, glucose, 7.5% sodium bicarbonate, ionomycin, and 1M calcium chloride were from Invitrogen (Carlsbad, CA, USA).
pH Level Adjustment of Media and Buffers
pH of all buffers and media were tested with pH meter (Thermo Fisher Scientific). For experiments with different pH levels, pH was adjusted with HCl and NaOH. In all other experiments, HEPES was used to adjust pH of buffers and media to 7.2. pH levels of buffers adjusted with HEPES at the start and the end of each experiment were tested (pH levels were between 7.0 and 7.4 after experiments).
Western Blot Analysis
Equivalent amounts of cells were separated by SDS-polyacrylamide gel electrophoresis (4–12% gel) and then transferred to a nitrocellulose membrane. Membranes were then probed using anti-citrullinated histone H3 (Ab176843) and anti-total histone H3 (Ab24834) antibodies using the iBind (Invitrogen) system with 1:1,000 dilution. An HRP-conjugated anti-mouse IgG and anti-rabbit IgG were used as the secondary detection antibodies (1:5,000 dilution) before visualization of immunoreactive bands with an ECL reagent (Thermo Fisher). F95 western blot detection of pan citrullination used methods described before (57).
Citrullination Assays
Recombinant human PAD2 (5 ng) or PAD4 (20 ng) was incubated with histone H3 (5 µg) in different media for 1 h at 37°C with 5% CO2. One million neutrophils were resuspended with 100 µl media and stimulated with 1 µM ionomycin for 30 min at 37°C. All reactions were performed in closed capped Eppendorf tubes and stopped with the addition of lithium dodecyl sulfate sample buffer (Invitrogen) and boiling.
Fibrinogen Citrullination ELISA Assay
Nunc Maxisorp plates were coated with 1 µg/ml human fibrinogen overnight at 4°C. After blocking with PBS containing 1% BSA, rhuPAD2 and rhuPAD4 were titrated in Deimination buffer [40 mM Tris–HCl (pH 7.5), 5 mM NaCl, 1 mM DTT] and added to coated plates. After a 90-min incubation at 37°C with 5% CO2, anti-citrullinated Fibrinogen IgG (20B2) followed by rabbit anti-mouse IgG HRP (ab6728) were added to the plates followed by detection with a colorimetric substrate (TMB and Stop Solution, KPL). The absorbance was read on a plate reader at 450 nm and the data were analyzed using Softmax Pro software. Statistical analysis was performed with Prism GraphPad (GraphPad Software, La Jolla, CA, USA).
Ethics Statement
Blood from healthy volunteers was obtained with informed consent under MedImmune, LLC’s blood donation program, and studies using human cells were performed in accordance with the Institutional Review Board guidelines.
Author Contributions
YZ and GS generated ideas for this study. YZ and NM performed experiments and analyzed data. YZ, NM, and GS wrote the manuscript. All authors reviewed the manuscript for content, provided suggestions, and approved the final manuscript.
Conflict of Interest Statement
YZ, NM, and GPS are full-time employees of MedImmune, a member of the AstraZeneca group.
The reviewer ML and handling editor declared their shared affiliation.
Acknowledgments
We thank Caroline Colley for providing insights about enzyme biology.
Funding
The study was funded be MedImmune LLC.
