- 1Shaanxi Key Laboratory of Brain Disorders, Institute of Basic Medical Sciences, Xi’an Medical University, Xi’an, China
- 2Key Lab for Thin Film and Microfabrication Technology, Department of Instrument Science and Engineering, School of Electronic Information and Electronic Engineering, Institute of Nano Biomedicine and Engineering, Ministry of Education, Shanghai Jiao Tong University, Shanghai, China
Myeloid-derived suppressor cells (MDSCs) are a heterogeneous group of myeloid progenitor and precursor cells at different stages of differentiation, which play an important role in tumor immunosuppression. Glioma is the most common and deadliest primary malignant tumor of the brain, and ample evidence supports key contributions of MDSCs to the immunosuppressive tumor microenvironment, which is a key factor stimulating glioma progression. In this review, we summarize the source and characterization of MDSCs, discuss their immunosuppressive functions, and current approaches that target MDSCs for tumor control. Overall, the review provides insights into the roles of MDSC immunosuppression in the glioma microenvironment and suggests that MDSC control is a powerful cellular therapeutic target for currently incurable glioma tumors.
Introduction
Glioma is the most common primary malignant tumor of the brain, and is characterized by high proliferation rates, and migration and invasion abilities. Comprehensive treatment includes surgical resection combined with radiotherapy and chemotherapy; however, such approaches are generally ineffective. The median survival time of patients with the most malignant glioma is approximately 1–2 years despite aggressive therapy, including surgery, radiotherapy and chemotherapy (1). Hence, development of more effective treatments is urgently required. Recently, immunotherapeutic approaches have been developed for cancer therapy, with exciting progress for some cancers; however, there are specific challenges for glioma immunotherapy (2). Many factors may contribute to these difficulties, including the blood-brain barrier (BBB), antigenic and genetic heterogeneity, and the tumor microenvironment (TME).
The Immune Microenvironment in Glioma
The TME is the dynamic milieu of a tumor, including the extracellular matrix (ECM), signaling molecules, stromal cells, and immune cells, which influence the growth and evolution of tumor cells. The glioma microenvironment differs from other solid tumors, because gliomas are located in the brain, which is an immune privileged organ, protected by the BBB, where cells of the peripheral immune system are prevented from entering under normal conditions. However, inflammation and tumor growth can disrupt the BBB (3, 4). The strong immunosuppressive TME of gliomas has led them to be referred to in the literature as “cold tumors” (5). Many studies have demonstrated that cytokines, chemokines, and regulatory immune-suppressive cells (6, 7), such as TGF-β, IL-10, prostaglandin E2, NKT cells, T/B regulatory cells (T/Breg), tumor-associated macrophages/microglia (TAMs), and myeloid-derived suppressor cells (MDSCs) (8), create a specific immunosuppressive TME, which is important for anti-tumor responses and glioma progression. All these proved that MDSCs are powerful inhibitors of anti-tumor immune responses in glioma, hence targeting MDSCs will be beneficial for patients with these tumors. Here, we review the phenotypic characteristics of MDSCs, as well as their mechanisms of development and activation, and strategies for MDSC-depletion.
Source and Characterization of MDSCS
Myeloid-derived suppressor cells are a heterogeneous group of myeloid progenitor and precursor cells, comprising macrophages, granulocytes, and dendritic cells (DCs) at different stages of differentiation. In healthy individuals, immature myeloid cells (IMCs) quickly differentiate into mature macrophages, granulocytes, or DCs (9); however, under pathological conditions, such as in patients with cancer, chronic inflammatory conditions prevent IMC differentiation into mature myeloid cells, resulting in MDSC accumulation (10). In cancer patients, MDSCs are defined as cells that co-express the myeloid differentiation markers, CD11b and CD33, while lacking markers of mature lymphoid and myeloid cells, such as the MHC class II molecule, HLA-DR (11). There are three main types of MDSCs: granulocytic or polymorphic nuclear MDSCs (G/PMN-MDSCs), mononuclear MDSCs (M-MDSC), and early-stage MDSCs (eMDSCs). In humans, M-MDSCs are characterized as CD11b+CD14+CD33+HLA-DRlow/−CD15–, PMN-MDSCs as CD11b+CD14–CD33+HLA-DRlow/−CD15+ (or CD66+), and eMDSCs as Lin– (i.e., CD3–, CD14–, CD15–, CD19–, CD56–, HLA-DR–, and CD33+. In mouse, MDSCs are defined as cells that co-express CD11b and Gr-1 (12), where Gr-1 is a cell surface antigen, and can be divided into Ly6C and Ly6G. Hence, M-MDSCs are characterized as CD11b+, Ly6C+, Ly6G–, according to their relative expression levels of Ly6G and Ly6C, whereas PMN-MDSCs are CD11b+, Ly6C–, Ly6G+, and eMDSCs have yet to be defined.
In humans, M-MDSCs can be distinguished from monocytes base on the expression of the MHC class II molecule HLA-DR (13). The PMN-MDSCs and neutrophils can be separated on the base of their low-density properties when using a standard Ficoll gradient. Recently, Condamine T. et al. identified that lectin-type oxidized low-density lipoprotein receptor-1 (LOX-1) as a potential marker of human PMN-MDSC (12). Moreover, the M-MDSCs and PMN-MDSCs also display specific cell-death-associated program. Haverkamp et al. demonstrated that the anti-apoptotic molecules c-FLIP [cellular FLICE (FADD-like IL-1β-converting enzyme)-inhibitory protein] is required for monocytic MDSC development (14). Fiore et al. reported that c-FLIP can activate many transcription of several immunosuppression-related genes in part through nuclear factor kappa-light-chain-enhancer of activated B cells (NFκB) activation, and then plays an important role in re-programming monocytes into MDSCs without affecting cell survival, but not affects the conversion from neutrophils into PMN-MDSCs (15).
As we known, the basic functional characteristic of MDSCs is suppressing immune cells, mainly T-cells and lesser B and NK cells (16). Groth et al. reported that M-MDSCs showed a higher immunosuppressive capacity compared to PMN-MDSC, which directly suppress T cell function or induce the generation of Treg cells by secreting TGF-β and IL-10 (17). Trovato et al. showed that M-MDSCs are the most potent myeloid subset to halt T cell proliferation in patients with pancreatic ductal carcinoma (18). Moreover, plasticity and function of MDSCs are strictly regulated by the activation of specific molecular pathways (13), preferentially driven by signal transducer and activator of transcription 3 (STAT3), and CCAAT/enhancer binding proteinβ (C/EBPβ). Upregulation of STAT3 is a hallmark of MDSCs, which plays a central role in regulating MDSC expansion and tolerogenicity (19). Upregulation of C/EBP-β is also associated with the expansion of MDSCs populations (19). Furthermore, C/EBP-β controls the controls the immunosuppressive activity of MDSC through regulating the expression of arginase (ARG1) and inducible nitric oxide synthase (iNOS) (20, 21).
Myeloid-Derived Suppressor Cells in Glioma
Glioma is a central nervous system (CNS) tumor, and the immunosuppressive TME of glioma likely depends on depressed T cell function, through accumulation of immunosuppressive leukocytes, such as MDSCs and regulatory T-cells (Tregs). MDSCs number is usually relatively larger in the spleen, blood, and tumors, and correlates with the tumor stage and chemotherapy response (22). In glioma patients, the intratumoral density of MDSCs also increases during glioma progression and correlates with patient survival (23). Gielen et al. reported that MDSCs increased in blood samples from patients with glioma compared with healthy donors. (24) and Raychaudhuri et al. found that patients with glioblastoma multiforme (GBM) had elevated levels of MDSCs in their peripheral blood (25), and the majority of the MDSCs were neutrophilic CD15+ CD14– (PMN-MDSC, 82%), followed by lineage-negative (eMDSCs, 15%), and monocytic (M-MDSC, 3%). Gielen et al. showed that MDSCs were significantly increased among peripheral blood mononuclear cells from patients with GBM, but only slightly and non-significantly increased in patients with grade II or III glioma, and MDSCs found in tumor material are almost exclusively CD15-positive (24). Dubinski et al. also revealed that the frequency of CD14highCD15pos M-MDSCs and CD14lowCD15pos PMN-MDSCs was significantly higher in peripheral blood of GBM patients compared with healthy donors (26). These studies indicate the expansion of PMN and M-MDSC subtypes in patients with glioma, and M-MDSCs were the most abundant MDSC subpopulation in the blood, while PMN-MDSCs were dominant in glioma tissue. Further, Raychaudhuri et al. found that MDSCs represented 5.4 ± 1.8% of total cells in human GBM tumors; the majority were lineage negative (CD14–CD15–) eMDSCs, followed by PMN-MDSC (CD15+CD14–), and M-MDSC (CD15–CD14+) subtypes. In murine GBM tumors, MDSCs comprised 8.06 ± 0.78% of total cells, of which more were M-MDSC than G-MDSC (27). Hence, it is established that the types of MDSCs differ in tumor tissues compared with the circulation (blood), while detection of the predominant MDSC type in glioma requires further research, since the TME may impact MDSC migration or differentiation.
MDSC Expansion and Recruitment
Myeloid-derived suppressor cells are present at relatively low levels under normal conditions, but are recruited and differentiate in various pathologic situations, such as during autoimmune encephalitis, bacterial infections, and tumors. Jennifer et al. reported that the healthy donor human CD14+ monocytes could acquire MDSC phenotypes (reduced CD14 but not CD11b expression) and immunosuppressive properties (increased immunosuppressive interleukin-10, transforming growth factor-β (TGF-β), and B7-H1 expression) when cultured with human glioblastoma cell lines (28). Kumar et al. also found that normal human monocytes could become M-MDSCs when cultured in glioma-conditioned media under hypoxic conditions (29).
