- 1PhD Program in Clinical and Experimental Medicine and Medical Humanities, University of Insubria, Varese, Italy
- 2Neurology Unit, Department of Translational Medicine, Interdisciplinary Research Centre of Autoimmune Diseases, Movement Disorders Centre, University of Piemonte Orientale, Novara, Italy
- 3Biolab Research Srl, Research and Development, Novara, Italy
Background: Parkinson's disease (PD) is characterized by loss of dopaminergic neurons and intraneuronal accumulation of alpha-synuclein, both in the basal ganglia and in peripheral sites, such as the gut. Peripheral immune activation and reactive oxygen species (ROS) production are important pathogenetic features of PD. In this context, the present study focused on the assessment of in vitro effects of probiotic bacterial strains in PBMCs isolated from PD patients vs. healthy controls.
Methods: 40 PD patients and 40 matched controls have been enrolled. Peripheral blood mononuclear cells (PBMCs) were isolated and co-cultured with a selection of probiotics microorganisms belonging to the lactobacillus and bifidobacterium genus. In vitro release of the major pro- (Tumor Necrosis Factor-alpha and Interleukin-17A and 6) and anti-inflammatory (Interleukin 4 and 10) cytokines by PBMCs, as well as the production of ROS was investigated. Furthermore, we assessed the ability of probiotics to influence membrane integrity, antagonize the growth of potential pathogen bacteria, such as Escherichia coli and Klebsiella pneumoniae and encode tyrosine decarboxylase genes (tdc).
Results: All probiotic strains were able to inhibit inflammatory cytokines and ROS production in both patients and controls. The most striking results were obtained in PD subjects with L. salivarius LS01 and L. acidophilus which significantly reduced pro-inflammatory and increased the anti-inflammatory cytokines (p < 0.05). Furthermore, most strains determined restoration of membrane integrity and inhibition of E. coli and K. pneumoniae. Finally, we also showed that all the strains do not carry tdc gene, which is known to decrease levodopa bioavailability in PD patients under treatment.
Conclusions: Probiotics exert promising in vitro results in decreasing pro-inflammatory cytokines, oxidative stress and potentially pathogenic bacterial overgrowth. In vivo longitudinal data are mandatory to support the use of bacteriotherapy in PD.
Introduction
Parkinson's disease (PD) is a common neurodegenerative disease, characterized by loss of dopaminergic neurons and intracellular accumulation of alpha-synuclein (α-syn) in the surviving neurons (1). Involvement of inflammatory mechanisms, with an imbalance between detrimental and protective immune functions (2), as well as neurotoxicity of reactive oxygen species (ROS) have been documented by several studies (3, 4). Both neuroinflammation and ROS may favor α-syn aggregation which may in turn increase pro-inflammatory cytokines and oxidative stress, thus triggering a vicious circle (5). Clinical presentation of PD is classically defined by the presence of motor symptoms such as bradykinesia, rest tremor, and rigidity. On the other hand, patients often complain of non-motor symptoms like hyposmia, constipation, pain and psychiatric conditions (e.g., anxiety, depression) that in many cases may precede the onset of clinically established disease (6, 7). Seminal work by Braak et al. hypothesized an initial aggregation of α-syn in the gut with subsequent propagation along the vagus nerve to the brain to reach the substantia nigra in the mesencephalon (8). Moreover, constipation represents a relevant symptom of PD, affecting about 70–80% of patients (9) and may precede motor symptoms by 20 years (10). The loss of enteric dopaminergic neurons determines an impairment of gastric mobility with an increased dopaminergic content and overexpression of dopaminergic receptors in the stomach (11). Furthermore, PD patients present an increased intestinal permeability and higher expression of colonic pro-inflammatory cytokines (12). Accordingly, in an α-syn overexpressing murine model of PD, gut microbiota is necessary for both microglia activation and motor impairment (13). In addition, a direct correlation between gut bacterial count and disease progression was found in PD patients (14). PD patients have a different composition of gut microbiota compared to healthy subjects (15) with reduced levels of Prevotellaceae and abundance of Enterobacteriaceae (16). Prevotella are in fact involved in the production of thiamine and folate, both of which are important for proper intestinal homeostasis (17). On the contrary, increased levels of Enterobacteriaceae have been associated to a severe PD phenotype with postural instability and gait difficulty. (16).
Of note, there is evidence that probiotics may modulate not only inflammation through cytokines production (18, 19), but also oxidative damage through a down-modulation of ROS (20). Another relevant aspect of the host-microbial interaction is the established role of infections in accelerating clinical decline in PD patients (21). In a recent investigation, focused on the clinical features and therapeutic outcomes of infected patients with or without PD, the incidence of respiratory tract and urinary tract infections was higher in PD than in age and sex-matched non-PD patients. Additionally, a longer mean hospitalization time was observed in the PD group (22). In this regard, specific probiotic strains may potentially counteract the growth of common pathogens, such as Escherichia coli (E. coli) and Klebsiella pneumoniae (K. pneumoniae) (23, 24). Furthermore, Van Kessel et al. recently reported that some probiotic strains produce tyrosine decarboxylase (TDC) (25). This bacterial enzyme efficiently converts levodopa to dopamine in the gut, even in the presence of human decarboxylase inhibitors or tyrosine, a competitive substrate. Accordingly, in situ levels of levodopa in PD patients are decreased by significant abundance of gut bacterial TDC (25). TDC genes (tdc) have been detected in particular in the genome of numerous bacterial species within the genera Lactobacillus and Enterococcus (26, 27). Abundance of bacterial tdc in stool specimens of PD patients was indeed correlated with increased daily dosage requirement of levodopa (25).
On this background, the aim of our study was to investigate the in vitro effects of probiotics on samples from a group of PD patients compared to healthy subjects. To do that, we assessed cytokine and reactive oxygen species (ROS) release by peripheral blood mononuclear cells (PBMCs), and restoration of artificial membrane permeability. In addition, we investigated the ability of the selected probiotics to directly inhibit E. coli and K. pneumoniae. Finally, we verified the absence of tdc within the genome of the selected probiotic strains.