References
1. Vossenaar ER, Zendman AJ, van Venrooij WJ, Pruijn GJ. PAD, a growing family of citrullinating enzymes: genes, features and involvement in disease. Bioessays (2003) 25(11):1106–18. doi:10.1002/bies.10357
2. Baka Z, Gyorgy B, Geher P, Buzas EI, Falus A, Nagy G. Citrullination under physiological and pathological conditions. Joint Bone Spine (2012) 79(5):431–6. doi:10.1016/j.jbspin.2012.01.008
3. Wang S, Wang Y. Peptidylarginine deiminases in citrullination, gene regulation, health and pathogenesis. Biochim Biophys Acta (2013) 1829(10):1126–35. doi:10.1016/j.bbagrm.2013.07.003
4. Tutturen AE, Fleckenstein B, de Souza GA. Assessing the citrullinome in rheumatoid arthritis synovial fluid with and without enrichment of citrullinated peptides. J Proteome Res (2014) 13(6):2867–73. doi:10.1021/pr500030x
5. van Beers JJ, Schwarte CM, Stammen-Vogelzangs J, Oosterink E, Bozic B, Pruijn GJ. The rheumatoid arthritis synovial fluid citrullinome reveals novel citrullinated epitopes in apolipoprotein E, myeloid nuclear differentiation antigen, and beta-actin. Arthritis Rheum (2013) 65(1):69–80. doi:10.1002/art.37720
6. Vossenaar ER, van Venrooij WJ. Citrullinated proteins: sparks that may ignite the fire in rheumatoid arthritis. Arthritis Res Ther (2004) 6(3):107–11. doi:10.1186/ar1057
7. Chang X, Yamada R, Suzuki A, Sawada T, Yoshino S, Tokuhiro S, et al. Localization of peptidylarginine deiminase 4 (PADI4) and citrullinated protein in synovial tissue of rheumatoid arthritis. Rheumatology (2005) 44(1):40–50. doi:10.1093/rheumatology/keh414
8. Schellekens GA, de Jong BA, van den Hoogen FH, van de Putte LB, van Venrooij WJ. Citrulline is an essential constituent of antigenic determinants recognized by rheumatoid arthritis-specific autoantibodies. J Clin Invest (1998) 101(1):273–81. doi:10.1172/JCI1316
9. van Venrooij WJ, van Beers JJ, Pruijn GJ. Anti-CCP antibodies: the past, the present and the future. Nat Rev Rheumatol (2011) 7(7):391–8. doi:10.1038/nrrheum.2011.76
10. Willemze A, Trouw LA, Toes RE, Huizinga TW. The influence of ACPA status and characteristics on the course of RA. Nat Rev Rheumatol (2012) 8(3):144–52. doi:10.1038/nrrheum.2011.204
11. Nakashima K, Hagiwara T, Yamada M. Nuclear localization of peptidylarginine deiminase V and histone deimination in granulocytes. J Biol Chem (2002) 277(51):49562–8. doi:10.1074/jbc.M208795200
12. Neeli I, Khan SN, Radic M. Histone deimination as a response to inflammatory stimuli in neutrophils. J Immunol (2008) 180(3):1895–902. doi:10.4049/jimmunol.180.3.1895
13. Christophorou MA, Castelo-Branco G, Halley-Stott RP, Oliveira CS, Loos R, Radzisheuskaya A, et al. Citrullination regulates pluripotency and histone H1 binding to chromatin. Nature (2014) 507(7490):104–8. doi:10.1038/nature12942
14. Li P, Li M, Lindberg MR, Kennett MJ, Xiong N, Wang Y. PAD4 is essential for antibacterial innate immunity mediated by neutrophil extracellular traps. J Exp Med (2010) 207(9):1853–62. doi:10.1084/jem.20100239
15. Hakkim A, Furnrohr BG, Amann K, Laube B, Abed UA, Brinkmann V, et al. Impairment of neutrophil extracellular trap degradation is associated with lupus nephritis. Proc Natl Acad Sci U S A (2010) 107(21):9813–8. doi:10.1073/pnas.0909927107
16. Garcia-Romo GS, Caielli S, Vega B, Connolly J, Allantaz F, Xu Z, et al. Netting neutrophils are major inducers of type I IFN production in pediatric systemic lupus erythematosus. Sci Transl Med (2011) 3(73):73ra20. doi:10.1126/scitranslmed.3001201
17. Kessenbrock K, Krumbholz M, Schonermarck U, Back W, Gross WL, Werb Z, et al. Netting neutrophils in autoimmune small-vessel vasculitis. Nat Med (2009) 15(6):623–5. doi:10.1038/nm.1959
18. Kelley JM, Monach PA, Ji C, Zhou Y, Wu J, Tanaka S, et al. IgA and IgG antineutrophil cytoplasmic antibody engagement of Fc receptor genetic variants influences granulomatosis with polyangiitis. Proc Natl Acad Sci U S A (2011) 108(51):20736–41. doi:10.1073/pnas.1109227109
19. Darrah E, Andrade F. NETs: the missing link between cell death and systemic autoimmune diseases? Front Immunol (2012) 3:428. doi:10.3389/fimmu.2012.00428