A variety of inflammatory mediators can induce MDSC expression and recruitment (Figure 1). Jiang et al. reported that interleukin-6 (IL-6) can promote the amplification and immunosuppressive function of breast cancer MDSCs in vitro and in vivo, by inducing dysfunction of SOCS3 and activation of signal transducer and transcription activator 3 (STAT3)-signaling (30). Gielen et al. reported that STAT3 also regulates MDSC amplification through expression of S100A8/9 in gliomas (31). Tumor-derived granulocyte macrophage colony-stimulating factor (GM-CSF) has an important role in the expansion of MDSCs, both in vitro and in vivo (32–34). Importantly, Marigo et al. found that GM-CSF and IL-6 allowed rapid and efficient generation of MDSCs from precursors present in mouse and human bone marrow (35). Moreover, various other tumor-derived factors, such as prostaglandin-E2 (PGE2) (36), IL-10 (37), VEGF (38), and TGF-β (39–41), have been suggested to contribute to the induction and expansion of MDSCs (36), and these factors are also derived from glioma cells. Albulescu et al. showed that IL-6, IL-1β, TNF-α, IL-10, VEGF, FGF-2, IL-8, IL-2, and GM-CSF were upregulated in gliomas (42). Further, many studies have shown that PGE2 is overexpressed in glioma (43). Together, these data suggest that glioma cells can stimulate the expansion of MDSCs by secreting numerous well-studied factors (IL-6, IL-10, VEGF, PGE-2, GM-CSF, and TGF-β2).
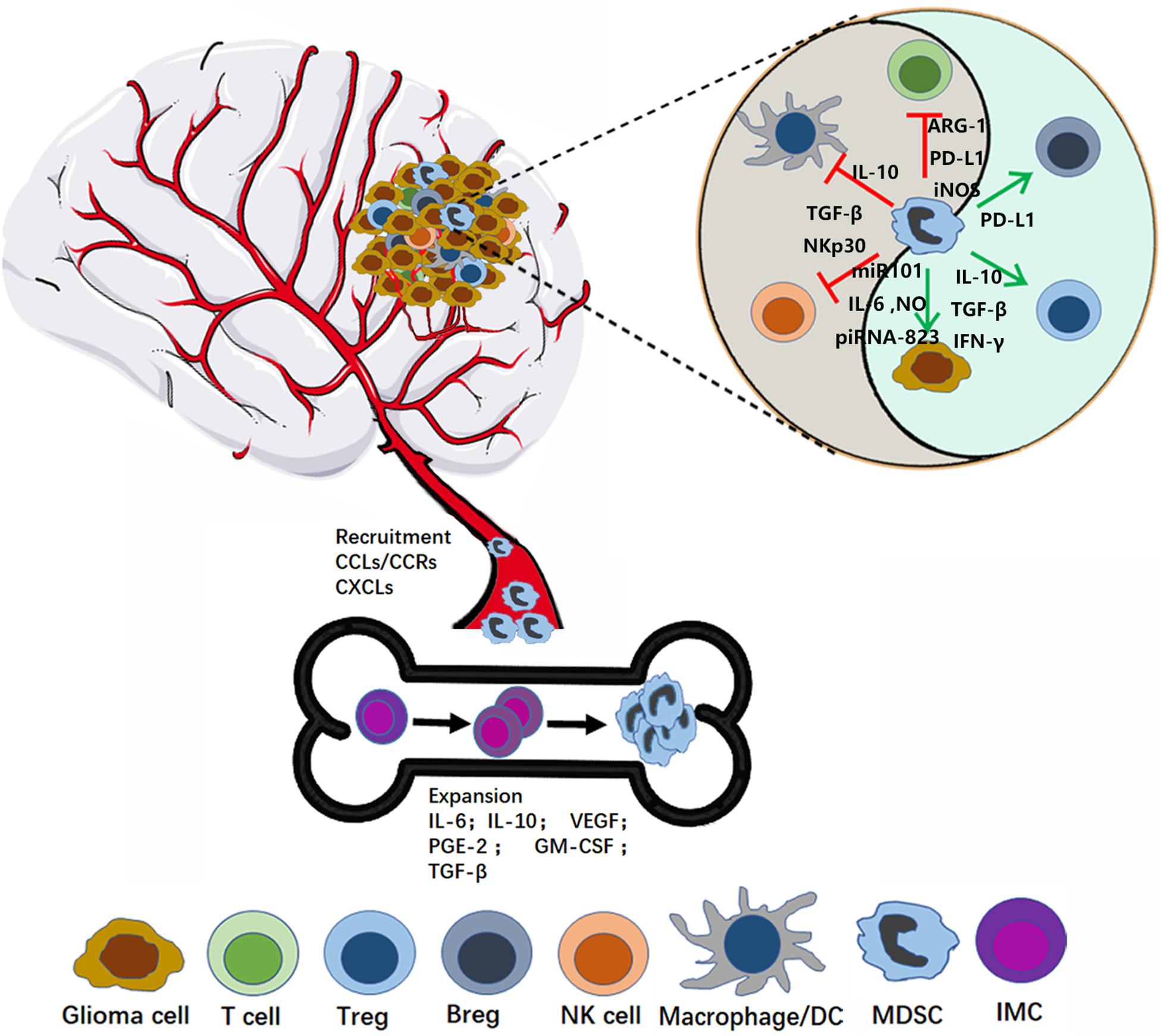
Figure 1. MDSC recruitment and activation in glioma microenvironment. In the bone marrow, MDSCs originate from immature myeloid cells (IMC), and then expand and migrate to the glioma site through the interaction between CCR and respective chemokines (CCL). In the tumor microenvironment, MDSCs play immunosuppression role by inhibiting the anti-tumor activity of cytotoxic T cells, suppressing the NK, Macrophage and Dendritic cells (DCs) function, expansion, and promoting Tregs and Bregs.
Chemokines are a family of 8–14 kDa chemoattractant cytokines secreted by cells, which have important roles in regulating cells trafficking (44). Multiple chemokines are involved in recruiting MDSCs in different cancer models (45–47). Chemokine (C-C motif) ligand (CCL) 2 and its receptors, chemokine (C-C motif) receptor (CCR) 2, 4, and 5, have key roles in attraction of M-MDSCs (48, 49). In particular, microenvironment-derived CCL-2 can recruit MDSCs to cancer sites via CCL2-CCR2 interaction (50). Furthermore, Vakilian et al. reviewed the CCL2/CCR2 signaling pathway in glioma and found that it plays a dual role in mediating early tumor immunosurveillance and sustaining tumor growth and progression (51). IL-8 (CXCL8) is a pro-inflammatory chemokine produced by many cell types, including glioma, and can promote MDSC trafficking into the tumor microenvironment through the IL-8/IL-8R axis (52, 53). CXC chemokine ligand 2 (CXCL2), also referred to as macrophage inflammatory protein-2 (MIP-2), has a pivotal role in recruiting MDSCs to tumor stroma (54). Kammerer et al. found that CXCL2 was an immune response gene in glioma; however, whether expression of this gene is altered in tumor cells or cells in the TME was not determined (55). Interestingly, Bruyère et al. found that inhibition of CXCL2 expression in Hs683 glioma cells using siRNA markedly impaired cell proliferation (56). Overall, these results suggest that high levels of CXCL2 expression are important for glioma progression; however, the mechanism regulating MDSC recruitment requires clarification.
MDSC-Induced Immunosuppression in Gliomas
Myeloid-derived suppressor cells indisputably induce immunosuppression and promote tumor development. Numerous mechanisms by which MDSCs inhibit immune responses have been reported, inducing inhibition of the anti-tumor activity of cytotoxic T cells, suppression of NK cell, macrophage, and dendritic cell (DC) function, and induction of Tregs and Bregs. In this section, we summarize the function of MDSCs in glioma development in detail (Figure 1).
Inhibition of T Cell Function
T cells, particularly cytotoxic T cells, have important roles in tumor-inhibition, and there is substantial evidence that MDSCs can inhibit T cell function via multiple mechanisms. MDSCs are well known to induce oxidative stress by secreting ROS and nitrogen species (RNS). The main pathways of ROS production are related to the NADPH oxidases (NOX) (57), and RNS are produced by the activation of ARG1 or iNOS (NOS2) in different MDSC subsets (58). These reactive species can inhibit T cell growth through interfering with the expression of the CD3ζ chain and induction of apoptosis (59, 60). Moreover, intratumoral RNS production can inhibit the T cell migration by inducing the CCL2 chemokine nitration (61). MDSC can also deplete metabolites and factors which are critical for T cell functions. MDSCs deplete L-arginine which inhibits T cell growth and induce apoptosis from the microenvironment by enhancing the activity of ARG1, inducible iNOS and increase the uptake mediated by the CAT-2B transporter (62, 63). Tryptophan (Trp)-catabolizing enzymes such as Indoleamine 2,3-dioxygenase (IDO) have been shown to be involved in tumor immune escape. Upregulation of IDO1 in MDSC and tumor cells leads to Trp depletion that impairs cytotoxic T cell responses and survival (64–66). HIF1-α is produced in response to hypoxia in the TME and can induce PD-L1 expression on MDSCs. Further, blockade of PD-L1 can inhibit MDSC-mediated T cell suppression, through modulating MDSC cytokine production (67).
Inhibiting NK Cell Function
NK cells are a critical component of innate immunity and can eradicate gliomas without T cell cooperation (68). Fortin et al. found that the MDSCs can suppress the function of NK cells via reactive oxygen species (ROS) production (69), while Li et al. showed that membrane-bound TGF-β1 on MDSCs can induce NK cell anergy (70). Further, Hoechst et al. reported that MDSCs suppress NK cell cytotoxicity and cytokine release through contact with the NK cell receptor, NKp30 (71). Bruno et al. recently reviewed the interactions of MDSCs and NK cells and determined that cross-talk between these types of cells can impact tumor progression and angiogenesis (72).
Inhibiting Macrophage and DC Function
Microglia/macrophages are among the most common cells in brain tumors (73), and MDSCs can modulate functions of macrophages (74). Sinha et al. proved that cross-talk between MDSCs and macrophages skews macrophages toward an M2 phenotype by cell-cell contact, and decreasethe production of IL-12 of macrophage (75). The downregulation of IL-12 is further exacerbated by the macrophages themselves, because the production of IL-10 of macrophages is also promoted by MDSCs (76). Interestingly, Pinton et al. found that the bone marrow-derived macrophages (BMDM) exerted a strong immunosuppression in center of the glioma, but brain-resident microglial cells (MG) showed little or no suppression (77). So, the relationship between the MDSC and macrophages need further research in tumor especially glioma. DCs are the most powerful antigen-presenting cells and a DC vaccine has potential to improve clinical outcomes of patients with glioma (78, 79). Hu et al. proved that MDSCs can inhibit IL-12 production and suppress T cell stimulation of DCs through IL-10 production (80). These results suggest that MDSCs can modulate the functions of macrophages and DCs through different mechanisms.