Patients and Methods
Patients
We enrolled 40 patients with PD (15 women and 25 men, mean age 70 ± 8 years) and 40 age-matched healthy donors (HD, 18 women and 22 men, mean age 68 ± 7 years). PD diagnosis was performed according to the Movement Disorders Society (MDS) diagnostic criteria, e.g., when: (a) subjects presented with a parkinsonism, defined as bradykinesia, associated to rest tremor or rigidity without signs of atypical parkinsonism; (b) exclusion criteria, red flags and supportive criteria were assessed (28).
Patients were regularly followed-up at the Movement Disorder Center of Maggiore Hospital in Novara (Italy). For each patient the following parameters were considered: gender, age at onset, disease duration, Hoehn & Yahr stage (29), UPDRS III score (30), and PD therapy calculating the levodopa equivalent doses (LED) of each drug according to Tomlinson et al. (31).
Subjects with past or concomitant autoimmune disease and with a previous or ongoing immune-modulating or suppressive therapy were excluded. All subjects underwent a complete blood cell (CBC) analysis including C-Reactive Protein (CRP) and erythrocyte sedimentation rate in order to exclude both defects or activation of the immune system. All subjects were of Italian origin.
This study was approved by the local Ethics Committee (CE 65/16). Patients were included in the study after having read and signed an informed consent form for research purpose.
Cell Cultures
Twenty milliliters of blood were drawn by venipuncture in vacuum tubes containing heparin on the same day of the clinical assessment. In order to rule out any confounding factors caused by circadian rhythm, all samples were collected at the same time of the day. Human PBMCs were isolated from heparinized blood by Healthy Donors (HD-PBMCs) and PD patients (PD-PBMCs). For cell isolation, standard techniques of dextran sedimentation and Histopaque (density = 1.077 g/cm3) gradient centrifugation (400 × g, 30 min, room temperature) were used. Cells were then recovered by thin suction at the interface. Isolated cells were then re-suspended in RPMI 1640 medium supplemented with 5% fetal bovine serum (FBS) and 2 mM glutamine. Cell viability (trypan blue dye exclusion) was usually >98%.
Bacteria and Growth Conditions
Six probiotic strains (Lactobacillus salivarius LS01 DSM 22775, Lactobacillus plantarum LP01 LMG P-21021, Lactobacillus acidophilus LA02 DSM 21717, Lactobacillus rhamnosus LR06 DSM 21981, Bifidobacterium animalis subsp. lactis BS01 LMG P-21384, Bifidobacterium breve BR03 DSM 16604), from the Probiotical SpA collection, have been used in the present study: probiotic strains were stored in 20% glycerol at −80°C. More than 90% of the cells were alive upon thawing. Before use, microorganisms were grown in anaerobic conditions with CO2-generating kits (Anaerocult A; Merck, Darmstadt, Germany) overnight at 37°C in de Man-Rogosa-Sharpe (MRS) broth containing 0.05% cysteine hydrochloride, and then sub-cultured until the mid-log phase. For the enumeration of live bacteria, the BD Cell Viability Kit (BD Biosciences, Milan, Italy) were used as instructed by the manufacturer. For stimulation experiments, bacteria were suspended in RPMI-1640 medium [Invitrogen, Italy] and added to PBMCs cultures.
Cytokine Release
Cytokine release by PBMCs was measured with an enzyme-linked immunoassay kit according to the manufacturer's instructions (ELISA Ready-SET-Go! Affymetrix eBioscience, USA). Interleukin 17A (IL-17A), tumor necrosis factor α (TNF-α), and IL-10 were assessed in both healthy controls and PD patients, whereas IL-6 and IL-4 were assessed only in PD-PBMCs (before and after probiotic stimulation). The levels of each cytokine were calculated in ρg/ml, in accordance with the manufacturer's instructions. For these experiments, HD and PD PBMCs (1 × 106 cells/plate) were pre-treated for 24 h with the indicated probiotic strains in 1:1 ratio. Only HD PBMCs were treated previously with purified lipopolysaccharide (LPS) from E. coli 055:B5 (Sigma Chemicals, Milan, Italy) at a concentration of 10 g/mL.
Superoxide Anion () Production
HD- and PD-PBMCs (1 × 106 cells/plate) were treated for 24 h with probiotic strains in 1:1 ratio. production was evaluated by the superoxide dismutase-sensitive cytochrome C reduction assay and calculated as nmol reduced cytochrome C/106 cells/30 min, using an extinction coefficient of 21.1 mM. To avoid interference with spectrophotometrical recordings, cells were incubated with RPMI 1,640 without phenol red and FBS. Basal values ( production from unstimulated PBMCs) in HD were 2.2 ± 0.4 nmol reduced cytochrome C/106 cells/30', and in PD patient-PBMCs were 140 ± 12 nmol reduced cytochrome C/106 cells/30'. PMA is a stimulus known to induce a strong and significant respiratory burst. In line with this, PMA 10−7 M exposure determined a significant increase of cytochrome C levels in HD-PBMCs: 52 ± 4.5 nmol reduced cytochrome C/106 cells/30′.
Measurements of TransEpithelial Electrical Resistance (TEER)
Caco-2 cells are human colonic adenocarcinoma cells that form confluent, polarized epithelial monolayers with well-differentiated intercellular tight junctions structures. The integrity of the barrier function can be measured with TransEpithelial Electrical Resistance (TEER). TEER is an in vitro measurement of the movement of ions across the paracellular pathway.
