- Department of Medical Microbiology and Immunology, University of Wisconsin-Madison, Madison, WI, United States
Neisseria gonorrhoeae is an obligate human pathogen that causes mucosal surface infections of male and female reproductive tracts, pharynx, rectum, and conjunctiva. Asymptomatic or unnoticed infections in the lower reproductive tract of women can lead to serious, long-term consequences if these infections ascend into the fallopian tube. The damage caused by gonococcal infection and the subsequent inflammatory response produce the condition known as pelvic inflammatory disease (PID). Infection can lead to tubal scarring, occlusion of the oviduct, and loss of critical ciliated cells. Consequences of the damage sustained on the fallopian tube epithelium include increased risk of ectopic pregnancy and tubal-factor infertility. Additionally, the resolution of infection can produce new adhesions between internal tissues, which can tear and reform, producing chronic pelvic pain. As a bacterium adapted to life in a human host, the gonococcus presents a challenge to the development of model systems for probing host-microbe interactions. Advances in small-animal models have yielded previously unattainable data on systemic immune responses, but the specificity of N. gonorrhoeae for many known (and unknown) host targets remains a constant hurdle. Infections of human volunteers are possible, though they present ethical and logistical challenges, and are necessarily limited to males due to the risk of severe complications in women. It is routine, however, that normal, healthy fallopian tubes are removed in the course of different gynecological surgeries (namely hysterectomy), making the very tissue most consequentially damaged during ascending gonococcal infection available for laboratory research. The study of fallopian tube organ cultures has allowed the opportunity to observe gonococcal biology and immune responses in a complex, multi-layered tissue from a natural host. Forty-five years since the first published example of human fallopian tube being infected ex vivo with N. gonorrhoeae, we review what modeling infections in human tissue explants has taught us about the gonococcus, what we have learned about the defenses mounted by the human host in the upper female reproductive tract, what other fields have taught us about ciliated and non-ciliated cell development, and ultimately offer suggestions regarding the next generation of model systems to help expand our ability to study gonococcal pathogenesis.
Introduction
Infections with Neisseria gonorrhoeae (gonococcus, GC) most commonly begin at the cervix in females, which marks the dividing line between the lower reproductive tract (vagina, ectocervix) and the upper reproductive tract (uterus, fallopian tubes, ovaries, and endometrium). Cervical infections can be symptomatic or asymptomatic, but without treatment 10–20% of cervical infections ascend to cause infection of the upper female reproductive tract, including the endometrium and fallopian tubes (1). While ascending infection of the fallopian tube may be a dead-end for gonococcal transmission, it is a particularly consequential outcome for the unfortunate host. Fallopian tube infection leads to inflammation (salpingitis) and pelvic inflammatory disease (PID). Following PID, a woman's risk for ectopic pregnancy increases to 9% (from < 2%), tubal-factor infertility increases to 16% (from < 3%) (2), and chronic pelvic pain is experienced by 36% of patients (3). While the proportion of PID cases that are attributable to N. gonorrhoeae (< 40%) has fallen relative to Chlamydia trachomatis (~60%), gonococcal PID typically presents with more severe symptoms (4). The sharp rise in antibiotic-resistant gonococci raises the risk of reversing gains in preventing gonococcal PID (5).
Unlike many commonly studied bacterial pathogens, N. gonorrhoeae is not readily adaptable to laboratory animal models due to its exquisite adaptation to the human host. A female mouse model was developed nearly 20 years ago (6). With refinement in the intervening time, this model has proven very useful, especially in the understanding of complex systemic immune responses model reviewed here (7). Estradiol-treated mice become colonized following intravaginal inoculation and GC can ascend at least as far into the upper reproductive tract as the uterus (8). However, colonization is maintained for only about 10 days and resumption of the murine estrous cycle clears infection (7). Bypassing the vagina via transcervical inoculation allows for transient colonization of the uterus, with successful infection of the majority of animals for up to 24 h. The majority of animals then clear infection by 48 h (9). Despite the success of mouse models, there exist numerous biochemical, physiological, and morphological differences between murine and human female reproductive tracts, as well as between mouse and human immune systems. GC has evolved to exploit human versions of proteins for epithelial cell binding, iron acquisition, and immune evasion, among other features. For modeling human infections, a faithful reproduction of human disease occurs in experimental infection of chimpanzees (10, 11). Studies also can be performed on the infection of human male volunteers. However, both of these models are expensive and not practical for large-scale use. Moreover, human experimental infection necessarily excludes the use of females due to the risk of severe complications. Though the male urethral infection model continues to provide many important insights into host and pathogen biology, this review will focus primarily on modeling infection of the human upper female reproductive tract as the male model has been reviewed elsewhere (12, 13).
As an alternative to animal models for understanding ascending infections and the development of PID, portions of human oviducts (fallopian tubes) can be maintained in culture for days to weeks (14). While pre-menopausal samples are the best for assuring vigorous ciliary activity (15), the hormonal status of donors has no noticeable effect on ciliary activity (16). Therefore, samples obtained from any stage of the menstrual cycle are suitable for use in organ culture. Explants provide an opportunity to study gonococcal infections on a human female epithelial surface that is targeted during natural infection, complete with the complex mixture of ciliated and secretory epithelial cells and multi-layered tissue architecture. This review is intended to summarize what we have learned from fallopian tube organ culture infections with gonococci, what is known about the immunological capabilities of the fallopian tube, and how this immunology relates to our understanding of gonococcal host-pathogen interactions. Lastly, we address how the improvement of human organ and organ-like models are expanding our ability to probe specific molecular and genetic interactions between N. gonorrhoeae and the human host.
Invading the Fallopian Tube
Defining a Tissue Explant Infection Model for N. gonorrhoeae
Prior to the use of human fallopian tube organ cultures as a model ciliated epithelium, tracheal cultures had been used to study infectious agents. Trachea were shown to survive ex vivo, displaying ciliary activity for 3–5 weeks (17). In the first published attempt to model gonococcal interactions with ciliated epithelia, fallopian tubes were used alongside tracheal samples from embryonic chicken, cow, human, and adult mouse (16). Non-piliated gonococci were found to grow well on fallopian tube tissue, with an inoculum as low as 10 colony forming units (CFU) reaching a maximal density (107-1010 CFU/mL) in 2–4 days. A higher inoculum (103 CFU) reached a maximum density after 1–2 days and caused a complete loss of ciliary activity by 5–6 days. For comparison, uninfected fallopian tube tissue maintained beating cilia for between 14 days and 1 month (or more). Tissue culture media such as Eagle's Minimal Essential Medium (MEM) is sufficient to support tissue survival, though gonococci grow poorly in this media (15). Gonococci will grow, however, in conditioned media from fallopian tube or media supplemented with tissue homogenate. Work that compared rabbit oviducts in culture to human fallopian tube revealed that, similar to the previous work in trachea, the presence of any tissue supports the multiplication of GC in culture. Gonococci, however, neither bind to nor damage rabbit oviduct, and do not cause any reduction in ciliated cell activity (18). Piliated gonococci likewise adhered poorly to the mucosal epithelium of rabbit, pig, cow (19), and guinea pig (20). In all cases, no noticeable histopathology or loss of ciliary activity was observed in animal oviducts compared to human fallopian tubes infected in parallel. One of the seminal observations, made with both piliated and non-piliated strains, is that gonococci bind preferentially to secretory (non-ciliated) cells, though it is the ciliated cells that later die (18, 21). Gonococci are also able to quickly invade the apical side of non-ciliated cells (within ~20 min) (18), and then exit from the basolateral side (transcytosis) but are not observed invading or residing inside ciliated cells (21). When non-pathogenic Neisseria pharyngis (now known as N. cinerea) was grown in fallopian tube organ culture, the bacteria survived at similar levels to N. gonorrhoeae, but failed to elicit any of the decrease in ciliary activity that is characteristic of GC (15), suggesting that human pathogenic Neisseria possesses unique factors capable of damaging human female reproductive tract epithelia.
Epithelial Damage and the Inflammatory Response to Gonococci
Early work in the human fallopian tube organ culture model established that GC could colonize explants and recapitulate the damage seen in patients with gonorrhea (22). The specific damage of primary concern is the death of ciliated epithelial cells, which have physiologic functions important in fertility. Loss of the ability of ciliated cells to participate in transporting the fertilized ovum to the uterus is considered a major predisposing factor for tubal factor infertility and ectopic pregnancy (23). In culture, uninfected fallopian tube explants display robust ciliated activity for more than 2 weeks, but tissues infected with non-piliated gonococci show decreases in ciliary activity starting at about 36 h post-infection and exfoliation of ciliated cells starting around 64 h (18). Complete loss of ciliated cell activity occurs by 4–6 days post-infection (16, 18). Multiple groups observed that a decrease in ciliary beat frequency precedes the appearance of visible damage of the epithelial surface (24, 25). The magnitude of reduction in ciliary activity and rapidity of sloughing is more pronounced in infections with piliated gonococci compared to non-piliated, even with equal or fewer CFU recovered from tissues (25).
Ciliated cells are the first to die despite the attachment of gonococci to non-ciliated cells. This observation led to a search for soluble factors (such as toxins) present in filter-sterilized gonococcal broth cultures (cell-free supernatant) and filter-sterilized media from gonococci-infected fallopian tube cultures. Both supernatants were found to contain a heat-stabile, toxic component that is partially removed by adsorption to limulus amoebocyte lysate (LAL), and capable of causing the same ciliated cell death as observed in gonococcal infections (26). This toxic effect was attributed to lipopolysaccharide (LPS), which is present in gonococci as a low-molecular weight version lacking the repeating O-antigen and referred to as lipooligosaccharide (LOS). LOS was purified and shown to decrease ciliary activity at lower concentrations than the microgram quantities measured in infected organ cultures (27). The toxic effect of gonococcal LOS, like damage from gonococcal infection, also appeared to be a human-specific phenomenon, not affecting the ciliary activity of rabbit, pig, or cow oviducts (28). This observation would seem curious now, as LPS/LOS signaling via the eukaryotic immune sensor Toll-like receptor 4 (TLR4) has been characterized as a conserved pathway leading to inflammatory responses in all of the above species (29, 30). Adsorption of LOS with LAL, however, did not eliminate all of the soluble toxic activity produced by gonococci, implicating another factor(s) causing toxicity. Around the same time, it was just beginning to be recognized that gonococci release soluble fragments of peptidoglycan (PG), in particular monomeric fragments consisting of N-acetylmuramic acid and N-acetylglucosamine sugars linked to three- or four-amino acid long peptide chains (with 80% as tripeptide, 20% as tetrapeptide) (31, 32). Treatment of organ cultures with isolated PG monomers, reduces ciliary activity and causes death and sloughing of ciliated cells (33). The later isolation from Bordetella pertussis of tracheal cytotoxin (TCT), a tetrapeptide PG monomer identical to the minority monomer species released by gonococci, reinforced the idea that small PG monomers can cause ciliated cell cytotoxicity, in this case in the trachea of hamsters (34, 35). The further discovery that PG is found as a contaminant in crudely purified LPS preparations (36), and the recognition that PG monomers are sensed differently by the same receptor in different species (37), implicates PG as a major mediator of species-specific oviduct toxicity. Certainly, both LOS and PG are toxic products, abundantly released from growing gonococci and capable of contributing individually to inflammation. Together, it is likely they have an even greater impact, as PG monomers are known to synergize with LPS to provoke a larger host response than either alone (38).
While gonococcal LOS and PG fragments are ascribed causative roles in epithelial damage, neither is a “toxin” in the same sense as a lytic pore-forming toxin or a compound capable of poisoning cellular processes to create a direct toxic effect. Rather, LOS and PG are recognized by the host as pathogen-associated molecular patterns (PAMPs) and induce programmed defense responses. One of the first host inflammatory responses measured from gonococcal infection of fallopian tube organ cultures was the induction of tumor necrosis factor (TNF) (39). Addition of TNFα to fallopian tube explants reduces ciliary activity in a dose-dependent manner, and reproduces the characteristic death and sloughing of ciliated cells (Figure 1). Rising concentrations of TNFα during gonococcal infection correlate linearly with a decrease in ciliary activity (40). In the analogous B. pertussis system, it should be noted that interleukin (IL) 1 (and not TNFα) was implicated as the host factor driving respiratory epithelial damage. IL-1 addition was shown to be capable of inducing ciliated cell death similar to that seen in TCT treatment of hamster tracheal rings (41). Though the host organism and infection system are different, the role of IL-1 is noteworthy as this cytokine is also implicated as the driver of epithelial damage in Chlamydia trachomatis infections of human fallopian tube organ cultures (42).
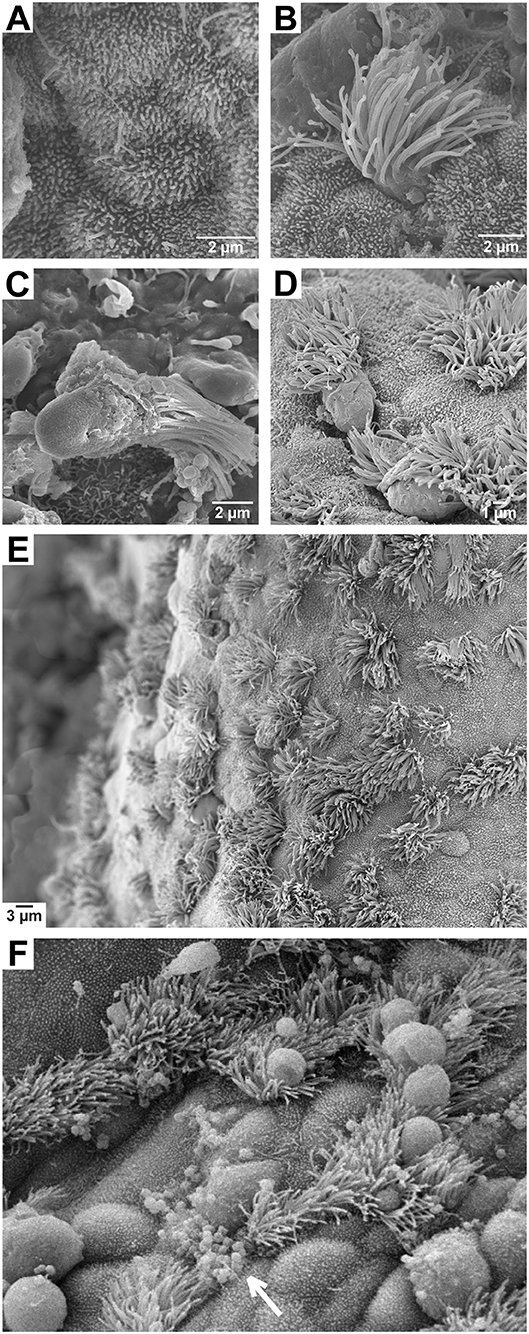
Figure 1. Scanning electron micrographs of fallopian tube explants. (A) Non-ciliated (secretory) epithelial cell; (B) ciliated epithelial cell; (C) ciliated epithelial cell sloughed during gonococcal infection; (D) ciliated cells sloughing following a 24 h treatment with 2 ng/mL TNFα; (E) an untreated epithelial layer displaying normal cell morphology; (F) an epithelial layer during gonococcal infection showing binding of most bacteria to non-ciliated cell surfaces (arrow) and swelling of cells that precedes ciliated cell sloughing.