Treg Expansion and Differentiation
Tregs are suppressor T cells, which can inhibit the induction and proliferation of cytokine-secreting effector T cells (81). Induction of Treg activity is important for the evasion of immunosurveillance by malignant gliomas and correlates with glioma progression (82–84). Huang et al. found that MDSCs can induce Treg generation, dependent on soluble factors, such as IL-10, TGF-β, and IFN-γ (85, 86). In addition, Hoechst et al. showed that MDCSs can catalyze the trans-differentiation of Th17 cells into Tregs, dependent on MDSC-derived TGF-β and retinoic acid (87). These data demonstrate that MDSCs can regulate Treg expansion and induce Th17 cells to differentiate into Tregs.
Promotion of Immunosuppressive B Cells
Regulatory B cells (Bregs) are a population of immunosuppressive cells that support immunological tolerance (88). Recently, glioma-infiltrating B cells were shown to have strong immune suppressor functions in suppressing CD8+ T cells and inducing Tregs (89). Lee-Chang et al. found that MDSCs produced numerous microvesicles (MV) containing PD-L1. Bregs can take up these MVs via receptor-mediated endocytosis, followed by endocytic recycling toward the plasma membrane. These results indicate that MDSCs can promote Breg-mediated immunosuppressive functions, at least in part via transfer of membrane-bound PD-L1.
Besides, MDSC can also directly support tumor (or cancer stem cells) growth through impacting on angiogenesis, invasion and metastasis, and promote cancer cell stemeness (74). MDSCs can produce angiogenic factors such as VEGF and basic fibroblast growth factor (bFGF) to promote tumor angiogenesis (90, 91). Moreover, Li et al. even found that MDSCs directly incorporated into tumor endothelium through acquiring endothelial cell (EC) properties (91). Furthermore, MDSCs promote tumor invasion and metastasis by two mechanisms: (i) increasing the production of multiple matrix metalloproteinases (MMPs), which play an important role in matrix degradation, and chemokines to create a pre-metastatic environment, and (ii) fusing with tumor cells to promote the metastatic process (92, 93). Several studies showed that MDSCs promote cancer cell stemness through inducing miRNA (94), production of IL-6 and NO (95), inducing piRNA-823 expression and DNMT3B activation (96) (Figure 1).
Targeting MDSCS in Glioma Therapy
Myeloid-derived suppressor cells are established as a central immunosuppressive factor, which promotes tumor progression. Therefore, control of the number and/or function of MDSCs would be a powerful anti-tumor therapy. Below, we summarize current targeting approaches for MDSC control (Figure 2).
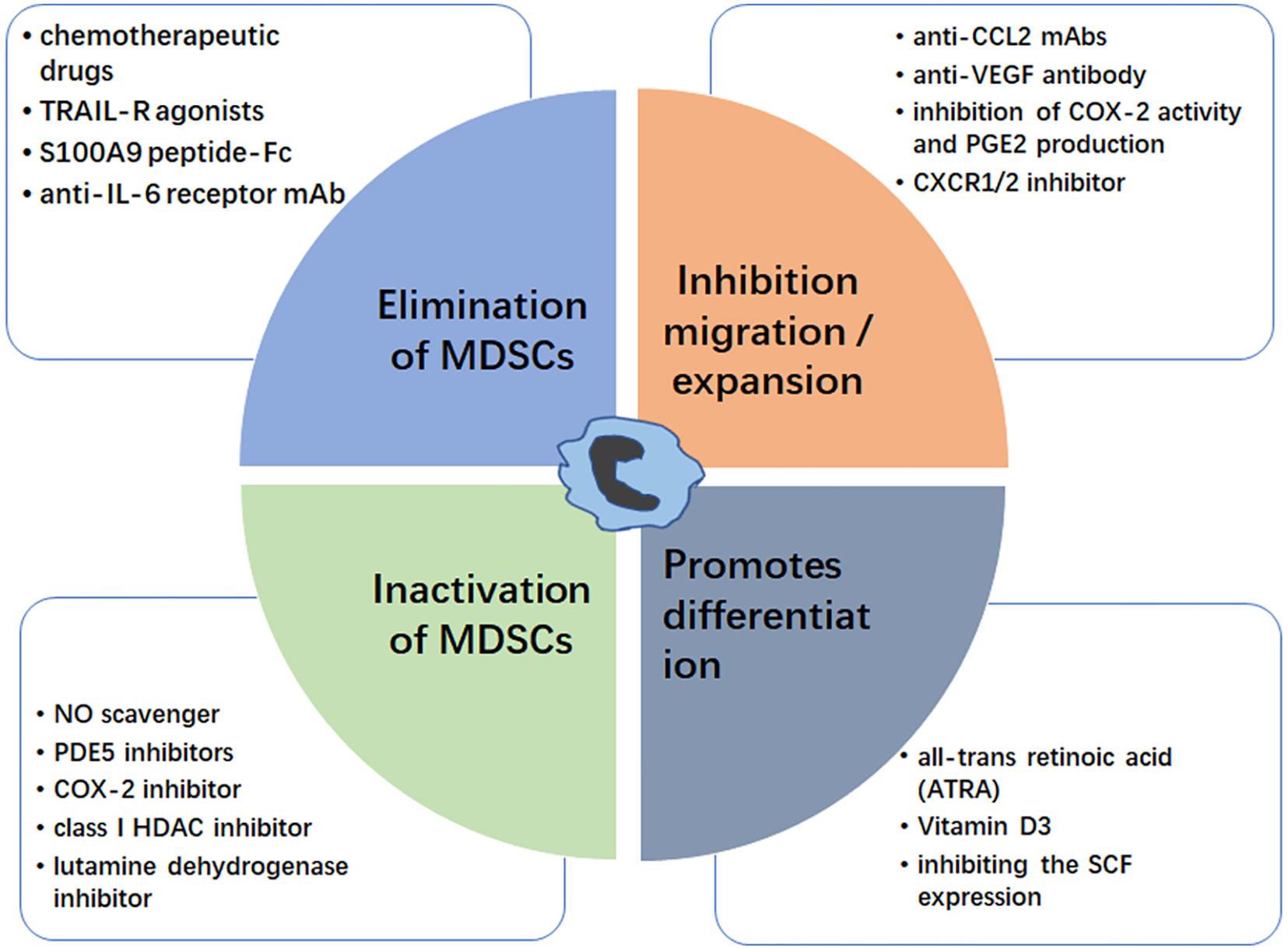
Figure 2. Strategies for targeting MDSC. The MDSC modulation could be achieved by elimination of MDSCs (blue box), inhibition of MDSC migration/expansion (orange box), inactivation of MDSCs (green box), and promoting MDSC differentiation (gray box). Examples for each therapeutic approach are shown.
Elimination of MDSCs
Elimination of MDSCs inhibits tumor progression by enhancing antitumor responses, and there are many reports that chemotherapy drugs can eliminate MDSCs. Suzuki et al. first proved that the chemotherapeutic drug, gemcitabine, can specifically reduce MDSCs, with no significant reductions in other immune cells, such as T cells, B cells, macrophages, or NK cells, in tumor-bearing animals (97). This immunomodulating capacity of gemcitabine could be useful to treat glioma (98). Otvos et al. showed that gemcitabine and 5-FU were selectively cytotoxic to MDSCs, with 5-FU showing greater efficacy in depleting MDSCs and inducing MDSC apoptosis in vivo and in vitro (99). Ugel et al. proved that gemcitabine and 5-FU mainly target M-MDSCs (100). Furthermore, low dose 5-FU can selectively deplete MDSCs, resulting in prolonged survival in a glioma mouse model (101). Recently, David et al. found that the orally bioavailable 5-FU prodrug, capecitabine in combination with bevacizumab can reduce the circulating levels of MDSC in GBM patients (102). Many studies support a role for NF-related apoptosis-induced ligand receptors (TRAIL-Rs) act as potential targets for selective elimination of MDSCs (103). Dominguez et al. proved that DS-8273a, an agonistic TRAIL-R2 (DR5) antibody, could eliminate MDSCs without affecting lymphoid or mature myeloid cells (104), and Nagane et al. found that anti-DR5 mAb treatments significantly suppressed growth of subcutaneous glioma xenografts until complete regression (105). Murat et al. found that glioma patients with high expression of S100A8 and S100A9 are related with short survival (106). Recently, Qin et al. proved that S100A9 peptide-Fc fusion (pepti-body) reagents can deplete blood and splenic MDSCs in mouse tumor models (107, 108). Further, many researches proved that anti IL-6 therapy showed potential benefits for treating various human cancers including glioma (109, 110), Sumida et al. found that an anti-IL-6 receptor monoclonal antibody (mAb) could eliminate MDSCs and inhibit tumor growth by enhancing T-cell responses, and that its therapeutic effect was enhanced by combination with gemcitabine (111). Together, these data suggest that MDSCs can be directly depleted using various agents, including chemotherapy drugs, peptides, and mAbs; however, the mechanisms underlying MDSC elimination require further elucidation.