A reduction in TEER may represent an early expression of cell damage and indicates that the barrier function of the intestine is decreased. Caco-2 cell lines have been extensively used over the last 20 years as a model of the intestinal barrier. The experiment was performed with an inflammatory stressor (a combination of TNF-α and IL1-ß), which is known to reduce the relative TEER of Caco-2 cells. The monolayer of Caco-2 cells was first exposed to the probiotic bacteria for 1 h, followed by exposure to the inflammatory stressor in the presence of the same probiotic bacteria, also for 1 h. After a recovery time of 24 h the TEER of the monolayer was measured. The results were compared to the TEER of a monolayer that was exposed to the stressor alone and to an unexposed sample. Cells were grown in Dulbecco's Modified Eagle Medium (DMEM) with 10% fetal bovine serum. Caco-2 (1 × 106 cells/well) differentiated at 20 days were provided by the Anemocyte s.r.l. (Gerenzano, Varese, Italy). TEER was measured in each monolayer before adding 106 AFU [Active Fluorescent Units were evaluated with cytofluorimetric analytical method ISO 19344:2015 (E)-IDF 232:2015 (E)] of probiotic strains onto the apical surface for 24 h prior to treatment of the basolateral medium with TNF-α and IL1-ß (10 ng/ml; Thermo Scientific, USA). It was determined that 106 AFU of probiotic strains did not cause deleterious effects on epithelial cells over the time-course of the experiments and that the medium used in this experiment did not cause bacterial overgrowth.
Spot-on Lawn Antimicrobial Assay/Agar Spot Antimicrobial Assay
The antimicrobial activity against E. coli and K. pneumoniae was assessed according to the protocol described by Santini C. (32). Briefly, 5 μl of probiotic overnight fresh cultures with an optical density (OD) at 600 nm close to 1 were spotted on the surface of MRS agar plates and incubated anaerobically for 5 h at 37°C to allow strain development (spot). The E. coli ATCC 8739 strain or K. pneumoniae ATCC 13883 strain was inoculated in Brain Heart Infusion (BHI) soft agar and dispensed onto spot plates. When the top agar was solid, the plates were inverted and incubated in conditions of anaerobiosis at 37°C for 48 h. At the end of incubation, plates were examined for the appearance of clear zones showing the antagonistic activity. The plate inhibition technique experiments were carried out on triplicates, and the mean values of growth inhibition zones around the disks were measured using a ruler (mm) and recorded.
Search for Tyrosine Decarboxylase (TDC) Genes
To identify whether the genomes of the probiotic strains employed encoded tdc, the TDC protein sequence (EOT87933) from Enterococcus faecalis v583 was used as a query along with E. faecalis v583 as a positive control using the BLAST program of PATRIC suite (www.patricbrc.org). E. faecalis V583 TDC protein sequence (NCBI accession: EOT87933) was downloaded in FASTA format. PATRIC suite was used for the Annotation of the six bacterial strains using the RAST tool kit encoded within the software. Annotated genomes were grouped together and BLASTed against the TDC protein sequences.
Statistical Analysis
Results are expressed as the means ± SEM of duplicate measures determined in three independent experiments. Differences between unstimulated and stimulated samples were tested using the t test with GraphPad Prism 6.0 software. Correlations between probiotic strain and clinical-demographic variables were calculated using Spearman test. Values of p < 0.05 were considered significant.
Results
Cytokine Release
IL-10 and IL-4 are important cytokines in the regulation of immune responses, counterbalancing the pro-inflammatory effects of TNF-α, IL-6 and IL-17A. Cytokines modulation by different probiotic strains was measured in PBMCs from both PD patients and healthy donors (Table 1 and Figure 1; Table S1). In PD-PBMCs, most probiotic strains determined a statistically significant reduction of pro-inflammatory cytokines production (TNF-α, IL-6, and IL-17A) and an increase of the anti-inflammatory IL-4 and IL-10. The most striking results were obtained with LS01 (TNF-α: baseline 255.52 ± 29.55 pg/ml, after stimulus 146.69 ± 28.67 pg/ml, p < 0.001; IL-6 baseline 197.2 ± 18.2 pg/ml, after stimulus 132.5 ± 4.2 pg/ml, p < 0.001; IL17-A baseline 114.08 ± 15.41 pg/ml, after stimulus 52.48 ± 9.25 pg/ml, p < 0.001; IL-4 baseline 102.00 ± 16.4 pg/ml, after stimulus 149.6 ± 34.7 pg/ml, p < 0.001; IL-10: baseline 140.12 ± 18.01 pg/ml, after stimulus 194.24 ± 15.42 pg/ml, p < 0.001) and LA02 (TNF-α: baseline 228.90 ± 26.89 pg/ml, after stimulus 195.15 ± 33.52 pg/ml, p < 0.001; IL-6 baseline 180.2 ± 92.5 pg/ml, after stimulus 92.5 ± 5.9 pg/ml, p < 0.001; IL17-A baseline 112.81 ± 15.28 pg/ml, after stimulus 87.99 ± 12.80 pg/ml, p < 0.001; IL-4 baseline 26.3 ± 2.5 pg/ml, after stimulus 45.6 ± 4.9 pg/ml, p < 0.05; IL-10: baseline 165.33 ± 20.53 pg/ml, after stimulus 192.18 ± 23.22 pg/ml, p < 0.001). The remaining data, including all p values, are shown in Table 1.