20. Gupta S, Kaplan MJ. The role of neutrophils and NETosis in autoimmune and renal diseases. Nat Rev Nephrol (2016) 12(7):402–13. doi:10.1038/nrneph.2016.71
21. Blachere NE, Parveen S, Fak J, Frank MO, Orange DE. Inflammatory but not apoptotic death of granulocytes citrullinates fibrinogen. Arthritis Res Ther (2015) 17(1):369. doi:10.1186/s13075-015-0890-0
22. Corsiero E, Pratesi F, Prediletto E, Bombardieri M, Migliorini P. NETosis as source of autoantigens in rheumatoid arthritis. Front Immunol (2016) 7:485. doi:10.3389/fimmu.2016.00485
23. Khandpur R, Carmona-Rivera C, Vivekanandan-Giri A, Gizinski A, Yalavarthi S, Knight JS, et al. NETs are a source of citrullinated autoantigens and stimulate inflammatory responses in rheumatoid arthritis. Sci Transl Med (2013) 5(178):178ra40. doi:10.1126/scitranslmed.3005580
24. Spengler J, Lugonja B, Ytterberg AJ, Zubarev RA, Creese AJ, Pearson MJ, et al. Release of active peptidyl arginine deiminases by neutrophils can explain production of extracellular citrullinated autoantigens in rheumatoid arthritis synovial fluid. Arthritis Rheumatol (2015) 67(12):3135–45. doi:10.1002/art.39313
25. Romero V, Fert-Bober J, Nigrovic PA, Darrah E, Haque UJ, Lee DM, et al. Immune-mediated pore-forming pathways induce cellular hypercitrullination and generate citrullinated autoantigens in rheumatoid arthritis. Sci Transl Med (2013) 5(209):209ra150. doi:10.1126/scitranslmed.3006869
26. Lewis HD, Nacht M. iPAD or PADi-‘tablets’ with therapeutic disease potential? Curr Opin Chem Biol (2016) 33:169–78. doi:10.1016/j.cbpa.2016.06.020
27. Kusunoki Y, Nakazawa D, Shida H, Hattanda F, Miyoshi A, Masuda S, et al. Peptidylarginine deiminase inhibitor suppresses neutrophil extracellular trap formation and MPO-ANCA production. Front Immunol (2016) 7:227. doi:10.3389/fimmu.2016.00227
28. Sarswat A, Wasilewski E, Chakka SK, Bello AM, Caprariello AV, Muthuramu CM, et al. Inhibitors of protein arginine deiminases and their efficacy in animal models of multiple sclerosis. Bioorg Med Chem (2017) 25(9):2643–56. doi:10.1016/j.bmc.2017.03.006
29. Biron BM, Chung CS, O’Brien XM, Chen Y, Reichner JS, Ayala A. Cl-amidine prevents histone 3 citrullination and neutrophil extracellular trap formation, and improves survival in a murine sepsis model. J Innate Immun (2017) 9(1):22–32. doi:10.1159/000448808
30. Willis VC, Banda NK, Cordova KN, Chandra PE, Robinson WH, Cooper DC, et al. Protein arginine deiminase 4 inhibition is sufficient for the amelioration of collagen-induced arthritis. Clin Exp Immunol (2017) 188(2):263–74. doi:10.1111/cei.12932
31. Suzuki A, Kochi Y, Shoda H, Seri Y, Fujio K, Sawada T, et al. Decreased severity of experimental autoimmune arthritis in peptidylarginine deiminase type 4 knockout mice. BMC Musculoskelet Disord (2016) 17(1):205. doi:10.1186/s12891-016-1055-2
32. Bicker KL, Thompson PR. The protein arginine deiminases: structure, function, inhibition, and disease. Biopolymers (2013) 99(2):155–63. doi:10.1002/bip.22127
33. Arita K, Hashimoto H, Shimizu T, Nakashima K, Yamada M, Sato M. Structural basis for Ca(2+)-induced activation of human PAD4. Nat Struct Mol Biol (2004) 11(8):777–83. doi:10.1038/nsmb799
34. Liu YL, Tsai IC, Chang CW, Liao YF, Liu GY, Hung HC. Functional roles of the non-catalytic calcium-binding sites in the N-terminal domain of human peptidylarginine deiminase 4. PLoS One (2013) 8(1):e51660. doi:10.1371/journal.pone.0051660
35. Damgaard D, Bjorn ME, Steffensen MA, Pruijn GJ, Nielsen CH. Reduced glutathione as a physiological co-activator in the activation of peptidylarginine deiminase. Arthritis Res Ther (2016) 18(1):102. doi:10.1186/s13075-016-1000-7
36. Damgaard D, Bjorn ME, Jensen PO, Nielsen CH. Reactive oxygen species inhibit catalytic activity of peptidylarginine deiminase. J Enzyme Inhib Med Chem (2017) 32(1):1203–8. doi:10.1080/14756366.2017.1368505
37. Burtis CA, Ashwood ER, Bruns DE, Tietz NW. Tietz Textbook of Clinical Chemistry and Molecular Diagnostics. 5th ed. St. Louis, MO: Saunders (2013). xviii, 2238 p.