Inhibition of MDSC Migration and Expansion
Inhibiting MDSC migration and expansion is another strategy for tumor therapy. Glioma cells are known to secrete factors important for MDSC migration and expansion. Zhu et al. proved that systemic administration of neutralizing anti-CCL2 mAbs can block recruitment and decrease the number of MDSCs in the TME, providing significant survival benefits in mouse GL261 glioma and human U87 glioma xenograft models (112). Chen et al. reported that anti-VEGF antibody can effectively decrease the recruitment of MDSCs to tumor tissue (113), while anti-VEGF treatment has been proposed to enhance the survival and quality of life in glioma patients (114, 115). Many studies proved the protective effect of COX-2 inhibitors on glioma (116, 117), and the use of non-steroidal anti-inflammatory drugs was significantly associated with a lower risk of glioma (118, 119). Obermajer et al. demonstrated that inhibition of cyclooxygenase (COX-2) activity and PGE2 production can reduce the accumulation of human MDSCs in the ovarian cancer environment (45, 120). Sinha et al. proved that the COX-2 inhibitor, SC58236, delays primary tumor growth and reduces MDSC accumulation in spontaneously metastatic BALB/c-derived 4T1 mammary carcinoma mouse (121). The chemokine receptor CXCR2 is a receptor of CXCL2 which was highly expressed in PMN-MDSC, andplay an important role in MDSCs recruitment (122). SX-682, a CXCR1/2 inhibitor, could block tumor MDSC recruitment and enhance T cell activation and anti-tumor immunity through various forms of immunotherapy (123, 124).
Inactivation of MDSCs
Once MDSCs migrate into the TME, they suppress anti-tumor responses via various mechanisms, hence impairment of MDSC activity is another strategy to target these cells. Increased production of NO and ROS facilitates MDSCs to suppress CD8+ T cell responses. The ROS scavenger, N-acetylcysteine (NAC), can inhibit MDSC function (108), while the NO scavenger, carboxy-PTIO (C-PTIO), can reduce the immunosuppressive activity of MDSCs and restore impaired CTL function by inhibiting the NO production (125). Activation of iNOS and ARG-1 also has key roles in MDSC activation. Phosphodiesterase-5 (PDE5) inhibitors includesildenafil, tadalafil, and vardenafil, they are emerged as a promising approach to inhibit proliferation, motility and invasion of certain cancer cells including glioma (126). These inhibitors are reported to suppress both iNOS and ARG-1 activities in MDSC, thereby decrease MDSC immunosuppressive functions (127, 128). Moreover, COX-2 is required for the production of PGE2 (129) and correlates positively with ARG-1 expression in MDSCs (130); hence, COX-2 inhibitors, such as celecoxib or acetylsalicylic acid, can suppress gliomagenesis by MDSC activation (131, 132). Entinostat (MS-275), a class I HDAC inhibitor in pre-clinical testing for glioblastoma, can inhibit MDSC function and exhibit antitumor effect in murine models of lung and renal cell carcinoma (133, 134).
It has been reported that the glutaminolysis contributes to MDSC function (135), this means glutamate metabolism (maintaining optimal glutamine or glutamate levels) is critical in MDSC-mediated immunosuppression phenomenon. As we know, glutamate is one of the main excitatory neurotransmitters in the central nervous system (CNS) (136). And in glioma microenvironments, the glutamate concentration is 400 times that of normal brain tissue (137). This high glutamate concentration is benefit for glioma cell growth and affects MDSCs function, so nhibition of glutamine metabolism is suggested as an attractive and druggable therapeutic target especially in glioma (138). The glutamine dehydrogenase inhibitor, epigallocatechin gallate (EGCG), which has been proved to induce apoptosis and inhibit proliferation of glioma cell (139), also could reverse MDSC activity (140).
Promotion of MDSC Differentiation
Promotion of MDSC differentiation into mature myeloid cells is a simple approach to inhibition of the immune-suppressive functions of MDSCs. All-trans retinoic acid (ATRA), a natural oxidative metabolite of vitamin A, can promote differentiation of MDSCs into mature myeloid cells (141). Mirza et al. reported that patients with cancer treated with ATRA exhibit improved myeloid/lymphoid DC ratios and immune responses (142). Further, Nefedova et al. found that the ATRA can active ERK1/2 MAPK signaling pathway, which increases the production of glutathione and reduces ROS levels, thus promoting MDSC differentiation (143). Recently, wang et al. proved that ATRA can induce asymmetric division of GSCs from the U87MG cell line, suggesting a therapeutic potential for glioma (144, 145). Similar to ATRA, vitamin D3 can induce immature MDSCs to be differentiated into dendritic and macrophages cells, and then displays anti-proliferative effects in a wide variety of cancer types including glioblastoma multiforme (GBM), (146–148). Moreover, Pan et al. proved that stem-cell factor (SCF), which is secreted by various cancers, including glioma (149), can decrease MDSC differentiation, resulting in MDSC expansion, while inhibiting SCF expression using siRNA can reduce MDSC accumulation (150).
Conclusion and Future Prospects
To date, immunotherapeutic strategies have proven to be effective against various tumors, and researchers are increasingly focusing on immunotherapy for patients with glioma. Although significant progress has been made, some challenges must be overcome (7). There is substantial evidence that MDSCs are important immunosuppressors (11), hence targeting MDSC immune suppressive features has potential as an anti-tumor therapy approach in glioma (151); however, the mechanisms underlying MDSC activity in glioma require further elucidation. In recent years, several strategies have been investigated, such as elimination of MDSCs, inhibition of MDSC migration and expansion, inactivation of MDSCs, and promotion of MDSC differentiation. In summary, control of MDSCs is a powerful cellular therapeutic target for patients with glioma; nevertheless, further basic and clinical research is required in this field.
Author Contributions
YM, NG, and JL conceived and structured the manuscript. JC, ZH, and PJ drafted the manuscript and figure. XG evaluated and reviewed the manuscript structure, ideas, and science. WJ conceived the topic and revised the manuscript. All authors read and approved the final manuscript.
Funding
This work was supported by the National Natural Science Foundation of China (Nos. 81873740 and 81601376), Shaanxi Youth Talents Project, Scientific Research Program funded by the Shaanxi Provincial Education Department (No. 19JS057), Natural Science Basic Research Plan in Shaanxi Province of China (Nos. 2020JQ-879 and 2020JQ-877), Xi’an Medical University’s Key Disciplines of Molecular Immunology and Foundation of Xi’an Medical University’s (Nos. 2018GJFY05, 2018DOC02, 2018PT08, 18NBZD08, and 18NBZD09), and the Youth Innovation Team of Shaanxi Universities.
Conflict of Interest
The authors declare that the research was conducted in the absence of any commercial or financial relationships that could be construed as a potential conflict of interest.
References
1. Davis FG, McCarthy BJ. Current epidemiological trends and surveillance issues in brain tumors. Expert Rev Anticancer Ther. (2001) 3:395–401. doi: 10.1586/14737140.1.3.395
2. Buerki RA, Chheda ZS, Okada H. Immunotherapy of primary brain tumors: facts and hopes. Clin Cancer Res. (2018) 21:5198–205. doi: 10.1158/1078-0432.CCR-17-2769
3. Davies DC. Blood-brain barrier breakdown in septic encephalopathy and brain tumours. J Anat. (2002) 6:639–46. doi: 10.1046/j.1469-7580.2002.00065.x
4. Rascher G, Fischmann A, Kroger S, Duffner F, Grote EH, Wolburg H. Extracellular matrix and the blood-brain barrier in glioblastoma multiforme: spatial segregation of tenascin and agrin. Acta Neuropathol. (2002) 1:85–91. doi: 10.1007/s00401-002-0524-x
5. Tomaszewski W, Sanchez-Perez L, Gajewski TF, Sampson JH. Brain tumor microenvironment and host state: implications for immunotherapy. Clin Cancer Res. (2019) 14:4202–10. doi: 10.1158/1078-0432.CCR-18-1627
6. Perng P, Lim M. Immunosuppressive mechanisms of malignant gliomas: parallels at Non-CNS sites. Front Oncol. (2015) 5:153. doi: 10.3389/fonc.2015.00153
7. Kamran N, Alghamri MS, Nunez FJ, Shah D, Asad AS, Candolfi M, et al. Current state and future prospects of immunotherapy for glioma. Immunotherapy. (2018) 4:317–39. doi: 10.2217/imt-2017-0122