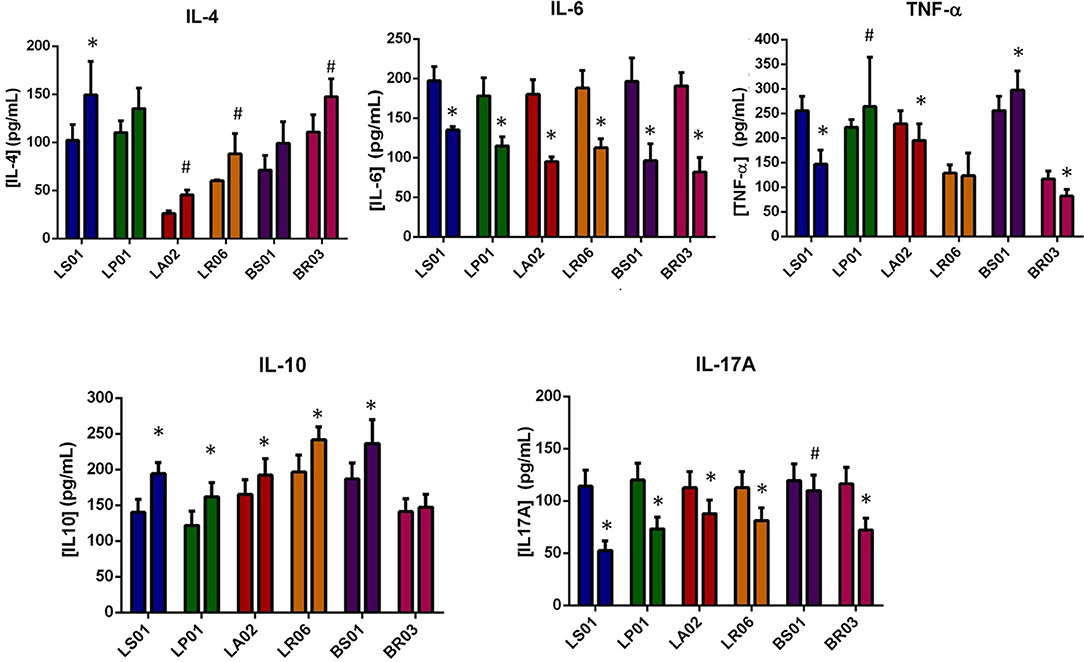
Figure 1. Modulation of cytokine production by probiotic strains. For each probiotic strain the first column indicates the baseline values, while the second after probiotic administration. *p < 0.001; #p < 0.05 post vs. pre-exposure from PD-PBMCs.
Superoxide Anion () Production
First, we found a statistically significant difference in baseline O2− production from unstimulated PBMCs between patients and controls. In fact, reduced cytochrome C in HD was 2.2 ± 0.4 nmol vs. 140 ± 12 nmol/106 cells/30' in PD-PBMCs (p < 0.001). To test the antioxidant effects of probiotics on HD-PBMCs we induced a toxic condition using PMA, a stimulus known to determine a strong and significant respiratory burst. Consistently, PMA exposure in HD-PBMCs increased levels of reduced cytochrome c (52 ± 4.5 nmol). As depicted in Figure 2, after exposure to probiotic strains, we found an overall decrease of production in unstimulated PBMCs from PD patients and in PMA-stimulated PBMCs from HD. In detail, LS01, LP01, LA02, LR06, BS01 caused a robust decrease of from PD-PBMCs (p < 0.01 post vs. pre-exposure). A weaker, though statistically significant, effect was obtained from PMA-stimulated HD-PBMCs after exposure to LS01, LP01, BR03 (p < 0.05 post vs. pre-exposure).
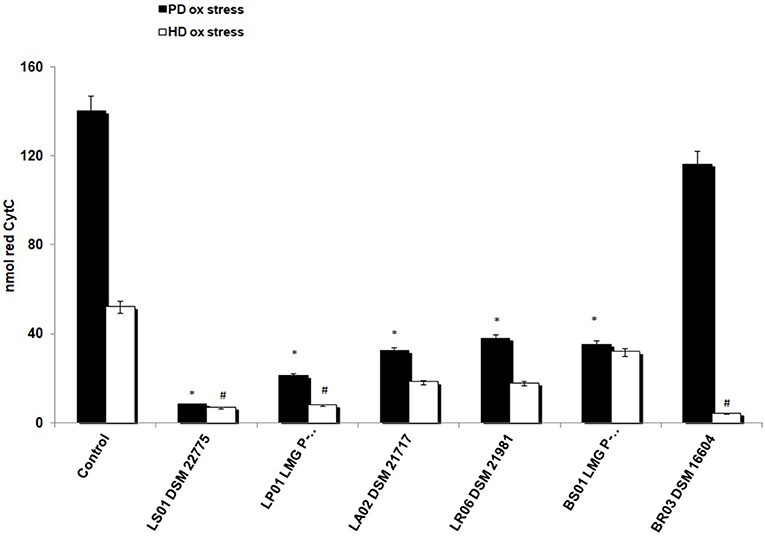
Figure 2. Reduction of superoxide anion () production. *p < 0.01 post vs. pre-exposure from PD-PBMCs. #p < 0.05 post vs. pre-exposure from PMA-exposed HD-PBMCs.
TEER Evaluation
Three strains (LP01, LR06, and BR03) provided higher protection of epithelial cells against the cytokine-induced barrier dysfunction (p < 0.001), whereas three others (LS01, LA02, and BS01) had a lower, though still significant, effect (p < 0.05). The TEER ratio was measured before adding the bacterial inoculum (CTR) and in a damage-tissue model and after addition and incubation of the probiotic strains on Caco-2 monolayer. The results are shown in Table 2 and Figure S1.
Inhibition of E. coli and K. pneumoniae
As shown in Table 3, most probiotic strains showed a robust inhibitory capacity against the target pathogen strains E. coli and K. pneumoniae involved in the comorbidities of PD. Particularly, LP01 and LR06 exerted the highest inhibition. Moreover, such inhibition was not observed when a negative control (MRS acidified at pH 4.3 but without any probiotic strains; data not shown) was tested, thus confirming the specificity of the detected antagonistic activity.
Tyrosine Decarboxylase (TDC) Genes
Only E. faecalis V583 gave an Identity value equal to 100% with a Query Cover of 100%, whilst the other probiotic strains scored below 35% of Identity, thus excluding the expression of a tyrosine decarboxylase activity within the six tested probiotic strains (data not shown).
Clinical Analysis
Demographic Results
Mean age at PD onset was 65 ± 8 years. In detail, at study enrollment, 4 patients were drug naïve, 26 were taking levodopa (one of them was on Duodopa) and 10 were taking dopaminergic treatment other than levodopa (dopamine agonists and MAO inhibitors). Mean LED was 469.9 mg/day ± 354. Mean UPDRS III was 12.45 ± 6.9 points.
Gender
Comparing responses to probiotics in relation to gender, we found a statistically significant difference between male and female PD patients in ROS production from PBMCs. The effect of LR06 was more pronounced in samples from male vs. female donors (81 and 60% reduction compared to baseline levels, respectively; p < 0.05; Table 4). On the contrary, BS01 was more effective in samples from female than male patients (84 and 69% reduction compared to baseline levels, respectively; p < 0.05; Table 4).