Gonococcal infection is capable of inducing transcription of numerous cytokines and chemokines in epithelial cell lines such as ME-180, HeLa, and HaCaT via activation of the transcription factor nuclear factor kappa-B (NF-κB) (43). In fallopian tube explants, TNFα and IL-1β, as well as the inflammatory cytokines/chemokines IL-6, monocyte chemoattractant protein-1 (MCP-1, CCL2), macrophage inflammatory protein-1β (MIP-1β,CCL4), and granulocyte-macrophage colony-stimulating factor (GM-CSF) are made in response to gonococcal infection and detected by enzyme-linked immunosorbent assay (ELISA) after as little as 3 h (44, 45). While levels of TNFα, IL-1β, IL-6, and MCP-1 seem to generally increase out to 24 h, MIP-1β and GM-CSF appear to peak at 12 h and decline by 24 h. The kinetics of some cytokine responses were shown to vary between piliated and non-piliated variants, with cytokine induction by piliated gonococci potentially delayed by several hours (45). Since the vast majority of gonococci recovered from symptomatic natural infections are piliated (46, 47), a delay in cytokine induction in fallopian tube explants may indicate a role for pili in suppressing certain immune responses during ascending infection.
Nitric oxide (NO) is another component of the inflammatory host response that is produced on mucosal epithelia, in particular NO made by inducible nitric oxide synthase (iNOS, encoded by nos2). In hamster tracheal epithelial (HTE) cells, TCT and IL-1 were shown to induce nitrite production (a proxy for NO), and applying nitric oxide synthase inhibitors prevented the loss of ciliary activity in hamster tracheal rings treated with TCT (48). In the hamster trachea, NO production localizes only to the non-ciliated (secretory) epithelial cells (49). Though intriguing, this finding regarding the distribution of NO production has not been reproduced in humans or in reproductive tract tissues. During gonococcal infection of fallopian tube organ cultures, iNOS transcription is induced ~35-fold, but treatment of uninfected fallopian tube tissues with the NO donor S-Nitroso-N-acetylpenicillamine (SNAP) did not induce cellular damage as measured by lactate dehydrogenase (LDH) release (50). Treatment of infected tissues with iNOS inhibitors also produced mixed results in reducing cellular damage (50). The inhibition of fallopian tube damage that was seen during treatment with N(G)-Nitro-L-arginine methyl ester (L-NAME) corresponded with a reduction in bacterial CFU. A decrease in bacterial proliferation presumably accounted for a corresponding reduction in tissue damage. Interestingly, a reduction in viable gonococci during inhibition of iNOS aligns with data that iNOS activity increases gonococcal survival within primary human cervical epithelial (pex) cells (51). While it is unclear whether NO participates directly in the damage observed in the fallopian tube during gonococcal infections, GC has adapted methods to avoid killing and perhaps even exploit NO during host colonization.
Matrix metalloproteinases (MMPs) are host enzymes that function in the degradation of extracellular matrix, disassembly of cellular junctions (including for immune cell infiltration), and activation (by cleavage) of cytokines and growth factors (52). Numerous bacteria or bacterial products (including PG and LPS) have been shown to induce various MMPs (including MMP1, 2, 3, 7, 9, 10, and 11) (53–56). When bacteria induce MMPs, host tissue can sustain collateral damage, such as when Gram-positive Enterococcus faecalis activates MMP9, contributing to adverse outcomes of surgical wound infections (57). During gonococcal infection of fallopian tube epithelial cells, MMP2 accumulates intracellularly while MMP9 secretion is increased (MMP3 and MMP8 levels are unchanged) (58). In fallopian tube explants, MMP8 expression is increased following GC infection, while transcription of MMP3 and MMP9 (as well as the TIMP metallopeptidase inhibitor 1, TIMP-1) are unchanged (59). The functions and localization of MMPs, when coupled with data indicating their induction during fallopian tube infection, makes these enzymes prime candidates for mechanistic involvement in epithelial damage. In cell culture, gonococci cause disruption of E-cadherin and β-catenin from adherens junctions between fallopian tube epithelial cells (60). GC also disrupt the apical junctions of polarized HEC-1-B (endometrial) and T84 (colonic epithelial) cells by activating epidermal growth factor receptor (EGFR) signaling (which also involves redistribution of β-catenin) (61). The disassembly of apical junctions is also seen in an endocervical tissue model of infection, where GC activates intracellular non-muscle myosin II and induces calcium flux to promote the exfoliation of columnar epithelial cells (62). Additional investigation is needed into the composition of cellular junctions between ciliated and non-ciliated fallopian tube epithelial cells, the activity of bacterially-induced MMPs, and the intracellular signaling pathways activated by gonococci to determine what factors make ciliated cells especially sensitive to exfoliation.
The exact mechanisms by which ciliated cells die and are removed from the epithelial layer remain unknown. Morphologically, cells do not appear to lyse as would be expected from necrosis or pyroptosis (though IL-1β is released during infections). In cultured human fallopian tube epithelial cells, GC inoculated at various multiplicities of infection (MOI = 1, 10, or 100) all result in production of TNFα, which can cause apoptosis. However, only the lowest MOI showed significant Caspase-3 activation (indicative of apoptotic cell death) (63). Since TNFα addition alone can induce apoptosis and addition of more GC (higher MOI) blocks the TNFα-dependent apoptosis, it can be hypothesized that GC contact or some GC-produced soluble factor produces an anti-apoptotic effect. Indeed, GC is known to increase the expression of anti-apoptotic factors in human urethral epithelial cells (64, 65), and human End1 endocervical epithelial cells (66). GC also confers protection from apoptosis in neutrophils (67, 68). However, GC also induces pro-apoptotic genes in HeLa cells (69). The outer membrane porin PorB has been implicated in induction of apoptosis by (1) trafficking to mitochondria in murine bone marrow-derived macrophages (BMDMs) and human THP-1 macrophages, and (2) initiating the intrinsic pathway of apoptosis in mouse BMDMs (70). During fallopian tube explant infections, GC were more often seen contacting (or in close proximity to) non-ciliated cells, which could facilitate the induction of a protective response. Ultimately, a better understanding is needed of the differences between ciliated and non-ciliated cells in their ability to sense and respond to TNFα and other components of the host defense response.
Factors Conferring Adherence to Fallopian Tube Epithelia
Adhering to host surfaces, whether to facilitate physical anchoring for replication or to induce uptake into host cells, is a critical step in the life cycle of many bacterial pathogens. Such is the case for GC, which can multiply extracellularly on mucosal surfaces, or proceed through a stepwise process of first binding tightly to columnar epithelial cells, being endocytosed, transiting through epithelial cells, and exiting into the subepithelial space (71, 72). Early observations of N. gonorrhoeae adherence during human fallopian tube organ culture infection focused on the two best known (and most easily observable) mediators of adherence: pili and opacity (Opa) proteins. Piliated GC bind to (and damage) the mucosal surface more rapidly than non-piliated variants of the same strain (25). Piliated gonococci were also observed binding to the tips and surfaces of microvilli on non-ciliated cells (73), a phenomenon later observed in infections of cultured HEC-1-B endometrial carcinoma cells as a precursor to cellular invasion (74). The exact receptor that gonococcal pili engage during fallopian tube infection for either adherence or invasion is unknown. Both CR3 (a CD11b/CD18 integrin heterodimer) and CD46 (MCP) have been implicated as ligands for pili (75, 76), and both are found on the fallopian tube epithelium (77, 78). Expression of CR3, however, decreases progressively in ascending tissues, reaching its lowest relative level in the fallopian tube (77). In addition, questions remain as to whether the interaction of pili with CD46 functions to mediate adherence or rather a downstream intracellular signaling event (79–82). In the endometrium, carcinoembryonic antigen-related cell adhesion molecules (CEACAMs/CD66), asialoglycoprotein receptor (ASGP-R), and CR3 have each been implicated in gonococcal adherence (83).
Gonococci can still bind to and damage fallopian tube epithelia through pilus-independent mechanisms (18), and Opa proteins alone are sufficient to confer adherence to fallopian tube epithelium (84). In the only study to look exclusively at the role of Opa proteins in attachment to fallopian tube, Opa- variants of F62-SF were found to bind exclusively to non-ciliated cells (as observed previously) as did OpaC+ and OpaD+ strains (which bound to microvilli). Strains expressing OpaB, however, were seen on both ciliated and non-ciliated cells, with OpaA-expressing strains binding to ciliated cells in large clumps (85). Interestingly, the OpaC and OpaD-expressing variants were judged the most damaging to epithelia while OpaA-expressing were the least damaging. Despite Opa proteins being well characterized as adhesins and routinely associated with successful human infections (86), early work in fallopian tube organ culture suggested that piliated and transparent (Opa-) bacteria bind better (by 10–100x) to fallopian tube explants than piliated and opaque (Opa+) bacteria (22, 73). In laparoscopic sampling from the fallopian tubes of women with salpingitis, the same group recovered predominantly GC with transparent colony phenotypes (>95% Opa-) while endocervical isolates were 50% or greater Opa+ (87). The majority of Opa proteins (which vary in number between gonococcal strains) bind to distinct CEACAMs, though at least one Opa binds instead to heparin-sulfate proteoglycans (HSPG) (88). In a study of low-passage isolates of GC from cervix and male urethra, the majority of strains bound to transfected Lec11 cells (a CHO derivative) stably expressing CEACAM1, CEACAM5, or CEACAM6. Fewer showed robust binding to CEACAM3 and very few bound CEACAM8 (89). Human CEACAM5, when expressed in mice, also contributes to increased recovery of GC during murine lower genital tract infection (90). The expression levels of particular CEACAMs on cells of the human female genital tract has been questioned, however, with evidence that CEACAM1, 3, 5 (CEA), 6, and 8 are not highly expressed on primary fallopian tube, primary cervix, HEC-1-B endometrial cells, or HeLa cervical carcinoma cells (91). It is not known which CEACAM (or HSPG) is the most available target of Opa proteins in the fallopian tube, nor is it known what threshold level of expression is needed for Opa-CEACAM binding in any given tissue. It is clear that Opa proteins facilitate adherence to cultured epithelial cells and contribute to bacterial fitness during successful colonization of the murine lower genital tract (92). In primary human ectocervical and endocervical cells, however, the absence of Opa expression does not decrease adherence (76). It is still possible that Opa proteins contribute to the mix of adhesins needed to address the changing receptor availability and immunological context encountered during the progression of ascending from the endocervix to the fallopian tube (83). In a study of seven patients with acute salpingitis (fallopian tube inflammation), laparoscopic isolation of bacteria from fallopian tube and cul-du-sac revealed a higher proportion of Opa- than Opa+ in these tissues (22). The laparoscopy samples also had a consistently higher proportion of Opa- GC than matched cervical isolates from the same patient. These data suggest that whatever factors are driving Opa protein production at the endocervix, Opa proteins may be turned off or selected against when bacteria are infecting the fallopian tube.
Gonococcal lipooligosaccharide has already been discussed as an important trigger of host inflammatory responses, but LOS on the cell surface may also function in bacterial binding and invasion of fallopian tube epithelial cells. Several different gonococcal LOS variants bind to Galectin-3, a β-galactoside-binding protein expressed on non-ciliated cells of fallopian tube epithelium as well as on HEC-1-B endometrial adenocarcinoma and PC3 human prostate adenocarcinoma cells (93). The “triggering receptor expressed on myeloid cell-2” (TREM-2) was also identified as a ligand for gonococcal LOS, and is constitutively expressed on human fallopian tube epithelium, ME-180 and HeLa cervical carcinoma cells, ectocervical Ect1/E6E7 cells, endocervical End1/E6E7 cells, vaginal Vk2/E6E7 cells, and THP-1 monocytic cells (94). The resident microbiota in the female lower reproductive tract contribute sialidase activity that processes the terminal sialylation present on LOS, a process that has been implicated in promoting transmission to males by unmasking a terminal galactose that binds to ASGP-R on urethral epithelial cells (95, 96). It is unclear how the sialylation state of LOS impacts the pathogenesis of fallopian tube infection. Increasing LOS sialylation is known to decrease Opa-dependent invasion of Chang conjunctival and ME-180 cervical epithelial cells (97). Several studies also report rapid internalization of gonococci into fallopian tube secretory epithelial cells (21, 22), perhaps implicating a low level of LOS sialylation during ascending infection.
Natural hormonal cycling likely plays a role in the expression of gonococcal factors important for adhesion, immune evasion and virulence. Before specific Opa proteins were recognized as the factors determining colony opacity/transparency, variations in the visual appearance of isolates were noticed to change (along with the likelihood of recovering viable gonococci) based on the stage of the menstrual cycle (47). Isolates obtained in close temporal proximity to menstruation were found to lack the surface proteins associated with opacity. The overall variation in recovery phenotypes during the menstrual cycle was attributed to the action of progesterone (or substances acting similarly to progesterone). While progesterone has been investigated for its ability to enhance survival of gonococci in primary cervical epithelial (pex) cells (51), its role in ascending infection is unknown. However, since progesterone causes a significant lowering of ciliary beat frequency in fallopian tube (98), progesterone could reasonably be expected to have some impact on gonococcal adherence or colonization in this tissue. Additionally, fallopian tube tissue from users of both hormonal and non-hormonal (copper intrauterine device) contraception display changes in the surface expression of CD46 and the HSPG syndecan-1, but not CD66 (CEACAM) (78). Much remains to be determined about how bacteria, adherent at the cervix, transit the uterus to enter the fallopian tube. It is likely that gonococci have adapted to utilize numerous host receptors in sequence (83), and are able to adjust accordingly to whatever tissue environment they find themselves in as they make their way into the upper female reproductive tract.
Insights From Chlamydia trachomatis Infections in Fallopian Tube Explants
Chlamydia trachomatis (Ct) is the most commonly reported sexually transmitted infection (STI) and another bacterium that can ascend to the fallopian tubes, causing PID and tubal-factor infertility. Fallopian tube organ culture explants have also been used to study the pathogenesis of Ct, with early observations in this system noting that Ct is able to bind to and infect both ciliated and non-ciliated cells (99). Unlike GC infection, ciliated cell function did not appear to be affected by Ct, though microvilli were lost on infected non-ciliated cells, which became rounded and lost attachment to neighboring cells. By 72 h post-infection, ruptured cells could be observed, characteristic of the cell lysis that results from release of infectious elementary bodies (EBs) at the end of the Ct intracellular life cycle. The observed loss of cellular polarity and disruption of cell-cell junctions in Ct-infected fallopian tube is accompanied by β-catenin recruitment from adherens junctions to Chlamydial inclusions (100). While disassembly of junctions likely contributes directly to epithelial disruption, β-catenin is also tightly regulated at the cellular level as a component of the developmentally important Wnt signaling pathway. Increased Wnt signaling during Ct infection induces numerous changes in fallopian tube tissue, including up-regulation of the stem-cell marker Olfactomedin 4 (OLFM4) (100), which is a target of NF-κB and Notch signaling pathways. OLFM4 also participates in the curtailing of innate immune responses by negatively regulating nucleotide-binding oligomerization domain-containing protein 1 (NOD1)- and NOD2-dependent NF-κB activation (101).