8. Lindau D, Gielen P, Kroesen M, Wesseling P, Adema GJ. The immunosuppressive tumour network: myeloid-derived suppressor cells, regulatory T cells and natural killer T cells. Immunology. (2013) 2:105–15. doi: 10.1111/imm.12036
9. Kusmartsev S, Gabrilovich DI. Inhibition of myeloid cell differentiation in cancer: the role of reactive oxygen species. J Leuk Biol. (2003) 2:186–96. doi: 10.1189/jlb.0103010
10. Meyer C, Sevko A, Ramacher M, Bazhin AV, Falk CS, Osen W, et al. Chronic inflammation promotes myeloid-derived suppressor cell activation blocking antitumor immunity in transgenic mouse melanoma model. Proc Natl Acad Sci USA. (2011) 41:17111–6. doi: 10.1073/pnas.1108121108
11. Gieryng A, Kaminska B. Myeloid-derived suppressor cells in gliomas. Contemp Oncol. (2016) 5:345–51. doi: 10.5114/wo.2016.64592
12. Condamine T, Dominguez GA, Youn JI, Kossenkov AV, Mony S, Alicea-Torres K, et al. Lectin-type oxidized LDL receptor-1 distinguishes population of human polymorphonuclear myeloid-derived suppressor cells in cancer patients. Sci Immunol. (2016) 2:aaf8943. doi: 10.1126/sciimmunol.aaf8943
13. Veglia F, Perego M, Gabrilovich D. Myeloid-derived suppressor cells coming of age. Nat Immunol. (2018) 2:108–19. doi: 10.1038/s41590-017-0022-x
14. Haverkamp JM, Smith AM, Weinlich R, Dillon CP, Qualls JE, Neale G, et al. Myeloid-derived suppressor activity is mediated by monocytic lineages maintained by continuous inhibition of extrinsic and intrinsic death pathways. Immunity. (2014) 6:947–59. doi: 10.1016/j.immuni.2014.10.020
15. Fiore A, Ugel S, De Sanctis F, Sandri S, Fracasso G, Trovato R, et al. Induction of immunosuppressive functions and NF-kappaB by FLIP in monocytes. Nat Commun.. (2018) 1:5193. doi: 10.1038/s41467-018-07654-4
16. Gabrilovich DI. Myeloid-derived suppressor cells. Cancer Immunol Res. (2017) 1:3–8. doi: 10.1158/2326-6066.CIR-16-0297
17. Groth C, Hu X, Weber R, Fleming V, Altevogt P, Utikal J, et al. Immunosuppression mediated by myeloid-derived suppressor cells (MDSCs) during tumour progression. Br J Cancer. (2019) 1:16–25. doi: 10.1038/s41416-018-0333-1
18. Trovato R, Fiore A, Sartori S, Cane S, Giugno R, Cascione L, et al. Immunosuppression by monocytic myeloid-derived suppressor cells in patients with pancreatic ductal carcinoma is orchestrated by STAT3. J Immunother Cancer. (2019) 1:255. doi: 10.1186/s40425-019-0734-6
19. Trovato R, Cane S, Petrova V, Sartoris S, Ugel S, De Sanctis F. The engagement between MDSCs and metastases: partners in crime. Front Oncol. (2020) 10:165. doi: 10.3389/fonc.2020.00165
20. Wang W, Xia X, Mao L, Wang S. The CCAAT/enhancer-binding protein family: its roles in MDSC expansion and function. Front Immunol. (2019) 10:1804. doi: 10.3389/fimmu.2019.01804
21. Haverkamp JM, Crist SA, Elzey BD, Cimen C, Ratliff TL. In vivo suppressive function of myeloid-derived suppressor cells is limited to the inflammatory site. Eur J Immunol. (2011) 3:749–59. doi: 10.1002/eji.201041069
22. Youn JI, Gabrilovich DI. The biology of myeloid-derived suppressor cells: the blessing and the curse of morphological and functional heterogeneity. Eur J Immunol. (2010) 11:2969–75. doi: 10.1002/eji.201040895
23. Gieryng A, Pszczolkowska D, Walentynowicz KA, Rajan WD, Kaminska B. Immune microenvironment of gliomas. Lab Investigat J Tech Methods Pathol. (2017) 5:498–518. doi: 10.1038/labinvest.2017.19
24. Gielen PR, Schulte BM, Kers-Rebel, Verrijp K, Petersen-Baltussen HM, Laan M, et al. Increase in both CD14-positive and CD15-positive myeloid-derived suppressor cell subpopulations in the blood of patients with glioma but predominance of CD15-positive myeloid-derived suppressor cells in glioma tissue. J Neuropathol Exp Neurol. (2015) 5:390–400. doi: 10.1097/NEN.0000000000000183
25. Raychaudhuri B, Rayman P, Ireland J, Ko J, Rini B, Borden EC, et al. Myeloid-derived suppressor cell accumulation and function in patients with newly diagnosed glioblastoma. Neuro Oncol. (2011) 6:591–9. doi: 10.1093/neuonc/nor042
26. Dubinski D, Wolfer J, Hasselblatt M, Schneider-Hohendorf T, Bogdahn U, Stummer W, et al. CD44 T effector memory cell dysfunction is associated with the accumulation of granulocytic myeloid-derived suppressor cells in glioblastoma patients. Neuro Oncol. (2016) 6:807–18. doi: 10.1093/neuonc/nov280
27. Raychaudhuri B, Rayman P, Huang P, Grabowski M, Hambardzumyan D, Finke JH, et al. Myeloid derived suppressor cell infiltration of murine and human gliomas is associated with reduction of tumor infiltrating lymphocytes. J Neuro Oncol. (2015) 2:293–301. doi: 10.1007/s11060-015-1720-6
28. Rodrigues JC, Gonzalez GC, Zhang L, Ibrahim G, Kelly JJ, Gustafson MP, et al. Normal human monocytes exposed to glioma cells acquire myeloid-derived suppressor cell-like properties. Neuro Oncol. (2010) 4:351–65. doi: 10.1093/neuonc/nop023
29. Kumar R, de Mooij T, Peterson TE, Kaptzan T, Johnson AJ, Daniels DJ, et al. Modulating glioma-mediated myeloid-derived suppressor cell development with sulforaphane. PLoS One. (2017) 6:e0179012. doi: 10.1371/journal.pone.0179012
30. Jiang M, Chen J, Zhang W, Zhang R, Ye Y, Liu P, et al. Interleukin-6 trans-signaling pathway promotes immunosuppressive myeloid-derived suppressor cells via suppression of suppressor of cytokine signaling 3 in breast cancer. Front Immunol. (2017) 8:1840. doi: 10.3389/fimmu.2017.01840
31. Gielen PR, Schulte BM, Kers-Rebel ED, Verrijp K, Bossman SA, Ter Laan M, et al. Elevated levels of polymorphonuclear myeloid-derived suppressor cells in patients with glioblastoma highly express S100A8/9 and arginase and suppress T cell function. Neuro Oncol. (2016) 9:1253–64. doi: 10.1093/neuonc/now034
32. Morales JK, Kmieciak M, Knutson KL, Bear HD, Manjili MH. GM-CSF is one of the main breast tumor-derived soluble factors involved in the differentiation of CD11b-Gr1- bone marrow progenitor cells into myeloid-derived suppressor cells. Breast Cancer Res Treat. (2010) 1:39–49. doi: 10.1007/s10549-009-0622-8
33. Dolcetti L, Peranzoni E, Ugel S, Marigo I, Fernandez Gomez A, Mesa C, et al. Hierarchy of immunosuppressive strength among myeloid-derived suppressor cell subsets is determined by GM-CSF. Eur J Immunol. (2010) 1:22–35. doi: 10.1002/eji.200939903
34. Li L, Zhang J, Diao W, Wang D, Wei Y, Zhang CY, et al. MicroRNA-155 and MicroRNA-21 promote the expansion of functional myeloid-derived suppressor cells. J Immunol. (2014) 3:1034–43. doi: 10.4049/jimmunol.1301309
35. Marigo I, Bosio E, Solito S, Mesa C, Fernandez A, Dolcetti L, et al. Tumor-induced tolerance and immune suppression depend on the C/EBPbeta transcription factor. Immunity. (2010) 6:790–802. doi: 10.1016/j.immuni.2010.05.010
36. Mao Y, Sarhan D, Steven A, Seliger B, Kiessling R, Lundqvist A. Inhibition of tumor-derived prostaglandin-e2 blocks the induction of myeloid-derived suppressor cells and recovers natural killer cell activity. Clin Cancer Res. (2014) 15:4096–106. doi: 10.1158/1078-0432.CCR-14-0635
37. Bah I, Kumbhare A, Nguyen L, McCall CE, El Gazzar M. IL-10 induces an immune repressor pathway in sepsis by promoting S100A9 nuclear localization and MDSC development. Cell Immunol. (2018) 332:32–8. doi: 10.1016/j.cellimm.2018.07.003
38. Casella I, Feccia T, Chelucci C, Samoggia P, Castelli G, Guerriero R, et al. Autocrine-paracrine VEGF loops potentiate the maturation of megakaryocytic precursors through Flt1 receptor. Blood. (2003) 4:1316–23. doi: 10.1182/blood-2002-07-2184
39. Lechner MG, Megiel C, Russell SM, Bingham B, Arger N, Woo T, et al. Functional characterization of human Cd33+ and Cd11b+ myeloid-derived suppressor cell subsets induced from peripheral blood mononuclear cells co-cultured with a diverse set of human tumor cell lines. J Trans Med. (2011) 9:90. doi: 10.1186/1479-5876-9-90
40. Sade-Feldman M, Kanterman J, Ish-Shalom E, Elnekave M, Horwitz E, Baniyash M. Tumor necrosis factor-alpha blocks differentiation and enhances suppressive activity of immature myeloid cells during chronic inflammation. Immunity. (2013) 3:541–54. doi: 10.1016/j.immuni.2013.02.007
41. Soderberg SS, Karlsson G, Karlsson S. Complex and context dependent regulation of hematopoiesis by TGF-beta superfamily signaling. Ann N Y Acad Sci. (2009) 1176:55–69. doi: 10.1111/j.1749-6632.2009.04569.x
42. Albulescu R, Codrici E, Popescu ID, Mihai S, Necula LG, Petrescu D, et al. Cytokine patterns in brain tumour progression. Med Inflamm. (2013) 2103:979748. doi: 10.1155/2013/979748