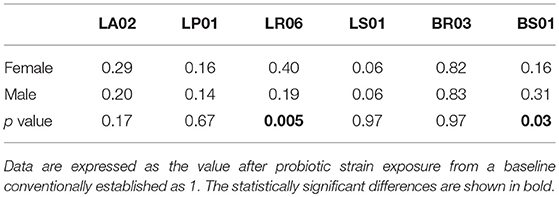
Table 4. Comparison of ROS production after each probiotic stimulation between female and male patients.
Disease Duration
We analyzed ROS and cytokine levels from PD-PBMCs exposed to different probiotic strains in relation to disease duration. We found that LA02 provided a robust anti-oxidant effect, which decreased significantly in samples of patients with longer disease duration (rho = 0.22, p < 0.05 Table 5, Figure 3). Such correlation was not detected with other strains. Furthermore, we found no other statistically significant correlation between the effect of the different strains and the remaining clinical variables (H&Y stage, UPDRS score, LED, data not shown).
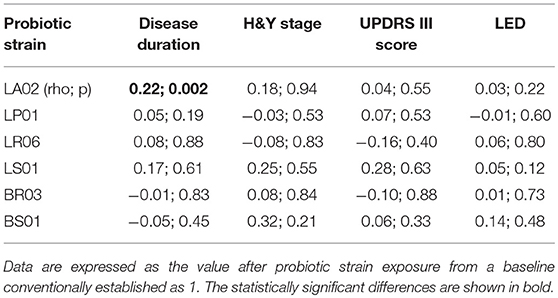
Table 5. Correlations between probiotic strains modulation of ROS production and clinical characteristics of PD patients.
Discussion
In this study we showed that probiotic strains modulate the release of cytokines and ROS by PBMCs of PD patients and healthy controls. Particularly, L. salivarius (LS01) and L. acidophilus (LA02) showed the best profiles in PD-PBMCs, being able to significantly decrease all the pro-inflammatory cytokines and increase the anti-inflammatory ones. The same strains were also able to significantly reduce ROS production in both PD and HD-PBMCs. In addition, all the tested probiotic strains restored epithelial damage in Caco-2 cells. Finally, the tested probiotic strains exerted a robust capacity of inhibiting E. coli and K. pneumoniae. These Gram-negative bacteria are frequently detected in blood cultures of septic PD patients (22). Such antagonistic activity might be ascribed to the active bacteriocins secreted by probiotic strains. Probiotics have been studied in PD for their potential symptomatic effect on constipation (33) and probiotic strains BS01, LP01, and BR03 have shown improvement in constipation and associated symptoms in healthy adults (34) and more interestingly in chronically constipated elderly (35). However, despite the great interest that recently arose around the gut-brain axis in health and disease, our study is the first to specifically address the effect of probiotics on mediators of inflammation and oxidative damage in PBMCs of PD patients. On the other hand, experimental evidence on the anti-inflammatory and anti-oxidative effects of probiotics is rapidly growing. L. plantarum displayed the capacity of decreasing the histopathological damages in a murine model of Alzheimer's disease (AD) leading to increased production of acetylcholine with consequent clinical improvement (36). Accordingly, it was shown that probiotics administration was effective in modulating cognitive functions in a group of AD patients (37). Probiotics may also be helpful in other contexts of neuroinflammation such as post-traumatic stress disorder, amyotrophic lateral sclerosis and cognitive dysfunction after surgery (38–40). Of note, in a murine model of stress, modification of gut microbiota provided both behavioral and immunological beneficial effects (41).
Altogether, such findings suggest that probiotics may represent a promising strategy to counteract the detrimental immune activation that takes place in PD. In fact, work by independent groups is indicating that peripheral and central immune responses are strictly interconnected in PD and the study of such mechanisms may provide relevant advances in both diagnostic and therapeutic areas (42, 43). An important breakthrough in this area was the demonstration of the antigenic role of α-syn on peripheral T cells: such epitopes can in fact drive T helper and cytotoxic responses in PD patients (44). Moreover, recent work by Kustrimovic et al. showed that PD patients display a predominance of Th1 mediated responses compared to healthy controls (45). A pro-inflammatory profile, with increased production of IL-1α, IL-1β, and CXCL8 was also detected in stool samples of PD patients, further supporting the involvement of intestinal immunity in PD (46). The results of the present study support the concept of a predominantly pro-inflammatory environment in the periphery, since pro-inflammatory cytokines production was significantly increased in PD patients vs. controls. Notably, the probiotic strains tested in our in vitro experiments were able to counterbalance such pro-inflammatory response. Our results also suggest the involvement of IL17A in PD: patients present in fact higher levels of IL17A than healthy controls. The role of IL17 producing T helper cells (Th17) in the context of neurodegeneration has not yet been completely elucidated (47). There is evidence showing that Th17 cause cell death in a human iPSC-based model of PD and also evidence that Th17 can be induced and regulated by the intestinal microbiota (48, 49).
Our study has indeed some limitations: sample size is relatively small, and the cross-sectional design suggests caution in the interpretation of results. Furthermore, our data derive from in vitro experiments, which might not reflect precisely the complex pathophysiological dynamics of PD and should therefore be reproduced in vivo. Possible strategies may involve a study on an animal model of PD, or alternatively the direct evaluation of the clinical and biological effects of probiotics administration in PD patients. In both cases, a longitudinal study in which biomarkers and clinical findings are collected before and after probiotics administration would likely provide important responses.
Our data also suggest that the effect of probiotics might be different with respect to disease stage and gender. Accordingly, we found that LA02 provided a down-modulation of ROS that was more pronounced in the early stages of disease. These data need further confirmation since previous studies did not detect any correlations between clinical or biological variables and disease stage in PD patients treated with probiotics (46). Moreover, LR06 and BS01 displayed different anti-inflammatory and anti-oxidant activities in PBMCs from male compared to female PD patients. Of note, it was previously reported that microbiota composition may differ between male and female subjects and that this in turn may influence immune functions (50). One last open question regards the relationships between probiotics, peripheral immune function and dopaminergic therapy, especially considering that, to date, the influence of PD treatment on peripheral immunity is still controversial (51–53). Definite answers to this question, as well as to whether and how probiotic administration should be personalized, will likely come from longitudinal in vivo studies.