Cultured epithelial cell lines (HeLa, SiHa, HT-29, SW620, and primary endocervical) infected with Ct secrete proinflammatory cytokines (IL-8, IL-6, GM-CSF, and GROα/CXCL1), but cytokine secretion is delayed until 20–24 h post-infection, after lysing cells release IL-1α (102). Blocking IL-1α blunts the cytokine burst, suggesting that neighboring epithelial cells are sensing IL-1α and initiating an amplification of the inflammatory response. In fallopian tube organ cultures, addition of exogenous IL-1α was shown by scanning electron microscopy (SEM) and histological staining to cause damage similar to Ct infection, with IL-1 receptor antagonist (IL-1RA) and p38 mitogen-activated protein (MAP)-kinase blockade (downstream of IL-1 sensing) reducing epithelial damage (42). The ability of cytokines (TNFα in the case of GC, IL-1α for Ct) to exacerbate immune-driven pathology is common to both infections, as is potentially the role of matrix metalloproteinases (MMPs) in tissue disruption. Transcriptional analysis of Ct infecting monolayers of HEp-2 cells revealed induction of MMP2 and MMP9 (103), the same MMPs with altered expression during GC infection of fallopian tube (58). Though Ct and GC have different lifestyles that bring them into conflict with the host in different ways, both organisms induce a pro-inflammatory response in the fallopian tube epithelium, where the long-term sequelae of infection appear to be the result of host-driven inflammatory pathology.
Defending the Fallopian Tube
Pattern Recognition Receptors in the Innate Immune Response
Colonization of GC in the upper female reproductive tract (FRT) involves bacteria binding to and interacting with epithelial cells. Epithelial cells are ready to respond to the presence of invading pathogens through the expression of a variety of microbial pattern recognition receptors (PRRs). Surveys of PRR expression throughout the FRT revealed expression of all identified toll-like receptors (TLR1-10), as well as cytosolic sensors NOD1, NOD2, retinoic acid-inducible gene I (RIG-I) and melanoma differentiation-associated protein 5 (MDA5) (104–107). In general, receptor expression appears to be constitutive and nearly ubiquitous across different regions of the FRT, but notable differences exist in the levels of receptor expression between tissues. TLR2 transcripts are higher in the fallopian tube and cervix than other FRT sites, while TLR4 transcript is higher in the fallopian tube and endometrium with lower expression in the lower tract (104). Immunohistochemical staining confirmed the presence of TLR1-3 and 5-6 throughout the FRT, and indicated detectable TLR4 only in the fallopian tubes, uterus, endometrium, and endocervix (TLR7-10 were not tested in this work) (105). The absence of TLR4 from the ectocervix and vagina would presumably reduce the triggering of inflammatory signaling that may be disadvantageous for maintaining a healthy vaginal commensal community. Transcript for TLR10 is perhaps the most restricted, having first been reported in the endometrium (108). That finding was disputed by later work that surveyed the entire FRT and found detectable expression only in the fallopian tube (106). Differences in detection may be due in part to variations in expression that occur at different points in the menstrual cycle reviewed in (109). A high relative expression of TLR10 in the fallopian tube is particularly interesting since TLR10 appears to act as an anti-inflammatory TLR. TLR10 heterodimerizes with TLR2 (which also has high expression in the fallopian tube) to inhibit inflammatory signaling and promote the production of anti-inflammatory IL-1 receptor antagonist (IL-1Ra) (110). The presence of TLR10 may then serve as a check on out-of-control inflammation in the Fallopian tube. The localization of TLRs can also be further restricted (or enriched) at the cellular level within the fallopian tube. Though TLR4 expression was reported to be generally high in fallopian tube, this receptor may be preferentially present on the surface of oviductal stromal fibroblasts, underneath the epithelial layer, and not on the epithelial cells themselves (111). The absence of TLR4 on epithelial cells could function to protect against the spurious activation of pathologic inflammation in the fallopian tube. However, the demonstrated ability of GC to disrupt epithelial integrity and invade through the epithelial layer (18, 21) likely puts the bacterium in proximity to TLR4 on fibroblasts. Some evidence also exists that ciliated and non-ciliated epithelial cells express TLRs at different levels, with ciliated cells expressing all 10 TLRs at higher levels than non-ciliated cells (112). It is unclear as of yet how differential responsiveness to TLR agonists might relate to the different cell fates seen during GC infections.
The cytosolic PRRs NOD1 and NOD2 recognize various fragments of PG (113), which are shed in abundance by gonococci and known to cause damage during fallopian tube infection (33). Synthetic muramyl peptides, some of which are similar to those naturally released by gonococci, can be endocytosed by HEK293T cells to activate NOD1 and NOD2 (114). When the fallopian tube, endometrium, endocervix, and ectocervix regions were surveyed for NOD receptor expression, all tissues were found to express NOD1, NOD2, and the adaptor receptor interacting serine/threonine kinase 2 (RIPK2) (106). Fallopian tube was shown to have the highest relative expression of both NOD1 and NOD2 compared to other FRT tissues (107). Primary fallopian tube epithelial cells were also shown to be capable of generating an IL-8/CXCL8 response to the addition of the NOD1 agonist D-gamma-Glu-meso-diaminopimelic acid (iE-DAP) and the NOD2 agonist muramyl dipeptide (MDP) (106). Fallopian tube organ cultures treated with conditioned media from N. gonorrhoeae or N. meningitidis broth cultures both induce an IL-8 response proportional to the release of human NOD1 agonist (115).
Gonococcal products, whether on the bacterial surface, secreted, or delivered by membrane vesicles, have been shown to interact with several TLRs and NOD receptors, which likely initiate the early innate immune signaling during ascending infection. Cell-free supernatant and gonococcal lysates (containing whole PG sacculi) activate NF-κB via human NOD1 and NOD2 (116). The NOD1 activation is dependent upon the periplasmic conversion of a gonococcal cell wall rich in tetrapeptide-stem PG into soluble fragments with primarily tripeptide stems that are agonists for human NOD1 (117). Gonococci can activate human NOD2 with released glycosidically-linked peptidoglycan dimers, and with multimeric PG (as might result from cell lysis) that is converted by host lysozyme to produce reducing disaccharide-containing PG fragments (118). Gonococcal lipooligosaccharide activates TLR4 signaling, including both the MyD88 pathway that activates NF-κB and the interferon regulatory factor 3 (IRF3) pathway that activates Type I interferons (119). The acylation state of the lipid A molecule is critical to gonococcal TLR4 activation, with the wild-type hexa-acylated version required for TLR4-dependent signaling (120). The placement of various sialic acid moieties (sialylation) on LOS appears not to influence TLR4 activation (121), while the presence of phosphoryl substituents on the lipid A molecule does influence TLR4 activation (119). Pathogenic Neisseria are potent activators of TLR4 signaling in part because they are prone to membrane blebbing, or the release of outer membrane vesicles, which functions to spread LOS, membrane proteins and associated intracellular cargo out from the cell, increasing the potential for bacterial products to contact PRRs (122–124).
Despite the lack of TLR4 expression in certain regions of the FRT, and a confirmed lack of expression in isolated vaginal, ectocervical and endocervical cells lines, GC is still able to activate NF-κB and induce proinflammatory cytokines in End-1 endocervical cells in the absence of TLR4 (125). TLR2 has long been recognized as another PRR activated by pathogenic Neisseria, an activity that has been largely attributed to recognition of the major outer membrane porin (PorB) (126, 127). Most studies of porin-TLR2 interaction have involved PorB from N. meningitidis, which is considered a stronger TLR2 agonist than N. lactamica PorB, which has been investigated for its vaccine adjuvant potential (128, 129). Gonococcal PorB, however, has recently been shown to have low TLR2 agonist activity when protein is refolded during purification, and higher TLR2 agonist activity when PorB is unfolded or aggregated (130). The outer membrane lipoprotein, Lip (also known as H.8 antigen) from GC has been shown to activate an NF-κB reporter in HEK293 cells expressing TLR2, and induce IL-6 and IL-8 from End-1 endocervical epithelial cells (131), confirming that GC possesses multiple potential TLR2 agonists.
Gonococci are able to activate additional cellular surveillance pathways, in particular activation of TRAF-interacting protein with forkhead-associated domain (TIFA) (132), and cyclic-GMP-AMP synthase (cGAS) (133). TIFA-dependent signaling is initiated by the intracellular detection of heptose-1,7-bisphosphate (HBP), a byproduct of gonococcal LOS production. Though a role for HBP has not yet been explored in fallopian tube infections, gonococcal supernatant containing HBP induces inflammatory responses in cultured End-1 endocervical epithelial cells, human macrophages, and human neutrophils (132). HBP has been shown in Helicobacter pylori infections to produce an earlier NF-κB response than NOD1 agonist (134). The cGAS enzyme is activated by detection of cytosolic double-stranded DNA (dsDNA) and initiates STING/TBK-1/IRF3 activation leading to induction of type I interferon. During GC infection of human monocytes (THP-1 cells), TLR4 and cGAS synergize to induce production of interferon-β (IFN-β) (133). Even with this observation, it is uncertain what role IFN-α or IFN-β has during gonococcal infection, since IFN-β can be both beneficial and detrimental to the host in clearing infection. In addition, the source of cytosolic gonococcal dsDNA sensed by cGAS remains uncertain, though presumed to originate from spontaneous bacteriolysis. It is therefore plausible that the proliferation of extracellular bacteria releasing TLR4 agonist, while some bacteria are phagocytosed and lyse inside responding macrophages, creates a scenario for increased IFN-β production during fallopian tube infection.
Immune Cells in Fallopian Tube Surveillance
Like other mucosal surfaces, the fallopian tube contains a complement of resident immune cells that can be found within the epithelial layer and just behind the epithelium in the lamina propria. A flow cytometry-based survey of the entire human female reproductive tract across 28 patients ranging from 26 to 66 years old revealed 6–20% of the total cellular content to be leukocytes (CD45+), with fallopian tube and uterus containing a higher proportion of immune cells than the cervix or vagina (135). Across all tissues, 30–60% of the leukocytes were T cells (CD3+), with granulocytes (CD66b+, likely polymorphonuclear leukocytes, PMNs) as the major population observed in the fallopian tube. However, a later study that focused exclusively on the fallopian tube epithelium and utilized both flow-cytometry and immunohistochemistry with an updated and expanded set of cellular markers reached a somewhat different conclusion on leukocyte distributions (136). Ardighieri et al. (136) surveyed 10 patients (5 pre-menopausal, 26-35; 5 post-menopausal, 63-73) and observed macrophages (CD68+ CD163+) and dendritic cells (DCs) (CD11c+) as the most abundant innate immune cells present in the fallopian tube, at ratios (to total nucleated cells) of ~1/30 and 1/21, respectively. While all combinations of CD11c+/− and CD163+/− cells (overlapping macrophage and DC markers) were present, CD11c+ CD163− DCs were abundant. These cells were observed at regular intervals, with cell bodies in the basal lamina and long intraepithelial projections facing toward the lumen. CD11c+ CD163+ cells were the most abundant population in the lamina propria and muscle wall (under the epithelium). In this same analysis (136), neutrophils were noted as a minor population localized almost exclusively intravascularly and present only in the lamina propria and muscle wall, the same localization as minor populations of mast cells (CD117+), plasmacytoid DCs (CD303+), plasma cells (CD138+), and regulatory T cells (CD3+Foxp3+). Whether or not neutrophils are a major population in the normal fallopian tube, these cells are well characterized as important components of the cellular response to GC infections. Neutrophils migrate rapidly from the bloodstream to sites of tissue inflammation and are found in high numbers in gonococcal infection of the male urethra and female cervix. As the role of neutrophils in GC infection of the female reproductive tract has recently been reviewed (137), the following section will focus on other fallopian tube-resident immune cell types and the outcomes of their potential interactions with gonococci.
Dendritic cells are critical front-line phagocytes and potent antigen-presenting cells, which function in the activation of T cells (138). DCs that encounter antigen in the presence of proinflammatory signals are induced to produce IL-12 and costimulatory molecules that encourage the development of T helper cells. Contact with GC, however, has been shown to induce expression of anti-inflammatory molecules (IL-10, PD-L1) from human and murine DCs, and both human and murine DCs exposed to GC are less able to stimulate proliferation of CD4+ T cells (139, 140). Porin (PorB) delivered via outer membrane vesicles (OMVs), has been shown to contribute to the suppressive effect of GC on DCs, in spite of any ability of porin to engage stimulatory TLR2 signaling (130). Though the mechanics of GC suppression of DC activity are still incompletely understood, several potentially important receptor-ligand interactions have been identified. Gonococcal LOS binds to C-type lectin receptors on DCs, with variations in glycosylation influencing which receptor is engaged (141). Wild type LOS from strain F62 with terminal N-acetylgalactosamine (GalNAc) binds to “macrophage galactose-type lectin” (MGL, also present on DCs). An lgtD mutant with terminal galactose is recognized through an unknown receptor. While an lgtB mutant with terminal N-acetylglucosamine (GlcNAc) binds to dendritic cell-specific intercellular adhesion molecule-3-grabbing non-integrin (DC-SIGN). The lgtD and lgtB deletions mirror the phenotypes that occur naturally due to slipped-strand mispairing. All of these variants have the ability to stimulate DCs to activate T cells, although LOS with a terminal GalNAc induces less IL-10 than other variants. This finding suggests that interaction with DCs may exert selective pressure for GC to vary LOS during host infection. Opa proteins, through their engagement of CEACAM1, have been shown to reduce the ability of DCs to stimulate memory responses from T cells against human immunodeficiency virus-1 (HIV-1), in part through down-regulation of the costimulatory molecule CD83 (140). It should be noted, however, that other groups have previously observed up-regulation of CD83 (and major histocompatibility complex class I, MHC-I) on DCs exposed to GC, as part of investigating how GC promotes HIV-1 infection of DCs (142). The interaction of GC with DCs is particularly interesting since DCs are critical target cells for HIV-1 infection (both pathogens share DC-SIGN as a receptor). Infections with GC have been shown to create an increased risk for HIV-1 infection through multifactorial mechanisms that remain unclear (143).