43. Mattila S, Tuominen H, Koivukangas J, Stenback F. The terminal prostaglandin synthases mPGES-1, mPGES-2, and cPGES are all overexpressed in human gliomas. Neuropathology. (2009) 2:156–65. doi: 10.1111/j.1440-1789.2008.00963.x
44. Umansky V, Blattner C, Gebhardt C, Utikal J. The role of myeloid-derived suppressor cells (MDSC) in cancer progression. Vaccines. (2016) 4:36. doi: 10.3390/vaccines4040036
45. Obermajer N, Muthuswamy R, Odunsi K, Edwards RP, Kalinski P. PGE(2)-induced CXCL12 production and CXCR4 expression controls the accumulation of human MDSCs in ovarian cancer environment. Cancer Res. (2011) 24:7463–70. doi: 10.1158/0008-5472.CAN-11-2449
46. Connolly MK, Mallen-St Clair J, Bedrosian AS, Malhotra A, Vera V, Ibrahim J, et al. Distinct populations of metastases-enabling myeloid cells expand in the liver of mice harboring invasive and preinvasive intra-abdominal tumor. J Leuk Biol. (2010) 4:713–25. doi: 10.1189/jlb.0909607
47. Wang SW, Liu SC, Sun HL, Huang TY, Chan CH, Yang CY, et al. CCL5/CCR5 axis induces vascular endothelial growth factor-mediated tumor angiogenesis in human osteosarcoma microenvironment. Carcinogenesis. (2015) 1:104–14. doi: 10.1093/carcin/bgu218
48. Lesokhin AM, Hohl TM, Kitano S, Cortez C, Hirschhorn-Cymerman D, Avogadri F, et al. Monocytic CCR2+ myeloid-derived suppressor cells promote immune escape by limiting activated CD8 T-cell infiltration into the tumor microenvironment. Cancer Res. (2012) 4:876–86. doi: 10.1158/0008-5472.CAN-11-1792
49. Zhang J, Patel L, Pienta KJ. CC chemokine ligand 2 (CCL2) promotes prostate cancer tumorigenesis and metastasis. Cytok Growth Fact Rev. (2010) 1:41–8. doi: 10.1016/j.cytogfr.2009.11.009
50. Chang AL, Miska J, Wainwright DA, Dey M, Rivetta CV, Yu D, et al. CCL2 Produced by the glioma microenvironment is essential for the recruitment of regulatory T cells and myeloid-derived suppressor cells. Cancer Res. (2016) 19:5671–82. doi: 10.1158/0008-5472.CAN-16-0144
51. Vakilian A, Khorramdelazad H, Heidari P, Sheikh Rezaei Z, Hassanshahi G. CCL2/CCR2 signaling pathway in glioblastoma multiforme. Neurochem Int. (2017) 103:1–7. doi: 10.1016/j.neuint.2016.12.013
52. Alfaro C, Teijeira A, Onate C, Perez G, Sanmamed MF, Andueza MP, et al. Tumor-produced interleukin-8 attracts human myeloid-derived suppressor cells and elicits extrusion of neutrophil extracellular traps (NETs). Clin Cancer Res. (2016) 15:3924–36. doi: 10.1158/1078-0432.CCR-15-2463
53. David JM, Dominguez C, Hamilton DH, Palena C. The IL-8/IL-8R axis: a double agent in tumor immune resistance. Vaccines. (2016) 3:22. doi: 10.3390/vaccines4030022
54. Katoh H, Wang D, Daikoku T, Sun H, Dey SK, Dubois RN. CXCR2-expressing myeloid-derived suppressor cells are essential to promote colitis-associated tumorigenesis. Cancer Cell. (2013) 5:631–44. doi: 10.1016/j.ccr.2013.10.009
55. Kammerer R, Buchner A, Palluch P, Pongratz T, Oboukhovskij K, Beyer W, et al. Induction of immune mediators in glioma and prostate cancer cells by non-lethal photodynamic therapy. PLoS One. (2011) 6:e21834. doi: 10.1371/journal.pone.0021834
56. Bruyere C, Mijatovic T, Lonez C, Spiegl-Kreinecker S, Berger W, Kast RE, et al. Temozolomide-induced modification of the CXC chemokine network in experimental gliomas. Int J Oncol. (2011) 5:1453–64. doi: 10.3892/ijo.2011.964
57. Liu Y, Wei J, Guo G, Zhou J. Norepinephrine-induced myeloid-derived suppressor cells block T-cell responses via generation of reactive oxygen species. Immunopharmacol Immunotoxicol. (2015) 4:359–65. doi: 10.3109/08923973.2015.1059442
58. Raber PL, Thevenot P, Sierra R, Wyczechowska D, Halle D, Ramirez ME, et al. Subpopulations of myeloid-derived suppressor cells impair T cell responses through independent nitric oxide-related pathways. Int J Cancer. (2014) 12:2853–64. doi: 10.1002/ijc.28622
59. Raber P, Ochoa AC, Rodriguez PC. Metabolism of L-arginine by myeloid-derived suppressor cells in cancer: mechanisms of T cell suppression and therapeutic perspectives. Immunol Investigat. (2012) 41:614–34. doi: 10.3109/08820139.2012.680634
60. Nagaraj S, Schrum AG, Cho HI, Celis E, Gabrilovich DI. Mechanism of T cell tolerance induced by myeloid-derived suppressor cells. J Immunol. (2010) 6:3106–16. doi: 10.4049/jimmunol.0902661
61. Molon B, Ugel S, Del Pozzo F, Soldani C, Zilio S, Avella D, et al. Chemokine nitration prevents intratumoral infiltration of antigen-specific T cells. J Exp Med. (2011) 10:1949–62. doi: 10.1084/jem.20101956
62. Bronte V, Zanovello P. Regulation of immune responses by L-arginine metabolism. Nat Rev Immunol. (2005) 8:641–54. doi: 10.1038/nri1668
63. Rodriguez PC, Quiceno DG, Zabaleta J, Ortiz B, Zea AH, Piazuelo MB, et al. Arginase I production in the tumor microenvironment by mature myeloid cells inhibits T-cell receptor expression and antigen-specific T-cell responses. Cancer Res. (2004) 16:5839–49. doi: 10.1158/0008-5472.CAN-04-0465
64. Schafer CC, Wang Y, Hough KP, Sawant A, Grant SC, Thannickal VJ, et al. Indoleamine 2,3-dioxygenase regulates anti-tumor immunity in lung cancer by metabolic reprogramming of immune cells in the tumor microenvironment. Oncotarget. (2016) 46:75407–24. doi: 10.18632/oncotarget.12249
65. Parker KH, Beury DW, Ostrand-Rosenberg S. Myeloid-derived suppressor cells: critical cells driving immune suppression in the tumor microenvironment. Adv Cancer Res. (2015) 128:95–139. doi: 10.1016/bs.acr.2015.04.002
66. Vetsika EK, Koukos A, Kotsakis A. Myeloid-derived suppressor cells: major figures that shape the immunosuppressive and angiogenic network in cancer. Cells. (2019) 12:1647. doi: 10.3390/cells8121647
67. Noman MZ, Desantis G, Janji B, Hasmim M, Karray S, Dessen P, et al. PD-L1 is a novel direct target of HIF-1alpha, and its blockade under hypoxia enhanced MDSC-mediated T cell activation. J Exp Med. (2014) 5:781–90. doi: 10.1084/jem.20131916
68. Baker GJ, Chockley P, Yadav VN, Doherty R, Ritt M, Sivaramakrishnan S, et al. Natural killer cells eradicate galectin-1-deficient glioma in the absence of adaptive immunity. Cancer Res. (2014) 18:5079–90. doi: 10.1158/0008-5472.CAN-14-1203
69. Fortin C, Huang X, Yang Y. NK cell response to vaccinia virus is regulated by myeloid-derived suppressor cells. J Immunol. (2012) 4:1843–9. doi: 10.4049/jimmunol.1200584
70. Li H, Han Y, Guo Q, Zhang M, Cao X. Cancer-expanded myeloid-derived suppressor cells induce anergy of NK cells through membrane-bound TGF-beta 1. J Immunol. (2009) 1:240–9. doi: 10.4049/jimmunol.182.1.240
71. Hoechst B, Voigtlaender T, Ormandy L, Gamrekelashvili J, Zhao F, Wedemeyer H, et al. Myeloid derived suppressor cells inhibit natural killer cells in patients with hepatocellular carcinoma via the NKp30 receptor. Hepatology. (2009) 3:799–807. doi: 10.1002/hep.23054
72. Bruno A, Mortara L, Baci D, Noonan DM, Albini A. Myeloid derived suppressor cells interactions with natural killer cells and pro-angiogenic activities: roles in tumor progression. Front Immunol. (2019) 10:771. doi: 10.3389/fimmu.2019.00771
73. da Fonseca AC, Badie B. Microglia and macrophages in malignant gliomas: recent discoveries and implications for promising therapies. Clin Dev Immunol. (2013) 2013:264124. doi: 10.1155/2013/264124
74. Won WJ, Deshane JS, Leavenworth JW, Oliva CR, Griguer CE. Metabolic and functional reprogramming of myeloid-derived suppressor cells and their therapeutic control in glioblastoma. Cell Stress. (2019) 2:47–65. doi: 10.15698/cst2019.02.176
75. Ostrand-Rosenberg S, Sinha P, Beury DW, Clements VK. Cross-talk between myeloid-derived suppressor cells (MDSC), macrophages, and dendritic cells enhances tumor-induced immune suppression. Semi Cancer Biol. (2012) 4:275–81. doi: 10.1016/j.semcancer.2012.01.011
76. Gabrilovich DI, Ostrand-Rosenberg S, Bronte V. Coordinated regulation of myeloid cells by tumours. Nat Rev Immunol. (2012) 4:253–68. doi: 10.1038/nri3175
77. Pinton L, Masetto E, Vettore M, Solito S, Magri S, D’Andolfi M, et al. The immune suppressive microenvironment of human gliomas depends on the accumulation of bone marrow-derived macrophages in the center of the lesion. J Immunother Cancer. (2019) 1:58. doi: 10.1186/s40425-019-0536-x
78. Marsh JC, Goldfarb J, Shafman TD, Diaz AZ. Current status of immunotherapy and gene therapy for high-grade gliomas. Cancer Cont. (2013) 1:43–8. doi: 10.1177/107327481302000107
79. Yang L, Guo G, Niu XY, Liu J. Dendritic cell-based immunotherapy treatment for glioblastoma multiforme. Biomed Res Int. (2015) 2015:717530. doi: 10.1155/2015/717530