Overall, our preliminary findings suggest a potential role for probiotic strains in modulating inflammation and oxidative stress and protecting the epithelium from gut permeability. Further relevant findings include a possible inhibitory effect against E. coli and K. pneumoniae, which might be exerted without interfering with levodopa levels.
Ethics Statement
This study was approved by the local Ethics Committee (CE 65/16). Patients were included in the study after having read and signed an informed consent form for research purpose.
Author Contributions
All authors contributed to manuscript revision, read and approved the submitted version. CC, MP, LMo, and RC contributed to manuscript revision. CC, MP, LMa, and AA contributed to conception and design of the study. LMa and AA organized the database, performed the statistical analysis, wrote the first draft of the manuscript. MP, TG, and AA contributed acquisition, analysis or interpretation of data.
Funding
This study was funded by the AGING PROJECT—Department of Excellence—Università del Piemonte Orientale.
Conflict of Interest Statement
AA, LMo, TG, and MP were employed by company Biolab Research srl, Novara, Italy.
The remaining authors declare that the research was conducted in the absence of any commercial or financial relationships that could be construed as a potential conflict of interest.
Acknowledgments
We thank Dr. Thomas Fleetwood for proofreading the manuscript.
Supplementary Material
The Supplementary Material for this article can be found online at: https://www.frontiersin.org/articles/10.3389/fimmu.2019.00969/full#supplementary-material
References
1. Srivanitchapoom P, Pitakpatapee Y, Suengtaworn A. Parkinsonian syndromes: a review. Neurol India. (2018) 66:S15–25. doi: 10.4103/0028-3886.226459
2. Comi C, Tondo G. Insights into the protective role of immunity in neurodegenerative disease. Neural Regen Res. (2017) 12:64–5. doi: 10.4103/1673-5374.198980
3. Cappellano G, Carecchio M, Fleetwood T, Magistrelli L, Cantello R, Dianzani U, et al. Immunity and inflammation in neurodegenerative diseases. Am J Neurodegener Dis. (2013) 21:89–107.
4. Liu X, Yamada N, Maruyama W, Osawa T. Formation of dopamine adducts derived from brain polyunsaturated fatty acids: mechanism for Parkinson disease. J Biol Chem. (2008) 283:34887–95. doi: 10.1074/jbc.M805682200
5. Dias V, Junn E, Mouradian JJ. The role of oxidative stress in Parkinson disease. J Parkinsons Dis. (2013) 3:461–91. doi: 10.3233/JPD-130230
6. Cerosimo MG, Raina GB, Pecci C, Pellene A, Calandra Cr, Gutiérrez C, et al. Gastrointestinal manifestations in Parkinson's disease: prevalence and occurrence before motor symptoms. J Neurol. (2013) 260:1332–8. doi: 10.1007/s00415-012-6801-2
7. Comi C, Magistrelli L, Oggioni GD, Carecchio M, Fleetwood T, Cantello R, et al. Peripheral nervous system involvement in Parkinson's disease: evidence and controversies. Parkinsonism Relat Disord. (2014) 20:1329–34. doi: 10.1016/j.parkreldis.2014.10.010
8. McCann H, Cartwright H, Halliday GM. Neuropathology of asynuclein propagation and Braak hypothesis. Mov Disord. (2016) 31:152–60. doi: 10.1002/mds.26421
9. Fasano A, Visanji NP, Liu LW, Lang AE, Pfeiffer RF. Gastrointestinal dysfunction in Parkinson's disease. Lancet Neurol. (2015) 14:625–39. doi: 10.1016/S1474-4422(15)00007-1
10. Felice VD, Quigley EM, Sullivan AM, Gerard AM, O'Keeffe GW, O'Mahony SM. Microbiota-gut-brain signalling in Parkinson's disease: implications for non-motor symptoms. Parkinsonism Relat Disord. (2016) 27:1–8. doi: 10.1016/j.parkreldis.2016.03.012
11. Zheng LF, Song J, Fan RF, Chen QZ, Ren XL, Zhang XL, et al. The role of vagal pathway and gastric dopamine in the rats after a 6-hydroxydopamine microinjection in the substantia nigra. Acta Physiol Oxf. (2014) 211:434–46. doi: 10.1111/apha.12229
12. Forsyth CB, Shannon KM, Kordower JH, Voigt RM, Shaikh M, Jaglin JA, et al. Increased intestinal permeability correlates with sigmoid mucosa alpha-synuclein staining and endotoxin exposure markers in early Parkinson's disease. PLoS ONE. (2011) 6:e28032. doi: 10.1371/journal.pone.0028032
13. Sampson TR, Debelius JW Thron T, Janssen S, Shastri GG, Esra Ilhan Z, et al. Gut microbiota regulate motor deficits and neuroinflammation in a model of Parkinson's disease. Cell. (2016) 167:1469–80. doi: 10.1016/j.cell.2016.11.018
14. Minato T, Maeda T, Fujisawa Y, Tsuji H, Nomonoto K, Ohno K, et al. Progression of Parkinson's disease is associated with gut dysbiosis:two-year follow-up study. PLoS ONE. (2017) 12:e0187307. doi: 10.1371/journal.pone.0187307
15. Keshavarzian A, Green SJ, Engen PA, Voigt RM, Naqib A, Forsyth CB. Colonic bacterial composition in Parkinson's disease. Mov Disord. (2015) 30:1351–60. doi: 10.1002/mds.26307
16. Sheperijans F, Aho V, Pereira PAB, Koskinen K, Paulin L, Pekkonen E, et al. Gut microbiota are related to Parkinson's disease and clinical phenotype. Mov Disord. (2015) 30:350–8. doi: 10.1002/mds.26069