Macrophages, the next most abundant immune cell type in the fallopian tube, are primary phagocytes that are likely to encounter GC that has invaded through or between epithelial cells and entered the submucosa. Fallopian tube explants release cytokines and chemokines capable of recruiting macrophages during gonococcal infection, such as MCP-1 (CCL2) and MIP-1β (CCL4) (45). Macrophages exposed to GC produce proinflammatory cytokines such as TNFα, IL-1β, IL-6, MCP-1 (CCL2), MIP-1α (CCL3), MIP-1β (CCL4), RANTES (CCL5), GROα (CXCL1), and CXCL10, which also likely promote PMN and T cell recruitment (144–146). Macrophages are able to phagocytose GC, with in vitro infections of human peripheral blood mononuclear cells (PBMCs) resulting in near complete internalization within 1 h of exposure and the killing of >60% of internalized bacteria in that same period (147). Similar results were reported for THP-1 monocytes, with >60% killing by 2 h post-phagocytosis and >80% by 5 h (146). Despite rapid eradication of the majority of the population inside PBMCs, by 6 h post-infection GC are seen persisting in macrophages. Survival occurs, in part, through the activity of the lipoprotein Ng-MIP (in the macrophage infectivity potentiator family of peptidyl-prolyl cis/trans isomerases) and by gonococcal manipulation of host gene expression to acquire necessary iron while inside (or in close association with) macrophages (146, 147). Gonococci that have been phagocytosed by macrophages are then capable of manipulating or inducing various cell death pathways. In human monocyte-derived macrophages (MDMs), intracellular gonococci are associated with increased macrophage death, with infection inducing caspase 1 and 4, indicative of pro-inflammatory (pyroptotic) cell death (148). Both THP-1 monocytes and PMBCs activate the NLRP3 inflammasome, promoting rapid cell death via pyronecrosis (145). In cultured macrophage lines, GC strain FA1090 protects U937 macrophages from staurosporine-induced apoptosis (anti-apoptotic activity), though infection induces apoptosis in the THP-1 cell line (149). Interestingly, U937 and THP-1 cell lines expressed different cytokine profiles during GC infection. For example, THP-1 cells secreted more IL-1α and IL-6, while U937 secreted more IL-10, an indication that these cell lines may have different pre-existing set points on the continuum of macrophage polarization states (150). Polarization of macrophages may itself be a relevant occurrence during infections, as primary human MDMs have been shown to polarize toward an M2 (specifically M2b) phenotype during infection with piliated, Opa+ gonococci. This is despite the fact that cytokines expressed by these macrophages include classically M1 and M2 molecules (matching the cytokines mentioned above) (151). A similar promotion of a tolerogenic phenotype (up-regulation of IL-10 and transformaing growth factor- β, TGF-β) is observed in infections of mouse macrophages (152). Increased expression of the immunosuppressive molecule PD-L1 on human MDMs along with a reduced ability of GC-infected MDMs to stimulate CD4+ T cells (151), mirrors the observations from DCs (139, 140). GC is therefore capable of manipulating both of these antigen-presenting cell types to discourage a productive adaptive immune response.
The major point of agreement between the two studies that estimated fallopian tube leukocytes is the abundance of T cells in this tissue (135, 136). In particular, the later study specifies cytotoxic (CD8+) T cells as the overwhelming majority of the CD3+ population in the intraepithelial compartment (1/15 ratio of CD8+ cells to epithelial cells). By contrast, gamma delta (TCRγδ+) T cells were observed at 1/120 and T helper (CD4+) cells were observed at only 1/400 in the intraepithelial space. Natural killer T (NKT) cells with invariant Vα24-JαQ TCRα were absent. Little to nothing is known about the role of T cells in the human response to gonococci in the fallopian tube, rather, most of what is known about T cells during gonococcal infection has come from studies of mouse models of intravaginal infection. During mouse infection, GC induces cytokines that indicate the presence of a T helper 17 (Th17) cell response (including the cytokine IL-17A) and not the cytokines expected from a Th1 response (153). In the same work, isolated mouse PBMCs and the human THP-1 cell line were both shown to make Th17-associated cytokines in response to GC infection or OMV treatment. Interestingly, blocking IL-17A or knocking out the receptor in mice, decreased neutrophil recruitment, increased recovered CFUs, and prolonged infection. These results indicate a disruption in an otherwise productive response that could clear gonococcal infection. One mechanism by which gonococci induce a Th17 response is through TLR4 recognition of gonococcal LOS, as Th17 responses are diminished in TLR4-mutant mice (154). Opa proteins then act to influence the Th17/Th1 balance, suppressing Th1 and Th2 responses (155). Central to determining the nature of the host T cell response, is the induction of TGF-β, IL-10, and type 1 regulatory T cells during gonococcal infection, which together promote Th17 responses and suppresses Th1 and Th2 responses (155, 156). Regulatory T cells (Tregs), including both TGF-β1-positive CD4+ cells and CD4+ CD25+ FoxP3+ cells, had been previously observed following mouse intravaginal infections (8). Tregs increase in number in the mouse genital tract draining lymph nodes following intravaginal gonococcal infections and TGF-β1+ CD4+ cells are observed in the mouse uterus. Though mice represent an imperfect proxy for human ascending infection and PID, the mouse model has provided valuable insight into the possible mechanistic basis of key observations in humans. Namely, why people are unable to mount a protective memory T cell response to a noticeably inflammatory bacterial infection. Future work is needed to determine the location of specific T cell subsets and their activation state during infections modeled in human tissue or in situ during or following natural infections.
In their long evolution with humans, gonococci have evolved methods to evade our most potent and useful defenses. GC can survive on and in epithelial cells, while facilitating passage through epithelial layers. GC is able to suppress the activity of dendritic cells and macrophages, and induce regulatory T cells to prevent CD4+ T cell responses. All of this suppression occurs while triggering immune responses through TLR2, TLR4, NOD1, NOD2, TIFA, and cGAS/STING to produce an inflammatory state. When neutrophils and macrophages arrive to clear gonococci, bacteria manage to survive within these professional phagocytes and, in some cases, promote cell death. Within this cytokine/chemokine milieu, pro-inflammatory Th17 cells are induced and Th17-dependent responses act to suppress Th1 and Th2 cells that would help generate immunologic memory. To date, we have assembled a working understanding of the complement of resident immune cells and distribution of receptors in the human fallopian tube. In addition, human fallopian tube explant experiments have revealed important properties of the innate immune response to GC, while animal models have contributed information on the adaptive immune response. By combining what is known from each of these different experimental sources, we are beginning to assemble a more complete picture of how GC promotes and exploits the inflammatory environment that causes PID (Figure 2).
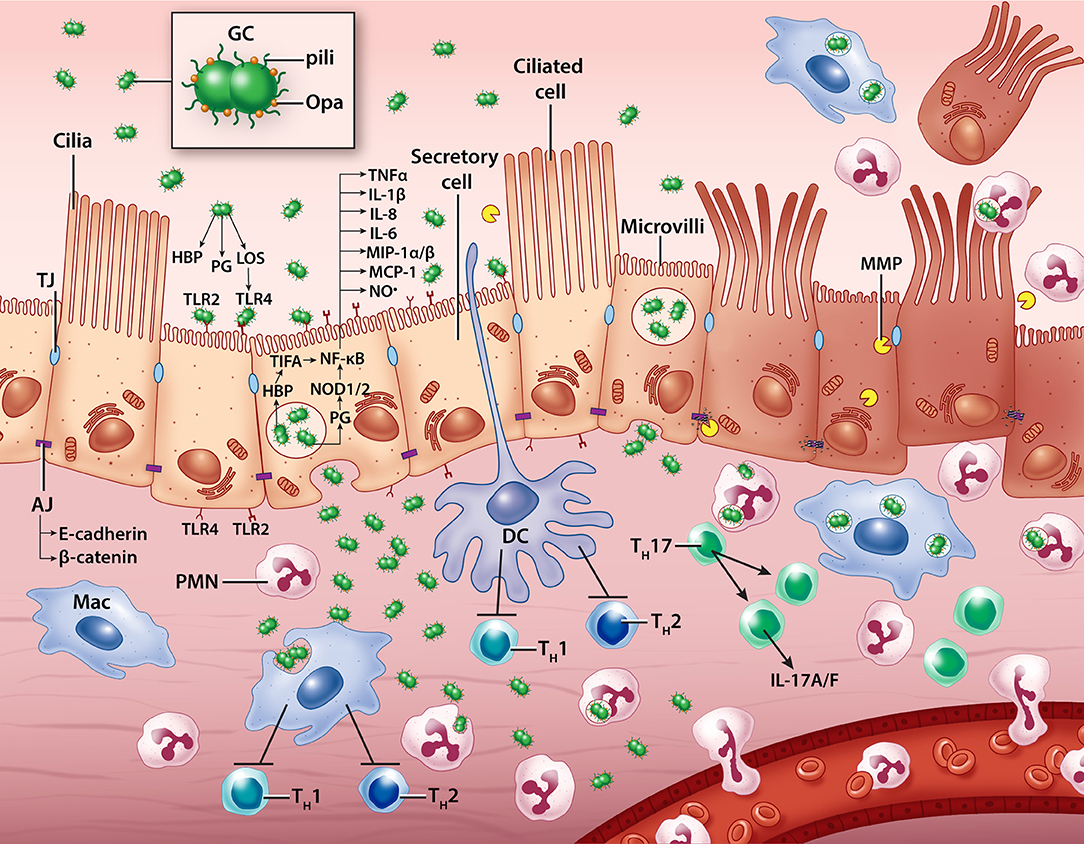
Figure 2. A model of N. gonorrhoeae pathogenesis in the human Fallopian tube. Extracellular gonococci interact with secretory (non-ciliated) epithelial cells, inducing cytokine, chemokine, nitric oxide, and matrix metalloproteinase production. Some bacteria transcytose through epithelial cells to invade the subepithelial space where they likely encounter resident macrophages, dendritic cells, and neutrophils which can help clear infection. Immune cells are also influenced by contact with GC and the building epithelial immune response to promote pro-inflammatory T cell activity and discourage productive memory T cell responses. Ciliated cells die and are sloughed from the epithelium, possibly with the help of GC-induced host processes that disassemble cell-cell junctions. GC, gonococcus; PMN, polymorphonuclear leukocyte (neutrophil); Mac, macrophage; DC, dendritic cell; TH, T helper cell; LOS, lipooligosaccharide; PG, peptidoglycan; HBP, heptose-1,7-bisphosphate; TLR, Toll-like receptor; MMP, matrix metalloproteinase; TJ, tight junction; AJ, adherens junction; NO•, nitric oxide.
The “Sterile” Fallopian Tube
The human female lower reproductive tract is an environment rich with commensal microbiota, containing around 108 bacteria per gram of vaginal fluid (157), and dominated by Lactobacillus species in the majority of women (158). Once past the cervix, however, the uterus, fallopian tubes, and ovaries were thought to be effectively sterile to protect the reproductive process. Three recent studies have utilized quantitative polymerase chain reaction (qPCR) and/or Next-Generation Sequencing (NGS) of 16S ribosomal RNA (rRNA) genes to detect bacteria in fallopian tubes (159–161). Two of these studies confirmed their findings with culture of fallopian tube-resident bacteria (159, 161). Bacteria above the cervix are indeed found at much lower abundance than below the cervix, by about four orders of magnitude. The Fallopian tube appears to contain a diverse community including members of the Gram-negative proteobacteria (Acinetobacter, Pseudomonas, Comamonas), Gram-negative anaerobes (Bacteroides), and Gram-positives (Clostridium, Enterococci, Staphylococci, Vagococcus, Proprionibacterium). Rather than being dominated by any one genus, both studies point to a more diverse polymicrobial community trending toward aerobes and facultative anaerobes that prefer slightly alkaline conditions. As with the vaginal microbiome, the microbiome analyses of the Fallopian tube do not overlap perfectly in their findings, variations that may be accounted for by geographic or ethnographic differences (one study featured 110 reproductive-age women from Shenzhen, China, another just 16 women from Brisbane, Australia).
In the lower FRT, Lactobacilli are known to prevent gonococcal establishment and colonization through multiple mechanisms. Lactobacilli keep the vaginal pH low, produce inhibitory H2O2 (162), compete for binding sites on epithelial cells (163), and produce surface molecules that control GC in a contact-dependent manner (164, 165). Once infection is established at the cervix and has ascended as far as the fallopian tube, it is unclear if resident microbes have any effect on the progression of inflammatory disease. The identification of a resident microbial community, however, suggests an additional variable to consider, on top of host genetics, when considering an individual's risk of negative outcomes from gonorrhea.
Thinking Outside the Tube
For human female upper reproductive tract infections, ex vivo or in vitro methods are the only ethical means for studying direct interactions between gonococci and their native host. Primary human-derived tissue is the nearest substitute for natural infection, but other alternatives either currently exist or could be applied to the fallopian tube given the state of the art for other tissues. Alternative technologies include human-derived primary cells, organoids (three-dimensional single or mixed cells), or organs-on-a-chip (OOC). Primary cells have been useful, to date, in the study of gonococcal infection in both males and females. Cells have been successfully derived from many of the principal tissues encountered during infection including cervical epithelium (166), endometrium (167), fallopian tube (60), and male urethral epithelium (168). Key findings regarding differences in receptor engagement and inflammatory responses between males and females (as mentioned above) have been determined using primary cells. The principle drawback to primary cells is the loss of multicellularity characteristic of intact tissues. In tissues, multiple stratified layers may be involved in immunological cross-talk or in engaging directly with bacteria during the progression of infection. In addition, adaptation to passage in cell culture and the loss of normal development/differentiation signals can impact the performance of primary cells in culture. For fallopian tubes in particular, the mixture of ciliated and non-ciliated cells, and the differences in their behavior during infection is key to the observed pathology.
Modern methods for isolating epithelial cells from Fallopian tube have not emerged from the field of infectious disease, but rather from studies on the origins of ovarian cancer, in particular high-grade serous ovarian carcinoma (HGSOC) (169). The desire to have models for studying oncogenic transformation of fallopian tube secretory cells has led to the development of isolation methods for primary fallopian tube secretory epithelial cells (FTSECs) and immortalized lines derived from these primary cells (170). Unfortunately, a side effect of fallopian tube epithelial cell isolation is a gradual loss of ciliated cells from culture upon passage (169). Indeed, ciliated cells appear to be a terminally differentiated cell type. In the murine airway epithelium, stem/progenitor cells known as basal cells give rise to secretory epithelial cells, which can then undergo terminal differentiation into ciliated cells (171). The maintenance of a secretory phenotype in this system is dependent on continuous signaling through the intercellular signaling receptor Notch2, without which secretory cells become ciliated cells. A similar linage relationship was also established for the epithelial cells of murine oviduct, where secretory cells are capable of self-renewal, as well as capable of differentiating into ciliated cells (172). In the oviduct, the balance of Wnt/β-catenin signaling is critical in both the maintenance of the secretory cell population and the differentiation into ciliated cells. While the human fallopian tube may not directly replicate the murine system, understanding the signals that are needed to maintain healthy mixed populations in culture allows us to improve how we establish and maintain in vitro models. Host developmental signaling pathways themselves may also be targets for pathogens, as evidenced by the ability of C. trachomatis to disrupt Wnt signaling in infected (and neighboring) epithelial cells in fallopian tube explants (100).
Defining an epithelial stem cell niche in the oviduct and determining the signals necessary to promote ciliated and secretory cell differentiation raises the possibility of using a patient's own stem cells to correct damage. Such repair could potentially restore fertility following tissue injury incurred from an STI or chemotherapy. Stem cells also provide additional possibilities for the generation of human-derived research materials. Induced pluripotent stem cell (iPSC) lines have been used to generate fallopian tube epithelium de novo (173). iPSCs first need to be induced to differentiate into intermediate mesoderm-like cells, and can subsequently be treated with Wnt pathway agonists (Wnt4 and Follistatin) to generate a fallopian tube epithelium that contains both ciliated and secretory cells. It is also possible to isolate multipotent mesenchymal stem cells (MSCs) from whole human fallopian tube tissue, as well as a subset of less proliferative MSCs from the mucosal portion of the tissue alone (174). These cells are capable of differentiation on adipogenic, osteogenic, and chondrogenic pathways and produce cytokines such as GM-CSF, IL-4, IL-6, TNFα and IFNγ. Kessler et al. demonstrated the isolation of adult stem cells from fallopian tube tissue, as well as defined the necessary culturing process to generate epithelia with a mixture of ciliated and secretory cells (175).