80. Hu CE, Gan J, Zhang RD, Cheng YR, Huang GJ. Up-regulated myeloid-derived suppressor cell contributes to hepatocellular carcinoma development by impairing dendritic cell function. Scand J Gastroenterol. (2011) 2:156–64. doi: 10.3109/00365521.2010.516450
81. Bettelli E, Carrier Y, Gao W, Korn T, Strom TB, Oukka M, et al. Reciprocal developmental pathways for the generation of pathogenic effector TH17 and regulatory T cells. Nature. (2006) 7090:235–8. doi: 10.1038/nature04753
82. Ooi YC, Tran P, Ung N, Thill K, Trang A, Fong BM, et al. The role of regulatory T-cells in glioma immunology. Clin Neurol Neurosur. (2014) 119:125–32. doi: 10.1016/j.clineuro.2013.12.004
83. Wainwright DA, Dey M, Chang A, Lesniak MS. Targeting tregs in malignant brain cancer: overcoming IDO. Front Immunol. (2013) 4:116. doi: 10.3389/fimmu.2013.00116
84. Wei J, Wu A, Kong LY, Wang Y, Fuller G, Fokt I, et al. Hypoxia potentiates glioma-mediated immunosuppression. PLoS One. (2011) 1:e16195. doi: 10.1371/journal.pone.0016195
85. Huang B, Pan PY, Li Q, Sato AI, Levy DE, Bromberg J, et al. Gr-1+CD115+ immature myeloid suppressor cells mediate the development of tumor-induced T regulatory cells and T-cell anergy in tumor-bearing host. Cancer Res. (2006) 2:1123–31. doi: 10.1158/0008-5472.CAN-05-1299
86. Park MJ, Lee SH, Kim EK, Lee EJ, Baek JA, Park SH, et al. Interleukin-10 produced by myeloid-derived suppressor cells is critical for the induction of Tregs and attenuation of rheumatoid inflammation in mice. Sci Rep. (2018) 1:3753. doi: 10.1038/s41598-018-21856-2
87. Hoechst B, Gamrekelashvili J, Manns MP, Greten TF, Korangy F. Plasticity of human Th17 cells and iTregs is orchestrated by different subsets of myeloid cells. Blood. (2011) 24:6532–41. doi: 10.1182/blood-2010-11-317321
88. Rosser EC, Mauri C. Regulatory B cells: origin, phenotype, and function. Immunity. (2015) 4:607–12. doi: 10.1016/j.immuni.2015.04.005
89. Ye ZP, He HY, Wang H, Li WS, Luo L, Huang ZC, et al. Glioma-derived ADAM10 induces regulatory B cells to suppress CD8+ T cells. PLoS One. (2014) 8:e105350. doi: 10.1371/journal.pone.0105350
90. Kujawski M, Kortylewski M, Lee H, Herrmann A, Kay H, Yu H. Stat3 mediates myeloid cell-dependent tumor angiogenesis in mice. J Clin Investigat. (2008) 10:3367–77. doi: 10.1172/JCI35213
91. Yang L, DeBusk LM, Fukuda K, Fingleton B, Green-Jarvis B, Shyr Y, et al. Expansion of myeloid immune suppressor Gr+CD11b+ cells in tumor-bearing host directly promotes tumor angiogenesis. Cancer Cell. (2004) 4:409–21. doi: 10.1016/j.ccr.2004.08.031
92. Pawelek JM, Chakraborty AK. Fusion of tumour cells with bone marrow-derived cells: a unifying explanation for metastasis. Nat Rev Cancer. (2008) 5:377–86. doi: 10.1038/nrc2371
93. Du R, Lu KV, Petritsch C, Liu P, Ganss R, Passegue E, et al. HIF1alpha induces the recruitment of bone marrow-derived vascular modulatory cells to regulate tumor angiogenesis and invasion. Cancer Cell. (2008) 3:206–20. doi: 10.1016/j.ccr.2008.01.034
94. Cui TX, Kryczek I, Zhao L, Zhao E, Kuick R, Roh MH, et al. Myeloid-derived suppressor cells enhance stemness of cancer cells by inducing microRNA101 and suppressing the corepressor CtBP2. Immunity. (2013) 3:611–21. doi: 10.1016/j.immuni.2013.08.025
95. Peng D, Tanikawa T, Li W, Zhao L, Vatan L, Szeliga W, et al. Myeloid-derived suppressor cells endow stem-like qualities to breast cancer cells through IL6/STAT3 and NO/NOTCH cross-talk signaling. Cancer Res. (2016) 11:3156–65. doi: 10.1158/0008-5472.CAN-15-2528
96. Ai L, Mu S, Sun C, Fan F, Yan H, Qin Y, et al. Myeloid-derived suppressor cells endow stem-like qualities to multiple myeloma cells by inducing piRNA-823 expression and DNMT3B activation. Mol Cancer. (2019) 1:88. doi: 10.1186/s12943-019-1011-5
97. Suzuki E, Kapoor V, Jassar AS, Kaiser LR, Albelda SM. Gemcitabine selectively eliminates splenic Gr-1+/CD11b+ myeloid suppressor cells in tumor-bearing animals and enhances antitumor immune activity. Clin Cancer Res. (2005) 18:6713–21. doi: 10.1158/1078-0432.CCR-05-0883
98. Bastiancich C, Bastiat G, Lagarce F. Gemcitabine and glioblastoma: challenges and current perspectives. Drug Discover Today. (2018) 2:416–23. doi: 10.1016/j.drudis.2017.10.010
99. Vincent J, Mignot G, Chalmin F, Ladoire S, Bruchard M, Chevriaux A, et al. 5-Fluorouracil selectively kills tumor-associated myeloid-derived suppressor cells resulting in enhanced T cell-dependent antitumor immunity. Cancer Res. (2010) 8:3052–61. doi: 10.1158/0008-5472.CAN-09-3690
100. Ugel S, Peranzoni E, Desantis G, Chioda M, Walter S, Weinschenk T, et al. Immune tolerance to tumor antigens occurs in a specialized environment of the spleen. Cell Rep. (2012) 3:628–39. doi: 10.1016/j.celrep.2012.08.006
101. Otvos B, Silver DJ, Mulkearns-Hubert EE, Alvarado AG, Turaga SM, Sorensen MD, et al. Cancer stem cell-secreted macrophage migration inhibitory factor stimulates myeloid derived suppressor cell function and facilitates glioblastoma immune evasion. Stem Cells. (2016) 8:2026–39. doi: 10.1002/stem.2393
102. Peereboom DM, Alban TJ, Grabowski MM, Alvarado AG, Otvos B, Bayik D, et al. Metronomic capecitabine as an immune modulator in glioblastoma patients reduces myeloid-derived suppressor cells. JCI Insight. (2019) 4:e130748. doi: 10.1172/jci.insight.130748
103. Condamine T, Kumar V, Ramachandran IR, Youn JI, Celis E, Finnberg N, et al. ER stress regulates myeloid-derived suppressor cell fate through TRAIL-R-mediated apoptosis. J Clin Investigat. (2014) 6:2626–39. doi: 10.1172/JCI74056
104. Dominguez GA, Condamine T, Mony S, Hashimoto A, Wang F, Liu Q, et al. Selective targeting of myeloid-derived suppressor cells in cancer patients using DS-8273a, an agonistic TRAIL-R2 antibody. Clin Cancer Res. (2017) 12:2942–50. doi: 10.1158/1078-0432.CCR-16-1784
105. Nagane M, Shimizu S, Mori E, Kataoka S, Shiokawa Y. Predominant antitumor effects by fully human anti-TRAIL-receptor 2 (DR5) monoclonal antibodies in human glioma cells in vitro and in vivo. Neuro Oncol. (2010) 7:687–700. doi: 10.1093/neuonc/nop069
106. Murat A, Migliavacca E, Hussain SF, Heimberger AB, Desbaillets I, Hamou MF, et al. Modulation of angiogenic and inflammatory response in glioblastoma by hypoxia. PLoS One. (2009) 6:e5947. doi: 10.1371/journal.pone.0005947
107. Qin H, Lerman B, Sakamaki I, Wei G, Cha SC, Rao SS, et al. Generation of a new therapeutic peptide that depletes myeloid-derived suppressor cells in tumor-bearing mice. Nat Med. (2014) 6:676–81. doi: 10.1038/nm.3560
108. Alicea-Torres K, Gabrilovich DI. Biology of myeloid-derived suppressor cells. In: Zitvogel L Kroemer G editors. Oncoimmunology. Cham: Springer (2017).
109. Rossi JF, Lu ZY, Jourdan M, Klein B. Interleukin-6 as a therapeutic target. Clin Cancer Res. (2015) 6:1248–57. doi: 10.1158/1078-0432.CCR-14-2291
110. Xue H, Yuan G, Guo X, Liu Q, Zhang J, Gao X, et al. A novel tumor-promoting mechanism of IL6 and the therapeutic efficacy of tocilizumab: Hypoxia-induced IL6 is a potent autophagy initiator in glioblastoma via the p-STAT3-MIR155-3p-CREBRF pathway. Autophagy. (2016) 7:1129–52. doi: 10.1080/15548627.2016.1178446
111. Sumida K, Wakita D, Narita Y, Masuko K, Terada S, Watanabe K, et al. Anti-IL-6 receptor mAb eliminates myeloid-derived suppressor cells and inhibits tumor growth by enhancing T-cell responses. Eur J Immunol. (2012) 8:2060–72. doi: 10.1002/eji.201142335
112. Zhu X, Fujita M, Snyder LA, Okada H. Systemic delivery of neutralizing antibody targeting CCL2 for glioma therapy. J Neuro Oncol. (2011) 1:83–92. doi: 10.1007/s11060-010-0473-5
113. Chen XW, Sun JG, Zhang LP, Liao XY, Liao RX. Recruitment of CD11b(+)Ly6C(+) monocytes in non-small cell lung cancer xenografts challenged by anti-VEGF antibody. Oncol Lett. (2017) 1:615–22. doi: 10.3892/ol.2017.6236
114. Rubenstein JL, Kim J, Ozawa T, Zhang M, Westphal M, Deen DF, et al. Anti-VEGF antibody treatment of glioblastoma prolongs survival but results in increased vascular cooption. Neoplasia. (2000) 4:306–14. doi: 10.1038/sj.neo.7900102
115. Xiao Q, Yang S, Ding G, Luo M. Anti-vascular endothelial growth factor in glioblastoma: a systematic review and meta-analysis. Neurol Sci. (2018) 12:2021–31. doi: 10.1007/s10072-018-3568-y
116. Mirghorbani M, Van Gool S, Rezaei N. Myeloid-derived suppressor cells in glioma. Exp Rev Neurother. (2013) 12:1395–406. doi: 10.1586/14737175.2013.857603
117. Gao X, Guo N, Xu H, Pan T, Lei H, Yan A, et al. Ibuprofen induces ferroptosis of glioblastoma cells via downregulation of nuclear factor erythroid 2-related factor 2 signaling pathway. Anti Cancer Drugs. (2020) 1:27–34. doi: 10.1097/CAD.0000000000000825