17. Nair AT, Ramachandran V, Jorghee NM, Antony S, Ramalingam G. Gut microbiota dysfunction as reliable non-invasive early diagnosis biomearkers in the pathophysiology of Parkinson's disease: a critical review. J Neurogastroenterol Motil. (2018) 1:30–42. doi: 10.5056/jnm17105
18. Nicola S, Amoruso A, Deidda F, Pane M, Allesina S, Mogna L, et al. Searching for the perfect homeostasis: five strains of Bifidobacterium longum from centenarians have a similar behavior in the production of cytokines. J Clin Gastroenterol. (2016) 50 (Suppl 2):S126–30. doi: 10.1097/MCG.0000000000000678
19. Fujiwara D, Inoue S, Wakabayashi H, Fujii T. The anti-allergic effects of lactic acid bacteria are strain dependent and mediated effects on both Th1/Th2 cytokine expression and balance. Int Arch Allergy Immunol. (2004) 135:205–15. doi: 10.1159/000081305
20. Nowak A, Paliwoda A, Blasiak J. Anti-proliferative, pro-apoptotic and anti-oxidative activity of Lactobacillus and Bifidobacterium strains: a review of mechanisms and therapeutic perspectives. Crit Rev Food Sci Nutr. (2018) 16:1–42. doi: 10.1080/10408398.2018.1494539
21. Kelly B, Blake C, Lennon O. Acute hospital admissions of individuals with a known Parkinson's disease diagnosis in Ireland 2009-2012: a short report. J Parkinson Dis. (2016) 6:709–16. doi: 10.3233/JPD-160839
22. Su CM, Kung CT, Chen FC, Cheng HH, Hsiao SY, Lai YR, et al. Manifestations and outcomes of patients with Parkinson's disease and serious infection in the emergency department. Biomed Res Int. (2018) 17:6014896. doi: 10.1155/2018/6014896
23. Mogna L, Deidda F, Nicola S, Amoruso A, Del Piano M, Mogna G. In vitro inhibition of Klebsiella pneumoniae by Lactobacillus delbrueckii subsp. delbrueckii LDD01 (DSM 22106): an innovative strategy to possibly counteract such infections in humans? J Clin Gastroenterol. (2016) 50 (Suppl 2):S136–9. doi: 10.1097/MCG.0000000000000680
24. Mogna L, Del Piano M, Deidda F, Nicola S, Soattini L, Debiaggi R, et al. Assessment of the in vitro inhibitory activity of specific probiotic bacteria against different Escherichia coli strains. J Clin Gastroenterol. (2012) 46 Suppl:S29–32. doi: 10.1097/MCG.0b013e31826852b7
25. van Kessel SP, Frye AK, El-Gendy AO, Castejon M, Keshavarzian A, van Dijk G, et al. Gut bacterial tyrosine decarboxylases restrict levels of levodopa in the treatment of Parkinson's disease. Nat Commun. (2019) 10:310. doi: 10.1038/s41467-019-08294-y
26. Perez M, Calles-Enríquez M, Nes I, Martin MC, Fernandez M, Ladero V, et al. Tyramine biosynthesis is transcriptionally induced at low pH and improves the fitness of Enterococcus faecalis in acidic environments. Appl Microbiol Biotechnol. (2015) 99:3547–58. doi: 10.1007/s00253-014-6301-7
27. Perez M, Ladero V, Del Rio B, Redruello B, de Jong A, Kuipers OP, et al. Transcriptome profiling of TDC cluster deletion mutant of Enterococcus faecalis V583. Genom Data. (2016) 9:67–9. doi: 10.1016/j.gdata.2016.06.012
28. Postuma RB, Berg D, Stern M, Poewe W, Olanow CW, Oertel, et al. MDS clinical diagnostic criteria for Parkinson's disease. Mov Disord. (2015) 30:1591–601. doi: 10.1002/mds.26424
29. Goetz CG, Poewe W, Rascol O, Sampaio C, Stebbins GT, Counsell C, et al. Movement disorder. society task force on rating scales for Parkinson's disease. Movement disorder society task force report on the Hoehn and Yahr staging scale: status and recommendations. Mov Disord. (2004) 19:1020. doi: 10.1002/mds.20213
30. Goetz CG, Tilley BC, Shaftman SR, Stebbins GT, Fahn S, Martinez-Martin P, et al. Movement disorder society UPDRS revision task force. Movement disorder society sponsored revision of the unified Parkinson's disease rating scale (MDSUPDRS): scale presentation and clinimetric testing results. Mov Disord. (2008) 23:2129. doi: 10.1002/mds.22340
31. Tomlinson CL, Stowe R, Patel S, Rick C, Gray R, Clarke CE. Systematic review of levodopa dose equivalency reporting in Parkinson's disease. Mov Disord. (2010) 25:2649–53. doi: 10.1002/mds.23429
32. Tharmaraj N, Shah NP. Antimicrobial effects of probiotics against selected pathogenic and spoilage bacteria in cheese-based dips. Int Food Res J. (2009) 16:261–76.
33. Barichella M, Pacchetti C, Bolliri C, Cassani E, Iorio L, Pusani C, et al. Probiotics and prebiotic fiber for constipation associated with Parkinson's disease. Neurology. (2016) 87:1274–80. doi: 10.1212/WNL.0000000000003127
34. Del Piano M, Carmagnola S, Anderloni A, Andorno S, Ballarè M, Balzarini M, et al. The use of probiotics in healthy volunteers with evacuation disorders and hard stools: a double-blind, randomized, placebo-controlled study. J Clin Gastroenterol. (2010) 44 (Suppl 1):S30–4. doi: 10.1097/MCG.0b013e3181ee31c3
35. Del Piano M, Montino F, Carmagnola S, Anderloni AM, Orsello E, Garello F, et al. The use of probiotics in the treatment of constipation in the elderly. Cibus. (2005) 1:23–30.