38. Wong SL, Demers M, Martinod K, Gallant M, Wang Y, Goldfine AB, et al. Diabetes primes neutrophils to undergo NETosis, which impairs wound healing. Nat Med (2015) 21(7):815–9. doi:10.1038/nm.3887
39. Buck J, Levin LR. Physiological sensing of carbon dioxide/bicarbonate/pH via cyclic nucleotide signaling. Sensors (Basel) (2011) 11(2):2112–28. doi:10.3390/s110202112
40. Merten OW. Introduction to animal cell culture technology-past, present and future. Cytotechnology (2006) 50(1–3):1–7. doi:10.1007/s10616-006-9009-4
41. Knuckley B, Bhatia M, Thompson PR. Protein arginine deiminase 4: evidence for a reverse protonation mechanism. Biochemistry (2007) 46(22):6578–87. doi:10.1021/bi700095s
42. Leppkes M, Maueroder C, Hirth S, Nowecki S, Gunther C, Billmeier U, et al. Externalized decondensed neutrophil chromatin occludes pancreatic ducts and drives pancreatitis. Nat Commun (2016) 7:10973. doi:10.1038/ncomms10973
43. Behnen M, Moller S, Brozek A, Klinger M, Laskay T. Extracellular acidification inhibits the ROS-dependent formation of neutrophil extracellular traps. Front Immunol (2017) 8:184. doi:10.3389/fimmu.2017.00184
44. Maueroder C, Mahajan A, Paulus S, Gosswein S, Hahn J, Kienhofer D, et al. Menage-a-trois: the ratio of bicarbonate to CO2 and the pH regulate the capacity of neutrophils to form NETs. Front Immunol (2016) 7:583. doi:10.3389/fimmu.2016.00583
45. Damgaard D, Palarasah Y, Skjodt K, Catrina AI, Hensen SM, Pruijn GJ, et al. Generation of monoclonal antibodies against peptidylarginine deiminase 2 (PAD2) and development of a PAD2-specific enzyme-linked immunosorbent assay. J Immunol Methods (2014) 405:15–22. doi:10.1016/j.jim.2013.12.008
46. Damgaard D, Senolt L, Nielsen MF, Pruijn GJ, Nielsen CH. Demonstration of extracellular peptidylarginine deiminase (PAD) activity in synovial fluid of patients with rheumatoid arthritis using a novel assay for citrullination of fibrinogen. Arthritis Res Ther (2014) 16(6):498. doi:10.1186/s13075-014-0498-9
47. Buck J, Sinclair ML, Schapal L, Cann MJ, Levin LR. Cytosolic adenylyl cyclase defines a unique signaling molecule in mammals. Proc Natl Acad Sci U S A (1999) 96(1):79–84. doi:10.1073/pnas.96.1.79
48. Wuttke MS, Buck J, Levin LR. Bicarbonate-regulated soluble adenylyl cyclase. J Pancreas (Online) (2001) 2(4 Suppl):154–8.