118. Zhang T, Yang X, Liu P, Zhou J, Luo J, Wang H, et al. Association between nonsteroidal anti-inflammatory drugs use and risk of central nervous system tumors: a dose-response meta analysis. Oncotarget. (2017) 60:102486–98. doi: 10.18632/oncotarget.21829
119. Scheurer ME, El-Zein R, Thompson PA, Aldape KD, Levin VA, Gilbert MR, et al. Long-term anti-inflammatory and antihistamine medication use and adult glioma risk. Cancer Epidemiol Biomark Preven. (2008) 5:1277–81. doi: 10.1158/1055-9965.EPI-07-2621
120. Condamine T, Mastio J, Gabrilovich DI. Transcriptional regulation of myeloid-derived suppressor cells. J Leu Biol. (2015) 6:913–22. doi: 10.1189/jlb.4RI0515-204R
121. Sinha P, Clements VK, Fulton AM, Ostrand-Rosenberg S. Prostaglandin E2 promotes tumor progression by inducing myeloid-derived suppressor cells. Cancer Res. (2007) 9:4507–13. doi: 10.1158/0008-5472.CAN-06-4174
122. Highfill SL, Cui Y, Giles AJ, Smith JP, Zhang H, Morse E, et al. Disruption of CXCR2-mediated MDSC tumor trafficking enhances anti-PD1 efficacy. Sci Trans Med. (2014) 237:237ra267. doi: 10.1126/scitranslmed.3007974
123. Sun L, Clavijo PE, Robbins Y, Patel P, Friedman J, Greene S, et al. Inhibiting myeloid-derived suppressor cell trafficking enhances T cell immunotherapy. JCI Insight. (2019) 4:e126853. doi: 10.1172/jci.insight.126853
124. Greene S, Robbins Y, Mydlarz WK, Huynh AP, Schmitt NC, Friedman J, et al. Inhibition of MDSC Trafficking with SX-682, a CXCR1/2 inhibitor, enhances NK-cell immunotherapy in head and neck cancer models. Clin Cancer Res. (2019) 26:1420–31. doi: 10.1158/1078-0432.CCR-19-2625
125. Hirano K, Hosoi A, Matsushita H, Iino T, Ueha S, Matsushima K, et al. The nitric oxide radical scavenger carboxy-PTIO reduces the immunosuppressive activity of myeloid-derived suppressor cells and potentiates the antitumor activity of adoptive cytotoxic T lymphocyte immunotherapy. Oncoimmunology. (2015) 8:e1019195. doi: 10.1080/2162402X.2015.1019195
126. Barone I, Giordano C, Bonofiglio D, Ando S, Catalano S. Phosphodiesterase type 5 and cancers: progress and challenges. Oncotarget. (2017) 58:99179–202. doi: 10.18632/oncotarget.21837
127. Capuano G, Rigamonti N, Grioni M, Freschi M, Bellone M. Modulators of arginine metabolism support cancer immunosurveillance. BMC Immunol. (2009) 10:1. doi: 10.1186/1471-2172-10-1
128. Serafini P, Meckel K, Kelso M, Noonan K, Califano J, Koch W, et al. Phosphodiesterase-5 inhibition augments endogenous antitumor immunity by reducing myeloid-derived suppressor cell function. J Exp Med. (2006) 12:2691–702. doi: 10.1084/jem.20061104
129. Lim JW, Kim H, Kim KH. Nuclear factor-kappaB regulates cyclooxygenase-2 expression and cell proliferation in human gastric cancer cells. Lab Investigat. (2001) 3:349–60. doi: 10.1038/labinvest.3780243
130. Condamine T, Gabrilovich DI. Molecular mechanisms regulating myeloid-derived suppressor cell differentiation and function. Trends Immunol. (2011) 1:19–25. doi: 10.1016/j.it.2010.10.002
131. Fujita M, Kohanbash G, Fellows-Mayle W, Hamilton RL, Komohara Y, Decker SA, et al. COX-2 blockade suppresses gliomagenesis by inhibiting myeloid-derived suppressor cells. Cancer Res. (2011) 7:2664–74. doi: 10.1158/0008-5472.CAN-10-3055
132. Srivastava MK, Andersson A, Zhu L, Harris-White M, Lee JM, Dubinett S, et al. Myeloid suppressor cells and immune modulation in lung cancer. Immunotherapy. (2012) 3:291–304. doi: 10.2217/imt.11.178
133. Orillion A, Hashimoto A, Damayanti N, Shen L, Adelaiye-Ogala R, Arisa S, et al. Entinostat neutralizes myeloid-derived suppressor cells and enhances the antitumor effect of PD-1 inhibition in murine models of lung and renal cell carcinoma. Clin Cancer Res. (2017) 17:5187–201. doi: 10.1158/1078-0432.CCR-17-0741
134. Lee P, Murphy B, Miller R, Menon V, Banik NL, Giglio P, et al. Mechanisms and clinical significance of histone deacetylase inhibitors: epigenetic glioblastoma therapy. Anticancer Res. (2015) 2: 615–25.
135. Hammami I, Chen J, Bronte V, DeCrescenzo G, Jolicoeur ML-. glutamine is a key parameter in the immunosuppression phenomenon. Biochem Biophys Res Commun. (2012) 4:724–9. doi: 10.1016/j.bbrc.2012.07.139
136. Meldrum BS. Glutamate as a neurotransmitter in the brain: review of physiology and pathology. J Nutr. (2000) 4:1007S–15S. doi: 10.1093/jn/130.4.1007S
137. Robert SM, Sontheimer H. Glutamate transporters in the biology of malignant gliomas. Cell Mol Life Sci CMLS. (2014) 10:1839–54. doi: 10.1007/s00018-013-1521-z
138. Natarajan SK, Venneti S. Glutamine metabolism in brain tumors. Cancers. (2019) 11:1628. doi: 10.3390/cancers11111628
139. Li H, Li Z, Xu YM, Wu Y, Yu KK, Zhang C, et al. Epigallocatechin-3-gallate induces apoptosis, inhibits proliferation and decreases invasion of glioma cell. Neurosci Bull. (2014) 1:67–73. doi: 10.1007/s12264-013-1394-z
140. Orentas RJ. Reading the tea leaves of tumor-mediated immunosuppression. Clin Cancer Res. (2013) 5:955–7. doi: 10.1158/1078-0432.CCR-12-3792
141. Hengesbach LM, Hoag KA. Physiological concentrations of retinoic acid favor myeloid dendritic cell development over granulocyte development in cultures of bone marrow cells from mice. J Nutr. (2004) 10:2653–9. doi: 10.1093/jn/134.10.2653
142. Mirza N, Fishman M, Fricke I, Dunn M, Neuger AM, Frost TJ, et al. All-trans-retinoic acid improves differentiation of myeloid cells and immune response in cancer patients. Cancer Res. (2006) 18:9299–307. doi: 10.1158/0008-5472.CAN-06-1690
143. Nefedova Y, Fishman M, Sherman S, Wang X, Beg AA, Gabrilovich DI. Mechanism of all-trans retinoic acid effect on tumor-associated myeloid-derived suppressor cells. Cancer Res. (2007) 22:11021–8. doi: 10.1158/0008-5472.CAN-07-2593
144. Wang R, Liu C. All-trans retinoic acid therapy induces asymmetric division of glioma stem cells from the U87MG cell line. Oncol Lett. (2019) 4:3646–54. doi: 10.3892/ol.2019.10691
145. Karsy M, Albert L, Tobias ME, Murali R, Jhanwar-Uniyal M. All-trans retinoic acid modulates cancer stem cells of glioblastoma multiforme in an MAPK-dependent manner. Anticancer Res. (2010) 12:4915–20.
146. Wiers KM, Lathers DM, Wright MA, Young MR. Vitamin D3 treatment to diminish the levels of immune suppressive CD34+ cells increases the effectiveness of adoptive immunotherapy. J Immunother. (2000) 1:115–24. doi: 10.1097/00002371-200001000-00014
147. Salomon DG, Fermento ME, Gandini NA, Ferronato MJ, Arevalo J, Blasco J, et al. Vitamin D receptor expression is associated with improved overall survival in human glioblastoma multiforme. J Neuro Oncol. (2014) 1:49–60. doi: 10.1007/s11060-014-1416-3
148. Walsh JE, Clark AM, Day TA, Gillespie MB, Young MR. Use of alpha,25-dihydroxyvitamin D3 treatment to stimulate immune infiltration into head and neck squamous cell carcinoma. Hum Immunol. (2010) 7:659–65. doi: 10.1016/j.humimm.2010.04.008
149. Sun L, Hui AM, Su Q, Vortmeyer A, Kotliarov Y, Pastorino S, et al. Neuronal and glioma-derived stem cell factor induces angiogenesis within the brain. Cancer Cell. (2006) 4:287–300. doi: 10.1016/j.ccr.2006.03.003
150. Pan PY, Wang GX, Yin B, Ozao J, Ku T, Divino CM, et al. Reversion of immune tolerance in advanced malignancy: modulation of myeloid-derived suppressor cell development by blockade of stem-cell factor function. Blood. (2008) 1:219–28. doi: 10.1182/blood-2007-04-086835
Keywords: MDSC, glioma, immunosuppression, tumor microenvironment, therapeutic target
Citation: Mi Y, Guo N, Luan J, Cheng J, Hu Z, Jiang P, Jin W and Gao X (2020) The Emerging Role of Myeloid-Derived Suppressor Cells in the Glioma Immune Suppressive Microenvironment. Front. Immunol. 11:737. doi: 10.3389/fimmu.2020.00737
Received: 16 December 2019; Accepted: 31 March 2020;
Published: 24 April 2020.
Edited by:
Nurit Hollander, Tel Aviv University, IsraelReviewed by:
Stefano Ugel, University of Verona, ItalyIlaria Marigo, Istituto Oncologico Veneto (IRCCS), Italy
Copyright © 2020 Mi, Guo, Luan, Cheng, Hu, Jiang, Jin and Gao. This is an open-access article distributed under the terms of the Creative Commons Attribution License (CC BY). The use, distribution or reproduction in other forums is permitted, provided the original author(s) and the copyright owner(s) are credited and that the original publication in this journal is cited, in accordance with accepted academic practice. No use, distribution or reproduction is permitted which does not comply with these terms.
*Correspondence: Weilin Jin, d2VpbGluamluQHlhaG9vLmNvbQ==; Xingchun Gao, Z3hjMTk5MjgxMDAzQDE2My5jb20=
†These authors have contributed equally to this work