Successful culture of primary cells, stem cells, and/or iPSCs often requires suspension in (or grown on) an extracellular matrix (frequently Martigel, derived from Engelbreth-Holm-Swarm cells or minimally a collagen- or fibronectin-coated substrate). When placed in three-dimensional culture, rather than when adhered to a cell dish in monolayer, a single cell type (given proper inputs) can develop distinct polarity and cellular differentiation. These differentiated, three-dimensional cell clusters are known as spheroids or organoids. To generate faithful reproductions of fallopian tube epithelia, both iPSCs (173) and adult stem cells from dissected fallopian tube (175) need to be grown as organoids. Organoids hold tremendous promise for the study of complex cellular systems: they have the richness of signals provided by multiple cell types, spatial growth cues that monolayer cells lack, and they can be expanded in culture over long periods of time. Kessler et al. propagated continuously dividing, morphologically stable fallopian tube organoids for 10 months. In particular, for pathogens where animal models are lacking or do not faithfully recapitulate key aspects of infection, organoids can provide a middle ground between cell culture models and the natural infection seen in humans (176). Organoid systems have already gained traction for the study for intestinal pathogenic bacteria (177), where intestinal organoids (also known as enteroids) have been used to study bacterial adherence and invasion. For gonococci, where humans are the only natural host, the development of organoids that recapitulate the tissue architecture of fallopian tube present an exciting development and a promising future direction for host-pathogen interaction research. Since organoids can be derived from single (stem) cells, genome editing technologies including CRISPR/Cas9 could be used to alter host genes targeted during infection, prior to the growth of cells into mature organoids. These techniques have already been applied to edit the genomes of both adult stem cell- and pluripotent stem cell-derived organoids to study a variety of human diseases reviewed in (178, 179).
Another technology that could be used to replicate in vitro the biological complexity seen in vivo is organs-on-chips (OOCs), which come from a fusion of biological and engineering fields. OOCs are composed of either cell lines or primary cells from one or more tissues, cultured in custom-engineered microfluidic devices that can mimic multi-organ systems and incorporate complex biomechanical features like gas-exchange or liquid mixing (180). While OOC technology has room to improve in handling patient-derived or induced-pluripotent stem cells, microfluidic devices generally produce more reproducible results than organoids, which rely on meticulous culturing and development. Recently, a microfluidic model was constructed for the human female reproductive tract that was capable of mimicking the menstrual cycle (181). During ascending female reproductive tract infection that results in PID, gonococci must presumably interact with multiple cell types in sequence (endo/ectocervix, uterus, and fallopian tube) en route from their inoculation site. Having an interconnected, multicellular system would allow for the study of bacteria-host interactions throughout the process of gonococcal ascension to the fallopian tube. It is also unclear what bacterial or host factors predispose women to an asymptomatic cervical infection and whether this relates to their likelihood of progression to upper FRT infection. One possible contributing factor is hormonal cycles, which dramatically influence the tissue environment encountered by bacteria, and could be replicated in OOC systems. Hormonal changes are known to impact (1) primary human ectocervical epithelial cell (HECEC) responsiveness to peptidoglycan and LPS (182), (2) gonococcal survival during primary human cervical epithelial (pex) cell infection (51), and (3) the adherence and internalization rates for gonococci on fallopian tube epithelial cells (78). However, the full impact of hormonal signaling is difficult to assess in models that feature only one cell type or even one multicellular tissue. As technology evolves, more opportunities will exist to develop robust, flexible, in vitro models that retain the relevance of experimenting on primary human tissue.
Concluding Remarks
Neisseria gonorrhoeae remains a challenging organism for undertaking studies of host-pathogen interactions owing to its evolutionary adaptation to a life cycle in human hosts. However, the increasing utility of mouse models that incorporate human components, the availability of surgical samples from human tissues, and more adaptable human-like model systems, together provide great promise for understanding the biology of an important human pathogen. The use of fallopian tube explants provides a unique window into natural infection. Studying its multicellular epithelium has revealed how infection is linked to decreased fertility via bacteria- and host-factor toxicity to ciliated cells. Its three-dimensional structure has allowed observations about the speed of cellular invasion, the process of transcytosis and colonization of the sub-epithelial space. Thanks to insights from the fields of developmental biology, cancer biology, and gynecology, we know more than ever before about the process of ciliated cell maturation, how ciliated cells are maintained in tissues, and what immune responses are most likely to take place in the fallopian tube.
There are still several gaps that remain in our understanding of the molecular mechanisms that underlie gonococcal salpingitis. Chief among them are the cellular events surrounding ciliated cell death and extrusion from the epithelium. Prior to cell death, cellular changes result in a decrease in ciliary beat frequency (CBF). Loss of ciliated cell activity then precedes visible death and sloughing of ciliated cells. These phenomena have been difficult to measure, as the most widely accepted methods for quantitatively studying CBF involve high-speed video microscopy. Standard video microscopy is not ideal for whole tissue samples that lack optical transparency, or for monitoring changes in CBF across multiple time points in multiple samples. Many of the early works that describe decreases in CBF during gonococcal infection, and form the core of our knowledge on that topic, rely on essentially subjective visual estimates such as “percent peripheral ciliary activity (PPCA)” or “ciliary vigor” (15, 25). Future advances in live-cell, non-destructive, three-dimensional imaging of surfaces, such as light-sheet microscopy (183, 184), or methods that allow real-time in vivo or ex vivo measurements such as micro-scale tomography (185), will hopefully provide more robust measurement methods. With quantitative measurement methods, we can better probe what factors precipitate loss of CBF and what cellular mechanisms are involved in ciliated cell death and extrusion.
To understand how gonococcal infection results in permanently damaging salpingitis, we still need a clearer idea of how gonococci ascend into the fallopian tube. Current hypotheses include bacterial twitching motility, gonococcal survival inside phagocytes, or transport on sperm cells (186). We also need to understand the interplay of host factors that may predispose some women to more detrimental outcomes of ascending infection. Certainly, evidence has been presented that suggests differences in timing of infection during the menstrual cycle may influence the chance of negative outcomes. Additional investigation of host polymorphisms in immune receptors or adhesion ligands and their effects on gonococcal salpingitis represents an area for potential future investigation, especially with the increased accessibility of human genome sequencing. For example, some evidence exists that TLR2 polymorphisms and potentially other host differences can affect the outcome of C. trachomatis infection (187, 188). Understanding patient history, including more accurate diagnosis and documenting of instances of reproductive tract infection, is especially important now that we are aware of epigenetic mechanisms that influence future epithelial responses to repeated inflammatory stimuli (189, 190). At the center of any discussion of how to model infectious diseases should be a clear understanding of how the knowledge we gain into basic biological mechanisms translates into the features we see (or seek to prevent) in human disease. Fallopian tubes, and other human tissues, provide an excellent model system for answering certain questions about how gonococci interact with, and provoke immune responses from diverse human genetic backgrounds. Along with cellular and whole-animal systems, organ cultures represent a vital piece of the research puzzle that together allows us to assemble a more complete picture of N. gonorrhoeae pathogenesis.
Author Contributions
JL and JD conceived and outlined the manuscript. JL wrote the manuscript and designed figures. JD edited the manuscript and figures.
Funding
This work was supported by R01 AI097157 from the National Institutes of Health (USA).
Conflict of Interest Statement
The authors declare that the research was conducted in the absence of any commercial or financial relationships that could be construed as a potential conflict of interest.
Acknowledgments
The authors would like to thank Kathleen T. Hackett for providing images used in Figure 1 and Catherine Stanley, artist in the Media Solutions office at the UW School of Medicine and Public Health for production support in generating Figure 2.
References
1. Eschenbach DA, Buchanan TM, Pollock HM, Forsyth PS, Alexander ER, Lin JS, et al. Polymicrobial etiology of acute pelvic inflammatory disease. N Engl J Med. (1975) 293:166–71. doi: 10.1056/NEJM197507242930403
2. Westrom L, Joesoef R, Reynolds G, Hagdu A, Thompson SE. Pelvic inflammatory disease and fertility. A cohort study of 1,844 women with laparoscopically verified disease and 657 control women with normal laparoscopic results. Sex Transm Dis. (1992) 19:185–92. doi: 10.1097/00007435-199207000-00001
3. Haggerty CL, Peipert JF, Weitzen S, Hendrix SL, Holley RL, Nelson DB, et al. Predictors of chronic pelvic pain in an urban population of women with symptoms and signs of pelvic inflammatory disease. Sex Transm Dis. (2005) 32:293–9. doi: 10.1097/01.olq.0000162361.69041.a5
4. Taylor BD, Ness RB, Darville T, Haggerty CL. Microbial correlates of delayed care for pelvic inflammatory disease. Sex Transm Dis. (2011) 38:434–8. doi: 10.1097/OLQ.0b013e3181ffa7c7
5. Fifer H, Natarajan U, Jones L, Alexander S, Hughes G, Golparian D, et al. Failure of dual antimicrobial therapy in treatment of gonorrhea. N Engl J Med. (2016) 374:2504–6. doi: 10.1056/NEJMc1512757
6. Jerse AE. Experimental gonococcal genital tract infection and opacity protein expression in estradiol-treated mice. Infect Immun. (1999) 67:5699–708.
7. Jerse AE, Wu H, Packiam M, Vonck RA, Begum AA, Garvin LE. Estradiol-treated female mice as surrogate hosts for Neisseria gonorrhoeae genital tract infections. Front Microbiol. (2011) 2:107. doi: 10.3389/fmicb.2011.00107
8. Imarai M, Candia E, Rodriguez-Tirado C, Tognarelli J, Pardo M, Perez T, et al. Regulatory T cells are locally induced during intravaginal infection of mice with Neisseria gonorrhoeae. Infect Immun. (2008) 76:5456–65. doi: 10.1128/IAI.00552-08
9. Islam EA, Shaik-Dasthagirisaheb Y, Kaushic C, Wetzler LM, Gray-Owen SD. The reproductive cycle is a pathogenic determinant during gonococcal pelvic inflammatory disease in mice. Mucosal Immunol. (2016) 9:1051–64. doi: 10.1038/mi.2015.122
10. Lucas CT, Chandler F Jr Martin JE Jr Schmale JD. Transfer of gonococcal urethritis from man to chimpanzee. An Anim Model Gonorrhea JAMA (1971) 216:1612–4. doi: 10.1001/jama.1971.03180360058007
11. Brown WJ, Lucas CT, Kuhn US. Gonorrhoea in the chimpanzee. Infection with laboratory-passed gonococci and by natural transmission. Br J Vener Dis. (1972) 48:177–8. doi: 10.1136/sti.48.3.177
12. Cohen MS, Cannon JG, Jerse AE, Charniga LM, Isbey SF, Whicker LG. Human experimentation with Neisseria gonorrhoeae: rationale, methods, and implications for the biology of infection and vaccine development. J Infect Dis. (1994) 169:532–7. doi: 10.1093/infdis/169.3.532
13. Cohen MS, Cannon JG. Human experimentation with Neisseria gonorrhoeae: progress and goals. J Infect Dis. (1999) 179(Suppl. 2):S375–9. doi: 10.1086/513847
14. McGee ZA, Woods ML Jr. Use of organ cultures in microbiological research. Annu Rev Microbiol. (1987) 41:291–300. doi: 10.1146/annurev.mi.41.100187.001451
15. McGee ZA, Johnson AP, Taylor-Robinson D. Human fallopian tubes in organ culture: preparation, maintenance, and quantitation of damage by pathogenic microorganisms. Infect Immun. (1976) 13:608–18.
16. Carney FE Jr Taylor-Robinson D. Growth and effect of Neisseria gonorrhoeae in organ cultures. Br J Vener Dis. (1973) 49:435–40. doi: 10.1136/sti.49.5.435
18. Taylor-Robinson D, Whytock S, Green CJ, Carney FE Jr. Effect of Neisseria gonorrhoeae on human and rabbit oviducts. Br J Vener Dis. (1974) 50:279–88. doi: 10.1136/sti.50.4.279
19. Johnson AP, Taylor-Robinson D, McGee ZA. Species specificity of attachment and damage to oviduct mucosa by Neisseria gonorrhoeae. Infect Immun. (1977) 18:833–9.
20. Johnson AP, Clark JB, Osborn MF, Taylor-Robinson D. A comparison of the association of Neisseria gonorrhoeae with human and guinea-pig genital mucosa maintained in organ culture. Br J Exp Pathol. (1980) 61:521–7.
21. Ward ME, Watt PJ, Robertson JN. The human fallopian tube: a laboratory model for gonococcal infection. J Infect Dis. (1974) 129:650–9. doi: 10.1093/infdis/129.6.650
22. Draper DL, Donegan EA, James JF, Sweet RL, Brooks GF. In vitro modeling of acute salpingitis caused by Neisseria gonorrhoeae. Am J Obstet Gynecol. (1980) 138:996–1002. doi: 10.1016/0002-9378(80)91095-9
23. Lyons RA, Saridogan E, Djahanbakhch O. The reproductive significance of human Fallopian tube cilia. Hum Reprod Update (2006) 12:363–72. doi: 10.1093/humupd/dml012
24. Mardh PA, Baldetorp B, Hakansson CH, Fritz H, Westrom L. Studies of ciliated epithelia of the human genital tract. 3: mucociliary wave activity in organ cultures of human Fallopian tubes challenged with Neisseria gonorrhoeae and gonococcal endotoxin. Br J Vener Dis. (1979) 55:256–64. doi: 10.1136/sti.55.4.256
25. McGee ZA, Johnson AP, Taylor-Robinson D. Pathogenic mechanisms of Neisseria gonorrhoeae: observations on damage to human fallopian tubes in organ culture by gonococci of colony type 1 or type 4. J Infect Dis. (1981) 143:413–22. doi: 10.1093/infdis/143.3.413
26. Melly MA, Gregg CR, McGee ZA. Studies of toxicity of Neisseria gonorrhoeae for human fallopian tube mucosa. J Infect Dis. (1981) 143:423–31. doi: 10.1093/infdis/143.3.423
27. Gregg CR, Melly M, Hellerqvist CG, Coniglio JG, McGee ZA. Toxic activity of purified lipopolysaccharide of Neisseria gonorrhoeae for human fallopian tube mucosa. J Infect Dis. (1981) 143:432–9. doi: 10.1093/infdis/143.3.432
28. Gregg CR, Johnson AP, Taylor-Robinson D, Melly MA, McGee ZA. Host species-specific damage to oviduct mucosa by Neisseria gonorrhoeae lipopolysaccharide. Infect Immun. (1981) 34:1056–8.
29. Herath S, Fischer DP, Werling D, Williams EJ, Lilly ST, Dobson H, et al. Expression and function of Toll-like receptor 4 in the endometrial cells of the uterus. Endocrinology (2006) 147:562–70. doi: 10.1210/en.2005-1113
30. Vaure C, Liu Y. A comparative review of toll-like receptor 4 expression and functionality in different animal species. Front Immunol. (2014) 5:316. doi: 10.3389/fimmu.2014.00316
31. Rosenthal RS. Release of soluble peptidoglycan from growing gonococci: hexaminidase and amidase activities. Infect Immun. (1979) 24:869–78.
32. Sinha RK, Rosenthal RS. Release of soluble peptidoglycan from growing gonococci: demonstration of anhydro-muramyl-containing fragments. Infect Immun. (1980) 29:914–25.