49. Litvin TN, Kamenetsky M, Zarifyan A, Buck J, Levin LR. Kinetic properties of “soluble” adenylyl cyclase. Synergism between calcium and bicarbonate. J Biol Chem (2003) 278(18):15922–6. doi:10.1074/jbc.M212475200
50. Choi HB, Gordon GR, Zhou N, Tai C, Rungta RL, Martinez J, et al. Metabolic communication between astrocytes and neurons via bicarbonate-responsive soluble adenylyl cyclase. Neuron (2012) 75(6):1094–104. doi:10.1016/j.neuron.2012.08.032
51. Xie F, Garcia MA, Carlson AE, Schuh SM, Babcock DF, Jaiswal BS, et al. Soluble adenylyl cyclase (sAC) is indispensable for sperm function and fertilization. Dev Biol (2006) 296(2):353–62. doi:10.1016/j.ydbio.2006.05.038
52. Steegborn C, Litvin TN, Levin LR, Buck J, Wu H. Bicarbonate activation of adenylyl cyclase via promotion of catalytic active site closure and metal recruitment. Nat Struct Mol Biol (2005) 12(1):32–7. doi:10.1038/nsmb880
53. Kleinboelting S, Diaz A, Moniot S, van den Heuvel J, Weyand M, Levin LR, et al. Crystal structures of human soluble adenylyl cyclase reveal mechanisms of catalysis and of its activation through bicarbonate. Proc Natl Acad Sci U S A (2014) 111(10):3727–32. doi:10.1073/pnas.1322778111
54. Zhou Y, Chen B, Mittereder N, Chaerkady R, Strain M, An LL, et al. Spontaneous secretion of the citrullination enzyme PAD2 and cell surface exposure of PAD4 by neutrophils. Front Immunol (2017) 8:1200. doi:10.3389/fimmu.2017.01200
55. Thompson PR, Fast W. Histone citrullination by protein arginine deiminase: is arginine methylation a green light or a roadblock? ACS Chem Biol (2006) 1(7):433–41. doi:10.1021/cb6002306
56. Fuhrmann J, Thompson PR. Protein arginine methylation and citrullination in epigenetic regulation. ACS Chem Biol (2016) 11(3):654–68. doi:10.1021/acschembio.5b00942
57. Zhou Y, Di Pucchio T, Sims GP, Mittereder N, Mustelin T. Characterization of the hypercitrullination reaction in human neutrophils and other leukocytes. Mediators Inflamm (2015) 2015:236451. doi:10.1155/2015/236451
58. Darrah E, Giles JT, Ols ML, Bull HG, Andrade F, Rosen A. Erosive rheumatoid arthritis is associated with antibodies that activate PAD4 by increasing calcium sensitivity. Sci Transl Med (2013) 5(186):186ra65. doi:10.1126/scitranslmed.3005370
59. Shi J, Darrah E, Sims GP, Mustelin T, Sampson K, Konig MF, et al. Affinity maturation shapes the function of agonistic antibodies to peptidylarginine deiminase type 4 in rheumatoid arthritis. Ann Rheum Dis (2017) 77(1):141–8. doi:10.1136/annrheumdis-2017-211489
60. Konig MF, Abusleme L, Reinholdt J, Palmer RJ, Teles RP, Sampson K, et al. Aggregatibacter actinomycetemcomitans-induced hypercitrullination links periodontal infection to autoimmunity in rheumatoid arthritis. Sci Transl Med (2016) 8(369):369ra176. doi:10.1126/scitranslmed.aaj1921
61. Konig MF, Andrade F. A critical reappraisal of neutrophil extracellular traps and NETosis mimics based on differential requirements for protein citrullination. Front Immunol (2016) 7:461. doi:10.3389/fimmu.2016.00461
Keywords: neutrophil, citrullination, bicarbonate, PAD2, PAD4
Citation: Zhou Y, Mittereder N and Sims GP (2018) Perspective on Protein Arginine Deiminase Activity—Bicarbonate Is a pH-Independent Regulator of Citrullination. Front. Immunol. 9:34. doi: 10.3389/fimmu.2018.00034
Received: 01 December 2017; Accepted: 04 January 2018;
Published: 18 January 2018
Edited by:
Martin Herrmann, Universitätsklinikum Erlangen, GermanyReviewed by:
Christian Maueröder, VIB-UGent Center for Inflammation Research (IRC), BelgiumMoritz Leppkes, Universitätsklinikum Erlangen, Germany
Copyright: © 2018 Zhou, Mittereder and Sims. This is an open-access article distributed under the terms of the Creative Commons Attribution License (CC BY). The use, distribution or reproduction in other forums is permitted, provided the original author(s) or licensor are credited and that the original publication in this journal is cited, in accordance with accepted academic practice. No use, distribution or reproduction is permitted which does not comply with these terms.
*Correspondence: Yebin Zhou, yebinzhou@gmail.com;
Gary P. Sims, simsg@medimmune.com