36. Nimgampalle M, Kuna Y. Anti-Alzheimer properties of probiotic, Lactobacillus plantarum MTCC 1325 in Alzheimer's disease induced albino rats. J Clin Diagn Res. (2017) 11:KC01–KC05. doi: 10.7860/JCDR/2017/26106.10428
37. Akbari E, Asemi Z, Daneshvar Kakhaki R, Bahmani F, Kouchaki E, Tamtaji OR. Effect of probiotic supplementation on cognitive function and metabolic status in Alzheimer's disease: a randomized, double-blind and controlled trial. Front Aging Neurosci. (2016) 8:256. doi: 10.3389/fnagi.2016.00256
38. Frank MG, Fonken LK, Watkins LR, Maier SF, Lowry CA. Could probiotics be used to mitigate neuroinflammation? ACS Chem Neurosci. (2018) 10:13–5. doi: 10.1021/acschemneuro.8b00386
39. Mazzini L, Mogna L, De Marchi F, Amoruso A, Pane M, Aloisio I, et al Potential role of gut microbiota in ALS pathogenesis and possible novel therapeutic strategies. J Clin Gastroenterol. (2018) 52 (Suppl 1):S68–70. doi: 10.1097/MCG.0000000000001042
40. Skvarc DR, Berk M, Byrne LK, Dean OM, Dodd S, Lewis M, et al. Post-operative cognitive dysfunction: an exploration of the inflammatory hypothesis and novel therapies. Neurosci Biobehav Rev. (2018) 84:116–33. doi: 10.1016/j.neubiorev.2017.11.011
41. Reber SO, Siebler PH, Donner NC, Morton JT, Smith DG, Kopelman JM, et al. Immunization with a heat-killed preparation of the environmental bacterium Mycobacterium vaccae promotes stress resilience in mice. Proc Natl Acad Sci USA. (2016) 113:E3130–9. doi: 10.1073/pnas.1600324113
42. Sommer A, Winner B, Prots I. The Trojan horse - neuroinflammatory impact of T cells in neurodegenerative diseases. Mol Neurodegener. (2017) 12:78. doi: 10.1186/s13024-017-0222-8
43. Alberio T, Pippione AC, Zibetti M, Olgiati S, Cecconi D, Comi C, et al. Discovery and verification of panels of T-lymphocyte proteins as biomarkers of Parkinson's disease. Sci Rep. (2012) 2:953. doi: 10.1038/srep00953
44. Sulzer D, Alcalay RN, Garretti F, Cote L, Kanter E, Agin-Liebes J, et al. T cells from patients with Parkinson's disease recognize α-synuclein peptides. Nature. (2017) 546:656–61. doi: 10.1038/nature22815
45. Kustrimovic N, Comi C, Magistrelli L, Rasini E, Legnaro M, Bombelli R, et al. Parkinson's disease patients have a complex phenotypic and functional Th1 bias: cross-sectional studies of Th1/Th17/Th2 and Treg in drug-naïve and drug-treated patients. J Neuroinflamm. (2018) 15:205. doi: 10.1186/s12974-018-1248-8
46. Houser MC, Chang J, Factor SA, Molho ES, Zabetian CP, Hill-Burns EM, et al. Stool immune profiles evince gastrointestinal inflammation in Parkinson's disease. Mov Disord. (2018) 33:793–804. doi: 10.1002/mds.27326
47. Storelli E, Cassina N, Rasini E, Marino F, Cosentino M. Do Th17 Lymphocytes and IL-17 contribute to Parkinson's disease? A systematic review of available evidence. Front Neurol. (2019) 10:13. doi: 10.3389/fneur.2019.00013
48. Sommer A, Maxreiter F, Krach F, Fadler T, Grosch J, Maroni M, et al. Th17 lymphocytes induce neuronal cell death in a human iPSC-based model of Parkinson's disease. Cell Stem Cell. (2018) 23:123–31. doi: 10.1016/j.stem.2018.06.015
49. Ivanov II, Atarashi K, Manel N, Brodie EL, Shima T, Karaoz U, et al. Induction of intestinal Th17 cells by segmented filamentous bacteria. Cell. (2009) 139:485–98. doi: 10.1016/j.cell.2009.09.033
50. Fransen F, van Beek AA, Borghuis T, Meijer B, Hugenholtz F, van der Gaast-de Jongh C, et al. The impact of gut microbiota on gender-specific differences in immunity. Front Immunol. (2017) 8:754. doi: 10.3389/fimmu.2017.00754
51. Kustrimovic N, Rasini E, Legnaro M, Bombelli R, Aleksic I, Blandini F, et al. Dopaminergic receptors on CD4+ T naive and memory lymphocytes correlate with motor impairment in patients with Parkinson's disease. Sci Rep. (2016) 6:33738. doi: 10.1038/srep33738
52. Alberio T, Pippione AC, Comi C, Olgiati S, Cecconi D, Zibetti M, et al. Dopaminergic therapies modulate the T-CELL proteome of patients with Parkinson's disease. IUBMB Life. (2012) 64:846–52. doi: 10.1002/iub.1073
Keywords: probiotic, neuroinflammation, Parkinson's disease (PD), oxidative stress, cytokines
Citation: Magistrelli L, Amoruso A, Mogna L, Graziano T, Cantello R, Pane M and Comi C (2019) Probiotics May Have Beneficial Effects in Parkinson's Disease: In vitro Evidence. Front. Immunol. 10:969. doi: 10.3389/fimmu.2019.00969
Received: 06 September 2018; Accepted: 15 April 2019;
Published: 07 May 2019.
Edited by:
Letizia Leocani, San Raffaele Hospital (IRCCS), ItalyReviewed by:
Clara Ballerini, University of Florence, ItalyMahmoud Salami, Kashan University of Medical Sciences, Iran
Copyright © 2019 Magistrelli, Amoruso, Mogna, Graziano, Cantello, Pane and Comi. This is an open-access article distributed under the terms of the Creative Commons Attribution License (CC BY). The use, distribution or reproduction in other forums is permitted, provided the original author(s) and the copyright owner(s) are credited and that the original publication in this journal is cited, in accordance with accepted academic practice. No use, distribution or reproduction is permitted which does not comply with these terms.
*Correspondence: Cristoforo Comi, comi@med.uniupo.it
orcid.org/0000-0002-6862-9468