33. Melly MA, McGee ZA, Rosenthal RS. Ability of monomeric peptidoglycan fragments from Neisseria gonorrhoeae to damage human fallopian-tube mucosa. J Infect Dis. (1984) 149:378–86. doi: 10.1093/infdis/149.3.378
34. Goldman WE, Cookson BT. Structure and functions of the Bordetella tracheal cytotoxin. Tokai J. Exp. Clin. Med. (1988) 13(Suppl.):187–91.
35. Cookson BT, Cho HL, Herwaldt LA, Goldman WE. Biological activities and chemical composition of purified tracheal cytotoxin of Bordetella pertussis. Infect Immun. (1989) 57:2223–9.
36. Girardin SE, Boneca IG, Carneiro LA, Antignac A, Jehanno M, Viala J, et al. Nod1 detects a unique muropeptide from Gram-negative bacterial peptidoglycan. Science (2003) 300:1584–7. doi: 10.1126/science.1084677
37. Magalhaes JG, Philpott DJ, Nahori MA, Jehanno M, Fritz J, Le Bourhis L, et al. Murine Nod1 but not its human orthologue mediates innate immune detection of tracheal cytotoxin. EMBO Rep. (2005) 6:1201–7. doi: 10.1038/sj.embor.7400552
38. Flak TA, Heiss LN, Engle JT, Goldman WE. Synergistic epithelial responses to endotoxin and a naturally occurring muramyl peptide. Infect Immun. (2000) 68:1235–42. doi: 10.1128/IAI.68.3.1235-1242.2000
39. McGee ZA, Clemens CM, Jensen RL, Klein JJ, Barley LR, Gorby GL. Local induction of tumor necrosis factor as a molecular mechanism of mucosal damage by gonococci. Microb Pathog. (1992) 12:333–41. doi: 10.1016/0882-4010(92)90096-7
40. McGee ZA, Jensen RL, Clemens CM, Taylor-Robinson D, Johnson AP, Gregg CR. Gonococcal infection of human fallopian tube mucosa in organ culture: relationship of mucosal tissue TNF-alpha concentration to sloughing of ciliated cells. J Sex Transm Dis. (1999) 26:160–5. doi: 10.1097/00007435-199903000-00007
41. Heiss LN, Moser SA, Unanue ER, Goldman WE. Interleukin-1 is linked to the respiratory epithelial cytopathology of pertussis. Infect Immun. (1993) 61:3123–8.
42. Hvid M, Baczynska A, Deleuran B, Fedder J, Knudsen HJ, Christiansen G, et al. Interleukin-1 is the initiator of Fallopian tube destruction during Chlamydia trachomatis infection. Cell Microbiol. (2007) 9:2795–803. doi: 10.1111/j.1462-5822.2007.00996.x
43. Naumann M, Wessler S, Bartsch C, Wieland B, Meyer TF. Neisseria gonorrhoeae epithelial cell interaction leads to the activation of the transcription factors nuclear factor kappaB and activator protein 1 and the induction of inflammatory cytokines. J Exp Med. (1997) 186:247–58. doi: 10.1084/jem.186.2.247
44. Maisey K, Nardocci G, Imarai M, Cardenas H, Rios M, Croxatto HB, et al. Expression of proinflammatory cytokines and receptors by human fallopian tubes in organ culture following challenge with Neisseria gonorrhoeae. Infect Immun. (2003) 71:527–32. doi: 10.1128/IAI.71.1.527-532.2003
45. Velasquez L, García K, Morales F, Heckels JE, Orihuela P, Rodas PI, et al. Neisseria gonorrhoeae pilus attenuates cytokine response of human fallopian tube explants. J Biomed Biotechnol. (2012) 2012:491298. doi: 10.1155/2012/491298
46. Swanson J, Kraus SJ, Gotschlich EC. Studies on gonococcus infection. I Pili and zones of adhesion: their relation to gonococcal growth patterns J Exp Med. (1971) 134:886–906. doi: 10.1084/jem.134.4.886
47. James JF, Swanson J. Studies on gonococcus infection. XIII Occurrence of color/opacity colonial variants in clinical cultures Infect Immun. (1978) 19:332–40.
48. Heiss LN, Lancaster JR Jr Corbett JA, Goldman WE. Epithelial autotoxicity of nitric oxide: role in the respiratory cytopathology of pertussis. Proc Natl Acad Sci USA. (1994) 91:267–70. doi: 10.1073/pnas.91.1.267
49. Flak TA, Goldman WE. Signalling and cellular specificity of airway nitric oxide production in pertussis. Cell Microbiol. (1999) 1:51–60. doi: 10.1046/j.1462-5822.1999.00004.x
50. García KP, Rubilar PS, Vargas MF, Cardenas H, Rios MA, Orihuela PA, et al. Nitric oxide is not involved in Neisseria gonorrhoeae-induced cellular damage of human fallopian tubes in vitro. Biol Res. (2010) 43:39–50. doi: 10.4067/S0716-97602010000100006
51. Edwards JL. Neisseria gonorrhoeae survival during primary human cervical epithelial cell infection requires nitric oxide and Is augmented by progesterone. Infect Immun. (2010) 78:1202–13. doi: 10.1128/IAI.01085-09
52. Parks WC, Wilson CL, Lopez-Boado YS. Matrix metalloproteinases as modulators of inflammation and innate immunity. Nat Rev Immunol. (2004) 4:617–29. doi: 10.1038/nri1418
53. Lopez-Boado YS, Wilson CL, Hooper LV, Gordon JI, Hultgren SJ, Parks WC. Bacterial exposure induces and activates matrilysin in mucosal epithelial cells. J Cell Biol. (2000) 148:1305–15. doi: 10.1083/jcb.148.6.1305
54. Zeisel MB, Druet VA, Wachsmann D, Sibilia J. MMP-3 expression and release by rheumatoid arthritis fibroblast-like synoviocytes induced with a bacterial ligand of integrin alpha5beta1. Arthritis Res Ther. (2005) 7:R118–126. doi: 10.1186/ar1462
55. Kanangat S, Postlethwaite A, Hasty K, Kang A, Smeltzer M, Appling W, et al. Induction of multiple matrix metalloproteinases in human dermal and synovial fibroblasts by Staphylococcus aureus: implications in the pathogenesis of septic arthritis and other soft tissue infections. Arthritis Res Ther. (2006) 8:R176. doi: 10.1186/ar2086
56. Vissers M, Hartman Y, Groh L, De Jong DJ, De Jonge MI, Ferwerda G. Recognition of Streptococcus pneumoniae and muramyl dipeptide by NOD2 results in potent induction of MMP-9, which can be controlled by lipopolysaccharide stimulation. Infect Immun. (2014) 82:4952–8. doi: 10.1128/IAI.02150-14
57. Shogan BD, Belogortseva N, Luong PM, Zaborin A, Lax S, Bethel C, et al. Collagen degradation and MMP9 activation by Enterococcus faecalis contribute to intestinal anastomotic leak. Sci Transl Med. (2015) 7:286ra268. doi: 10.1126/scitranslmed.3010658
58. Rodas PI, Perez D, Jauffret C, Gonzalez Y, Carreno C, Tapia CV, et al. Modified profile of Matrix Metalloproteinase 2 and 9 production by human fallopian tube epithelial cells after infection in vitro with Neisseria gonorrhoeae. J Infect Dis. (2017) 215:452–5. doi: 10.1093/infdis/jiw568
59. Juica NE, Rodas PI, Solar P, Borda P, Vargas R, Munoz C, et al. Neisseria gonorrhoeae challenge increases Matrix Metalloproteinase-8 expression in fallopian tube explants. Front Cell Infect Microbiol. (2017) 7:399. doi: 10.3389/fcimb.2017.00399
60. Rodríguez-Tirado C, Maisey K, Rodríguez FE, Reyes-Cerpa S, Reyes-López FE, Imarai M. Neisseria gonorrhoeae induced disruption of cell junction complexes in epithelial cells of the human genital tract. Microbes Infect. (2012) 14:290–300. doi: 10.1016/j.micinf.2011.11.002
61. Edwards VL, Wang LC, Dawson V, Stein DC, Song W. Neisseria gonorrhoeae breaches the apical junction of polarized epithelial cells for transmigration by activating EGFR. Cell Microbiol. (2013) 15:1042–57. doi: 10.1111/cmi.12099
62. Wang LC, Yu Q, Edwards V, Lin B, Qiu J, Turner JR, et al. Neisseria gonorrhoeae infects the human endocervix by activating non-muscle myosin II-mediated epithelial exfoliation. PLoS Pathog. (2017) 13:e1006269. doi: 10.1371/journal.ppat.1006269
63. Morales P, Reyes P, Vargas M, Rios M, Imarai M, Cardenas H, et al. Infection of human fallopian tube epithelial cells with Neisseria gonorrhoeae protects cells from tumor necrosis factor alpha-induced apoptosis. Infect Immun. (2006) 74:3643–50. doi: 10.1128/IAI.00012-06
64. Binnicker MJ, Williams RD, Apicella MA. Infection of human urethral epithelium with Neisseria gonorrhoeae elicits an upregulation of host anti-apoptotic factors and protects cells from staurosporine-induced apoptosis. Cell Microbiol. (2003) 5:549–60. doi: 10.1046/j.1462-5822.2003.00300.x
65. Binnicker MJ, Williams RD, Apicella MA. Gonococcal porin IB activates NF-kappaB in human urethral epithelium and increases the expression of host antiapoptotic factors. Infect Immun. (2004) 72:6408–17. doi: 10.1128/IAI.72.11.6408-6417.2004
66. Nudel K, Massari P, Genco CA. Neisseria gonorrhoeae Modulates Cell Death in Human Endocervical Epithelial Cells through Export of Exosome-Associated cIAP2. Infect Immun. (2015) 83:3410–7. doi: 10.1128/IAI.00732-15
67. Simons MP, Nauseef WM, Griffith TS, Apicella MA. Neisseria gonorrhoeae delays the onset of apoptosis in polymorphonuclear leukocytes. Cell Microbiol. (2006) 8:1780–90. doi: 10.1111/j.1462-5822.2006.00748.x
68. Chen A, Seifert HS. Neisseria gonorrhoeae-mediated inhibition of apoptotic signalling in polymorphonuclear leukocytes. Infect Immun. (2011) 79:4447–58. doi: 10.1128/IAI.01267-10
69. Kepp O, Gottschalk K, Churin Y, Rajalingam K, Brinkmann V, Machuy N, et al. Bim and Bmf synergize to induce apoptosis in Neisseria gonorrhoeae infection. PLoS Pathog. (2009) 5:e1000348. doi: 10.1371/journal.ppat.1000348
70. Deo P, Chow SH, Hay ID, Kleifeld O, Costin A, Elgass KD, et al. Outer membrane vesicles from Neisseria gonorrhoeae target PorB to mitochondria and induce apoptosis. PLoS Pathog (2018) 14:e1006945. doi: 10.1371/journal.ppat.1006945
71. McGee ZA, Stephens DS, Hoffman LH, Schlech WF III, Horn RG. Mechanisms of mucosal invasion by pathogenic Neisseria. Rev Infect Dis. (1983) 5(Suppl. 4):S708–14. doi: 10.1093/clinids/5.Supplement_4.S708
72. Merz AJ, Rifenbery DB, Arvidson CG, So M. Traversal of a polarized epithelium by pathogenic Neisseriae: facilitation by type IV pili and maintenance of epithelial barrier function. Mol Med. (1996) 2:745–54. doi: 10.1007/BF03401658
73. Draper DL, Donegan EA, James JF, Sweet RL, Brooks GF. Scanning electron microscopy of attachment of Neisseria gonorrhoeae colony phenotypes to surfaces of human genital epithelia. Am J Obstet Gynecol. (1980) 138:818–26. doi: 10.1016/S0002-9378(16)32743-0
74. Griffiss JM, Lammel CJ, Wang J, Dekker NP, Brooks GF. Neisseria gonorrhoeae coordinately uses Pili and Opa to activate HEC-1-B cell microvilli, which causes engulfment of the gonococci. Infect Immun. (1999) 67:3469–80.
75. Kallstrom H, Blackmer Gill D, Albiger B, Liszewski MK, Atkinson JP, Jonsson AB. Attachment of Neisseria gonorrhoeae to the cellular pilus receptor CD46: identification of domains important for bacterial adherence. Cell Microbiol. (2001) 3:133–43. doi: 10.1046/j.1462-5822.2001.00095.x
76. Edwards JL, Brown EJ, Uk-Nham S, Cannon JG, Blake MS, Apicella MA. A co-operative interaction between Neisseria gonorrhoeae and complement receptor 3 mediates infection of primary cervical epithelial cells. Cell Microbiol. (2002) 4:571–84. doi: 10.1046/j.1462-5822.2002.t01-1-00215.x
77. Edwards JL, Brown EJ, Ault KA, Apicella MA. The role of complement receptor 3 (CR3) in Neisseria gonorrhoeae infection of human cervical epithelia. Cell Microbiol. (2001) 3:611–22. doi: 10.1046/j.1462-5822.2001.00140.x
78. Fernandez R, Nelson P, Delgado J, Aguilera J, Massai R, Velasquez L, et al. Increased adhesiveness and internalization of Neisseria gonorrhoeae and changes in the expression of epithelial gonococcal receptors in the fallopian tube of copper T and Norplant users. Hum Reprod. (2001) 16:463–8. doi: 10.1093/humrep/16.3.463
79. Tobiason DM, Seifert HS. Inverse relationship between pilus-mediated gonococcal adherence and surface expression of the pilus receptor, CD46. Microbiology (2001) 147:2333–40. doi: 10.1099/00221287-147-8-2333
80. Gill DB, Koomey M, Cannon JG, Atkinson JP. Down-regulation of CD46 by piliated Neisseria gonorrhoeae. J Exp Med. (2003) 198:1313–22. doi: 10.1084/jem.20031159
81. Edwards JL, Apicella MA. I-domain-containing integrins serve as pilus receptors for Neisseria gonorrhoeae adherence to human epithelial cells. Cell Microbiol. (2005) 7:1197–211. doi: 10.1111/j.1462-5822.2005.00547.x
82. Kirchner M, Heuer D, Meyer TF. CD46-independent binding of neisserial type IV pili and the major pilus adhesin, PilC, to human epithelial cells. Infect Immun. (2005) 73:3072–82. doi: 10.1128/IAI.73.5.3072-3082.2005
83. Timmerman MM, Shao JQ, Apicella MA. Ultrastructural analysis of the pathogenesis of Neisseria gonorrhoeae endometrial infection. Cell Microbiol. (2005) 7:627–36. doi: 10.1111/j.1462-5822.2005.00491.x
84. Gorby G, Simon D, Rest RF. Escherichia coli that express Neisseria gonorrhoeae opacity-associated proteins attach to and invade human fallopian tube epithelium. Ann N Y Acad Sci. (1994) 730:286–9. doi: 10.1111/j.1749-6632.1994.tb44267.x
85. Dekker NP, Lammel CJ, Mandrell RE, Brooks GF. Opa (protein II) influences gonococcal organization in colonies, surface appearance, size and attachment to human fallopian tube tissues. Microb Pathog. (1990) 9:19–31. doi: 10.1016/0882-4010(90)90037-Q
86. Ison C, Crabtree H, Lammel C, Brooks G. Expression of protein II by clinical isolates of Neisseria gonorrhoeae. In: Achtman KP, Marchal M Morelli C, Seiler G, Thiesen A, editors. Neisseriae. New York, NY: Walter de Gruyter (1990). p. 597–602.
87. Draper DL, James JF, Brooks GF, Sweet RL. Comparison of virulence markers of peritoneal and fallopian tube isolates with endocervical Neisseria gonorrhoeae isolates from women with acute salpingitis. Infect Immun. (1980) 27:882–8.
88. Naumann M, Rudel T, Meyer TF. Host cell interactions and signalling with Neisseria gonorrhoeae. Curr Opin Microbiol. (1999) 2:62–70. doi: 10.1016/S1369-5274(99)80011-3
89. Sintsova A, Wong H, Macdonald KS, Kaul R, Virji M, Gray-Owen SD. Selection for a CEACAM receptor-specific binding phenotype during Neisseria gonorrhoeae infection of the human genital tract. Infect Immun. (2015) 83:1372–83. doi: 10.1128/IAI.03123-14
90. Muenzner P, Bachmann V, Zimmermann W, Hentschel J, Hauck CR. Human-restricted bacterial pathogens block shedding of epithelial cells by stimulating integrin activation. Science (2010) 329:1197–201. doi: 10.1126/science.1190892
91. Swanson KV, Jarvis GA, Brooks G, Barham BJ, Cooper MD, Griffiss JML. CEACAM is not necessary for Neisseria gonorrhoeae to adhere to and invade female genital epithelial cells. Cell Microbiol. (2001) 3:681–91. doi: 10.1046/j.1462-5822.2001.00147.x
92. Cole JG, Fulcher NB, Jerse AE. Opacity proteins increase Neisseria gonorrhoeae fitness in the female genital tract due to a factor under ovarian control. Infect Immun. (2010) 78:1629–41. doi: 10.1128/IAI.00996-09
93. John CM, Jarvis GA, Swanson KV, Leffler H, Cooper MD, Huflejt ME, et al. Galectin-3 binds lactosaminylated lipooligosaccharides from Neisseria gonorrhoeae and is selectively expressed by mucosal epithelial cells that are infected. Cell Microbiol. (2002) 4:649–62. doi: 10.1046/j.1462-5822.2002.00219.x
94. Quan DN, Cooper MD, Potter JL, Roberts MH, Cheng H, Jarvis GA. TREM-2 binds to lipooligosaccharides of Neisseria gonorrhoeae and is expressed on reproductive tract epithelial cells. Mucosal Immunol. (2008) 1:229–38. doi: 10.1038/mi.2008.1
95. Harvey HA, Jennings MP, Campbell CA, Williams R, Apicella MA. Receptor-mediated endocytosis of Neisseria gonorrhoeae into primary human urethral epithelial cells: the role of the asialoglycoprotein receptor. Mol Microbiol. (2001) 42:659–72. doi: 10.1046/j.1365-2958.2001.02666.x
96. Ketterer MR, Rice PA, Gulati S, Kiel S, Byerly L, Fortenberry JD, et al. Desialylation of Neisseria gonorrhoeae lipooligosaccharide by cervicovaginal microbiome sialidases: the potential for enhancing infectivity in men. J Infect Dis. (2016) 214:1621–8. doi: 10.1093/infdis/jiw329
97. Van Putten JP. Phase variation of lipopolysaccharide directs interconversion of invasive and immuno-resistant phenotypes of Neisseria gonorrhoeae. EMBO J. (1993) 12:4043–51. doi: 10.1002/j.1460-2075.1993.tb06088.x
98. Bylander A, Lind K, Goksor M, Billig H, Larsson DG. The classical progesterone receptor mediates the rapid reduction of fallopian tube ciliary beat frequency by progesterone. Reprod Biol Endocrinol. (2013) 11:33. doi: 10.1186/1477-7827-11-33
99. Cooper MD, Rapp J, Jeffery-Wiseman C, Barnes RC, Stephens DS. Chlamydia trachomatis infection of human fallopian tube organ cultures. J Gen Microbiol. (1990) 136:1109–15. doi: 10.1099/00221287-136-6-1109
100. Kessler M, Zielecki J, Thieck O, Mollenkopf HJ, Fotopoulou C, Meyer TF. Chlamydia trachomatis disturbs epithelial tissue homeostasis in Fallopian tubes via paracrine Wnt signaling. Am J Pathol. (2012) 180:186–98. doi: 10.1016/j.ajpath.2011.09.015
101. Liu W, Yan M, Liu Y, Wang R, Li C, Deng C, et al. Olfactomedin 4 down-regulates innate immunity against Helicobacter pylori infection. Proc Natl Acad Sci USA. (2010b) 107:11056–61. doi: 10.1073/pnas.1001269107
102. Rasmussen SJ, Eckmann L, Quayle AJ, Shen L, Zhang YX, Anderson DJ, et al. Secretion of proinflammatory cytokines by epithelial cells in response to Chlamydia infection suggests a central role for epithelial cells in chlamydial pathogenesis. J Clin Invest. (1997) 99:77–87. doi: 10.1172/JCI119136
103. Humphrys MS, Creasy T, Sun Y, Shetty AC, Chibucos MC, Drabek EF, et al. Simultaneous transcriptional profiling of bacteria and their host cells. PLoS ONE (2013) 8:e80597. doi: 10.1371/journal.pone.0080597
104. Pioli PA, Amiel E, Schaefer TM, Connolly JE, Wira CR, Guyre PM. Differential expression of Toll-like receptors 2 and 4 in tissues of the human female reproductive tract. Infect Immun. (2004) 72:5799–806. doi: 10.1128/IAI.72.10.5799-5806.2004
105. Fazeli A, Bruce C, Anumba DO. Characterization of Toll-like receptors in the female reproductive tract in humans. Hum Reprod. (2005) 20:1372–8. doi: 10.1093/humrep/deh775
106. Hart KM, Murphy AJ, Barrett KT, Wira CR, Guyre PM, Pioli PA. Functional expression of pattern recognition receptors in tissues of the human female reproductive tract. J Reprod Immunol. (2009) 80:33–40. doi: 10.1016/j.jri.2008.12.004
107. Ghosh M, Shen Z, Fahey JV, Crist SG, Patel M, Smith JM, et al. Pathogen recognition in the human female reproductive tract: expression of intracellular cytosolic Sensors NOD1, NOD2, RIG-1, and MDA5 and response to HIV-1 and Neisseria gonorrhea. Am J Reprod Immunol. (2012) 69:41–51. doi: 10.1111/aji.12019
108. Aflatoonian R, Tuckerman E, Elliott SL, Bruce C, Aflatoonian A, Li TC, et al. Menstrual cycle-dependent changes of Toll-like receptors in endometrium. Hum Reprod. (2007) 22:586–93. doi: 10.1093/humrep/del388
109. Aflatoonian R, Fazeli A. Toll-like receptors in female reproductive tract and their menstrual cycle dependent expression. J Reprod Immunol. (2008) 77:7–13. doi: 10.1016/j.jri.2007.03.014
110. Oosting M, Cheng SC, Bolscher JM, Vestering-Stenger R, Plantinga TS, Verschueren IC, et al. Human TLR10 is an anti-inflammatory pattern-recognition receptor. Proc Natl Acad Sci USA. (2014) 111:E4478–4484. doi: 10.1073/pnas.1410293111
111. Itoh H, Nasu K, Nishida M, Matsumoto H, Yuge A, Narahara H. Human oviductal stromal fibroblasts, but not oviductal epithelial cells, express Toll-like receptor 4: the site-specific mucosal immunity of the human fallopian tube against bacterial infection. Am J Reprod Immunol. (2006) 56:91–101. doi: 10.1111/j.1600-0897.2006.00389.x
112. Amjadi F, Zandieh Z, Salehi E, Jafari R, Ghasemi N, Aflatoonian A, et al. Variable localization of Toll-like receptors in human fallopian tube epithelial cells. Clin Exp Reprod Med. (2018) 45:1–9. doi: 10.5653/cerm.2018.45.1.1
113. Moreira LO, Zamboni DS. NOD1 and NOD2 signaling in infection and inflammation. Front Immunol. (2012) 3:328. doi: 10.3389/fimmu.2012.00328
114. Lee J, Tattoli I, Wojtal KA, Vavricka SR, Philpott DJ, Girardin SE. pH-dependent internalization of muramyl peptides from early endosomes enables Nod1 and Nod2 signaling. J Biol Chem. (2009) 284:23818–29. doi: 10.1074/jbc.M109.033670
115. Woodhams KL, Chan JM, Lenz JD, Hackett KT, Dillard JP. Peptidoglycan fragment release from Neisseria meningitidis. Infect Immun. (2013) 81:3490–8. doi: 10.1128/IAI.00279-13
116. Mavrogiorgos N, Mekasha S, Yang Y, Kelliher MA, Ingalls RR. Activation of NOD receptors by Neisseria gonorrhoeae modulates the innate immune response. Innate Immun. (2014) 20:377–89. doi: 10.1177/1753425913493453
117. Lenz JD, Hackett KT, Dillard JP. A single dual-function enzyme controls the production of inflammatory NOD agonist peptidoglycan fragments by Neisseria gonorrhoeae. mBio (2017) 8:e01464–01417. doi: 10.1128/mBio.01464-17
118. Knilans KJ, Hackett KT, Anderson JE, Weng C, Dillard JP, Duncan JA. Neisseria gonorrhoeae lytic transglycosylases LtgA and LtgD reduce host innate immune signaling through TLR2 and NOD2. ACS Infect Dis. (2017) 3:624–33. doi: 10.1021/acsinfecdis.6b00088
119. Liu M, John CM, Jarvis GA. Phosphoryl moieties of lipid A from Neisseria meningitidis and N. gonorrhoeae lipooligosaccharides play an important role in activation of both MyD88- and TRIF-dependent TLR4-MD-2 signaling pathways. J Immunol. (2010) 185:6974–84. doi: 10.4049/jimmunol.1000953
120. Zhou X, Gao X, Broglie PM, Kebaier C, Anderson JE, Thom N, et al. Hexa-acylated lipid A is required for host inflammatory response to Neisseria gonorrhoeae in experimental gonorrhea. Infect Immun. (2014) 82:184–92. doi: 10.1128/IAI.00890-13
121. Pridmore AC, Jarvis GA, John CM, Jack DL, Dower SK, Read RC. Activation of toll-like receptor 2 (TLR2) and TLR4/MD2 by Neisseria is independent of capsule and lipooligosaccharide (LOS) sialylation but varies widely among LOS from different strains. Infect Immun. (2003) 71:3901–8. doi: 10.1128/IAI.71.7.3901-3908.2003
122. Devoe IW, Gilchrist JE. Release of endotoxin in the form of cell wall blebs during in vitro growth of Neisseria meningitidis. J Exp Med. (1973) 138:1156–67. doi: 10.1084/jem.138.5.1156
123. Pettit RK, Judd RC. The interaction of naturally elaborated blebs from serum-susceptible and serum-resistant strains of Neisseria gonorrhoeae with normal human serum. Mol Microbiol. (1992) 6:729–34. doi: 10.1111/j.1365-2958.1992.tb01522.x
124. Zielke RA, Wierzbicki IH, Weber JV, Gafken PR, Sikora AE. Quantitative proteomics of the Neisseria gonorrhoeae cell envelope and membrane vesicles for the discovery of potential therapeutic targets. Mol Cell Proteomics (2014) 13:1299–317. doi: 10.1074/mcp.M113.029538
125. Fichorova RN, Cronin AO, Lien E, Anderson DJ, Ingalls RR. Response to Neisseria gonorrhoeae by cervicovaginal epithelial cells occurs in the absence of toll-like receptor 4-mediated signaling. J Immunol. (2002) 168:2424–32. doi: 10.4049/jimmunol.168.5.2424
126. Massari P, Henneke P, Ho Y, Latz E, Golenbock DT, Wetzler LM. Cutting edge: Immune stimulation by neisserial porins is toll-like receptor 2 and MyD88 dependent. J Immunol. (2002) 168:1533–7. doi: 10.4049/jimmunol.168.4.1533
127. Massari P, Visintin A, Gunawardana J, Halmen KA, King CA, Golenbock DT, et al. Meningococcal porin PorB binds to TLR2 and requires TLR1 for signaling. J Immunol. (2006) 176:2373–80. doi: 10.4049/jimmunol.176.4.2373
128. Liu X, Wetzler LM, Massari P. The PorB porin from commensal Neisseria lactamica induces Th1 and Th2 immune responses to ovalbumin in mice and is a potential immune adjuvant. Vaccine (2008) 26:786–96. doi: 10.1016/j.vaccine.2007.11.080
129. Toussi DN, Carraway M, Wetzler LM, Lewis LA, Liu X, Massari P. The amino acid sequence of Neisseria lactamica PorB surface-exposed loops influences Toll-like receptor 2-dependent cell activation. Infect Immun. (2012) 80:3417–28. doi: 10.1128/IAI.00683-12
130. Zhu W, Tomberg J, Knilans KJ, Anderson JE, McKinnon KP, Sempowski GD, et al. Properly folded and functional PorB from Neisseria gonorrhoeae inhibits dendritic cell stimulation of CD4(+) T cell proliferation. J Biol Chem. (2018) 293:11218–29. doi: 10.1074/jbc.RA117.001209
131. Fisette PL, Ram S, Andersen JM, Guo W, Ingalls RR. The Lip lipoprotein from Neisseria gonorrhoeae stimulates cytokine release and NF-kappaB activation in epithelial cells in a Toll-like receptor 2-dependent manner. J Biol Chem. (2003) 278:46252–60. doi: 10.1074/jbc.M306587200
132. Gaudet RG, Sintsova A, Buckwalter CM, Leung N, Cochrane A, Li J, et al. Cytosolic detection of the bacterial metabolite HBP activates TIFA-dependent innate immunity. Science (2015) 348:1251–5. doi: 10.1126/science.aaa4921
133. Andrade WA, Agarwal S, Mo S, Shaffer SA, Dillard JP, Schmidt T, et al. Type I Interferon induction by Neisseria gonorrhoeae: dual requirement of cyclic GMP-AMP synthase and Toll-like receptor 4. Cell Rep. (2016) 15:2438–48. doi: 10.1016/j.celrep.2016.05.030
134. Gall A, Gaudet RG, Gray-Owen SD, Salama NR. TIFA signaling in gastric epithelial cells initiates the cag Type 4 Secretion System-dependent innate immune response to Helicobacter pylori infection. mBio (2017) 8:e01168–17. doi: 10.1128/mBio.01168-17
135. Givan AL, White HD, Stern JE, Colby E, Gosselin EJ, Guyre PM, et al. Flow cytometric analysis of leukocytes in the human female reproductive tract: comparison of fallopian tube, uterus, cervix, and vagina. Am J Reprod Immunol. (1997) 38:350–9. doi: 10.1111/j.1600-0897.1997.tb00311.x
136. Ardighieri L, Lonardi S, Moratto D, Facchetti F, Shih Ie M, Vermi W, et al. Characterization of the immune cell repertoire in the normal fallopian tube. Int J Gynecol Pathol. (2014) 33:581–91. doi: 10.1097/PGP.0000000000000095
137. Stevens JS, Criss AK. Pathogenesis of Neisseria gonorrhoeae in the female reproductive tract: neutrophilic host response, sustained infection, and clinical sequelae. Curr Opin Hematol. (2018) 25:13–21. doi: 10.1097/MOH.0000000000000394
138. Murphy K, Travers P, Walport M, Janeway C. Janeway's Immunobiology. New York, NY: Garland Science (2012).
139. Zhu W, Ventevogel MS, Knilans KJ, Anderson JE, Oldach LM, McKinnon KP, et al. Neisseria gonorrhoeae suppresses dendritic cell-induced, antigen-dependent CD4 T cell proliferation. PLoS ONE (2012) 7:e41260. doi: 10.1371/journal.pone.0041260
140. Yu Q, Chow EM, Mccaw SE, Hu N, Byrd D, Amet T, et al. Association of Neisseria gonorrhoeae Opa(CEA) with dendritic cells suppresses their ability to elicit an HIV-1-specific T cell memory response. PLoS ONE (2013) 8:e56705. doi: 10.1371/journal.pone.0056705
141. Van Vliet SJ, Steeghs L, Bruijns SC, Vaezirad MM, Snijders Blok C, Arenas Busto JA, et al. Variation of Neisseria gonorrhoeae lipooligosaccharide directs dendritic cell-induced T helper responses. PLoS Pathog. (2009) 5:e1000625. doi: 10.1371/journal.ppat.1000625
142. Zhang J, Li G, Bafica A, Pantelic M, Zhang P, Broxmeyer H, et al. Neisseria gonorrhoeae enhances infection of dendritic cells by HIV type 1. J Immunol. (2005) 174:7995–8002. doi: 10.4049/jimmunol.174.12.7995
143. Fleming DT, Wasserheit JN. From epidemiological synergy to public health policy and practice: the contribution of other sexually transmitted diseases to sexual transmission of HIV infection. Sex Transm Infect. (1999) 75:3–17. doi: 10.1136/sti.75.1.3
144. Makepeace BL, Watt PJ, Heckels JE, Christodoulides M. Interactions of Neisseria gonorrhoeae with mature human macrophage opacity proteins influence production of proinflammatory cytokines. Infect Immun. (2001) 69:1909–13. doi: 10.1128/IAI.69.3.1909-1913.2001
145. Duncan JA, Gao X, Huang MT, O'connor BP, Thomas CE, Willingham SB, et al. Neisseria gonorrhoeae activates the proteinase cathepsin B to mediate the signaling activities of the NLRP3 and ASC-containing inflammasome. J Immunol. (2009) 182:6460–9. doi: 10.4049/jimmunol.0802696
146. Zughaier SM, Kandler JL, Shafer WM. Neisseria gonorrhoeae modulates iron-limiting innate immune defenses in macrophages. PLoS ONE (2014) 9:e87688. doi: 10.1371/journal.pone.0087688
147. Leuzzi R, Serino L, Scarselli M, Savino S, Fontana MR, Monaci E, et al. Ng-MIP, a surface-exposed lipoprotein of Neisseria gonorrhoeae, has a peptidyl-prolyl cis/trans isomerase (PPIase) activity and is involved in persistence in macrophages. Mol Microbiol. (2005) 58:669–81. doi: 10.1111/j.1365-2958.2005.04859.x
148. Ritter JL, Genco CA. Neisseria gonorrhoeae-induced inflammatory pyroptosis in human macrophages is dependent on intracellular gonococci and lipooligosaccharide. J Cell Death (2018) 11:1–12. doi: 10.1177/1179066017750902
149. Chateau A, Seifert HS. Neisseria gonorrhoeae survives within and modulates apoptosis and inflammatory cytokine production of human macrophages. Cell Microbiol. (2016) 18:546–60. doi: 10.1111/cmi.12529
150. Murray PJ, Allen JE, Biswas SK, Fisher EA, Gilroy DW, Goerdt S, et al. Macrophage activation and polarization: nomenclature and experimental guidelines. Immunity (2014) 41:14–20. doi: 10.1016/j.immuni.2014.06.008
151. Ortiz MC, Lefimil C, Rodas PI, Vernal R, Lopez M, Acuna-Castillo C, et al. Neisseria gonorrhoeae modulates immunity by polarizing human macrophages to a M2 profile. PLoS ONE (2015) 10:e0130713. doi: 10.1371/journal.pone.0130713
152. Escobar A, Candia E, Reyes-Cerpa S, Villegas-Valdes B, Neira T, Lopez M, et al. Neisseria gonorrhoeae induces a tolerogenic phenotype in macrophages to modulate host immunity. Mediators Inflamm. (2013) 2013:127017. doi: 10.1155/2013/127017
153. Feinen B, Jerse AE, Gaffen SL, Russell MW. Critical role of Th17 responses in a murine model of Neisseria gonorrhoeae genital infection. Mucosal Immunol. (2010) 3:312–21. doi: 10.1038/mi.2009.139
154. Packiam M, Wu H, Veit SJ, Mavrogiorgos N, Jerse AE, Ingalls RR. Protective role of Toll-like receptor 4 in experimental gonococcal infection of female mice. Mucosal Immunol. (2012) 5:19–29. doi: 10.1038/mi.2011.38
155. Liu Y, Islam EA, Jarvis GA, Gray-Owen SD, Russell MW. Neisseria gonorrhoeae selectively suppresses the development of Th1 and Th2 cells, and enhances Th17 cell responses, through TGF-beta-dependent mechanisms. Mucosal Immunol. (2012) 5:320–31. doi: 10.1038/mi.2012.12
156. Liu Y, Liu W, Russell MW. Suppression of host adaptive immune responses by Neisseria gonorrhoeae: role of interleukin 10 and type 1 regulatory T cells. Mucosal Immunol. (2014) 7:165–76. doi: 10.1038/mi.2013.36
157. Delaney ML, Onderdonk AB. Nugent score related to vaginal culture in pregnant women. Obstet Gynecol. (2001) 98:79–84. doi: 10.1016/S0029-7844(01)01402-8
158. Anahtar MN, Gootenberg DB, Mitchell CM, Kwon DS. Cervicovaginal microbiota and reproductive health: the virtue of simplicity. Cell Host Microbe (2018) 23:159–68. doi: 10.1016/j.chom.2018.01.013
159. Chen C, Song X, Wei W, Zhong H, Dai J, Lan Z, et al. The microbiota continuum along the female reproductive tract and its relation to uterine-related diseases. Nat Commun. (2017) 8:875. doi: 10.1038/s41467-017-00901-0
160. Miles SM, Hardy BL, Merrell DS. Investigation of the microbiota of the reproductive tract in women undergoing a total hysterectomy and bilateral salpingo-oopherectomy. Fertil Steril. 107:813–20 e811. doi: 10.1016/j.fertnstert.2016.11.028
161. Pelzer ES, Willner D, Buttini M, Hafner LM, Theodoropoulos C, Huygens F. The fallopian tube microbiome: implications for reproductive health. Oncotarget (2018) 9:21541–51. doi: 10.18632/oncotarget.25059
162. St Amant DC, Valentin-Bon IE, Jerse AE. Inhibition of Neisseria gonorrhoeae by Lactobacillus species that are commonly isolated from the female genital tract. Infect Immun. (2002) 70:7169–71. doi: 10.1128/IAI.70.12.7169-7171.2002
163. Spurbeck RR, Arvidson CG. Inhibition of Neisseria gonorrhoeae epithelial cell interactions by vaginal Lactobacillus species. Infect Immun. (2008) 76:3124–30. doi: 10.1128/IAI.00101-08
164. Ruiz FO, Pascual L, Giordano W, Barberis L. Bacteriocins and other bioactive substances of probiotic lactobacilli as biological weapons against Neisseria gonorrhoeae. Pathog Dis. (2015) 73: ftv013. doi: 10.1093/femspd/ftv013
165. Foschi C, Salvo M, Cevenini R, Parolin C, Vitali B, Marangoni A. Vaginal lactobacilli reduce Neisseria gonorrhoeae viability through multiple strategies: an in vitro study. Front Cell Infect Microbiol. (2017) 7:502. doi: 10.3389/fcimb.2017.00502
166. Edwards JL, Shao JQ, Ault KA, Apicella MA. Neisseria gonorrhoeae elicits membrane ruffling and cytoskeletal rearrangements upon infection of primary human endocervical and ectocervical cells. Infect Immun. (2000) 68:5354–63. doi: 10.1128/IAI.68.9.5354-5363.2000
167. Christodoulides M, Everson JS, Liu BL, Lambden PR, Watt PJ, Thomas EJ, et al. Interaction of primary human endometrial cells with Neisseria gonorrhoeae expressing green fluorescent protein. Mol Microbiol. (2002) 35:32–43. doi: 10.1046/j.1365-2958.2000.01694.x
168. Harvey HA, Ketterer MR, Preston A, Lubaroff D, Williams R, Apicella MA. Ultrastructural analysis of primary human urethral epithelial cell cultures infected with Neisseria gonorrhoeae. Infect Immun. (1997) 65:2420–7.
169. Karst AM, Levanon K, Drapkin R. Modeling high-grade serous ovarian carcinogenesis from the fallopian tube. Proc Natl Acad SciUSA. (2011) 108:7547–52. doi: 10.1073/pnas.1017300108
170. Karst AM, Drapkin R. Primary culture and immortalization of human fallopian tube secretory epithelial cells. Nat Protoc. (2012) 7:1755–64. doi: 10.1038/nprot.2012.097
171. Pardo-Saganta A, Tata PR, Law BM, Saez B, Chow RD, Prabhu M, et al. Parent stem cells can serve as niches for their daughter cells. Nature (2015) 523:597–601. doi: 10.1038/nature14553
172. Ghosh A, Syed SM, Tanwar PS. In vivo genetic cell lineage tracing reveals that oviductal secretory cells self-renew and give rise to ciliated cells. Development (2017) 144:3031–41. doi: 10.1242/dev.149989
173. Yucer N, Holzapfel M, Jenkins Vogel T, Lenaeus L, Ornelas L, Laury A, et al. Directed differentiation of human induced pluripotent stem cells into fallopian tube epithelium. Sci Rep. (2017) 7:10741. doi: 10.1038/s41598-017-05519-2
174. Wang J, Zhao Y, Wu X, Yin S, Chuai Y, Wang A. The utility of human fallopian tube mucosa as a novel source of multipotent stem cells for the treatment of autologous reproductive tract injury. Stem Cell Res Ther. (2015a) 6:98. doi: 10.1186/s13287-015-0094-1
175. Kessler M, Hoffmann K, Brinkmann V, Thieck O, Jackisch S, Toelle B, et al. The Notch and Wnt pathways regulate stemness and differentiation in human fallopian tube organoids. Nat Commun. (2015) 6:8989. doi: 10.1038/ncomms9989
176. Iakobachvili N, Peters PJ. Humans in a dish: the potential of organoids in modeling immunity and infectious diseases. Front Microbiol. (2017) 8:2402. doi: 10.3389/fmicb.2017.02402
177. Poole NM, Rajan A, Maresso AW. Human intestinal enteroids for the study of bacterial adherence, invasion, and translocation. Curr Protoc Microbiol. (2018) 50:e55. doi: 10.1002/cpmc.55
178. Driehuis E, Clevers H. CRISPR/Cas 9 genome editing and its applications in organoids. Am J Physiol Gastrointest Liver Physiol. (2017) 312:G257–65. doi: 10.1152/ajpgi.00410.2016
179. Nie J, Hashino E. Organoid technologies meet genome engineering. EMBO Rep. (2017) 18:367–76. doi: 10.15252/embr.201643732
180. Ronaldson-Bouchard K, Vunjak-Novakovic G. Organs-on-a-chip: a fast track for engineered human tissues in drug development. Cell Stem Cell (2018) 22:310–24. doi: 10.1016/j.stem.2018.02.011
181. Xiao S, Coppeta JR, Rogers HB, Isenberg BC, Zhu J, Olalekan SA, et al. A microfluidic culture model of the human reproductive tract and 28-day menstrual cycle. Nat Commun. (2017) 8:14584. doi: 10.1038/ncomms14584
182. Lashkari BS, Anumba DO. Estradiol alters the immune-responsiveness of cervical epithelial cells stimulated with ligands of Toll-like receptors 2 and 4. PLoS ONE (2017) 12:e0173646. doi: 10.1371/journal.pone.0173646
183. Glaser AK, Reder NP, Chen Y, Mccarty EF, Yin C, Wei L, et al. Light-sheet microscopy for slide-free non-destructive pathology of large clinical specimens. Nat Biomed Eng. (2017) 1. doi: 10.1038/s41551-017-0084
184. Liu TL, Upadhyayula S, Milkie DE, Singh V, Wang K, Swinburne IA, et al. Observing the cell in its native state: Imaging subcellular dynamics in multicellular organisms. Science (2018) 360. doi: 10.1126/science.aaq1392
185. Wang S, Burton JC, Behringer RR, Larina IV. In vivo micro-scale tomography of ciliary behavior in the mammalian oviduct. Sci Rep. (2015) 5:13216. doi: 10.1038/srep13216
186. Harvey HA, Porat N, Campbell CA, Jennings M, Gibson BW, Phillips NJ, et al. Gonococcal lipooligosaccharide is a ligand for the asialoglycoprotein receptor on human sperm. Mol Microbiol. (2000) 36:1059–70. doi: 10.1046/j.1365-2958.2000.01938.x
187. Den Hartog JE, Ouburg S, Land JA, Lyons JM, Ito JI, Pena AS, et al. Do host genetic traits in the bacterial sensing system play a role in the development of Chlamydia trachomatis-associated tubal pathology in subfertile women? BMC Infect Dis. (2006) 6:122. doi: 10.1186/1471-2334-6-122
188. Karimi O, Ouburg S, De Vries HJ, Pena AS, Pleijster J, Land JA, et al. TLR2 haplotypes in the susceptibility to and severity of Chlamydia trachomatis infections in Dutch women. Drugs Today (2009) 45(Suppl. B):67–74.
189. Naik S, Larsen SB, Gomez NC, Alaverdyan K, Sendoel A, Yuan S, et al. Inflammatory memory sensitizes skin epithelial stem cells to tissue damage. Nature (2017) 550:475–80. doi: 10.1038/nature24271
Keywords: Neisseria gonorrhoeae, fallopian tube, oviduct, organ culture, tissue explant, peptidoglycan, cilia, pelvic inflammatory disease
Citation: Lenz JD and Dillard JP (2018) Pathogenesis of Neisseria gonorrhoeae and the Host Defense in Ascending Infections of Human Fallopian Tube. Front. Immunol. 9:2710. doi: 10.3389/fimmu.2018.02710
Received: 31 August 2018; Accepted: 02 November 2018;
Published: 21 November 2018.
Edited by:
Michael W. Russell, University at Buffalo, United StatesReviewed by:
Wenxia Song, University of Maryland, College Park, United StatesJennifer L. Edwards, The Ohio State University, United States
Copyright © 2018 Lenz and Dillard. This is an open-access article distributed under the terms of the Creative Commons Attribution License (CC BY). The use, distribution or reproduction in other forums is permitted, provided the original author(s) and the copyright owner(s) are credited and that the original publication in this journal is cited, in accordance with accepted academic practice. No use, distribution or reproduction is permitted which does not comply with these terms.
*Correspondence: Joseph P. Dillard, anBkaWxsYXJkQHdpc2MuZWR1